- 1School of Clinical Medicine, Changchun University of Chinese Medicine, Changchun, China
- 2School of Pharmacy, Changchun University of Chinese Medicine, Changchun, China
Tendinopathy has a high incidence in athletes and the aging population. It can cause pain and movement disorders, and is one of the most difficult problems in orthopedics. Animal models of tendinopathy provide potentially efficient and effective means to develop understanding of human tendinopathy and its underlying pathological mechanisms and treatments. The selection of preclinical models is essential to ensure the successful translation of effective and innovative treatments into clinical practice. Large animals can be used in both micro- and macro-level research owing to their similarity to humans in size, structure, and function. This article reviews the application of large animal models in tendinopathy regarding injuries to four tendons: rotator cuff, patellar ligament, Achilles tendon, and flexor tendon. The advantages and disadvantages of studying tendinopathy with large animal models are summarized. It is hoped that, with further development of animal models of tendinopathy, new strategies for the prevention and treatment of tendinopathy in humans will be developed.
Introduction
Tendons are dense connective tissues that connect muscle to bone and transmit the forces created by muscles to the bones, which causes movement. Trauma, strenuous exercise, or overuse can lead to acute tendon rupture or chronic degenerative disease, resulting in pain, movement disorders and other clinical symptoms (Dean et al., 2017). Tendon injuries often occur in areas with frequent movements and high stress. The frequent sites of tendon injuries in humans are demonstrated the schematic diagram in Figure 1. Tendon injury is very common and can be debilitating, but tendon repair remains a clinical challenge for orthopedic medicine.
Tendon biology is poorly understood compared to other components of the musculoskeletal system (Gaut and Duprez, 2016). Development of effective therapeutics is hindered by the lack of fundamental guiding data on the biology of tendon development, signal transduction, mechanotransduction, and the basic mechanisms underlying tendon pathogenesis and healing (Andarawis-Puri et al., 2015). The ability to perform invasive assays in animal models provides researchers with powerful opportunities to improve understanding of many aspects of tendinopathy (Warden, 2007). The efficacy and safety of stem cells, growth factors, drugs, tissue-engineered tendons, and other therapeutic methods must be validated in animal models before using them in clinical trials. Therefore, to study the pathogenesis and treatment of tendinopathy, animal models are indispensable (Habets et al., 2018; Longo et al., 2018).
The pathology and pathophysiology of tendinopathy in humans is currently poorly understood, meaning that the validation of animal models is difficult. Two common and established features associated with tendinopathy in humans are histopathological changes and mechanical weakening of the tendon (Warden, 2007). The prominent histological and molecular features of tendinopathy include increased immune cells and inflammatory mediators, enhanced cellular apoptosis, dysregulated extracellular matrix homeostasis, disorganization of collagen fibers, an increase in the microvasculature, and sensory nerve innervation (Millar et al., 2021). The most reasonable way to generate tendinopathy in animal models is to introduce known and potential causative factors. At present, the most used methods of modeling tendinopathy include mechanical stimulation, chemical induction, and surgical operation.
The principal animals used in the study of tendinopathy include rats, mice, chickens, rabbits, sheep, horses, dogs, pigs, and so on. The research objectives of tendinopathy are stratified by two main concerns (Liu et al., 2021). One is at the micro level, such as the biological characteristics of tendon-bone healing, the potential signaling pathway and the genetic mechanism of the disease, etc.; verified small animal models can usually meet the needs of such research. The second is at the macro level, such as biomechanical modeling, optimization of surgical techniques, evaluation of new instruments or devices, etc. This type of research requires animal models to be highly similar to humans in structure and function, so large animal models, especially non-human primate models, are usually more suitable. In most cases, these two goals are not separate; both micro and macro levels need to be considered. Large animals have more prospects for application because they can be used for both microscopic and macroscopic research.
This article reviews and analyzes the large animal models used in the study of tendinopathy in recent years with respect to four aspects: rotator cuff injury, patellar ligament injury, Achilles tendon injury and flexor tendon injury. The article aims to provide reference for further research.
Rotator cuff injury model
Rotator cuff injury is the most common shoulder injury. After injury, the tissue often presents with irreversible muscle atrophy, stiffness and fatty infiltration (Derwin et al., 2007). The ideal animal model of rotator cuff injury should have a similar anatomical structure and function to humans to be able to simulate the local microenvironment and histological changes after tendon injury. Previous studies have evaluated the application of various experimental animals in rotator cuff injury modeling (Takase et al., 2017; Lebaschi et al., 2018). But, in fact, except for humans, most animals rely on limbs for support. Even if there is occasional standing behavior supported on double hind limbs for a short time, the double forelimbs still have more weight-bearing functions than humans. Thus, the anatomy of the shoulder is different between humans and most other animals. The shoulder structure of nonhuman primates is the closest anatomical and physiological analog to that of humans. A schematic diagram of the scapula structure of human and different animals is shown in Figure 2. Large animal models of rotator cuff injury are mainly rabbits, sheep, dogs and cattle, which are suitable for studying the mechanisms of muscle degeneration, stent repair technology and improved surgical methods (Bisson et al., 2008; Liu et al., 2018; Smith et al., 2018; Roßbach et al., 2020).
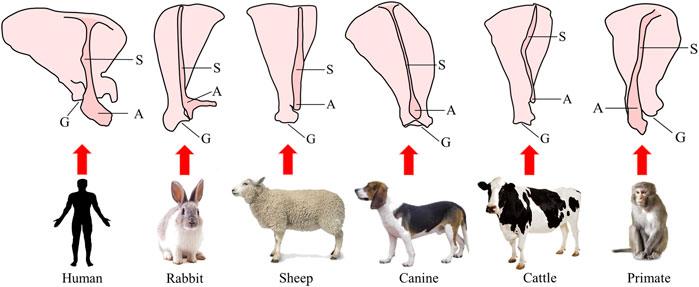
FIGURE 2. Schematic diagram of the structure of the scapula in humans and different animals. S, scapular spine; A, acromion; G, glenoid cavity.
Rabbits are one of the commonly used animal models in orthopedic studies. Hyman et al. presented a detailed architectural and physiological analysis of chronic tear and repair compared with age-matched control rabbit supraspinatus muscles (Hyman et al., 2021). Previous studies have used rabbit supraspinatus tendon or infraspinatus tendon tear models to analyze the tendon-bone healing effects of platelet-rich plasma and ozone therapy (Gurger et al., 2021), microfracture apertures (Sun et al., 2020), decellularized tendon sheets (Liu et al., 2018), preservation of native enthesis (Su et al., 2018) and nano-calcium silicate mineralized fish scale scaffolds (Han et al., 2023). Lee et al. studied the histological and biomechanical changes in a rabbit model of chronic rotator cuff tears repaired by human dermal fibroblasts (Lee et al., 2021). Yildiz et al. used a rabbit irreparable rotator cuff tear model to compare the healing of two types of upper joint capsule reconstruction grafts (Yildiz et al., 2019). Xu et al. explored the in vivo biomechanical and histological processes of the rerouting biceps tendon to treat chronic irreparable rotator cuff tears in a rabbit model (Xu et al., 2022). In addition, Grumet et al. proposed that the rabbit subscapularis muscle model could be used for the study of rotator cuff lesions. They found that the rabbit glenohumeral joint had a bone channel formed by the supraglenoid tubercle, coracoid process and infraglenoid tubercle. The rabbit subscapularis muscle passes through this bone channel and to the lesser tubercle of the humerus, similar to the human supraspinatus tendon that passes under the acromion to the greater tubercle of the humerus. More importantly, the structure traversed within this bone tunnel is the tendon part of the subscapularis muscle. Another advantage of this model is the presence of fatty infiltration of the muscle after the tendon is severed (Grumet et al., 2009). Vargas-Vila et al. studied the progression of muscle loss and fat accumulation in a rabbit model of rotator cuff tear (Vargas-Vila et al., 2022). Wang et al. studied that adipose stem cell-derived exosomes decreased fatty infiltration and enhanced rotator cuff healing in a rabbit model of chronic tears (Wang et al., 2020).
Sheep can be used as a practical, large animal model. This model has been gradually used in various orthopedic studies, including studies on rotator cuff repair, due to its easy availability, ease of rearing, and relatively low cost. The large size of the infraspinatus tendon in sheep makes them particularly suitable for in vitro biomechanical studies (Camenzind et al., 2016; Guo et al., 2016). In addition, the sheep model has also been used to investigate the effect on rotator cuff repair of engineered tissue grafting (Novakova et al., 2018), perforated anchors, or collagen scaffolds loaded with tenocytes (Roßbach et al., 2020). Coleman et al. successfully constructed a chronic rotator cuff injury repair model by wrapping the broken end of the ovine infraspinatus tendon with a membrane (Coleman et al., 2003). Sener et al. used two different fixation methods to investigate the biomechanical and histological characteristics of patellar tendon-bone autografting and free flexor-tendon autografting in reconstruction of an infraspinatus defect in sheep (Sener et al., 2004). Romeo et al. evaluated the mechanical, structural, and histologic quality of rotator cuff repairs augmented with an interposition electrospun nanofiber scaffold in an acute sheep model (Romeo et al., 2022). Luan et al. found that muscle fatty infiltration and fibrosis would also occur after repair of acute rotator cuff injury in sheep (Luan et al., 2015). In a sheep rotator cuff model, tenotomy predominantly induces fatty infiltration, and denervation induces mostly muscle atrophy (Wieser et al., 2021). Some scholars have further revealed the possible mechanisms of muscle atrophy and degeneration after rotator cuff injury through sheep models, which is expected to provide a new target for clinical treatment (Gerber et al., 2017; Ruoss et al., 2018a; Ruoss et al., 2018b).
The canine rotator cuff injury model has been used to study a variety of rotator cuff repair materials or surgical methods (Adams et al., 2006; Derwin et al., 2007; Roller et al., 2018; Smith et al., 2018). Adams et al. used a full-thickness tear model of the Canis infraspinatus tendon to evaluate the effect of using human acellular dermal matrix grafting to enhance rotator cuff repair (Adams et al., 2006). Smith et al. compared the augmentation of partial rotator cuff tears of biologic scaffolds in a canine model (Smith et al., 2020). Derwin et al. evaluated the applicability of the canine rotator cuff acute full-thickness injury repair model; their results demonstrated that the repair strength immediately after surgery depended on the suture type and method, although all repaired tendons had retears shortly after surgery (Derwin et al., 2007). Derwin et al. evaluated the extent to which augmentation of acute repair of rotator cuff tendons with a newly designed poly-L-lactide repair device would improve functional and biomechanical outcomes in a canine model (Derwin et al., 2009). In addition, the canine model can replicate muscle atrophy and fatty infiltration following rotator cuff injury in humans. Safran et al. developed a canine rotator cuff chronic injury model to explore the dynamic performance, muscle volume, and fat infiltration of infraspinatus muscles over time (Safran et al., 2005). The canine rotator cuff injury repair model can also tolerate various postoperative rehabilitation programs, such as plaster fixation, suspension fixation, and treadmill exercises (Lebaschi et al., 2016). Ji et al. tested a non-weight-bearing (NWB) canine model for rotator cuff repair research. In this model, the extensor muscles of the elbow and wrist were denervated by cutting the radial nerve proximal to the innervation region of the triceps brachii, to prevent weight bearing and muscle contraction of the affected limb after surgery (Ji et al., 2015). Liu et al. reported a novel biomaterial with engineered tendon-fibrocartilage-bone composite and bone marrow-derived mesenchymal stem cell sheet; the construct was tested for augmentation of rotator cuff repair using a canine NWB model (Liu et al., 2019).
The rotator cuff injury model of cattle is used in biomechanical research. Previous studies have investigated the biomechanical differences between single-row and double-row rotator cuff repairs (Mahar et al., 2007), the initial fixation strength of different suture methods under cyclic loading (Anderl et al., 2012), the influence of suture materials on the biomechanics of the suture–tendon interface (Bisson et al., 2008), and the pull out strength of different stitches (Bisson and Manohar, 2010). Park et al. evaluated the interface pressure of the rotator cuff tendon to the greater tuberosity for different rotator cuff repair techniques. Simulated rotator cuff tears over a 1 x 2 cm infraspinatus insertion footprint were created in 25 bovine shoulders. A transosseous tunnel simple suture technique, suture anchor simple technique, and suture anchor mattress technique were used for repair. Their results showed that the transosseous tunnel rotator cuff repair technique created significantly more contact and greater overall pressure distribution over a defined footprint when compared with suture anchor techniques (Park et al., 2005). The main advantage of the bovine rotator cuff injury model is that there is little difference in rotator cuff size and quality of tissue between different individuals, which helps to ensure the consistency of the replication model (Liu et al., 2021).
From the perspective of translational medicine, nonhuman primates are undoubtedly the most ideal species for shoulder joint research, as they are the most similar to humans in anatomical structure and physiology. Plate et al. studied age-related degenerative functional, radiographic, and histological changes of the shoulder in nonhuman primates (Plate et al., 2013). Sonnabend et al. stated that baboons make the best animal model for rotator cuff repair research based on their shoulders’ similarity to the human shoulder joint (Sonnabend et al., 2010). Xu et al. used the African green monkey rotator cuff injury model to evaluate the repair effect and immune response of a non-cross-linked porcine dermal extracellular matrix graft; the results of this study demonstrated that the graft integrated well with the host tendon tissue and did not cause significant inflammatory reactions (Xu et al., 2012). However, the high cost of primate rearing, complexity of management, and difficulties in ethical approval limit its experimental application (Warden, 2007).
Patellar tendon injury model
The patellar tendon is located on the surface of the knee joint, connecting the patella and the tibia of the lower leg. It is one of the knee extensor devices. Due to the constant movement of the human knee joint, the patellar tendon is under periodic load for a long time, and the patellar tendon has become a common and frequent site of tendinopathy (Hast et al., 2014). In general, the patellar tendon is relatively large and its anatomical position is superficial and easy to access (Longo et al., 2011). The animal model of this part is convenient for biomechanical testing and research because the dual osseous attachment of the patellar tendon can be pulled without direct injury to the tendon material. However, the patellar tendon is wide and thin, which makes it difficult to inject drugs into. It requires delicate local operations, which require higher technical requirements for operators. Large animal models used in the study of tendinopathy include rabbit, sheep, dog, cow, and pig.
Patellar tendinitis in athletes is a chronic injury caused by repeated pulling on the patellar tip attachment of the patellar tendon due to excessive training, which can overload the patella and patellar tendon. To study the pathogenesis and development of patellar tendon fibrosis, a rabbit model of patellar tendon fibrosis was established by electrical stimulation-induced jumping (Liu et al., 2020). Intense exercise can cause acute injury to the proximal patella. Wang et al. detected the effect of the time of training after injury on healing (Wang et al., 2017). Xu et al. established a rabbit model of partial patellar resection to verify whether combined magnetic field technology can promote the healing of the bone–tendon junction through endochondral ossification (Xu et al., 2014). Kim et al. evaluated the healing capacity of injected atelocollagen as a treatment scaffold for patellar tendon defects (Kim et al., 2021). Other studies have compared the effects of human recombinant epidermal growth factor and platelet-rich plasma on the histological healing process and gene expression profile using a rabbit patellar tendon incision model (Lyras et al., 2016; Sarıkaya et al., 2017).
The sheep knee joint is often used as a model in orthopedic research. Kayser et al. provided ultrasound images of the sheep knee joint that were relevant in musculoskeletal research (Kayser et al., 2019). The biomechanical characteristics of the patellar tendon, such as elasticity and stiffness, are of paramount importance and constitute major outcome measures in research. Kayser et al. assessed whether the stiffness of the healthy ovine patellar tendon changed with age and weight in a population of normal animals (Kayser et al., 2022). Thangarajah et al. used a sheep model of acute tendon contraction to study the role of the demineralized bone matrix in the treatment of tendon tear retractions. The patellar tendon was detached from the tibial tuberosity and a complete distal tendon transverse defect measuring 1 cm was created. The tendon was reconnected with suture anchors, and the defect was bridged with a demineralized bone matrix and minimally invasive mesenchymal stem cells (Thangarajah et al., 2016). Enea et al. performed surgery on the right hind leg of 48 Welsh goats, removed the central third of the patellar tendon, replaced it with an implant, and studied the effect of implanted collagen on tendon and ligament tissue regeneration in vivo (Enea et al., 2013).
One study examined the biomechanical and histological properties of the medial third of the patellar tendon in dogs; the results found a direct contrast to those of the central third (Linder et al., 1994). Gersoff et al. used the canine patellar tendon defect model to perform full-thickness proximal and distal flap defects of the patellar tendon in eight purpose-bred research mongrel dogs, and compared the healing of the Artelon patch-augmented tendon and tendon repair alone (Gersoff et al., 2019). A total of 27 patellar tendons in male Beagles were surgically subjected to stretching by a small diameter or a large diameter rod to induce damage due to strain, and were evaluated at 4- and 8-week intervals using quantitative magnetic resonance imaging, biomechanical testing, and histology (Pownder et al., 2021). de Moya and Kim quantified changes in the patellar tendon length following surgical correction of medial patellar luxation in dogs and evaluated the potential risk factors associated with patellar tendon elongation using dogs that underwent surgery for medial patellar luxation correction and had 2–3 months follow ups (de Moya and Kim, 2020).
In animal models of tendinopathy, the main ex vivo measurements of interest are the mechanical properties of the tendon. Reduced mechanical properties leading to an increased likelihood of spontaneous rupture is the result of clinical tendinopathy. The bovine patellar tendon is often used in biomechanical studies owing to its large size. Flanigan et al. evaluated the biomechanical properties of FiberWire, a novel suture material, compared with standard Ethibond sutures for tendon injury repair in the bovine knee joint (Flanigan et al., 2011). A three-step tensile stress-relaxation test was conducted on the patellar tendons of bovines, and the result revealed that long-term relaxation behavior is affected or implied by proteoglycans and crimp angle, possibly relating to slow structural reorganization of the tissue (Ristaniemi et al., 2021). To describe and compare the characteristics and coordination between knee ligaments and patellar tendons, dumbbell-shaped tensile specimens were cut from bovine knee ligament and patellar tendon for tensile testing. This study improved the understanding of the elasticity, viscoelasticity and failure characteristics of knee ligaments and patellar tendons (Ristaniemi et al., 2018).
Neovascular embolization is a therapeutic strategy for chronic musculoskeletal pain. Ghelfi et al. established a large animal model of patellar tendinopathy with neovascularization by percutaneous injection of increased doses of collagenase in nine 3-month-old male piglets. The model is feasible, safe and reproducible, which is helpful for the study of a new treatment for direct endovascular embolization of neovascularization (Ghelfi et al., 2021).
Achilles tendon injury model
As the largest and longest tendon of the human body, the Achilles tendon can typically bear more than 12.5 times the weight of the individual. Long-term, high-intensity load increases the incidence of Achilles tendinopathy (Komi, 1990). The Achilles tendon is also one of the most thoroughly researched elements of animal models of tendinopathy. It is advantageous to study because of its superficial parts, convenience of operation, and ease of sampling, which are conducive to the study of the mechanisms of tendinopathy. The Achilles tendon has been widely used in a variety of animal models (Hast et al., 2014; Loiselle et al., 2016). Large animals such as sheep or cattle are popular due to the appropriate size of their Achilles tendon, the weight bearing similar to humans, and their suitability for clinical evaluation. Rabbits are also suitable for Achilles tendon injury models because their Achilles tendon size allows for surgical approaches and accurate specimen examination (Doherty et al., 2006).
Skalec et al. conducted an anatomical and histological analysis on eight female New Zealand rabbits and comprehensively described the macroscopic and microscopic morphology of their Achilles tendon and its related structures (Skalec et al., 2019). The Achilles tendon transection model is a common model of injury that is used to study the biomechanical properties of healed tendons and the degree of adhesion formation (Meier Bürgisser et al., 2016), as well as the time-dependent changes of strain ratios (SRs) and the correlation between SRs and mechanical and histological properties of healed tissue (Yamamoto et al., 2017). It was also used to compare the effects of early activity and fixation on postoperative healing of rabbit Achilles tendon rupture (Jielile et al., 2016). The healing of tendons through open and percutaneous repair techniques was compared by histological, electron microscopic and biomechanical investigation (Yılmaz et al., 2014). Achilles tendon defects occur frequently in traumatic injuries. The rabbit Achilles tendon defect model was used to evaluate the repair effect of decellularized bovine tendon sheets (Zhang et al., 2018), polyethylene terephthalate artificial ligaments (Li et al., 2016), and collagen implants with or without a polydioxanone sheath for Achilles tendon defect reconstruction (Meimandi-Parizi et al., 2013). The Achilles tendinopathy model induced by bilateral Achilles tendon injection of collagenase in rabbits accurately represents the progressive histological and biomechanical characteristics of human chronic Achilles tendinopathy (de Cesar Netto et al., 2018). A rabbit model of ischemic injury caused by Achilles tendon ligation was used to compare a series of changes in Achilles tendon morphology and strain in the early stage of Achilles tendinopathy (Ahn et al., 2017).
Achilles tendon rupture is common in sheep Achilles tendon injury models. Previous studies have evaluated the repair effect of cross-linked acellular porcine dermal patches, platelet-rich plasma fibrin matrixes (Sarrafian et al., 2010), exogenous growth differentiation factor CDMP-2 (Virchenko et al., 2008) and plasma rich in growth factors (Fernández-Sarmiento et al., 2013) on sheep Achilles tendon ruptures. Bruns et al. studied the spontaneous healing process of sheep Achilles tendons after transection and partial resection by means of histological and biomechanical analyses (Bruns et al., 2000). Dündar et al. used the sheep Achilles tendon tear model to compare the biomechanical properties of modified Kessler, Bunnell and Tsuge technology in repairing sheep Achilles tendon tears (Dündar et al., 2020). Leung et al. simulated bone–bone, bone–tendon and tendon–tendon repairs with osteotomy of the calcaneus, reattachment of Achilles tendon to the calcaneus after removal of the insertion, and tenotomy of the Achilles tendon resection in 47 goats (Leung et al., 2015). There have also been studies using collagenase injections to create Achilles tendon injury models. Serrani et al. used real-time elastosonography to monitor the progress of Achilles tendon healing after an experimentally induced tendinopathy (Serrani et al., 2021). Facon-Poroszewska et al. compared the efficacy of radial pressure wave therapy with injections of autologous adipose-derived stem cells or platelet rich plasma in the therapeutic procedure for collagenase-induced Achilles tendinopathy in sheep (Facon-Poroszewska et al., 2019).
Cattle Achilles tendons are larger and are often used for improvements in surgical suture techniques. Tian et al. designed the Locking Block Modified Krackow (LBMK) peri-tendon fixation technique for minimally invasive surgery and then compared the biomechanics of LBMK with Kessler and percutaneous Achilles repair system techniques with a simulated early rehabilitation program (Tian et al., 2020b).Tian et al. used 20 fresh bovine Achilles tendon specimens and randomly divided them into two groups, which were respectively sutured by open Giftbox Achilles tendon repair and minimally invasive LBMK techniques. The early rehabilitation simulation scheme was used to compare the biomechanics of the two techniques (Tian et al., 2020a).
There are few studies on pigs as animal models for Achilles tendon injury. Previous studies used pigs to study the biological characteristics of Achilles tendons or collected the Achilles tendons of pigs as materials for tendon injury repair. Zhang et al. characterized the structural components, vascularity, and resident tendon cells of the porcine Achilles tendon (Zhang et al., 2021). Lohan et al. achieved tendon-like tissue formation by implanting decellularized porcine Achilles tendons that were recellularized with human hamstring tendon-derived tenocytes into nude mice (Lohan et al., 2018).
Flexor tendon injury model
Animal models involving flexor tendons include the superficial flexor digitorum and flexor digitorum profunda tendons. The tendon healing process here is very slow due to the presence of ischemic and cell deficiency, which is consistent with the healing characteristics of tendinopathy (Adams and Habbu, 2015). Flexor tendons are relatively small, limiting their use in small animals. At the same time, large animals have the advantage of naturally occurring tendinopathy (Longo et al., 2011). At present, large mammals such as sheep and horses are the main animal models of flexor tendon injuries. However, large animals cost more, and rabbits offer a good compromise. Moreover, rabbit flexor tendons are more like human tendons in diameter and the presence of a perceptible synovial sheath (Bottagisio and Lovati, 2017).
To understand the repair process of flexor tendon injuries, studies have used the rabbit flexor tendon injury model to detect the expression of mast cells, fibroblasts, neuropeptides (Berglund et al., 2010) and growth response factor-1 (Derby et al., 2012). Progressive tendon adhesion is a major challenge in flexor tendon repair. Liao et al. developed an anti-adhesion scaffold for surgical repair of the flexor tendon in a rabbit model (Liao et al., 2018). Chen et al. investigated the preventive effects and mechanism of chitosan on tendon repair adhesion in rabbit flexor tendons (Chen et al., 2015). Previous studies have explored the effects on the healing of flexor tendon injury in rabbits investigated fibrin glue (He et al., 2013), autologous platelet-rich fibrin (Liao et al., 2017), bone marrow mesenchymal stem cells (He et al., 2015), adipose-derived stem cells (de Lima Santos et al., 2019), growth differentiation factor-5 (Henn et al., 2010), and lactoferrin peptide (Håkansson et al., 2012). A reinforced tubular, medicated electrospun construct was developed for rabbit deep flexor tendon repair that combines mechanical strength with the release of anti-inflammatory and anti-adhesion drugs (Peeters et al., 2022).
Sheep flexor tendon injury models include flexor tendon transection, collagenase induction, and partial tendon resection to create defects. To explore the mechanism of flexor tendon injury, Biasutti et al. recorded the gene expression, and histopathology and biomechanical changes that occur throughout the superficial digital flexor tendon up to 16 weeks post-injury in a sheep surgical model (Biasutti et al., 2017). Previous studies have investigated the repair effects of synovial multipotent cells (Khan et al., 2020), peripheral blood-derived mesenchymal stromal cells, and platelet-rich plasma (Martinello et al., 2013) on experimentally injured deep digital flexor tendons of sheep. The effects of the multiwave locked system were investigated in the acute phase of collagenase-induced tendon lesions in six adult sheep (Iacopetti et al., 2015). De Mattos et al. examined the effect of nano light emitting diode phototherapy on tendinopathy by partial tenotomies measuring 0.2 × 0.5 cm on the second third of the superficial flexor tendons of 10 healthy sheep (de Mattos et al., 2015). The sheep flexor tendon injury model is also used to improve surgical suture techniques. Uslu et al. randomly divided 60 fresh sheep forelimb flexor digitorum profundus tendons into three groups, and used two-, four-, and eight-strand suture techniques, respectively, to investigate the biomechanical relationship between the diameter of the core suture and the final repair strength of the surrounding suture with an increase of the number of suture lines (Uslu et al., 2014). Doğramaci et al. used 20 fresh flexor digitorum profundus tendons from the forelimbs of healthy adult sheep to improve suture techniques and evaluate their mechanical properties after repair (Doğramaci et al., 2009).
The flexor digitorum superficialis tendon of horses is a frequently injured structure that is functionally equivalent to the human Achilles tendon. Both play a key role in energy storage systems during high-speed exercise and can accumulate microdamage related to exercise and age, and are prone to rupture during strenuous activities (Patterson-Kane et al., 2012). To study the biological mechanism of age-related tendon injury, some studies have performed qualitative and quantitative analyses on the gene expression and collagen fiber diameters of the flexor digitorum superficially and the flexor digitorum profunda tendons of horses at different ages (Ribitsch et al., 2020). Some researchers studied the therapeutic effect of fetal-derived embryonic-like stem cells using a collagenase gel-physical defect model in the mid-metacarpal region of the superficial digital flexor tendon of horses (Watts et al., 2011). Durgam et al. described the value of intralesional tendon-derived progenitor cell injections in equine flexor tendinitis using collagenase-induced tendinitis in both front superficial digital flexor tendons of horses (Durgam et al., 2016). Some studies examined superficial flexor tendon injuries in both horse forelimbs to explore the safety and effectiveness of equine allogeneic tenogenic primed mesenchymal stem cells in the treatment of tendon injury (Depuydt et al., 2021), and compared the changes of imaging, histology, and biochemical and biomechanical parameters (Johnson et al., 2021). Nelson et al. compared the intra- and postoperative clinical features of desmotomy of the accessory ligament of the superficial digital flexor tendon using a Saber radiofrequency electrosurgical probe versus sharp transection with a tenotomy knife (Nelson et al., 2015). With in-depth research on equine tendinopathy in recent years, the application of flexor tendon modeling is more common, and further promotes the translation of research results from large animal models to clinical practice on humans.
Conclusion
The selection of preclinical models is essential to ensure that the efficacy and safety of studied treatments is successfully translated into clinical practice. The selection of animal models requires consideration of scientific criteria, economics, and ethical issues. Indeed, the costs associated with animal rearing and management significantly influence the selection of animal species because an adequate sample size is necessary to obtain reliable results. Therefore, the selection of animal models for proof-of-concept studies needs to balance the ethical justification, cost-effectiveness, and appropriateness of the model itself, such as anatomical location, size, surgical approach, and biomechanical properties. When considering the efficacy of a new therapy, for the validity of preclinical models, the identification of appropriate controls, optimal study duration and intermediate time points, and the provision of the most in-depth analysis must be considered (Bottagisio and Lovati, 2017).
Rodents are the most used animal model for the study of the genetic and molecular mechanisms of tendinopathy due to their minimal cost and ethical compliance. Rats are often used to explore the factors affecting tendon-bone healing (Degen et al., 2016; Arimura et al., 2017; Nakagawa et al., 2017; Yonemitsu et al., 2019), the molecular mechanisms underlying ectopic ossification in tendinopathy (Geng et al., 2020) and inflammation and scar formation in the injury tendon healing (Wang et al., 2019; Geng et al., 2020). The advantage of mouse model is the ability to examine the role of specific signal transduction pathways and molecules in tendon degeneration and repair by gene knockout (Kuenzler et al., 2017; Jensen et al., 2018). Despite their low cost and ease of rearing, the small size of rats and mice has an impact on surgical approaches, non-invasive imaging techniques, and biomechanical testing. Moreover, the relevant results still need to be applied to large animals and clinical trials to ensure safety and effectiveness.
Compared with small animals, large animals share many similar characteristics with humans in genetics, anatomy, physiology, pathology, and so on. Therefore, large animals are more suitable for macroscopic research on topics such as tendon biomechanics, scaffold repair technology, and surgical method optimization. The advantages and disadvantages of large animal models in tendinopathy research are summarized in Table 1. However, due to the prohibitive cost of rearing and managing large animals and the difficulty of ethical approval, typically only small sample studies can be conducted. Rabbits offer a satisfactory compromise. The size and biomechanical properties of rabbit tendons are similar to those of human flexor tendons (Goodman and Choueka, 2005; Nagasawa et al., 2008), allowing for surgical and in vivo imaging analysis. The docility of rabbits allows evaluation of postoperative rehabilitation effects by immobilization or active loading. Rabbit models can also be used to evaluate the role of autologous cell therapy and regenerative medicine (Chong et al., 2007). Sheep are readily available, moderately expensive, and are used to study chronic tendon injuries and various suture techniques to reduce postoperative adverse conditions. Dogs are used to explore the repair effect of tendon injury mediated by internal factors and postoperative rehabilitation schemes, owing to their high degree of cooperation. However, public opinion pressure against the animal testing dogs hinders the selection of this species. Some experiments have used specially bred dogs as models of tendinopathy. The horse is a natural tendon injury model due to its frequent sports. Horses can be used to study the mechanisms and repair methods of tendon injury. Porcine tendons and ligaments are the best animal sources for xenogeneic tendon transplantation.
The application of animal models has promoted the progress of tendinopathy research but, given the complexity of human tendinopathy, there are still significant differences between animal experimental models and clinical human tendon injuries. In addition, more validated animal models are needed, as no single model can answer all the questions (Warden, 2007). It is hoped that, with the further development of animal models of tendinopathy, new strategies for the prevention and treatment of human tendinopathy can be provided.
Author contributions
GZ was responsible for the writing of the manuscript, XZ for the editing of the graphs and charts, SH for the editing and recording of the tables, YJ and ZQ for the revision and final review of the manuscript. All authors were involved in the creation and were responsible for the content of the work.
Funding
This work was supported by the National Natural Science Foundation of China (Grant No. 81973712, 82003985). Jilin Province Science and Technology Development Project in China (Grant No. 20210204013YY, 20200504005YY). Jilin Province Science and Technology Development Plan Project (Grant No. 20200708081YY). Jilin Province Traditional Chinese Medicine Science and Technology Project (Grant No. 2022012). Jilin Provincial Development and Reform Commission Innovation Capacity Building Project (Grant No. 2021C035-5).
Conflict of interest
The authors declare that the research was conducted in the absence of any commercial or financial relationships that could be construed as a potential conflict of interest.
Publisher’s note
All claims expressed in this article are solely those of the authors and do not necessarily represent those of their affiliated organizations, or those of the publisher, the editors and the reviewers. Any product that may be evaluated in this article, or claim that may be made by its manufacturer, is not guaranteed or endorsed by the publisher.
References
Adams, J. E., and Habbu, R. (2015). Tendinopathies of the hand and wrist. J. Am. Acad. Orthop. Surg. 23 (12), 741–750. doi:10.5435/jaaos-d-14-00216
Adams, J. E., Zobitz, M. E., Reach, J. S., An, K. N., and Steinmann, S. P. (2006). Rotator cuff repair using an acellular dermal matrix graft: an in vivo study in a canine model. Arthroscopy 22 (7), 700–709. doi:10.1016/j.arthro.2006.03.016
Ahn, K. S., Lee, N. J., Kang, C. H., Lee, Y. H., and Jeon, H. J. (2017). Serial changes of tendon histomorphology and strain elastography after induced achilles tendinopathy in rabbits: An in vivo study. J. Ultrasound Med. 36 (4), 767–774. doi:10.7863/ultra.16.02059
Andarawis-Puri, N., Flatow, E. L., and Soslowsky, L. J. (2015). Tendon basic science: Development, repair, regeneration, and healing. J. Orthop. Res. 33 (6), 780–784. doi:10.1002/jor.22869
Anderl, W., Heuberer, P. R., Laky, B., Kriegleder, B., Reihsner, R., and Eberhardsteiner, J. (2012). Superiority of bridging techniques with medial fixation on initial strength. Knee Surg. Sports Traumatol. Arthrosc. 20 (12), 2559–2566. doi:10.1007/s00167-012-1922-9
Arimura, H., Shukunami, C., Tokunaga, T., Karasugi, T., Okamoto, N., Taniwaki, T., et al. (2017). TGF-β1 improves biomechanical strength by extracellular matrix accumulation without increasing the number of tenogenic lineage cells in a rat rotator cuff repair model. Am. J. Sports Med. 45 (10), 2394–2404. doi:10.1177/0363546517707940
Berglund, M. E., Hildebrand, K. A., Zhang, M., Hart, D. A., and Wiig, M. E. (2010). Neuropeptide, mast cell, and myofibroblast expression after rabbit deep flexor tendon repair. J. Hand Surg. Am. 35 (11), 1842–1849. doi:10.1016/j.jhsa.2010.06.031
Biasutti, S., Dart, A., Smith, M., Blaker, C., Clarke, E., Jeffcott, L., et al. (2017). Spatiotemporal variations in gene expression, histology and biomechanics in an ovine model of tendinopathy. PLoS One 12 (10), e0185282. doi:10.1371/journal.pone.0185282
Bisson, L. J., and Manohar, L. M. (2010). A biomechanical comparison of the pullout strength of No. 2 FiberWire suture and 2-mm FiberWire tape in bovine rotator cuff tendons. Arthroscopy 26 (11), 1463–1468. doi:10.1016/j.arthro.2010.04.075
Bisson, L. J., Manohar, L. M., Wilkins, R. D., Gurske-Deperio, J., and Ehrensberger, M. T. (2008). Influence of suture material on the biomechanical behavior of suture-tendon specimens: a controlled study in bovine rotator cuff. Am. J. Sports Med. 36 (5), 907–912. doi:10.1177/0363546508314793
Bottagisio, M., and Lovati, A. B. (2017). A review on animal models and treatments for the reconstruction of Achilles and flexor tendons. J. Mater. Sci. Mater. Med. 28 (3), 45. doi:10.1007/s10856-017-5858-y
Bruns, J., Kampen, J., Kahrs, J., and Plitz, W. (2000). Achilles tendon rupture: experimental results on spontaneous repair in a sheep-model. Knee Surg. Sports Traumatol. Arthrosc. 8 (6), 364–369. doi:10.1007/s001670000149
Camenzind, R. S., Wieser, K., Fessel, G., Meyer, D. C., and Snedeker, J. G. (2016). Tendon collagen crosslinking offers potential to improve suture pullout in rotator cuff repair: An ex vivo sheep study. Clin. Orthop. Relat. Res. 474 (8), 1778–1785. doi:10.1007/s11999-016-4838-8
Chen, Q., Lu, H., and Yang, H. (2015). Chitosan prevents adhesion during rabbit flexor tendon repair via the sirtuin 1 signaling pathway. Mol. Med. Rep. 12 (3), 4598–4603. doi:10.3892/mmr.2015.4007
Chong, A. K., Ang, A. D., Goh, J. C., Hui, J. H., Lim, A. Y., Lee, E. H., et al. (2007). Bone marrow-derived mesenchymal stem cells influence early tendon-healing in a rabbit achilles tendon model. J. Bone Jt. Surg. Am. 89 (1), 74–81. doi:10.2106/jbjs.E.01396
Coleman, S. H., Fealy, S., Ehteshami, J. R., MacGillivray, J. D., Altchek, D. W., Warren, R. F., et al. (2003). Chronic rotator cuff injury and repair model in sheep. J. Bone Jt. Surg. Am. 85 (12), 2391–2402. doi:10.2106/00004623-200312000-00018
de Cesar Netto, C., Godoy-Santos, A. L., Augusto Pontin, P., Natalino, R. J. M., Pereira, C. A. M., Lima, F. D. O., et al. (2018). Novel animal model for Achilles tendinopathy: Controlled experimental study of serial injections of collagenase in rabbits. PLoS One 13 (2), e0192769. doi:10.1371/journal.pone.0192769
de Lima Santos, A., Silva, C. G. D., de Sá Barretto, L. S., Franciozi, C., Tamaoki, M. J. S., de Almeida, F. G., et al. (2019). Biomechanical evaluation of tendon regeneration with adipose-derived stem cell. J. Orthop. Res. 37 (6), 1281–1286. doi:10.1002/jor.24182
de Mattos, L. H., Álvarez, L. E., Yamada, A. L., Hussni, C. A., Rodrigues, C. A., Watanabe, M. J., et al. (2015). Effect of phototherapy with light-emitting diodes (890 nm) on tendon repair: an experimental model in sheep. Lasers Med. Sci. 30 (1), 193–201. doi:10.1007/s10103-014-1641-1
de Moya, K., and Kim, S. (2020). Radiographic evaluation of patellar tendon length following corrective surgical procedures for medial patellar luxation in dogs. PLoS One 15 (9), e0238598. doi:10.1371/journal.pone.0238598
Dean, B. J. F., Dakin, S. G., Millar, N. L., and Carr, A. J. (2017). Review: Emerging concepts in the pathogenesis of tendinopathy. Surgeon 15 (6), 349–354. doi:10.1016/j.surge.2017.05.005
Degen, R. M., Carbone, A., Carballo, C., Zong, J., Chen, T., Lebaschi, A., et al. (2016). The effect of purified human bone marrow-derived mesenchymal stem cells on rotator cuff tendon healing in an athymic rat. Arthroscopy 32 (12), 2435–2443. doi:10.1016/j.arthro.2016.04.019
Depuydt, E., Broeckx, S. Y., Van Hecke, L., Chiers, K., Van Brantegem, L., van Schie, H., et al. (2021). The evaluation of equine allogeneic tenogenic primed mesenchymal stem cells in a surgically induced superficial digital flexor tendon lesion model. Front. Vet. Sci. 8, 641441. doi:10.3389/fvets.2021.641441
Derby, B. M., Reichensperger, J., Chambers, C., Bueno, R. A., Suchy, H., and Neumeister, M. W. (2012). Early growth response factor-1: expression in a rabbit flexor tendon scar model. Plast. Reconstr. Surg. 129 (3), 435e–442e. doi:10.1097/PRS.0b013e3182402d81
Derwin, K. A., Baker, A. R., Codsi, M. J., and Iannotti, J. P. (2007). Assessment of the canine model of rotator cuff injury and repair. J. Shoulder Elb. Surg. 16, S140–S148. doi:10.1016/j.jse.2007.04.002
Derwin, K. A., Codsi, M. A., Baker, A. R., and McCarron, J. A. (2009). Rotator cuff repair augmentation in a canine model with use of a woven poly-L-lactide device. J. Bone Joint Surg. Am. 91 (5), 1159–1171. doi:10.2106/jbjs.H.00775
Doğramaci, Y., Kalaci, A., Sevinç, T. T., Süner, G., Emir, A., and Yanat, A. N. (2009). Single side locking on the opposite of the modified kessler tendon repair prevents gap formation and suture pull-out: a biomechanical evaluation in sheep tendons. Eklem Hast. Cerrahisi 20 (2), 102–106.
Doherty, G. P., Koike, Y., Uhthoff, H. K., Lecompte, M., and Trudel, G. (2006). Comparative anatomy of rabbit and human achilles tendons with magnetic resonance and ultrasound imaging. Comp. Med. 56 (1), 68–74.
Dündar, N., Güneri, B., Uzel, M., and Doğaner, A. (2020). Biomechanical comparison of Bunnell, modified Kessler, and Tsuge tendon repair techniques using two suture types. Acta Orthop. Traumatol. Turc. 54 (1), 104–113. doi:10.5152/j.aott.2020.01.411
Durgam, S. S., Stewart, A. A., Sivaguru, M., Wagoner Johnson, A. J., and Stewart, M. C. (2016). Tendon-derived progenitor cells improve healing of collagenase-induced flexor tendinitis. J. Orthop. Res. 34 (12), 2162–2171. doi:10.1002/jor.23251
Enea, D., Gwynne, J., Kew, S., Arumugam, M., Shepherd, J., Brooks, R., et al. (2013). Collagen fibre implant for tendon and ligament biological augmentation. in vivo study in an ovine model. Knee Surg. Sports Traumatol. Arthrosc. 21 (8), 1783–1793. doi:10.1007/s00167-012-2102-7
Facon-Poroszewska, M., Kiełbowicz, Z., and Prządka, P. (2019). Influence of radial pressure wave therapy (RPWT) on collagenase-induced achilles tendinopathy treated with platelet rich plasma and autologous adipose derived stem cells. Pol. J. Vet. Sci. 22 (4), 743–751. doi:10.24425/pjvs.2019.131405
Fernández-Sarmiento, J. A., Domínguez, J. M., Granados, M. M., Morgaz, J., Navarrete, R., Carrillo, J. M., et al. (2013). Histological study of the influence of plasma rich in growth factors (PRGF) on the healing of divided Achilles tendons in sheep. J. Bone Jt. Surg. Am. 95 (3), 246–255. doi:10.2106/jbjs.K.01659
Flanigan, D. C., Bloomfield, M., and Koh, J. (2011). A biomechanical comparison of patellar tendon repair materials in a bovine model. Orthopedics 34 (8), e344–e348. doi:10.3928/01477447-20110627-13
Fransson, B. A., Gavin, P. R., and Lahmers, K. K. (2005). Supraspinatus tendinosis associated with biceps brachii tendon displacement in a dog. J. Am. Vet. Med. Assoc. 227 (9), 1429–1433. doi:10.2460/javma.2005.227.1429
Gaut, L., and Duprez, D. (2016). Tendon development and diseases. Wiley Interdiscip. Rev. Dev. Biol. 5 (1), 5–23. doi:10.1002/wdev.201
Geng, Y., Zhao, X., Xu, J., Zhang, X., Hu, G., Fu, S. C., et al. (2020). Overexpression of mechanical sensitive miR-337-3p alleviates ectopic ossification in rat tendinopathy model via targeting IRS1 and Nox4 of tendon-derived stem cells. J. Mol. Cell Biol. 12 (4), 305–317. doi:10.1093/jmcb/mjz030
Gerber, C., Meyer, D. C., Schneeberger, A. G., Hoppeler, H., and von Rechenberg, B. (2004). Effect of tendon release and delayed repair on the structure of the muscles of the rotator cuff: an experimental study in sheep. J. Bone Jt. Surg. Am. 86 (9), 1973–1982. doi:10.2106/00004623-200409000-00016
Gerber, C., Meyer, D. C., Flück, M., Valdivieso, P., von Rechenberg, B., Benn, M. C., et al. (2017). Muscle degeneration associated with rotator cuff tendon release and/or denervation in sheep. Am. J. Sports Med. 45 (3), 651–658. doi:10.1177/0363546516677254
Gersoff, W. K., Bozynski, C. C., Cook, C. R., Pfeiffer, F. M., Kuroki, K., and Cook, J. L. (2019). Evaluation of a novel degradable synthetic biomaterial patch for augmentation of tendon healing in a large animal model. J. Knee Surg. 32 (5), 434–440. doi:10.1055/s-0038-1646930
Ghelfi, J., Bacle, M., Stephanov, O., de Forges, H., Soulairol, I., Roger, P., et al. (2021). Collagenase-induced patellar tendinopathy with neovascularization: First results towards a piglet model of musculoskeletal embolization. Biomedicines 10 (1), 2. doi:10.3390/biomedicines10010002
Goodman, H. J., and Choueka, J. (2005). Biomechanics of the flexor tendons. Hand Clin. 21 (2), 129–149. doi:10.1016/j.hcl.2004.11.002
Grumet, R. C., Hadley, S., Diltz, M. V., Lee, T. Q., and Gupta, R. (2009). Development of a new model for rotator cuff pathology: the rabbit subscapularis muscle. Acta Orthop. 80 (1), 97–103. doi:10.1080/17453670902807425
Guo, Q., Li, C., Qi, W., Li, H., Lu, X., Shen, X., et al. (2016). A novel suture anchor constructed of cortical bone for rotator cuff repair: a biomechanical study on sheep humerus specimens. Int. Orthop. 40 (9), 1913–1918. doi:10.1007/s00264-016-3185-4
Gurger, M., Once, G., Yilmaz, E., Demir, S., Calik, I., Say, Y., et al. (2021). The effect of the platelet-rich plasma and ozone therapy on tendon-to-bone healing in the rabbit rotator cuff repair model. J. Orthop. Surg. Res. 16 (1), 202. doi:10.1186/s13018-021-02320-w
Habets, B., van den Broek, A. G., Huisstede, B. M. A., Backx, F. J. G., and van Cingel, R. E. H. (2018). Return to sport in athletes with midportion achilles tendinopathy: A qualitative systematic review regarding definitions and criteria. Sports Med. 48 (3), 705–723. doi:10.1007/s40279-017-0833-9
Håkansson, J., Mahlapuu, M., Ekström, L., Olmarker, K., and Wiig, M. (2012). Effect of lactoferrin peptide (PXL01) on rabbit digit mobility after flexor tendon repair. J. Hand Surg. Am. 37 (12), 2519–2525. doi:10.1016/j.jhsa.2012.09.019
Han, F., Li, T., Li, M., Zhang, B., Wang, Y., Zhu, Y., et al. (2023). Nano-calcium silicate mineralized fish scale scaffolds for enhancing tendon-bone healing. Bioact. Mater. 20, 29–40. doi:10.1016/j.bioactmat.2022.04.030
Hast, M. W., Zuskov, A., and Soslowsky, L. J. (2014). The role of animal models in tendon research. Bone Jt. Res. 3 (6), 193–202. doi:10.1302/2046-3758.36.2000281
He, M., Gan, A. W., Lim, A. Y., Goh, J. C., Hui, J. H., Lee, E. H., et al. (2013). The effect of fibrin glue on tendon healing and adhesion formation in a rabbit model of flexor tendon injury and repair. J. Plast. Surg. Hand Surg. 47 (6), 509–512. doi:10.3109/2000656x.2013.789037
He, M., Gan, A. W., Lim, A. Y., Goh, J. C., Hui, J. H., and Chong, A. K. (2015). Bone marrow derived mesenchymal stem cell augmentation of rabbit flexor tendon healing. Hand Surg. 20 (3), 421–429. doi:10.1142/s0218810415500343
Henn, R. F., Kuo, C. E., Kessler, M. W., Razzano, P., Grande, D. P., and Wolfe, S. W. (2010). Augmentation of zone II flexor tendon repair using growth differentiation factor 5 in a rabbit model. J. Hand Surg. Am. 35 (11), 1825–1832. doi:10.1016/j.jhsa.2010.08.031
Hyman, S. A., Wu, I. T., Vasquez-Bolanos, L. S., Norman, M. B., Esparza, M. C., Bremner, S. N., et al. (2021). Supraspinatus muscle architecture and physiology in a rabbit model of tenotomy and repair. J. Appl. Physiol. 131 (6), 1708–1717. doi:10.1152/japplphysiol.01119.2020
Iacopetti, I., Perazzi, A., Maniero, V., Martinello, T., Patruno, M., Glazar, M., et al. (2015). Effect of MLS(®) laser therapy with different dose regimes for the treatment of experimentally induced tendinopathy in sheep: pilot study. Photomed. Laser Surg. 33 (3), 154–163. doi:10.1089/pho.2014.3775
Jensen, A. R., Kelley, B. V., Mosich, G. M., Ariniello, A., Eliasberg, C. D., Vu, B., et al. (2018). Neer Award 2018: Platelet-derived growth factor receptor α co-expression typifies a subset of platelet-derived growth factor receptor β-positive progenitor cells that contribute to fatty degeneration and fibrosis of the murine rotator cuff. J. Shoulder Elb. Surg. 27 (7), 1149–1161. doi:10.1016/j.jse.2018.02.040
Ji, X., Bao, N., An, K. N., Amadio, P.C., Steinmann, S. P., and Zhao, C. (2015). A canine non-weight-bearing model with radial neurectomy for rotator cuff repair. PLoS One 10 (6), e0130576. doi:10.1371/journal.pone.0130576
Jielile, J., Asilehan, B., Wupuer, A., Qianman, B., Jialihasi, A., Tangkejie, W., et al. (2016). Early ankle mobilization promotes healing in a rabbit model of achilles tendon rupture. Orthopedics 39 (1), e117–e126. doi:10.3928/01477447-20160106-01
Johnson, S. A., Valdés-Martínez, A., Turk, P. J., Wayne McIlwraith, C., Barrett, M. F., McGilvray, K. C., et al. (2021). Longitudinal tendon healing assessed with multi-modality advanced imaging and tissue analysis. Equine Vet. J. 54, 766–781. doi:10.1111/evj.13478
Kayser, F., Hontoir, F., Clegg, P., Kirschvink, N., Dugdale, A., and Vandeweerd, J. M. (2019). Ultrasound anatomy of the normal stifle in the sheep. Anat. Histol. Embryol. 48 (1), 87–96. doi:10.1111/ahe.12414
Kayser, F., Bori, E., Fourny, S., Hontoir, F., Clegg, P., Dugdale, A., et al. (2022). Ex vivo study correlating the stiffness of the ovine patellar tendon to age and weight. Int. Biomech. 9 (1), 1–9. doi:10.1080/23335432.2022.2108899
Khan, M. R., Smith, R. K., David, F., Lam, R., Hughes, G., De Godoy, R., et al. (2020). Evaluation of the effects of synovial multipotent cells on deep digital flexor tendon repair in a large animal model of intra-synovial tendinopathy. J. Orthop. Res. 38 (1), 128–138. doi:10.1002/jor.24423
Kim, D. K., Ahn, J., Kim, S. A., Go, E. J., Lee, D. H., Park, S. C., et al. (2021). Improved healing of rabbit patellar tendon defects after an atelocollagen injection. Am. J. Sports Med. 49 (11), 2924–2932. doi:10.1177/03635465211030508
Komi, P. V. (1990). Relevance of in vivo force measurements to human biomechanics. J. Biomech. 23, 23–34. doi:10.1016/0021-9290(90)90038-5
Kuenzler, M. B., Nuss, K., Karol, A., Schär, M. O., Hottiger, M., Raniga, S., et al. (2017). Neer award 2016: reduced muscle degeneration and decreased fatty infiltration after rotator cuff tear in a poly(ADP-ribose) polymerase 1 (PARP-1) knock-out mouse model. J. Shoulder Elb. Surg. 26 (5), 733–744. doi:10.1016/j.jse.2016.11.009
Kwon, J., Kim, Y. H., Rhee, S. M., Kim, T. I., Lee, J., Jeon, S., et al. (2018). Effects of allogenic dermal fibroblasts on rotator cuff healing in a rabbit model of chronic tear. Am. J. Sports Med. 46 (8), 1901–1908. doi:10.1177/0363546518770428
Lebaschi, A., Deng, X. H., Zong, J., Cong, G. T., Carballo, C. B., Album, Z. M., et al. (2016). Animal models for rotator cuff repair. Ann. N. Y. Acad. Sci. 1383 (1), 43–57. doi:10.1111/nyas.13203
Lebaschi, A. H., Deng, X. H., Camp, C. L., Zong, J., Cong, G. T., Carballo, C. B., et al. (2018). Biomechanical, histologic, and molecular evaluation of tendon healing in a new murine model of rotator cuff repair. Arthroscopy 34 (4), 1173–1183. doi:10.1016/j.arthro.2017.10.045
Lee, J. H., Kim, Y. H., Rhee, S. M., Han, J., Jeong, H. J., Park, J. H., et al. (2021). Rotator cuff tendon healing using human dermal fibroblasts: Histological and biomechanical analyses in a rabbit model of chronic rotator cuff tears. Am. J. Sports Med. 49 (13), 3669–3679. doi:10.1177/03635465211041102
Leung, K. S., Chong, W. S., Chow, D. H., Zhang, P., Cheung, W. H., Wong, M. W., et al. (2015). A comparative study on the biomechanical and histological properties of bone-to-bone, bone-to-tendon, and tendon-to-tendon healing: An achilles tendon-calcaneus model in goats. Am. J. Sports Med. 43 (6), 1413–1421. doi:10.1177/0363546515576904
Li, S., Ma, K., Li, H., Jiang, J., and Chen, S. (2016). The effect of sodium hyaluronate on ligamentation and biomechanical property of tendon in repair of achilles tendon defect with polyethylene terephthalate artificial ligament: A rabbit tendon repair model. Biomed. Res. Int. 2016, 8684231. doi:10.1155/2016/8684231
Liao, J. C. Y., He, M., Gan, A. W. T., and Chong, A. K. S. (2017). The effects of autologous platelet-rich fibrin on flexor tendon healing in a rabbit model. J. Hand Surg. Am. 42 (11), 928.e921–928. doi:10.1016/j.jhsa.2017.06.098
Liao, J. C. Y., He, M., Gan, A. W. T., Wen, F., Tan, L. P., and Chong, A. K. S. (2018). The effects of bi-functional anti-adhesion scaffolds on flexor tendon healing in a rabbit model. J. Biomed. Mater. Res. B Appl. Biomater. 106 (7), 2605–2614. doi:10.1002/jbm.b.34077
Linder, L. H., Sukin, D. L., Burks, R. T., and Haut, R. C. (1994). Biomechanical and histological properties of the canine patellar tendon after removal of its medial third. Am. J. Sports Med. 22 (1), 136–142. doi:10.1177/036354659402200121
Liu, G. M., Pan, J., Zhang, Y., Ning, L. J., Luo, J. C., Huang, F. G., et al. (2018). Bridging repair of large rotator cuff tears using a multilayer decellularized tendon slices graft in a rabbit model. Arthroscopy 34 (9), 2569–2578. doi:10.1016/j.arthro.2018.04.019
Liu, P., Zhu, W., and Liu, Q. (2021). Animal models for study on rotator cuff injury. Zhong Nan Da Xue Xue Bao Yi Xue Ban 46 (4), 426–431. doi:10.11817/j.issn.1672-7347.2021.200064
Liu, Q., Yu, Y., Reisdorf, R. L., Qi, J., Lu, C. K., Berglund, L. J., et al. (2019). Engineered tendon-fibrocartilage-bone composite and bone marrow-derived mesenchymal stem cell sheet augmentation promotes rotator cuff healing in a non-weight-bearing canine model. Biomaterials 192, 189–198. doi:10.1016/j.biomaterials.2018.10.037
Liu, H., Gao, F., Liang, X., Chen, X., Qu, Y., and Wang, L. (2020). Pathogenesis and development of patellar tendon fibrosis in a rabbit overuse model. Am. J. Sports Med. 48 (5), 1141–1150. doi:10.1177/0363546520902447
Lohan, A., Kohl, B., Meier, C., and Schulze-Tanzil, G. (2018). Tenogenesis of decellularized porcine achilles tendon matrix reseeded with human tenocytes in the nude mice xenograft model. Int. J. Mol. Sci. 19 (7), E2059. doi:10.3390/ijms19072059
Loiselle, A. E., Kelly, M., and Hammert, W. C. (2016). Biological augmentation of flexor tendon repair: A challenging cellular landscape. J. Hand Surg. Am. 41 (1), 144–149. quiz 149. doi:10.1016/j.jhsa.2015.07.002
Longo, U. G., Forriol, F., Campi, S., Maffulli, N., and Denaro, V. (2011). Animal models for translational research on shoulder pathologies: from bench to bedside. Sports Med. Arthrosc. Rev. 19 (3), 184–193. doi:10.1097/JSA.0b013e318205470e
Longo, U. G., Ronga, M., and Maffulli, N. (2018). Achilles tendinopathy. Sports Med. Arthrosc. Rev. 26 (1), 16–30. doi:10.1097/jsa.0000000000000185
Luan, T., Liu, X., Easley, J. T., Ravishankar, B., Puttlitz, C., and Feeley, B. T. (2015). Muscle atrophy and fatty infiltration after an acute rotator cuff repair in a sheep model. Muscle Ligaments Tendons J. 5 (2), 106–112. doi:10.32098/mltj.02.2015.09
Lui, P. P., Maffulli, N., Rolf, C., and Smith, R. K. (2011). What are the validated animal models for tendinopathy? Scand. J. Med. Sci. Sports 21 (1), 3–17. doi:10.1111/j.1600-0838.2010.01164.x
Lyras, D. N., Kazakos, K., Tilkeridis, K., Kokka, A., Ververidis, A., Botaitis, S., et al. (2016). Temporal and spatial expression of TGF-b1 in the early phase of patellar tendon healing after application of platelet rich plasma. Arch. Bone Jt. Surg. 4 (2), 156–160.
Mahar, A., Tamborlane, J., Oka, R., Esch, J., and Pedowitz, R. A. (2007). Single-row suture anchor repair of the rotator cuff is biomechanically equivalent to double-row repair in a bovine model. Arthroscopy 23 (12), 1265–1270. doi:10.1016/j.arthro.2007.07.010
Martinello, T., Bronzini, I., Perazzi, A., Testoni, S., De Benedictis, G. M., Negro, A., et al. (2013). Effects of in vivo applications of peripheral blood-derived mesenchymal stromal cells (PB-MSCs) and platlet-rich plasma (PRP) on experimentally injured deep digital flexor tendons of sheep. J. Orthop. Res. 31 (2), 306–314. doi:10.1002/jor.22205
Meier Bürgisser, G., Calcagni, M., Bachmann, E., Fessel, G., Snedeker, J. G., Giovanoli, P., et al. (2016). Rabbit Achilles tendon full transection model - wound healing, adhesion formation and biomechanics at 3, 6 and 12 weeks post-surgery. Biol. Open 5 (9), 1324–1333. doi:10.1242/bio.020644
Meimandi-Parizi, A., Oryan, A., and Moshiri, A. (2013). Tendon tissue engineering and its role on healing of the experimentally induced large tendon defect model in rabbits: a comprehensive in vivo study. PLoS One 8 (9), e73016. doi:10.1371/journal.pone.0073016
Millar, N. L., Silbernagel, K. G., Thorborg, K., Kirwan, P. D., Galatz, L. M., Abrams, G. D., et al. (2021). Tendinopathy. Nat. Rev. Dis. Prim. 7 (1), 1. doi:10.1038/s41572-020-00234-1
Nagasawa, K., Noguchi, M., Ikoma, K., and Kubo, T. (2008). Static and dynamic biomechanical properties of the regenerating rabbit Achilles tendon. Clin. Biomech. 23 (6), 832–838. doi:10.1016/j.clinbiomech.2008.02.002
Nakagawa, H., Morihara, T., Fujiwara, H., Kabuto, Y., Sukenari, T., Kida, Y., et al. (2017). Effect of footprint preparation on tendon-to-bone healing: A histologic and biomechanical study in a rat rotator cuff repair model. Arthroscopy 33 (8), 1482–1492. doi:10.1016/j.arthro.2017.03.031
Nelson, B. B., Kawcak, C. E., Ehrhart, E. J., and Goodrich, L. R. (2015). Radiofrequency probe and sharp transection for tenoscopic-guided desmotomy of the accessory ligament of the superficial digital flexor tendon. Vet. Surg. 44 (6), 713–722. doi:10.1111/vsu.12328
Novakova, S. S., Mahalingam, V. D., Florida, S. E., Mendias, C. L., Allen, A., Arruda, E. M., et al. (2018). Tissue-engineered tendon constructs for rotator cuff repair in sheep. J. Orthop. Res. 36 (1), 289–299. doi:10.1002/jor.23642
Park, M. C., Cadet, E. R., Levine, W. N., Bigliani, L. U., and Ahmad, C.S. (2005). Tendon-to-bone pressure distributions at a repaired rotator cuff footprint using transosseous suture and suture anchor fixation techniques. Am. J. Sports Med. 33 (8), 1154–1159. doi:10.1177/0363546504273053
Patterson-Kane, J. C., Becker, D. L., and Rich, T. (2012). The pathogenesis of tendon microdamage in athletes: the horse as a natural model for basic cellular research. J. Comp. Pathol. 147 (2-3), 227–247. doi:10.1016/j.jcpa.2012.05.010
Peeters, I., Pien, N., Mignon, A., Van Damme, L., Dubruel, P., Van Vlierberghe, S., et al. (2022). Flexor tendon repair using a reinforced tubular, medicated electrospun construct. J. Orthop. Res. 40 (3), 750–760. doi:10.1002/jor.25103
Plate, J. F, Bates, C. M., Mannava, S., Smith, T. L., Jorgensen, M. J., Register, T. C., et al. (2013). Age-related degenerative functional, radiographic, and histological changes of the shoulder in nonhuman primates. J. Shoulder Elbow Surg. 22 (8), 1019–1029. doi:10.1016/j.jse.2012.11.004
Pownder, S. L., Hayashi, K., Lin, B. Q., Meyers, K. N., Caserto, B. G., Breighner, R. E., et al. (2021). Differences in the magnetic resonance imaging parameter T2* may be identified during the course of canine patellar tendon healing: a pilot study. Quant. Imaging Med. Surg. 11 (4), 1234–1246. doi:10.21037/qims-20-684
Ribitsch, I., Gueltekin, S., Keith, M. F., Minichmair, K., Peham, C., Jenner, F., et al. (2020). Age-related changes of tendon fibril micro-morphology and gene expression. J. Anat. 236 (4), 688–700. doi:10.1111/joa.13125
Ristaniemi, A., Stenroth, L., Mikkonen, S., and Korhonen, R. K. (2018). Comparison of elastic, viscoelastic and failure tensile material properties of knee ligaments and patellar tendon. J. Biomech. 79, 31–38. doi:10.1016/j.jbiomech.2018.07.031
Ristaniemi, A., Regmi, D., Mondal, D., Torniainen, J., Tanska, P., Stenroth, L., et al. (2021). Structure, composition and fibril-reinforced poroviscoelastic properties of bovine knee ligaments and patellar tendon. J. R. Soc. Interface 18 (174), 20200737. doi:10.1098/rsif.2020.0737
Roller, B. L., Kuroki, K., Bozynski, C. C., Pfeiffer, F. M., and Cook, J. L. (2018). Use of a novel magnesium-based resorbable bone cement for augmenting anchor and tendon fixation. Am. J. Orthop. 47 (2). doi:10.12788/ajo.2018.0010
Romeo, A., Easley, J., Regan, D., Hackett, E., Johnson, J., Johnson, J., et al. (2022). Rotator cuff repair using a bioresorbable nanofiber interposition scaffold: a biomechanical and histologic analysis in sheep. J. Shoulder Elbow Surg. 31 (2), 402–412. doi:10.1016/j.jse.2021.07.018
Roßbach, B. P., Gülecyüz, M. F., Kempfert, L., Pietschmann, M. F., Ullamann, T., Ficklscherer, A., et al. (2020). Rotator cuff repair with autologous tenocytes and biodegradable collagen scaffold: A histological and biomechanical study in sheep. Am. J. Sports Med. 48 (2), 450–459. doi:10.1177/0363546519892580
Ruoss, S., Kindt, P., Oberholzer, L., Rohner, M., Jungck, L., Abdel-Aziz, S., et al. (2018a). Inhibition of calpain delays early muscle atrophy after rotator cuff tendon release in sheep. Physiol. Rep. 6 (21), e13833. doi:10.14814/phy2.13833
Ruoss, S., Möhl, C. B., Benn, M. C., von Rechenberg, B., Wieser, K., Meyer, D. C., et al. (2018b). Costamere protein expression and tissue composition of rotator cuff muscle after tendon release in sheep. J. Orthop. Res. 36 (1), 272–281. doi:10.1002/jor.23624
Safran, O., Derwin, K. A., Powell, K., and Iannotti, J. P. (2005). Changes in rotator cuff muscle volume, fat content, and passive mechanics after chronic detachment in a canine model. J. Bone Jt. Surg. Am. 87 (12), 2662–2670. doi:10.2106/jbjs.D.02421
Sarıkaya, B., Yumuşak, N., Yigin, A., Sipahioğlu, S., Yavuz, Ü., and Altay, M. A. (2017). Comparison of the effects of human recombinant epidermal growth factor and platelet-rich plasma on healing of rabbit patellar tendon. Eklem Hast. Cerrahisi 28 (2), 92–99. doi:10.5606/ehc.2017.55396
Sarrafian, T. L., Wang, H., Hackett, E. S., Yao, J. Q., Shih, M. S., Ramsay, H. L., et al. (2010). Comparison of Achilles tendon repair techniques in a sheep model using a cross-linked acellular porcine dermal patch and platelet-rich plasma fibrin matrix for augmentation. J. Foot Ankle Surg. 49 (2), 128–134. doi:10.1053/j.jfas.2009.12.005
Sener, M., Altay, M. A., Baki, C., Turhan, A. U., and Cobanoglu, U. (2004). The comparison of patellar tendon-bone autografting and free flexor-tendon autografting in infraspinatus defect of the shoulder: biomechanical and histological evaluation in a sheep model. Knee Surg. Sports Traumatol. Arthrosc. 12 (3), 235–240. doi:10.1007/s00167-003-0473-5
Serrani, D., Volta, A., Cingolani, F., Pennasilico, L., Di Bella, C., Bonazzi, M., et al. (2021). Serial ultrasonographic and real-time elastosonographic assessment of the ovine common calcaneal tendon, after an experimentally induced tendinopathy. Vet. Sci. 8 (4), 54. doi:10.3390/vetsci8040054
Skalec, A., Janeczek, M., and Czerski, A. (2019). Anatomy and histology of the rabbit common calcanean tendon. Anat. Histol. Embryol. 48 (5), 466–475. doi:10.1111/ahe.12468
Smith, M. J., Pfeiffer, F. M., Cook, C. R., Kuroki, K., and Cook, J. L. (2018). Rotator cuff healing using demineralized cancellous bone matrix sponge interposition compared to standard repair in a preclinical canine model. J. Orthop. Res. 36 (3), 906–912. doi:10.1002/jor.23680
Smith, M. J., Bozynski, C. C., Kuroki, K., Cook, C. R., Stoker, A. M., and Cook, J. L. (2020). Comparison of biologic scaffolds for augmentation of partial rotator cuff tears in a canine model. J. Shoulder Elb. Surg. 29 (8), 1573–1583. doi:10.1016/j.jse.2019.11.028
Sonnabend, D. H., Howlett, C. R., and Young, A. A. (2010). Histological evaluation of repair of the rotator cuff in a primate model. J. Bone Jt. Surg. Br. 92 (4), 586–594. doi:10.1302/0301-620x.92b4.22371
Su, W., Li, X., Zhao, S., Shen, P., Dong, S., Jiang, J., et al. (2018). Native enthesis preservation versus removal in rotator cuff repair in a rabbit model. Arthroscopy 34 (7), 2054–2062. doi:10.1016/j.arthro.2018.03.005
Suh, D. S., Lee, J. K., Yoo, J. C., Woo, S. H., Kim, G. R., Kim, J. W., et al. (2017). Atelocollagen enhances the healing of rotator cuff tendon in rabbit model. Am. J. Sports Med. 45 (9), 2019–2027. doi:10.1177/0363546517703336
Sun, Y., Kwak, J. M., Kholinne, E., Zhou, Y., Tan, J., Koh, K. H., et al. (2020). Small subchondral drill holes improve marrow stimulation of rotator cuff repair in a rabbit model of chronic rotator cuff tear. Am. J. Sports Med. 48 (3), 706–714. doi:10.1177/0363546519896350
Takase, F., Inui, A., Mifune, Y., Sakata, R., Muto, T., Harada, Y., et al. (2017). Effect of platelet-rich plasma on degeneration change of rotator cuff muscles: In vitro and in vivo evaluations. J. Orthop. Res. 35 (8), 1806–1815. doi:10.1002/jor.23451
Thangarajah, T., Shahbazi, S., Pendegrass, C. J., Lambert, S., Alexander, S., and Blunn, G. W. (2016). Tendon reattachment to bone in an ovine tendon defect model of retraction using allogenic and xenogenic demineralised bone matrix incorporated with mesenchymal stem cells. PLoS One 11 (9), e0161473. doi:10.1371/journal.pone.0161473
Tian, J., Rui, R., Xu, Y., Yang, W., Chen, X., Zhang, X., et al. (2020a). Achilles tendon rupture repair: Biomechanical comparison of the locking block modified Krackow technique and the Giftbox technique. Injury 51 (2), 559–564. doi:10.1016/j.injury.2019.10.019
Tian, J., Rui, Y., Xu, Y., Yang, W., Xu, T., Chen, X., et al. (2020b). A biomechanical comparison of Achilles tendon suture repair techniques: Locking Block Modified Krackow, Kessler, and Percutaneous Achilles Repair System with the early rehabilitation program in vitro bovine model. Arch. Orthop. Trauma Surg. 140 (11), 1775–1782. doi:10.1007/s00402-020-03535-y
Uslu, M., Isik, C., Ozsahin, M., Ozkan, A., Yasar, M., Orhan, Z., et al. (2014). Flexor tendons repair: effect of core sutures caliber with increased number of suture strands and peripheral sutures. A sheep model. Orthop. Traumatol. Surg. Res. 100 (6), 611–616. doi:10.1016/j.otsr.2014.05.009
Vargas-Vila, M. A., Gibbons, M. C., Wu, I. T., Esparza, M. C., Kato, K., Johnson, S. D., et al. (2022). Progression of muscle loss and fat accumulation in a rabbit model of rotator cuff tear. J. Orthop. Res. 40 (5), 1016–1025. doi:10.1002/jor.25160
Virchenko, O., Fahlgren, A., Rundgren, M., and Aspenberg, P. (2008). Early Achilles tendon healing in sheep. Arch. Orthop. Trauma Surg. 128 (9), 1001–1006. doi:10.1007/s00402-008-0691-x
Wang, C., Hu, Q., Song, W., Yu, W., and He, Y. (2020). Adipose stem cell-derived exosomes decrease fatty infiltration and enhance rotator cuff healing in a rabbit model of chronic tears. Am. J. Sports Med. 48 (6), 1456–1464. doi:10.1177/0363546520908847
Wang, L., Xiong, K., Wang, B., Liang, X., Li, H., Liu, H., et al. (2017). Effects of time to start training after acute patellar tendon enthesis injuries on healing of the injury in a rabbit model. Am. J. Sports Med. 45 (10), 2405–2410. doi:10.1177/0363546517712223
Wang, Y., He, G., Tang, H., Shi, Y., Kang, X., Lyu, J., et al. (2019). Aspirin inhibits inflammation and scar formation in the injury tendon healing through regulating JNK/STAT-3 signalling pathway. Cell Prolif. 52 (4), e12650. doi:10.1111/cpr.12650
Warden, S. J. (2007). Animal models for the study of tendinopathy. Br. J. Sports Med. 41 (4), 232–240. doi:10.1136/bjsm.2006.032342
Watts, A. E., Yeager, A. E., Kopyov, O. V., and Nixon, A. J. (2011). Fetal derived embryonic-like stem cells improve healing in a large animal flexor tendonitis model. Stem Cell Res. Ther. 2 (1), 4. doi:10.1186/scrt45
Wieser, K., Grubhofer, F., Hasler, A., Götschi, T., Beeler, S., Meyer, D., et al. (2021). Muscle Degeneration Induced by Sequential Release and Denervation of the Rotator Cuff Tendon in Sheep. J. Sports Med. 9 (8), 23259671211025302. doi:10.1177/23259671211025302
Xu, H., Sandor, M., Qi, S., Lombardi, J., Connor, J., McQuillan, D. J., et al. (2012). Implantation of a porcine acellular dermal graft in a primate model of rotator cuff repair. J. Shoulder Elb. Surg. 21 (5), 580–588. doi:10.1016/j.jse.2011.03.014
Xu, J., Li, Y., Zhang, X., Han, K., Ye, Z., Wu, C., et al. (2022). The Biomechanical and Histological Processes of Rerouting Biceps to Treat Chronic Irreparable Rotator Cuff Tears in a Rabbit Model. Am. J. Sports Med . 50 (2), 347–361. doi:10.1177/03635465211062914
Xu, D., Zhang, T., Qu, J., Hu, J., and Lu, H. (2014). Enhanced patella-patellar tendon healing using combined magnetic fields in a rabbit model. Am. J. Sports Med. 42 (10), 2495–2501. doi:10.1177/0363546514541539
Yamamoto, Y., Yamaguchi, S., Sasho, T., Fukawa, T., Akatsu, Y., Akagi, R., et al. (2017). Quantitative US elastography can Be used to quantify mechanical and histologic tendon healing in a rabbit model of achilles tendon transection. Radiology 283 (2), 408–417. doi:10.1148/radiol.2016160695
Yildiz, F., Bilsel, K., Pulatkan, A., Kapicioglu, M., Uzer, G., Çetindamar, T., et al. (2019). Comparison of two different superior capsule reconstruction methods in the treatment of chronic irreparable rotator cuff tears: a biomechanical and histologic study in rabbit models. J. Shoulder Elb. Surg. 28 (3), 530–538. doi:10.1016/j.jse.2018.08.022
Yılmaz, G., Doral, M. N., Turhan, E., Dönmez, G., Atay, A., and Kaya, D. (2014). Surgical treatment of achilles tendon ruptures: the comparison of open and percutaneous methods in a rabbit model. Ulus. Travma Acil Cerrahi Derg. 20 (5), 311–318. doi:10.5505/tjtes.2014.42716
Yonemitsu, R., Tokunaga, T., Shukunami, C., Ideo, K., Arimura, H., Karasugi, T., et al. (2019). Fibroblast growth factor 2 enhances tendon-to-bone healing in a rat rotator cuff repair of chronic tears. Am. J. Sports Med. 47 (7), 1701–1712. doi:10.1177/0363546519836959
Yoon, J. P., Lee, C. H., Jung, J. W., Lee, H. J., Lee, Y. S., Kim, J. Y., et al. (2018). Sustained delivery of transforming growth factor β1 by use of absorbable alginate scaffold enhances rotator cuff healing in a rabbit model. Am. J. Sports Med. 46 (6), 1441–1450. doi:10.1177/0363546518757759
Zhang, C. H., Jiang, Y. L., Ning, L. J., Li, Q., Fu, W. L., Zhang, Y. J., et al. (2018). Evaluation of decellularized bovine tendon sheets for achilles tendon defect reconstruction in a rabbit model. Am. J. Sports Med. 46 (11), 2687–2699. doi:10.1177/0363546518787515
Keywords: large animal model, tendinopathy, rotator cuff injury, patellar tendon injury, Achilles tendon injury, flexor tendon injury
Citation: Zhang G, Zhou X, Hu S, Jin Y and Qiu Z (2022) Large animal models for the study of tendinopathy. Front. Cell Dev. Biol. 10:1031638. doi: 10.3389/fcell.2022.1031638
Received: 30 August 2022; Accepted: 12 October 2022;
Published: 25 October 2022.
Edited by:
Yongye Huang, Northeastern University, ChinaCopyright © 2022 Zhang, Zhou, Hu, Jin and Qiu. This is an open-access article distributed under the terms of the Creative Commons Attribution License (CC BY). The use, distribution or reproduction in other forums is permitted, provided the original author(s) and the copyright owner(s) are credited and that the original publication in this journal is cited, in accordance with accepted academic practice. No use, distribution or reproduction is permitted which does not comply with these terms.
*Correspondence: Ye Jin, anlfY2N1Y21AMTYzLmNvbQ==; Zhidong Qiu, cXpkY2N6eUAxNjMuY29t