- 1Department of Otolaryngology, Hospital Universitario Central de Asturias and Instituto de Investigación Sanitaria Del Principado de Asturias (ISPA), Instituto Universitario de Oncología Del Principado de Asturias (IUOPA), University of Oviedo, Oviedo, Spain
- 2CIBERONC, Instituto de Salud Carlos III, Madrid, Spain
Annexins are an extensive superfamily of structurally related calcium- and phospholipid-binding proteins, largely conserved and widely distributed among species. Twelve human annexins have been identified, referred to as Annexin A1-13 (A12 remains as of yet unassigned), whose genes are spread throughout the genome on eight different chromosomes. According to their distinct tissue distribution and subcellular localization, annexins have been functionally implicated in a variety of biological processes relevant to both physiological and pathological conditions. Dysregulation of annexin expression patterns and functions has been revealed as a common feature in multiple cancers, thereby emerging as potential biomarkers and molecular targets for clinical application. Nevertheless, translation of this knowledge to the clinic requires in-depth functional and mechanistic characterization of dysregulated annexins for each individual cancer type, since each protein exhibits varying expression levels and phenotypic specificity depending on the tumor types. This review specifically and thoroughly examines the current knowledge on annexin dysfunctions in carcinogenesis. Hence, available data on expression levels, mechanism of action and pathophysiological effects of Annexin A1-13 among different cancers will be dissected, also further discussing future perspectives for potential applications as biomarkers for early diagnosis, prognosis and molecular-targeted therapies. Special attention is devoted to head and neck cancers (HNC), a complex and heterogeneous group of aggressive malignancies, often lately diagnosed, with high mortality, and scarce therapeutic options.
Introduction
Annexins are an extensive multigene superfamily of proteins that possess high structural and biological homology (40–60%) (Gerke and Moss, 2002; Guo et al., 2013b) and whose main biochemical property is the binding or “annexing” to phospholipid membranes in a Ca2+-dependent manner (Moss and Morgan, 2004; Lim and Pervaiz, 2007). There are more than 500 annexins described in different species, which are widely distributed among eukaryotes, but largely absent in prokaryotes and yeasts (Fernandez and Morgan, 2003). The high evolutionary conservation of annexins among species and their presence in all higher eukaryotic organisms suggest an indispensable role in cell biology (Moss and Morgan, 2004). In humans, there are twelve annexins described (Figure 1), conventionally referred to as Annexin A1-13 (the ANXA12 gene is unassigned) (Morgan et al., 1999a), whose genes are spread throughout the genome on chromosomes 1, 2, 4, 5, 8, 9, 10 and 15 (Table 1) (Moss and Morgan, 2004).
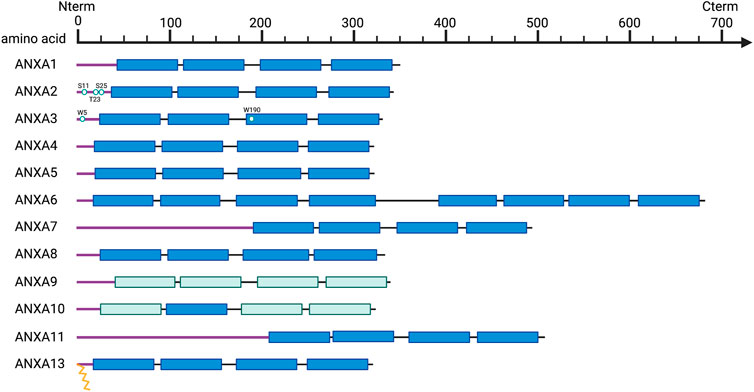
FIGURE 1. Schematic overview of annexin structural organization. Purple line, N-terminal tails; Dark blue, C-terminal core domains including four annexin repeats (duplicated to eight in ANXA6); Light blue, annexin repeats harboring non-functional type II calcium binding sites; Key residues are indicated and represented as spheres, and myristoylation as yellow zig-zag lines.
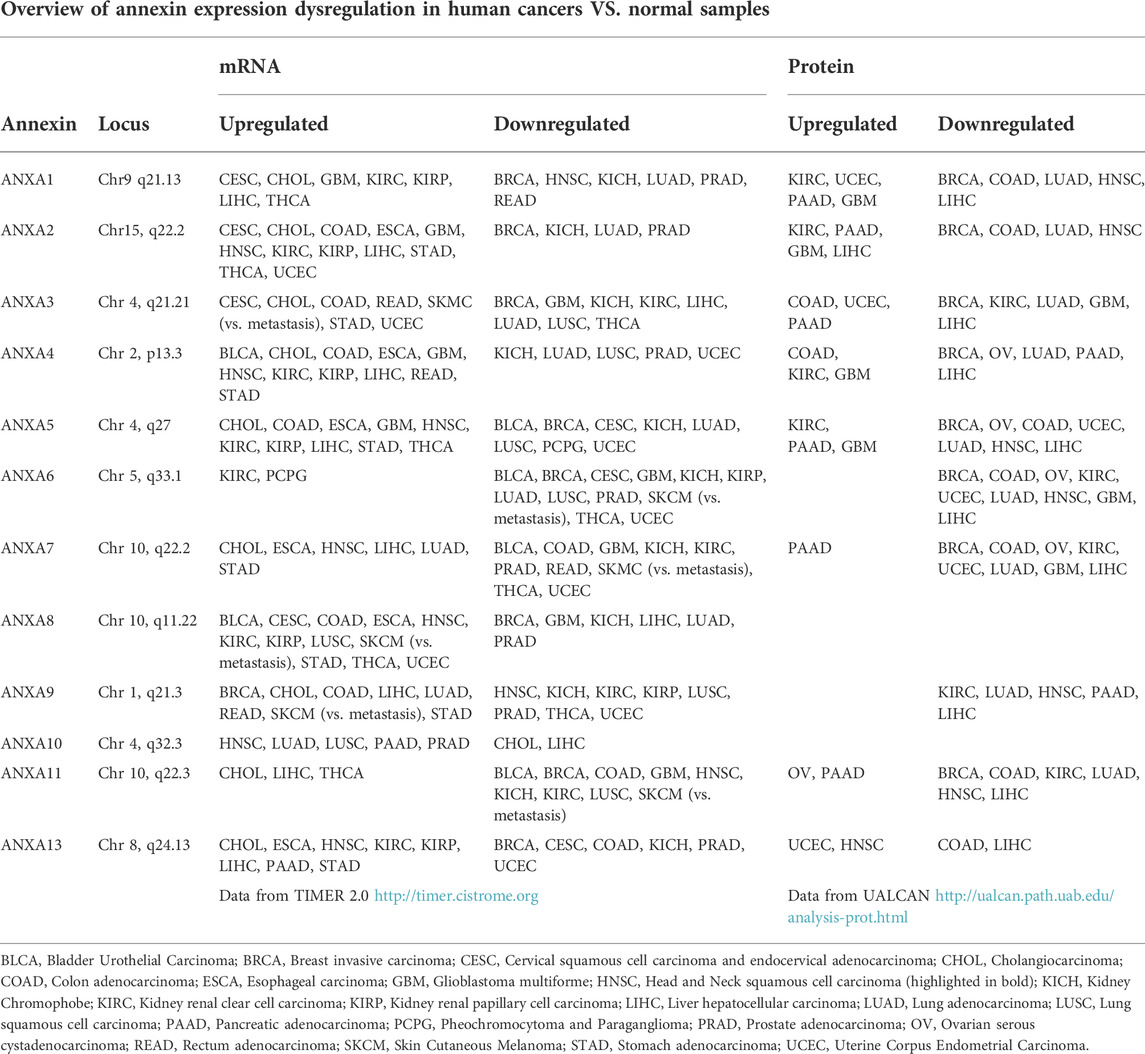
TABLE 1. Overview of annexin expression dysregulation in human cancers vs. normal samples (unless stated otherwise). Transcriptomic and protein data was obtained from TCGA and CPTAC, respectively. TIMER 2.0 (http://timer.cistrome.org/) was used to asset differences in expression level whereas UALCAN webtool (http://ualcan.path.uab.edu/) was used for protein levels.
Structurally, annexins are characterized by a highly conserved C-terminal core domain composed of at least four conserved structural repeats (each one of 70 amino acids long), where the calcium and phospholipids binding domains are located (Figure 1) (Mirsaeidi et al., 2016). In addition, each annexin has a unique N-terminal domain with a variable length and amino acid sequence, involved in protein-protein interactions and responsible for their biological and functional specificity (Figure 1) (Gerke and Moss, 2002; Mirsaeidi et al., 2016). This variable N-terminal region contains binding sites for multiple protein partners, including members of the calcium binding S100 family (Rescher and Gerke, 2008; Rintala-Dempsey et al., 2008), and also for various kinases related to signaling pathways, such as the proto-oncogene tyrosine-kinase Src and the calcium-controlled serine threonine kinase PKC (Kheifets et al., 2006; Hayes and Moss, 2009).
Annexins are usually cytosolic and soluble proteins with a stable form, but also detectable in the nucleus and the cell surface (Mirsaeidi et al., 2016). In response to specific stimuli, these proteins could be translocated through various types of intracellular membranes, and transported to the cell exterior via an endoplasmic reticulum/Golgi-dependent pathway (Boudhraa et al., 2016).
Regarding their functions, annexins have been implicated in a large variety of biological processes, and in the regulation of several cellular membrane functions, such as cell adhesion and morphology, vesicle organization, endo- and exocytosis, membrane trafficking and scaffolding, maintaining membrane stability under stress conditions or regulation of cytoskeleton dynamics (Hayes et al., 2004; Rescher and Gerke, 2004; Draeger et al., 2011; Schloer et al., 2018). Accordingly, these biochemical properties make annexins perfect candidates to transduce the extracellular stimulus across the membrane into the activation of intracellular signaling pathways to trigger multiple cellular responses such as proliferation, apoptosis, inflammatory activity, angiogenesis, immune response regulation, cell differentiation and also cell motility and invasion (Gerke and Moss, 2002; Ling et al., 2004; Schloer et al., 2018).
The expression levels and tissue distribution vary widely in both physiological and pathological conditions. Thus, some annexins such as Annexins A1, A2, A3, A4, A5, A6, A7 and A11 exhibit an ubiquitous expression, whereas others show very restrictive expression patterns, such as Annexin A8 in placenta and skin, Annexin A10 in stomach and Annexin A13 in small intestine (Fernandez and Morgan, 2003) (Table 2). Although direct evidence for a causative role of annexins in human diseases has not yet been demonstrated, several pathologies such as diabetes, cardiovascular and autoimmune diseases, infection and cancer have been associated with annexin dysfunctions, so termed “annexinopathies” (Rand, 1999; Hayes et al., 2007).
Growing evidences have revealed that annexins are frequently and commonly dysregulated in multiple cancers, including HNC. As summarized in Table 1, altered expression levels of each annexin (both mRNA and protein) are frequently detected either upregulated or downregulated depending on the cancer type. Not surprisingly, annexin expression changes cause widespread functional effects on multiple biological and cellular processes including various cancer hallmarks (Table 2). On this basis, several annexins have emerged as potential biomarkers for cancer diagnosis, prognosis, disease monitoring, prediction of treatment response and/or therapeutic targets (Table 3).
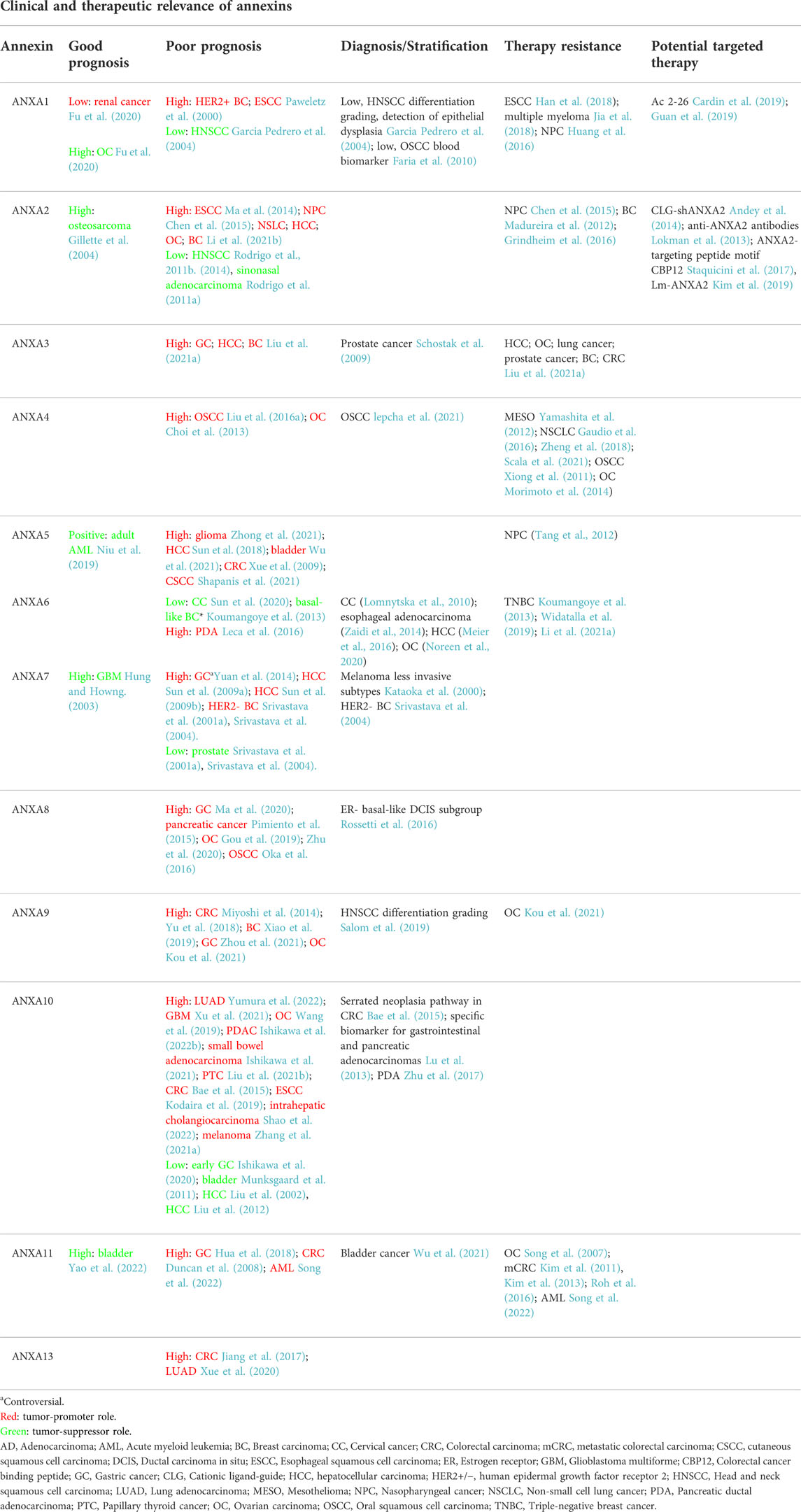
TABLE 3. Clinical significance and potential therapeutic implications of annexin dysregulation in human cancers.
Head and neck cancers (HNC) represent the seventh most common cancer worldwide, comprising a highly diverse and heterogeneous group of malignancies (Mody et al., 2021). Most head and neck malignancies are diagnosed at a late stage due to the scarcity of specific symptoms. Mortality remains high mainly related to locoregional recurrences and second primary tumors (Leemans et al., 2011). Despite continuous advancements in the different treatment modalities (surgery, radio- and chemotherapy), survival rates for HNSCC patients have not been substantially improved in recent decades. At present, there are only few molecular-targeted therapies approved by the FDA: cetuximab (anti-EGFR) (Vermorken et al., 2007), nivolumab and pembrolizumab (anti-PD-1/PD-L1) (Cramer et al., 2019); however, these treatments only benefit 20–30% of patients (Cohen et al., 2019). Hence, there is an urgent need for novel therapeutic targets and more accurate prognosticators and early diagnosis markers that augment the limited predictability of the current clinicopathologic criteria. This review thoroughly and critically examines current knowledge and reported data on annexin dysregulation in human cancers with a special focus on HNC pathogenesis. An overview of all the annexins aberrantly expressed in HNC (either upregulated or downregulated) compared to the levels in normal tissue is depicted in Figure 2 (both mRNA and protein). In the following subsections, available data on expression dysregulation of Annexin A1-13 and the clinical and pathobiological relevance of each protein will be jointly reviewed, also further discussing future perspectives for their potential applications as biomarkers for early diagnosis, prognosis and molecular-targeted therapies.
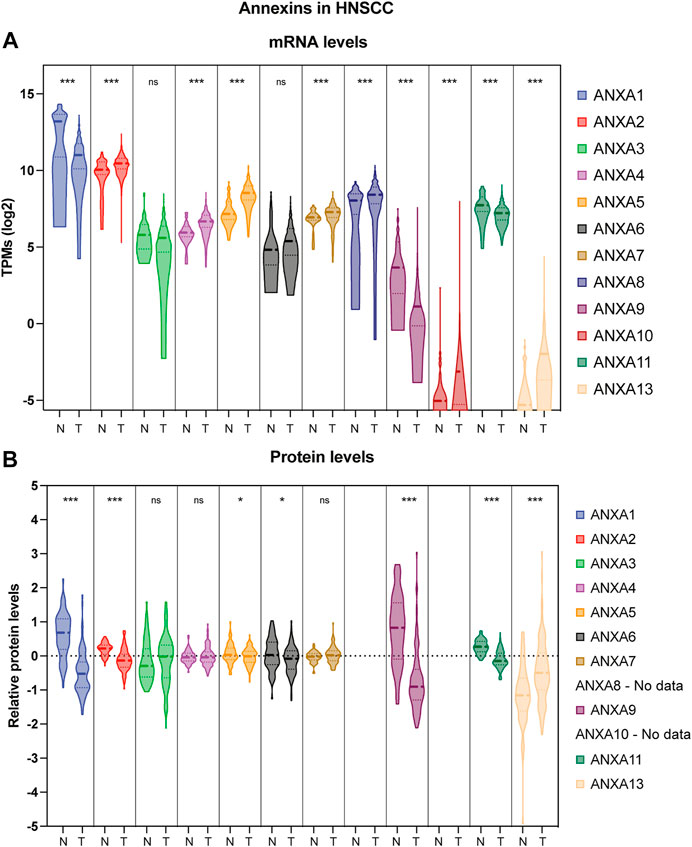
FIGURE 2. Violin plot comparison of annexin expression levels in HNSCC patient samples versus normal adjacent tissue. (A) Transcriptomic expression data were obtained at Xena repository (Goldman et al., 2020) from the TCGA-HNSCC cohort consisting of 44 normal adjacent tissue (N) and 522 primary tumors (T). Transcript per million (TPMs) are shown as log2 transformed (***p < 0.001 by t-test using Welch’s correction; ns, not significant). (B) Proteomic expression data from 72 normal adjacent tissue (N) and 110 primary tumors (T) were obtained from Proteomic Data Commons (https://pdc.cancer.gov/pdc/study/PDC000221). Ion intensity is shown as log2 transformed (***p < 0.001, *p < 0.05 by t-test using Welch’s correction; ns, not significant).
Annexin A1
Structural and functional characteristics
Annexin A1 (ANXA1) was first described in the 1970s, characterized as the first member of the annexin superfamily, and called macrocortin, renocortin and lipomodulin, lipocortin-1 and lastly named Annexin A1. The ANXA1 gene maps in the chromosomal region 9q12-q21.2 and encodes a 37-kDa protein, whose structural features are similar to those of the annexin superfamily members, and with a variable N-terminal regulatory region that contains sites of phosphorylation, glycosylation, acetylation and proteolysis, conferring as such specific biological properties (Buckingham et al., 2006; Guo et al., 2013b). ANXA1 can be found in alternatively spliced isoforms, as proteolytic fragments of its bioactive N-terminus, interacting with different ligands, and hence could be localized in the nucleus, cytoplasm, membrane or extracellular matrix (Boudhraa et al., 2016). ANXA1 was first identified as an endogenous mediator of the anti-inflammatory effects of the glucocorticoids. Initially, ANXA1 was studied in neutrophils, eosinophils and monocytes where it is highly expressed (Perretti et al., 1996; Hannon et al., 2003; Perretti and Solito, 2004), and thereafter, it was widely detected in different tissues and involved in multiple cell processes, including cell survival, proliferation, apoptosis, differentiation and migration (Lim and Pervaiz, 2007).
ANXA1 is well-known by its participation in the inhibition of glucocorticoid-induced eicosanoid and phospholipase A2 (PLA2) synthesis (Raynal and Pollard, 1994; Lim and Pervaiz, 2007). ANXA1 is considered a mediator of the anti-inflammatory, immunosuppressive and antipyretic action of glucocorticoids, thereby regulating their expression and secretion (Davidson et al., 1991; Gobbetti and Cooray, 2016). It has also been reported that ANXA1 may act as an endogenous inhibitor of NFκB, inducible in response to anti-inflammatory agents (Zhang et al., 2010). Moreover, ANXA1 is a major substrate for numerous kinases, such as epidermal growth factor receptor (EGFR), hepatocyte growth factor receptor (HGFR/MET), platelet-derived growth factor receptor (PDGFR) and protein kinase C (PKC) (Lim and Pervaiz, 2007). These phosphorylation events lead to the activation of several signaling pathways (e.g., ERK-MAPK pathway), regulating cell proliferation and differentiation (Alldridge and Bryant, 2003). Seemingly, ANXA1 may create varying regulatory signals on different pathways that could explain its dual role, either promoting or inhibiting cell proliferation, as well as distinct functions and phenotypes depending on the cellular and tissue context.
ANXA1 may induce apoptosis through calcium release and BAD proteins dephosphorylation, allowing BAD association to mitochondria (Solito et al., 2003). During apoptosis, ANXA1 itself translocate to the nucleus, which can be inhibited by Bcl-2. ANXA1 can also regulate apoptosis through TRAIL (Petrella et al., 2005). By contrast, some groups have reported that ANXA1 exhibits anti-apoptotic properties, probably because its role depends on the cell type or cellular differentiation status. ANXA1 is also able to mediate the phagocytosis of apoptotic cells when it is recruited to the cell surface, where it binds to phosphatidylserine (PS) (Dalli et al., 2012).
ANXA1 has also been involved in motility and invasion processes, since mice with ANXA1 overexpression exhibited a significantly higher wound closure ability, and conversely, ANXA1 inhibition led to a reduction in the wound healing ability (Yi and Schnitzer, 2009). It has been described that the participation of ANXA1 in would healing is mediated by different signaling pathways such as MAPK, Rho-GTPases and NFκB (Bist et al., 2015).
Besides, special attention has been focused on studying the functional roles in membrane remodeling, cell adhesion, migration and cell signaling through the formyl peptide receptor (FPR), known receptors for externalized ANXA1 (Cooray et al., 2013; Gastardelo et al., 2014). FPRs may cause potent and opposite effects depending on the ligand (Cooray et al., 2013), and ANXA1, having the strongest affinity for FPR2, triggers different regulatory signaling pathways in a dose-dependent manner (Karlsson et al., 2005). This could plausibly explain how ANXA1 may elicit distinct regulatory signals and pleiotropic functions in different tissues (Boudhraa et al., 2016).
Noteworthy, during the last years, ANXA1 has emerged as an immunomodulatory protein and great effort has been devoted to understand its specific immune-suppressive role during malignant transformation. In physiological conditions, ANXA1 promotes immune suppression to counteract inflammatory process, specifically enhancing the differentiation of macrophages into M2 (Li et al., 2011). In cancer context, ANXA1 regulates macrophages activation by inhibiting the expression and activation of the inducible nitric oxide synthase (iNOS) (Smyth et al., 2006) or regulating nuclear EGFR and EGFR/STAT3 signaling pathway to ultimately create an immunosuppressive environment that facilitates cancer progression (Araújo et al., 2021).
Altered expression and pathobiological role in cancer
ANXA1 dysregulation has been frequently detected in many types of cancer; however, its specific role has not yet been fully deciphered (Fu et al., 2020). ANXA1 has been found overexpressed in gastric cancer (Hippo et al., 2001), pancreatic and hepatocellular carcinoma (Masaki et al., 1996), colorectal cancer (Roth et al., 2010), lung cancer (Rong et al., 2014), melanoma (Rondepierre et al., 2009), skin cancer (Hummerich et al., 2006) and endometrial carcinoma (Voisin et al., 2011). On the other hand, ANXA1 is markedly down-regulated in breast cancer (Anbalagan et al., 2014; Yuan et al., 2016), prostate cancer (Kang et al., 2002), esophageal cancer (Paweletz et al., 2000), cervical cancer (Liu et al., 2011), lymphoma (Santos et al., 2009), hilar cholangiocarcinoma (Wang et al., 2010), intestinal-type sinonasal adenocarcinoma (Rodrigo et al., 2011a) and also in HNSCC (Garcia Pedrero et al., 2004).
The opposite expression levels of ANXA1 in different tumor types makes difficult to understand precisely the role played by ANXA1 during tumorigenesis. Actually, its action shows cellular or tissue specificity that could be due to post-translational modifications, potential site re-processing or epigenetic regulation among others. In fact, the contrasting patterns of ANXA1 expression in different tumor types is just one of the enigmas in deciphering the underlying regulatory mechanisms and phenotypic specificity of ANXA1.
Therapeutic implications
ANXA1 has been related to treatment resistance in several cancers. It has been reported that serum ANXA1 levels increased after chemoradiotherapy in esophageal squamous cell carcinoma (ESCC) patients (Han et al., 2018). ANXA1 knockdown enhances the antitumor effect of bortezomib in multiple myeloma (Jia et al., 2018). In HNSCC and nasopharyngeal cancer (NPC), ANXA1 expression has been correlated with radiation resistance (Suh et al., 2015; Huang et al., 2016). These data underscore that ANXA1 could serve as a novel predictive biomarker of treatment response, and emerge as a potential co-adjuvant treatment to improve chemosensitivity in different types of cancer, including HNSCC.
It has been demonstrated that ANXA1 promotes the switching of macrophages to the protumoral M2 phenotype preventing the induction of cytotoxic T cell response, thus creating an immunosuppressed tumor microenvironment that facilitates tumor progression and dissemination (Araújo et al., 2021).
ANXA1 was the first annexin found to be implicated in the plasma membrane repair response. It is recruited within seconds at the wound region after an injury, demonstrating its function as stress-responsive protein (McNeil et al., 2006). In this context, cancer cells are normally under higher stress levels than normal cells, leading to plasma membrane damage, so ANXA1 could be a promising adjuvant treatment combined with current oncologic treatments to prevent plasma membrane repair in cancer cells after anti-proliferative treatments.
ANXA1 could be therapeutically exploited through its known receptors, FPR1 and FPR2. These receptors are predominantly expressed on the surface of several types of immune cells (macrophages, dendritic cells, neutrophils … ) as well as endothelial and epithelial cells (Rescher et al., 2002). Since ANXA1 expression is closely related to inflammatory processes, the signaling axis ANXA1/FPR could constitute an attractive immunomodulatory target for cancer therapies. ANXA1 regulates apoptosis and clearance of neutrophils and promotes monocytes recruitment during inflammatory events. However, the mechanisms responsible for the immunomodulatory role of ANXA1 have not been completely elucidated. Seemingly, it has been reported different results depending the experimental settings and cellular models tested (D’Acquisto et al., 2008; Yang et al., 2013).
Furthermore, ANXA1 N-terminal mimetic peptide Ac 2–26 has been proposed as a potential therapeutic strategy for cancer treatment, targeting ANXA1-mediated functions and related signaling pathways. As a proof of principle, it has been demonstrated that Ac 2–26 is able to decrease the proliferation of cervical cancer cells, through the activation of the MAPK family and targeting p53 to arrest the cell cycle (Cardin et al., 2019). It has also been showed that Ac 2–26 could be used to treat non-small cell lung cancer (NSCLC), regulating NFkB pathway (Guan et al., 2019). Remarkably, this ANXA1 mimetic has demonstrated cardioprotective actions against in vivo myocardial infarction, thereby effectively reducing cardiac inflammation, fibrosis and apoptosis (Qin et al., 2019). Fredman and coworkers designed nanoparticles coupled to Ac 2–26 to target collagen IV as a treatment for advanced atherosclerotic lesions, which caused a reduced lesion instability in an FPR2-dependent manner (Fredman et al., 2015). Hence, the combination of Ac 2–26 with nanoparticles emerges as a useful and promising therapeutic approach for cancer treatment.
Clinical and biological roles in HNC
A pioneer study led by our research group demonstrated the downregulation of ANXA1 protein expression in HNSCC tissue specimens by both Western blot analysis and immunohistochemistry (Garcia Pedrero et al., 2004). The loss of ANXA1 expression was significantly associated with poor prognostic parameters, i.e., larger tumors, locoregional metastases, poor differentiation, and advanced disease stages. ANXA1 expression in HNSCC was closely related with the tumor differentiation and therefore it emerged as a differentiation marker potentially applicable for histopathological grading. In addition, our results unprecedentedly revealed the clinical utility of ANXA1 for early and accurate detection of epithelial dysplasia, since ANXA1 loss occurred in early tumorigenesis and was detected in all dysplastic precancerous lesions (Garcia Pedrero et al., 2004). We also further contributed to uncover a transcriptional regulatory mechanism underlying ANXA1 downregulation in HNSCC. We thus found that miR-196a/b levels inversely correlated with ANXA1 expression in paired HNSCC tissue samples and that ANXA1 was a direct target of these miRNAs (Álvarez-Teijeiro et al., 2017a; Álvarez-Teijeiro et al., 2017b).
Studies from other groups have confirmed ANXA1 down-regulation in HNSCC, and further contributed to functionally and mechanistically characterize the specific role of ANXA1 in the progression of these tumors. Thus, ANXA1 expression has been linked to the regulation of different cellular processes in HNC thereby affecting multiple hallmarks of cancer (Figure 3). It has been reported that ANXA1 expression inversely correlates with EGFR, and it regulates the intensity and duration of the EGFR-dependent signaling events and the exosome phopho-EGFR release affecting cell-cell communication (Raulf et al., 2018). In NPC, ANXA1 was found to compete with Cbl for binding to EphA2, increasing its stability by inhibiting EphA2 ubiquitination and degradation mediated by Cbl. Consistently, ANXA1 binding to EphA2 increased EphA2 levels and its oncogenic activity, enhancing tumor growth and metastatic dissemination in vitro and in vivo. Moreover, patients with high expression of both proteins showed a poorer disease-free survival and overall survival compared to patients with high expression of only one protein (Feng et al., 2021).
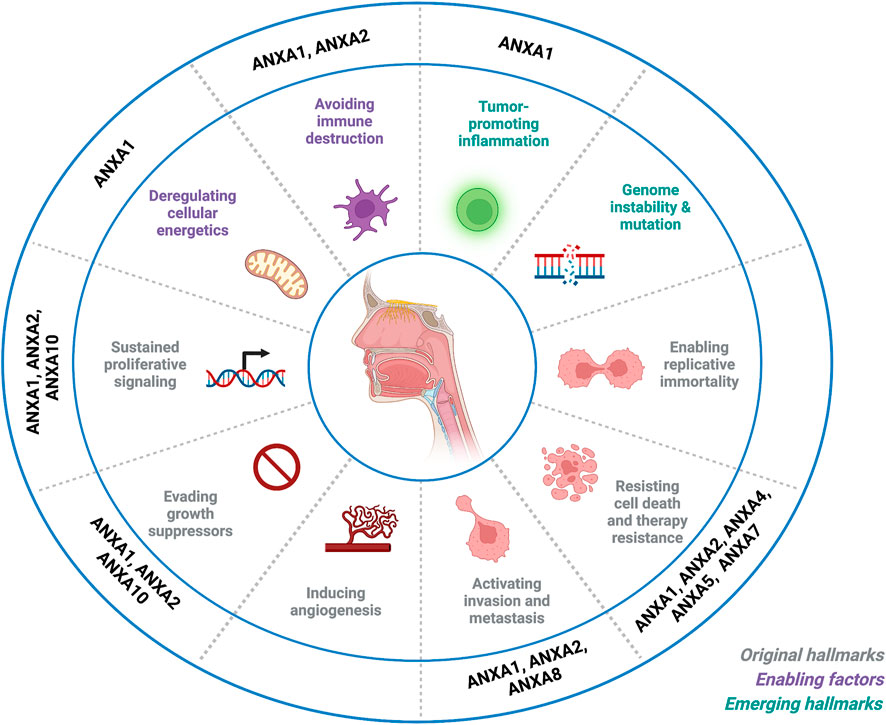
FIGURE 3. Impact of annexin dysregulation on the hallmarks of head and neck cancer. Schematic representation that summarizes current knowledge on annexin dysregulation in relation to the hallmarks of cancer defined by Hanahan and Weinberg. Image created with BioRender.com.
ANXA1 also regulates autophagy in NPC cells through the inhibition of the proteins BECN1 and ATG5, promoting cell migration, invasion and metastasis. ANXA1-mediated autophagy suppression involves the PI3K/Akt signaling pathway (Zhu et al., 2018). Likewise, ANXA1 can also exert a pro-tumorigenic role in HNC by regulating tumor growth and metastasis through FPR2 (Gastardelo et al., 2014). ANXA1 has also been linked to radio- and chemoresistance (Zhuwang et al., 2013; Suh et al., 2015). Moreover, ANXA1 mRNA levels were found to diminish in OSCC patients compared to controls and detectable in liquid biopsy, postulating its application as a blood-based biomarker (Faria et al., 2010).
Annexin A2
Structural and functional characteristics
Annexin A2 (ANXA2; also known as lipocortin II or calpactin-1 heavy chain) is a peripheral membrane-binding protein encoded by ANXA2 gene, located at 15q22.2. There are three pseudogenes located on chromosomes 4, 9, and 10. ANXA2 protein is mainly expressed at the surface of several cell types, including epithelial cells, macrophages and mononuclear cells (Hedhli et al., 2012; Wang and Lin, 2014). It can be detected as a monomer, heterodimer or heterotetramer (Waisman, 1995). It is mainly found as a multifunctional heterotetrametric form composed by two subunits of ANXA2 bridged non-covalently with a S100A10 dimer (A2t or AIIt) (Thiel et al., 1992; Deora et al., 2004). It results in an enhanced binding affinity to membrane phospholipids; consequently, this complex is mainly found associated with the plasma membrane and specific membrane-bound structures. Its subunits contains three type II and two type III Ca (2+)-binding sites, being able to mediate the interaction between ANXA2 complex AIIt (S100A10 and S100A11) and anionic phospholipid, F-actin, and heparin (Liemann and Lewit-Bentley, 1995; Liemann and Huber, 1997; Filipenko and Waisman, 2001). Phosphorylation at tyrosine residue Tyr23 induces nuclear translocation, while phosphorylation of the serine residues Ser11 and Ser25 allows ANXA2 export from the nucleus (Liu J. et al., 2003).
ANXA2 participates in several cellular functions in both health and disease. It plays a prominent regulatory role of membrane trafficking, and actin-related membrane dynamics. ANXA2/S100A10 heterotetramer formed by Ca2+ presence binds to cortical actin network, regulating exocytosis (Creutz, 1992), micropinocytosis (Merrifield et al., 2001), and plasmatic membrane cytoarchitecture (Benaud et al., 2004). The heterotetrameric complex plays a role in the subcellular distribution of early and recycling endosome flow process (Harder and Gerke, 1993; Zobiack et al., 2003), whereas it is suggested that ANXA2 monomeric form has been related to cholesterol-mediated endocytosis (Morel and Gruenberg, 2007).
ANXA2 has also been implicated in the maintenance of fibrinolytic homeostasis by its interaction with tenascin-C and the tissue-type plasminogen activator on the cell surface, which is crucial for the degradation of fibrin (Gerke et al., 2005).
Altered expression and pathobiological role in cancer
ANXA2 dysregulation is a common feature in multiple cancers (Li Z. et al., 2021). Similar to ANXA1, ANXA2 may elicit either tumor-promoting or -suppressive mechanisms depending on the cancer type; however, ANXA2 is frequently enhanced in metastatic cancers. ANXA2 expression has been shown to promote proliferation, invasion and metastasis in gliomas (Zhai et al., 2011), ovarian cancer (Lokman et al., 2013), hepatomas (Zhang et al., 2013), breast cancer (Sharma et al., 2006) and pancreatic cancer (Zheng et al., 2011). Conversely, there are tumors where ANXA2 acts as a tumor suppressor. In prostate cancer, ANXA2 expression is reduced or lost in cell lines, and its overexpression inhibited cell migration (Liu J. W. et al., 2003). ANXA2 is also found downregulated in ESCC and significantly correlated with lymph node metastasis and pathological differentiation (Feng et al., 2012). In osteosarcomas, increased protein expression was correlated with lower metastatic potential (Gillette et al., 2004).
ANXA2 regulation by post-translational modifications plays a critical role. In particular, phosphorylation on serine residues Ser11, Ser25 and tyrosine Tyr23 may have important functional implications in a variety of cellular processes, which are also relevant for tumor cell biology as major hallmarks of cancer. Phosphorylation of Tyr23 residue on ANXA2 has been pointed as a key regulator of cell motility. Upon Tyr23 phosphorylation, ANXA2 binds to actin filaments to enhance or inhibit migration modulating cytoskeletal structure (de Graauw et al., 2008; Kpetemey et al., 2015).
Tyr23 phosphorylation may promote cell migration by regulating the DOCK3/β-catenin/WAVE2 axis in different models of hepatoma cells (Cui et al., 2016). It has also been reported that Tyr23 phosphorylation triggers epithelial-to-mesenchymal transition (EMT) mediated by Rho, transforming growth factor (TGF)-β and Twist/Snail pathways (Rescher et al., 2008; Zheng et al., 2011; Chen et al., 2015).
ANXA2 has been reported as a major cellular substrate of Src that phosphorylates ANXA2 on Tyr23 regulating actin interactions and subsequently cell migration (Shetty et al., 2012). Based on this, a recent report by Mahdi et al., 2020 demonstrated EGF-dependent ANXA2 phosphorylation on Tyr24, which was assumed but not demonstrated to be by Src (Mahdi et al., 2020). In addition, ANXA2 promotes cell invasion mediated by Src/ANXA2/STAT3 pathway (Rocha et al., 2018). Similarly, YES1 phosphorylates ANXA2 on Tyr24, which drives gastric cancer invasion and metastasis by the activation of EphA2/YES1/ANXA2 axis (Mao et al., 2021).
ANXA2 is indirectly involved in Akt pathway regulation. When cells are exposed to irradiation, Akt protein binds to the heat shock protein 27 (HSP27), which ameliorates the effects on DNA damage and apoptosis by radiation (Kanagasabai et al., 2010).
ANXA2 influences p53 level by activating JNK/c-Jun signaling and suppressing p53 expression and its downstream target genes, p21, BAX and GADD45, which regulate apoptosis (Wang et al., 2012; Feng et al., 2017). It is well-known that nutrition and oxygen are indispensable for tumor cells to grow and survive. So, in the early stage of tumor starvation, ANXA2 may support starving cells by inducing autophagy (Moreau et al., 2015).
There are also evidences for a potential application of ANXA2 as a therapeutic target. Ricciardelli et al. used an anti-ANXA2 antibody to reduce both tumor growth and metastasis in an ovarian cancer mice model (SK-OV3) (Lokman et al., 2013). One year later, Mandip Singh and others inserted short hairpin (sh)RNA targeting ANXA2 (shANXA2) into a cationic ligand-guide (CLG, a liposomal carrier) to construct a CLG-ANXA2 compound. The CLG-ANXA2 was designed to recognize cancer cells and CSCs in a lung cancer mouse model (H1650). After CLG-ANXA2 was taken up by tumor cells, shANXA2 reduced ANXA2 mRNA and protein levels. The CLGshANXA2 subgroup showed reduced tumor growth (72–75% relative to the control) (Andey et al., 2014). It has also been recently described an ANXA2-targeting peptide motif CBP12 with highly selectivity and affinity to ANXA2 and proved ability to specifically target colorectal cancer cells, therefore emerging as a candidate for ANXA2-targeted therapeutic strategies (Staquicini et al., 2017). Interestingly, listeria-based anti-ANXA2 targeting vaccine (Lm-ANXA2) in combination with anti-PD-1 antibodies have demonstrated to be effective in PDAC mice models (Kim et al., 2019).
Noteworthy, ANXA2 gene silencing has demonstrated to downregulate the expression of proangiogenic proteins including vascular endothelial growth factor as (VEGF)-R2, VEGF-C, matrix metalloproteinases such as MMP-2, MMP-9, MT1-MMP and also the metalloproteinase inhibitor TIMP-2 (Bao et al., 2009).
ANXA2 may play an immunomodulatory role, since ANXA2 interacts with dendritic cell (DC)-specific intracellular adhesion molecule (ICAM)-3 grabbing non-integrin (DC-SIGN, CD209) leading to immunosuppression (Chao et al., 2015). This suppression might influence the clinical efficacy and outcome of anticancer therapies.
ANXA2 has also been linked to treatment resistance. Intracellular ANXA2 binds the p50 subunit of nuclear factor (NF)-κB by exposure of pancreatic cancer cells to genotoxic agents. This complex is translocated to the nucleus to activate the NF-κB signaling pathway, which modulates cell apoptosis and drug resistance (Jung et al., 2015). Nuclear translocation of ANXA2 also exhibited a protective effect against DNA damage caused by irradiation in human lung and breast cancer cells (Madureira et al., 2012; Grindheim et al., 2016).
Besides, it has been described a reciprocal regulation between ANXA2 and ERG oncoprotein in prostate cancer (Griner et al., 2015) and with HER2 in breast cancer (Shetty et al., 2012)
Clinical and biological roles in HNC
There are controversial findings on the function of ANXA2 in HNSCC. Different studies have reported relationships of ANXA2 with poor or good prognosis depending on the tumor location or the studied cohort. These discrepant results could be related to differences on patient characteristics, such as race (Beebe-Dimmer and Cooney, 2019), smoking (Bezerra et al., 2018), oral health habits (Kawakita et al., 2017), HPV infection (Hübbers and Akgül, 2015), etc.
ANXA2 is upregulated in several tumor subtypes but in HNSCC is usually found downregulated, and low ANXA2 levels associated with poorly differentiated tumors and metastasis in OSCC, laryngeal/pharyngeal squamous cell carcinoma (Pena-Alonso et al., 2008; Rodrigo et al., 2011b, 2014). Also, intestinal-type sinonasal adenocarcinomas with loss of expression of ANXA2 are associated to the more aggressive histopathological types (Rodrigo et al., 2011a). By contrast, another study reported high ANXA2 protein expression in OSCC associated with disease stage, differentiation grade, lymph node metastasis and poor patient survival (Ma and Wang, 2021). This study also found that ANXA2 silencing by siRNA was able to significantly reduce the proliferation, migration and invasion of OSCC cells.
Some studies have associated high ANXA2 expression levels to poor overall survival and disease-free survival, and advanced disease status in esophageal carcinoma (Ma et al., 2014), and NPC (Chen et al., 2015). Notably, ANXA2 participates in facilitating host cell infection by HPV16 resulting in a high risk of tumor progression (Woodham et al., 2012). Moreover, ANXA2 has been linked to resistance to radio and chemotherapy in NPC (Chen et al., 2015), while ANXA2 interaction with dendritic cells caused immunosuppression mediated by IL-10 production (Chao et al., 2015).
In summary, various ANXA2-targeted therapeutic strategies have already been developed and tested, demonstrating antitumor efficacy in some preclinical cancer models. However, despite widespread dysregulation of ANXA2 expression in different cancers, the biological and clinical impact of ANXA2 targeting may considerably vary depending on cellular and tissue specificity, Therefore, a potential clinical application will require individual testing and deep functional and mechanistic characterization for each cancer type. Furthermore, ANXA2 role modulating radio and chemotherapy as well as immune response exposes it as a combination target to overcome resistance and to improve treatment response.
Annexin A3
Annexin A3 (ANXA3), also known as lipocortin III or placental anticoagulant protein III, is a calcium-dependent phospholipid-binding protein that affects membrane trafficking and organization (Gerke and Moss, 2002). It has two isoforms that vary slightly in their molecular weights (33 and 36 kDa) (Moss and Morgan, 2004), result from the alternative splicing of exon 3 within the ANXA3 gene (Bianchi et al., 2010) located at chromosome region 4q13-q22 (Tait et al., 1991). This protein contains two special tryptophan residues, which can influence its interaction with phospholipid membranes (Sopkova et al., 2009). Regarding its functions, ANXA3 was first shown to inhibit PLA2 (Coméra et al., 1990) and to cleave the cyclic bond of inositol 1,2-cyclic phosphate to form inositol 1-phosphate (Ross et al., 1990). Nevertheless, later studies have confirmed its involvement in a wide variety of biophysiological processes, including vesicular transport (Faugaret et al., 2011), cell migration (Zhang Z. et al., 2021), angiogenesis (Park et al., 2005), apoptosis (Chong et al., 2010), inflammation (Meng et al., 2019) or sepsis (Toufiq et al., 2020) among others.
Similar to other members of the annexin family, aberrant expression of ANXA3 has been linked to cancer pathogenesis, although it is highly dependent on the tissue of origin. Accordingly, ANXA3 expression has been found upregulated in breast, colorectal or pancreatic tumors, while downregulated levels have been detected in renal, thyroid or prostate cancers. Research performed assessing the functional role of ANXA3 in cancer has demonstrated its involvement in vital processes for tumor development and progression (Liu C. et al., 2021; Yang et al., 2021), such as sustaining proliferative signals from ERK and JNK pathways in colorectal (Xu R. et al., 2019) and hepatocellular carcinomas (Tong et al., 2015), or PI3K/Akt pathway in pancreatic cancer (Wan et al., 2020). In addition, aberrant ANXA3 expression has also been shown to downregulate multiple pro-apoptotic proteins, promote tumor invasion and metastasis, induce angiogenesis and even regulate drug resistance in different tumor types (Liu C. et al., 2021; Yang et al., 2021).
Regarding its clinical relevance, high ANXA3 expression has been correlated with a poor prognosis in gastric, hepatocellular and breast carcinomas (Liu C. et al., 2021), and it has also been proposed as a diagnostic marker in prostate cancer (Schostak et al., 2009).
Despite mounting evidence supporting the involvement of ANXA3 in cancer development and progression, as of yet, very little has been unraveled regarding its specific contribution to HNC. Only one study has been published in which ANXA3 was identified as a downstream target of EGFR using a functional proteomic approach in NPC-derived cells treated with TGF-α (Ruan et al., 2010). This approach confirmed that treatment of CNE2 cells with TGF-α induced tyrosine phosphorylation of ANXA3, which was blocked by treatment with the EGFR inhibitor PD153035 (Ruan et al., 2010). Even though the relevance of ANXA3 phosphorylation/dysregulation has not yet been determined, these results suggest a potential impact on NPC treatment, and encourage deepening on ANXA3 contribution in HNC since it could positively impact patient management and disease outcome.
Annexin A4
Annexin A4 (ANXA4), also known as lipocortin IV or placental anticoagulant protein II, has 45–59% identity with other members of its family and shares a similar size and exon-intron organization (Moss and Morgan, 2004). It is a calcium and phospholipid binding protein that promotes membrane fusion and is involved in exocytosis (Oling et al., 2000; Kaetzel et al., 2001). Physiologically, ANXA4 has been shown to inhibit adenylyl cyclase 5 (Heinick et al., 2015, 2020), an enzyme that catalyzes the conversion of ATP to adenosine 3′,5′-cyclic monophosphate (cAMP), and also to interfere with sulfatide-induced plasma coagulation (Nakayama et al., 2020). ANXA4 acts as a sensor of negative membrane curvatures suggesting a role in the restoration of plasma membrane (Florentsen et al., 2021). In addition, it has been implicated in the migration of both skin fibroblasts (Wang et al., 2020) and airway epithelial progenitor cells (Jiang et al., 2018), as well as in trophoblast invasion via the PI3K/Akt/eNOS pathway (Xu Y. et al., 2019).
Accordingly, ANXA4 has also been involved in cell migration and invasion in different cancers. Thus, reduced ANXA4 levels can hamper these two processes in cells derived from gastric cancer (Ji et al., 2022), gallbladder cancer (Yao et al., 2016) and ovarian clear cell adenocarcinoma (Wang et al., 2017); meanwhile its overexpression in colorectal cancer cells was able to promote their migratory and invasive potential (Peng et al., 2021). In addition, ANXA4 expression has been associated to poor prognosis in ovarian serous carcinoma (Choi et al., 2013).
Interestingly, ANXA4 translocation from plasma membrane to cytosol has been related to resistance to paclitaxel in lung cancer cells (Gaudio et al., 2016; Scala et al., 2021) and also its upregulation has been linked to cisplatin (CDDP) resistance in mesothelioma cells (Yamashita et al., 2012), ovarian clear cell carcinoma cells (Morimoto et al., 2014) and non-small cell lung cancer (NSCLC) cell lines (Zheng et al., 2018).
Very limited information has been published to date on the role of ANXA4 in HNC. The study by Deng et al. (Deng et al., 2012) was the first to explore ANXA4 expression in laryngeal tissues. Even though the analysis was only performed in 30 patient samples, it clearly showed that its expression was frequently detected (73%) in this cancer type (Deng et al., 2012). In addition, based on a differential proteomic expression analysis, ANXA4 has been postulated as a biomarker for OSCC diagnosis and prognosis (Liu W. et al., 2016). ANXA4 expression was early detected in oral premalignant lesions (oral submucous fibrosis, OSF) as well as in the corresponding patient-matched OSCC tissue samples, where expression was found to correlate with tumor stage and poor prognosis (Liu W. et al., 2016).
Another study compared proteomic profiles in saliva samples from healthy donors and tamol chewers, which tend to form ulcers in the oral cavity (Kumar et al., 2021) predisposing to develop oral cancer (Phukan et al., 2001; Borkotoky et al., 2020). ANXA4 expression levels showed a 20.22% increase in tamol chewers versus healthy controls, which led to propose ANXA4 as a population screening marker for the early detection of OSCC in tamol consumers (lepcha et al., 2021). It has also been reported that ANXA4 could serve to distinguish between two different types of benign tumors of the salivary glands, Warthin’s tumors (WT) and pleomorphic adenomas (PA), as PAs differentially express proteins associated with cell death, apoptosis and tumorigenesis, like ANXA4 and ANXA1 (Donadio et al., 2013). Moreover, it has been suggested a possible contribution of ANXA4 to OSCC chemoresistance, because this protein was found differentially expressed among others, in Sirt1-induced resistance to CDDP (Xiong et al., 2011). However, further investigation is needed to demonstrate a direct participation in OSCC chemoresistance, as well as to extend ANXA4 analysis to other HNSCC subsites.
Together these data emphasize the conceivable application of ANXA4 as a biomarker for OSCC diagnosis and prognosis, but also therapeutically, since targeting ANXA4 expression might hinder early tumorigenesis, progression and management of OSCC, and perhaps other HNC types.
Annexin A5
Annexin A5 (ANXA5) is the smallest member of the family with 36 kDa. Similar to ANXA4, it plays an important role in membrane repair (Bouter et al., 2015). However, probably the most known function of ANXA5 is its ability to bind PS, which is rapidly mobilized from the inner to the outer side of the membrane in cells undergoing apoptosis or necrosis (Rysavy et al., 2014). This characteristic together with the fact that ANXA5 is not toxic or immunogenic when used in vivo has made this protein a valuable tool for in vivo imaging of cell death processes (Chopra et al., 2012).
In the context of cancer, high ANXA5 expression has been associated with poor outcome in gliomas (Zhong et al., 2021), hepatocarcinomas (Sun et al., 2018), bladder (Wu et al., 2021), colorectal (Xue et al., 2009) and cutaneous squamous cell carcinomas (Shapanis et al., 2021). Interestingly, ANXA5 levels were higher in bladder cancer patients with lymph node involvement (Mitra et al., 2006), which is consistent with studies performed on cellular models of hepatocarcinoma. The authors demonstrated that the loss of ANXA5 expression decreased cell adhesion to lymph nodes, and also reduced the formation of lymph node metastasis in vivo (Sun et al., 2018). In addition, in vitro experiments have shown that ANXA5 depletion may hamper proliferation, migration, invasion and metastasis formation in various tumor cells from breast cancer (Bouvet et al., 2020), uterine cervical carcinoma (Li et al., 2018), hepatocarcinoma (Sun et al., 2018) and cholangiocarcinoma (Ding et al., 2017). In marked contrast to these data, it has been reported that positive ANXA5 expression correlates with a better prognosis in adult acute myeloid leukemia (AML) (Niu et al., 2019), and a tumor suppressor role has been described in gastric cancer cells through the repression of ERK pathway (Wang et al., 2021).
On the other hand, it has also been reported that PS is expressed in the surface of cancer cells and also vascular endothelial cells in tumors, whereas it cannot be detected in normal endothelium (Ran and Thorpe, 2002). Therefore, the ability of ANXA5 to bind to PS could be exploited for therapeutic purposes in cancer patients. According to this purpose, it has been demonstrated that ANXA5 may improve specific cancer cell targeting when combined with different therapeutic strategies, such as phototermal nanotubes (McKernan et al., 2021), vaccine antigens (Kang et al., 2020), or enzyme-prodrug systems (Virani et al., 2018). In all cases, treated mice experienced a prolonged survival without any noticeable toxic effects in healthy tissues (Virani et al., 2018; Kang et al., 2020; McKernan et al., 2021). Furthermore, ANXA5 administration in mice enhanced immunogenicity by binding to PS and inducing systemic cytotoxic T-cell responses, leading to tumor regression and reduced relapse (Li et al., 2020). It has also proved to rescue immune suppression after chemotherapy, thus contributing to generate a robust antitumor immunity (Kang et al., 2020).
In the specific context of HNC, ANXA5 was found overexpressed in 80% of the laryngeal cancer samples analyzed, although there is no available information on the clinical significance of this finding (Deng et al., 2012). Through a proteomic profiling approach, ANXA5 overexpression was also detected in malignant major salivary gland tumors (MSGT) versus the adjacent healthy tissue (Seccia et al., 2020); however, significant correlations between protein expression and the clinical parameters were not observed (Seccia et al., 2020). In a meta-analysis of gene expression in public datasets, ANXA5 was found among other 95 genes whose expression had a significant impact over OSCC patients’ survival (Bajrai et al., 2021). These data suggest that ANXA5 might play a role in OSCC progression, although a more in-depth analysis is needed to clarify and validate this finding. In addition, a comparative protein expression analysis in NPC-derived cells revealed that ANXA5 levels were increased in cis-diamminedichloroplatinum (cDDP)-resistant cells compared to the parental CNE2 cells (Tang et al., 2012). These results suggest that ANXA5 could contribute to cDDP resistance in NPC; however, more experiments are required to confirm its direct involvement, and to extend analysis to other HNC types.
These data evidence that the role of ANXA5 in HNC development and progression remains largely unknown. Therefore, more efforts are needed to unravel this, in particular, its therapeutic potential due to the high affinity of ANXA5 for PS. On this basis, ANXA5 targeting could emerge as a valuable strategy to counteract immune evasion in HNC, and ultimately contribute to trigger a potent antitumor immune response (Birge et al., 2016).
Annexin A6
Annexin A6 (ANXA6) is the only protein from this family structurally comprising 8 annexin repeats that form two cores in the C-terminal domain (Qi et al., 2015). This peculiarity may render specific membrane-interaction mechanisms (Buzhynskyy et al., 2009). ANXA6 is expressed in most human tissues, and mainly localized in plasma membrane and endosomes (Grewal et al., 2017). Physiologically, ANXA6 plays a crucial role as a scaffold protein and in the organization of membrane domains, where it interacts with many different partners into multifactorial complexes that orchestrates different signaling pathways. As a result, ANXA6 is involved in many events associated with membrane organization, such as cholesterol homeostasis, interactions with actin cytoskeleton proteins, regulation of endocytic trafficking and secretory events, modulates calcium flux and homeostasis and is involved in the reorganization of cytoskeleton during cell migration. In relation to its scaffolding properties, ANXA6 regulates several signaling pathways such as EGFR/Ras/MAPK and FAK/PI3K (Qi et al., 2015; Grewal et al., 2017). ANXA6 has been directly involved in regulating Ca2+ entry. Its stable membrane expression reduces calcium store-operated entry and reduces cell proliferation. Its interaction with plasma membrane and subsequent cortical actin stabilization attenuates calcium entry in vivo (Monastyrskaya et al., 2009). ANXA6 has also been involved in membrane repair mechanisms (Potez et al., 2011; Swaggart et al., 2014), inducing constriction forces and acting synergistically with ANXA4 (Boye et al., 2017).
ANXA6 has been involved in several biological processes such as cell proliferation, survival, membrane repair, migration and adhesion (Grewal et al., 2017), which are often dysregulated in cancer. In most cases, ANXA6 acts as a tumor suppressor, but some oncogenic roles have also been described depending on the cancer type and disease stage.
It has been demonstrated that ANXA6 present in extracellular vesicles may play a role in cancer. In pancreatic ductal adenocarcinoma (PDA), cancer-associated fibroblasts (CAF)-derived extracellular vesicles containing the complex ANXA6/LRP1/TSP1 enhanced tumor aggressiveness, and ANXA6 was crucial to promote aggressive phenotypes, invasion in vitro, and metastasis formation in vivo (Leca et al., 2016). Exosomal ANXA6 has also been involved in chemotherapy resistance. Exosomal ANXA6 from gemcitabine-resistant cells induced gemcitabine resistance in sensitive triple negative breast cancer (TNBC) cells, at least by downregulating EGFR (Li T. et al., 2021). Another study from Uchihara et al. showed that ANXA6 from CAF-derived extracellular vesicles induced drug resistance in gastric cancer cell lines via integrin beta1 stabilization in the cell surface and subsequent FAK-YAP pathway activation (Uchihara et al., 2020). In breast cancer, ANXA6 present in exosomes from stem cells promoted paclitaxel resistance via YAP1 upregulation. ANXA6 also promoted cell migration, autophagy, cell growth and stem properties (Guo et al., 2021). In addition, ANXA6 has been involved in resistance to EGFR-TKIs such as lapatinib in TNBC, where ANXA6 upregulation is part of an adaptive mechanism to acquire resistance (Widatalla et al., 2019). Another study from the same group showed that ANXA6 could also promote resistance to some EGFR-TKIs in breast cancer cells (Koumangoye et al., 2013).
ANXA6 has been extensively studied in breast cancer. ANXA6 is required for the cell-cell and cell-ECM contacts, and its loss contributes to tumor progression by promoting loss of cell contacts and anchorage-independent growth. ANXA6 was also found necessary for an efficient motility and invasion of breast cancer cells. Sakwe et al. discussed these apparent discrepancies and expound the varying levels of ANXA6 expression across breast cancer development (Sakwe et al., 2011). Hence, ANXA6 may function as a tumor suppressor or tumor promoter, depending on the cancer subtype and degree of malignancy. ANXA6 antitumor role may be more relevant in TNBC than in non-triple negative breast cancers (Korolkova et al., 2020b). The involvement of ANXA6 in plasma membrane repair may allow cancer cells to rapidly respond to small membrane injuries that arise frequently, as demonstrated in MCF-7 cells (Boye et al., 2017). Moreover, a reciprocal regulation between ANXA6 and the Ca2+ activated RasGRF2 (GRF2) has also been recently reported in TNBC, which could serve to distinguish rapidly growing tumors from those more aggressive and highly invasive (Korolkova et al., 2020a).
Ectopic expression of ANXA6 in the human A431 squamous epithelial carcinoma cells reduced tumor growth suggesting an antitumor role for ANXA6 in A431 cells (Theobald et al., 1995). ANXA6 overexpression also reduced LDL cholesterol-induced migration and invasion of A431 cells (Jose et al., 2022). Interestingly, ANXA6 reduced A431 cell migration and invasiveness, and enhanced EGFR-TKIs-mediated inhibition of growth and migration in these cells (Hoque et al., 2020). All the aforementioned studies highlight the anti-tumor role of ANXA6 in A431 squamous epithelial cells overexpressing EGFR. Given that EGFR overexpression is a common feature in HNSCC, an analogous role for ANXA6 could be plausible, which merits further investigation.
Several studies have demonstrated that ANXA6 is a scaffold protein that modulates EGFR/Ras/MAPK pathway. Thus, ANXA6 interacts with several proteins like p120 GAP, Raf1 and PKCalfa, downregulating this pathway, thereby acting as a tumor suppressor in several human cancers (Pons et al., 2001; Grewal et al., 2005; Muga et al., 2009; Cornely et al., 2011; Koese et al., 2013; Wang et al., 2013). This is a very important mechanism in HNSCC, where ANXA6 promotes PKCalfa-mediated EGFR inactivation, acting as a scaffold for PKCalfa and promoting its recruitment to plasma membrane and its interaction with EGFR. ANXA6 also promoted PKCalfa-dependent inhibitory feedback over EGFR pathway. It is also important to mention that downregulation of ANXA6 expression occurs in several malignancies with EGFR upregulation, maybe as a possible mechanism to prevent the antitumor role of ANXA6 (Koese et al., 2013). By contrast, it has been described a protumor role for ANXA6 and its involvement in drug resistance (Koumangoye et al., 2013; Widatalla et al., 2019), related to its ability to modulate biological membranes, and to stabilize activated receptors in the membrane (Cornely et al., 2011), such as EGFR (Koumangoye et al., 2013).
ANXA6 expression has been proposed as a useful biomarker in several human cancers. ANXA6 acts as a tumor suppressor in cervical cancer via autophagy induction in vitro and in vivo. Besides, ANXA6 expression levels may serve as a predictive biomarker of survival for cervical cancer patients (Sun et al., 2020). Nuclear ANXA6 expression has been proposed as a protein marker for squamous cervical cancer diagnosis (Lomnytska et al., 2010), which may improve cervical cancer diagnosis at early stages and patient monitoring (Lomnytska et al., 2011). ANXA6 has also been included as a serum biomarker in a 4-protein panel for esophageal adenocarcinoma detection (Zaidi et al., 2014). ANXA6 is downregulated in human hepatocellular carcinoma, and suggested as a putative biomarker for these tumors (Meier et al., 2016). In basal-like breast cancer, reduced ANXA6 expression was significantly associated with higher recurrence-free but lower distant metastasis-free and overall survival. Koumangoye et al. also proposed ANXA6 expression as a biomarker to identify patients likely to respond to EGFR-TKIs (Koumangoye et al., 2013). In addition, ANXA6 has emerged as a potential biomarker in ovarian cancer, since it was found significantly upregulated in tumor tissue samples, particularly in stages II-IV compared to health tissue (Noreen et al., 2020). High ANXA6 expression has been associated with a poor survival in PDA, and ANXA6 levels in circulating extracellular vesicles (EVs) postulated as a diagnostic and prognostic biomarker in PDA (Leca et al., 2016). Similarly, serum exosomal ANXA6 levels could serve as a predictive biomarker of response to gemcitabine-based chemotherapy in TNBC patients (Li T. et al., 2021).
Annexin A7
Annexin A7 (ANXA7), also known as synexin, presents a long hydrophobic N-terminal domain, rich in glycine, tyrosine and proline. This protein is predominantly located in plasma membrane, secretory vesicles and nuclear envelope (Guo et al., 2013a; Grewal et al., 2016). ANXA7 is deeply involved in calcium homeostasis, and it was actually the first annexin shown to function as a calcium channel. It is involved in exocytic secretion and aggregation of chromatin granules. It also displays a GTPase function, interacting and hydrolyzing GTP in several cellular processes, such as calcium/GTP-dependent exocytic trafficking (Gerke and Moss, 2002; Grewal et al., 2016). Besides its functions in calcium homeostasis and as a GTPase, ANXA7 negatively regulates COX-dependent prostaglandin (PGE2) production and is also involved in cardiac remodeling, as well as in cell proliferation regulation (Grewal et al., 2016).
ANXA7 has also been reported to play a role in cancer, either oncogenic or oncosuppressor depending on the tumor subtypes. As discussed below, ANXA7 may act as an oncogene in gastric cancer, hepatocellular carcinoma, nasopharyngeal carcinoma and breast cancer; or as an oncosuppressor in melanoma, prostate cancer, and glioblastoma.
In gastric cancer, the significance of ANXA7 expression remains controversial. Loss of ANXA7 expression has been associated with distant metastases in gastric cancer, hence suggesting an oncosuppressor role (Hsu et al., 2008). However, other studies have pointed to a protumor role of ANXA7 in gastric cancer. Yuan et al. demonstrated that high ANXA7 expression was an independent predictor of poor survival, which was associated with poor differentiation, and presence of lymph node metastasis (Yuan et al., 2014). ANXA7 expression was also found to be downregulated along gastric cancer progression and inversely correlated with apoptosis (Ye et al., 2018). These findings could reflect an anti-apoptotic role for ANXA7 in gastric pathogenesis and a potential biomarker for diagnosis, prognosis and treatment for gastric adenocarcinoma patients (Ye et al., 2018).
ANXA7 upregulation in hepatocellular carcinoma has been associated with enhanced invasion and lymphatic metastasis (Sun et al., 2009b; 2009a). In Her2-negative breast cancer, ANXA7 expression correlated with metastasis and low survival rate, and serves as a diagnostic and prognostic biomarker for these patients (Srivastava et al., 2004). In addition, Srivastava et al. showed that high expression of ANXA7 was a strong predictor of reduced disease-free survival (Srivastava et al., 2001a), and thus highlights its potential as a prognostic biomarker to predict breast cancer patients’ survival.
On the other hand, supporting a tumor-suppressor role, ANXA7 has been pointed as a marker for a less invasive phenotype in melanoma (Kataoka et al., 2000). ANXA7 also exhibits a suppressive role in prostate cancer. ANXA7 inhibits tumor growth and cell proliferation, and its loss of expression has been correlated with late-stage prostate cancer (Srivastava et al., 2001b). Moreover, reduced ANXA7 expression occurred significantly in metastatic and hormone refractory prostate cancer compared to benign hyperplasia (Srivastava et al., 2001a).
ANXA7 expression has been reported an independent outcome predictor in glioblastoma multiforme, and its expression correlated with longer survival in patients with GBM (Hung and Howng, 2003). ANXA7 has been shown to downregulate EGFR in glioblastoma and loss of ANXA7 mRNA expression was associated with poor survival and prognosis in glioblastoma patients. Altogether, these data support an oncosuppressive role of ANXA7 in these malignancies as well as an oncogenic synergistic effect between ANXA7 loss and EGFR amplification (Yadav et al., 2009).
In the context of HNC, it has only been reported that ANXA7 silencing enhanced radiosensitivity in NPC via apoptosis promotion (Gui et al., 2020).
Annexin A8
Annexin A8 (ANXA8), similar to other members, plays an important role in the organization of membrane domains, especially those that constitute sites of membrane-cytoskeleton interactions, for instance, by binding F-actin and certain phospholipids such as PtdIns(4,5)P2 (Goebeler et al., 2006). Moreover, ANXA8 is highly involved in the regulation of intracellular membrane trafficking and regulation of endocytosis (Goebeler et al., 2008). Related to this latter role, ANXA8 has been implicated in the adhesion of leukocytes to the endothelium, by modulating CD63 sorting and efficient membrane presentation (Poeter et al., 2014). ANXA8 also regulates adhesion to ECM proteins like integrin β1 (Heitzig et al., 2017). All these features make ANXA8 an important regulator of key cellular processes such as migration, invasion and adhesion, often commonly dysregulated in cancer. ANXA8 mainly acts as a tumor-promoting gene in human cancers. ANXA8 upregulation was first described in promyelocytic leukemia, harboring a PML-RARA fusion, in which dysregulation of RARA gene caused such overexpression (Chang et al., 1992).
Its biological relevance has been intensely studied in mammary gland development and breast cancer. ANXA8 is regulated by all-trans retinoid acid (RA) and RA-RARA-ANXA8 axis enhances a loop of aberrant morphogenesis, rendering an abnormal mammary gland structure (Rossetti and Sacchi, 2019). In fact, ANXA2 downregulation and ANXA8 upregulation were jointly sufficient to create abnormal ductal carcinoma in situ (DCIS) acinar-like structures, which resemble early breast cancer lesions. Moreover, ANXA8 upregulation is detected in DCIS compared to atypical ductal hyperplasia and normal mammary gland, and also highly upregulated in ER-negative tumors compared to ER-positive ones. In addition, ANXA8 expression significantly correlated with features of breast cancer progression such as tumor grade, stage and lymph node infiltration, arising as a putative biomarker to identify ER-negative basal-like breast cancers (Rossetti et al., 2016).
Meanwhile, ANXA8 overexpression in gastric cancer has been correlated with disease stage and differentiation grading, and it emerged as an independent predictor of worse OS and DFS and a potential poor prognosis biomarker for gastric cancer patients (Ma et al., 2020). ANXA8 has also been found upregulated in squamous cell carcinoma of the uterine cervix (Chao et al., 2006). Mechanistically, ANXA8 inhibition by miR-185-3p reduced the proliferation of cervical cancer cells (Zhang and Han, 2021).
In pancreatic cancer, ANXA8 overexpression was also associated with higher histological grades and a lower survival in I-II stage patients, thereby emerging as a poor prognosis biomarker for early stages of pancreatic cancer (Pimiento et al., 2015). Another study from Karanjawala et al. found ANXA8 overexpression in infiltrating ductal pancreatic adenocarcinomas compared to normal ducts, suggesting its possible use as a diagnostic biomarker (Karanjawala et al., 2008). There are also evidences for a protumor role of ANXA8 in bladder cancer. Expression was consistently found upregulated in bladder tumors and derived cell lines and promoted tumor growth and metastases in vitro and in vivo, while ANXA8 silencing reduced tumor growth, migration, invasion and EMT (Yuan et al., 2021).
In ovarian cancer, ANXA8 expression was also found to increase during tumor progression. High ANXA8 levels were significantly associated with advanced stages, differentiation grade and nodal metastases, and proposed as a poor prognosis biomarker in epithelial ovarian cancer (Zhu et al., 2020). ANXA8 mRNA levels were also upregulated and correlated with poor overall survival and progression-free survival in patients with ovarian serous tumors. Immunohistochemical ANXA8 expression in malignant ovarian tumors correlated with FIGO stages and tumor progression, and it was revealed as an independent predictor of outcome and survival and a powerful poor prognosis biomarker for these tumors (Gou et al., 2019).
Besides, it has been demonstrated that ANXA8 regulates VEGFR-driven angiogenesis, showing a crucial role for sprouting, invasion and adhesion of human umbilical vein endothelial cells (HUVECs) to ECM proteins (Heitzig et al., 2017). Given such implications, to deepen investigation on a plausible role of ANXA8 as a therapeutic target for anti-angiogenic strategies should be encouraged.
Despite all the aforementioned studies strongly supporting a relevant role for ANXA8 in numerous cancer types, it has so far been poorly investigated in HNC. A study from Kudo et al. reported ANXA8 upregulation in maxillary squamous carcinomas harboring TP53 mutation (Kudo et al., 2017). It has also been published that ANXA8 mRNA expression levels could serve as a biomarker to detect OSCC lymph node metastases that were histopathologically undetectable (Oka et al., 2016).
Annexin A9
Annexin A9 (ANXA9) was first discovered in 1999 (Morgan et al., 1999a). Its structure is similar to ANXA2 and displays certain conserved domains; however, ANXA9 is an atypical annexin member, unable to bind calcium since its sequence lacks the acidic cap residue in the calcium binding domains required for such interaction. This unique structural characteristic of ANXA9 whose biological function is not regulated by calcium, subsequently, leads to distinct subcellular locations, biochemical properties and biological functions beyond the scaffold features shared by other family members (Morgan et al., 1999a; Gerke and Moss, 2002; Goebeler et al., 2003).
Little is known about ANXA9 biological functions. Of special interest for epithelial biology is the finding that ANXA9 interacts with the N-terminal domain of periplakin, a protein that is especially relevant in the epidermis for the formation of epidermal cornified envelopes, and as a scaffold for several cytoskeleton proteins (Boczonadi and Määttä, 2012).
ANXA9 dysfunctions have been reported in several human cancers, showing a protumor role mainly linked to invasion and metastasis processes. ANXA9 expression has been involved in colorectal cancer invasion and metastases by regulating genes such as ADAM17, MMP-9, TIMP-1 and E-cadherin (Yu et al., 2018), and proposed as an independent factor of poor prognosis (Miyoshi et al., 2014; Yu et al., 2018). Likewise, ANXA9 expression has been associated with a higher incidence of bone metastasis in breast cancer and so included in a predictive gene panel (Cosphiadi et al., 2018), and related to breast cancer prognosis (Xiao et al., 2019).
In gastric cancer, ANXA9 upregulation has also been associated to poor prognosis in gastric cancer patients. In addition, ANXA9 was able to promote cell migration and growth in gastric cancer cell lines via TGF-β signaling, which reinforces its oncogenic role in these tumors (Zhou et al., 2021). ANXA9 expression was also associated with a poor prognosis in ovarian cancer and resistance to cisplatin in vitro and in vivo, posing ANXA9 as an interesting candidate for targeted therapies to overcome cisplatin-resistant cancers (Kou et al., 2021). Given that cisplatin-based chemotherapy is commonly used to treat HNSCC patients, it would be of major interest to address the role and expression of ANXA9 in cisplatin resistance in these tumors.
In the context of HNC, our group was first to evaluate by immunohistochemistry ANXA9 protein expression using a large homogeneous cohort of 372 surgically treated HPV-negative HNSCC patients. In normal tissues, ANXA9 expression was observed in the most differentiated layers of the squamous stratified epithelium but not proliferative basal cells. ANXA9 expression was downregulated in 42% of HNSCC samples compared to normal epithelia. This result was also further confirmed using transcriptomic data from the TCGA, which suggests possible transcriptional mechanisms underlying ANXA9 downregulation in HNSCC (Salom et al., 2019). Noteworthy, positive ANXA9 expression HNSCC was tightly associated with the histological differentiation grade, predominantly detected in well-differentiated tumors and oropharyngeal tumor location (Salom et al., 2019).
Annexin A10
Annexin A10 (ANXA10) was first identified in 1999 (Morgan et al., 1999b). Similar to ANXA9, changes and inactivation of well-conserved calcium binding sites provide unique membrane-binding properties and calcium-independent functions to ANXA9 and ANXA10, suggesting that both proteins could act in environments where calcium sensitivity is not functionally determining (Morgan et al., 1999b; Gerke and Moss, 2002). Some of these known singular functions for ANXA10 are related to a nuclear subcellular location, regulation of transcription and mRNA processing (Quiskamp et al., 2014).
ANXA10 plays a dual role in human cancers. Although most malignancies exhibit an oncopromoter role, there are also evidences for a tumor-suppressor role in several cancers, such as hepatocellular carcinoma, gastric cancer, bladder cancer and lung cancer. ANXA10 downregulation in hepatocellular carcinoma has been associated with poor survival rates, higher early intrahepatic tumor recurrence, higher vascular infiltration and a higher grade. Interestingly, this downregulation was correlated with mutated p53, and both alterations synergistically contributed to tumor progression (Liu et al., 2002, 2012). Loss of ANXA10 expression is frequent in early gastric cancer, and constitutes an independent biomarker of poor prognosis (Ishikawa et al., 2020). Kim et al. found that ANXA10 may display a tumor-suppressor role by reducing tumor growth and promoting apoptosis in gastric cancer cells (KIM et al., 2010). It has also been suggested that ANXA10 may constitute a predictive biomarker of chemotherapy response in gastric cancer (Ishikawa et al., 2022a). In bladder cancer, low ANXA10 expression was associated with a shorter progression-free survival and proposed as a poor prognosis biomarker in both early and advanced tumors (Munksgaard et al., 2011). In addition, ANXA10 has been proposed to be a tumor suppressor in NSCLC based on migration and invasion assays and in vivo studies conducted by Hung et al. (Hung et al., 2019). Opposing to this, high ANXA10 expression was detected in lung adenocarcinomas, and promoted the migration of A549 cells (Yumura et al., 2022).
In relation to its oncopromoter role, ANXA10 has been proposed as a prognostic biomarker and therapeutic target in many tumor types. Of special interest, high ANXA10 expression was reported as an independent poor prognostic biomarker in lung adenocarcinoma (Yumura et al., 2022), glioblastoma multiforme (Xu et al., 2021), serous epithelial ovarian cancer (Wang et al., 2019), pancreatic ductal adenocarcinoma (Ishikawa et al., 2022b), small bowel adenocarcinoma (Ishikawa et al., 2021) and papillary thyroid cancer (Liu X. et al., 2021). Interestingly, ANXA10 has also been proposed as a useful and highly specific biomarker for adenocarcinomas of the upper gastrointestinal tract and pancreatobiliary system, and also included in a panel of biomarkers to trace the origin of adenocarcinomas with unknown primary sites (Lu et al., 2013).
High ANXA10 expression has been correlated with depth of invasion and poor disease-free survival in ESCC patients. Moreover, ANXA10 promoted growth of ESCC cell lines via activation of Akt and ERK1/2 pathways (Kodaira et al., 2019). ANXA10 expression was also correlated with poor overall survival in colorectal cancer (Bae et al., 2015), and intrahepatic cholangiocarcinoma (Shao et al., 2022). In addition, ANXA10 expression was found upregulated in primary and metastatic melanoma and associated with tumor progression, observations reinforced by its promoting role of cell migration in vitro and metastases in vivo (Zhang X. et al., 2021). ANXA10 expression has been postulated as a useful biomarker for early detection of pancreatic ductal adenocarcinoma since it can be detected in precancerous lesions (Zhu et al., 2017).
In HNC, ANXA10 upregulation was detected in primary OSCC compared to normal tissue, which was more frequent in advanced cases and associated to tumor size. Mechanistically, it has been reported that ANXA10 promotes G1 cell cycle progression and cell proliferation enhancing ERK phosphorylation and ERK/MAPK pathway activation (Shimizu et al., 2012). These findings support a protumor role of ANXA10 in oral tumorigenesis by promoting proliferation and tumor growth. In accordance to these data, results from our group confirmed ANXA10 upregulation both at protein and mRNA level in HNSCC tissue samples compared to the normal counterpart (Salom et al., 2019). Thus, ANXA10 protein was detected in 64% of the HNSCC specimens but absent in normal epithelia. ANXA10 expression was significantly associated with differentiation grade, and higher in oropharyngeal tumors; however, no correlations with HNSCC patient survival were observed (Salom et al., 2019).
Annexin A11
Annexin A11 (ANXA11) displays a long N-terminal domain (aprox. 200 aminoacids), which resembles that of ANXA7, with a 3D structure that differs from the typical amphipathic helix motifs present in N-terminal domains from other family members. Together with ANXA7 and ANXA13, these are the earliest vertebrate annexins, and are considered the ancestor of the remaining annexin members (Gerke and Moss, 2002).
This protein is tightly involved in Ca2+-regulated exocytic processes, interrupting midbody formation during cytokinesis (and hence cell cycle progression), sex differentiation throughout gonad development and apoptosis regulation. Of note, ANXA11 interaction with S100A6 protein (calcyclin) is especially relevant for the aforementioned biological functions (Wang et al., 2014). As many other annexins, ANXA11 is involved in membrane curvature mechanisms, similar to ANXA7, and both proteins share their unique ability to form mobile lens structures in the bilayer (Boye et al., 2018).
ANXA11 dysfunction has been associated with several human diseases such as autoimmune diseases and thrombolysis (Wang et al., 2014), and especially with amyotrophic lateral sclerosis (ALS) (Siddique and T, 2001; Smith et al., 2017). Regarding human cancers, ANXA11 has been related to drug resistance and metastasis in several human malignancies, as discussed below.
ANXA11 acts as a tumor-suppressor in hepatocarcinoma regulating apoptosis, invasion and lymph node metastases. ANXA11 downregulation led to reduced apoptosis and enhanced invasion and metastatic abilities, as well as enhanced chemoresistance to 5-FU in both in vitro and in vivo models (Liu et al., 2015; Liu et al., 2016 S.). In bladder cancer, ANXA11 expression levels were decreased in tumor tissue compared to the normal counterpart, and together with other annexins, serves to identify luminal-subtype bladder tumors (Wu et al., 2021). Moreover, high ANXA11 levels were associated with a higher overall survival in bladder cancer patients (Yao et al., 2022), further supporting an oncosuppressor role in this malignancy.
ANXA11 was found upregulated in gastric tumors compared to normal tissue, and increased ANXA11 levels were significantly associated with tumor size and infiltration, lymph node metastasis, TNM stage and vascular invasion, emerging as a bad prognosis factor and a potential therapeutic target for this cancer type. In vitro ANXA11 silencing reduced cell proliferation and invasion and migration capabilities, supporting a protumor role in gastric cancer (Hua et al., 2018).
ANXA11 has been involved in chemotherapy resistance in several malignancies. Downregulated ANXA11 expression is associated with cisplatin resistance in ovarian cancer, and pointed as a useful biomarker to predict chemoresistance to platinum-based therapies and early tumor recurrence (Song et al., 2007). Given that cisplatin is the gold-standard therapy for HNC patients, it would be interesting to explore the possible implication of ANXA11 in HNC chemoresistance, and its potential as a predictive biomarker of cisplatin response. Even though ANXA11 expression has not been detected in HNC tissue samples (Song et al., 2007), the small sample size in the only published study demands further investigation.
ANXA11 has also been associated with chemotherapy response in metastatic colorectal cancer, and ANXA11 nsSNP rs1049550 has been proposed as a biomarker to predict bevacizumab sensitivity in these patients (Kim et al., 2011, 2013; Roh et al., 2016). ANXA11 is overexpressed in colon primary tumors compared to normal colon, and associated with tumor stage. Moreover, patients harboring high ANXA11 and ANXA4 expression displayed poorer survival than those with low annexin expression (Duncan et al., 2008). High ANXA11 mRNA levels have also been associated to a poor overall survival in patients with acute myeloid leukemia, and related to a resistance mechanism to DR5-targeting agents that induce apoptosis regulation (Song et al., 2022). Since ANXA11 plays a role in apoptosis modulation, it could represent an important player in cancer therapies based on targeting apoptotic pathways and/or the development of resistance mechanisms, and hence emerge as a valuable biomarker to predict treatment response or to identify/stratify the subset of patients who are likely to respond (Song et al., 2022).
Annexin A13
Annexin A13 (ANXA13) is the earliest annexin ancestor in vertebrates. Its expression is tightly restricted to intestine, and transcribed as two isoforms by alternative splicing (named A13a and A13b) (Iglesias et al., 2001; Gerke and Moss, 2002). Of note, it is the only member myristoylated at its N-terminal domain, allowing specific membrane association features that may act synergistically with canonical (Gerke and Moss, 2002; Turnay et al., 2005; McCulloch et al., 2019). Like other annexins, ANXA13 is involved in membrane biology, being of special relevance in lipid-raft dynamics involved in apical transport (Lafont et al., 1998; Plant et al., 2000).
In cancer, high ANXA13 expression has been associated with a poor outcome in colorectal cancer (Jiang et al., 2017), and lung adenocarcinoma where it promotes cell migration, invasion and EMT (Xue et al., 2020). To date, the clinical significance of ANXA13 in HNC remains unexplored.
Concluding remarks
According to the herein reviewed data, dysregulation of annexin expression is a common feature in multiple cancers (Table 1 and Figure 2) that causes widespread functional effects on multiple cellular processes critical for tumor biology, thereby affecting major hallmarks of cancer (Table 2 and Figure 3). Hence, it is not surprising that this family of proteins offers enormous opportunities for the identification of clinically relevant biomarkers and novel molecular targets for the development of personalized therapies. In fact, this review compiles comprehensive information evidencing the great potential of annexins as biomarkers for cancer diagnosis, prognosis, disease monitoring, prediction of treatment response and/or therapeutic targets (summarized in Table 3). Nevertheless, given that each protein exhibits varying expression levels and phenotypic specificity depending on the tumor type, in-depth functional and mechanistic characterization of annexin dysregulation for each individual cancer poses a fundamental task to safety translate this knowledge into clinical application.
Annexins display different expression patterns, some of them are found upregulated in certain tumor types while downregulated in others, and such imbalance may trigger a variety of downstream signaling pathways and functional regulatory effects. In addition, annexins show important differences in their calcium binding ability, subcellular locations, post-translational modifications and a high number of interaction partners. All these factors jointly contribute to complicate their precise functions in each cell and tissue. Ultimately, annexins cannot be overall classified as an oncogene or a tumor suppressor, they should actually be considered as double-edged swords depending on the cellular or tissue context.
HNC encompasses a complex and heterogeneous group of aggressive malignancies, whose main pitfalls are late diagnosis, poor prognostication, scarce molecular-targeted therapies, and drug resistance. Therefore, annexin research represents a growing area of interest to attempt to overcome the major challenges of this disease. Based on the existing published data, ANXA1 and ANXA2 are the most extensively investigated, revealed as both clinically and biologically relevant and, as such, highly promising for clinical application. It has been demonstrated that these two proteins participate in numerous hallmarks of cancer (Figure 3), which highlights their central role as key players and possible targets for therapy in HNC as in other cancers.
Even though targeted therapies based on annexin dysfunctions are not yet available for cancer treatment, annexins are potentially druggable targets that could be therapeutically exploited. Precisely, various strategies have already been developed successfully targeting ANXA1 and ANXA2 functions, which have shown efficacy on various preclinical tumor models. It is therefore strongly recommended to further extend testing to HNC models. In particular, we may anticipate that ANXA2 blocking strategies might be adequate in NPC and OSCC to specifically target its oncopromoter role, but not in other HNSCC subtypes, such as laryngeal and pharyngeal carcinomas, where ANXA2 expression is downregulated and oncosuppressive. This should also be taken into account when eventually designing clinical trials in the future, in order to adequately define the inclusion criteria, to optimize patients’ stratification and enrollment, and ultimately to maximize clinical efficacy as well as patient benefit and safety. Similarly, the ANXA1 mimetic peptide Ac 2-26 (with reported cardioprotective effects) could also represent a valuable therapeutic option for some HNC subtypes; however, this possibility requires in-depth functional characterization in disease-relevant preclinical models.
It is worth highlighting that ANXA1 and ANXA2 have been linked to radio-chemoresistance and immunosuppression in HNC and other cancers, which poses a plausible application as adjuvant treatments to jointly improve treatment response and antitumor immune response. Nevertheless, the mechanisms responsible for these immunomodulatory actions need a more precise elucidation, since different results have been reported depending on the experimental settings and cellular models tested. Other family members (i.e., ANXA4, ANXA5, and ANXA7) have been associated to treatment resistance mechanisms in HNC, which also merits deeper investigation.
On the other hand, from a biomarker point of view, various annexins have been pointed as potential diagnostic, prognostic and/or predictive biomarkers in HNC. Of great interest and application, results revealing ANXA1 lost as an early marker to facilitate histopathological diagnosis of epithelial dysplasia (Garcia Pedrero et al., 2004). This molecular test based on immunohistochemical detection has shown a 100% sensitivity, and it could be easily implemented to routine clinical practice. ANXA1 (Garcia Pedrero et al., 2004), A2 (Rodrigo et al., 2011b, 2014) and A9 (Salom et al., 2019) expression has been closely related to histopathological grading in HNSCC. Interestingly, since ANXA1 can be externalized (Cooray et al., 2013; Gastardelo et al., 2014), its expression is detectable in liquid biopsy, changes upon treatment, and ANXA1 mRNA levels have been proposed as a blood-based biomarker for OSCC detection (Faria et al., 2010). In addition, ANXA8 mRNA expression levels have been postulated as a biomarker to detect OSCC lymph node metastases histopathologically undetectable (Oka et al., 2016).
Author contributions
LP-F, SM, and SA-T contributed to the writing of the manuscript and the preparation of figures/tables. MO-R and FH-P contributed to the analysis of TCGA data and preparation of figures/tables. IM-J contributed to the writing of the manuscript. JG-P contributed to the writing and revision of the manuscript and figures/tables and providing funding support. All authors read and approved the final version of the manuscript.
Funding
This study was supported by the Instituto de Salud Carlos III (ISCIII) through the project grants PI19/00560 and CIBERONC (CB16/12/00390) and co-funded by the European Union; by FINBA-ISPA through the project grant 2021-047-INTRAMURAL NOV-ALTES (sponsored by Janssen), the Instituto de Investigación Sanitaria del Principado de Asturias (ISPA), Fundación Bancaria Cajastur-IUOPA, and Universidad de Oviedo. Also through the grant “Ayudas para Grupos de Investigación de Organismos del Principado de Asturias 2021–2023” (IDI/2021/000079), funded by Principado de Asturias through FICYT and the FEDER Funding Program from the European Union. SA-T is recipient of a Sara Borrell postdoctoral fellowship from ISCIII (CD20/00006), LP-F is recipient of an FPU-PhD fellowship from the Spanish Ministry of Education (FPU20/01588), and IM-J recipient of a Severo Ochoa predoctoral fellowship from the Principado de Asturias (BP20-152).
Conflict of interest
The authors declare that the research was conducted in the absence of any commercial or financial relationships that could be construed as a potential conflict of interest.
Publisher’s note
All claims expressed in this article are solely those of the authors and do not necessarily represent those of their affiliated organizations, or those of the publisher, the editors and the reviewers. Any product that may be evaluated in this article, or claim that may be made by its manufacturer, is not guaranteed or endorsed by the publisher.
References
Alldridge, L. C., and Bryant, C. E. (2003). Annexin 1 regulates cell proliferation by disruption of cell morphology and inhibition of cyclin D1 expression through sustained activation of the ERK1/2 MAPK signal. Exp. Cell Res. 290, 93–107. doi:10.1016/S0014-4827(03)00310-0
Álvarez-Teijeiro, S., Menéndez, S. T., Villaronga, M. Á., Pena-Alonso, E., Rodrigo, J. P., Morgan, R. O., et al. (2017a). Annexin A1 down-regulation in head and neck squamous cell carcinoma is mediated via transcriptional control with direct involvement of miR-196a/b. Sci. Rep. 7, 6790. doi:10.1038/s41598-017-07169-w
Álvarez-Teijeiro, S., Menéndez, S. T., Villaronga, M. Á., Rodrigo, J. P., Manterola, L., De Villalaín, L., et al. (2017b). Dysregulation of miR-196b in head and neck cancers leads to pleiotropic effects in the tumor cells and surrounding stromal fibroblasts. Sci. Rep. 7, 17785. doi:10.1038/s41598-017-18138-8
Anbalagan, D., Yap, G., Yuan, Y., Pandey, V. K., Lau, W. H., Arora, S., et al. (2014). Annexin-A1 regulates MicroRNA-26b∗ and MicroRNA-562 to directly target NF-κB and angiogenesis in breast cancer cells. PLoS One 9, e114507. doi:10.1371/journal.pone.0114507
Andey, T., Marepally, S., Patel, A., Jackson, T., Sarkar, S., O’Connell, M., et al. (2014). Cationic lipid guided short-hairpin RNA interference of annexin A2 attenuates tumor growth and metastasis in a mouse lung cancer stem cell model. J. Control. Release 184, 67–78. doi:10.1016/j.jconrel.2014.03.049
Araújo, T. G., Mota, S. T. S., Ferreira, H. S. V., Ribeiro, M. A., Goulart, L. R., and Vecchi, L. (2021). Annexin a1 as a regulator of immune response in cancer. Cells. doi:10.3390/cells10092245
Bae, J. M., Kim, J. H., Rhee, Y. Y., Cho, N. Y., Kim, T. Y., and Kang, G. H. (2015). Annexin A10 expression in colorectal cancers with emphasis on the serrated neoplasia pathway. World J. Gastroenterol. 21, 9749–9757. doi:10.3748/wjg.v21.i33.9749
Bajrai, L. H., Sohrab, S. S., Mobashir, M., Kamal, M. A., Rizvi, M. A., and Azhar, E. I. (2021). Understanding the role of potential pathways and its components including hypoxia and immune system in case of oral cancer. Sci. Rep. 11, 19576. doi:10.1038/s41598-021-98031-7
Bao, H., Jiang, M., Zhu, M., Sheng, F., Ruan, J., and Ruan, C. (2009). Overexpression of Annexin II affects the proliferation, apoptosis, invasion and production of proangiogenic factors in multiple myeloma. Int. J. Hematol. 90, 177–185. doi:10.1007/s12185-009-0356-8
Beebe-Dimmer, J. L., and Cooney, K. A. (2019). Mitochondrial alterations may underlie race-specific differences in cancer risk and outcome. J. Clin. Invest. 129, 2187–2188. doi:10.1172/JCI128707
Benaud, C., Gentil, B. J., Assard, N., Court, M., Garin, J., Delphin, C., et al. (2004). AHNAK interaction with the annexin 2/S100A10 complex regulates cell membrane cytoarchitecture. J. Cell Biol. 164, 133–144. doi:10.1083/jcb.200307098
Bezerra, N. V. F., Leite, K. L. de F., de Medeiros, M. M. D., Martins, M. L., Cardoso, A. M. R., Alves, P. M., et al. (2018). Impact of the anatomical location, alcoholism and smoking on the prevalence of advanced oral cancer in Brazil. Med. Oral Patol. Oral Cir. Bucal 23, e295–e301. doi:10.4317/medoral.22318
Bianchi, C., Bombelli, S., Raimondo, F., Torsello, B., Angeloni, V., Ferrero, S., et al. (2010). Primary cell cultures from human renal cortex and renal-cell carcinoma evidence a differential expression of two spliced isoforms of annexin A3. Am. J. Pathol. 176, 1660–1670. doi:10.2353/ajpath.2010.090402
Birge, R. B., Boeltz, S., Kumar, S., Carlson, J., Wanderley, J., Calianese, D., et al. (2016). Phosphatidylserine is a global immunosuppressive signal in efferocytosis, infectious disease, and cancer. Cell Death Differ. 23, 962–978. doi:10.1038/cdd.2016.11
Bist, P., Phua, Q. H., Shu, S., Yi, Y., Anbalagan, D., Lee, L. H., et al. (2015). Annexin-A1 controls an ERK-RhoA-NFκB activation loop in breast cancer cells. Biochem. Biophys. Res. Commun. 461, 47–53. doi:10.1016/j.bbrc.2015.03.166
Boczonadi, V., and Määttä, A. (2012). Annexin A9 is a periplakin interacting partner in membrane-targeted cytoskeletal linker protein complexes. FEBS Lett. 586, 3090–3096. doi:10.1016/j.febslet.2012.07.057
Borkotoky, R., Malakar, B., Saikia, A. K., Mukherjee, S. N., and Bose, S. (2020). Alterations in XRCC1 gene profile in pathogenesis of oral cavity cancer: A population-based study from kamrup urban district of Assam, India. Curr. Probl. Cancer 44, 100473. doi:10.1016/j.currproblcancer.2019.03.003
Boudhraa, Z., Bouchon, B., Viallard, C., D’Incan, M., and Degoul, F. (2016). Annexin A1 localization and its relevance to cancer. Clin. Sci. 130, 205–220. doi:10.1042/CS20150415
Bouter, A., Carmeille, R., Gounou, C., Bouvet, F., Degrelle, S. A., Evain-Brion, D., et al. (2015). Review: Annexin-A5 and cell membrane repair. Placenta 36, S43–S49. doi:10.1016/j.placenta.2015.01.193
Bouvet, F., Ros, M., Bonedeau, E., Croissant, C., Frelin, L., Saltel, F., et al. (2020). Defective membrane repair machinery impairs survival of invasive cancer cells. Sci. Rep. 10, 21821. doi:10.1038/s41598-020-77902-5
Boye, T. L., Jeppesen, J. C., Maeda, K., Pezeshkian, W., Solovyeva, V., Nylandsted, J., et al. (2018). Annexins induce curvature on free-edge membranes displaying distinct morphologies. Sci. Rep. 8, 10309–10314. doi:10.1038/s41598-018-28481-z
Boye, T. L., Maeda, K., Pezeshkian, W., Sønder, S. L., Haeger, S. C., Gerke, V., et al. (2017). Annexin A4 and A6 induce membrane curvature and constriction during cell membrane repair. Nat. Commun. 8, 1623. doi:10.1038/s41467-017-01743-6
Buckingham, J. C., John, C. D., Solito, E., Tierney, T., Flower, R. J., Christian, H., et al. (2006). “Annexin 1, glucocorticoids, and the neuroendocrine-immune interface,” in Annals of the New York Academy of sciences. doi:10.1196/annals.1366.002
Buzhynskyy, N., Golczak, M., Lai-kee-him, J., Lambert, O., Tessier, B., Gounou, C., et al. (2009). Annexin-A6 presents two modes of association with phospholipid membranes . A combined QCM-D , AFM and cryo-TEM study. J. Struct. Biol. 168, 107–116. doi:10.1016/j.jsb.2009.03.007
Cardin, L. T., Prates, J., da Cunha, B. R., Tajara, E. H., Oliani, S. M., and Rodrigues-Lisoni, F. C. (2019). Annexin A1 peptide and endothelial cell-conditioned medium modulate cervical tumorigenesis. FEBS Open Bio 9, 668–681. doi:10.1002/2211-5463.12603
Chang, K., Wang, G., Freireich, E., Daly, M., Naylor, S., Trujillo, J., et al. (1992). Specific expression of the annexin VIII gene in acute promyelocytic leukemia. Blood 79, 1802–1810. doi:10.1182/blood.v79.7.1802.1802
Chao, A., Wang, T. H., Lee, Y. S., Hsueh, S., Chao, A. S., Chang, T. C., et al. (2006). Molecular characterization of adenocarcinoma and squamous carcinoma of the uterine cervix using microarray analysis of gene expression. Int. J. Cancer 119, 91–98. doi:10.1002/ijc.21813
Chao, P. Z., Hsieh, M. S., Cheng, C. W., Hsu, T. J., Lin, Y. T., Lai, C. H., et al. (2015). Dendritic cells respond to nasopharygeal carcinoma cells through annexin A2-recognizing DC-SIGN. Oncotarget 6, 159–170. doi:10.18632/oncotarget.2700
Chen, C. Y., Lin, Y. S., Chen, C. L., Chao, P. Z., Chiou, J. F., Kuo, C. C., et al. (2015). Targeting annexin A2 reduces tumorigenesis and therapeutic resistance of nasopharyngeal carcinoma. Oncotarget 6, 26946–26959. doi:10.18632/oncotarget.4521
Choi, C. H., Sung, C. O., Kim, H. J., Lee, Y. Y., Song, S. Y., Song, T., et al. (2013). Overexpression of annexin A4 is associated with chemoresistance in papillary serous adenocarcinoma of the ovary. Hum. Pathol. 44, 1017–1023. doi:10.1016/j.humpath.2012.08.024
Chong, K. W. Y., Chen, M. J., Koay, E. S. C., Wong, B. S., Lee, A. Y. W., Russo-Marie, F., et al. (2010). Annexin A3 is associated with cell death in lactacystin-mediated neuronal injury. Neurosci. Lett. 485, 129–133. doi:10.1016/j.neulet.2010.08.089
Chopra, A., Shan, L., Eckelman, W. C., Leung, K., Latterner, M., Bryant, S. H., et al. (2012). Molecular imaging and contrast agent database (MICAD): Evolution and progress. Mol. Imaging Biol. 14, 4–13. doi:10.1007/s11307-011-0521-3
Cohen, E. E. W., Soulières, D., Le Tourneau, C., Dinis, J., Licitra, L., Ahn, M. J., et al. (2019). Pembrolizumab versus methotrexate, docetaxel, or cetuximab for recurrent or metastatic head-and-neck squamous cell carcinoma (KEYNOTE-040): A randomised, open-label, phase 3 study. Lancet 393, 156–167. doi:10.1016/S0140-6736(18)31999-8
Coméra, C., Rothhut, B., and Russo-Marie, F. (1990). Identification and characterization of phospholipase A2 inhibitory proteins in human mononuclear cells. Eur. J. Biochem. 188, 139–146. doi:10.1111/j.1432-1033.1990.tb15381.x
Cooray, S. N., Gobbetti, T., Montero-Melendez, T., McArthur, S., Thompson, D., Clark, A. J. L., et al. (2013). Ligand-specific conformational change of the G-protein-coupled receptor ALX/FPR2 determines proresolving functional responses. Proc. Natl. Acad. Sci. U. S. A. 110, 18232–18237. doi:10.1073/pnas.1308253110
Cornely, R., Rentero, C., Enrich, C., Grewal, T., and Gaus, K. (2011). Annexin A6 is an organizer of membrane microdomains to regulate receptor localization and signalling. IUBMB Life 63, 1009–1017. doi:10.1002/iub.540
Cosphiadi, I., Atmakusumah, T. D., Siregar, N. C., Muthalib, A., Harahap, A., and Mansyur, M. (2018). Bone metastasis in advanced breast cancer: Analysis of gene expression microarray. Clin. Breast Cancer 18, e1117–e1122. doi:10.1016/j.clbc.2018.03.001
Cramer, J. D., Burtness, B., and Ferris, R. L. (2019). Immunotherapy for head and neck cancer: Recent advances and future directions. Oral Oncol. 99, 104460. doi:10.1016/j.oraloncology.2019.104460
Creutz, C. E. (1992). The annexins and exocytosis. Science 258, 924–931. doi:10.1126/science.1439804
Cui, H. Y., Wang, S. J., Miao, J. Y., Fu, Z. G., Feng, F., Wu, J., et al. (2016). CD147 regulates cancer migration via direct interaction with Annexin A2 and DOCK3-β-catenin-WAVE2 signaling. Oncotarget 7, 5613–5629. doi:10.18632/oncotarget.6723
D’Acquisto, F., Perretti, M., and Flower, R. J. (2008). Annexin-A1: A pivotal regulator of the innate and adaptive immune systems. Br. J. Pharmacol. 155, 152–169. doi:10.1038/bjp.2008.252
Dalli, J., Jones, C. P., Cavalcanti, D. M., Farsky, S. H., Perretti, M., and Rankin, S. M. (2012). Annexin A1 regulates neutrophil clearance by macrophages in the mouse bone marrow. FASEB J. 26, 387–396. doi:10.1096/fj.11-182089
Davidson, J., Flower, R. J., Milton, A. S., Peers, S. H., and Rotondo, D. (1991). Antipyretic actions of human recombinant lipocortin-1. Br. J. Pharmacol. 102, 7–9. doi:10.1111/j.1476-5381.1991.tb12122.x
de Graauw, M., Tijdens, I., Smeets, M. B., Hensbergen, P. J., Deelder, A. M., and van de Water, B. (2008). Annexin A2 phosphorylation mediates cell scattering and branching morphogenesis via cofilin activation. Mol. Cell. Biol. 28, 1029–1040. doi:10.1128/mcb.01247-07
Deng, S., Wang, J., Hou, L., Li, J., Chen, G., Jing, B., et al. (2012). Annexin A1, A2, A4 and A5 play important roles in breast cancer, pancreatic cancer and laryngeal carcinoma, alone and/or synergistically. Oncol. Lett. 5, 107–112. doi:10.3892/ol.2012.959
Deora, A. B., Kreitzer, G., Jacovina, A. T., and Hajjar, K. A. (2004). An annexin 2 phosphorylation switch mediates p11-dependent translocation of annexin 2 to the cell surface. J. Biol. Chem. 279, 43411–43418. doi:10.1074/jbc.M408078200
Ding, X. M., Li, J. X., Wang, K., Wu, Z. S., Yao, A. H., Jiao, C. Y., et al. (2017). Effects of silencing annexin A5 on proliferation and invasion of human cholangiocarcinoma cell line. Eur. Rev. Med. Pharmacol. Sci. 21, 1477–1488.
Donadio, E., Giusti, L., Seccia, V., Ciregia, F., da Valle, Y., Dallan, I., et al. (2013). New insight into benign tumours of major salivary glands by proteomic approach. PLoS One 8, e71874. doi:10.1371/journal.pone.0071874
Draeger, A., Monastyrskaya, K., and Babiychuk, E. B. (2011). Plasma membrane repair and cellular damage control: The annexin survival kit. Biochem. Pharmacol. 81, 703–712. doi:10.1016/j.bcp.2010.12.027
Duncan, R., Carpenter, B., Main, L. C., Telfer, C., and Murray, G. I. (2008). Characterisation and protein expression profiling of annexins in colorectal cancer. Br. J. Cancer 98, 426–433. doi:10.1038/sj.bjc.6604128
Faria, P. C. B., Sena, A. A. S., Nascimento, R., Carvalho, W. J., Loyola, A. M., Silva, S. J., et al. (2010). Expression of annexin A1 mRNA in peripheral blood from oral squamous cell carcinoma patients. Oral Oncol. 46, 25–30. doi:10.1016/j.oraloncology.2009.09.003
Faugaret, D., Chouinard, F. C., Harbour, D., El azreq, M. A., and Bourgoin, S. G. (2011). An essential role for phospholipase D in the recruitment of vesicle amine transport protein-1 to membranes in human neutrophils. Biochem. Pharmacol. 81, 144–156. doi:10.1016/j.bcp.2010.09.014
Feng, J. G., Liu, Q., Qin, X., Geng, Y. H., Zheng, S. T., Liu, T., et al. (2012). Clinicopathological pattern and Annexin A2 and Cdc42 status in patients presenting with differentiation and lymphnode metastasis of esophageal squamous cell carcinomas. Mol. Biol. Rep. 39, 1267–1274. doi:10.1007/s11033-011-0859-2
Feng, J., Lu, S. S., Xiao, T., Huang, W., Yi, H., Zhu, W., et al. (2021). ANXA1 binds and stabilizes EphA2 to promote nasopharyngeal carcinoma growth and metastasis. Cancer Res. 80, 4386–4398. doi:10.1158/0008-5472.CAN-20-0560
Feng, X., Liu, H., Zhang, Z., Gu, Y., Qiu, H., and He, Z. (2017). Annexin A2 contributes to cisplatin resistance by activation of JNK-p53 pathway in non-small cell lung cancer cells. J. Exp. Clin. Cancer Res. 36, 123. doi:10.1186/s13046-017-0594-1
Fernandez, M. P., and Morgan, R. O. (2003). Structure, function and evolution of the annexin gene superfamily. doi:10.1007/978-1-4419-9214-7_2
Filipenko, N. R., and Waisman, D. M. (2001). The C terminus of annexin II mediates binding to F-actin. J. Biol. Chem. 276, 5310–5315. doi:10.1074/jbc.M009710200
Florentsen, C. D., Kamp-Sonne, A., Moreno-Pescador, G., Pezeshkian, W., Hakami Zanjani, A. A., Khandelia, H., et al. (2021). Annexin A4 trimers are recruited by high membrane curvatures in giant plasma membrane vesicles. Soft Matter 17, 308–318. doi:10.1039/d0sm00241k
Fredman, G., Kamaly, N., Spolitu, S., Milton, J., Ghorpade, D., Chiasson, R., et al. (2015). Targeted nanoparticles containing the proresolving peptide Ac2-26 protect against advanced atherosclerosis in hypercholesterolemic mice. Sci. Transl. Med. 7, 275ra20. doi:10.1126/scitranslmed.aaa1065
Fu, Z., Zhang, S., Wang, B., Huang, W., Zheng, L., and Cheng, A. (2020). Annexin A1: A double-edged sword as novel cancer biomarker. Clin. Chim. Acta. 504, 36–42. doi:10.1016/j.cca.2020.01.022
Garcia Pedrero, J. M., Fernandez, M. P., Morgan, R. O., Herrero Zapatero, A., Gonzalez, M. V., Suarez Nieto, C., et al. (2004). Annexin A1 down-regulation in head and neck cancer is associated with epithelial differentiation status. Am. J. Pathol. 164, 73–79. doi:10.1016/S0002-9440(10)63098-2
Gastardelo, T. S., Cunha, B. R., Raposo, L. S. R., Maniglia, J. V., Cury, P. M., Lisoni, F. V. C. R., et al. (2014). Inflammation and cancer: Role of annexin a1 and FPR2/ALX in proliferation and metastasis in human laryngeal squamous cell carcinoma. PLoS One 9, e111317. doi:10.1371/journal.pone.0111317
Gaudio, E., Paduano, F., Ngankeu, A., Ortuso, F., Lovat, F., Pinton, S., et al. (2016). A Fhit-mimetic peptide suppresses annexin A4-mediated chemoresistance to paclitaxel in lung cancer cells. Oncotarget 7, 29927–29936. doi:10.18632/oncotarget.9179
Gerke, V., Creutz, C. E., and Moss, S. E. (2005). Annexins: Linking Ca2+ signalling to membrane dynamics. Nat. Rev. Mol. Cell Biol. 6, 449–461. doi:10.1038/nrm1661
Gerke, V., and Moss, S. E. (2002). Annexins : From structure to function. Physiol. Rev. 82, 331–371. doi:10.1152/physrev.00030.2001
Gillette, J. M., Chan, D. C., and Nielsen-Preiss, S. M. (2004). Annexin 2 expression is reduced in human osteosarcoma metastases. J. Cell. Biochem. 92, 820–832. doi:10.1002/jcb.20117
Gobbetti, T., and Cooray, S. N. (2016). Annexin A1 and resolution of inflammation: Tissue repairing properties and signalling signature. Biol. Chem. 397, 981–993. doi:10.1515/hsz-2016-0200
Goebeler, V., Poeter, M., Zeuschner, D., Gerke, V., and Rescher, U. (2008). Annexin A8 regulates late endosome organization and function. Mol. Biol. Cell 19, 5267–5278. doi:10.1091/mbc.e08-04-0383
Goebeler, V., Ruhe, D., Gerke, V., and Rescher, U. (2006). Annexin A8 displays unique phospholipid and F-actin binding properties. FEBS Lett. 580, 2430–2434. doi:10.1016/j.febslet.2006.03.076
Goebeler, V., Ruhe, D., Gerke, V., and Rescher, U. (2003). Atypical properties displayed by annexin A9, a novel member of the annexin family of Ca2+ and lipid binding proteins. FEBS Lett. 546, 359–364. doi:10.1016/S0014-5793(03)00634-3
Goldman, M. J., Craft, B., Hastie, M., Repečka, K., McDade, F., Kamath, A., et al. (2020). Visualizing and interpreting cancer genomics data via the Xena platform. Nat. Biotechnol. 38, 675–678. doi:10.1038/s41587-020-0546-8
Gou, R., Zhu, L., Zheng, M., Guo, Q., Hu, Y., Li, X., et al. (2019). Annexin A8 can serve as potential prognostic biomarker and therapeutic target for ovarian cancer: Based on the comprehensive analysis of Annexins. J. Transl. Med. 17, 275–296. doi:10.1186/s12967-019-2023-z
Grewal, T., Evans, R., Rentero, C., Tebar, F., Cubells, L., De Diego, I., et al. (2005). Annexin A6 stimulates the membrane recruitment of p120GAP to modulate Ras and Raf-1 activity. Oncogene 24, 5809–5820. doi:10.1038/sj.onc.1208743
Grewal, T., Hoque, M., Conway, J. R. W., Reverter, M., Wahba, M., Beevi, S. S., et al. (2017). Annexin A6—a multifunctional scaffold in cell motility. Cell adh. Migr. 11, 288–304. doi:10.1080/19336918.2016.1268318
Grewal, T., Wason, S. J., Enrich, C., and Rentero, C. (2016). Annexins-insights from knockout mice. Biol. Chem. 397, 1031–1053. doi:10.1515/hsz-2016-0168
Grindheim, A. K., Hollås, H., Raddum, A. M., Saraste, J., and Vedeler, A. (2016). Reactive oxygen species exert opposite effects on Tyr23 phosphorylation of the nuclear and cortical pools of annexin A2. J. Cell Sci. 129, 314–328. doi:10.1242/jcs.173195
Griner, N. B., Young, D., Chaudhary, P., Mohamed, A. A., Huang, W., Chen, Y., et al. (2015). ERG oncoprotein inhibits ANXA2 expression and function in prostate cancer. Mol. Cancer Res. 13, 368–379. doi:10.1158/1541-7786.MCR-14-0275-T
Guan, X., Fang, Y., Long, J., and Zhang, Y. (2019). Annexin 1–nuclear factor-κB–microRNA-26a regulatory pathway in the metastasis of non-small cell lung cancer. Thorac. Cancer 10, 665–675. doi:10.1111/1759-7714.12982
Gui, S. J., Ding, R. L., Wan, Y. P., Zhou, L., Chen, X. J., Zeng, G. Q., et al. (2020). Knockdown of annexin VII enhances nasopharyngeal carcinoma cell radiosensitivity in vivo and in vitro. Cancer Biomark. 28, 129–139. doi:10.3233/CBM-190739
Guo, C., Liu, S., Greenaway, F., and Sun, M. Z. (2013a). Potential role of annexin A7 in cancers. Clin. Chim. Acta. 423, 83–89. doi:10.1016/j.cca.2013.04.018
Guo, C., Liu, S., and Sun, M. Z. (2013b). Potential role of Anxa1 in cancer. Future Oncol. 9, 1773–1793. doi:10.2217/fon.13.114
Guo, Z., Guo, A., and Zhou, C. (2021). Breast cancer stem cell-derived ANXA6-containing exosomes sustain paclitaxel resistance and cancer aggressiveness in breast cancer. Front. Cell Dev. Biol. 9, 718721–718732. doi:10.3389/fcell.2021.718721
Han, G. H., Lu, K. J., Huang, J. X., Zhang, L. X., Dai, S. Bin, and Dai, C. L. (2018). Association of serum annexin A1 with treatment response and prognosis in patients with esophageal squamous cell carcinoma. J. Cancer Res. Ther. 14, S667–S674. doi:10.4103/0973-1482.187297
Hannon, R., Croxtall, J. D., Getting, S. J., Roviezzo, F., Yona, S., Paul-Clark, M. J., et al. (2003). Aberrant inflammation and resistance to glucocorticoids in annexin 1-/- mouse. FASEB J. 17, 253–255. doi:10.1096/fj.02-0239fje
Harder, T., and Gerke, V. (1993). The subcellular distribution of early endosomes is affected by the annexin II2p112 complex. J. Cell Biol. 123, 1119–1132. doi:10.1083/jcb.123.5.1119
Hayes, M. J., Longbottom, R. E., Evans, M. A., and Moss, S. E. (2007). Annexinopathies. Annex. Subcell. Biochem. 45, 1–28. doi:10.1007/978-1-4020-6191-2_1
Hayes, M. J., and Moss, S. E. (2009). Annexin 2 has a dual role as regulator and effector of v-Src in cell transformation. J. Biol. Chem. 284, 10202–10210. doi:10.1074/jbc.M807043200
Hayes, M. J., Rescher, U., Gerke, V., and Moss, S. E. (2004). Annexin-Actin interactions. Traffic 5, 571–576. doi:10.1111/j.1600-0854.2004.00210.x
Hedhli, N., Falcone, D. J., Huang, B., Cesarman-maus, G., Kraemer, R., Zhai, H., et al. (2012). The annexin A2/S100A10 system in health and disease : Emerging paradigms. J. Biomed. Biotechnol. 2012, 406273. doi:10.1155/2012/406273
Heinick, A., Husser, X., Himmler, K., Kirchhefer, U., Nunes, F., Schulte, J. S., et al. (2015). Annexin A4 is a novel direct regulator of adenylyl cyclase type 5. FASEB J. 29, 3773–3787. doi:10.1096/fj.14-269837
Heinick, A., Pluteanu, F., Hermes, C., Klemme, A., Domnik, M., Husser, X., et al. (2020). Annexin A4 N-terminal peptide inhibits adenylyl cyclase 5 and limits β-adrenoceptor-mediated prolongation of cardiac action potential. FASEB J. 34, 10489–10504. doi:10.1096/fj.201902094RR
Heitzig, N., Brinkmann, B. F., Koerdt, S. N., Rosso, G., Shahin, V., and Rescher, U. (2017). Annexin A8 promotes VEGF-A driven endothelial cell sprouting. Cell adh. Migr. 11, 275–287. doi:10.1080/19336918.2016.1264559
Hippo, Y., Yashiro, M., Ishii, M., Taniguchi, H., Tsutsumi, S., Hirakawa, K., et al. (2001). Differential gene expression profiles of scirrhous gastric cancer cells with high metastatic potential to peritoneum or lymph nodes. Cancer Res. 61, 889–895.
Hoque, M., Elmaghrabi, Y. A., Köse, M., Beevi, S. S., Jose, J., Meneses-Salas, E., et al. (2020). Annexin A6 improves anti-migratory and anti-invasive properties of tyrosine kinase inhibitors in EGFR overexpressing human squamous epithelial cells. FEBS J. 287, 2961–2978. doi:10.1111/febs.15186
Hsu, P. I., Huang, M. S., Chen, H. C., Hsu, P. N., Lai, T. C., Wang, J. L., et al. (2008). The significance of ANXA7 expression and its correlation with poor cellular differentiation and enhanced metastatic potential of gastric cancer. J. Surg. Oncol. 97, 609–614. doi:10.1002/jso.21046
Hua, K., Li, Y., Zhao, Q., Fan, L., Tan, B., and Gu, J. (2018). Downregulation of annexin A11 (ANXA11) inhibits cell proliferation, invasion, and migration via the AKT/GSK-3β pathway in gastric cancer. Med. Sci. Monit. 24, 149–160. doi:10.12659/MSM.905372
Huang, L., Liao, L., Wan, Y., Cheng, A., Li, M., Chen, S., et al. (2016). Downregulation of annexin A1 is correlated with radioresistance in nasopharyngeal carcinoma. Oncol. Lett. 12, 5229–5234. doi:10.3892/ol.2016.5324
Hübbers, C. U., and Akgül, B. (2015). HPV and cancer of the oral cavity. Virulence 6, 244–248. doi:10.1080/21505594.2014.999570
Hummerich, L., Müller, R., Hess, J., Kokocinski, F., Hahn, M., Fürstenberger, G., et al. (2006). Identification of novel tumour-associated genes differentially expressed in the process of squamous cell cancer development. Oncogene 25, 111–121. doi:10.1038/sj.onc.1209016
Hung, K.-S., and Howng, S.-L. (2003). Prognostic significance of annexin VII expression in glioblastomas multiforme in humans. J. Neurosurg. 99, 886–892. doi:10.3171/jns.2003.99.5.0886
Hung, M. S., Chen, Y. C., Lin, P. Y., Li, Y. C., Hsu, C. C., Lung, J. H., et al. (2019). Cul4A modulates invasion and metastasis of lung cancer through regulation of ANXA10. Cancers (Basel) 11, E618. doi:10.3390/cancers11050618
Iglesias, J., Morgan, R. O., Jenkins, N. A., Copeland, N. G., Gilbert, D. J., and Fernandez, M. (2001). Comparative genetics and evolution of annexin A13 as the founder gene of vertebrate annexins, 608–618.
Ishikawa, A., Kuraoka, K., Zaitsu, J., Saito, A., Kuwai, T., Shimizu, Y., et al. (2021). Annexin A10 expression is associated with poor prognosis in small bowel adenocarcinoma. Anticancer Res. 41, 1349–1355. doi:10.21873/anticanres.14892
Ishikawa, A., Kuraoka, K., Zaitsu, J., Saito, A., Kuwai, T., Suzuki, T., et al. (2020). Loss of annexin a10 expression is associated with poor prognosis in early gastric cancer. Acta histochem. cytochem. 53, 113–119. doi:10.1267/ahc.20-00014
Ishikawa, A., Kuraoka, K., Zaitsu, J., Saito, A., Kuwai, T., Tazawa, H., et al. (2022a). Transcriptomic analysis of annexin A10 and chemosensitivity in gastric adenocarcinoma cells. Anticancer Res. 42, 1707–1717. doi:10.21873/anticanres.15647
Ishikawa, A., Kuraoka, K., Zaitsu, J., Saito, A., Yamaguchi, A., Kuwai, T., et al. (2022b). High Annexin A10 expression is correlated with poor prognosis in pancreatic ductal adenocarcinoma. Histol. Histopathol. 37, 243–250. doi:10.14670/HH-18-397
Ji, Z., Diao, W., and Shang, J. (2022). Circular RNA circ_0000592 elevates ANXA4 expression via sponging miR-1179 to facilitate tumor progression in gastric cancer. Anticancer. Drugs 33, e644–e654. doi:10.1097/CAD.0000000000001216
Jia, C., Kong, D., Guo, Y., Li, L., and Quan, L. (2018). Enhanced antitumor effect of combination of annexin A1 knockdown and bortezomib treatment in multiple myeloma in vitro and in vivo. Biochem. Biophys. Res. Commun. 505, 720–725. doi:10.1016/j.bbrc.2018.09.140
Jiang, G., Wang, P., Wang, W., Li, W., Dai, L., and Chen, K. (2017). Annexin A13 promotes tumor cell invasion in vitro and is associated with metastasis in human colorectal cancer. Oncotarget 8, 21663–21673. doi:10.18632/oncotarget.15523
Jiang, K., Tang, Z., Li, J., Wang, F., and Tang, N. (2018). Anxa4 mediated airway progenitor cell migration promotes distal epithelial cell fate specification. Sci. Rep. 8, 14344. doi:10.1038/s41598-018-32494-z
Jose, J., Hoque, M., Engel, J., Beevi, S. S., Wahba, M., Georgieva, M. I., et al. (2022). Annexin A6 and NPC1 regulate LDL-inducible cell migration and distribution of focal adhesions. Sci. Rep. 12, 596–612. doi:10.1038/s41598-021-04584-y
Jung, H., Kim, J. S., Kim, W. K., Oh, K. J., Kim, J. M., Lee, H. J., et al. (2015). Intracellular annexin A2 regulates NF-κB signaling by binding to the p50 subunit: Implications for gemcitabine resistance in pancreatic cancer. Cell Death Dis. 6, e1606. doi:10.1038/cddis.2014.558
Kaetzel, M. A., Mo, Y. D., Mealy, T. R., Campos, B., Bergsma-Schutter, W., Brisson, A., et al. (2001). Phosphorylation mutants elucidate the mechanism of annexin IV-mediated membrane aggregation. Biochemistry 40, 4192–4199. doi:10.1021/bi002507s
Kanagasabai, R., Karthikeyan, K., Vedam, K., Qien, W., Zhu, Q., and Ilangovan, G. (2010). Hsp27 protects adenocarcinoma cells from UV-induced apoptosis by Akt and p21-dependent pathways of survival. Mol. Cancer Res. 8, 1399–1412. doi:10.1158/1541-7786.MCR-10-0181
Kang, J. S., Calvo, B. F., Maygarden, S. J., Caskey, L. S., Mohler, J. L., and Ornstein, D. K. (2002). Dysregulation of annexin I protein expression in high-grade prostatic intraepithelial neoplasia and prostate cancer. Clin. Cancer Res. 8, 117–123.
Kang, T. H., Park, J. H., Yang, A., Park, H. J., Lee, S. E., Kim, Y. S., et al. (2020). Annexin A5 as an immune checkpoint inhibitor and tumor-homing molecule for cancer treatment. Nat. Commun. 11, 1137. doi:10.1038/s41467-020-14821-z
Karanjawala, Z. E., Illei, P. B., Ashfaq, R., Infante, J. R., Murphy, K., Pandey, A., et al. (2008). New markers of pancreatic cancer identified through differential gene expression analyses: Claudin 18 and annexin A8. Am. J. Surg. Pathol. 32, 188–196. doi:10.1097/PAS.0b013e31815701f3
Karlsson, J., Fu, H., Boulay, F., Dahlgren, C., Hellstrand, K., and Movitz, C. (2005). Neutrophil NADPH-oxidase activation by an annexin AI peptide is transduced by the formyl peptide receptor (FPR), whereas an inhibitory signal is generated independently of the FPR family receptors. J. Leukoc. Biol. 78, 762–771. doi:10.1189/jlb.0305153
Kataoka, T. R., Akihiko, I., Asada, H., Watabe, K., Nishiyama, K., Nakamoto, K., et al. (2000). Annexin VII as a novel marker for invasive phenotype of malignant melanoma. Jpn. J. Cancer Res. 91, 75–83. doi:10.1111/j.1349-7006.2000.tb00862.x
Kawakita, D., Lee, Y. C. A., Li, Q., Chen, Y., Chen, C. J., Hsu, W. L., et al. (2017). Impact of oral hygiene on head and neck cancer risk in a Chinese population. Head. Neck 39, 2549–2557. doi:10.1002/hed.24929
Kheifets, V., Bright, R., Inagaki, K., Schechtman, D., and Mochly-Rosen, D. (2006). Protein kinase C delta (deltaPKC)-annexin V interaction: A required step in deltaPKC translocation and function. J. Biol. Chem. 281, 23218–23226. doi:10.1074/jbc.M602075200
Kim, J. C., Ha, Y. J., Roh, S. A., Choi, E. Y., Yoon, Y. S., Kim, K. P., et al. (2013). Feasibility of proposed single-nucleotide polymorphisms as predictive markers for targeted regimens in metastatic colorectal cancer. Br. J. Cancer 108, 1862–1869. doi:10.1038/bjc.2013.163
Kim, J. C., Kim, S. Y., Cho, D. H., Ha, Y. J., Choi, E. Y., Kim, C. W., et al. (2011). Novel chemosensitive single-nucleotide polymorphism markers to targeted regimens in metastatic colorectal cancer. Clin. Cancer Res. 17, 1200–1209. doi:10.1158/1078-0432.CCR-10-1907
Kim, J. K., Kim, P. J., Jung, K. H., Noh, J. H., Eun, J. W., Bae, H. J., et al. (2010). Decreased expression of Annexin A10 in gastric cancer and its overexpression in tumor cell growth suppression. Oncol. Rep. 24, 607–612.
Kim, V. M., Blair, A. B., Lauer, P., Foley, K., Che, X., Soares, K., et al. (2019). Anti-pancreatic tumor efficacy of a Listeria-based, Annexin A2-targeting immunotherapy in combination with anti-PD-1 antibodies. J. Immunother. Cancer 7, 132. doi:10.1186/s40425-019-0601-5
Kodaira, H., Koma, Y. I., Hosono, M., Higashino, N., Suemune, K., Nishio, M., et al. (2019). ANXA10 induction by interaction with tumor-associated macrophages promotes the growth of esophageal squamous cell carcinoma. Pathol. Int. 69, 135–147. doi:10.1111/pin.12771
Koese, M., Rentero, C., Kota, B. P., Hoque, M., Cairns, R., Wood, P., et al. (2013). Annexin A6 is a scaffold for PKCα to promote EGFR inactivation. Oncogene 32, 2858–2872. doi:10.1038/onc.2012.303
Korolkova, O. Y., Widatalla, S. E., Whalen, D. S., Nangami, G. N., Abimbola, A., Williams, S. D., et al. (2020a). Reciprocal expression of Annexin A6 and RasGRF2 discriminates rapidly growing from invasive triple negative breast cancer subsets. PLoS One 15, e0231711. doi:10.1371/journal.pone.0231711
Korolkova, O. Y., Widatalla, S. E., Williams, S. D., Whalen, D. S., Beasley, H. K., Ochieng, J., et al. (2020b). EGFR-targeted therapies. Cancer Metastasis Rev. 2, 1–22.
Kou, X., Ding, H., Li, L., and Chao, H. (2021). Hsa-miR-105-1 regulates cisplatin-resistance in ovarian carcinoma cells by targeting ANXA9. Anal. Cell. Pathol. 2021, 6662486. doi:10.1155/2021/6662486
Koumangoye, R. B., Nangami, G. N., Thompson, P. D., Agboto, V. K., Ochieng, J., and Sakwe, A. M. (2013). Reduced annexin A6 expression promotes the degradation of activated epidermal growth factor receptor and sensitizes invasive breast cancer cells to EGFR-targeted tyrosine kinase inhibitors. Mol. Cancer 12, 167–180. doi:10.1186/1476-4598-12-167
Kpetemey, M., Dasgupta, S., Rajendiran, S., Das, S., Gibbs, L. D., Shetty, P., et al. (2015). MIEN1, a novel interactor of Annexin A2, promotes tumor cell migration by enhancing AnxA2 cell surface expression. Mol. Cancer 14, 156. doi:10.1186/s12943-015-0428-8
Kudo, I., Esumi, M., Kusumi, Y., Furusaka, T., and Oshima, T. (2017). Particular gene upregulation and p53 heterogeneous expression in TP53-mutated maxillary carcinoma. Oncol. Lett. 14, 4633–4640. doi:10.3892/ol.2017.6751
Kumar, A., Oswal, K., Singh, R., Kharodia, N., Pradhan, A., Sethuraman, L., et al. (2021). Assessment of areca nut use, practice and dependency among people in guwahati, Assam: A cross-sectional study. Ecancermedicalscience 15, 1198. doi:10.3332/ECANCER.2021.1198
Lafont, F., Lecat, S., Verkade, P., and Simons, K. (1998). Annexin XIIIb associates with lipid microdomains to function in apical delivery. J. Cell Biol. 142, 1413–1427. doi:10.1083/jcb.142.6.1413
Leca, J., Martinez, S., Lac, S., Nigri, J., Secq, V., Rubis, M., et al. (2016). Cancer-associated fibroblast-derived annexin A6+ extracellular vesicles support pancreatic cancer aggressiveness. J. Clin. Invest. 126, 4140–4156. doi:10.1172/JCI87734
Leemans, C. R., Braakhuis, B. J. M., and Brakenhoff, R. H. (2011). The molecular biology of head and neck cancer. Nat. Rev. Cancer 11, 9–22. doi:10.1038/nrc2982
lepcha, L., Sarma, M. P., Kataki, A. C., Wankhar, W., and Unni, B. G. (2021). Protein profile of human saliva as a predictive and prognostic tool for OSCC in tamol chewer’s population in Assam. Asian pac. J. Cancer Prev. 22, 1837–1841. doi:10.31557/APJCP.2021.22.6.1837
Li, L., Zou, J., Dai, Y., Fan, W., Niu, G., Yang, Z., et al. (2020). Burst release of encapsulated annexin A5 in tumours boosts cytotoxic T-cell responses by blocking the phagocytosis of apoptotic cells. Nat. Biomed. Eng. 4, 1102–1116. doi:10.1038/s41551-020-0599-5
Li, T., Tao, Z., Zhu, Y., Liu, X., Wang, L., Du, Y., et al. (2021a). Exosomal annexin A6 induces gemcitabine resistance by inhibiting ubiquitination and degradation of EGFR in triple-negative breast cancer. Cell Death Dis. 12, 684. doi:10.1038/s41419-021-03963-7
Li, X., Ma, W., Wang, X., Ci, Y., and Zhao, Y. (2018). Annexin A5 overexpression might suppress proliferation and metastasis of human uterine cervical carcinoma cells. Cancer Biomark. 23, 23–32. doi:10.3233/CBM-171040
Li, Y., Cai, L., Wang, H., Wu, P., Gu, W., Chen, Y., et al. (2011). Pleiotropic regulation of macrophage polarization and tumorigenesis by formyl peptide receptor-2. Oncogene 30, 3887–3899. doi:10.1038/onc.2011.112
Li, Z., Yu, L., Hu, B., Chen, L., Jv, M., Wang, L., et al. (2021b). Advances in cancer treatment: A new therapeutic target, annexin A2. J. Cancer 12, 3587–3596. doi:10.7150/JCA.55173
Liemann, S., and Huber, R. (1997). Three-dimensional structure of annexins. Cell. Mol. Life Sci. 53, 516–521. doi:10.1007/s000180050065
Liemann, S., and Lewit-Bentley, A. (1995). Annexins: A novel family of calcium- and membrane-binding proteins in search of a function. Structure 3, 233–237. doi:10.1016/S0969-2126(01)00152-6
Lim, L. H. K., and Pervaiz, S. (2007). Annexin 1: The new face of an old molecule. FASEB J. 21, 968–975. doi:10.1096/fj.06-7464rev
Ling, Q., Jacovina, A. T., Deora, A., Febbraio, M., Simantov, R., Silverstein, R. L., et al. (2004). Annexin II regulates fibrin homeostasis and neoangiogenesis in vivo. J. Clin. Invest. 113, 38–48. doi:10.1172/JCI19684
Liu, C., Li, N., Liu, G., and Feng, X. (2021a). Annexin A3 and cancer. Oncol. Lett. 22, 834. doi:10.3892/ol.2021.13095
Liu, H., Han, Y., Mi, R., Zhang, Y., Su, G., Wang, H., et al. (2011). Identification of cervical cancer proteins associated with treatment with paclitaxel and cisplatin in patients. Int. J. Gynecol. Cancer 21, 1452–1457. doi:10.1097/IGC.0b013e31822491d0
Liu, J., Rothermund, C. A., Ayala-Sanmartin, J., and Vishwanatha, J. K. (2003a). Nuclear annexin II negatively regulates growth of LNCaP cells and substitution of ser 11 and 25 to glu prevents nucleo-cytoplasmic shuttling of annexin II. BMC Biochem. 4, 10. doi:10.1186/1471-2091-4-10
Liu, J. W., Shen, J. J., Tanzillo-Swarts, A., Bhatia, B., Maldonado, C. M., Person, M. D., et al. (2003b). Annexin II expression is reduced or lost in prostate cancer cells and its re-expression inhibits prostate cancer cell migration. Oncogene 22, 1475–1485. doi:10.1038/sj.onc.1206196
Liu, S., Guo, C., Wang, J., Wang, B., Qi, H., and Sun, M. Z. (2016a). ANXA11 regulates the tumorigenesis, lymph node metastasis and 5-fluorouracil sensitivity of murine hepatocarcinoma Hca-P cells by targeting c-Jun. Oncotarget 7, 16297–16310. doi:10.18632/oncotarget.7484
Liu, S. H., Lin, C. Y., Peng, S. Y., Jeng, Y. M., Pan, H. W., Lai, P. L., et al. (2002). Down-regulation of annexin A10 in hepatocellular carcinoma is associated with vascular invasion, early recurrence, and poor prognosis in synergy with p53 mutation. Am. J. Pathol. 160, 1831–1837. doi:10.1016/S0002-9440(10)61129-7
Liu, S., Wang, J., Guo, C., Qi, H., and Sun, M. Z. (2015). Annexin A11 knockdown inhibits in vitro proliferation and enhances survival of Hca-F cell via Akt2/FoxO1 pathway and MMP-9 expression. Biomed. Pharmacother. 70, 58–63. doi:10.1016/j.biopha.2015.01.011
Liu, W., Zeng, L., Li, N., Wang, F., Jiang, C., Guo, F., et al. (2016b). Quantitative proteomic analysis for novel biomarkers of buccal squamous cell carcinoma arising in background of oral submucous fibrosis. BMC Cancer 16, 584. doi:10.1186/s12885-016-2650-1
Liu, X., Peng, X., Hu, Z., Zhao, Q., He, J., Li, J., et al. (2012). Effects of over-expression of ANXA10 gene on proliferation and apoptosis of hepatocellular carcinoma cell line HepG2. J. Huazhong Univ. Sci. Technol. - Med. Sci. 32, 669–674. doi:10.1007/s11596-012-1015-5
Liu, X., Yang, M., Guo, Y., and Lu, X. (2021b). Annexin A10 is a novel prognostic biomarker of papillary thyroid cancer. Ir. J. Med. Sci. 190, 59–65. doi:10.1007/s11845-020-02263-x
Lokman, N. A., Elder, A. S. F., Ween, M. P., Pyragius, C. E., Hoffmann, P., Oehler, M. K., et al. (2013). Annexin A2 is regulated by ovarian cancer-peritoneal cell interactions and promotes metastasis. Oncotarget 4, 1199–1211. doi:10.18632/oncotarget.1122
Lomnytska, M. I., Becker, S., Bodin, I., Olsson, A., Hellman, K., Hellström, A. C., et al. (2011). Differential expression of ANXA6, HSP27, PRDX2, NCF2, and TPM4 during uterine cervix carcinogenesis: Diagnostic and prognostic value. Br. J. Cancer 104, 110–119. doi:10.1038/sj.bjc.6605992
Lomnytska, M. I., Becker, S., Hellman, K., Hellström, A. C., Souchelnytskyi, S., Mints, M., et al. (2010). Diagnostic protein marker patterns in squamous cervical cancer. Proteomics. Clin. Appl. 4, 17–31. doi:10.1002/prca.200900086
Lu, S. H., Yuan, R. H., Chen, Y. L., Hsu, H. C., and Jeng, Y. M. (2013). Annexin A10 is an immunohistochemical marker for adenocarcinoma of the upper gastrointestinal tract and pancreatobiliary system. Histopathology 63, 640–648. doi:10.1111/his.12229
Ma, F., Li, X., Fang, H., Jin, Y., Sun, Q., and Li, X. (2020). Prognostic value of ANXA8 in gastric carcinoma. J. Cancer 11, 3551–3558. doi:10.7150/jca.40010
Ma, R. L., Shen, L. Y., and Chen, K. N. (2014). Coexpression of ANXA2, SOD2 and HOXA13 predicts poor prognosis of esophageal squamous cell carcinoma. Oncol. Rep. 31, 2157–2164. doi:10.3892/or.2014.3088
Ma, Y., and Wang, H. (2021). Clinical significance of Annexin A2 expression in oral squamous cell carcinoma and its influence on cell proliferation, migration and invasion. Sci. Rep. 11, 5033–5042. doi:10.1038/s41598-021-84675-y
Madureira, P. A., Hill, R., Lee, P. W. K., and Waisman, D. M. (2012). Genotoxic agents promote the nuclear accumulation of annexin A2: Role of annexin A2 in mitigating DNA damage. PLoS One 7, e50591. doi:10.1371/journal.pone.0050591
Mahdi, A. F., Malacrida, B., Nolan, J., McCumiskey, M. E., Merrigan, A. B., Lal, A., et al. (2020). Expression of annexin A2 promotes cancer progression in estrogen receptor negative breast cancers. Cells 9. doi:10.3390/cells9071582
Mao, L., Yuan, W., Cai, K., Lai, C., Huang, C., Xu, Y., et al. (2021). EphA2–YES1–ANXA2 pathway promotes gastric cancer progression and metastasis. Oncogene 40, 3610–3623. doi:10.1038/s41388-021-01786-6
Masaki, T., Tokuda, M., Ohnishi, M., Watanabe, S., Fujimura, T., Miyamoto, K., et al. (1996). Enhanced expression of the protein kinase substrate annexin in human hepatocellular carcinoma. Hepatology 24, 72–81. doi:10.1053/jhep.1996.v24.pm0008707286
McCulloch, K. M., Yamakawa, I., Shifrin, D. A., Mcconnell, R. E., Foegeding, N. J., Singh, P. K., et al. (2019). An alternative N-terminal fold of the intestine-specific annexin A13a induces dimerization and regulates membrane-binding. J. Biol. Chem. 294, 3454–3463. doi:10.1074/jbc.RA118.004571
McKernan, P., Virani, N. A., Faria, G. N. F., Karch, C. G., Prada Silvy, R., Resasco, D. E., et al. (2021). Targeted single-walled carbon nanotubes for photothermal therapy combined with immune checkpoint inhibition for the treatment of metastatic breast cancer. Nanoscale Res. Lett. 16, 9. doi:10.1186/s11671-020-03459-x
McNeil, A. K., Rescher, U., Gerke, V., and McNeil, P. L. (2006). Requirement for annexin A1 in plasma membrane repair. J. Biol. Chem. 281, 35202–35207. doi:10.1074/jbc.M606406200
Meier, E. M., Rein-Fischboeck, L., Pohl, R., Wanninger, J., Hoy, A. J., Grewal, T., et al. (2016). Annexin A6 protein is downregulated in human hepatocellular carcinoma. Mol. Cell. Biochem. 418, 81–90. doi:10.1007/s11010-016-2735-9
Meng, H., Zhang, Y., An, S. T., and Chen, Y. (2019). Annexin A3 gene silencing promotes myocardial cell repair through activation of the PI3K/Akt signaling pathway in rats with acute myocardial infarction. J. Cell. Physiol. 234, 10535–10546. doi:10.1002/jcp.27717
Merrifield, C. J., Rescher, U., Almers, W., Proust, J., Gerke, V., Sechi, A. S., et al. (2001). Annexin 2 has an essential role in actin-based macropinocytic rocketing. Curr. Biol. 11, 1136–1141. doi:10.1016/S0960-9822(01)00321-9
Mirsaeidi, M., Gidfar, S., Vu, A., and Schraufnagel, D. (2016). Annexins family: Insights into their functions and potential role in pathogenesis of sarcoidosis. J. Transl. Med. 14, 89. doi:10.1186/s12967-016-0843-7
Mitra, A. P., Almal, A. A., George, B., Fry, D. W., Lenehan, P. F., Pagliarulo, V., et al. (2006). The use of genetic programming in the analysis of quantitative gene expression profiles for identification of nodal status in bladder cancer. BMC Cancer 6, 159. doi:10.1186/1471-2407-6-159
Miyoshi, N., Yamamoto, H., Mimori, K., Yamashita, S., Miyazaki, S., Nakagawa, S., et al. (2014). Anxa9 gene expression in colorectal cancer: A novel marker for prognosis. Oncol. Lett. 8, 2313–2317. doi:10.3892/ol.2014.2477
Mody, M. D., Rocco, J. W., Yom, S. S., Haddad, R. I., and Saba, N. F. (2021). Head and neck cancer. Lancet 398, 2289–2299. doi:10.1016/S0140-6736(21)01550-6
Monastyrskaya, K., Babiychuk, E. B., Hostettler, A., Wood, P., Grewal, T., and Draeger, A. (2009). Plasma membrane-associated annexin A6 reduces Ca2+ entry by stabilizing the cortical actin cytoskeleton. J. Biol. Chem. 284, 17227–17242. doi:10.1074/jbc.M109.004457
Moreau, K., Ghislat, G., Hochfeld, W., Renna, M., Zavodszky, E., Runwal, G., et al. (2015). Transcriptional regulation of Annexin A2 promotes starvation-induced autophagy. Nat. Commun. 6, 8045. doi:10.1038/ncomms9045
Morel, E., and Gruenberg, J. (2007). The p11/S100A10 light chain of annexin A2 is dispensable for annexin A2 association to endosomes and functions in endosomal transport. PLoS One 2, e1118. doi:10.1371/journal.pone.0001118
Morgan, R. O., Bell, D. W., Testa, J. R., and Fernandez, M. P. (1999a). Human annexin 31 genetic mapping and origin. Gene 227, 33–38. doi:10.1016/S0378-1119(98)00597-6
Morgan, R. O., Jenkins, N. A., Gilbert, D. J., Copeland, N. G., Balsara, B. R., Testa, J. R., et al. (1999b). Novel human and mouse annexin A10 are linked to the genome duplications during early chordate evolution. Genomics 60, 40–49. doi:10.1006/geno.1999.5895
Morimoto, A., Serada, S., Enomoto, T., Kim, A., Matsuzaki, S., Takahashi, T., et al. (2014). Annexin A4 induces platinum resistance in a chloride-and calcium-dependent manner. Oncotarget 5, 7776–7787. doi:10.18632/oncotarget.2306
Moss, S. E., and Morgan, R. O. (2004). The annexins. Genome Biol. 5, 219. doi:10.1186/gb-2004-5-4-219
Muga, S. V., Timpson, P., Cubells, L., Evans, R., Hayes, T. E., Rentero, C., et al. (2009). Annexin A6 inhibits Ras signalling in breast cancer cells. Oncogene 28, 363–377. doi:10.1038/onc.2008.386
Munksgaard, P. P., Mansilla, F., Brems Eskildsen, A. S., Fristrup, N., Birkenkamp-Demtröder, K., Ulhøi, B. P., et al. (2011). Low ANXA10 expression is associated with disease aggressiveness in bladder cancer. Br. J. Cancer 105, 1379–1387. doi:10.1038/bjc.2011.404
Nakayama, M., Miyagawa, H., Kuranami, Y., Tsunooka-Ota, M., Yamaguchi, Y., and Kojima-Aikawa, K. (2020). Annexin A4 inhibits sulfatide-induced activation of coagulation factor XII. J. Thromb. Haemost. 18, 1357–1369. doi:10.1111/jth.14789
Niu, Y., Yang, X., Chen, Y., Jin, X., Xie, Y., Tang, Y., et al. (2019). Distinct prognostic values of Annexin family members expression in acute myeloid leukemia. Clin. Transl. Oncol. 21, 1186–1196. doi:10.1007/s12094-019-02045-7
Noreen, S., Gardner, Q. A., Fatima, I., Sadaf, S., and Akhtar, M. W. (2020). Upregulated expression of calcium-dependent annexin A6: A potential biomarker of ovarian carcinoma. Proteomics. Clin. Appl. 14, e1900078–9. doi:10.1002/prca.201900078
Oka, R., Nakashiro, K. I., Goda, H., Iwamoto, K., Tokuzen, N., and Hamakawa, H. (2016). Annexin A8 is a novel molecular marker for detecting lymph node metastasis in oral squamous cell carcinoma. Oncotarget 7, 4882–4889. doi:10.18632/oncotarget.6639
Oling, F., Santos, J. S. D. O., Govorukhina, N., Mazères-Dubut, C., Bergsma-Schutter, W., Oostergetel, G., et al. (2000). Structure of membrane-bound annexin A5 trimers: A hybrid cryo-EM - X-ray crystallography study. J. Mol. Biol. 304, 561–573. doi:10.1006/jmbi.2000.4183
Park, J. E., Do, H. L., Lee, J. A., Sung, G. P., Kim, N. S., Park, B. C., et al. (2005). Annexin A3 is a potential angiogenic mediator. Biochem. Biophys. Res. Commun. 337, 1283–1287. doi:10.1016/j.bbrc.2005.10.004
Paweletz, C. P., Ornstein, D. K., Roth, M. J., Bichsel, V. E., Gillespie, J. W., Calvert, V. S., et al. (2000). Loss of annexin 1 correlates with early onset of tumorigenesis in esophageal and prostate carcinoma. Cancer Res. 60, 6293–6297.
Pena-Alonso, E., Rodrigo, J. P., Parra, I. C., Pedrero, J. M. G., Meana, M. V. G., Nieto, C. S., et al. (2008). Annexin A2 localizes to the basal epithelial layer and is down-regulated in dysplasia and head and neck squamous cell carcinoma. Cancer Lett. 263, 89–98. doi:10.1016/j.canlet.2007.12.029
Peng, Y., Zhang, Z., Zhang, A., Liu, C., Sun, Y., Peng, Z., et al. (2021). Membrane-cytoplasm translocation of annexin A4 is involved in the metastasis of colorectal carcinoma. Aging 13, 10312–10325. doi:10.18632/aging.202793
Perretti, M., Croxtall, J. D., Wheller, S. K., Goulding, N. J., Hannon, R., and Flower, R. J. (1996). Mobilizing lipocortin 1 in adherent human leukocytes downregulates their transmigration. Nat. Med. 2, 1259–1262. doi:10.1038/nm1196-1259
Perretti, M., and Solito, E. (2004). Annexin 1 and neutrophil apoptosis. Biochem. Soc. Trans. 32, 507–510. doi:10.1042/BST0320507
Petrella, A., Festa, M., Ercolino, S. F., Zerilli, M., Stassi, G., Solito, E., et al. (2005). Induction of annexin-1 during TRAIL-induced apoptosis in thyroid carcinoma cells. Cell Death Differ. 12, 1358–1360. doi:10.1038/sj.cdd.4401645
Phukan, R. K., Ali, M. S., Chetia, C. K., and Mahanta, J. (2001). Betel nut and tobacco chewing; potential risk factors of cancer of oesophagus in Assam, India. Br. J. Cancer 85, 661–667. doi:10.1054/bjoc.2001.1920
Pimiento, J. M., Chen, D. T., Centeno, B. A., Davis-Yadley, A. H., Husain, K., Fulp, W. J., et al. (2015). Annexin A8 is a prognostic marker and potential therapeutic target for pancreatic cancer. Pancreas 44, 122–127. doi:10.1097/MPA.0000000000000218
Plant, P. J., Lafont, F., Lecat, S., Verkade, P., Simons, K., and Rotin, D. (2000). Apical membrane targeting of Nedd 4 is mediated by an association of its C2 domain with annexin XIIIb. J. Cell Biol. 149, 1473–1484. doi:10.1083/jcb.149.7.1473
Poeter, M., Brandherm, I., Rossaint, J., Rosso, G., Shahin, V., Skryabin, B. V., et al. (2014). Annexin A8 controls leukocyte recruitment to activated endothelial cells via cell surface delivery of CD63. Nat. Commun. 5, 3738. doi:10.1038/ncomms4738
Pons, M., Tebar, F., Kirchho, M., Peiro, S., Diego, D., Grewal, T., et al. (2001). Activation of Raf-1 is defective in annexin 6 overexpressing Chinese hamster ovary cells. FEBS Lett. 501, 69–73. doi:10.1016/s0014-5793(01)02635-7
Potez, S., Luginbu, M., Monastyrskaya, K., Hostettler, A., Draeger, A., and Babiychuk, E. B. (2011). Tailored protection against plasmalemmal injury by annexins with different Ca2+ sensitivities. J. Biol. Chem. 286, 17982–17991. doi:10.1074/jbc.M110.187625
Qi, H., Liu, S., Guo, C., Wang, J., Greenaway, F. T., and Sun, M. Z. (2015). Role of annexin A6 in cancer. Oncol. Lett. 10, 1947–1952. doi:10.3892/ol.2015.3498
Qin, C. X., Rosli, S., Deo, M., Cao, N., Walsh, J., Tate, M., et al. (2019). Cardioprotective actions of the annexin-A1 N-terminal peptide, AC2-26, against myocardial infarction. Front. Pharmacol. 10, 269–284. doi:10.3389/fphar.2019.00269
Quiskamp, N., Poeter, M., Raabe, C. A., Hohenester, U. M., König, S., Gerke, V., et al. (2014). The tumor suppressor annexin A10 is a novel component of nuclear paraspeckles. Cell. Mol. Life Sci. 71, 311–329. doi:10.1007/s00018-013-1375-4
Ran, S., and Thorpe, P. E. (2002). Phosphatidylserine is a marker of tumor vasculature and a potential target for cancer imaging and therapy. Int. J. Radiat. Oncol. Biol. Phys. 54, 1479–1484. doi:10.1016/S0360-3016(02)03928-7
Rand, J. H. (1999). Annexinopathies” A new class of diseases. N. Engl. J. Med. 340, 1035–1036. doi:10.1056/nejm199904013401310
Raulf, N., Lucarelli, P., Thavaraj, S., Brown, S., Vicencio, J. M., Sauter, T., et al. (2018). Annexin A1 regulates EGFR activity and alters EGFR-containing tumour-derived exosomes in head and neck cancers. Eur. J. Cancer 102, 52–68. doi:10.1016/j.ejca.2018.07.123
Raynal, P., and Pollard, H. B. (1994). Annexins: The problem of assessing the biological role for a gene family of multifunctional calcium- and phospholipid-binding proteins. Biochim. Biophys. Acta 1197, 63–93. doi:10.1016/0304-4157(94)90019-1
Rescher, U., Danielczyk, A., Markoff, A., and Gerke, V. (2002). Functional activation of the formyl peptide receptor by a new endogenous ligand in human lung A549 cells. J. Immunol. 169, 1500–1504. doi:10.4049/jimmunol.169.3.1500
Rescher, U., and Gerke, V. (2004). Annexins - unique membrane binding proteins with diverse functions. J. Cell Sci. 117, 2631–2639. doi:10.1242/jcs.01245
Rescher, U., and Gerke, V. (2008). S100A10/p11: Family, friends and functions. Pflugers Arch. 455, 575–582. doi:10.1007/s00424-007-0313-4
Rescher, U., Ludwig, C., Konietzko, V., Kharitonenkov, A., and Gerke, V. (2008). Tyrosine phosphorylation of annexin A2 regulates Rho-mediated actin rearrangement and cell adhesion. J. Cell Sci. 121, 2177–2185. doi:10.1242/jcs.028415
Rintala-Dempsey, A. C., Rezvanpour, A., and Shaw, G. S. (2008). S100-annexin complexes - structural insights. FEBS J. 275, 4956–4966. doi:10.1111/j.1742-4658.2008.06654.x
Rocha, M. R., Barcellos-de-Souza, P., Sousa-Squiavinato, A. C. M., Fernandes, P. V., de Oliveira, I. M., Boroni, M., et al. (2018). Annexin A2 overexpression associates with colorectal cancer invasiveness and TGF-ß induced epithelial mesenchymal transition via Src/ANXA2/STAT3. Sci. Rep. 8, 11285. doi:10.1038/s41598-018-29703-0
Rodrigo, J. P., García-Pedrero, J. M., Llorente, J. L., Fresno, M. F., Allonca, E., Suarez, C., et al. (2011a). Down-regulation of annexin A1 and A2 protein expression in intestinal-type sinonasal adenocarcinomas. Hum. Pathol. 42, 88–94. doi:10.1016/j.humpath.2010.05.017
Rodrigo, J. P., Lequerica-Fernández, P., Rosado, P., Allonca, E., García-Pedrero, J. M., and De Vicente, J. C. (2011b). Clinical significance of annexin A2 downregulation in oral squamous cell carcinoma. Head. Neck 33, 1708–1714. doi:10.1002/hed.21661
Rodrigo, J. P., Martińez, P., Allonca, E., Alonso-Durań, L., Suaŕez, C., Astudillo, A., et al. (2014). Immunohistochemical markers of distant metastasis in laryngeal and hypopharyngeal squamous cell carcinomas. Clin. Exp. Metastasis 31, 317–325. doi:10.1007/s10585-013-9630-5
Roh, S. A., Park, I. J., Yoon, Y. S., Kwon, Y. H., Chung, J. H., Kim, T. W., et al. (2016). Feasibility of novel PPP1R15A and proposed ANXA11 single nucleotide polymorphisms as predictive markers for bevacizumab regimen in metastatic colorectal cancer. J. Cancer Res. Clin. Oncol. 142, 1705–1714. doi:10.1007/s00432-016-2177-5
Rondepierre, F., Bouchon, B., Papon, J., Bonnet-Duquennoy, M., Kintossou, R., Moins, N., et al. (2009). Proteomic studies of B16 lines: Involvement of Annexin A1 in melanoma dissemination. Biochim. Biophys. Acta 1794, 61–69. doi:10.1016/j.bbapap.2008.09.014
Rong, B., Zhao, C., Liu, H., Ming, Z., Cai, X., Gao, W., et al. (2014). Elevated serum annexin A1 as potential diagnostic marker for lung cancer: A retrospective case-control study. Am. J. Transl. Res. 6, 558–569.
Ross, T. S., Tait, J. F., and Majerus, P. W. (1990). Identity of inositol 1, 2-cyclic phosphate 2-phosphohydrolase with lipocortin III. Science 248, 605–607. doi:10.1126/science.2159184
Rossetti, S., Bshara, W., Reiners, J. A., Corlazzoli, F., Miller, A., and Sacchi, N. (2016). Harnessing 3D models of mammary epithelial morphogenesis: An off the beaten path approach to identify candidate biomarkers of early stage breast cancer. Cancer Lett. 380, 375–383. doi:10.1016/j.canlet.2016.07.003
Rossetti, S., and Sacchi, N. (2019). 3D mammary epithelial cell models: A goldmine of dcis biomarkers and morphogenetic mechanisms. Cancers (Basel) 11, E130–E138. doi:10.3390/cancers11020130
Roth, U., Razawi, H., Hommer, J., Engelmann, K., Schwientek, T., Müller, S., et al. (2010). Differential expression proteomics of human colorectal cancer based on a syngeneic cellular model for the progression of adenoma to carcinoma. Proteomics 10, 194–202. doi:10.1002/pmic.200900614
Ruan, L., Wang, G. L., Chen, Y., Yi, H., Tang, C. E., Zhang, P. F., et al. (2010). Identification of tyrosine phosphoproteins in signaling pathway triggered TGF-a by using functional proteomics technology. Med. Oncol. 27, 1407–1414. doi:10.1007/s12032-009-9394-6
Rysavy, N. M., Shimoda, L. M. N., Dixon, A. M., Speck, M., Stokes, A. J., Turner, H., et al. (2014). Beyond apoptosis: The mechanism and function of phosphatidylserine asymmetry in the membrane of activating mast cells. Bioarchitecture 4, 127–137. doi:10.1080/19490992.2014.995516
Sakwe, A. M., Koumangoye, R., Guillory, B., and Ochieng, J. (2011). Annexin A6 contributes to the invasiveness of breast carcinoma cells by influencing the organization and localization of functional focal adhesions. Exp. Cell Res. 317, 823–837. doi:10.1016/j.yexcr.2010.12.008
Salom, C., Álvarez-Teijeiro, S., Fernández, M. P., Morgan, R. O., Allonca, E., Vallina, A., et al. (2019). Frequent alteration of annexin A9 and A10 in HPV-negative head and neck squamous cell carcinomas: Correlation with the histopathological differentiation grade. J. Clin. Med. 8, E229. doi:10.3390/jcm8020229
Santos, J., González-Sánchez, L., Matabuena-deYzaguirre, M., Villa-Morales, M., Cozar, P., López-Nieva, P., et al. (2009). A role for stroma-derived annexin A1 as mediator in the control of genetic susceptibility to T-cell lymphoblastic malignancies through prostaglandin e 2 secretion. Cancer Res. 69, 2577–2587. doi:10.1158/0008-5472.CAN-08-1821
Scala, M. C., Di Micco, S., Lanzillotta, D., Musella, S., Di Sarno, V., Parrino, B., et al. (2021). Overcome chemoresistance: Biophysical and structural analysis of synthetic FHIT-derived peptides. Front. Mol. Biosci. 8, 715263. doi:10.3389/fmolb.2021.715263
Schloer, S., Pajonczyk, D., and Rescher, U. (2018). Annexins in translational research: Hidden treasures to be found. Int. J. Mol. Sci. 19, E1781. doi:10.3390/ijms19061781
Schostak, M., Schwall, G. P., Poznanović, S., Groebe, K., Müller, M., Messinger, D., et al. (2009). Annexin A3 in urine: A highly specific noninvasive marker for prostate cancer early detection. J. Urol. 181, 343–353. doi:10.1016/j.juro.2008.08.119
Seccia, V., Navari, E., Donadio, E., Boldrini, C., Ciregia, F., Ronci, M., et al. (2020). Proteomic investigation of malignant major salivary gland tumors. Head. Neck Pathol. 14, 362–373. doi:10.1007/s12105-019-01040-2
Shao, Y. Y., Kuo, H. Y., Jeng, Y. M., Wu, Y. M., Wang, H. P., Hsu, C., et al. (2022). Association of annexin A10 expression with poor prognosis of intrahepatic cholangiocarcinoma. BMC Cancer 22, 219–226. doi:10.1186/s12885-022-09288-8
Shapanis, A., Lai, C., Smith, S., Coltart, G., Sommerlad, M., Schofield, J., et al. (2021). Identification of proteins associated with development of metastasis from cutaneous squamous cell carcinomas (cSCCs) via proteomic analysis of primary cSCCs. Br. J. Dermatol. 184, 709–721. doi:10.1111/bjd.19485
Sharma, M. R., Koltowski, L., Ownbey, R. T., Tuszynski, G. P., and Sharma, M. C. (2006). Angiogenesis-associated protein annexin II in breast cancer: Selective expression in invasive breast cancer and contribution to tumor invasion and progression. Exp. Mol. Pathol. 81, 146–156. doi:10.1016/j.yexmp.2006.03.003
Shetty, P. K., Thamake, S. I., Biswas, S., Johansson, S. L., and Vishwanatha, J. K. (2012). Reciprocal regulation of annexin A2 and EGFR with her-2 in her-2 negative and herceptin-resistant breast cancer. PLoS One 7, e44299. doi:10.1371/journal.pone.0044299
Shimizu, T., Kasamatsu, A., Yamamoto, A., Koike, K., Ishige, S., Takatori, H., et al. (2012). Annexin A10 in human oral cancer: Biomarker for tumoral growth via G1/S transition by targeting MAPK signaling pathways. PLoS One 7, e45510–9. doi:10.1371/journal.pone.0045510
Siddique, N., and T, S. (2001). Amyotrophic lateral sclerosis overview. Washington, Seattle: Seattle Univ, 1993–2022. GeneReviews® [Internet].
Smith, B. N., Topp, S. D., Fallini, C., Shibata, H., Chen, H.-J., Troakes, C., et al. (2017). Mutations in the vesicular trafficking protein annexin A11 are associated with amyotrophic lateral sclerosis. Sci. Transl. Med. 9, eaad9157. doi:10.1126/scitranslmed.aad9157
Smyth, T., Harris, H. J., Brown, A., Tötemeyer, S., Farnfield, B. A., Maskell, D. J., et al. (2006). Differential modulatory effects of annexin 1 on nitric oxide synthase induction by lipopolysaccharide in macrophages. Immunology 117, 340–349. doi:10.1111/j.1365-2567.2005.02307.x
Solito, E., Kamal, A., Russo-Marie, F., Buckingham, J. C., Marullo, S., and Perretti, M. (2003). A novel calcium-dependent proapoptotic effect of annexin 1 on human neutrophils. FASEB J. 17, 1544–1546. doi:10.1096/fj.02-0941fje
Song, J., Shih, I. M., Salani, R., Chan, D. W., and Zhang, Z. (2007). Annexin XI is associated with cisplatin resistance and related to tumor recurrence in ovarian cancer patients. Clin. Cancer Res. 13, 6842–6849. doi:10.1158/1078-0432.CCR-07-0569
Song, X., Wu, X., Zhang, Z., Cui, Z., Zheng, Y., and Sun, J. (2022). Subcellular proteome analysis reveals apoptotic vulnerability of T-cell acute lymphoblastic leukemia. Biomed. Res. Int. 2022, 5504475. doi:10.1155/2022/5504475
Sopkova, J., Raguenes-Nicol, C., Vincent, M., Chevalier, A., Lewit-Bentley, A., Russo-Marie, F., et al. (2009). Ca2+ and membrane binding to annexin 3 modulate the structure and dynamics of its N terminus and domain III. Protein Sci. 11, 1613–1625. doi:10.1110/ps.4230102
Srivastava, M., Bubendorf, L., Nolan, L., Glasman, M., Leighton, X., Miller, G., et al. (2001a). ANX7 as a bio-marker in prostate and breast cancer progression. Dis. Markers 17, 115–120. doi:10.1155/2001/239602
Srivastava, M., Bubendorf, L., Raffeld, M., Bucher, C., Torhorst, J., Sauter, G., et al. (2004). Prognostic impact of ANX7-GTPase in metastatic and HER2- negative breast cancer patients. Clin. Cancer Res. 10, 2344–2350. doi:10.1158/1078-0432.ccr-03-0278
Srivastava, M., Bubendorf, L., Srikantan, V., Fossom, L., Nolan, L., Glasman, M., et al. (2001b). Anx7, a candidate tumor suppressor gene for prostate cancer. Proc. Natl. Acad. Sci. U. S. A. 98, 4575–4580. doi:10.1073/pnas.071055798
Staquicini, D. I., Rangel, R., Guzman-Rojas, L., Staquicini, F. I., Dobroff, A. S., Tarleton, C. A., et al. (2017). Intracellular targeting of annexin A2 inhibits tumor cell adhesion, migration, and in vivo grafting. Sci. Rep. 7, 4243. doi:10.1038/s41598-017-03470-w
Suh, Y. E., Raulf, N., Gäken, J., Lawler, K., Urbano, T. G., Bullenkamp, J., et al. (2015). MicroRNA-196a promotes an oncogenic effect in head and neck cancer cells by suppressing annexin A1 and enhancing radioresistance. Int. J. Cancer 137, 1021–1034. doi:10.1002/ijc.29397
Sun, M.-Z., Liu, S., and Tang, J. (2009a). Targeting Annexin A7 in hepatocarcinoma lymphatic metastasis. Chin. J. Lung Cancer 12, 633–634.
Sun, M.-Z., Liu, S., Tang, J., Wang, Z., Gong, X., Sun, C., et al. (2009b). Proteomics analysis of two mice hepatocarcinoma ascites syngeneic cell lines with high and low lymph node metastasis rates provide potential protein markers for tumor malignancy attributes to lymphatic metastasis. Proteomics 9, 3285–3302. doi:10.1002/pmic.200701002
Sun, X., Liu, S., Wang, J., Wei, B., Guo, C., Chen, C., et al. (2018). Annexin A5 regulates hepatocarcinoma malignancy via CRKI/II-DOCK180-RAC1 integrin and MEK-ERK pathways. Cell Death Dis. 9, 637. doi:10.1038/s41419-018-0685-8
Sun, X., Shu, Y., Xu, M., Jiang, J., Wang, L., Wang, J., et al. (2020). ANXA6 suppresses the tumorigenesis of cervical cancer through autophagy induction. Clin. Transl. Med. 10, e208. doi:10.1002/ctm2.208
Swaggart, K. A., Demonbreun, A. R., Vo, A. H., Swanson, K. E., Kim, E. Y., Fahrenbach, J. P., et al. (2014). Annexin A6 modifies muscular dystrophy by mediating sarcolemmal repair. Proc. Natl. Acad. Sci. U. S. A. 111, 6004–6009. doi:10.1073/pnas.1324242111
Tait, J. F., Frankenberry, D. A., Miao, C. H., Killary, A. M., Adler, D. A., and Disteche, C. M. (1991). Chromosomal localization of the human annexin III (ANX3) gene. Genomics 10, 441–448. doi:10.1016/0888-7543(91)90330-H
Tang, S., Huang, W., Zhong, M., Yin, L., Jiang, H., Hou, S., et al. (2012). Identification Keratin 1 as a cDDP-resistant protein in nasopharyngeal carcinoma cell lines. J. Proteomics 75, 2352–2360. doi:10.1016/j.jprot.2012.02.003
Theobald, J., Hanby, A., Patel, K., and Moss, S. E. (1995). Annexin VI has tumour-suppressor activity in human A431 squamous epithelial carcinoma cells. Br. J. Cancer 71, 786–788. doi:10.1038/bjc.1995.152
Thiel, C., Osborn, M., and Gerke, V. (1992). The tight association of the tyrosine kinase substrate annexin II with the submembranous cytoskeleton depends on intact p11- and Ca2+-binding sites. J. Cell Sci. 103, 733–742. doi:10.1242/jcs.103.3.733
Tong, M., Fung, T. M., Luk, S. T., Ng, K. Y., Lee, T. K., Lin, C. H., et al. (2015). ANXA3/JNK signaling promotes self-renewal and tumor growth, and its blockade provides a therapeutic target for hepatocellular carcinoma. Stem Cell Rep. 5, 45–59. doi:10.1016/j.stemcr.2015.05.013
Toufiq, M., Roelands, J., Alfaki, M., Syed Ahamed Kabeer, B., Saadaoui, M., Lakshmanan, A. P., et al. (2020). Annexin A3 in sepsis: Novel perspectives from an exploration of public transcriptome data. Immunology 161, 291–302. doi:10.1111/imm.13239
Turnay, J., Lecona, E., Fernández-Lizarbe, S., Guzmán-Aránguez, A., Fernández, M. P., Olmo, N., et al. (2005). Structure-function relationship in annexin A13, the founder member of the vertebrate family of annexins. Biochem. J. 389, 899–911. doi:10.1042/BJ20041918
Uchihara, T., Miyake, K., Yonemura, A., Komohara, Y., Itoyama, R., Koiwa, M., et al. (2020). Extracellular vesicles from cancer-associated fibroblasts containing annexin A6 induces FAK-YAP activation by stabilizing β1 integrin, enhancing drug resistance. Cancer Res. 80, 3222–3235. doi:10.1158/0008-5472.CAN-19-3803
Vermorken, J. B., Trigo, J., Hitt, R., Koralewski, P., Diaz-Rubio, E., Rolland, F., et al. (2007). Open-label, uncontrolled, multicenter phase II study to evaluate the efficacy and toxicity of cetuximab as a single agent in patients with recurrent and/or metastatic squamous cell carcinoma of the head and neck who failed to respond to platinum-based therapy. J. Clin. Oncol. 25, 2171–2177. doi:10.1200/JCO.2006.06.7447
Virani, N. A., Thavathiru, E., McKernan, P., Moore, K., Benbrook, D. M., and Harrison, R. G. (2018). Anti-CD73 and anti-OX40 immunotherapy coupled with a novel biocompatible enzyme prodrug system for the treatment of recurrent, metastatic ovarian cancer. Cancer Lett. 425, 174–182. doi:10.1016/j.canlet.2018.03.027
Voisin, S. N., Krakovska, O., Matta, A., DeSouza, L. V., Romaschin, A. D., Colgan, T. J., et al. (2011). Identification of novel molecular targets for endometrial cancer using a drill-down LC-MS/MS Approach with iTRAQ. PLoS One 6, e16352. doi:10.1371/journal.pone.0016352
Waisman, D. M. (1995). Annexin II tetramer: Structure and function. Mol. Cell. Biochem. 149-150, 301–322. doi:10.1007/BF01076592
Wan, X., Guo, D., Zhu, Q., and Qu, R. (2020). microRNA-382 suppresses the progression of pancreatic cancer through the PI3K/Akt signaling pathway by inhibition of Anxa3. Am. J. Physiol. Gastrointest. Liver Physiol. 319, G309–G322. doi:10.1152/ajpgi.00322.2019
Wang, C., and Lin, C. (2014). Annexin A2 : Its molecular regulation and cellular expression in cancer development. Dis. Markers 2014, 308976. doi:10.1155/2014/308976
Wang, C. Y., Chen, C. L., Tseng, Y. L., Fang, Y. T., Lin, Y. S., Su, W. C., et al. (2012). Annexin A2 silencing induces G2 arrest of non-small cell lung cancer cells through p53-dependent and -independent mechanisms. J. Biol. Chem. 287, 32512–32524. doi:10.1074/jbc.M112.351957
Wang, D., Zhang, H., Fang, Z., and Yu, G. (2010). Annexin-1 downregulation is associated with clinical outcome in Chinese patients with hilar cholangiocarcinoma. Eur. Surg. Res. 45, 151–157. doi:10.1159/000320237
Wang, H., Deng, L., Cai, M., Zhuang, H., Zhu, L., Hao, Y., et al. (2017). Annexin A4 fucosylation enhances its interaction with the NF-kB p50 and promotes tumor progression of Ovarian clear cell Carcinoma. Oncotarget 8, 108093–108107. doi:10.18632/oncotarget.10226
Wang, J., Guo, C., Liu, S., Qi, H., Yin, Y., Liang, R., et al. (2014). Annexin A11 in disease. Clin. Chim. Acta. 431, 164–168. doi:10.1016/j.cca.2014.01.031
Wang, J., Zhao, S., Wang, F., Wang, J., and Zhang, Y. (2019). Prognostic significance of increased expression of annexin A10 (ANXA10) in serous epithelial ovarian cancer. Med. Sci. Monit. 25, 5666–5673. doi:10.12659/MSM.915911
Wang, X., Dai, Y., Zhao, Y., Li, M., Zhang, J., Ci, Y., et al. (2021). AnnexinA5 might suppress the phenotype of human gastric cancer cells via ERK pathway. Front. Oncol. 11, 665105. doi:10.3389/fonc.2021.665105
Wang, X., Zhang, S., Zhang, J., Lam, E., Liu, X., Sun, J., et al. (2013). Annexin A6 is down-regulated through promoter methylation in gastric cancer. Am. J. Transl. Res. 5, 555–562.
Wang, Y., Zheng, X., Wang, Q., Zheng, M., and Pang, L. (2020). GSK3β-Ikaros-ANXA4 signaling inhibits high-glucose-induced fibroblast migration. Biochem. Biophys. Res. Commun. 531, 543–551. doi:10.1016/j.bbrc.2020.07.142
Widatalla, S. E., Korolkova, O. Y., Whalen, D. S., Goodwin, J. S., Williams, K. P., Ochieng, J., et al. (2019). Lapatinib-induced annexin A6 upregulation as an adaptive response of triple-negative breast cancer cells to EGFR tyrosine kinase inhibitors. Carcinogenesis 40, 998–1009. doi:10.1093/carcin/bgy192
Woodham, A. W., da Silva, D. M., Skeate, J. G., Raff, A. B., Ambroso, M. R., Brand, H. E., et al. (2012). The S100A10 subunit of the annexin A2 heterotetramer facilitates L2-mediated human papillomavirus infection. PLoS One 7, e43519. doi:10.1371/journal.pone.0043519
Wu, W. B., Jia, G. Z., Chen, L., Liu, H. T., and Xia, S. J. (2021). Analysis of the expression and prognostic value of annexin family proteins in bladder cancer. Front. Genet. 12, 731625–731637. doi:10.3389/fgene.2021.731625
Xiao, B., Hang, J., Lei, T., He, Y., Kuang, Z., Wang, L., et al. (2019). Identification of key genes relevant to the prognosis of ER-positive and ER-negative breast cancer based on a prognostic prediction system. Mol. Biol. Rep. 46, 2111–2119. doi:10.1007/s11033-019-04663-4
Xiong, P., Li, Y. X., Tang, Y. T., and Chen, H. G. (2011). Proteomic analyses of Sirt1-mediated cisplatin resistance in OSCC cell line. Protein J. 30, 499–508. doi:10.1007/s10930-011-9354-9
Xu, H., Wu, X., Dou, Y., and Zheng, W. (2021). The prognostic significance of annexin A family in glioblastoma. Ir. J. Med. Sci. 3, 1539–1547. doi:10.1007/s11845-021-02737-6
Xu, R., Yin, J., Zhang, Y., and Zhang, S. (2019a). Annexin A3 depletion overcomes resistance to oxaliplatin in colorectal cancer via the MAPK signaling pathway. J. Cell. Biochem. 120, 14585–14593. doi:10.1002/jcb.28720
Xu, Y., Sui, L., Qiu, B., Yin, X., Liu, J., and Zhang, X. (2019b). ANXA4 promotes trophoblast invasion via the PI3K/akt/eNOS pathway in preeclampsia. Am. J. Physiol. Cell Physiol. 316, C481–C491. doi:10.1152/ajpcell.00404.2018
Xue, G.-L., Zhang, C., Zheng, G.-L., Zhang, L.-J., and Bi, J.-W. (2020). Annexin A13 predicts poor prognosis for lung adenocarcinoma patients and accelerates the proliferation and migration of lung adenocarcinoma cells by modulating epithelial-mesenchymal transition. Fundam. Clin. Pharmacol. 34, 687–696. doi:10.1111/fcp.12555
Xue, G., Hao, L. Q., Ding, F. X., Mei, Q., Huang, J. J., Fu, C. G., et al. (2009). Expression of annexin A5 is associated with higher tumor stage and poor prognosis in colorectal adenocarcinomas. J. Clin. Gastroenterol. 43, 831–837. doi:10.1097/MCG.0b013e31819cc731
Yadav, A. K., Renfrow, J. J., Scholtens, D. M., Xie, H., Duran, G. E., Bredel, C., et al. (2009). Monosomy of chromosome 10 associated with dysregulation of epidermal growth factor signaling in glioblastomas. JAMA - J. Am. Med. Assoc. 302, 276–289. doi:10.1001/jama.2009.1022
Yamashita, T., Nagano, K., Kanasaki, S. O., Maeda, Y., Furuya, T., Inoue, M., et al. (2012). Annexin A4 is a possible biomarker for cisplatin susceptibility of malignant mesothelioma cells. Biochem. Biophys. Res. Commun. 421, 140–144. doi:10.1016/j.bbrc.2012.03.144
Yang, L., Lu, P., Yang, X., Li, K., and Qu, S. (2021). Annexin A3, a calcium-dependent phospholipid-binding protein: Implication in cancer. Front. Mol. Biosci. 8, 716415. doi:10.3389/fmolb.2021.716415
Yang, Y. H., Song, W., Deane, J. A., Kao, W., Ooi, J. D., Ngo, D., et al. (2013). Deficiency of annexin A1 in CD4 + T cells exacerbates T cell–dependent inflammation. J. Immunol. 190, 997–1007. doi:10.4049/jimmunol.1202236
Yao, H. S., Sun, C., Li, X. X., Wang, Y., Jin, K. Z., Zhang, X. P., et al. (2016). Annexin A4-nuclear factor-κ B feedback circuit regulates cell malignant behavior and tumor growth in gallbladder cancer. Sci. Rep. 6, 31056. doi:10.1038/srep31056
Yao, X., Qi, X., Wang, Y., Zhang, B., He, T., Yan, T., et al. (2022). Identification and validation of an annexin-related prognostic signature and therapeutic targets for bladder cancer: Integrative analysis. Biol. (Basel) 11, 259. doi:10.3390/biology11020259
Ye, W., Li, Y., Fan, L., Zhao, Q., Yuan, H., Tan, B., et al. (2018). Annexin A7 expression is downregulated in late-stage gastric cancer and is negatively correlated with the differentiation grade and apoptosis rate. Oncol. Lett. 15, 9836–9844. doi:10.3892/ol.2018.8576
Yi, M., and Schnitzer, J. E. (2009). Impaired tumor growth, metastasis, angiogenesis and wound healing in annexin A1-null mice. Proc. Natl. Acad. Sci. U. S. A. 106, 17886–17891. doi:10.1073/pnas.0901324106
Yu, S., Bian, H., Gao, X., and Gui, L. (2018). Annexin A9 promotes invasion and metastasis of colorectal cancer and predicts poor prognosis. Int. J. Mol. Med. 41, 2185–2192. doi:10.3892/ijmm.2018.3432
Yuan, H. F., Li, Y., Zhao, Q., Fan, L. Q., Tan, B. B., and Ye, W. H. (2014). Expression of annexin A7 and its clinical significance in differentiation and metastasis of gastric carcinoma. Int. J. Clin. Exp. Pathol. 7, 6567–6574.
Yuan, J. B., Gu, L., Chen, L., Yin, Y., and Fan, B. Y. (2021). Annexin A8 regulated by lncRNA-TUG1/miR-140-3p axis promotes bladder cancer progression and metastasis. Mol. Ther. Oncolytics 22, 36–51. doi:10.1016/j.omto.2021.04.008
Yuan, Y., Anbalagan, D., Lee, L. H., Samy, R. P., Shanmugam, M. K., Kumar, A. P., et al. (2016). ANXA1 inhibits miRNA-196a in a negative feedback loop through NF-kB and C-Myc to reduce breast cancer proliferation. Oncotarget 7, 27007–27020. doi:10.18632/oncotarget.8875
Yumura, M., Nagano, T., Jimbo, N., Dokuni, R., Kiriu, T., Tanaka, Y., et al. (2022). Annexin A10 expression as a novel prognostic marker in lung adenocarcinoma. Anticancer Res. 42, 1289–1294. doi:10.21873/anticanres.15595
Zaidi, A. H., Gopalakrishnan, V., Kasi, P. M., Zeng, X., Malhotra, U., Balasubramanian, J., et al. (2014). Evaluation of a 4-protein serum biomarker panel-biglycan, annexin-A6, myeloperoxidase, and protein S100-A9 (B-AMP)-for the detection of esophageal adenocarcinoma. Cancer 120, 3902–3913. doi:10.1002/cncr.28963
Zhai, H., Acharya, S., Gravanis, I., Mehmood, S., Seidman, R. J., Shroyer, K. R., et al. (2011). Annexin A2 promotes glioma cell invasion and tumor progression. J. Neurosci. 31, 14346–14360. doi:10.1523/JNEUROSCI.3299-11.2011
Zhang, H. J., Yao, D. F., Yao, M., Huang, H., Wang, L., Yan, M. J., et al. (2013). Annexin A2 silencing inhibits invasion, migration, and tumorigenic potential of hepatoma cells. World J. Gastroenterol. 19, 3792–3801. doi:10.3748/wjg.v19.i24.3792
Zhang, W., and Han, D. (2021). miR-185-3p targets Annexin-A8 to inhibit proliferation in cervical cancer cells. Cytotechnology 73, 585–592. doi:10.1007/s10616-021-00479-y
Zhang, X., Hu, Z., Wang, X., Li, L., Zhu, B., Lin, X., et al. (2021a). ANXA10 promotes melanoma metastasis by suppressing E3 ligase TRIM41-directed PKD1 degradation. Cancer Lett. 519, 237–249. doi:10.1016/j.canlet.2021.07.033
Zhang, Z., Huang, L., Zhao, W., and Rigas, B. (2010). Annexin 1 induced by anti-inflammatory drugs binds to NF-kappaB and inhibits its activation: Anticancer effects in vitro and in vivo. Cancer Res. 70, 2379–2388. doi:10.1158/0008-5472.CAN-09-4204
Zhang, Z., Li, Z., Ma, Z., Deng, M., Xing, M., Wu, J., et al. (2021b). Annexin A3 as a marker protein for microglia in the central nervous system of rats. Neural Plast. 2021, 5575090. doi:10.1155/2021/5575090
Zheng, L., Foley, K., Huang, L., Leubner, A., Mo, G., Olino, K., et al. (2011). Tyrosine 23 phosphorylation-dependent cell-surface localization of annexin A2 is required for invasion and metastases of pancreatic cancer. PLoS One 6, e19390. doi:10.1371/journal.pone.0019390
Zheng, M. D., Wang, N. D., Li, X. L., Yan, J., Tang, J. H., Zhao, X. H., et al. (2018). Toosendanin mediates cisplatin sensitization through targeting Annexin A4/ATP7A in non-small cell lung cancer cells. J. Nat. Med. 72, 724–733. doi:10.1007/s11418-018-1211-0
Zhong, H., Liu, S., Cao, F., Zhao, Y., Zhou, J., Tang, F., et al. (2021). Dissecting tumor antigens and immune subtypes of glioma to develop mRNA vaccine. Front. Immunol. 12, 709986. doi:10.3389/fimmu.2021.709986
Zhou, Y., Qiu, C., Wang, T., Tao, L., Zhang, Z., and Yao, J. (2021). High expression of annexin A9 promotes cell proliferation and migration in gastric cancer via the TGF-β signaling pathway. J. Environ. Pathol. Toxicol. Oncol. 40, 87–94. doi:10.1615/JEnvironPatholToxicolOncol.2021038527
Zhu, J. F., Huang, W., Yi, H. M., Xiao, T., Li, J. Y., Feng, J., et al. (2018). Annexin A1-suppressed autophagy promotes nasopharyngeal carcinoma cell invasion and metastasis by PI3K/AKT signaling activation. Cell Death Dis. 9, 1154. doi:10.1038/s41419-018-1204-7
Zhu, J., Wu, J., Pei, X., Tan, Z., Shi, J., and Lubman, D. M. (2017). Annexin A10 is a candidate marker associated with the progression of pancreatic precursor lesions to adenocarcinoma. PLoS One 12, e0175039–19. doi:10.1371/journal.pone.0175039
Zhu, L., Gou, R., Guo, Q., Wang, J., Liu, Q., and Lin, B. (2020). High expression and potential synergy of human epididymis protein 4 and Annexin A8 promote progression and predict poor prognosis in epithelial ovarian cancer. Am. J. Transl. Res. 12, 4017–4030.
Zhuwang, D., Liu, Y., Yang, X., Yang, C. Z., Ma, J., Yang, X., et al. (2013). Low Annexin A1 expression predicts benefit from induction chemotherapy in oral cancer patients with moderate or poor pathologic differentiation grade. BMC Cancer 13, 301. doi:10.1186/1471-2407-13-301
Keywords: annexin, head and neck cancer, biomarker, diagnosis, therapeutic target
Citation: Prieto-Fernández L, Menéndez ST, Otero-Rosales M, Montoro-Jiménez I, Hermida-Prado F, García-Pedrero JM and Álvarez-Teijeiro S (2022) Pathobiological functions and clinical implications of annexin dysregulation in human cancers. Front. Cell Dev. Biol. 10:1009908. doi: 10.3389/fcell.2022.1009908
Received: 02 August 2022; Accepted: 29 August 2022;
Published: 28 September 2022.
Edited by:
Thomas Grewal, The University of Sydney, AustraliaReviewed by:
Pankaj Chaudhary, University of North Texas Health Science Center, United StatesNoor Alia Lokman, University of Adelaide, Australia
Praveenkumar Shetty, K. S. Hegde Medical Academy, India
Copyright © 2022 Prieto-Fernández, Menéndez, Otero-Rosales, Montoro-Jiménez, Hermida-Prado, García-Pedrero and Álvarez-Teijeiro. This is an open-access article distributed under the terms of the Creative Commons Attribution License (CC BY). The use, distribution or reproduction in other forums is permitted, provided the original author(s) and the copyright owner(s) are credited and that the original publication in this journal is cited, in accordance with accepted academic practice. No use, distribution or reproduction is permitted which does not comply with these terms.
*Correspondence: Juana M. García-Pedrero, juanagp.finba@gmail.com; Saúl Álvarez-Teijeiro, saul.teijeiro@gmail.com
†These authors have contributed equally to this work