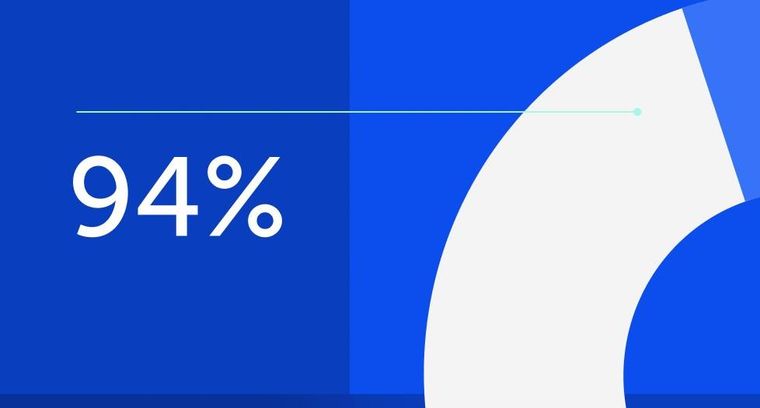
94% of researchers rate our articles as excellent or good
Learn more about the work of our research integrity team to safeguard the quality of each article we publish.
Find out more
ORIGINAL RESEARCH article
Front. Cell Dev. Biol., 25 November 2022
Sec. Nuclear Organization and Dynamics
Volume 10 - 2022 | https://doi.org/10.3389/fcell.2022.1050556
This article is part of the Research TopicMolecular Architecture and Dynamics of Meiotic ChromosomesView all 16 articles
Robertsonian translocation is the most common chromosomal rearrangement in mammals, and represents the type of chromosomal change that most effectively contributes to speciation in natural populations. Rb translocations involve double-strand DNA breaks at the centromere level in two telocentric chromosomes, followed by repair ligation of the respective long arms, creating a metacentric Rb chromosome. Many different chromosomal races have been described in Mus musculus domesticus that show reduced chromosome numbers due to the presence of Rb metacentric chromosomes. The crossroads between ancestral telocentrics and the new metacentric chromosomes should be resolved in the meiotic cells of the heterozygote individuals, which form trivalents. The preferential segregation of metacentric chromosomes to the egg during female meiosis I has been proposed to favor their fixation and eventual conversion of a telocentric karyotype to a metacentric karyotype. This biased segregation, a form of meiotic drive, explains the karyotype changes in mammalian species that have accumulated Rb fusions. We studied and compared the number of Rb chromosomes inherited by the offspring of multiple Rb heterozygous of M. domesticus in reciprocal crosses. We did not find that the Rb chromosomes were inherited preferentially with respect to the telocentric chromosomes; therefore, we found no evidence for the meiotic drive, nor was there a random distribution of Rb chromosomes inherited by the descendants.
Robertsonian (Rb) translocation is the most common chromosomal rearrangement in mammals (King 1993) and that most effectively contributes to variation or speciation in natural populations (Garagna et al., 2001a; Aniskin et al., 2006). In Mus musculus domesticus, many different chromosomal races have been described that show reduced chromosome numbers due to the presence of metacentric Rb chromosomes (Pialek et al., 2005). Rb translocations involve double-strand DNA breaks at the centromere level in two telocentric chromosomes, followed by fusion of the respective long arms, creating a metacentric Rb chromosome. The short arms (p) of the original telocentric chromosomes, including the proximal telomeres, part of the satellite DNA and, frequently one centromere, are lost (Nanda et al., 1995; Garagna et al., 2001a; Garagna et al., 2002). Most current models of chromosomal variation of natural populations assume that Rb chromosomes are negatively tolerated; only after a long period can the new chromosome could eventually overcome the meiotic restrictions and become established within a reproductive community (Baker and Bickham, 1986; Rieseberg 2001; Hauffe et al., 2012). However, six distinct chromosomal races of the house mice of the Madeira Island have emerged in less than 500 years. This remarkable example of chromosome evolution has been explained by reproductive chromosomal isolation and genetic drift (Britton-Davidian et al., 2000; Britton-Davidian et al., 2005). The crossroads between ancestral telocentrics and new metacentric chromosomes should be resolved in heterozygote individuals, wherein trivalents are formed during meiosis I (Wallace et al., 2002). The Mendelian distribution of metacentric/telocentric chromosomes in the heterozygote gametes will depend on the normal progression of the meiotic prophase, random arrangement of the trivalents at metaphase I, and alternate segregation between them at anaphase. The meiotic drive (also called segregation distortion) is the preferential selection of certain chromosomes or gametes that alters the gene ratio from the Mendelian expectations.
Although multiple Rb heterozygotes showed an increased loss of spermatocytes at prophase I, many of them entered meiotic divisions (Garagna et al., 2001b; Wallace et al., 2002; Manterola et al., 2009). The chromosomes of each trivalent move together into alignment at metaphase and separate from each other at anaphase I. Only gametes resulting from alternate segregation exhibit normal or balanced karyotypes. Gametes produced by adjacent segregations have unbalanced karyotypes, being nullisomic or disomic in one or more chromosomes. Selective mechanisms, such as the metaphase checkpoint, appear to be effective because spermatids resulting from alternate segregation prevail in heterozygotes (Eaker et al., 2001; Anton et al., 2004; Pylyp et al., 2013; Manieu et al., 2014; Lamotte et al., 2018). Additionally, the direction of alternate segregation during female meiosis I could potentially determine whether metacentric chromosomes are transmitted to the offspring (Yoshida and Kitano, 2012).
According to the meiotic drive hypothesis, Rb metacentrics segregated preferentially to the egg in populations that have fixed multiple different metacentrics; and preferentially segregate to the polar body in other populations that have remained telocentric (de Villena and Sapienza 2001; White et al., 2010). It is still unclear what determines the direction of the drive and how that directions can differ between populations, so that some of them retain the fusions and change karyotype, while others do not. The relative centromere strength of Rb metacentric versus homologous telocentrics has been proposed to determine the direction of the meiotic drive, with stronger centromeres preferentially remaining in the egg (Chmátal et al., 2014). In addition, asymmetries in the meiotic spindle orientation, tubulin and microtubule-organizing center, and associated meiotic drivers may contribute to explain the meiotic drive in oocytes (Akera et al., 2017; Wu et al., 2018; Kruger and Mueller., 2021).
In this study we attempted to determine whether the meiotic drive could be taking place during the meiosis of Robertsonian heterozygotes of Mus musculus domesticus who carry eight Rb chromosomes, by studying the number of Rb chromosomes (0–8), inherited by the descendants of parental males or females who are multiple heterozygous Rb.
Males and females of the Rb heterozygotes 2n = 32 group of Mus musculus domesticus were generated by crossing the homozygote strain CD1 2n = 40, with all their chromosomes telocentric, and the homozygote Milano II race 2n = 24, with eight pairs of Rb metacentric chromosomes. The heterozygotes were crossed with homozygotes (2n = 40), to obtain offspring who could inherit between 0 and 8 Rb metacentric chromosomes (Figure 1). The eight Rb chromosomes are: Rb 2.12, 3.4, 5.15, 6.7, 8.11, 9.14, 10.13, 16.17. Numbers correspond to the 2n = 40 standard karyotype. Nineteen crosses of heterozygous females (2n = 32) and homozygous males (2n = 40) were performed with litter, with an average of 4.4 offspring in each. Ten crosses of heterozygous males (2n = 32) and homozygous females (2n = 40) were made with litter, with an average of 5.5 offspring each.
FIGURE 1. Origin of the mice under study that can inherit between 0 and 8 Rb chromosomes. Males and females of Robertsonian (Rb) heterozygotes 2n = 32 of Mus musculus domesticus were generated by crossing homozygotes Milano II race 2n = 24, with eight pairs of Rb metacentric chromosomes, and homozygote strain CD1 2n = 40, with all chromosomes telocentric. In turn, heterozygotes were crossed with homozygotes (2n = 40) to obtain offspring who could inherit between 0 and 8 Rb metacentric chromosomes.
Mice were maintained at 22°C with a light/dark cycle of 12/12 h and fed ad libitum. The procedures involving the use of the mice were reviewed and approved by the Ethics Review Committee of the School of Medicine, Universidad de Chile (No. CBA #0441), and by the Ethics Review Committee of the Chilean National Science Foundation FONDECYT-CONICYT. The care and handling of laboratory animals was done following all institutional and national guidelines (protocol CBA #0441, FMUCH).
The number of Rb chromosomes inherited by 139 descendants of female or male Rb heterozygotes was studied in metaphase plate chromosomes obtained from bone marrow cells and stained with Giemsa or DAPI. Briefly, bone marrow cells were incubated for 10 min in 0.05 M KCl at 37°C, and the pellet was washed and fixed in 3:1 v/v methanol: acetic acid. Rb chromosomes and total chromosomes were counted in 10 metaphase plates per animal. After determining the number of Rb metacentric chromosomes, we verified that the telocentric chromosomes actually completed the corresponding diploid number.
The size of the pericentromeric region was measured in the Rb chromosomes inherited by each of the five offspring of a 2n = 32 heterozygous father and a 2n = 40 mother, which were respectively: Three, five, three, two, four, and five Rb chromosomes, respectively. Chromosomes were stained with DAPI (4′, 6-diamino-2-phenylindole), which distinguishes A-T base-rich pericentromeric heterochromatin. Metaphase plates were stained for 10 min in the dark with 10 µl DAPI (Thermo Fisher Scientific®) in 40 µl 1x PBS buffer, washed with 1x PBS buffer, and sealed with coverslips. The samples were observed under a Nikon® Optiphot fluorescence microscope and digitized using a Nikon® Digital Sight DS-5M camera.
The segmented tracing tool of the Fiji software (Just ImageJ, image processing and analysis in Java) was used to measure both chromatids and thus estimate the average total length of each chromosome (TL). The length of the centromeric region (CL) was measured in pixels and normalized with respect to the chromosomal total length (CL/TLx100), to reduce errors caused by differences in chromosome compaction. For each specimen, 20 metaphase plates were studied, and the total values were recorded according to the number of Rb chromosomes present in each metaphase plate. Hence, there were 160 values for the heterozygous parent that had eight Rb chromosomes and only 40 values for the son D that had only two Rb chromosomes. The estimated pericentromeric region length of Rb metacentric chromosomes (CL/TL) for each offspring was compared with the normalized average length of the pericentromeric regions of the eight Rb chromosomes of the parental heterozygote, which was also measured in 20 metaphase plates.
To test the null hypothesis that Rb chromosomes inherited by the offspring of multiple heterozygous parents have a random and equal distribution for the offspring of male and female heterozygous parents, a theoretical binomial distribution with probability p = 0.5 was generated, whose probability function is given as:
Based on this distribution, the expected frequencies of the appearance of offspring with the Rb chromosome were calculated, and the Chi-2 goodness-of-fit test was used to compare the expected frequencies with those observed. The theoretical distributions, as percentages, are shown in Table 1.
TABLE 1. Expected frequencies of the appearance of offspring with the 0 to 8 Rb chromosomes, calculated on the theoretical binomial distribution with probability p = 0.5.
We used the binomial proportion test to test the null hypothesis that 50% of the offspring could have a larger pericentromeric region of Rb chromosomes than the centromeric average size of 21.51 of the eight heterozygous father Rb chromosomes. The confidence interval was 95% and 5% was used for statistical significance. The data were processed using STATA v. 16.0.
Number of Rb chromosomes present in the offspring of crosses between Rb heterozygous male or female parents and 2n = 40 homozygotes of Mus domesticus.
We analyzed a total of 139 mice that were descended from crosses between heterozygous 2n = 32 with eight metacentric Rb chromosomes and homozygous 2n = 40 with all telocentric chromosomes were analyzed. Of these, 83 came from the cross of heterozygous females and homozygous males, and 56 from the crosses of heterozygous males and homozygous females (Table 2). In the chromosome set of offspring, 0 to 8 Rb metacentric chromosomes were found; however, in six offspring of heterozygous mothers, Rb chromosomes were absent, and no offspring showed seven Rb chromosomes (Table 2). The total number of Rb chromosomes present in the descendants of heterozygous females and males were 256 and 228, respectively. The average number of chromosomes per each descendants of heterozygous females was 3.08 (38.6%) and that of heterozygous males was 4.07 (50.7%), which shows significant difference (<0.01).
TABLE 2. Number of Rb chromosomes inherited by descendants of crosses between Rb heterozygous male or female parents and homozygotes 2n = 40, of Mus m domesticus mice.
Roughly, the set of 484 Rb chromosomes inherited by the total descendants would be equivalent to approximately 44% of the maximum possible number of 1,112 metacentric Rb chromosomes, considering eight Rb chromosomes per 139 descendants. This suggests that although the Rb chromosomes were highly inherited, they were not preferred over telocentric chromosomes.
To assess whether the number of Rb chromosomes inherited by the offspring of multiple heterozygous parents has a random and similar distribution for offspring of male and female heterozygous parents, a theoretical binomial distribution with probability p = 0.5 was generated and the observed data were compared.
In binomial distributions, the expected frequencies of descendants from heterozygous female or male parents according to the inherited Rb chromosome are shown in Table 3, and the comparison between the observed and the expected frequencies of descendants from female and male heterozygous parents is shown in Figure 2.
TABLE 3. Observed number of descendants by inherited Rb chromosome and expected frequencies according to the binomial distribution. Heterozygous females (p-value = 0.0000); Heterozygous males (p-value = 0.0000).
FIGURE 2. Observed frequencies do not fit the theoretical binomial distributions. (A) Observed (bars) descendants from heterozygous females by inherited Rb chromosome and expected (curve) frequencies according to the binomial distribution (p-value = 0.0000). (B) Observed (bars) descendants from heterozygous male by inherited Rb chromosome and expected (curve) frequencies according to the binomial distribution (p-value = 0.0000).
In both cases, the null hypothesis was rejected (p < 0.05), which means that the data do not follow a binomial distribution pattern; therefore, they can be associated with non-random inheritance.
Pericentromeric region size in Rb chromosomes present in the offspring of crosses between heterozygous Rb males and 2n = 40 homozygous females of Mus domesticus.
The size of the pericentromeric regions of the Rb chromosomes was measured in the eight Rb chromosomes of a 2n = 32 heterozygous male, and in the Rb chromosomes inherited by his six sons (Figure 3). The descendants named A, B, C, D, E, and F presented respectively three, five, three, two, four, and five Rb chromosomes and diploid numbers of 37, 35, 37, 38, 36, and 35, respectively (Table 4).
FIGURE 3. Mitotic chromosomes stained with DAPI. (A) Pericentromeric region length of a Rb chromosome; (A′,A′′) Sister chromatid lengths of same chromosome. (B) Heterozygous parent 2n = 32 metaphase plate. Arrows show 8 metacentric Rb chromosomes. (C) Descendent C, 2n = 37 metaphase plate. Arrows show 3 metacentric Rb chromosomes.
TABLE 4. Diploid number, Rb chromosomes and lengths of the pericentromeric regions of Rb chromosomes in the members of a family composed by a multiple Rb heterozygous male and a homozygous female 2n = 40 and their six offspring. The length of the centromeric region (CL) was measured in pixels and normalized with respect to the chromosomal total length (CL/TLx100).
The length of the pericentromeric regions was estimated as a percentage of CL to TL (Figure 3; Table 4). In five of the six descendants, the length of the pericentromeric region was greater than the average length of the pericentromeric regions of the Rb chromosomes of the father (Table 5). In the offspring of this family, the ratio 5/6 is greater than 0.5 (inheritance by chance) and p = 0.0512.
TABLE 5. In 5 of the 6 descendants, the length of the pericentromeric region was verified to be greater than the average length of the pericentromeric regions of the Rb chromosomes of the father. The ratio 5/6 is greater than 0.5 (inheritance by chance) p = 0.0512.
Since the first observation of chromosome behavior during meiosis in the grasshopper Brachystola (Sutton 1903; Carothers 1917), the segregation of homologous chromosomes during meiosis is widely considered as being fundamentally random. However, clear examples have been described in various organisms where meiotic drive or distortion with respect to random meiotic segregation occurs (Bongiorni et al., 2004; Presgraves, 2009; Dawe et al., 2018; Malinovskaya et al., 2020; Kruger and Mueller, 2021; Pajpach et al., 2021).
In natural populations of Mus domesticus, a totally telocentric ancestral karyotype, Robertsonian translocations or centric mergers occur that originate from Rb metacentric chromosomes and reduce the chromosome number (Pialek et al., 2005). Given the unusual speed with that Rb chromosomes have set in some populations, it has been proposed that Rb chromosomes can be inherited preferentially with respect to the ancestral telocentrics in the descendants of heterozygotes (Britton-Davidian et al., 2000; Britton-Davidian et al., 2005). In these cases, the meiotic drive or preferential segregation of some of the chromosomal forms were observed (de Villena and Sapienza, 2001; White et al., 2010; Clark and Akera, 2021).
Crossroads between both chromosomal forms are found in heterozygotes; in the first meiotic prophase, the metacentric Rb chromosome synapsed with the telocentrics, homologous to their arms, forming a trivalent, and subsequently, must segregate between them (Wallace et al., 2002). Although multiple Rb heterozygotes have a loss of spermatocytes at prophase I and majorly at meiotic divisions produce gametes and are fertile (Garagna et al., 2001b; Wallace et al., 2002; Manterola et al., 2009).
In Rb heterozygous males, gametes produced by alternate meiotic segregation, in which the Rb metacentric chromosome separates from the telocentric chromosomes, seem to predominate (Manieu et al., 2014). In human males heterozygous for one Robertsonian chromosome, 70%–80% of the total gametes are normal or balanced gametes (Wang et al., 2017; Lamotte et al., 2018).
In this study, we analyzed whether Rb chromosomes were preferentially inherited with respect to the telocentric chromosomes, by the offspring of heterozygous males and females for eight Rb chromosomes. Our data showed that offspring inherited between 0 and 8 Rb chromosomes and that the distribution of frequencies was not binomial. The non-binomial distribution of the descendants of any of the heterozygous parents suggests that the number of inherited Rb chromosomes would not be a random process, at least in quantitative terms, because Rb chromosomes have not been distinguished between them. We did not find that descendants of heterozygous females inherited more Rb chromosomes than those of heterozygous males. In contrast, we found that male descendants presented a significantly higher number of Rb chromosomes.
Obviously, these results were obtained from heterozygotes of crosses of the CD1 strain (2n = 40) with the Milano II race (2n = 24), and therefore cannot be directly extrapolated to all the Mus musculus domesticus strains/races.
The configuration of the trivalent in the meiotic prophase of Rb heterozygotes could favor the alternating segregation of the anaphase, but this chromosomal configuration could also be relevant in the fixation of the Rb chromosomes in hybrid populations. This could be because this synaptic configuration ensures an obligatory encounter between the Rb chromosome and its telocentric homologous chromosomes, giving rise to the closeness of the abundant pericentromeric heterochromatin of the three gathered chromosomes (Berríos et al., 2014; Berríos 2017). This chromosomal conjunction, which is reiterated in thousands of cells, may also contribute to a second-centric fusion. In addition to the mentioned chromosomal configuration of first meiotic prophase, the intense DNA nicking and repair activity (Neale and Keeney 2006) and the homology of satDNA sequences shared by the mouse telocentric chromosomes create the conditions for new chromosomal rearrangements (Garagna et al., 2001a; Kalitsis et al., 2006). In this way, the concurrence of elements that could favor new chromosome-centric fusions would enhance the chance of those chromosomes regaining the homozygous condition.
The successful fixation of an Rb chromosome in natural populations is the result of the meeting of heterozygotes and the production of homozygous descendants. Any meiotic condition that favors Rb chromosomes in gametes also contributes to the fixation of Rb chromosomes.
The hypothesis that heterozygous females would transfer more Rb chromosomes to their offspring than males arises from findings in different organisms (de Villena and Sapienza, 2001; Fishman and Saunders, 2008; Presgraves, 2009; Dudka and Lampson, 2022). In addition, segregational asymmetry is difficult to explain in males, whereas in female meiosis, such asymmetry is possible because the chromosomes that eventually remain in the oocyte can be inherited, while those that remain in the polocytes are necessarily lost (Lindholm et al., 2016). In this scenario, the differences in centromere strength has been shown to predict the direction of driving. Stronger centromeres, manifested by increased kinetochore protein levels and specific interactions with spindle microtubules, would produce chromosomes that are preferentially retained in the egg (Chmatal et al., 2014; Akera et al., 2017; Wu et al., 2018).
Considering that the main cellular mechanism proposed has been focused on centromere size and its segregational advantage, we found it interesting to show what was observed in the measurement of the pericentromeric and centromeric regions of Rb chromosomes in six descendants of an Rb heterozygote. In mice, the centromeric region contains repetitive centromeric DNA sequences that are enriched in minor satellite repeats, which are prominent sites for centromeric proteins CENP-A and CENP-B assembly (Guenatri et al., 2004; Pajpach et al., 2021). The mouse pericentromeric region contains inactive chromatin and is composed of major satellite repeats, that are required for heterochromatin formation (Guenatri et al., 2004; Pajpach et al., 2021). In 5/6 of the sons, the average size of the centromeric region of the inherited Rb chromosomes was found to be significantly larger than the average size of the same region in the eight Rb chromosomes of the heterozygous male parent. The measurement made involved the centromeric DNA and the abundant pericentromeric heterochromatin located in the proximal p and q regions of the Rb chromosomes. These observations are not comparable with the cellular analyses of segregational efficiency in meiosis of the Rb chromosome according to the size and strength of its centromere because our measurements included the pericentromeric heterochromatin that we do not know how much it may contribute to the meiotic segregation of chromosomes (Chmatal et al., 2014; Iwata-Otsubo et al., 2017). On the other hand, the centromeric region sizes estimated here are on Rb chromosomes that have successfully appeared in the descendants, after overcoming meiotic segregation, gamete differentiation, fertilization, embryonic development and birth. This observation suggests that the larger size of the entire centromeric region is advantageous to the inherited Rb chromosomes.
The raw data supporting the conclusion of this article will be made available by the authors, without undue reservation.
The animal study was reviewed and approved by Ethics Review Committee of the School of Medicine, Universidad de Chile (No. CBA #0441), and by the Ethics Review Committee of the Chilean National Science Foundation FONDECYT-CONICYT.
SB wrote most of the manuscript. GC performed the statistical analyses. EA was responsible for the cross and karyotype analysis. TA made the metaphase plates and AM measured the centromeric regions. All authors have contributed to the manuscript and approved the submitted version.
This work was supported by the Comisión Superior de Investigación Científica (CONICYT-Chile) grant to SB.
Male and female specimens from the original natural populations were donated to our laboratory by Dr. Carlo Redi and Dr. Silvia Garagna from Pavia University, Italy, as part of a collaborative research project.
The authors declare that the research was conducted in the absence of any commercial or financial relationships that could be construed as a potential conflict of interest.
All claims expressed in this article are solely those of the authors and do not necessarily represent those of their affiliated organizations, or those of the publisher, the editors and the reviewers. Any product that may be evaluated in this article, or claim that may be made by its manufacturer, is not guaranteed or endorsed by the publisher.
Akera, T., Chmátal, L., Trimm, E., Yang, K., Aonbangkhen, C., Chenoweth, D. M., et al. (2017). Spindle asymmetry drives non-Mendelian chromosome segregation. Science 358, 668–672. doi:10.1126/science.aan0092
Aniskin, V. M., Benazzou, T., Biltueva, L., Dobigny, G., Granjon, L., and Volobouev, V. (2006). Unusually extensive karyotype reorganization in four congeneric Gerbillus species (Muridae: Gerbillinae). Cytogenet. Genome Res. 112, 131–140. doi:10.1159/000087525
Anton, E., Blanco, J., Egozcue, J., and Vidal, F. (2004). Sperm FISH studies in seven male carriers of robertsonian translocation t(13;14)(q10; q10). Hum. Reprod. 19, 1345–1351. doi:10.1093/humrep/deh232
Baker, R. J., and Bickham, J. W. (1986). Speciation by monobrachial centric fusions. Proc. Natl. Acad. Sci. U. S. A. 83 (21), 8245–8248. doi:10.1073/pnas.83.21.8245
Berríos, S. (2017). Nuclear architecture of mouse spermatocytes: Chromosome topology, heterochromatin, and nucleolus. Cytogenet. Genome Res. 151, 61–71. doi:10.1159/000460811
Berríos, S., Manieu, C., Page, J., Ayarza, E., Lopez-Fenner, J., Manterola, M., et al. (2014). Robertsonian chromosomes and the nuclear architecture of mouse meiotic prophase spermatocytes. Biol. Res. 47 (1), 16–24. doi:10.1186/0717-6287-47-16
Bongiorni, S., Fiorenzo, P., Pippoletti, D., and Prantera, G. (2004). Inverted meiosis and meiotic drive in mealybugs. Chromosoma 112, 331–341. doi:10.1007/s00412-004-0278-4
Britton-Davidian, J., Catalan, J., da Gracia Ramalhinho, M., Ganem, G., Auffray, J. C., Capela, R., et al. (2000). Rapid chromosomal evolution in island mice. Nature 403, 158. doi:10.1038/35003116
Britton-Davidian, J., Catalan, J., Ramalhinho, M. G., Auffray, J. C., Nunes, A. C., Gazave, E., et al. (2005). Chromosomal phylogeny of robertsonian races of the house mouse on the island of Madeira: Testing between alternative mutational processes. Genet. Res. 86, 171–183. doi:10.1017/S0016672305007809
Carothers, E. E. (1917). The segregation and recombination of homologous chromosomes as found in two genera of Acrididae (Orthoptera). J. Morphol. 26, 445–521. doi:10.1002/jmor.1050280205
Chmátal, L., Gabriel, S. I., Mitsainas, G. P., Martínez-Vargas, J., Ventura, J., Searle, J. B., et al. (2014). Centromere strength provides the cell biological basis for meiotic drive and karyotype evolution in mice. Curr. Biol. 24 (19), 2295–2300. doi:10.1016/j.cub.2014.08.017
Clark, F. E., and Akera, T. (2021). Unravelling the mystery of female meiotic drive: Where we are. Open Biol. 11 (9), 210074. doi:10.1098/rsob.210074
Dawe, R. K., Lowry, E. G., Gent, J. I., Harkess, A. E., Hodges, A. L., Hiatt, E. N., et al. (2018). A kinesin-14 motor activates neocentromeres to promote meiotic drive in maize. Cell 173, 839–850. doi:10.1016/j.cell.2018.03.009
de Villena, F. P. M., and Sapienza, C. (2001). Female meiosis drives karyotypic evolution in mammals. Genetics 159, 1179–1189. doi:10.1093/genetics/159.3.1179
Dudka, D., and Lampson, M. A. (2022). Centromere drive: Model systems and experimental progress. Chromosome Res. 30, 187–203. doi:10.1007/s10577-022-09696-3
Eaker, S., Pyle, A., Cobb, J., and Handel, M. A. (2001). Evidence for meiotic spindle checkpoint from analysis of spermatocytes from Robertsonian chromosome heterozygous mice. J. Cell Sci. 114, 2953–2965. doi:10.1242/jcs.114.16.2953
Fishman, L., and Saunders, A. (2008). Centromere-associated female meiotic drive entails male fitness costs in monkeyflowers. Science 322 (5907), 1559–1562. doi:10.1126/science.1161406
Garagna, S., Marziliano, N., Zuccotti, M., Searle, J. B., Capanna, E., and Redi, C. A. (2001a). Pericentromeric organization at the fusion point of mouse Robertsonian translocation chromosomes. Proc. Natl. Acad. Sci. U. S. A. 98, 171–175. doi:10.1073/pnas.98.1.171
Garagna, S., Zuccotti, M., Capanna, E., and Redi, C. A. (2002). High-resolution organization of mouse telomeric and pericentromeric DNA. Cytogenet. Genome Res. 96, 125–129. doi:10.1159/000063028
Garagna, S., Zuccotti, M., Thornhill, A., Fernández-Donoso, R., Berrios, S., Capanna, E., et al. (2001b). Alteration of nuclear architecture in male germ cells of chromosomally derived subfertile mice. J. Cell Sci. 114, 4429–4434. doi:10.1242/jcs.114.24.4429
Guenatri, M., Bailly, D., Maison, C., and Almouzni, G. (2004). Mouse centric and pericentric satellite repeats form distinct functional heterochromatin. J. Cell Biol. 166, 493–505. doi:10.1083/jcb.200403109
Hauffe, H. C., Gimenez, M. D., and Searle, J. B. (2012). “Chromosomal hybrid zones in the house mouse,” in Evolution of the house mouse. Editors M. Macholán, S. J. E. Baird, P. Munclinger, and J. Piálek (Cambridge: Cambridge University Press), 407–430.
Iwata-Otsubo, A., Dawicki-McKenna, J. M., Akera, T., Falk, S. J., Chmátal, L., Yang, K., et al. (2017). Expanded satellite repeats amplify a discrete CENP-A nucleosome assembly site on chromosomes that drive in female meiosis. Curr. Biol. 7 (2715), 2365–2373. e8. doi:10.1016/j.cub.2017.06.069
Kalitsis, P., Griffiths, B., and Choo, K. H. A. (2006). Mouse telocentric sequences reveal a high rate of homogenization and possible role in Robertsonian translocation. Proc. Natl. Acad. Sci. U. S. A. 103, 8786–8791. doi:10.1073/pnas.0600250103
King, M. (1993). Species evolution: The role of chromosome change. Cambridge (UK): Cambridge University Press.
Kruger, A. N., and Mueller, J. L. (2021). Mechanisms of meiotic drive in symmetric and asymmetric meiosis. Cell. Mol. Life Sci. 78 (7), 3205–3218. doi:10.1007/s00018-020-03735-0
Lamotte, A., Martinez, G., Devillard, F., Hograindleur, J. P., Satre, V., Coutton, C., et al. (2018). Is sperm FISH analysis still useful for robertsonian translocations? Meiotic analysis for 23 patients and review of the literature. Basic Clin. Androl. 28 (5), 5–11. doi:10.1186/s12610-018-0069-z
Lindholm, A. K., Dyer, K. A., Firman, R. C., Fishman, L., Forstmeier, W., Holman, L., et al. (2016). The ecology and evolutionary dynamics of meiotic drive. Trends Ecol. Evol. 31, 315–326. doi:10.1016/j.tree.2016.02.001
Malinovskaya, L. P., Zadesenets, K. S., Karamysheva, T. V., Akberdina, E. A., Kizilova, E. A., Romanenko, M. V., et al. (2020). Germline-restricted chromosome (GRC) in the sand Martin and the pale Martin (Hirundinidae, Aves): Synapsis, recombination and copy number variation. Sci. Rep. 10, 1058–1110. doi:10.1038/s41598-020-58032-4
Manieu, C., González, M., López-Fenner, J., Page, J., Ayarza, E., Fernández-Donoso, R., et al. (2014). Aneuploidy in spermatids of Robertsonian (Rb) chromosome heterozygous mice. Chromosome Res. 22, 545–557. doi:10.1007/s10577-014-9443-7
Manterola, M., Page, J., Vasco, C., Berríos, S., Parra, M. T., Viera, A., et al. (2009). A high incidence of meiotic silencing of unsynapsed chromatin is not associated with substantial pachytene loss in heterozygous male mice carrying multiple simple Robertsonian translocations. PLoS Genet. 5, e1000625. doi:10.1371/journal.pgen.1000625
Nanda, I., Schneider-Rasp, S., Winking, H., and Schmid, M. (1995). Loss of telomeric sites in the chromosomes of Mus musculus domesticus (Rodentia: Muridae) during Robertsonian rearrangements. Chromosome Res. 3, 399–409. doi:10.1007/BF00713889
Pajpach, F., Wu, T., Shearwin-Whyatt, L., Jones, K., and Grützner, F. (2021). Flavors of non-random meiotic segregation of autosomes and sex chromosomes. Genes (Basel) 12 (9), 1338–1360. doi:10.3390/genes12091338
Pialek, J., Hauffe, H. C., and Searle, J. B. (2005). Chromosomal variation in the house mouse. Biol. J. Linn. Soc. Lond. 84, 535–563. doi:10.1111/j.1095-8312.2005.00454.x
Presgraves, D. (2009). “Drive and sperm,” in The evolution and genetics of male meiotic drive. Editors T. Birkhead, D. Hosken, and S. Pitnick. 1st ed. (Amsterdam, Netherlands: Elsevier). 9780123725684.
Pylyp, L. Y., Zukin, V. D., and Bilko, N. M. (2013). Chromosomal segregation in sperm of Robertsonian translocation carriers. J. Assist. Reprod. Genet. 30 (9), 1141–1145. doi:10.1007/s10815-013-0067-1
Rieseberg, L. H. (2001). Chromosomal rearrangements and speciation. Trends Ecol. Evol. 16, 351–358. doi:10.1016/s0169-5347(01)02187-5
Wallace, B. M. N., Searle, J. B., and Everett, C. A. (2002). The effect of multiple simple Robertsonian heterozygosity on chromosome pairing and fertility of wild-stock house mice (Mus musculus domesticus). Cytogenet. Genome Res. 96, 276–286. doi:10.1159/000063054
Wang, B., Nie, B., Tang, D., Li, R., Liu, X., Song, J., et al. (2017). Analysis of meiotic segregation patterns and interchromosomal effects in sperm from 13 robertsonian translocations. Balk. J. Med. Genet. 20 (1), 43–50. doi:10.1515/bjmg-2017-0003
White, T. A., Bordewich, M., and Searle, J. B. (2010). A network approach to study karyotypic evolution: The chromosomal races of the common shrew (Sorex araneus) and house mouse (Mus musculus) as model systems. Syst. Biol. 59, 262–276. doi:10.1093/sysbio/syq004
Wu, T., Lane, S. I. R., Morgan, S. L., and Jones, K. T. (2018). Spindle tubulin and MTOC asymmetries may explain meiotic drive in oocytes. Nat. Commun. 9, 2952. doi:10.1038/s41467-018-05338-7
Keywords: Robertsonian chromosomes, meiotic drive, meiosis, heterozygotes, Mus domesticus
Citation: Ayarza E, Cavada G, Arévalo T, Molina A and Berríos S (2022) Quantitative analysis of Robertsonian chromosomes inherited by descendants from multiple Rb heterozygotes of Mus m. Domesticus. Front. Cell Dev. Biol. 10:1050556. doi: 10.3389/fcell.2022.1050556
Received: 21 September 2022; Accepted: 14 November 2022;
Published: 25 November 2022.
Edited by:
Ricardo Benavente, Julius Maximilian University of Würzburg, GermanyReviewed by:
Alberto Viera, Autonomous University of Madrid, SpainCopyright © 2022 Ayarza, Cavada, Arévalo, Molina and Berríos. This is an open-access article distributed under the terms of the Creative Commons Attribution License (CC BY). The use, distribution or reproduction in other forums is permitted, provided the original author(s) and the copyright owner(s) are credited and that the original publication in this journal is cited, in accordance with accepted academic practice. No use, distribution or reproduction is permitted which does not comply with these terms.
*Correspondence: Soledad Berríos, c2JlcnJpb3NAbWVkLnVjaGlsZS5jbA==
Disclaimer: All claims expressed in this article are solely those of the authors and do not necessarily represent those of their affiliated organizations, or those of the publisher, the editors and the reviewers. Any product that may be evaluated in this article or claim that may be made by its manufacturer is not guaranteed or endorsed by the publisher.
Research integrity at Frontiers
Learn more about the work of our research integrity team to safeguard the quality of each article we publish.