- Division of Human Reproduction and Developmental Genetics, Women’s Hospital, and Institute of Genetics, Zhejiang University School of Medicine, Hangzhou, China
Totipotency represents the highest developmental potency. By definition, totipotent stem cells are capable of giving rise to all embryonic and extraembryonic cell types. In mammalian embryos, totipotency occurs around the zygotic genome activation period, which is around the 2-cell stage in mouse embryo or the 4-to 8-cell stage in human embryo. Currently, with the development of in vitro totipotent-like models and the advances in small-scale genomic methods, an in-depth mechanistic understanding of the totipotency state and regulation was enabled. In this review, we explored and summarized the current views about totipotency from various angles, including genetic and epigenetic aspects. This will hopefully formulate a panoramic view of totipotency from the available research works until now. It can also help delineate the scaffold and formulate new hypotheses on totipotency for future research works.
1 Introduction
The very first demonstration of totipotent cells in the embryo was from the works of Tarkowski, in which a single blastomere isolated from a 2-cell (2C) stage embryo was proven to be able to generate a whole adult mouse (Tarkowski, 1959). By definition, totipotency refers to the developmental potential of cells capable of giving rise to the whole fertile organism (Redo and Brickman, 2020; Malik and Wang, 2022). Sometimes, functional assays, which revealed the capacity of stem cells being able to differentiate into all embryonic and extraembryonic lineages, are also recognized as a demonstration of totipotency. Apart from the definition in the perspective of developmental potentials, molecular criteria, including transcriptomic and epigenetic features, are also used to assess the totipotency state (Malik and Wang, 2022). In mouse embryos, totipotency emerges from the zygote to the 2-cell embryonic stage (Lu and Zhang, 2015). In addition, embryonic cells up to the 4-cell stage possess differentiation capacity toward all embryonic and extraembryonic lineages, thus also recognized as totipotent. Following these stages, the embryonic cells start to commit to fate segregation, first toward inner cell mass (ICM), which gives rise to fetal tissues, and trophectoderm (TE), which gives rise to extraembryonic placental tissues.
The transcription network regulating totipotency undergoes dynamic wiring from 1C to 4C stages and expands the zygotic genome activation (ZGA) period. Shortly after fertilization, maternal RNAs and proteins stored in the oocyte start to be degraded, a process known as maternal decay; at the same time, the zygotic genome begins to be transcribed, known as ZGA (Sha et al., 2019). ZGA usually occurs in two subsequent steps: a minor ZGA which predominantly takes place in the paternal pronucleus and a more substantial major ZGA which occurs in a 2-cell mouse embryo or 8-cell human embryo (Eckersley-Maslin et al., 2019). Minor ZGA is a prerequisite of major ZGA. Transient inhibition of minor ZGA with transcription inhibitors resulted in embryonic arrest at the 2-cell stage and major ZGA defect (Abe et al., 2018). How ZGA is triggered and how it contributes to the wiring of the totipotency transcription network and developmental potential are essential questions in the early embryogenesis field. So far, various biological pathways, such as transcription, cell cycle, nuclear/cytoplasmic ratio, or translation of key ZGA transcription factors (TFs), have been implicated in ZGA initiation and are also found to be important for totipotency establishment (Jukam et al., 2017).
Yet, comprehensive studies about totipotency and ZGA regulators have been limited due to the availability of cells in early embryos. However, identification and establishment of in vitro totipotent-like stem cell culture systems have significantly enabled the dissection of the totipotency regulation. Currently, different in vitro totipotency models share similarities but also bear substantial differences compared to in vivo totipotency states. Under serum/leukemia inhibitory factor (LIF) culture conditions, around 1%–2% of mouse embryonic stem cells (mESCs) were found to exhibit transcription features such as the 2C embryos and were known as 2C-like embryonic stem cells (2CLCs) (Macfarlan et al., 2012; Li and Izpisua Belmonte, 2018). 2CLCs possess the ability to contribute to both embryonic and extraembryonic tissues, although the functionality of differentiated cells remains to be evaluated (Macfarlan et al., 2012). The somatic cell nuclear transfer (SCNT) technique is also another way of reprogramming to access totipotent stem cells (Gurdon, 1962). In this context, 2CLCs have higher efficiencies of SCNT due to their similarity to 2C embryos (Ishiuchi et al., 2015). Since the identification of 2CLCs in 2012, a large number of the molecular mechanisms regulating the totipotency state have been revealed using this in vitro totipotency model. Recently, human 8-cell-like cells (8CLCs) were also discovered to exist in the heterogeneous human naïve embryonic stem cells, which transcriptionally resemble the 8-cell human embryos (Mazid et al., 2022; Taubenschmid-Stowers et al., 2022). In addition, small molecules targeting different signaling pathways have been used to generate different totipotent-like models in vitro. Expanded potential stem cells (EPSCs) were first established by Liu and Deng laboratories (Yang J. et al., 2017; Yang Y. et al., 2017), which can contribute to both embryo proper and trophectoderm lineages in chimera assays (Yang J. et al., 2017; Yang Y. et al., 2017). Transcriptionally, EPSCs derived correlated with E4.5 to E5.5 epiblast or EpiSCs (Posfai et al., 2021). Recently, using a splicing inhibitor pladienolide B (PlaB), the totipotent blastomere-like cell (TBLC) model was established (Shen et al., 2021; Taubenschmid-Stowers et al., 2022). TBLCs show epigenetic and transcriptomic profiles similar to those of 2C–4C embryos but distinct from those of 2CLCs. It has robust embryonic and extraembryonic differentiation potential. Similarly, totipotent-like stem cell (TLSC) models were established from mESCs using small-molecule induction, in which the transcriptional and epigenetic states were found to be closer to those of 2C embryos (Yang et al., 2022). However, both TBLCs and TLSCs differentiated poorly toward some of PrE- and TE-derived lineages (Shen et al., 2021; Yang et al., 2022), questioning the developmental potential of these in vivo totipotent cells. Recently, the Ding laboratory recently generated a culture condition to reprogram cells to chemically induce totipotent stem cells (ciTotiSCs), resembling mouse totipotent 2C embryo stage cells at transcriptional, epigenetic, and metabolic levels (Hu et al., 2022). Excitingly, the culture conditions in the Deng laboratory can derive totipotent potential stem (TPS) cells from 2C embryos. In vivo chimera formation assays show that these cells have embryonic and extraembryonic developmental potentials at the single-cell level and can induce blastocyst-like structures from TPS cells in vitro (Xu Y. et al., 2022). In general, all these in vitro totipotent-like models possess chimera formation, transcriptional, and epigenetic features similar to those of totipotent embryonic cells. However, more robust functional demonstration of their developmental totipotency remains to be assessed.
Here, we explored and summarized the current understanding on regulation of totipotent stem cells and totipotency in early embryos from various genetic and epigenetic perspectives, such as transcriptional regulation, epigenetic regulation, cell cycle, DNA damage, and RNA metabolism. From the current emerging evidence, the panoramic regulation of the totipotency state will surface more.
2 Transcriptional regulation of the totipotency state
Specific cell states are shaped by various pathways, and among all, gene expression plays essential roles. The emergence of totipotency is accompanied by the transcription network sustaining ZGA. Both cis- and trans-genomic elements and coding and non-coding genes are actively engaged in ZGA and the establishment of the totipotent state.
2.1 Transcription factors in regulation of the totipotency state
Transcription factors work by recognizing specific DNA sequences and recruiting RNA polymerase for specific gene transcription. Totipotent stem cells have their own unique transcriptional regulatory network. Currently, the understanding of the totipotent transcriptional regulatory network is just beginning. A few positive and negative transcription regulators have been identified from the in vitro and in vivo totipotency models.
Among all totipotency-related TFs, Dux, a double homeodomain transcription factor, was the most significantly studied, which was initially found to reprogram pluripotent mESCs toward a 2C-like state (De Iaco et al., 2017; Hendrickson et al., 2017; Whiddon et al., 2017). Upon Dux induction, 2C-related genes including the Zscan4 family, Zfp352, and 2C-specific transposable elements (TEs) were transcriptionally activated. The chromatin landscape was also reorganized to resemble that of mouse 2C embryos (Hendrickson et al., 2017). Similarly, over-expression of the Dux homolog DUX4 in human ESCs can also push a totipotent-like state (De Iaco et al., 2017; Hendrickson et al., 2017; Sugie et al., 2020; Taubenschmid-Stowers et al., 2022). So far, in the 2CLC model, various transcription regulation mechanisms uncovered are centered around Dux. However, despite the fact that Dux is at the central node of the 2CLC state, knocking out Dux cluster genes in mouse embryos led to no dramatic developmental defect, and the mouse can survive to adulthood. Correspondingly, the transcriptome of Dux knock-out embryos had minimal defects around ZGA (Chen and Zhang, 2019); ZGA can still occur normally despite a slight delay (Guo et al., 2019). This implies that Dux plays a supportive rather than an essential role in the activation of the ZGA transcription network in early embryonic development, and parallel or redundant mechanisms exist to initiate ZGA in vivo to sustain the totipotent transcription network (Chen and Zhang, 2019; Guo et al., 2019; De Iaco et al., 2020; Bosnakovski et al., 2021).
Recently, a multi-copy mouse homeobox gene, Obox4, was identified, which potentially acted in parallel with Dux to initiate the ZGA network. Single knockdown of Obox4 or Dux was tolerated by embryogenesis, whereas Obox4/Dux double knockdown completely abolished embryonic development after 2C (Guo et al., 2022). Dux was rapidly degraded at the end of the 2C embryo stage, which is thought to be necessary for exit from the totipotency state both in vivo and in vitro. Prolonged expression of Dux in 2C/4C embryos enhanced the expression of ZGA genes and TEs and led to sustenance of the 2C transcription network (Percharde et al., 2018; Guo et al., 2019). Upon ectopic Dux expression, embryos stalled development and eventually died after one more division (Percharde et al., 2018; Guo et al., 2019; Ruebel et al., 2019). Moreover, exogenously injected Dux RNAs were rapidly degraded in embryos (Guo et al., 2019). In vitro, prolonging Dux over-expression in mESCs not only resulted in increased 2CLCs but also DNA damage and apoptosis (Olbrich et al., 2021). Thus, it is possible that, for proper early embryonic development, precise quantitative and temporal regulation of Dux is crucial.
Maternal RNAs and proteins are transmitted to the zygote, which are important upstream mediators of ZGA. The maternal factor is required to activate Dux (Hendrickson et al., 2017). Paralog maternal factors Dppa2 and Dppa4 were identified to bind the promoters of Dux and activated Dux expression (De Iaco et al., 2019; Yan et al., 2019). In addition, another maternal factor, the pause release factor Nelfa, can also activate Dux through its interaction with DNA topoisomerase 2a (Top2a) (Hu et al., 2020). On the other hand, Dux expression reversely activated Nelfa (Grow et al., 2021). In addition to maternal factors, P53 and DNA-damage response (DDR) pathways were also reported to activate Dux and promote totipotency transcription. These pathways were also reported to be active in mouse early embryos (Atashpaz et al., 2020; Grow et al., 2021). Recently, it was reported that nuclear receptor Nr5a2 was a crucial pioneer activator of major ZGA by promoting chromatin accessibility and was necessary for progression beyond the 2C stage (Gassler et al., 2022). Thus, multi-mechanisms may redundantly ensure the precise timing and regulation of ZGA and totipotency establishment.
Zscan4 family members are also highly expressed transcription factors in mouse and human totipotent cells, among which Zscan4d is the most highly transcribed in 2C embryos, and Zscan4c is a major transcript in mESCs (Falco et al., 2007). Zscan4c interacted with 2C-specific transposable element MT2 (LTR of MERVL) through the SCAN domain, which interacted with the GBAF chromatin remodeling complex to promote the enhancer activity of MT2 (Zhang W. et al., 2019). Reduction in Zscan4 transcript levels slowed down the transition from 2C–4C embryos (Falco et al., 2007). The Zscan4 family is downstream of Dux. Interestingly, Dppa2 and Dppa4, the activators of Dux, can also be activated by Zscan4. In contrast to Dux, Zscan4c cannot induce 2CLCs in Dppa2/4 double-knockout cells (Eckersley-Maslin et al., 2019). The evidence reflects a complex transcription feedback loop between Zscan4, Dppa2/4, and Dux in vitro and in vivo totipotency states (Zhang W. et al., 2019).
In addition to activators, repressors of 2C states were also identified, many of which are active in the pluripotent state of mESCs. Ythdc1, an m6A reader, is required for maintenance of pluripotent mESCs, while the deletion of Ythdc1 facilitated the reversion of mESCs to the 2C-like state through activation of Dux (Liu et al., 2021). Totipotency repressor, zinc finger protein Zfp281, can prominently inhibit Dux-activated transcripts and prevent mESCs from transiting back to the 2C-like state (Wen et al., 2022).
2.2 Transposable elements and the regulation of totipotency
TEs have the ability to transposit in the genome and constitute around 30–50% of mammalian genomes (Elbarbary et al., 2016; Fueyo et al., 2022). Mammalian totipotency has been linked with an extensive transposon-based regulatory mechanism. TEs consist of DNA and RNA transposons (or retrotransposons) (Slotkin and Martienssen, 2007; Kunarso et al., 2010). The retrotransposon was further classified into short interspersed nuclear elements (SINEs), long interspersed nuclear elements (LINEs), and endogenous retroviruses (ERVs). MERVL, the mouse ERVL sub-families, is specifically upregulated in the 2C embryos (Figure 1). Similarly, HERVL expression is also enriched in the human 8C stage (Göke et al., 2015). Both MERVL and HERVL can be bound by DUX or DUX4 (Hendrickson et al., 2017). Efficient and precise activation of MERVL plays a crucial role in early embryogenesis. Loss of MERVL expression impaired developmental progression (Huang et al., 2017; Sakashita et al., 2022), while ectopic activation of MERVL loci in mESCs switched on the 2C-related gene expression (Yang et al., 2020). Many 2C-specific ZGA genes rely on the LTR of MERVL as a promoter or enhancer (Macfarlan et al., 2011, 2012). In addition, the transcribed RNAs from MERVL can also form chimeric transcripts with coding genes to promote the totipotency state (Peaston et al., 2004; Macfarlan et al., 2012). In addition to MERVL, LINE-1 element transcription is observed most in a 2C embryo (Jachowicz et al., 2017), which can function as a nuclear RNA scaffold to recruit the KAP1/nucleolin (NCL) complex to repress Dux in mESCs and pre-implantation mouse embryos (Percharde et al., 2018). In early human embryos, hominoid-specific retrotransposon SINE-VNTR-Alu (SVA) elements are activated by H3K9me3 reprogramming, leading to activation of major ZGA gene expression (Xu R. et al., 2022; Yu et al., 2022).
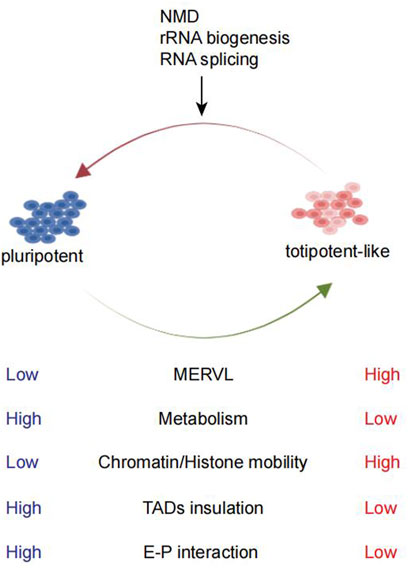
FIGURE 1. Comparison between pluripotent and totipotent-like cells and the pathways studied for totipotent-like exit. mESCs can fluctuate in and out of a transient totipotent-like state and exhibit similarities to totipotent 2C embryos. Totipotent-like cells exhibit high MERVL expression, low metabolism, high chromatin/histone mobility, weakening of TAD insulation, and E–P interaction. NMD, rRNA biogenesis, and RNA splicing are shown to promote totipotent-like exit.
Despite the emerging evidence of TEs’ function in ZGA and totipotency regulation, how their specific activation and repression are coupled to the totipotent transcription network remains poorly understood. ERVs bear motifs to recruit transcription factors or epigenetic modifiers. In the case of MERVL, it can be bound and activated by maternal factor Stella (Huang et al., 2017). Long non-coding RNAs and small RNAs involved in totipotency also participate in regulation of MERVL (Svoboda, 2017), such as miR-34a and miR-344. miR-34a has been demonstrated to be important for activating MERVL and promoting the 2C totipotency state through activating Gata2 expression (Choi et al., 2017). Meanwhile, miR-344 activated by Dux can post-transcriptionally repress ZMYM2-LSD1, a transcriptional corepressor complex, leading to MERVL activation (Yang et al., 2020). Totipotency-related TEs can also be regulated by various epigenetic factors, such as histone H3 variants (Elsässer et al., 2015), H3K9 methyltransferases (Rowe et al., 2010; Maksakova et al., 2013), and histone chaperones (Hatanaka et al., 2015; Ishiuchi et al., 2015; Yang et al., 2015). The facilitates chromatin transcription (FACT) complex is a histone H2A/H2B chaperone, which can repress MERVL and ERV-chimeric transcripts (Chen et al., 2020) through regulating the expression of Nsd2 (Gao et al., 2021). YTHDC1, the m6A reader, can bind and direct ERVK, intracisternal A particles, (IAPs) and LINE1 RNAs to their loci, which led to silencing of these retrotransposons through deposition of histone H3K9me3, facilitating the reprogramming toward totipotent-like states (Liu et al., 2021). In addition, the zinc finger protein, ZFP809, can bind to MERVL through the zinc finger domain and silence MERVL through its KRAB domains by recruiting TRIM28, NURD (histone deacetylase), and SETDB1 (histone methyltransferase) (Wolf et al., 2015; Wang and Goff, 2017).
In conclusion, although numerous regulating factors have been studied so far that can promote the emergence of totipotent-like cells which acquire a generally similar chromatin landscape and epigenetic modifications as 2C embryos, only a few genes acquire the epigenetic features of 2C embryos (Zhang Y. et al., 2021), and further epigenetic regulation needs to be revealed.
3 Epigenetic regulation of totipotency
Totipotency is associated with globally less repressive histone marks and increased chromatin accessibility (Ishiuchi et al., 2015; Eckersley-Maslin et al., 2016). On the other hand, during the transition toward pluripotency, decreased histone mobility can be observed (Bošković et al., 2014) (Figure 1). Recently, further remodeling of pericentromeric heterochromatin and reconstruction of a pluripotency-specific broad H3K4me3 domain facilitated more efficient reprogramming of pluripotent ESCs toward TLSCs, which bears more epigenetic resemblance to 2C embryos (Yang et al., 2022). This suggests that epigenetic reprogramming endues the essential features for the totipotency state. Currently, given that only a few genes acquire 2C-embryo epigenetic signatures (Zhang Y. et al., 2021), further efforts to reprogram the epigenetic modification toward the 2C embryo state remain a major challenge.
3.1 Regulation of DNA methylation in the totipotency state
Global DNA demethylation occurs soon after fertilization until the blastocyst stage, while increasing methylation was observed during differentiation (Smith et al., 2012). Wolf Reik group, identified a global DNA methylation dynamic during the reprogramming of ESCs toward totipotent-like cells (Eckersley-Maslin et al., 2016). Loss of DNMTs led to the reprogramming into the MERVL+Zscan4+ totipotent-like state which was triggered by the genome-wide DNA demethylation. In contrast, during the exit from the totipotent state, the restoration of DNMT protein levels led to establishment of genome-wide DNA methylation (Eckersley-Maslin et al., 2016). DNA demethylation allows the activation of transcription factors, leading to the up-regulation of the MERVL and chromatin decompaction. Furthermore, the DNA demethylase TET may act as an epigenetic barrier for the transition from pluripotent to intermediate and 2C-like states by facilitating KAP1 recruitment (Lu et al., 2014). Correspondingly, Tet1/2/3 triple knock-out cells (TKOs) were more poised to transition to a 2C-like state (Lu et al., 2014; Qiu et al., 2020). In apparent contradiction to these findings, Gadd45, the regulator of TET-mediated DNA demethylation, promoted 2C-like state transition. Gadd45a/b/g TKOs exhibited DNA hypermethylation with impaired transition to a 2C-like state. Gadd45a/b double knock-out mouse embryos showed embryonic lethality, with abrogated ZGA and developmental arrest (Schüle et al., 2019). A negative regulator of Tet, Smchd1, which is involved in X chromosome inactivation in female cells (Blewitt et al., 2008), can also inhibit the 2C transcription program (Huang et al., 2021). In general, Tet may play a dual role in the regulation of the transition toward the 2C stage: first, repression of LINE1 through the KAP1/TRIM28/NCL complex led to silencing of Dux (Lu et al., 2014; Percharde et al., 2018; Schüle et al., 2019). Second, Gadd45 and Smchd1 targeted Tets to promote the 2C conversion via demethylation and protection against de novo methylation (Schüle et al., 2019).
In conclusion, totipotent embryos and totipotent-like cells are both hypomethylated; however, blastocyst and naïve pluripotent stem cells also possess the hypomethylated genome (Leitch et al., 2013). Therefore, hypomethylation could be a general epigenetic feature of early embryo development but not specific for totipotency regulation.
3.2 Regulation of histone modification in the totipotency state
Histone modifications play important roles in many biological processes by regulating chromatin states. Appropriate remodeling of histone modification is critical for embryonic development and totipotency reprogramming (Yang et al., 2021). Treatment with histone deacetylase (HDAC) inhibitors on mESCs can result in the activation of MERVL and/or Zscan4, with the emergence of 2CLCs (Dan et al., 2015; Walter et al., 2016), while knocking down the chromatin remodeler Chd5 (Hayashi et al., 2016), Hnrnpk (Thompson et al., 2015), or members in the repressive chromatin complexes such as Kap-1, Kdm1a, G9a, Hp1, or Rybp similarly led to 2C-like transition (Ishiuchi and Torres-Padilla, 2013).
Core histones H2A, H3.1, and H3.2 are expressed at a high level in 2C embryos but gradually declined as embryos develop (Tian et al., 2020). Caf-1 is responsible for deposition of histones H3 and H4 during DNA synthesis, and depletion of the p150 subunit of CAF-1 leads to chromocenter loss in pluripotent ESCs and transition into 2CLCs (Houlard et al., 2006; Ishiuchi et al., 2015). In addition, H3.3 was directly associated with Dux loci and inhibited Dux expression (Tian et al., 2020). H3.3 is also enriched around MERVL in 2CLCs and 2C embryos, coincident with the onset of MERVL expression (Nakatani et al., 2022). Induction of 2CLCs was found following p150 depletion (Yang et al., 2022), supporting that Caf-1 depletion-induced 2C-like states was associated with altered histone deposition.
It was found that incomplete depletion of H3K9me3 was a key reason underlying the arrest of somatic cloned embryos at the ZGA stage (Matoba et al., 2014; Liu et al., 2016), and abnormal enrichment of H3K9me3 also severely hindered pre-implantation embryonic development in mice (Burton et al., 2020). The correct establishment of H3K9me3 modifications on ERVs was recently found to be depending on Dux (Xu R. et al., 2022). At the human 8C stage, H3K9me3 was reprogrammed on homology-specific retrotransposons of SVA (SINE-VNTR-Alu elements), remodeling the enhancer function of SVA to promote totipotent gene expression (Yu et al., 2022). Setdb1, a histone H3K9 methyltransferase, is essential for embryonic development. Setdb1 deficiency initiated 2C-like transition in a Dux-dependent manner (Wu et al., 2020). Kdm5b, an H3K4 demethylase, can recruit SETDB1 to repress ERVs such as MMVL30 (Zhang S.-M. et al., 2021). Inhibition of Kdm5b promoted the remodeling of broad H3K4me3 regions, which facilitated the stabilization of totipotency transcription (Yang et al., 2022). Aberrant acetylated regions were epigenetic barriers for efficient totipotency reprogramming in SCNT, and histone deacetylase inhibitor TSA can improve the efficiency of Dux cluster gene activation in SCNT (Yang et al., 2021). Globally, MERVL+ cells have increased levels of histone acetylation (Macfarlan et al., 2012; Ishiuchi et al., 2015). Similarly, Zscan4+ cells showed increase in H3K27ac modification in both totipotent genes and retrotransposons (Akiyama et al., 2015).
In mouse 2C embryos, chromatins are associated with a broad decrease in H3K27me3, H3K36me3 and H3K9me3 after fertilization, while a broad increase in H3K27ac modification in the 2C stage is observed (Fu et al., 2020b). A unique chromatin domain structure relating to H3K27me3 is maintained up to the 8C stage and diminishes thereafter (Zheng et al., 2016; Collombet et al., 2020). Most of the differentially expressed genes in 2CLCs displayed globally decreased H3K4me3 and H3K27me3 levels, while de novo H3K4me3 deposition in the promoter regions was found in highly upregulated 2C genes (Zhang Y. et al., 2021). Augmented H3K27ac deposition on MT2 was also observed (Zhang W. et al., 2019). During early human embryogenesis, wider promoter H3K4me3 marks were easily observable at the 4C stage, and a subset of them remained at the 8C stage. However, compared to promoter H3K4me3, the weaker but widespread distal H3K4me3 mark decreased at the 8C stage. Also, there are no H3K27me3 signals in human embryos at the ZGA stage (Xia et al., 2019; Xu et al., 2021). Overall, in the perspective of histone modifications, in vitro totipotent-like cells displayed some similarities toward totipotent embryos, including broad decreased levels of H3K27me3 and H3K4me3 and an increased level of H3K27ac (Akiyama et al., 2015; Zhang Y. et al., 2021).
SUMOylation is largely observed on histones and potentially modulates chromatin structure and transcription (Cubeñas-Potts and Matunis, 2013). The experiments disrupting SUMOylation in embryos revealed its importance on mammalian development (Nacerddine et al., 2005; Lomelí and Vázquez, 2011). SUMOylation can act on chromatin to maintain cellular identity. For example, loss of SUMOylation in ESCs leads to a genome-wide decline of H3K9me3-dependent heterochromatin and converts ESCs into a 2C-like state by releasing PRC1.6 and KAP1/SETDB1 complexes from the Dux loci (Cossec et al., 2018; Theurillat et al., 2020). In addition, histone H1, which is crucial for maintaining heterochromatin, was controlled by SUMO2/3. This modification fixated H1 on the heterochromatin in ESCs and prevented the expression of 2C genes. Depletion of SUMOylation resulted in chromatin condensation and H1 expulsion. As a result, the 2C transcriptional program was activated with an increased H3K27ac level (Sheban et al., 2022). The DPPA2 protein can also be SUMOylated (Theurillat et al., 2020), and this was negatively regulated by SUMO E3 ligase PIAS4. Depleting Pias4 is sufficient to activate Dppa2 and the 2C-like transcription program (Yan et al., 2019). Although SUMOylation is important during mammalian development and 2CLC reprogramming, its function in in vivo totipotent embryos still awaits further elucidation.
3.3 Metabolic influence of totipotency epigenetics
The metabolic state of a cell is a key feature of cellular identity and has been linked to cellular plasticity. During reprogramming to 2CLCs, the metabolic state of cells changed. 2C embryos and 2CLCs exhibited substantial distinctions compared to pluripotent mESCs (Zhao et al., 2021) and generally displayed a low metabolism (Figure 1) level with decreased glycolysis and respiratory chain activity and lower reactive oxygen species (ROS) levels but increased glucose uptake (Rodriguez-Terrones et al., 2020). A screening revealed metabolites such as sodium l-lactate, d-ribose, and sodium acetate as 2CLC inducers (Rodriguez-Terrones et al., 2020). Likewise, related studies have found that chemical inhibition of glycolysis was sufficient to promote fate conversion toward 2CLC (Hu et al., 2020). The underlying influences of metabolism on cell fate decision largely depended on the effect of metabolites in epigenome remodeling (Britt et al., 2020; Zhao et al., 2021), such as the reduction in l-2-hydroxyglutarate was required for embryonic early histone demethylation (Zhao et al., 2021). However, with PCA analysis, 2CLCs were extremely different from 2C embryos and 2CLCs were very close to ESCs (Zhao et al., 2021). Perhaps, we could establish a unique totipotent stem cell model which is closer to the 2C embryo by altering the metabolome of ESCs.
3.4 Regulation of the 3D genome in the totipotency state
In mammalian cells, three-dimensional (3D) genome is critical for gene regulation and is highly dynamic during embryo development. The DNA in the nucleus is hierarchically organized into chromosome territories, compartments, TADs, and chromatin loops. In general, dramatic reprogramming of the 3D chromatin architecture occurs after fertilization. Also, substantial chromatin compaction occurs during the 1-cell to 2-cell transition, resulting in the formation of large chromatin blocks that accumulate near the nuclear envelope (Zheng and Xie, 2019). A higher-order chromatin structure and TADs start to be established from the late 2C stage in mouse embryos (Ke et al., 2017; Fu et al., 2020b), while during human embryonic development, TAD structure is gradually established after fertilization. Notably, blocking ZGA can inhibit TAD establishment in human embryos but not in mice (Chen et al., 2019).
Previous studies revealed both 2CLCs and 2C embryos display more relaxed chromatin structure than mESCs, including an overall weakened enhancer–promoter (E–P) interactions and TAD insulation (Ishiuchi et al., 2015; Zhu et al., 2021b) (Figure 1). While the weakened E–P interaction was associated with mESC enhancer inactivation and down-regulation of pluripotency genes, the weakened TAD insulation may promote contacts between the putative new 2C enhancers and neighboring 2C genes (Zhu et al., 2021b). Typically, chromocenters and major satellite transcription are features of the totipotency state (Houlard et al., 2006). However, whether the change in 3D chromatin structures is a cause or consequence of the totipotency state is still worth exploring. Recently, it was found that the inhibition of Dot1l can induce 2C-like transcription state with collapsed chromocenters and reduced H3K79me3, representing the remodeled pericentromeric heterochromatin. As a result, MERVL loci were released from the heterochromatic environment and become activated (Xu Y. et al., 2022; Yang et al., 2022).
In addition, when nucleoli were disrupted by blocking RNA polymerase I activity or preventing nucleolar phase separation, Dux loci can dissociate from the nucleolar surface and get activated, promoting the transition to 2CLCs (Xie et al., 2022). In mouse embryos, rRNA biogenesis and nucleolus defect prevented nucleolar maturation, resulting in developmental arrest at the 2C–4C stage (Zhu et al., 2021a; Xie et al., 2022).
The topology in the genome is mediated by CTCF (CCCTC-binding factor) and cohesin (Merkenschlager and Nora, 2016). CTCF can act as a transcriptional activator through interaction with RNA PolII (Chernukhin et al., 2007), and it can also act as a transcription repressor when functioning together with the histone deacetylase complex (Lutz et al., 2000). Depletion of CTCF or cohesion (Zhu et al., 2021b) facilitated ESCs to transition into 2CLCs (Song et al., 2022) with chromatin relaxation and activation of Dux loci (Olbrich et al., 2021). The transition from totipotency to pluripotency during embryonic development was also characterized by the progressive accumulation of CTCF and maturation of TADs (Du et al., 2017; Ke et al., 2017; Chen et al., 2019). CTCF also modulated the 3D genome to enable pluripotency reprogramming by acting as an insulator along with imitation switch chromatin remodeler Smarca5 (Song et al., 2022). However, another study showed that Smarca5 positively regulates 2C-like transcription in a Dppa2-dependent manner (Alda-Catalinas et al., 2020). Thus, it was possible that Smarca5 and CTCF may potentially act together or independently to exert cell state-specific transcription functions.
In general, 3D genome is closely related to the chromatin organization and also influences the rewiring of the transcription network.
4 Cell cycle and DNA damage response in totipotency
Cell fate decision processes, such as differentiation and reprogramming, are typically coupled with cell cycle progression (Gonzales et al., 2015; Gruenheit et al., 2018). Totipotent stem cells potentially possess distinct cell cycle patterns compared with cells from other developmental stages. For instance, 2CLCs, and also 2C embryo cells, have been shown to slow down the DNA replication fork speed (Nakatani et al., 2022). 2CLCs were found to emerge along with slow DNA replication, and genes highly expressed in 2CLCs had distinct replication timing (RT), mainly during the early S-phase. The slow replication fork speed enabled the remodeling of replication timing in MERVL-enriched specific genomic regions toward the early S-phase, which led to their activation (Nakatani et al., 2022). In general, slowing down the replication fork speed induced 2CLCs and improved the reprogramming efficiency of somatic cell nuclear transfer (Nakatani et al., 2022).
In addition, the totipotency state is usually associated with prolonged G2/M phases (Eckersley-Maslin et al., 2016; Atashpaz et al., 2020; Zhu et al., 2021a). In Dux over-expression-induced 2CLCs, those with higher MERVL expression (2C-IN) exhibited a prolonged G1 phase, while cells with lower MERVL expression (2C-OUT) exhibited a prolonged G2/M phase (Zhu et al., 2021a). However, whether and how specific cell cycle-related pathways and proteins play a role during the 2CLC transition from ESCs remains elusive. The influence of the cell cycle on the 2C-like state may be partly due to the regulation of the nucleolar structure. During the cell cycle progression, dynamic remodeling occurs in the nucleolus (Probst et al., 2009; Padeken and Heun, 2014; Nagano et al., 2017; Zhang H. et al., 2019). Immature nucleolus structure and reduced rRNA synthesis were found in 2CLCs and 2C embryos (Xie et al., 2022). Interestingly, it was demonstrated that LIN28 could interact with the TRIM28/NCL complex to mediate the repression of Dux in mESCs and pre-implantation embryos (Percharde et al., 2018; Sun et al., 2022). When rRNA biogenesis was inhibited, the TRIM28/NCL/LIN28 complex dissociated from peri-nucleolar heterochromatin (PNH), which led to Dux loci releasing from PNH and activation of MERVL and 2C genes (Yu et al., 2021). Similar changes in nucleoli were also observed when mESCs were arrested by DNA synthesis inhibitor cytarabine CYT (Zhu et al., 2021a). CYT triggered 2C gene activation independent of the ATR-CHK1 and the p53 pathways (Zhu et al., 2021a).
Cell cycle progression and DNA synthesis also potentiate the generation of DNA damage. Critically, multiple sources contribute to endogenous DNA damage in early embryo development (Ziegler-Birling et al., 2009; Wossidlo et al., 2010; Srinivasan et al., 2020). Similarly, in Dux-induced 2CLCs, researchers observed increased DNA damage and cell death (Guo et al., 2019; Olbrich et al., 2021). DNA damage triggered the activation of ATM/ATR and CHKs, which activated Grsf1 (G-rich sequence factor 1) to stabilize Dux mRNA and induce 2C-like states (Atashpaz et al., 2020). Moreover, p53, the downstream effector of DDR, was rapidly activated after fertilization and promoted Dux function and ZGA gene expression. Importantly, p53 expression can rescue Dux activation in Dppa2/4 double knock-out mESCs (Grow et al., 2021). This suggests that the DDR/P53 pathway can participate in the timely activation of totipotent genes.
Although DNA damage and CHK1 activation induced 2CLCs (Atashpaz et al., 2020; Grow et al., 2021), no increase in γH2AX was observed in 2CLCs. Furthermore, depletion of checkpoint proteins did not affect the generation of 2CLCs, leaving the necessity of the DDR pathway in totipotency to be elucidated (Nakatani et al., 2022). In mammalian pre-implantation embryos, DNA replication stress predisposed gene-poor regions to chromatin instability, which impaired developmental potential of cells (Palmerola et al., 2022). The DNA damage resulted in reduced replication fork speed (Tercero and Diffley, 2001). A possible cause of replication fork stalling is DNA demethylation (Guo et al., 2014). Also, γH2AX foci were found in regions with active DNA demethylation in mouse pre-implantation embryos (Wossidlo et al., 2010).
Overall, totipotent-like cells and totipotent embryos potentially possess distinct cell cycle patterns compared with other cells. Meanwhile, DNA replication stress is abundant during mammalian pre-implantation embryos and can activate the 2CLC network (Atashpaz et al., 2020; Grow et al., 2021). In the future, the mechanism of how DNA damage occurs in early embryos and how cell cycle affects embryonic development potential will need to be further elucidated.
5 RNA metabolism and totipotency regulation
RNA metabolism, including RNA synthesis, modification, and degradation, can also contribute to gene expression dynamics (Qiu et al., 2020). RNA splicing can regulate mRNA stability and plays an important role in vertebrate development. Interestingly, screening of factors promoting the 2C-like state revealed a large number of candidates belonging to spliceosome and RNA splicing pathways (Rodriguez-Terrones et al., 2018). Recently, Zrsr1 and Zrsr2, minor splicing factors, were found to be essential for ZGA and the transition to 2CLCs from mESCs. Zrsr1/2 double knock-out embryos arrested at 2–4C stages and RNA-seq revealed remarkable upregulation of RNAs of early embryo development. This was linked to a blockade of maternal RNA degradation (Gómez-Redondo et al., 2020). Remarkably, a broad-spectrum spliceosome inhibitor, plaB, can reverse mESCs into a totipotent transcription network and promote the 2C-like state conversion (Shen et al., 2021). Apart from splicing, the nonsense-mediated mRNA decay (NMD) pathway was also linked to 2C and totipotency states. It was identified to degrade Dux RNA upon entry into the 2C state (Fu et al., 2020a). However, as a general spectrum RNA quality surveillance pathway, how NMD specifically facilitate 2C-related RNA degradation during early development is still unknown. In addition, using an unbiased genome-scale CRISPR knock-out screen in mESCs, m6A modification-related genes, such as Mettl3/14, were found to repress the RNA abundance of IAPs and ERVK elements, thus promoting the 2C-like transition from mESCs (Chelmicki et al., 2021). With increasing evidence demonstrating the effect of RNA metabolism on the totipotency transcription network and totipotency reprogramming, still more effects are needed to elucidate the molecular bases of RNA metabolism in the context of early development and totipotency.
6 Regulation of the signaling pathway
The signaling pathway is an essential way of signal transmission between cells and with the external environment. Key signaling pathways are of pressing importance during embryonic development. The expanded developmental potential of EPSCs was thought to be mediated by high BMP4 and low activin/nodal signaling activity. In a similar manner, human blastoid formation was improved by triple inhibition of the Hippo, TGF-β, and ERK pathways with chemical inhibitors (Malik and Wang, 2022). The active Akt signaling pathway supports self-renewal of ESCs; in contrast, Akt-specific inhibitors API-2 and MK2206 could induce 2C arrest and significantly downregulated the mRNA of MERVL and eIF-1A, implying p-Ser473-Akt may be a potential player in the totipotency transcription in 2C mouse embryos (Chen et al., 2016).
Retinoic acid (RA), a metabolite of vitamin A, displays important roles during embryonic development. Several studies reported that RA drove a NELFA-mediated 2C-like state under S/LIF culture conditions (Sharova et al., 2016; Tagliaferri et al., 2020; Iturbide, 2021; Wang et al., 2021) through a coordinated expression of Dux and Duxbl1 and the activation of Prame family member Gm12794c (Napolitano et al., 2020). Not only transcriptional and developmental potentials but also DNA methylation, histone modification, glycolysis, and protein synthesis exhibit similarities to 2C embryos in RA-induced 2C-like cells (Wang et al., 2021). However, RA-treated cells gradually died or differentiated, and they failed to maintain a stable totipotent state (Hu et al., 2022). In the pluripotent state, RA counteracted the differentiation and maintains pluripotency, through the activation of WNT signaling (Wang et al., 2008), Nanog expression (Chen et al., 2007), and phosphoinositide 3-kinase (PI3K) activation (Chen and Khillan, 2010). Chemical manipulation of signaling pathways could further improve the totipotency reprogramming efficiency and may lead to the conversion toward a more functional totipotency state in vitro (Malik and Wang, 2022).
7 Understanding the totipotency state from integrated angles
The establishment of a stable in vitro totipotent stem cell culture system has been a long-term goal of the stem cell and early developmental biology field. Currently, various in vitro culture systems have been identified or established, including 2CLCs (Macfarlan et al., 2012), EPSCs (Yang Y. et al., 2017; Yang et al., 2017 Y.), TBLCs (Shen et al., 2021), TLSCs (Yang et al., 2022), ciTotiSCs (Hu et al., 2022), and TPS cells (Xu Y. et al., 2022). Cells from these models mimicked totipotent transcriptome to different extents and can give rise to intra- and extraembryonic lineages under different experimental contexts.
In vitro totipotent state is a dynamic state, and the cells induced to reprogram the 2C-like state were found to spontaneously roll back to the original pluripotent state (Macfarlan et al., 2012; Rodriguez-Terrones et al., 2018; Olbrich et al., 2021). It is thought that dynamic alteration in the transcriptome may be the underlying cause. Negative feedback responses were evident in the 2CLC transcription network, which may be associated with the instability of 2CLCs. For instance, Dppa2 and Dppa4 formed a negative feedback loop involving Dux and Dux repressors LINE-1 (De Iaco et al., 2019). Otherwise, ZSCAN4 bound to a CA repeat embedded in the Dux upstream of the TSS unit and downregulated Dux expression in the presence of double-stranded DNA breaks (Grow et al., 2021). In addition, Duxbl1 expression and 2CL state were induced by the treatment of RA (Sharova et al., 2016; Tagliaferri et al., 2020; Iturbide, 2021; Wang et al., 2021); however, in the meantime, Duxbl1 can directly downregulate Zscan4 and endogenous Duxbl1 (Tagliaferri et al., 2020). These shreds of evidence indicated negative feedback regulation exists in the 2CLC transcriptional regulatory network and may underlie the instability of in vitro totipotency obtained so far. Moreover, early totipotent embryos and totipotent-like cells exhibit low metabolism and DNA damage activation. These properties argue against a self-sustainable totipotency state which can be stably maintained in the long term.
Using transcriptome profiling of the Dux-induced 2CLC system, Fu et al. (2020a) found that the entry and exit from the 2C-like state follow distinct transcription pathways. NMD-mediated mRNA decay of Dux seems to play a very important role during the 2C exit process. Apart from RNA metabolism, biological processes negatively regulating the 2C state may be involved in the exit from totipotency and embryonic development after 2C. RNA splicing, RNA decay (Fu et al., 2020a; Shen et al., 2021), and rRNA biosynthesis (Grow et al., 2021; Yu et al., 2021) were all shown to exhibit negative regulatory effects on 2CLCs (Figure 1). Prdm14a, a pluripotency transcription factor, acted as a barrier in the transition of mESCs to 2CLCs (Nakaki and Saitou, 2014). Myc can prevent the transition to the 2C-like state by actively maintaining the pluripotent transcriptome (Fu et al., 2019). Otx2, a member of the OTX family highly expressed in mouse blastocysts and ESCs, negatively regulated Dux, Zscan4c, MERVL, and other ZGA genes in mESCs (Guo et al., 2021). Highly abundant TRIM66/DAX1 in mESCs served as a negative regulator of the 2C-like state through repressing Dux transcription (Zuo et al., 2022). These factors may all be involved in the dissolution of the 2C-like state.
Therefore, the exit from the in vitro 2C-like state also mimics the developmental process from 2C to morula and blastocyst stages. Understanding whether 2C embryos also exhibit similar 2C exit mechanisms as in vitro 2C-like cells and whether the totipotency state is intrinsically associated with a dynamically wired and unstable transcription state has great implications for us to understand the transcriptional properties in early embryo development.
8 Summary and outlook
The establishment of a totipotent-like system in vitro greatly helped us chart the map of embryonic development. Given the knowledge about totipotency obtained so far, large gaps still exist in fully understanding the regulatory and functional properties of the totipotency state. Totipotency represents the highest developmental potential in early embryogenesis and thus potentially can contribute toward the widest applications of stem cells such as reproduction, development, and tissue/organ regeneration. It is evident that further understanding of totipotency regulation and optimizing chemically defined culture conditions will further increase the efficiency of totipotency reprogramming and create more functional in vitro totipotency models for basic research and regenerative medicine (Malik and Wang, 2022).
Author contributions
HX and HL conceived and wrote the review manuscript. All authors have read and agreed to the published version of the manuscript.
Funding
This work was supported by the National Natural Science Foundation of China (Nos. 31871372 and 31950410535) and Key Research and Development Program of Zhejiang Province (LZ22C120002).
Acknowledgments
The authors thank Danya Wu, Zhipeng Ai, Junjiao Wang, and Yangquan Xiang for comments on the manuscript. Also, the authors are grateful for the funding by the National Natural Science Foundation of China (No.31871372, 31950410535) and Key Research and Development Program of Zhejiang Province (LZ22C120002).
Conflict of interest
The authors declare that the research was conducted in the absence of any commercial or financial relationships that could be construed as a potential conflict of interest.
Publisher’s note
All claims expressed in this article are solely those of the authors and do not necessarily represent those of their affiliated organizations, or those of the publisher, the editors, and the reviewers. Any product that may be evaluated in this article, or claim that may be made by its manufacturer, is not guaranteed or endorsed by the publisher.
References
Abe, K.-I., Funaya, S., Tsukioka, D., Kawamura, M., Suzuki, Y., Suzuki, M. G., et al. (2018). Minor zygotic gene activation is essential for mouse preimplantation development. Proc. Natl. Acad. Sci. U. S. A. 115, E6780–E6788. doi:10.1073/pnas.1804309115
Akiyama, T., Xin, L., Oda, M., Sharov, A. A., Amano, M., Piao, Y., et al. (2015). Transient bursts of Zscan4 expression are accompanied by the rapid derepression of heterochromatin in mouse embryonic stem cells. DNA Res. 22, 307–318. doi:10.1093/dnares/dsv013
Alda-Catalinas, C., Bredikhin, D., Hernando-Herraez, I., Santos, F., Kubinyecz, O., Eckersley-Maslin, M. A., et al. (2020). A single-cell transcriptomics CRISPR-activation screen identifies epigenetic regulators of the zygotic genome activation program. Cell Syst. 11, 25–41. e9. doi:10.1016/j.cels.2020.06.004
Atashpaz, S., Samadi Shams, S., Gonzalez, J. M., Sebestyén, E., Arghavanifard, N., Gnocchi, A., et al. (2020). ATR expands embryonic stem cell fate potential in response to replication stress. eLife 9, e54756. doi:10.7554/eLife.54756
Blewitt, M. E., Gendrel, A.-V., Pang, Z., Sparrow, D. B., Whitelaw, N., Craig, J. M., et al. (2008). SmcHD1, containing a structural-maintenance-of-chromosomes hinge domain, has a critical role in X inactivation. Nat. Genet. 40, 663–669. doi:10.1038/ng.142
Bošković, A., Eid, A., Pontabry, J., Ishiuchi, T., Spiegelhalter, C., Raghu Ram, E. V. S., et al. (2014). Higher chromatin mobility supports totipotency and precedes pluripotency in vivo. Genes Dev. 28, 1042–1047. doi:10.1101/gad.238881.114
Bosnakovski, D., Gearhart, M. D., Ho Choi, S., and Kyba, M. (2021). Dux facilitates post-implantation development, but is not essential for zygotic genome activation. Biol. Reprod. 104, 83–93. doi:10.1093/biolre/ioaa179
Britt, E. C., John, S. V., Locasale, J. W., and Fan, J. (2020). Metabolic regulation of epigenetic remodeling in immune cells. Curr. Opin. Biotechnol. 63, 111–117. doi:10.1016/j.copbio.2019.12.008
Burton, A., Brochard, V., Galan, C., Ruiz-Morales, E. R., Rovira, Q., Rodriguez-Terrones, D., et al. (2020). Heterochromatin establishment during early mammalian development is regulated by pericentromeric RNA and characterized by non-repressive H3K9me3. Nat. Cell Biol. 22, 767–778. doi:10.1038/s41556-020-0536-6
Chelmicki, T., Roger, E., Teissandier, A., Dura, M., Bonneville, L., Rucli, S., et al. (2021). m6A RNA methylation regulates the fate of endogenous retroviruses. Nature 591, 312–316. doi:10.1038/s41586-020-03135-1
Chen, F., Zhang, W., Xie, D., Gao, T., Dong, Z., and Lu, X. (2020). Histone chaperone FACT represses retrotransposon MERVL and MERVL-derived cryptic promoters. Nucleic Acids Res. 48, 10211–10225. doi:10.1093/nar/gkaa732
Chen, J., Lian, X., Du, J., Xu, S., Wei, J., Pang, L., et al. (2016). Inhibition of phosphorylated Ser473-Akt from translocating into the nucleus contributes to 2-cell arrest and defective zygotic genome activation in mouse preimplantation embryogenesis. Dev. Growth Differ. 58, 280–292. doi:10.1111/dgd.12273
Chen, L., and Khillan, J. S. (2010). A novel signaling by vitamin A/retinol promotes self renewal of mouse embryonic stem cells by activating PI3K/Akt signaling pathway via insulin-like growth factor-1 receptor. Stem Cells 28, 57–63. doi:10.1002/stem.251
Chen, L., Yang, M., Dawes, J., and Khillan, J. S. (2007). Suppression of ES cell differentiation by retinol (vitamin A) via the overexpression of Nanog. Differentiation. 75, 682–693. doi:10.1111/j.1432-0436.2007.00169.x
Chen, X., Ke, Y., Wu, K., Zhao, H., Sun, Y., Gao, L., et al. (2019). Key role for CTCF in establishing chromatin structure in human embryos. Nature 576, 306–310. doi:10.1038/s41586-019-1812-0
Chen, Z., and Zhang, Y. (2019). Loss of DUX causes minor defects in zygotic genome activation and is compatible with mouse development. Nat. Genet. 51, 947–951. doi:10.1038/s41588-019-0418-7
Chernukhin, I., Shamsuddin, S., Kang, S. Y., Bergström, R., Kwon, Y.-W., Yu, W., et al. (2007). CTCF interacts with and recruits the largest subunit of RNA polymerase II to CTCF target sites genome-wide. Mol. Cell. Biol. 27, 1631–1648. doi:10.1128/MCB.01993-06
Choi, Y. J., Lin, C.-P., Risso, D., Chen, S., Kim, T. A., Tan, M. H., et al. (2017). Deficiency of microRNA miR-34a expands cell fate potential in pluripotent stem cells. Science 355, eaag1927. doi:10.1126/science.aag1927
Collombet, S., Ranisavljevic, N., Nagano, T., Varnai, C., Shisode, T., Leung, W., et al. (2020). Parental-to-embryo switch of chromosome organization in early embryogenesis. Nature 580, 142–146. doi:10.1038/s41586-020-2125-z
Cossec, J.-C., Theurillat, I., Chica, C., Búa Aguín, S., Gaume, X., Andrieux, A., et al. (2018). SUMO safeguards somatic and pluripotent cell identities by enforcing distinct chromatin states. Cell Stem Cell 23, 742–757. e8. doi:10.1016/j.stem.2018.10.001
Cubeñas-Potts, C., and Matunis, M. J. (2013). Sumo: A multifaceted modifier of chromatin structure and function. Dev. Cell 24, 1–12. doi:10.1016/j.devcel.2012.11.020
Dan, J., Yang, J., Liu, Y., Xiao, A., and Liu, L. (2015). Roles for histone acetylation in regulation of telomere elongation and two-cell state in mouse ES cells. J. Cell. Physiol. 230, 2337–2344. doi:10.1002/jcp.24980
De Iaco, A., Coudray, A., Duc, J., and Trono, D. (2019). DPPA2 and DPPA4 are necessary to establish a 2C‐like state in mouse embryonic stem cells. EMBO Rep. 20, e47382. doi:10.15252/embr.201847382
De Iaco, A., Planet, E., Coluccio, A., Verp, S., Duc, J., and Trono, D. (2017). DUX-family transcription factors regulate zygotic genome activation in placental mammals. Nat. Genet. 49, 941–945. doi:10.1038/ng.3858
De Iaco, A., Verp, S., Offner, S., Grun, D., and Trono, D. (2020). DUX is a non-essential synchronizer of zygotic genome activation. Development 147, dev177725. doi:10.1242/dev.177725
Du, Z., Zheng, H., Huang, B., Ma, R., Wu, J., Zhang, X., et al. (2017). Allelic reprogramming of 3D chromatin architecture during early mammalian development. Nature 547, 232–235. doi:10.1038/nature23263
Eckersley-Maslin, M., Alda-Catalinas, C., Blotenburg, M., Kreibich, E., Krueger, C., and Reik, W. (2019). Dppa2 and Dppa4 directly regulate the Dux-driven zygotic transcriptional program. Genes Dev. 33, 194–208. doi:10.1101/gad.321174.118
Eckersley-Maslin, M. A., Svensson, V., Krueger, C., Stubbs, T. M., Giehr, P., Krueger, F., et al. (2016). MERVL/Zscan4 network activation results in transient genome-wide DNA demethylation of mESCs. Cell Rep. 17, 179–192. doi:10.1016/j.celrep.2016.08.087
Elbarbary, R. A., Lucas, B. A., and Maquat, L. E. (2016). Retrotransposons as regulators of gene expression. Science 351, aac7247. doi:10.1126/science.aac7247
Elsässer, S. J., Noh, K.-M., Diaz, N., Allis, C. D., and Banaszynski, L. A. (2015). Histone H3.3 is required for endogenous retroviral element silencing in embryonic stem cells. Nature 522, 240–244. doi:10.1038/nature14345
Falco, G., Lee, S.-L., Stanghellini, I., Bassey, U. C., Hamatani, T., and Ko, M. S. H. (2007). Zscan4: A novel gene expressed exclusively in late 2-cell embryos and embryonic stem cells. Dev. Biol. 307, 539–550. doi:10.1016/j.ydbio.2007.05.003
Fu, X., Djekidel, M. N., and Zhang, Y. (2020a). A transcriptional roadmap for 2C-like–to–pluripotent state transition. Sci. Adv. 6, eaay5181. doi:10.1126/sciadv.aay5181
Fu, X., Wu, X., Djekidel, M. N., and Zhang, Y. (2019). Myc and Dnmt1 impede the pluripotent to totipotent state transition in embryonic stem cells. Nat. Cell Biol. 21, 835–844. doi:10.1038/s41556-019-0343-0
Fu, X., Zhang, C., and Zhang, Y. (2020b). Epigenetic regulation of mouse preimplantation embryo development. Curr. Opin. Genet. Dev. 64, 13–20. doi:10.1016/j.gde.2020.05.015
Fueyo, R., Judd, J., Feschotte, C., and Wysocka, J. (2022). Roles of transposable elements in the regulation of mammalian transcription. Nat. Rev. Mol. Cell Biol. 23, 481–497. doi:10.1038/s41580-022-00457-y
Gao, T., Chen, F., Zhang, W., Zhao, X., Hu, X., and Lu, X. (2021). Nsd2 represses endogenous retrovirus MERVL in embryonic stem cells. Stem Cells Int. 2021, 1–8. doi:10.1155/2021/6663960
Gassler, J., Kobayashi, W., Gáspár, I., Ruangroengkulrith, S., Kümmecke, M., Kravchenko, P., et al. (2022). Zygotic genome activation by the totipotency pioneer factor Nr5a2. Developmental Biology. Available at: https://www.biorxiv.org/content/10.1101/2022.05.17.492379v1.full.pdf (Accessed May 17, 2022).doi:10.1101/2022.05.17.492379
Göke, J., Lu, X., Chan, Y.-S., Ng, H.-H., Ly, L.-H., Sachs, F., et al. (2015). Dynamic transcription of distinct classes of endogenous retroviral elements marks specific populations of early human embryonic cells. Cell Stem Cell 16, 135–141. doi:10.1016/j.stem.2015.01.005
Gómez-Redondo, I., Ramos-Ibeas, P., Pericuesta, E., Fernández-González, R., Laguna-Barraza, R., and Gutiérrez-Adán, A. (2020). Minor splicing factors Zrsr1 and Zrsr2 are essential for early embryo development and 2-cell-like conversion. Int. J. Mol. Sci. 21, 4115. doi:10.3390/ijms21114115
Gonzales, K. A. U., Liang, H., Lim, Y.-S., Chan, Y.-S., Yeo, J.-C., Tan, C.-P., et al. (2015). Deterministic restriction on pluripotent state dissolution by cell-cycle pathways. Cell 162, 564–579. doi:10.1016/j.cell.2015.07.001
Grow, E. J., Weaver, B. D., Smith, C. M., Guo, J., Stein, P., Shadle, S. C., et al. (2021). p53 convergently activates Dux/DUX4 in embryonic stem cells and in facioscapulohumeral muscular dystrophy cell models. Nat. Genet. 53, 1207–1220. doi:10.1038/s41588-021-00893-0
Gruenheit, N., Parkinson, K., Brimson, C. A., Kuwana, S., Johnson, E. J., Nagayama, K., et al. (2018). Cell cycle heterogeneity can generate robust cell type proportioning. Dev. Cell 47, 494–508. e4. doi:10.1016/j.devcel.2018.09.023
Guo, H., Zhu, P., Yan, L., Li, R., Hu, B., Lian, Y., et al. (2014). The DNA methylation landscape of human early embryos. Nature 511, 606–610. doi:10.1038/nature13544
Guo, M., Zhang, Y., Zhou, J., Bi, Y., Xu, J., Xu, C., et al. (2019). Precise temporal regulation of Dux is important for embryo development. Cell Res. 29, 956–959. doi:10.1038/s41422-019-0238-4
Guo, S.-M., Mei, N.-H., Yang, J., and Zhou, L.-Q. (2021). Transcription factor OTX2 silences the expression of cleavage embryo genes and transposable elements. J. Reprod. Dev. 67, 223–228. doi:10.1262/jrd.2021-007
Guo, Y., Kitano, T., Murano, K., Li, T. D., Sakashita, A., Ishizu, H., et al. (2022). Obox4 secures zygotic genome activation upon loss of Dux. Genetics. Available at: https://www.biorxiv.org/content/biorxiv/early/2022/07/05/2022.07.04.498763.full.pdf (Accessed July 5, 2022).doi:10.1101/2022.07.04.498763
Gurdon, J. B. (1962). The developmental capacity of nuclei taken from intestinal epithelium cells of feeding tadpoles. Development 10, 622–640. doi:10.1242/dev.10.4.622
Hatanaka, Y., Inoue, K., Oikawa, M., Kamimura, S., Ogonuki, N., Kodama, E. N., et al. (2015). Histone chaperone CAF-1 mediates repressive histone modifications to protect preimplantation mouse embryos from endogenous retrotransposons. Proc. Natl. Acad. Sci. U. S. A. 112, 14641–14646. doi:10.1073/pnas.1512775112
Hayashi, M., Maehara, K., Harada, A., Semba, Y., Kudo, K., Takahashi, H., et al. (2016). Chd5 regulates MuERV-L/MERVL expression in mouse embryonic stem cells via H3K27me3 modification and histone H3.1/H3.2. J. Cell. Biochem. 117, 780–792. doi:10.1002/jcb.25368
Hendrickson, P. G., Doráis, J. A., Grow, E. J., Whiddon, J. L., Lim, J.-W., Wike, C. L., et al. (2017). Conserved roles of mouse DUX and human DUX4 in activating cleavage-stage genes and MERVL/HERVL retrotransposons. Nat. Genet. 49, 925–934. doi:10.1038/ng.3844
Houlard, M., Berlivet, S., Probst, A. V., Quivy, J.-P., Héry, P., Almouzni, G., et al. (2006). CAF-1 is essential for heterochromatin organization in pluripotent embryonic cells. PLoS Genet. 2, e181. doi:10.1371/journal.pgen.0020181
Hu, Y., Yang, Y., Tan, P., Zhang, Y., Han, M., Yu, J., et al. (2022). Induction of mouse totipotent stem cells by a defined chemical cocktail. Nature. Online ahead of print. doi:10.1038/s41586-022-04967-9
Hu, Z., Tan, D. E. K., Chia, G., Tan, H., Leong, H. F., Chen, B. J., et al. (2020). Maternal factor NELFA drives a 2C-like state in mouse embryonic stem cells. Nat. Cell Biol. 22, 175–186. doi:10.1038/s41556-019-0453-8
Huang, Y., Kim, J. K., Do, D. V., Lee, C., Penfold, C. A., Zylicz, J. J., et al. (2017). Stella modulates transcriptional and endogenous retrovirus programs during maternal-to-zygotic transition. Elife 6, e22345. doi:10.7554/eLife.22345
Huang, Z., Yu, J., Cui, W., Johnson, B. K., Kim, K., and Pfeifer, G. P. (2021). The chromosomal protein SMCHD1 regulates DNA methylation and the 2c-like state of embryonic stem cells by antagonizing TET proteins. Sci. Adv. 7, eabb9149. doi:10.1126/sciadv.abb9149
Ishiuchi, T., Enriquez-Gasca, R., Mizutani, E., Bošković, A., Ziegler-Birling, C., Rodriguez-Terrones, D., et al. (2015). Early embryonic-like cells are induced by downregulating replication-dependent chromatin assembly. Nat. Struct. Mol. Biol. 22, 662–671. doi:10.1038/nsmb.3066
Ishiuchi, T., and Torres-Padilla, M.-E. (2013). Towards an understanding of the regulatory mechanisms of totipotency. Curr. Opin. Genet. Dev. 23, 512–518. doi:10.1016/j.gde.2013.06.006
Iturbide, A., Ruiz Tejada Segura, M. L., Noll, C., Schorpp, K., Rothenaigner, I., Ruiz-Morales, E. R., et al. (2021). Retinoic acid signaling is critical during the totipotency window in early mammalian development. Nat. Struct. Mol. Biol. 28, 521–532. doi:10.1038/s41594-021-00590-w
Jachowicz, J. W., Bing, X., Pontabry, J., Bošković, A., Rando, O. J., and Torres-Padilla, M.-E. (2017). LINE-1 activation after fertilization regulates global chromatin accessibility in the early mouse embryo. Nat. Genet. 49, 1502–1510. doi:10.1038/ng.3945
Jukam, D., Shariati, S. A. M., and Skotheim, J. M. (2017). Zygotic genome activation in vertebrates. Dev. Cell 42, 316–332. doi:10.1016/j.devcel.2017.07.026
Ke, Y., Xu, Y., Chen, X., Feng, S., Liu, Z., Sun, Y., et al. (2017). 3D chromatin structures of mature gametes and structural reprogramming during mammalian embryogenesis. Cell 170, 367–381. e20. doi:10.1016/j.cell.2017.06.029
Kunarso, G., Chia, N.-Y., Jeyakani, J., Hwang, C., Lu, X., Chan, Y.-S., et al. (2010). Transposable elements have rewired the core regulatory network of human embryonic stem cells. Nat. Genet. 42, 631–634. doi:10.1038/ng.600
Leitch, H. G., McEwen, K. R., Turp, A., Encheva, V., Carroll, T., Grabole, N., et al. (2013). Naive pluripotency is associated with global DNA hypomethylation. Nat. Struct. Mol. Biol. 20, 311–316. doi:10.1038/nsmb.2510
Li, M., and Izpisua Belmonte, J. C. (2018). Deconstructing the pluripotency gene regulatory network. Nat. Cell Biol. 20, 382–392. doi:10.1038/s41556-018-0067-6
Liu, J., Gao, M., He, J., Wu, K., Lin, S., Jin, L., et al. (2021). The RNA m6A reader YTHDC1 silences retrotransposons and guards ES cell identity. Nature 591, 322–326. doi:10.1038/s41586-021-03313-9
Liu, W., Liu, X., Wang, C., Gao, Y., Gao, R., Kou, X., et al. (2016). Identification of key factors conquering developmental arrest of somatic cell cloned embryos by combining embryo biopsy and single-cell sequencing. Cell Discov. 2, 16010. doi:10.1038/celldisc.2016.10
Lomelí, H., and Vázquez, M. (2011). Emerging roles of the SUMO pathway in development. Cell. Mol. Life Sci. 68, 4045–4064. doi:10.1007/s00018-011-0792-5
Lu, F., Liu, Y., Jiang, L., Yamaguchi, S., and Zhang, Y. (2014). Role of Tet proteins in enhancer activity and telomere elongation. Genes Dev. 28, 2103–2119. doi:10.1101/gad.248005.114
Lu, F., and Zhang, Y. (2015). Cell totipotency: Molecular features, induction, and maintenance. Natl. Sci. Rev. 2, 217–225. doi:10.1093/nsr/nwv009
Lutz, M., Burke, L. J., Barreto, G., Goeman, F., Greb, H., Arnold, R., et al. (2000). Transcriptional repression by the insulator protein CTCF involves histone deacetylases. Nucleic Acids Res. 28, 1707–1713. doi:10.1093/nar/28.8.1707
Macfarlan, T. S., Gifford, W. D., Agarwal, S., Driscoll, S., Lettieri, K., Wang, J., et al. (2011). Endogenous retroviruses and neighboring genes are coordinately repressed by LSD1/KDM1A. Genes Dev. 25, 594–607. doi:10.1101/gad.2008511
Macfarlan, T. S., Gifford, W. D., Driscoll, S., Lettieri, K., Rowe, H. M., Bonanomi, D., et al. (2012). Embryonic stem cell potency fluctuates with endogenous retrovirus activity. Nature 487, 57–63. doi:10.1038/nature11244
Maksakova, I. A., Thompson, P. J., Goyal, P., Jones, S. J., Singh, P. B., Karimi, M. M., et al. (2013). Distinct roles of KAP1, HP1 and G9a/GLP in silencing of the two-cell-specific retrotransposon MERVL in mouse ES cells. Epigenetics Chromatin 6, 15. doi:10.1186/1756-8935-6-15
Malik, V., and Wang, J. (2022). Pursuing totipotency: Authentic totipotent stem cells in culture. Trends Genet. 38, 632–636. doi:10.1016/j.tig.2022.03.012
Matoba, S., Liu, Y., Lu, F., Iwabuchi, K. A., Shen, L., Inoue, A., et al. (2014). Embryonic development following somatic cell nuclear transfer impeded by persisting histone methylation. Cell 159, 884–895. doi:10.1016/j.cell.2014.09.055
Mazid, Md. A., Ward, C., Luo, Z., Liu, C., Li, Y., Lai, Y., et al. (2022). Rolling back human pluripotent stem cells to an eight-cell embryo-like stage. Nature 605, 315–324. doi:10.1038/s41586-022-04625-0
Merkenschlager, M., and Nora, E. P. (2016). CTCF and Cohesin in genome folding and transcriptional gene regulation. Annu. Rev. Genomics Hum. Genet. 17, 17–43. doi:10.1146/annurev-genom-083115-022339
Nacerddine, K., Lehembre, F., Bhaumik, M., Artus, J., Cohen-Tannoudji, M., Babinet, C., et al. (2005). The SUMO pathway is essential for nuclear integrity and chromosome segregation in mice. Dev. Cell 9, 769–779. doi:10.1016/j.devcel.2005.10.007
Nagano, T., Lubling, Y., Várnai, C., Dudley, C., Leung, W., Baran, Y., et al. (2017). Cell-cycle dynamics of chromosomal organization at single-cell resolution. Nature 547, 61–67. doi:10.1038/nature23001
Nakaki, F., and Saitou, M. (2014). PRDM14: A unique regulator for pluripotency and epigenetic reprogramming. Trends biochem. Sci. 39, 289–298. doi:10.1016/j.tibs.2014.04.003
Nakatani, T., Lin, J., Ji, F., Ettinger, A., Pontabry, J., Tokoro, M., et al. (2022). DNA replication fork speed underlies cell fate changes and promotes reprogramming. Nat. Genet. 54, 318–327. doi:10.1038/s41588-022-01023-0
Napolitano, G., Tagliaferri, D., Fusco, S., Cirillo, C., De Martino, I., Addeo, M., et al. (2020). A novel member of Prame family, Gm12794c, counteracts retinoic acid differentiation through the methyltransferase activity of PRC2. Cell Death Differ. 27, 345–362. doi:10.1038/s41418-019-0359-9
Olbrich, T., Vega-Sendino, M., Tillo, D., Wu, W., Zolnerowich, N., Pavani, R., et al. (2021). CTCF is a barrier for 2C-like reprogramming. Nat. Commun. 12, 4856. doi:10.1038/s41467-021-25072-x
Padeken, J., and Heun, P. (2014). Nucleolus and nuclear periphery: Velcro for heterochromatin. Curr. Opin. Cell Biol. 28, 54–60. doi:10.1016/j.ceb.2014.03.001
Palmerola, K. L., Amrane, S., De Los Angeles, A., Xu, S., Wang, N., de Pinho, J., et al. (2022). Replication stress impairs chromosome segregation and preimplantation development in human embryos. Cell 185, 2988–3007. e20. doi:10.1016/j.cell.2022.06.028
Peaston, A. E., Evsikov, A. V., Graber, J. H., de Vries, W. N., Holbrook, A. E., Solter, D., et al. (2004). Retrotransposons regulate host genes in mouse oocytes and preimplantation embryos. Dev. Cell 7, 597–606. doi:10.1016/j.devcel.2004.09.004
Percharde, M., Lin, C.-J., Yin, Y., Guan, J., Peixoto, G. A., Bulut-Karslioglu, A., et al. (2018). A LINE1-nucleolin partnership regulates early development and ESC identity. Cell 174, 391–405. e19. doi:10.1016/j.cell.2018.05.043
Posfai, E., Schell, J. P., Janiszewski, A., Rovic, I., Murray, A., Bradshaw, B., et al. (2021). Evaluating totipotency using criteria of increasing stringency. Nat. Cell Biol. 23, 49–60. doi:10.1038/s41556-020-00609-2
Probst, A. V., Dunleavy, E., and Almouzni, G. (2009). Epigenetic inheritance during the cell cycle. Nat. Rev. Mol. Cell Biol. 10, 192–206. doi:10.1038/nrm2640
Qiu, Q., Hu, P., Qiu, X., Govek, K. W., Cámara, P. G., and Wu, H. (2020). Massively parallel and time-resolved RNA sequencing in single cells with scNT-seq. Nat. Methods 17, 991–1001. doi:10.1038/s41592-020-0935-4
Redo, A., and Brickman, J. M. (2020). From pluripotency to totipotency: An experimentalist’s guide to cellular potency. Development 11, dev189845. doi:10.1242/dev.189845
Rodriguez-Terrones, D., Gaume, X., Ishiuchi, T., Weiss, A., Kopp, A., Kruse, K., et al. (2018). A molecular roadmap for the emergence of early-embryonic-like cells in culture. Nat. Genet. 50, 106–119. doi:10.1038/s41588-017-0016-5
Rodriguez-Terrones, D., Hartleben, G., Gaume, X., Eid, A., Guthmann, M., Iturbide, A., et al. (2020). A distinct metabolic state arises during the emergence of 2-cell-like cells. EMBO Rep. 21, e48354. doi:10.15252/embr.201948354
Rowe, H. M., Jakobsson, J., Mesnard, D., Rougemont, J., Reynard, S., Aktas, T., et al. (2010). KAP1 controls endogenous retroviruses in embryonic stem cells. Nature 463, 237–240. doi:10.1038/nature08674
Ruebel, M. L., Vincent, K. A., Schall, P. Z., Wang, K., and Latham, K. E. (2019). SMCHD1 terminates the first embryonic genome activation event in mouse two-cell embryos and contributes to a transcriptionally repressive state. Am. J. Physiol. Cell Physiol. 317, C655–C664. doi:10.1152/ajpcell.00116.2019
Sakashita, A., Kitano, T., Ishizu, H., Guo, Y., Masuda, H., Ariura, M., et al. (2022). Transcription of murine endogenous retrovirus MERVL is required for progression of development in early preimplantation embryos. Developmental Biology. Available at: https://www.biorxiv.org/content/10.1101/2022.07.20.500739v1.full.pdf (Accessed July 21, 2022).doi:10.1101/2022.07.20.500739
Schüle, K. M., Leichsenring, M., Andreani, T., Vastolo, V., Mallick, M., Musheev, M. U., et al. (2019). GADD45 promotes locus-specific DNA demethylation and 2C cycling in embryonic stem cells. Genes Dev. 33, 782–798. doi:10.1101/gad.325696.119
Sha, Q.-Q., Zhang, J., and Fan, H.-Y. (2019). A story of birth and death: mRNA translation and clearance at the onset of maternal-to-zygotic transition in mammals. Biol. Reprod. 101, 579–590. doi:10.1093/biolre/ioz012
Sharova, L. V., Sharov, A. A., Piao, Y., Stagg, C. A., Amano, T., Qian, Y., et al. (2016). Emergence of undifferentiated colonies from mouse embryonic stem cells undergoing differentiation by retinoic acid treatment. Vitro Cell. Dev. Biol. Anim. 52, 616–624. doi:10.1007/s11626-016-0013-5
Sheban, D., Shani, T., Maor, R., Aguilera-Castrejon, A., Mor, N., Oldak, B., et al. (2022). SUMOylation of linker histone H1 drives chromatin condensation and restriction of embryonic cell fate identity. Mol. Cell 82, 106–122. e9. doi:10.1016/j.molcel.2021.11.011
Shen, H., Yang, M., Li, S., Zhang, J., Peng, B., Wang, C., et al. (2021). Mouse totipotent stem cells captured and maintained through spliceosomal repression. Cell 184, 2843–2859. e20. doi:10.1016/j.cell.2021.04.020
Slotkin, R. K., and Martienssen, R. (2007). Transposable elements and the epigenetic regulation of the genome. Nat. Rev. Genet. 8, 272–285. doi:10.1038/nrg2072
Smith, Z. D., Chan, M. M., Mikkelsen, T. S., Gu, H., Gnirke, A., Regev, A., et al. (2012). A unique regulatory phase of DNA methylation in the early mammalian embryo. Nature 484, 339–344. doi:10.1038/nature10960
Song, Y., Liang, Z., Zhang, J., Hu, G., Wang, J., Li, Y., et al. (2022). CTCF functions as an insulator for somatic genes and a chromatin remodeler for pluripotency genes during reprogramming. Cell Rep. 39, 110626. doi:10.1016/j.celrep.2022.110626
Srinivasan, R., Nady, N., Arora, N., Hsieh, L. J., Swigut, T., Narlikar, G. J., et al. (2020). Zscan4 binds nucleosomal microsatellite DNA and protects mouse two-cell embryos from DNA damage. Sci. Adv. 6, eaaz9115. doi:10.1126/sciadv.aaz9115
Sugie, K., Funaya, S., Kawamura, M., Nakamura, T., Suzuki, M. G., and Aoki, F. (2020). Expression of Dux family genes in early preimplantation embryos. Sci. Rep. 10, 19396. doi:10.1038/s41598-020-76538-9
Sun, Z., Yu, H., Zhao, J., Tan, T., Pan, H., Zhu, Y., et al. (2022). LIN28 coordinately promotes nucleolar/ribosomal functions and represses the 2C-like transcriptional program in pluripotent stem cells. Protein Cell 13, 490–512. doi:10.1007/s13238-021-00864-5
Svoboda, P. (2017). Long and small noncoding RNAs during oocyte-to-embryo transition in mammals. Biochem. Soc. Trans. 45, 1117–1124. doi:10.1042/BST20170033
Tagliaferri, D., Mazzone, P., Noviello, T. M. R., Addeo, M., Angrisano, T., Del Vecchio, L., et al. (2020). Retinoic acid induces embryonic stem cells (ESCs) transition to 2 cell-like state through a coordinated expression of Dux and Duxbl1. Front. Cell Dev. Biol. 7, 385. doi:10.3389/fcell.2019.00385
Tarkowski, A. K. (1959). Experiments on the development of isolated blastomers of mouse eggs. Nature 184, 1286–1287. doi:10.1038/1841286a0
Taubenschmid-Stowers, J., Rostovskaya, M., Santos, F., Ljung, S., Argelaguet, R., Krueger, F., et al. (2022). 8C-like cells capture the human zygotic genome activation program in vitro. Cell Stem Cell 29, 449–459. e6. doi:10.1016/j.stem.2022.01.014
Tercero, J. A., and Diffley, J. F. (2001). Regulation of DNA replication fork progression through damaged DNA by the Mec1/Rad53 checkpoint. Nature 412, 553–557. doi:10.1038/35087607
Theurillat, I., Hendriks, I. A., Cossec, J.-C., Andrieux, A., Nielsen, M. L., and Dejean, A. (2020). Extensive SUMO modification of repressive chromatin factors distinguishes pluripotent from somatic cells. Cell Rep. 32, 108146. doi:10.1016/j.celrep.2020.108146
Thompson, P. J., Dulberg, V., Moon, K.-M., Foster, L. J., Chen, C., Karimi, M. M., et al. (2015). hnRNP K coordinates transcriptional silencing by SETDB1 in embryonic stem cells. PLoS Genet. 11, e1004933. doi:10.1371/journal.pgen.1004933
Tian, Q., Wang, X., Xie, S., Yin, Y., and Zhou, L. (2020). H3.3 impedes zygotic transcriptional program activated by Dux. Biochem. Biophys. Res. Commun. 522, 422–427. doi:10.1016/j.bbrc.2019.11.114
Walter, M., Teissandier, A., Pérez-Palacios, R., and Bourc’his, D. (2016). An epigenetic switch ensures transposon repression upon dynamic loss of DNA methylation in embryonic stem cells. Elife 5, e11418. doi:10.7554/eLife.11418
Wang, C., and Goff, S. P. (2017). Differential control of retrovirus silencing in embryonic cells by proteasomal regulation of the ZFP809 retroviral repressor. Proc. Natl. Acad. Sci. U. S. A. 114, E922–E930. doi:10.1073/pnas.1620879114
Wang, E. T., Sandberg, R., Luo, S., Khrebtukova, I., Zhang, L., Mayr, C., et al. (2008). Alternative isoform regulation in human tissue transcriptomes. Nature 456, 470–476. doi:10.1038/nature07509
Wang, Y., Na, Q., Li, X., Tee, W.-W., Wu, B., and Bao, S. (2021). Retinoic acid induces NELFA-mediated 2C-like state of mouse embryonic stem cells associates with epigenetic modifications and metabolic processes in chemically defined media. Cell Prolif. 54, e13049. doi:10.1111/cpr.13049
Wen, X., Lin, Z., Wu, H., and Fu, X. (2022). Zfp281 inhibits the pluripotent-to-totipotent state transition in mouse embryonic stem cells. Cell Biol. 10, 879428. doi:10.1101/2022.03.14.484036
Whiddon, J. L., Langford, A. T., Wong, C.-J., Zhong, J. W., and Tapscott, S. J. (2017). Conservation and innovation in the DUX4-family gene network. Nat. Genet. 49, 935–940. doi:10.1038/ng.3846
Wolf, G., Yang, P., Füchtbauer, A. C., Füchtbauer, E.-M., Silva, A. M., Park, C., et al. (2015). The KRAB zinc finger protein ZFP809 is required to initiate epigenetic silencing of endogenous retroviruses. Genes Dev. 29, 538–554. doi:10.1101/gad.252767.114
Wossidlo, M., Arand, J., Sebastiano, V., Lepikhov, K., Boiani, M., Reinhardt, R., et al. (2010). Dynamic link of DNA demethylation, DNA strand breaks and repair in mouse zygotes. EMBO J. 29, 1877–1888. doi:10.1038/emboj.2010.80
Wu, K., Liu, H., Wang, Y., He, J., Xu, S., Chen, Y., et al. (2020). SETDB1-Mediated cell fate transition between 2C-like and pluripotent states. Cell Rep. 30, 25–36. e6. doi:10.1016/j.celrep.2019.12.010
Xia, W., Xu, J., Yu, G., Yao, G., Xu, K., Ma, X., et al. (2019). Resetting histone modifications during human parental-to-zygotic transition. Science 365, 353–360. doi:10.1126/science.aaw5118
Xie, S. Q., Leeke, B. J., Whilding, C., Wagner, R. T., Garcia-Llagostera, F., Low, Y., et al. (2022). Nucleolar-based Dux repression is essential for embryonic two-cell stage exit. Genes Dev. 36, 331–347. doi:10.1101/gad.349172.121
Xu, R., Li, C., Liu, X., and Gao, S. (2021). Insights into epigenetic patterns in mammalian early embryos. Protein Cell 12, 7–28. doi:10.1007/s13238-020-00757-z
Xu, R., Li, S., Wu, Q., Li, C., Jiang, M., Guo, L., et al. (2022a). Stage-specific H3K9me3 occupancy ensures retrotransposon silencing in human pre-implantation embryos. Cell Stem Cell 29, 1051–1066.e8. e8. doi:10.1016/j.stem.2022.06.001
Xu, Y., Zhao, J., Ren, Y., Wang, X., Lyu, Y., Xie, B., et al. (2022b). Derivation of totipotent-like stem cells with blastocyst-like structure forming potential. Cell Res. 32, 513–529. doi:10.1038/s41422-022-00668-0
Yan, Y.-L., Zhang, C., Hao, J., Wang, X.-L., Ming, J., Mi, L., et al. (2019). DPPA2/4 and SUMO E3 ligase PIAS4 opposingly regulate zygotic transcriptional program. PLoS Biol. 17, e3000324. doi:10.1371/journal.pbio.3000324
Yang, B. X., El Farran, C. A., Guo, H. C., Yu, T., Fang, H. T., Wang, H. F., et al. (2015). Systematic identification of factors for provirus silencing in embryonic stem cells. Cell 163, 230–245. doi:10.1016/j.cell.2015.08.037
Yang, F., Huang, X., Zang, R., Chen, J., Fidalgo, M., Sanchez-Priego, C., et al. (2020). DUX-miR-344-ZMYM2-Mediated activation of MERVL LTRs induces a totipotent 2C-like state. Cell Stem Cell 26, 234–250. e7. doi:10.1016/j.stem.2020.01.004
Yang, G., Zhang, L., Liu, W., Qiao, Z., Shen, S., Zhu, Q., et al. (2021). Dux-mediated corrections of aberrant H3K9ac during 2-cell genome activation optimize efficiency of somatic cell nuclear transfer. Cell Stem Cell 28, 150–163. e5. doi:10.1016/j.stem.2020.09.006
Yang, J., Ryan, D. J., Wang, W., Tsang, J. C.-H., Lan, G., Masaki, H., et al. (2017a). Establishment of mouse expanded potential stem cells. Nature 550, 393–397. doi:10.1038/nature24052
Yang, M., Yu, H., Yu, X., Liang, S., Hu, Y., Luo, Y., et al. (2022). Chemical-induced chromatin remodeling reprograms mouse ESCs to totipotent-like stem cells. Cell Stem Cell 29, 400–418. e13. doi:10.1016/j.stem.2022.01.010
Yang, Y., Liu, B., Xu, J., Wang, J., Wu, J., Shi, C., et al. (2017b). Derivation of pluripotent stem cells with in vivo embryonic and extraembryonic potency. Cell 169, 243–257. e25. doi:10.1016/j.cell.2017.02.005
Yu, H., Chen, M., Hu, Y., Ou, S., Yu, X., Liang, S., et al. (2022). Dynamic reprogramming of H3K9me3 at hominoid-specific retrotransposons during human preimplantation development. Cell Stem Cell 29, 1031–1050. e12. doi:10.1016/j.stem.2022.06.006
Yu, H., Sun, Z., Tan, T., Pan, H., Zhao, J., Zhang, L., et al. (2021). rRNA biogenesis regulates mouse 2C-like state by 3D structure reorganization of peri-nucleolar heterochromatin. Nat. Commun. 12, 6365. doi:10.1038/s41467-021-26576-2
Zhang, H., Emerson, D. J., Gilgenast, T. G., Titus, K. R., Lan, Y., Huang, P., et al. (2019a). Chromatin structure dynamics during the mitosis-to-G1 phase transition. Nature 576, 158–162. doi:10.1038/s41586-019-1778-y
Zhang, S.-M., Cai, W. L., Liu, X., Thakral, D., Luo, J., Chan, L. H., et al. (2021a). KDM5B promotes immune evasion by recruiting SETDB1 to silence retroelements. Nature 598, 682–687. doi:10.1038/s41586-021-03994-2
Zhang, W., Chen, F., Chen, R., Xie, D., Yang, J., Zhao, X., et al. (2019b). Zscan4c activates endogenous retrovirus MERVL and cleavage embryo genes. Nucleic Acids Res. 47, 8485–8501. gkz594. doi:10.1093/nar/gkz594
Zhang, Y., Huang, Y., Dong, Y., Liu, X., Kou, X., Zhao, Y., et al. (2021b). Unique patterns of H3K4me3 and H3K27me3 in 2-Cell-like embryonic stem cells. Stem Cell Rep. 16, 458–469. doi:10.1016/j.stemcr.2021.01.020
Zhao, J., Yao, K., Yu, H., Zhang, L., Xu, Y., Chen, L., et al. (2021). Metabolic remodelling during early mouse embryo development. Nat. Metab. 3, 1372–1384. doi:10.1038/s42255-021-00464-x
Zheng, H., Huang, B., Zhang, B., Xiang, Y., Du, Z., Xu, Q., et al. (2016). Resetting epigenetic memory by reprogramming of histone modifications in mammals. Mol. Cell 63, 1066–1079. doi:10.1016/j.molcel.2016.08.032
Zheng, H., and Xie, W. (2019). The role of 3D genome organization in development and cell differentiation. Nat. Rev. Mol. Cell Biol. 20, 535–550. doi:10.1038/s41580-019-0132-4
Zhu, Y., Cheng, C., Chen, L., Zhang, L., Pan, H., Hou, L., et al. (2021a). Cell cycle heterogeneity directs spontaneous 2C state entry and exit in mouse embryonic stem cells. Stem Cell Rep. 16, 2659–2673. doi:10.1016/j.stemcr.2021.09.003
Zhu, Y., Yu, J., Gu, J., Xue, C., Zhang, L., Chen, J., et al. (2021b). Relaxed 3D genome conformation facilitates the pluripotent to totipotent-like state transition in embryonic stem cells. Nucleic Acids Res. 49, 12167–12177. doi:10.1093/nar/gkab1069
Ziegler-Birling, C., Helmrich, A., Tora, L., and Torres-Padilla, M.-E. (2009). Distribution of p53 binding protein 1 (53BP1) and phosphorylated H2A.X during mouse preimplantation development in the absence of DNA damage. Int. J. Dev. Biol. 53, 1003–1011. doi:10.1387/ijdb.082707cz
Keywords: embryonic stem cell, totipotency, transcription regulation, epigenetic regulation, developmental potential
Citation: Xu H and Liang H (2022) The regulation of totipotency transcription: Perspective from in vitro and in vivo totipotency. Front. Cell Dev. Biol. 10:1024093. doi: 10.3389/fcell.2022.1024093
Received: 21 August 2022; Accepted: 13 October 2022;
Published: 26 October 2022.
Edited by:
Qiang Wu, Macau University of Science and Technology, Macao, SAR ChinaCopyright © 2022 Xu and Liang. This is an open-access article distributed under the terms of the Creative Commons Attribution License (CC BY). The use, distribution or reproduction in other forums is permitted, provided the original author(s) and the copyright owner(s) are credited and that the original publication in this journal is cited, in accordance with accepted academic practice. No use, distribution or reproduction is permitted which does not comply with these terms.
*Correspondence: Hongqing Liang, bGlhbmdob25ncWluZ0B6anUuZWR1LmNu