- 1Department of Orthopaedics Surgery, Union Hospital, Tongji Medical College, Huazhong University of Science and Technology, Wuhan, China
- 2Institute of Hematology, Union Hospital, Tongji Medical College, Huazhong University of Science and Technology, Wuhan, China
- 3The First Clinical Medical College of Lanzhou University, Lanzhou, China
- 4The First Hospital of Lanzhou University, Lanzhou, China
Intervertebral disc degeneration (IVDD) has been reported to be the most prevalent contributor to low back pain, posing a significant strain on the healthcare systems on a global scale. Currently, there are no approved therapies available for the prevention of the progressive degeneration of intervertebral disc (IVD); however, emerging regenerative strategies that aim to restore the normal structure of the disc have been fundamentally promising. In the last decade, mesenchymal stem cells (MSCs) have received a significant deal of interest for the treatment of IVDD due to their differentiation potential, immunoregulatory capabilities, and capability to be cultured and regulated in a favorable environment. Recent investigations show that the pleiotropic impacts of MSCs are regulated by the production of soluble paracrine factors. Exosomes play an important role in regulating such effects. In this review, we have summarized the current treatments for disc degenerative diseases and their limitations and highlighted the therapeutic role and its underlying mechanism of MSC-derived exosomes in IVDD, as well as the possible future developments for exosomes.
1 Introduction
Low back pain (LBP) is a widespread health concern in modern society, resulting in high socioeconomic consequences (Katz, 2006; Kague et al., 2021). Epidemiological statistics show that approximately 80% of adults experience lower back pain at least once in their lifetime (Park et al., 2021); LBP is considered to be one of the most important reasons that limit the working capacity of people younger than 45 years (Deyo and Weinstein, 2001; Balague et al., 2012). Annually, the direct cost of treating LBP is approximately US$ 30 billion and the indirect socio-economic losses account for approximately US$ 100 billion in the United States (Binch et al., 2021). The incidence and prevalence of LBP in China have increased annually, and it has become one of the major diseases that affect both public health and people’s quality of life (Yao et al., 2011; Wu et al., 2019). Previous studies (Liao et al., 2019a; Au et al., 2020; Binch et al., 2021; Croft et al., 2021) showed that intervertebral disc (IVD) degeneration (IVDD) is the most prevalent contributor to LBP at present. Although the etiology of IVDD remains poorly understood, some influencing factors include metabolic disorders, aging, mechanical loading, trauma, infection, nutrition, and genetic predisposition (Deyo and Weinstein, 2001; Cherif et al., 2020). To date, the clinical treatment for LBP mainly includes bed rest, administration of non-steroid anti-inflammatory drugs (NSAIDs) and analgesics, lumbar discectomy, and interbody fusion (Binch et al., 2021). However, these approaches only focus on temporarily alleviating the symptoms rather than targeting the pathogenesis, and as such the progression of IVDD is neither delayed nor reversed (Hu et al., 2020; DiStefano et al., 2021). Therefore, novel treatment modalities are needed in IVDD therapy. In recent years, indeed, emerging strategies for the treatment of IVDD has gained the attention of researchers; MSC-based therapies and exosomes from MSCs show promising effects and have potential clinical applicability (Melrose, 2016; Richardson et al., 2016; Vadala et al., 2016; Loibl et al., 2019; Croft et al., 2021). The efficacy of MSCs in IVDD repair has been demonstrated in preclinical studies (Orozco et al., 2011; Peroglio et al., 2013; Zhang et al., 2021), and more recently in clinical trials (Elabd et al., 2016; Centeno et al., 2017; Pettine et al., 2017). Originally, it was thought that the injected MSCs could phenotypically differentiate into nucleus pulposus-like cells to replace the damaged tissue (Risbud et al., 2004a; Risbud et al., 2004b; Steck et al., 2005). However, this hypothesis was later challenged by the findings from numerous studies in the last decade. There exists a consensus that MSCs exert their therapeutic functions in vivo mostly via the paracrine process, such as the release of signaling molecules including growth factors, cytokines, and extracellular vesicles (Fayazi et al., 2021; Mohd Noor et al., 2021). Among them, exosomes, as the main transmission medium for intercellular communication, participate in a variety of pathological and physiological functions by means of influencing the gene expression, migration, proliferation, apoptosis, and metabolism in the recipient cells (Pourakbari et al., 2019; Shi et al., 2021). Recent years have witnessed an increase in the amount of research being carried out on the therapeutic value of exosomes for the treatment of IVDD. In view of this, we have concentrated our attention on IVDD, MSCs, and exosomes in the present research. Specifically, we focused on the potential mechanisms of MSC-derived exosomes for IVDD treatment.
2 Pathology of IVDD
The intervertebral consists of an outer annulus fibrosus (AF) and center gel-like nucleus pulposus (NP), as well as 2 cartilaginous endplates (Zhang et al., 2019; Binch et al., 2021). In the human body, the IVD is known to be the largest avascular tissue (Liao et al., 2019b), the IVD has a very limited intrinsic healing potential. Several pathophysiological processes of IVDD include decreased proliferation and increased apoptosis of nucleus pulposus cells, growth of nerves and blood vessels into intervertebral disc tissue, metabolic disorders (decreased synthesis and increased degradation) of extracellular matrix, aging of annulus fibrosus, and calcification of cartilage endplate (Balague et al., 2012). These eventually result in the clinical symptoms due to the inability to maintain the normal hydrophilic characteristics of the intervertebral disc, a large loss of water content, partial atrophy of nucleus pulposus, the disappearance of the normal borderline between annulus fibrosus and nucleus pulposus, and loss of intervertebral height (Deyo and Weinstein, 2001) (Figure 1).
3 Current Treatments for Intervertebral Disc Degeneration Diseases
3.1 Conservative and Surgical Treatments
At present, IVDD therapy generally includes conservative symptomatic and surgical treatments (Park et al., 2021). Conservative treatment usually exhibits good results for LBP (van Middelkoop et al., 2011; Quentin et al., 2021). It primarily includes oral drugs (including opioids, non-steroidal anti-inflammatory drugs (NSAIDs), skeletal muscle relaxants, acetaminophen), epidural steroid injections, and physical therapy. Surgical treatment tends to be restricted to people with severe or worsening symptoms, often after the conservative treatment has failed (Deyo and Weinstein, 2001; Binch et al., 2021). It includes interventional surgery (ozone radiofrequency ablation, etc.) and conventional surgery (discectomy, fusion, etc.) (Acosta et al., 2005; Binch et al., 2021). However, whether conservative treatment or surgical treatment are mainly targeted to the alleviation of pain symptoms rather than the underlying pathogenesis or reversing the process of IVDD and, although many patients respond in the short term, they generally have limited or no long-lasting clinical benefit. There are now a variety of biological and regeneration therapies aiming at targeting the illness at the cellular and molecular levels and reestablishing IVD functioning. As a result, these therapies have the potential to provide better clinical results.
3.2 Biologic and Small-Molecule Therapies
Since the exact mechanism of IVDD has not been completely understood, conservative therapies seem to be mostly inefficacious, and contemporary surgical interventions concentrate predominantly on removing pathological disc tissue and fusing the spine1. Various novel biologic therapies for IVDD degeneration have been evolved, including growth factor injection, platelet-rich plasma, gene therapy, cell-based therapy, and so on. Studies on small-molecule therapies have also been conducted.
3.2.1 Growth Factor-Based Therapy
The homeostasis of IVDD is regulated by actively maintaining the balance between catabolism and anabolism of IVD cells. Several growth factors have been reported to maintain this balance (Kennon et al., 2018). Han et al. (2015) have suggested that the direct injection of growth factors was among the most promising biological treatment modalities for the regeneration or repair of an IVD that has suffered from deterioration. Accumulating evidence implies that growth factors including growth and differentiation factor-5 (GDF-5), TGF-β, BMP-2, OP-1, basic fibroblast growth factor (bFGF/FGF2), epidermal growth factor (EGF), and IGF-1 in some models could promote cell proliferation and matrix synthesis (Imai et al., 2007; Ellman et al., 2008; Masuda, 2008; Cho et al., 2013; Travascio et al., 2014). It is worth noting that the response of different growth factors to different matrix structures and cellularity of the deteriorating disk is heterogeneous. Exogenous GDF-5, BMP-2, and TGF-βrepaired inner annular chondrocytes in degraded IVDs by promoting synthesis of NP and inner annulus, while IGF-1/b-FGF had little effect on these cells (Imai et al., 2007; Masuda, 2008; Cho et al., 2013; Travascio et al., 2014). Proteolytic enzymes such as ADAMTS and MMPs, as well as pro-inflammatory cytokines, act as an important role in the ECM degradation of NP. So, the injection of specific inhibitors or antagonists of these enzymes into the IVD is considered as a viable method to inhibit further degradation of ECM which was caused by the target protein hydrolases (Vo et al., 2013; Han et al., 2015). However, owing to the biological factors having short half-lives, the extremely low number of cells that are viable in terms of the capacity to be activated in IVDs that have already deteriorated IVDs, the limited effect of a single injection of growth factors, and the additional trauma and inflammatory reactions of repeated injections, all these factors make it difficult to apply growth factor-based therapy to clinical treatment (Mackiewicz et al., 2009; Kepler et al., 2013).
3.2.2 Platelet-Rich Plasma Therapy
Platelet-rich plasma (PRP) is known as an autologous blood concentrate of platelets. Once activated, it secretes a variety of chemokines, cytokines, growth factors, and other functional molecules (Wang et al., 2015). As a cocktail of multiple bioactive factors, PRP is widely clinically used as a therapeutic agent for tissue repair and regeneration. Several in vivo and in vitro investigations have shown the utility of PRP for IVD repair and regeneration (Obata et al., 2012; Wang et al., 2013; Pirvu et al., 2014). In addition as a therapeutic strategy, PRP has the merits of being low-cost, ease of preparation, ease of application, and autologous sources without rejection reaction (Chang and Park, 2021). Nevertheless, in view of the possible negative consequences of ossification, inflammation, and vascularization, more research on standardized formulations is of great necessity to validate the effectiveness and safety of PRP.
3.2.3 Gene Therapy
When compared to single injections of bio factors, gene therapy techniques are expected to possess a longer duration of action. Gene therapy is the process of delivering one or even more desirable genes into target cells with the aid of a vector (primarily a viral or non-viral vector) for the purpose of modifying the functioning state of targeted cells by releasing the product of the donor gene (Sakai and Grad, 2015). A large number of research reports have established strategies for delivering the desirable gene into IVD cells (Zhang et al., 2009; Liang et al., 2010; Ren et al., 2013). IVD regeneration has been investigated thoroughly using desired genetic encoding for anabolic factors (LMP-1, GDF-5, and BMPs), the transcription factor Sox-9 (Paul et al., 2003), anti-inflammatory agents (IL1ra) (Le Maitre et al., 2007), or metalloproteinase inhibitors (TIMP-1) (Wallach et al., 2003). Though gene therapy has shown promise, safety issues associated with immunogenicity, insertional mutations, and unregulated gene expression, as well as the assessment of long-term efficacy, must also be determined and addressed seriously. Furthermore, gene therapy is not a cost-effective solution because of the significant expense involved in the preparation of the vectors for gene delivery.
3.3.4. Small Molecules Therapy
Small molecular are defined as therapeutics with molecular weights of 600D or less, that can specifically bind to certain biological intracellular molecules and play a regulating role in particular biological processes (O'Shea et al., 2013). Unlike traditional drugs, small molecules are usually inhibitors of the relevant signaling pathway, which provides a promising target in the development of IVDD (Chen et al., 2017; Binch et al., 2021). Several studies have shown that small-molecule exhibited beneficial effects mainly through attenuation inflammatory signaling, apoptosis, catabolism, oxidative stress, and activation of anabolism by regulating a variety of signaling pathways (Colombier et al., 2014; Sakai and Grad, 2015; Chen et al., 2016; Li et al., 2017a; Pan et al., 2018; Zhang et al., 2018; Lin et al., 2019). Above, small molecular therapy is a positive alternative therapy for IVDD. Several of these small molecules have been shown to be effective in preventing disc cell deterioration. Furthermore, they are also crucial components in the regeneration of injured IVD in view of the fact that they regulate enzymes that are involved in the metabolism process (Kamali et al., 2021). Additionally, in contrast with cell- or growth factor-based treatment, small molecules are rarely biodegraded in vivo and are usually seen as a more cost-effective treatment option (Sun et al., 2019). However, small-molecule drugs still have relatively few clinical applications in IVDD. Currently, they do not show significantly better than NSAIDs based on existing clinical evidence (Daily et al., 2016; Chen et al., 2017; Grothe et al., 2017). The possible reasons are as listed: Firstly, small molecules always have weak specificity, which may affect other tissues resulting in unexpected side effects when systemically administered. Secondly, single-target strategies have not always brought optimistic results, due to complex diseases often needing to be tracked at various levels by modulating several targets simultaneously. The third reason is the limited number of clinical trials that have been conducted in this field, inadequate in vivo data as a consequence of a scarcity of comparable animal models, and the absence of patient stratification techniques to determine who would gain considerable benefit from a small molecule-based therapy.
Next-generation treatment strategies are based on regenerative medicine (Fernandez-Francos et al., 2021). The aim is to develop therapies that prevent LBP by delaying the IVD deterioration and promoting its repair. These strategies can be roughly split into two groups: cell-based and cell-free therapies (DiStefano et al., 2021); among the cell-free systems, exosomes have received a great deal of interest as a potentially effective therapeutic approach for IVDD in recent years.
4 Mesenchymal Stem Cell-Based Therapy in IVDD Treatment
MSCs are pluripotent non-hematopoietic stem cells that may be obtained from a wide range of sources, such as the umbilical cord blood, urine, adipose tissue, liver, kidney, bone marrow, placenta, and so on (Meng et al., 2013; Quang et al., 2018). A minimum of three conditions must be met by MSCs in accordance with the report released by the International Society for Cellular Therapy (ISCT) (Dominici et al., 2006). In addition, when developed in vitro, MSCs are considered to be plastic-adherent. Moreover, MSCs should express the surface markers CD105, CD90, and CD73, while expressing none of the hematopoietic markers CD34, CD45, CD11b or CD14, CD19 or CD79α and HLA-DR. Furthermore, when grown under particular circumstances, MSC should be differentiated into chondroblasts, adipocytes, and osteoblasts. Different MSCs have emerged as trendsetters in the field of cell therapy research due to their ease of isolation and specific biological functions (Wang X. et al., 2019). On the one hand, because of their pluripotency, MSCs were believed to replicate and differentiate into specialized cells to replace the damaged tissue (Richardson et al., 2016; Fernandez-Francos et al., 2021); MSCs, on the other hand, may produce bioactive molecules in response to their contact with the host niche including platelet-derived growth factor (PDGF), matrix metalloproteinase-9, matrix metalloproteinase-2 (MMP-2), prostaglandin E2 (PGE2), interleukin-6 (IL-6), and, insulin-like growth factor-1 (IGF-1), and ultimately have the functions of anti-apoptosis, immunomodulatory, anti-inflammatory, angiogenic and anti-fibrosis (Ha et al., 2020; Sun S.-J. et al., 2021; Shi et al., 2021). As a consequence, the utility of MSC-based therapeutics has been brought to the forefront of research. Actually, mesenchymal stem cells (MSCs) have shown safety as well as therapeutic significance in the field of regenerative medicine (Mocchi et al., 2020). Currently, about 1,000 clinical trials worldwide have been reported to use MSCs to treat various diseases (Pittenger et al., 2019; Kabat et al., 2020), including inflammatory and fibrotic diseases (Ryu et al., 2020); liver disease (Zhu et al., 2021), bone and joint diseases (Li N. et al., 2020), inflammatory bowel diseases (Zhang X. et al., 2020), diabetes (Kuang et al., 2021), cardiovascular diseases (Chen et al., 2021), neurodegenerative diseases (Fayazi et al., 2021), spinal cord injuries (Huang et al., 2021) and so on. Likewise, the emergence of cell-based therapies has also paved the way for utilizing MSCs in IVDD treatment. A large number of preclinical studies have established the effectiveness of MSCs in the treatment of IVDD (Vadala et al., 2016; Sinkemani et al., 2020). On the one hand, under appropriate culture conditions, mesenchymal stem cells may differentiate into NP-like cells. For example, Stoyanov et al. (2011) examined if it was feasible to guide bone marrow-derived MSCs to take on the phenotype of natural killer cells (NPs). They discovered that hypoxia, growth/differentiation factor 5 (GDF5), and induction of markers associated with NP-cell-like phenotype by NPC co-culture have been shown to have an effect on the expression profiles of bone marrow-derived MSCs in humans. More recently, a similar finding was reported by Clarke et al. (2014). Other growth factors, including transforming growth factor-beta 3 (TGF-β3) and especially growth/differentiation factor 6 (GDF6), in addition to GDF5, were shown to significantly elevate the NP marker genes expressions in MSCs. Moreover, in an animal experiment in vivo, Sakai et al. (2005) transplanted autologous bone marrow MSCs labeled with green fluorescent protein into a rabbit model of the IVDD. Two weeks after transplantation, a large number of cells expressing green fluorescent protein were found in the transplantation space. Compared with the non-transplantation group, the expression of type II collagen and proteoglycan in the intervertebral disc of the transplantation group was substantially elevated, which indicated that the transplanted MSC could differentiate into the nucleus pulposus cells in the hypoxic environment of the IVD.
On the other hand, MSCs are reported to produce a wide range of trophic factors to rescue and activate nucleus pulposus cells isolated from degenerative intervertebral discs by enhancing ECM synthesis, down-regulating pro-inflammatory cytokines, and up-regulating NP marker genes (including SOX9, type II collagen, and proteoglycans) within the diseased disc (Barakat et al., 2019). In many preclinical studies promoting IVD regeneration, the role of this MSC paracrine has been proved (Qi et al., 2019; Zeng et al., 2020). A study (Qi et al., 2019) that induced nucleus pulposus mesenchymal stem cells (NPMSCs) apoptosis through high glucose (HG) treatment found that MSC conditioned medium (MSC-CM) has resumed the collagen II and aggrecan expression in NPMSCs, thereby alleviating extracellular matrix degradation. Another study (Zeng et al., 2020) also demonstrated that CM derived from umbilical cord-derived MSCs (UCMSCs) might enhance the regeneration ability of nucleus pulpo-sus-derived progenitor/stem cells from degenerated disc (D-NPSCs) by enhancing their proliferative and stemness abilities, indicating the prospects of treatment approach to reinvigorate IVD progenitor/stem cells via paracrine signaling, which could be achieved with the aid of viable factors generated from MSCs.
The utility of cell-based MSC treatment, on the other hand, has certain limitations. First, although results of cell transplantation (through injection) are preliminary for the treatment of intervertebral disc degeneration, most studies showed that the transplanted cells could not adapt to intra-disc ischemia and hypoxia and thus could not survive (Binch et al., 2021). This greatly limits the therapeutic effect of stem cells. Second, MSC-based therapies may lead to the risk of granulocytosis, graft rejection, ectopic bone formation, microvascular embolism, and tumorigenicity (Ryu et al., 2020; Sun S.-J. et al., 2021). Third, the standardized culture procedures, the timings, the methods of treatment, and the selection of donor cells need to be studied further (Binch et al., 2021). Therefore, an effective treatment strategy should not include the direct participation of stem cells. Indeed, accumulating evidence showed that the efficacy of cell-based therapies is mainly attributed to paracrine signaling (Ha et al., 2020; Ratajczak and Ratajczak, 2020; Bao and He, 2021; DiStefano et al., 2021). Novel therapeutic methods are developed as a result of this discovery, including the creation of cell-free techniques on the basis of the utilization of secretome fractions such as vesicular and soluble fractions as a possibly more attractive option to cell treatment. The identification and application of soluble vesicles in the secretome as a substitute for cell-free therapy is a hot spot of current research. As most cell types, including MSCs, can produce exosomes with comparable therapeutic effects, exosomes containing various paracrine mediators are a potential and attractive cell-free therapeutic strategy for IVDD.
5 MSC-Derived Exosomes
5.1 Exosome Biogenesis
Exosomes, a subset of extracellular vesicle (EVs), are phospholipid vesicles that are membrane-bound and released by nearly all tissues, cells, and body fluids (plasma, urine, saliva, amniotic fluid, gastrointestinal secretions, semen, synovial fluid, and breast milk) (Pegtel and Gould, 2019). EVs are classified into three categories as follows: exosomes (30–100 nm), microparticles (100–1,000 nm), and apoptotic bodies (1,000–5000 nm) (Malekpour et al., 2021) (Table 1). In addition to the differences in their size, the biogenesis of exosomes is a hallmark feature that distinguishes them from other EVs. Unlike microvesicles which are generated directly from the plasma membrane and apoptotic bodies that are generated in the process of apoptosis, the exosomes have endosomal origins (Birtwistle et al., 2021). Exosomes are derived from an endosomal compartment, then released into the extracellular environment upon fusion of multivesicular bodies (MVBs) comprising of intraluminal vesicles (ILVs) with the plasma membrane. (Figure 2). The endosomal sorting complexes required for transport (ESCRT) located on MVBs regulate exosomes loading and biogenesis (Birtwistle et al., 2021). The ESCRT is a family of proteins that associate in successive complexes (ESCRT-0, -I, -II, and -III) at the membranes of MVBs for the purpose of regulating the production of ILVs as well as their cargo (Gupta and Pulliam, 2014). Many associated proteins (such as Tsg101, Alix, VPS4) also participate in these processes (Birtwistle et al., 2021). Tsg101 (typical tumor susceptibility gene 101 protein) is a component of the cellular ESCRT-I complex, whereas Alix (encoded by PDCD6IP) is an auxiliary protein of the ESCRT-III complex (Wang B. et al., 2019). Furthermore, exosome biogenesis may be regulated by ESCRT-independent machinery, which is reliant on Hsp70 binding phospholipids to construct MVBs (DiStefano et al., 2021). Moreover, the biogenesis, as well as the release of exosomes generated by MSC, are both dependent on external cues to function normally. Moreover, under various stimuli, different contents and compositions of exosomes secreted from the same parental cells were observed (Stoyanov et al., 2011; Babaei and Rezaie, 2021). Upon being produced from the cell, exosomes have been found to be either guided to and taken up by recipient cells that are present in the microenvironment or are transported to other regions by the body’s circulatory system. The exosomes are mostly taken up by the recipient cells via three routes: 1) ligand-receptor interaction; 2) endocytosis of target cells; 3) direct membrane fusion (Sun S.-J. et al., 2021; Fayazi et al., 2021; Liu et al., 2021) (Figure 2). The release of exosome contents into the cytoplasmic space of recipient cells occurs after the exosomes merge with recipient cells, facilitating the horizontal transmission of their cargo.
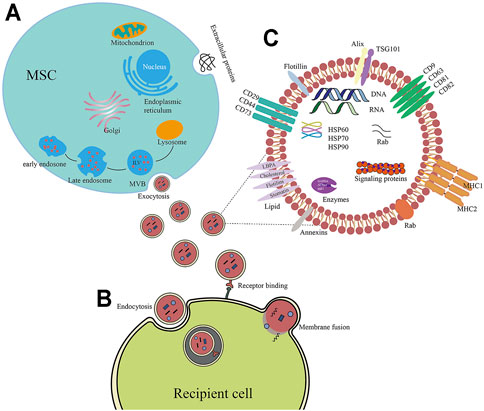
FIGURE 2. (A) The molecular composition of exosomes generated from MSCs. Exosomes generated from MSC contain adhesion molecules (CD73, CD44, and CD29), tetraspanins (CD9, CD63, and CD81), multivesicular body biogenesis-related proteins (Tsg101, Alix), and heat shock proteins. In addition to proteins, exosomes also comprise enzymes, lipids, and nucleic acids (DNA, miRNA, microRNA, and mRNA). (B) Biogenesis of exosomes produced from MSCs. As exosomes, these vesicles of endocytic origin are generated when the multivesicular body (MVB) membrane is subjected to inward budding, resulting in the formation of exosomes. ILV is found in MVBs. The production of exosomes occurs as a consequence of the fusion of MVBs with their plasma membrane. (C) Exosomes may infiltrate target cells by a variety of pathways, including membrane fusion, binding receptors, and endocytosis.
5.2 MSC-Derived Exosomes Components
Exosomes’ molecular structure is highly variable, depending on the parent cell type, epigenetic modifications, and numerous pathophysiological environmental factors (DiStefano et al., 2021). The exosomal components mainly include nucleic acids, proteins, and lipids and these determine the biological functions of the exosomes. In recent years, with the rapid development in biotechnology including transcriptomics, proteomics, lipidomics, and bioinformatics, the compositions of exosomes have been well studied, which provide a theoretical basis for exocrine therapy of diseases (Yu et al., 2014). The exosomal lipids mainly include phosphatidylserine, phosphatidic acid, cholesterol, sphingomyelin, arachidonic acid, prostaglandins, and leukotrienes; these not only participate in the formation of exosomes but also performs an instrumental function in the maintenance of their biological stability (Pegtel and Gould, 2019). Exosomes are also abundant in a variety of multifunctional proteins, including those involved in exosome synthesis (for example, the syntenin, ALIX, TSG101, and ESCRT complex), as well as membrane transporter and fusion proteins (for example, annexins, heat shock protein, and, Rab GTPases) (Xunian and Kalluri, 2020). In addition to proteins and lipids, various nucleic acids such as genomic DNA, cDNA, mitochondrial DNA (mtDNA), long noncoding RNAs (lncRNAs), circularRNAs, microRNAs (miRNAs), and mRNAs are excessively enriched in exosomes (Yu et al., 2014; Nakamura, 2019). These molecules have a role in the epigenetic remodeling of cells and the modulation of biological functions. Nevertheless, the cargo of each exosome colony differs significantly depending on the tissue and cell types of the secretory population (Ibrahim and Marban, 2016).
MSCs are known to produce more exosomes than do other types of primary cells (Yu et al., 2014). Proteomic analyses show that a total of 1,927 different proteins in MSC exosomes have multifaceted functions necessary for their functional and biogenesis characteristics (Li et al., 2020b). Not only do MSC-derived exosomes express ubiquitous exosome surface proteins including tetraspanins (CD81, CD63, and CD9), Alix, and Tsg101, as well as heat shock proteins (HSP90, HSP70, and HSP60), but also the MSC membrane proteins for several adhesion molecules such as CD73, CD44, and CD29. (Schorey and Bhatnagar, 2008; Colombo et al., 2014) (Figure 2). Like general exosomes, diverse types of nucleic acids including lncRNAs, miRNAs, and mRNA are enriched in MSC exosomes (Vlassov et al., 2012). They perform an integral function in changing the fate of recipient cells. Through the mechanism of exosome-cell contact, exosomal RNAs may be translated inside recipient cells, resulting in gene suppression and post-transcriptional modulation (Wu et al., 2020). Among them, micro-RNAs (miRNAs) have constantly garnered greater interest (Li et al., 2019; Zhang C. et al., 2020). miRNAs are thought to be a form of non-coding RNA which are approximately 22 nucleotides in length (Xie et al., 2020). They modulate post-transcriptional gene expression as they bind to the 30 UTR of the target mRNAs (Ma et al., 2020). miRs are among the major MSC exosomal effector molecules, which can participate in multiple biological processes including cell differentiation, apoptosis, angiogenesis, and inflammatory pathways. For example, tumor growth inhibitor miRs (miR-122, miR-125b, miR-24, miR-31, miR-223, miR-451, miR-214, and miR-23b) (Hassanzadeh et al., 2021) and inflammation modulatory miRs (miR-146 and miR-155) (Hulsmans and Holvoet, 2013) have been reported in MSC-derived exosomes. Notably, a single miR may control numerous messenger RNAs and a single messenger RNA could also be modulated by many miRs (Jin and Lee, 2015; Kong et al., 2015). These complex regulatory networks confer on MSC exosomes the potential to produce multiple functional effects, which in turn mediate various physiological and pathological processes.
6 Methods for Exosome Isolation and Identification
6.1 Isolation of Exosomes
There are several isolation techniques including centrifugation, ultrafiltration, size exclusion chromatography, polymer precipitation, immune-affinity capture, and microfluidics.
Centrifugation could either be ultracentrifugation or sucrose density gradient centrifugation. Ultracentrifugation is most commonly used and is known as the “gold standard” for separating exosomes (Ailuno et al., 2020). Ultracentrifugation is based on the differential sedimentation rate of proteins, vesicles, cell debris, and cells in uniform suspensions. By progressively elevating the centrifugal force and duration, cells and bigger particles can be eliminated. The exosomes are separated at ≥100,000 g/min and >2 h centrifugation (Gupta et al., 2018). As the density of exosomes is approximately 1.13∼ 1.19 g/ml (Raposo et al., 1996; Thery et al., 2006; Tauro et al., 2012), the density gradient centrifugation can be used for their separation. This method combines ultracentrifugation with a sucrose cushion or sucrose density gradient, and is based on the principle of separation and concentration according to gradient density after the removal of non-vesicular substances; exosomes with higher purity can be obtained using this method (Tauro et al., 2012). However, this method is time-consuming and has a lower yield. The quantity and quality of exosomes obtained are susceptible to uncertainties due to external factors. Further, it is difficult to separate other impurities of similar density, such as vesicles and proteins, from the obtained exosomes (Wang et al., 2021).
Size exclusion chromatography (SEC) is a chromatographic technique that separates small-molecule–protein complexes based on their size. (Veyel et al., 2018). The technique requires a chromatographic column made of porous polymer. First, the process involves removing cells as well as bigger particles via centrifugation at a low speed, subsequently filtering twice with a filter, and final purification by SEC (Sokolova et al., 2011). The most dazzling advantage of this method is that it requires only low centrifugal force, and hence avoids the demolition of the vesicle structure to a greater extent. However, the purity of the produced exosomes is lesser. The chromatogram column is easy to block and the adhesion leads to the loss of exosomes (Tauro et al., 2012; Xu et al., 2019).
Ultrafiltration (UF) is another technique of separation based on the size of exosomes (Sidhom et al., 2020). According to the diameter of exosomes, an ultrafiltration membrane that corresponds to the closest molecular weight is used to separate exosomes by centrifugation or pressurization (Li et al., 2017b). It is simple and rapid, but it has an obvious drawback that it is difficult to separate exosomes from other extracellular vesicles with similar diameters (Yu et al., 2018). The adhesion to the ultrafiltration membrane reduces the yield of exosomes, and the external force applied in the process of ultrafiltration may also cause the deformation or rupture of exosomes.
Isolation by immune-affinity chromatography is based on the interaction between antigens and antibodies with high specific affinity (Schiel and Hage, 2009). It is mainly used for the separation and purification of biological macromolecules. Certain antibodies against specific exosome surface indicators, including tetraspanins and TSG101 proteins, are generally utilized for exosome extraction and purification (Mondal and Whiteside, 2021).
Polymer precipitation is a process in which exosomes are precipitated from body fluids or cell cultures by altering their solubility or dispersion (Wei et al., 2021). It is usually used to precipitate exosomes from samples using polyethylene glycol or agglutinin. Currently, commercial kits such as ExoQuick and Exoquick-Tc kits from System Biosciences Company are available for extracting exosomes using polyethylene glycol (PEG) solutions. It is simple to use and does not require any special equipment; however, it is expensive. The reaction with body fluids or cell culture medium is incubated overnight at 4 °C and then centrifuged at a low speed.
Microfluidics is a new technology that uses micro-and nano-sized tubes to process and manipulate fluids (Chen et al., 2020). It can be divided into three categories: immune affinity, screening, and porous structure capture (Liga et al., 2015). Compared to the other techniques, this method is portable, fast, cost-effective, and automated, with a higher recovery rate. However, as it is a new emerging technology, it lacks standardization and large-scale sample data. Hence this method needs to be verified in future studies.
6.2 Identification of Exosomes
Currently, exosomal identification generally relies on the morphology, size, and their marker proteins using various methods including western blot analysis (WB), flow cytometry (FCM), nanoparticle tracking analysis (NTA), atomic force microscopy (AFM), scanning electron microscope (SEM), and transmission electron microscope (TEM) (Sokolova et al., 2011; Yang et al., 2019). The size and morphology of exosomes are usually observed using TEM, SEM, and AFM; among these TEM is most commonly used (Liu et al., 2018). NTA is used for the purpose of examining the exosome concentration and diameter. FCM and WB may detect exosomal surface marker proteins, including CD82, CD81, CD63, and CD9 (Yaghoubi et al., 2019). Usually, exosome identification involves a combination of two or more of these methods for a comprehensive assessment.
7 The Therapeutic Promise of Exosomes Generated From MSCs in a Variety of Tissues
Exosomes have been shown to have favorable effects in a significant number of experiments conducted over the last decade. Compared to the MSCs, MSC-derived exosomes not only play an indispensable role in tissue repair and regeneration but also have a more lasting, potent, and “easier-to-preserve” role (Mohd Noor et al., 2021). Owing to their small size, MSC-derived exosomes have greater stability and mitigate the risks associated with the administration of live cells, such as the possibility of microvascular blockage throughout the body. In addition, exosomes as small transporters can also easily get through the blood-brain barrier, while MSCs cannot.
In fact, many pre-clinical trials have illustrated the importance of exosomes derived from MSC in regaining tissue homeostasis and enhancing tissue regeneration. For example, exosomes derived from BM-MSCs (Collino et al., 2017) play a protective role in mice with acute renal injury. It involves the transfer of mRNAs and microRNAs to injured adrenocortical cells, which results in the reduction of apoptosis. Zhu et al. (2014) reported that MSC-derived exosomes alleviate acute lung injury (ALI) triggered by endotoxin in mice by transferring keratinocyte growth factor (KGF) mRNA to the injured alveoli. Previous studies also demonstrated that owning to the ability to cross the blood-brain barrier, MSC-derived exosomes may become an ideal therapy option for various degenerative brain disorders such as Parkinson’s disease, stroke, autism and Alzheimer’s disease (Perets et al., 2019; d'Angelo et al., 2020; Saint-Pol et al., 2020). Furthermore, modified exosomes were shown to be an effective vehicle for the administration of physiologically active components as well as medications. In addition to protecting payloads from enzymatic destruction, exosomes also enable them to target cells in a passive and particular manner. Overall, this is achieved due to their distinctive double-layered membrane structure (Liang et al., 2020; Han et al., 2021).
Moreover, in musculoskeletal repair and regeneration, the research using exosomes is still in its infancy. In previous studies (Pourakbari et al., 2019; Mohd Noor et al., 2021), the chondroprotective, anti-inflammatory, and antioxidant characteristics of MSC-derived exosomes have been found to deliver promising therapeutic benefits in the treatment of osteoarthritis (OA). As the most common forms of degenerative joint diseases, the phenotypic signs of OA and IVDD are very similar despite some obvious differences in their etiology and pathophysiology (Rustenburg et al., 2018). This suggests that at least a part of the treatment mechanism using MSC exosomes in OA can be used for the repair of intervertebral disc degeneration.
8 Impacts of MSC-Derived Exosomes on IVDD
Although the application of MSC-derived exosomes in IVDD treatment is still at an early stage, it is clear that there is growing interest in its use. Reduced ECM content, cell loss, inflammation, and increased oxidative stress are the pathophysiological hallmarks of IVDD. Encouragingly MSC-derived exosomes may restore the deteriorated IVD by targeting these four elements of the disease. Table 2 summarizes the findings of existing studies using MSC-derived exosomes in IVD regeneration and repair. In these 16 rescue-based research, MSC-derived exosomes were extracted from a number of cell sources, such as placenta-MSCs (1 study), umbilical cord (UC)-MSCs (1 study), adipose (AD)-MSCs (1 study), BM-MSCs (10 studies), unspecified (2 studies) tissues and human-induced pluripotent stem cells (1 study). Despite the differences in the tissue source, MSC-derived exosomes exert similar therapeutic effects in a pre-clinical model of IVDD mainly by restoring ECM homeostasis, promoting cell proliferation, reducing cell death, regulating inflammatory response, and attenuating oxidative stress. The specific mechanisms are described below:

TABLE 2. Pre-clinical studies investigating the therapeutic potential of stem cell-derived exosomes on IVDD.
8 1 Restoring Extracellular Matrix Homeostasis
One of the most significant characteristics of IVDD is the disruption of ECM homeostasis (Lu et al., 2019). Normally, anabolism and catabolism of ECM in IVD cells are in a dynamic balance, which is regulated by matrix catabolic factors (including matrix metalloproteinases (MMPs), matrix anabolic factors (including transforming growth factor (TGF)), and disintegrins and metalloprotease with thrombospondin motifs (ADAMTSs) (Chen et al., 2019). In addition, tissue inhibitors of metalloproteinases (TIMPs) can also regulate the activity of matrix-degrading enzymes (Chu et al., 2019). Earlier research reports have confirmed that when aging, injury, genetics or other exogenous stimuli disrupt the dynamic balance within the disc, certain pro-inflammatory cytokines including interleukin (IL)-1β and tumor necrosis factor (TNF)-a, may be secreted from IVD cells as well as infiltrating immune cells and lead to abnormal ECM remodeling via the mechanism of the up-modulation of catabolic enzymes (ADAMTSs and MMPs) and the downmodulation of ECM synthesis-related molecules (proteoglycan, aggrecan, and collagen type II) (Risbud and Shapiro, 2014) (Johnson et al., 2015). As a consequence, enhancing anabolism while simultaneously decreasing catabolism is critical for preserving the integrity of ECM and slowing the degeneration of intervertebral discs.
An accumulating amount of available data demonstrates that MSC-exosomes may effectively enhance the expression of extracellular matrix-related molecules and simultaneously inhibit their decomposition, thereby storing the ECM homeostasis (Bao and He, 2021; Mohd Noor et al., 2021). In previous studies, researchers used different in vitro cellular challenges (including IL-1β) (Zhu L. et al., 2020; Li Z.-q. et al., 2020), high glucose (Qi et al., 2019), LPS (Zhang J. et al., 2020), advanced glycation end products (AGEs) (Liao et al., 2019a), hydrogen peroxide (H2O2) (Bari et al., 2018; Xia et al., 2019), TNF-ɑ (Cheng et al., 2018; Zhu G. et al., 2020; Yuan et al., 2020), tert-butyl hydroperoxide (TBHP) (Xie et al., 2020), and acidic pH (Li et al., 2020a)) to simulate the degenerative microenvironment. For these biochemical challenges, exosomes produced from MSCs have been shown to have a protective impact on NPCs via the mechanism of increasing the expression of chondrocytes, aggrecan, and collagen II in the extracellular matrix and decreasing matrix disintegration via the mechanism of the down-modulation of matrix-degrading enzymes. Moreover, the effects of MSC-derived exosomes on ECM metabolism in IVD cells without any biochemical challenge have also been investigated (Lu et al., 2017; Hingert et al., 2020). One study (Lu et al., 2017) showed that after the intervention of exosomes derived from MSC, the ECM expression in degenerated nucleus pulposus cells increases significantly. The up-modulation of TIMP-1 and downmodulation of MMP-1/MMP-3 also indicate a healthier balance between catabolism and anabolism. Similar findings were presented by Hingert et al. (2020) When comparing degenerated disc cell pellets treated with MSC-derived exosomes to control cultures, it was observed that ECM synthesis was over three times greater in the treated pellets. MMP-1 secretion in the degenerated disc cells was similarly reduced as a result of the exosome therapy.
Furthermore, subsequent research has proven the strong relationship between miRNAs and the progression of IDD, which partially explains the effective role and its underlying mechanism of MSC exosomes in the IVD (Zhu G. et al., 2020; Wen et al., 2021). MicroRNA-199a in BMSC-secreted exosomes showed a superior protective role against ECM degradation by targeting GREM1 to inactivate the TGF-βpathway. Exosomes from BMSCs contain miR-532-5p, which inhibits matrix disintegration via the mechanism of targeting MMP-13 in degraded NPCs, resulting in the mitigation of IDD (Zhu G. et al., 2020). Moreover, Sun et al. demonstrated that iMSC-exosomes loaded exogenous miR-105-5p performed an integral function in the iMSC-sEV-mediated treatment impact by downregulating the catabolism markers of the ECM (ADAMTS-4 and MMP-3) and upregulating the anabolism markers (aggrecan and collagen II) (Sun Y. et al., 2021). This indicates that delivering exogenous micro-RNA via MSC exosomes might be a promising therapeutic approach for IVDD.
Together, the abovementioned findings suggest that MSC-derived exosomes have a pro-regenerative effect on terminally differentiated cells that have been derived from the degenerated IVD tissue via inhibition of catabolism and promotion of ECM synthesis.
8.2 Cell Proliferation Stimulation and Apoptosis Inhibition
In addition to an inflammatory environment, the central portion of degenerating discs comprises hypoxia, low glucose, elevated osmotic pressure, increased mechanical loading, low pH, and nutrient deficiency, which decreased cell viability and increased cell apoptosis (Ding et al., 2013; Sakai and Andersson, 2015). Exosomes produced from MSCs have been shown to enhance the proliferation of IVD cells while simultaneously inhibiting their apoptosis (Hu et al., 2020).
8.2.1 NP Cells
Most of the studies regarding IVD degeneration have been focused on nucleus pulposus cells (NPCs), which are the resident cells of the disc and perform instrumental functions in maintaining the normal function of IVD (Jia et al., 2016). Excessive nucleus pulposus cells apoptosis is one of the main reasons for the initiation and progression of IVDD (Guo et al., 2021). Previous studies showed that the co-culture of MSCs with nucleus pulposus cells not only stimulated the NPCs proliferation but also attenuated cell apoptosis in the degenerated IVD. Studies have shown that exosomes derived from MSC replicate these therapeutic effects of their parent cells (Faruqu et al., 2021; Zhu et al., 2021). For in vitro disc degeneration model induced by different biochemical challenges, including H2O2, LPS, IL-1β, TNF-ɑ, acidic pH, and high glucose, the administration of MSC-derived exosomes exerts pro-proliferation and anti-apoptosis effect via distinct mechanisms. One study (Cheng et al., 2018) reported that MSC-derived exosomes promoted NPC proliferation and prevented apoptosis in NPCs, and alleviate IVD degeneration through miR-21. Exosomal miR-21 restrains PTEN activity and thus regulates PI3K/Akt pathway in NPC apoptosis. Another study (Li et al., 2020a) demonstrated that MSC-derived exosomes were capable of preventing and attenuating NPC apoptosis by suppressing caspase-3 expression and alleviating caspase-3 cleavage triggered by acidic pH. In addition, Zhu G. et al. (2020) discovered that exosomes originating from BMSCs inhibit fibrotic accumulation, ECM disintegration, NPC apoptosis, and alleviate the progress of IDD through miR-532–5p. The RASSF5 has been shown to directly target the miR-532–5p gene which mediates the regulation of cell apoptosis. Similarly, miR-142-3p from MSC exosomes also stimulate NPCs proliferation and ameliorate the apoptosis of the NPC induced by IL1-β via targeting MLK3 to suppress MAPK signaling (Zhu L. et al., 2020).
Yuan et al. (2020) found that overexpression of miRNA-4450 in nucleus pulposus cells could promote cell apoptosis and inflammatory responses; conversely, the above results were opposite upon knocking out miRNA-4450 in the cells. Further bioinformatic analysis showed that miRNA-4450 could specifically bind to the zinc finger protein 121. When co-cultured with MSC-derived exosomes, the apoptosis rate in nucleus pulposus cells treated with TNF-ɑ decreased significantly. Thus, MSC-derived exosomes may reduce the apoptosis in nucleus pulposus cells by antagonizing the signaling pathways involving miRNA-4450 and zinc finger protein 121. Wen et al. (2021) also report that miR-199a from MSC exosomes promote the proliferation of NPCs and inhibit cell apoptosis via downregulating GREM1. Moreover, a recent study by our team also testified that exosomes originating from MSC might alleviate apoptosis induced by advanced glycation end products (AGEs) in vitro via activating AKT and ERK signaling pathways (Liao et al., 2019a).
8.2.2 Annulus Fibrosus Cells
Annulus fibrosus is a fibrous cartilage-like structure arranged in concentric circles outside the nucleus pulposus (van den Akker et al., 2016). It protects the jelly-like nucleus pulposus tissue and evenly distributes the pressure on it. Thus, the annulus fibrosus performs a critical function in maintaining the normal physiological function of the IVD. Li Z.-q. et al. (2020) found that BMSC-derived exosomes could not only promote proliferation but also significantly reduce the apoptosis induced by IL-1B in annulus fibrosus cells. Further studies show that IL-1B promotes autophagy-related proteins expression (LC3-II, LC3-I, and Beclin-1) and reduces the expression of P13K/mTOR/AKT signaling pathway-related proteins (p-mTOR and p-AKT). After the intervention using BMSC-derived exosomes, the effect of IL-1B could be reversed, and with the addition of rapamycin, an inhibitor of the H1TOR pathway, the expression of inflammatory cytokines and phagocytic associated proteins in BMSC-derived exosomes were significantly enhanced. Therefore, BMSC-derived exosomes may reduce the apoptosis in annulus fibrosus cells by inhibiting P13K/AKT/mTOR signaling pathway-mediated autophagy. Although there are few studies on the relationship between MSC-exosomes and annulus fibrosus apoptosis, given the important role of annulus fibrosus in IVDD, the inhibition of annulus fibrosus apoptosis by BMSC-derived exosomes need to be investigated in the future.
8.2.3 Cartilage Endplate Cells
The cartilage endplate (CEP) is known to be a hyaline cartilage layer existing between the vertebral bodies and intervertebral disc. Due to their rich micropore structure that allows nutrients and metabolites to pass through, the CEP is the predominant source of nutrients for the avascular IVD (Raj, 2008). Degeneration of the CEP, including calcification and excessive apoptosis of CEP cells, could impede nutrient diffusion, resulting in metabolic and functional disorders of IVD cells to initiate IVDD (Sakai and Grad, 2015). Therefore, CEP degeneration may be a pivotal initiating factor in IVDD pathogenesis, and inhibition of early CEP degeneration is very important to delay IVDD.
Currently, only a study focus on the relationship between MSC-derived exosomes and apoptosis in cartilage endplate cells (Xie et al., 2020). MSC-derived exosomes were shown to be effective in preventing and inhibiting apoptosis as well as calcification of cartilage endplate cells once exposed to oxidative stress caused by tert-butyl hydroperoxide (TBHP). Further analysis showed that MSC exosomes are rich in miRNA-31-5p, whereas down-modulation of miR-31-5p inhibited exosomal protective benefits on CEP. Pathway analysis showed that ATF6 was the miR-31-5p target, and the ATF6 expression was inversely modulated by miR-31-5p. It has also been shown thatATF6 can trigger cell apoptosis via the ER-stress pathway. CEPs were less susceptible to cell death and calcification when treated with MSC-exosomes, and the specific mechanism might be correlated with the pathway modulation of the miR-31-5p/ATF6-associated ER stress.
8.3 Anti-Oxidation Effects
Excessive oxidative stress is key in the early events of IVDD (Luo et al., 2019). It promotes the expression of catabolic factors, including MMP-3 and TNF-α (Song et al., 2018). Previous research shows that the pathological mechanisms underlying IVDD are closely associated with ROS and oxidative stress (Feng et al., 2017). Throughout the course of NP cell degradation, the homeostasis between the production of oxygen-free radicals and antioxidant defense is interrupted, thereby resulting in the buildup of reactive oxygen species (ROS), oxidative stress, and ultimately mitochondrial apoptosis in NPCs. Thus, anti-oxidative stress mechanisms are a feasible pathway for IVDD treatment. Recently, Bari et al. reported that although the freeze-dried secretome shows no free-radical scavenging activity, it was shown to be effective in protecting oxidative damage caused by oxidative stress at concentrations ranging from 5 to 50 mg/ml. According to the researchers, this capacity was due to the induction as well as the regeneration of physiologically active antioxidant systems inside cells (Bari et al., 2018). Xia et al. showed that attenuation of mitochondrial dysfunction caused by oxidative stress may greatly improve cellular homeostasis in NP cells once expose to IVDD. They hypothesized that MSC exosomes may provide mitochondrial proteins to NP cells, enabling the cells to repair the damaged mitochondrial components. The proteomic database developed by them also indicates that MSC exosomes contain antioxidant proteins including Prdx-1, Gpx 4, and Trap 1 (Xia et al., 2019). The separation and accurate identification of these proteins, nevertheless, would need more research. Moreover, our prior research showed that AGEs accelerated the onset of IDD by exacerbating mitochondrial dysfunction as well as oxidative stress. Inspired by this work and the therapeutic potential of MSC exosomes, we carried out a series of tests and demonstrated that MSC-derived exosomes might attenuate AGE-induced ER stress in vitro via the activation of the ERK and AKT signaling pathways. In addition, an in vivo trial demonstrated that the administration of MSC exosomes had a therapeutic impact on IVDD (Liao et al., 2019a).
8.4 Anti-Inflammatory Effects
Numerous studies have demonstrated that disc degeneration is associated with inflammatory response (Yang et al., 2015). During IVDD, degenerative intervertebral disc cells themselves produce proinflammatory chemokines includingCCL545, CCL4, CCL3, CXCL1, and XCL1 that are able to attract other leukocytes and produce pro-inflammatory cytokines including TNF-ɑ, IL-1, IL-2, IL-6, and IL-8 that quicken the IVDD progression by upmodulating the matrix catabolic enzymes (Cunha et al., 2018). As the degeneration cascade progresses, the production of pro-inflammatory molecules, particularly TNF–αand IL-1, increases rapidly. Although similar therapeutic effects were observed in these studies, the mechanism of action underlying MSC exosome-related alleviation of inflammation corresponds to the differences in the biochemical challenge. Exosomes from MSCs have been shown to have an anti-inflammatory effect on pathogenic NP cells via the mechanism of inhibiting the production of inflammatory markers and the activation of the NLRP3 inflammasome (Xia et al., 2019). MSC-exosomal miR-410 targets the 3′UTR of NLRP3 and reduces NPC pyroptosis after LPS treatment (Zhang J. et al., 2020).
Moreover, the accumulation of inflammatory factors can also increase the apoptosis of the annulus fibrosus, destroy its structure and function, and further aggravate the process of IVDD. Li et al. reported that exosomes generated from BMSC could not only enhance the proliferation of annulus fibrosus cells and reduce the apoptosis induced by IL-1B but could also reduce the production of inflammatory factors including prostaglandin E2, IL-6, and cyclooxygenase-2. Further analysis showed that BMSC-derived exosomes could reduce the inflammatory response in annulus fibrosus cells by inhibiting P13K/AKll/mTOR signaling pathway-mediated autophagy (Li Z.-q. et al., 2020). Zhu and his colleagues performed further investigations, demonstrating that BMMSCs-derived exosomes-packaged miR-142-3p mitigate inflammatory damage in NPCs via the mechanism of inhibiting the MAPK signaling pathway by targeting MLK3 (Zhu L. et al., 2020).
Therefore, the anti-inflammatory effects of MSC exosomes can combine the miRNA and corresponding messenger RNA functions to inhibit the formation of inflammatory bodies, the activation of inflammatory factors, and the inhibition of cell autophagy. Thus, reducing the series of inflammatory reactions could potentially delay IVDD.
9 Challenges and Prospects
Exosome-based therapy is a novel option for the regeneration and repair of intervertebral discs. During the last decade, many research reports have revealed the therapeutic potential of MSC-derived exosomes in the treatment of a variety of illnesses. However, current investigations into the therapy of IVDD using exosomes derived from MSCs are at the stage of exploration; the majority of the preclinical trials have revealed potential benefits of MSC exosomes on NPCs; only one study reported the effects of MSC exosomes in AF cells; one study used CEP chondrocytes. For future investigations on therapeutic application, studies should focus on a comprehensive understanding of the regeneration and protection characteristics of this treatment approach on all terminally differentiated types of cells in IVD. Furthermore, the functions of exosomes produced from primordial cells in the human IVD in the regulation of regeneration and repair in IVDD should be explored further in the future. Furthermore, most preclinical studies on IVDD have used rodents, which compared to humans, have inherent differences between species (Lisieski et al., 2018). In addition, drug-induced or acupuncture-induced IVD degenerative animal models and in vitro cell line models may not reflect the true effect of exosomes on human intervertebral disc degeneration.
As a carrier of natural source signal molecules, exosomes have the advantages of nano-scale size, the permeability of biological barriers, low immunogenicity, low cytotoxicity, convenient storage, and their clinical applications are rapidly emerging (Hade et al., 2021). Exosomes generated from MSCs have gained widespread attention in the field of regenerative medicine due to their promising potential to regenerate damaged tissues on a par with MSCs themselves (Sakai and Grad, 2015). Furthermore, earlier research has proven that MSC-derived exosomes are appropriate alternatives to available MSCs for the purpose of overcoming the limits of these cells (Akao et al., 2011). However, although many preclinical studies demonstrated the good therapeutic potential of MSC-derived exosomes for IVDD, several critical issues will require careful consideration before using MSC exosomes in clinical settings. First, the absence of worldwide uniformity in the purification, separation, and profiling of MSC exosomes may result in controversial outcomes across various laboratory-based investigations. Uniform consensus on these aspects should be adopted to facilitate comparative research. Moreover, the preservation, transit, and storage of exosomes are crucial, which should be taken into consideration seriously. In spite of the fact that a study has shown that freeze-drying can purify exosomes and facilitate transportation and storage (Bari et al., 2018); however, further studies are needed to prove whether freeze-drying can change the characteristics of exosomes. Second, although some research reports have shown that exosomes generated from MSC are effective and safe in IVDD treatment, the pharmacological characteristics, such as bio-distribution, bioavailability, targeting, and pharmacokinetics, of exosomes in vivo remain unknown (Ailuno et al., 2020). Further studies directed towards these characteristics are needed, so as to elucidate their mechanism of action. Third, the optimal route of administration (local or systemic), administration dosing, interval, potency assays, and minimizing dose toxicity has not been effectively solved at present (Pittenger et al., 2019). Fourth, it is necessary to optimize the culture conditions and isolation methods for exosomes (Elabd et al., 2016; Lui, 2021). In order to produce safer and more effective functionalized exosomes on a large scale to cater to the needs of clinical research, the selection of appropriate culture conditions is critical to the development of exosome-based treatments for IVDD in the future. In addition, effective and scalable exosomes isolation methods from MSCs culture medium also should be in compliance with good manufacturing practices capable of reproducible purity and potency, which are essential for the exosomes yield (Forsberg et al., 2020). Last, the therapeutic outcomes of exosomes follow mainly from their intrinsic cargoes like RNA, DNA, lipids, and proteins. Despite the protein and RNA compositions of these exosomes have been quantitatively characterized, the specific mechanisms involved in the regeneration process need to be further elucidated. Moreover, current studies have mostly focused on regulators of miRNA sorting into exosomes, the effects of other exosomal components such as ncRNA, lncRNA, circRNA, tRNA, lipids, and proteins on the physiological functions of exosomes need to be further studied in the future.
10 Conclusion
In conclusion, exosomes generated from a variety of MSC sources have been shown to effectively exhibit anti-apoptotic, anti-oxidative stress, anti-inflammatory, and ECM homeostatic capabilities when delivered to IVDD patients in pre-clinical investigations. Hence, their therapeutic biological functions may provide a novel potential cell-free treatment for the regeneration and repair of IVD while minimizing the risks associated with cell therapy. Our knowledge about the biology and application of MSC-derived exosomes, however, is still incomplete. Further studies on the optimal route, interval, and dose of administration, the underlying mechanisms of MSC exosomes biological action are essential for optimizing the therapeutic effects. Clinical trials of standardized and reproducible MSC-derived exosomes preparation are needed before they can be applied to clinical practices.
Author Contributions
Concept and design: LL, XW. Acquisition, analysis, or interpretation of data: LL, FG, HW, CT, SL, ZY, PL, YX, JZ. Drafting of the manuscript: LL and AX. Critical revision of the manuscript for important intellectual content: ZY, CT, CY, and XW. Obtained funding: ZY. Supervision: ZY and XW.
Funding
This work was supported by the National Natural Science Foundation of China (No. 81974349 and No.81974355) and the Science and Technology Commission of Hubei (grant no. 2018AAA067).
Conflict of Interest
The authors declare that the research was conducted in the absence of any commercial or financial relationships that could be construed as a potential conflict of interest.
Publisher’s Note
All claims expressed in this article are solely those of the authors and do not necessarily represent those of their affiliated organizations, or those of the publisher, the editors and the reviewers. Any product that may be evaluated in this article, or claim that may be made by its manufacturer, is not guaranteed or endorsed by the publisher.
References
Acosta, F. L., Lotz, J., and Ames, C. P. (2005). The Potential Role of Mesenchymal Stem Cell Therapy for Intervertebral Disc Degeneration: a Critical Overview. Foc 19 (3), 1–6. doi:10.3171/foc.2005.19.3.5
Ailuno, G., Baldassari, S., Lai, F., Florio, T., and Caviglioli, G. (2020). Exosomes and Extracellular Vesicles as Emerging Theranostic Platforms in Cancer Research. Cells 9 (12), 2569. doi:10.3390/cells9122569
Akao, Y., Iio, A., Itoh, T., Noguchi, S., Itoh, Y., Ohtsuki, Y., et al. (2011). Microvesicle-mediated RNA Molecule Delivery System Using Monocytes/macrophages. Mol. Ther. 19 (2), 395–399. doi:10.1038/mt.2010.254
Au, T. Y. K., Lam, T. K., Peng, Y., Wynn, S. L., Cheung, K. M. C., Cheah, K. S. E., et al. (2020). Transformation of Resident Notochord‐descendent Nucleus Pulposus Cells in Mouse Injury‐induced Fibrotic Intervertebral Discs. Aging Cell 19 (11), e13254. doi:10.1111/acel.13254
Babaei, M., and Rezaie, J. (2021). Application of Stem Cell-Derived Exosomes in Ischemic Diseases: Opportunity and Limitations. J. Transl Med. 19 (1), 196. doi:10.1186/s12967-021-02863-w
Balagué, F., Mannion, A. F., Pellisé, F., and Cedraschi, C. (2012). Non-specific Low Back Pain. The Lancet 379 (9814), 482–491. doi:10.1016/S0140-6736(11)60610-7
Bao, C., and He, C. (2021). The Role and Therapeutic Potential of MSC-Derived Exosomes in Osteoarthritis. Arch. Biochem. Biophys. 710, 109002. doi:10.1016/j.abb.2021.109002
Barakat, A. H., Elwell, V. A., and Lam, K. S. (2019). Stem Cell Therapy in Discogenic Back Pain. J. Spine Surg. 5 (4), 561–583. doi:10.21037/jss.2019.09.22
Bari, E., Perteghella, S., Di Silvestre, D., Sorlini, M., Catenacci, L., Sorrenti, M., et al. (2018). Pilot Production of Mesenchymal Stem/Stromal Freeze-Dried Secretome for Cell-free Regenerative Nanomedicine: A Validated GMP-Compliant Process. Cells 7 (11), 190. doi:10.3390/cells7110190
Binch, A. L. A., Fitzgerald, J. C., Growney, E. A., and Barry, F. (2021). Cell-based Strategies for IVD Repair: Clinical Progress and Translational Obstacles. Nat. Rev. Rheumatol. 17 (3), 158–175. doi:10.1038/s41584-020-00568-w
Birtwistle, L., Chen, X.-M., and Pollock, C. (2021). Mesenchymal Stem Cell-Derived Extracellular Vesicles to the Rescue of Renal Injury. Ijms 22 (12), 6596. doi:10.3390/ijms22126596
Centeno, C., Markle, J., Dodson, E., Stemper, I., Williams, C. J., Hyzy, M., et al. (2017). Treatment of Lumbar Degenerative Disc Disease-Associated Radicular Pain with Culture-Expanded Autologous Mesenchymal Stem Cells: a Pilot Study on Safety and Efficacy. J. Transl Med. 15 (1), 197. doi:10.1186/s12967-017-1300-y
Chang, M. C., and Park, D. (2021). The Effect of Intradiscal Platelet-Rich Plasma Injection for Management of Discogenic Lower Back Pain: A Meta-Analysis. Jpr Vol. 14, 505–512. doi:10.2147/JPR.S292335
Chen, D., Xia, D., Pan, Z., Xu, D., Zhou, Y., Wu, Y., et al. (2016). Metformin Protects against Apoptosis and Senescence in Nucleus Pulposus Cells and Ameliorates Disc Degeneration In Vivo. Cell Death Dis 7 (10)–e2441. doi:10.1038/cddis.2016.334
Chen, P., Li, S., Guo, Y., Zeng, X., and Liu, B.-F. (2020). A Review on Microfluidics Manipulation of the Extracellular Chemical Microenvironment and its Emerging Application to Cell Analysis. Analytica Chim. Acta 1125, 94–113. doi:10.1016/j.aca.2020.05.065
Chen, S., Liu, S., Ma, K., Zhao, L., Lin, H., and Shao, Z. (2019). TGF-β Signaling in Intervertebral Disc Health and Disease. Osteoarthritis and Cartilage 27 (8), 1109–1117. doi:10.1016/j.joca.2019.05.005
Chen, Y., Huang, J., Tang, C., Chen, X., Yin, Z., Heng, B. C., et al. (2017). Small Molecule Therapeutics for Inflammation-Associated Chronic Musculoskeletal Degenerative Diseases: Past, Present and Future. Exp. Cel Res. 359 (1), 1–9. doi:10.1016/j.yexcr.2017.07.027
Chen, Y., Shen, H., Ding, Y., Yu, Y., Shao, L., and Shen, Z. (2021). The Application of Umbilical Cord‐derived MSCs in Cardiovascular Diseases. J. Cel Mol Med 25, 8103–8114. doi:10.1111/jcmm.16830
Cheng, X., Zhang, G., Zhang, L., Hu, Y., Zhang, K., Sun, X., et al. (2018). Mesenchymal Stem Cells Deliver Exogenous miR-21viaexosomes to Inhibit Nucleus Pulposus Cell Apoptosis and Reduce Intervertebral Disc Degeneration. J. Cel. Mol. Med. 22 (1), 261–276. doi:10.1111/jcmm.13316
Cherif, H., Bisson, D. G., Mannarino, M., Rabau, O., Ouellet, J. A., and Haglund, L. (2020). Senotherapeutic Drugs for Human Intervertebral Disc Degeneration and Low Back Pain. Elife 9. doi:10.7554/eLife.54693
Cho, H., Lee, S., Park, S.-H., Huang, J., Hasty, K. A., and Kim, S.-J. (2013). Synergistic Effect of Combined Growth Factors in Porcine Intervertebral Disc Degeneration. Connect. Tissue Res. 54 (3), 181–186. doi:10.3109/03008207.2013.775258
Chu, Y., Wu, P.-Y., Chen, C.-W., Lyu, J.-L., Liu, Y.-J., Wen, K.-C., et al. (2019). Protective Effects and Mechanisms of N-Phenethyl Caffeamide from UVA-Induced Skin Damage in Human Epidermal Keratinocytes through Nrf2/HO-1 Regulation. Ijms 20 (1), 164. doi:10.3390/ijms20010164
Clarke, L. E., McConnell, J. C., Sherratt, M. J., Derby, B., Richardson, S. M., and Hoyland, J. A. (2014). Growth Differentiation Factor 6 and Transforming Growth Factor-Beta Differentially Mediate Mesenchymal Stem Cell Differentiation, Composition, and Micromechanical Properties of Nucleus Pulposus Constructs. Arthritis Res. Ther. 16 (2), R67. doi:10.1186/ar4505
Collino, F., Pomatto, M., Bruno, S., Lindoso, R. S., Tapparo, M., Sicheng, W., et al. (2017). Exosome and Microvesicle-Enriched Fractions Isolated from Mesenchymal Stem Cells by Gradient Separation Showed Different Molecular Signatures and Functions on Renal Tubular Epithelial Cells. Stem Cel Rev Rep 13 (2), 226–243. doi:10.1007/s12015-016-9713-1
Colombier, P., Clouet, J., Hamel, O., Lescaudron, L., and Guicheux, J. (2014). The Lumbar Intervertebral Disc: from Embryonic Development to Degeneration. Jt. Bone Spine 81 (2), 125–129. doi:10.1016/j.jbspin.2013.07.012
Colombo, M., Raposo, G., and Théry, C. (2014). Biogenesis, Secretion, and Intercellular Interactions of Exosomes and Other Extracellular Vesicles. Annu. Rev. Cel Dev. Biol. 30, 255–289. doi:10.1146/annurev-cellbio-101512-122326
Croft, A. S., Illien-Jünger, S., Grad, S., Guerrero, J., Wangler, S., and Gantenbein, B. (2021). The Application of Mesenchymal Stromal Cells and Their Homing Capabilities to Regenerate the Intervertebral Disc. Ijms 22 (7), 3519. doi:10.3390/ijms22073519
Cunha, C., Silva, A. J., Pereira, P., Vaz, R., Gonçalves, R. M., and Barbosa, M. A. (2018). The Inflammatory Response in the Regression of Lumbar Disc Herniation. Arthritis Res. Ther. 20 (1), 251. doi:10.1186/s13075-018-1743-4
Daily, J. W., Yang, M., and Park, S. (2016). Efficacy of Turmeric Extracts and Curcumin for Alleviating the Symptoms of Joint Arthritis: A Systematic Review and Meta-Analysis of Randomized Clinical Trials. J. Med. Food 19 (8), 717–729. doi:10.1089/jmf.2016.3705
d’Angelo, M., Cimini, A., and Castelli, V. (2020). Insights into the Effects of Mesenchymal Stem Cell-Derived Secretome in Parkinson's Disease. Ijms 21 (15), 5241. doi:10.3390/ijms21155241
Deyo, R. A., and Weinstein, J. N. (2001). Low Back Pain. N. Engl. J. Med. 344 (5), 363–370. doi:10.1056/NEJM200102013440508
Ding, F., Shao, Z.-w., and Xiong, L.-m. (2013). Cell Death in Intervertebral Disc Degeneration. Apoptosis 18 (7), 777–785. doi:10.1007/s10495-013-0839-1
DiStefano, T. J., Vaso, K., Danias, G., Chionuma, H. N., Weiser, J. R., and Iatridis, J. C. (2021). Extracellular Vesicles as an Emerging Treatment Option for Intervertebral Disc Degeneration: Therapeutic Potential, Translational Pathways, and Regulatory Considerations. Adv. Healthc. Mater., 2100596. doi:10.1002/adhm.202100596
Dominici, M., Le Blanc, K., Mueller, I., Slaper-Cortenbach, I., Marini, F. C., Krause, D. S., et al. (2006). Minimal Criteria for Defining Multipotent Mesenchymal Stromal Cells. The International Society for Cellular Therapy Position Statement. Cytotherapy 8 (4), 315–317. doi:10.1080/14653240600855905
Elabd, C., Centeno, C. J., Schultz, J. R., Lutz, G., Ichim, T., and Silva, F. J. (2016). Intra-discal Injection of Autologous, Hypoxic Cultured Bone Marrow-Derived Mesenchymal Stem Cells in Five Patients with Chronic Lower Back Pain: a Long-Term Safety and Feasibility Study. J. Transl Med. 14, 253. doi:10.1186/s12967-016-1015-5
Ellman, M. B., An, H. S., Muddasani, P., and Im, H.-J. (2008). Biological Impact of the Fibroblast Growth Factor Family on Articular Cartilage and Intervertebral Disc Homeostasis. Gene 420 (1), 82–89. doi:10.1016/j.gene.2008.04.019
Faruqu, F. N., Liam‐Or, R., Zhou, S., Nip, R., and Al‐Jamal, K. T. (2021). Defined Serum‐free Three‐dimensional Culture of Umbilical Cord‐derived Mesenchymal Stem Cells Yields Exosomes that Promote Fibroblast Proliferation and Migration In Vitro. FASEB j. 35 (1), e21206. doi:10.1096/fj.202001768RR
Fayazi, N., Sheykhhasan, M., Soleimani Asl, S., and Najafi, R. (2021). Stem Cell-Derived Exosomes: a New Strategy of Neurodegenerative Disease Treatment. Mol. Neurobiol. 58 (7), 3494–3514. doi:10.1007/s12035-021-02324-x
Feng, C., Yang, M., Lan, M., Liu, C., Zhang, Y., Huang, B., et al. (2017). ROS: Crucial Intermediators in the Pathogenesis of Intervertebral Disc Degeneration. Oxidative Med. Cell Longevity 2017, 1–12. doi:10.1155/2017/5601593
Fernández-Francos, S., Eiro, N., Costa, L. A., Escudero-Cernuda, S., Fernández-Sánchez, M. L., and Vizoso, F. J. (2021). Mesenchymal Stem Cells as a Cornerstone in a Galaxy of Intercellular Signals: Basis for a New Era of Medicine. Ijms 22 (7), 3576. doi:10.3390/ijms22073576
Forsberg, M. H., Kink, J. A., Hematti, P., and Capitini, C. M. (2020). Mesenchymal Stromal Cells and Exosomes: Progress and Challenges. Front. Cel Dev. Biol. 8, 665. doi:10.3389/fcell.2020.00665
Grothe, K., Flechsenhar, K., Paehler, T., Ritzeler, O., Beninga, J., Saas, J., et al. (2017). IκB Kinase Inhibition as a Potential Treatment of Osteoarthritis - Results of a Clinical Proof-Of-Concept Study. Osteoarthritis and Cartilage 25 (1), 46–52. doi:10.1016/j.joca.2016.08.010
Guo, Z., Qiu, C., Mecca, C., Zhang, Y., Bian, J., Wang, Y., et al. (2021). Elevated Lymphotoxin-α (TNFβ) Is Associated with Intervertebral Disc Degeneration. BMC Musculoskelet. Disord. 22 (1), 77. doi:10.1186/s12891-020-03934-7
Gupta, A., and Pulliam, L. (2014). Exosomes as Mediators of Neuroinflammation. J. Neuroinflammation 11, 68. doi:10.1186/1742-2094-11-68
Gupta, S., Rawat, S., Arora, V., Kottarath, S. K., Dinda, A. K., Vaishnav, P. K., et al. (2018). An Improvised One-step Sucrose Cushion Ultracentrifugation Method for Exosome Isolation from Culture Supernatants of Mesenchymal Stem Cells. Stem Cel Res Ther 9 (1), 180. doi:10.1186/s13287-018-0923-0
Ha, D. H., Kim, H.-k., Lee, J., Kwon, H. H., Park, G.-H., Yang, S. H., et al. (2020). Mesenchymal Stem/Stromal Cell-Derived Exosomes for Immunomodulatory Therapeutics and Skin Regeneration. Cells 9 (5), 1157. doi:10.3390/cells9051157
Hade, M. D., Suire, C. N., and Suo, Z. (2021). Mesenchymal Stem Cell-Derived Exosomes: Applications in Regenerative Medicine. Cells 10 (8), 1959. doi:10.3390/cells10081959
Han, I., Ropper, A. E., Konya, D., Kabatas, S., Toktas, Z., Aljuboori, Z., et al. (2015). Biological Approaches to Treating Intervertebral Disk Degeneration: Devising Stem Cell Therapies. Cel Transpl. 24 (11), 2197–2208. doi:10.3727/096368915X688650
Han, Q., Xie, Q. R., Li, F., Cheng, Y., Wu, T., Zhang, Y., et al. (2021). Targeted Inhibition of SIRT6 via Engineered Exosomes Impairs Tumorigenesis and Metastasis in Prostate Cancer. Theranostics 11 (13), 6526–6541. doi:10.7150/thno.53886
Hassanzadeh, A., Rahman, H. S., Markov, A., Endjun, J. J., Zekiy, A. O., Chartrand, M. S., et al. (2021). Mesenchymal Stem/stromal Cell-Derived Exosomes in Regenerative Medicine and Cancer; Overview of Development, Challenges, and Opportunities. Stem Cel Res Ther 12 (1), 297. doi:10.1186/s13287-021-02378-7
Hingert, D., Ekström, K., Aldridge, J., Crescitelli, R., and Brisby, H. (2020). Extracellular Vesicles from Human Mesenchymal Stem Cells Expedite Chondrogenesis in 3D Human Degenerative Disc Cell Cultures. Stem Cel Res Ther 11 (1), 323. doi:10.1186/s13287-020-01832-2
Hu, Z.-L., Li, H.-Y., Chang, X., Li, Y.-Y., Liu, C.-H., Gao, X.-X., et al. (2020). Exosomes Derived from Stem Cells as an Emerging Therapeutic Strategy for Intervertebral Disc Degeneration. Wjsc 12 (8), 803–813. doi:10.4252/wjsc.v12.i8.803
Huang, F., Gao, T., Wang, W., Wang, L., Xie, Y., Tai, C., et al. (2021). Engineered Basic Fibroblast Growth Factor-Overexpressing Human Umbilical Cord-Derived Mesenchymal Stem Cells Improve the Proliferation and Neuronal Differentiation of Endogenous Neural Stem Cells and Functional Recovery of Spinal Cord Injury by Activating the PI3K-Akt-GSK-3β Signaling Pathway. Stem Cel Res Ther 12 (1), 468. doi:10.1186/s13287-021-02537-w
Hulsmans, M., and Holvoet, P. (2013). MicroRNA-containing Microvesicles Regulating Inflammation in Association with Atherosclerotic Disease. Cardiovasc. Res. 100 (1), 7–18. doi:10.1093/cvr/cvt161
Ibrahim, A., and Marbán, E. (2016). Exosomes: Fundamental Biology and Roles in Cardiovascular Physiology. Annu. Rev. Physiol. 78, 67–83. doi:10.1146/annurev-physiol-021115-104929
Imai, Y., Okuma, M., An, H. S., Nakagawa, K., Yamada, M., Muehleman, C., et al. (2007). Restoration of Disc Height Loss by Recombinant Human Osteogenic Protein-1 Injection into Intervertebral Discs Undergoing Degeneration Induced by an Intradiscal Injection of Chondroitinase ABC. Spine 32 (11), 1197–1205. doi:10.1097/BRS.0b013e3180574d26
Jia, H., Ma, J., Lv, J., Ma, X., Xu, W., Yang, Y., et al. (2016). Oestrogen and Parathyroid Hormone Alleviate Lumbar Intervertebral Disc Degeneration in Ovariectomized Rats and Enhance Wnt/β-Catenin Pathway Activity. Sci. Rep. 6, 27521. doi:10.1038/srep27521
Jin, D., and Lee, H. (2015). A Computational Approach to Identifying Gene-microRNA Modules in Cancer. Plos Comput. Biol. 11 (1), e1004042. doi:10.1371/journal.pcbi.1004042
Johnson, Z., Schoepflin, Z. R., Schoepflin, Z., Choi, H., Shapiro, I., and Risbud, M. (2015). Disc in Flames: Roles of TNF-α and IL-1β in Intervertebral Disc Degeneration. eCM 30, 104–117. discussion 116-107. doi:10.22203/ecm.v030a08
Kabat, M., Bobkov, I., Kumar, S., and Grumet, M. (2020). Trends in Mesenchymal Stem Cell Clinical Trials 2004-2018: Is Efficacy Optimal in a Narrow Dose Range? Stem Cell Transl Med 9 (1), 17–27. doi:10.1002/sctm.19-0202
Kague, E., Turci, F., Newman, E., Yang, Y., Brown, K. R., Aglan, M. S., et al. (2021). 3D Assessment of Intervertebral Disc Degeneration in Zebrafish Identifies Changes in Bone Density that Prime Disc Disease. Bone Res. 9 (1), 39. doi:10.1038/s41413-021-00156-y
Kamali, A., Ziadlou, R., Lang, G., Pfannkuche, J., Cui, S., Li, Z., et al. (2021). Small Molecule-Based Treatment Approaches for Intervertebral Disc Degeneration: Current Options and Future Directions. Theranostics 11 (1), 27–47. doi:10.7150/thno.48987
Katz, J. N. (2006). Lumbar Disc Disorders and Low-Back Pain: Socioeconomic Factors and Consequences. J. Bone Jt. Surg Am 88 (Suppl. 2), 21–24. doi:10.2106/JBJS.E.01273
Kennon, J. C., Awad, M. E., Chutkan, N., DeVine, J., and Fulzele, S. (2018). Current Insights on Use of Growth Factors as Therapy for Intervertebral Disc Degeneration. Biomol. Concepts 9 (1), 43–52. doi:10.1515/bmc-2018-0003
Kepler, C. K., Ponnappan, R. K., Tannoury, C. A., Risbud, M. V., and Anderson, D. G. (2013). The Molecular Basis of Intervertebral Disc Degeneration. Spine J. 13 (3), 318–330. doi:10.1016/j.spinee.2012.12.003
Kong, X., Ding, X., Li, X., Gao, S., and Yang, Q. (2015). 53 BP 1 Suppresses Epithelial-Mesenchymal Transition by Downregulating ZEB 1 through Micro RNA ‐200b/429 in Breast Cancer. Cancer Sci. 106 (8), 982–989. doi:10.1111/cas.12699
Kuang, S., He, F., Liu, G., Sun, X., Dai, J., Chi, A., et al. (2021). CCR2-engineered Mesenchymal Stromal Cells Accelerate Diabetic Wound Healing by Restoring Immunological Homeostasis. Biomaterials 275, 120963. doi:10.1016/j.biomaterials.2021.120963
Le Maitre, C. L., Hoyland, J. A., and Freemont, A. J. (2007). Interleukin-1 Receptor Antagonist Delivered Directly and by Gene Therapy Inhibits Matrix Degradation in the Intact Degenerate Human Intervertebral Disc: an In Situ Zymographic and Gene Therapy Study. Arthritis Res. Ther. 9 (4), R83. doi:10.1186/ar2282
Li, M., Li, R., Yang, S., Yang, D., Gao, X., Sun, J., et al. (2020a). Exosomes Derived from Bone Marrow Mesenchymal Stem Cells Prevent Acidic pH-Induced Damage in Human Nucleus Pulposus Cells. Med. Sci. Monit. 26, e922928. doi:10.12659/MSM.922928
Li, M., Zhang, A., Li, J., Zhou, J., Zheng, Y., Zhang, C., et al. (2020b). Osteoblast/fibroblast Coculture Derived Bioactive ECM with Unique Matrisome Profile Facilitates Bone Regeneration. Bioactive Mater. 5 (4), 938–948. doi:10.1016/j.bioactmat.2020.06.017
Li, M., Zou, X., Xia, T., Wang, T., Liu, P., Zhou, X., et al. (2019). A Five‐miRNA Panel in Plasma Was Identified for Breast Cancer Diagnosis. Cancer Med. 8 (16), 7006–7017. doi:10.1002/cam4.2572
Li, N., Gao, J., Mi, L., Zhang, G., Zhang, L., Zhang, N., et al. (2020c). Synovial Membrane Mesenchymal Stem Cells: Past Life, Current Situation, and Application in Bone and Joint Diseases. Stem Cel Res Ther 11 (1), 381. doi:10.1186/s13287-020-01885-3
Li, P., Gan, Y., Xu, Y., Wang, L., Ouyang, B., Zhang, C., et al. (2017a). 17beta-estradiol Attenuates TNF-α-Induced Premature Senescence of Nucleus Pulposus Cells through Regulating the ROS/NF-κB Pathway. Int. J. Biol. Sci. 13 (2), 145–156. doi:10.7150/ijbs.16770
Li, P., Kaslan, M., Lee, S. H., Yao, J., and Gao, Z. (2017b). Progress in Exosome Isolation Techniques. Theranostics 7 (3), 789–804. doi:10.7150/thno.18133
Li, Z.-q., Kong, L., Liu, C., and Xu, H.-G. (2020d). Human Bone Marrow Mesenchymal Stem Cell-Derived Exosomes Attenuate IL-1β-induced Annulus Fibrosus Cell Damage. Am. J. Med. Sci. 360 (6), 693–700. doi:10.1016/j.amjms.2020.07.025
Liang, G., Zhu, Y., Ali, D. J., Tian, T., Xu, H., Si, K., et al. (2020). Engineered Exosomes for Targeted Co-delivery of miR-21 Inhibitor and Chemotherapeutics to Reverse Drug Resistance in colon Cancer. J. Nanobiotechnol 18 (1), 10. doi:10.1186/s12951-019-0563-2
Liang, H., Ma, S.-Y., Feng, G., Shen, F. H., and Joshua Li, X. (2010). Therapeutic Effects of Adenovirus-Mediated Growth and Differentiation Factor-5 in a Mice Disc Degeneration Model Induced by Annulus Needle Puncture. Spine J. 10 (1), 32–41. doi:10.1016/j.spinee.2009.10.006
Liao, Z., Luo, R., Li, G., Song, Y., Zhan, S., Zhao, K., et al. (2019a). Exosomes from Mesenchymal Stem Cells Modulate Endoplasmic Reticulum Stress to Protect against Nucleus Pulposus Cell Death and Ameliorate Intervertebral Disc Degeneration In Vivo. Theranostics 9 (14), 4084–4100. doi:10.7150/thno.33638
Liao, Z., Wu, X., Song, Y., Luo, R., Yin, H., Zhan, S., et al. (2019b). Angiopoietin‐like Protein 8 Expression and Association with Extracellular Matrix Metabolism and Inflammation during Intervertebral Disc Degeneration. J. Cel Mol Med 23 (8), 5737–5750. doi:10.1111/jcmm.14488
Liga, A., Vliegenthart, A. D. B., Oosthuyzen, W., Dear, J. W., and Kersaudy-Kerhoas, M. (2015). Exosome Isolation: a Microfluidic Road-Map. Lab. Chip 15 (11), 2388–2394. doi:10.1039/c5lc00240k
Lin, J., Chen, J., Zhang, Z., Xu, T., Shao, Z., Wang, X., et al. (2019). Luteoloside Inhibits IL-1β-Induced Apoptosis and Catabolism in Nucleus Pulposus Cells and Ameliorates Intervertebral Disk Degeneration. Front. Pharmacol. 10, 868. doi:10.3389/fphar.2019.00868
Lisieski, M. J., Eagle, A. L., Conti, A. C., Liberzon, I., and Perrine, S. A. (2018). Single-Prolonged Stress: A Review of Two Decades of Progress in a Rodent Model of Post-traumatic Stress Disorder. Front. Psychiatry 9, 196. doi:10.3389/fpsyt.2018.00196
Liu, W.-z., Ma, Z.-j., Li, J.-r., and Kang, X.-w. (2021). Mesenchymal Stem Cell-Derived Exosomes: Therapeutic Opportunities and Challenges for Spinal Cord Injury. Stem Cel Res Ther 12 (1), 102. doi:10.1186/s13287-021-02153-8
Liu, Y., Song, B., Wei, Y., Chen, F., Chi, Y., Fan, H., et al. (2018). Exosomes from Mesenchymal Stromal Cells Enhance Imatinib-Induced Apoptosis in Human Leukemia Cells via Activation of Caspase Signaling Pathway. Cytotherapy 20 (2), 181–188. doi:10.1016/j.jcyt.2017.11.006
Loibl, M., Wuertz‐Kozak, K., Vadala, G., Lang, S., Fairbank, J., and Urban, J. P. (2019). Controversies in Regenerative Medicine: Should Intervertebral Disc Degeneration Be Treated with Mesenchymal Stem Cells? JOR Spine 2 (1), e1043. doi:10.1002/jsp2.1043
Lu, K., Li, H.-y., Yang, K., Wu, J.-l., Cai, X.-w., Zhou, Y., et al. (2017). Exosomes as Potential Alternatives to Stem Cell Therapy for Intervertebral Disc Degeneration: In-Vitro Study on Exosomes in Interaction of Nucleus Pulposus Cells and Bone Marrow Mesenchymal Stem Cells. Stem Cel Res Ther 8 (1), 108. doi:10.1186/s13287-017-0563-9
Lu, L., Hu, J., Wu, Q., An, Y., Cui, W., Wang, J., et al. (2019). Berberine Prevents Human Nucleus Pulposus Cells from IL-1β-induced E-xtracellular M-atrix D-egradation and A-poptosis by I-nhibiting the NF-κB P-athway. Int. J. Mol. Med. 43 (4), 1679–1686. doi:10.3892/ijmm.2019.4105
Lui, P. P. Y. (2021). Mesenchymal Stem Cell-Derived Extracellular Vesicles for the Promotion of Tendon Repair - an Update of Literature. Stem Cel Rev Rep 17 (2), 379–389. doi:10.1007/s12015-020-10023-8
Luo, R., Song, Y., Liao, Z., Yin, H., Zhan, S., Wang, K., et al. (2019). Impaired Calcium Homeostasis via Advanced Glycation End Products Promotes Apoptosis through Endoplasmic Reticulum Stress in Human Nucleus Pulposus Cells and Exacerbates Intervertebral Disc Degeneration in Rats. FEBS J. 286 (21), 4356–4373. doi:10.1111/febs.14972
Ma, L., Pan, L., Liu, W., Liu, Y., Xiang, X., Pan, Y., et al. (2020). Agrin Influences Botulinum Neurotoxin A-Induced Nerve Sprouting via miR-144-Agrin-MuSK Signaling. Front. Cel Dev. Biol. 8, 15. doi:10.3389/fcell.2020.00015
Mackiewicz, Z., Salo, J., Konttinen, Y. T., Kaigle Holm, A., Indahl, A., Pajarinen, J., et al. (2009). Receptor Activator of Nuclear Factor Kappa B Ligand in an Experimental Intervertebral Disc Degeneration. Clin. Exp. Rheumatol. 27 (2), 299–306.
Malekpour, K., Hazrati, A., Zahar, M., Markov, A., Zekiy, A. O., Navashenaq, J. G., et al. (2021). The Potential Use of Mesenchymal Stem Cells and Their Derived Exosomes for Orthopedic Diseases Treatment. Stem Cel Rev Rep 24, 1–19. doi:10.1007/s12015-021-10185-z
Masuda, K. (2008). Biological Repair of the Degenerated Intervertebral Disc by the Injection of Growth Factors. Eur. Spine J. 17 (Suppl. 4), 441–451. doi:10.1007/s00586-008-0749-z
Melrose, J. (2016). Strategies in Regenerative Medicine for Intervertebral Disc Repair Using Mesenchymal Stem Cells and Bioscaffolds. Regenerative Med. 11 (7), 705–724. doi:10.2217/rme-2016-0069
Meng, X., Su, R.-J., Baylink, D. J., Neises, A., Kiroyan, J. B., Lee, W. Y.-W., et al. (2013). Rapid and Efficient Reprogramming of Human Fetal and Adult Blood CD34+ Cells into Mesenchymal Stem Cells with a Single Factor. Cell Res 23 (5), 658–672. doi:10.1038/cr.2013.40
Mocchi, M., Dotti, S., Del Bue, M., Villa, R., Bari, E., Perteghella, S., et al. (2020). Veterinary Regenerative Medicine for Musculoskeletal Disorders: Can Mesenchymal Stem/Stromal Cells and Their Secretome Be the New Frontier? Cells 9 (6), 1453. doi:10.3390/cells9061453
Mohd Noor, N. A., Abdullah Nurul, A., Ahmad Mohd Zain, M. R., Wan Nor Aduni, W. K., and Azlan, M. (2021). Extracellular Vesicles from Mesenchymal Stem Cells as Potential Treatments for Osteoarthritis. Cells 10 (6), 1287. doi:10.3390/cells10061287
Mondal, S. K., and Whiteside, T. L. (2021). Immunoaffinity-Based Isolation of Melanoma Cell-Derived and T Cell-Derived Exosomes from Plasma of Melanoma Patients. Methods Mol. Biol. 2265, 305–321. doi:10.1007/978-1-0716-1205-7_23
Nakamura, Y. (2019). Biomarkers for Immune Checkpoint Inhibitor-Mediated Tumor Response and Adverse Events. Front. Med. 6, 119. doi:10.3389/fmed.2019.00119
O'Shea, J. J., Holland, S. M., and Staudt, L. M. (2013). JAKs and STATs in Immunity, Immunodeficiency, and Cancer. N. Engl. J. Med. 368 (2), 161–170. doi:10.1056/NEJMra1202117
Obata, S., Akeda, K., Imanishi, T., Masuda, K., Bae, W., Morimoto, R., et al. (2012). Effect of Autologous Platelet-Rich Plasma-Releasate on Intervertebral Disc Degeneration in the Rabbit Anular Puncture Model: a Preclinical Study. Arthritis Res. Ther. 14 (6), R241. doi:10.1186/ar4084
Orozco, L., Soler, R., Morera, C., Alberca, M., Sánchez, A., and García-Sancho, J. (2011). Intervertebral Disc Repair by Autologous Mesenchymal Bone Marrow Cells: a Pilot Study. Transplantation 92 (7), 822–828. doi:10.1097/TP.0b013e3182298a15
Pan, Z., Sun, H., Xie, B., Xia, D., Zhang, X., Yu, D., et al. (2018). Therapeutic Effects of Gefitinib-Encapsulated Thermosensitive Injectable Hydrogel in Intervertebral Disc Degeneration. Biomaterials 160, 56–68. doi:10.1016/j.biomaterials.2018.01.016
Park, J.-H., Choi, K.-E., Kim, S.-G., Chu, H.-Y., Lee, S.-W., Kim, T.-J., et al. (2021). Long-Term Follow-Up of Inpatients with Failed Back Surgery Syndrome Who Received Integrative Korean Medicine Treatment: A Retrospective Analysis and Questionnaire Survey Study. Jcm 10 (8), 1703. doi:10.3390/jcm10081703
Paul, R., Haydon, R. C., Cheng, H., Ishikawa, A., Nenadovich, N., Jiang, W., et al. (2003). Potential Use of Sox9 Gene Therapy for Intervertebral Degenerative Disc Disease. Spine 28 (8), 755–763. doi:10.1097/01.brs.0000058946.64222.92
Pegtel, D. M., and Gould, S. J. (2019). Exosomes. Annu. Rev. Biochem. 88, 487–514. doi:10.1146/annurev-biochem-013118-111902
Perets, N., Betzer, O., Shapira, R., Brenstein, S., Angel, A., Sadan, T., et al. (2019). Golden Exosomes Selectively Target Brain Pathologies in Neurodegenerative and Neurodevelopmental Disorders. Nano Lett. 19 (6), 3422–3431. doi:10.1021/acs.nanolett.8b04148
Peroglio, M., Eglin, D., Benneker, L. M., Alini, M., and Grad, S. (2013). Thermoreversible Hyaluronan-Based Hydrogel Supports In Vitro and Ex Vivo Disc-like Differentiation of Human Mesenchymal Stem Cells. Spine J. 13 (11), 1627–1639. doi:10.1016/j.spinee.2013.05.029
Pettine, K. A., Suzuki, R. K., Sand, T. T., and Murphy, M. B. (2017). Autologous Bone Marrow Concentrate Intradiscal Injection for the Treatment of Degenerative Disc Disease with Three-Year Follow-Up. Int. Orthopaedics (Sicot) 41 (10), 2097–2103. doi:10.1007/s00264-017-3560-9
Pirvu, T. N., Schroeder, J. E., Peroglio, M., Verrier, S., Kaplan, L., Richards, R. G., et al. (2014). Platelet-rich Plasma Induces Annulus Fibrosus Cell Proliferation and Matrix Production. Eur. Spine J. 23 (4), 745–753. doi:10.1007/s00586-014-3198-x
Pittenger, M. F., Discher, D. E., Péault, B. M., Phinney, D. G., Hare, J. M., and Caplan, A. I. (2019). Mesenchymal Stem Cell Perspective: Cell Biology to Clinical Progress. NPJ Regen. Med. 4, 22. doi:10.1038/s41536-019-0083-6
Pourakbari, R., Khodadadi, M., Aghebati-Maleki, A., Aghebati-Maleki, L., and Yousefi, M. (2019). The Potential of Exosomes in the Therapy of the Cartilage and Bone Complications; Emphasis on Osteoarthritis. Life Sci. 236, 116861. doi:10.1016/j.lfs.2019.116861
Qi, L., Wang, R., Shi, Q., Yuan, M., Jin, M., and Li, D. (2019). Umbilical Cord Mesenchymal Stem Cell Conditioned Medium Restored the Expression of Collagen II and Aggrecan in Nucleus Pulposus Mesenchymal Stem Cells Exposed to High Glucose. J. Bone Miner Metab. 37 (3), 455–466. doi:10.1007/s00774-018-0953-9
Quang, H. V., Chang, C.-C., Song, P., Hauge, E.-M., and Kjems, J. (2018). Caveolae-mediated Mesenchymal Stem Cell Labelling by PSS-Coated PLGA PFOB Nano-Contrast Agent for MRI. Theranostics 8 (10), 2657–2671. doi:10.7150/thno.23206
Quentin, C., Bagheri, R., Ugbolue, U. C., Coudeyre, E., Pélissier, C., Descatha, A., et al. (2021). Effect of Home Exercise Training in Patients with Nonspecific Low-Back Pain: A Systematic Review and Meta-Analysis. Ijerph 18 (16), 8430. doi:10.3390/ijerph18168430
Raj, P. P. (2008). Intervertebral Disc: Anatomy-Physiology-Pathophysiology-Treatment. Pain Pract. 8 (1), 18–44. doi:10.1111/j.1533-2500.2007.00171.x
Raposo, G., Nijman, H. W., Stoorvogel, W., Liejendekker, R., Harding, C. V., Melief, C. J., et al. (1996). B Lymphocytes Secrete Antigen-Presenting Vesicles. J. Exp. Med. 183 (3), 1161–1172. doi:10.1084/jem.183.3.1161
Ratajczak, M. Z., and Ratajczak, J. (2020). Extracellular Microvesicles/exosomes: Discovery, Disbelief, Acceptance, and the Future? Leukemia 34 (12), 3126–3135. doi:10.1038/s41375-020-01041-z
Ren, S., Liu, Y., Ma, J., Liu, Y., Diao, Z., Yang, D., et al. (2013). Treatment of Rabbit Intervertebral Disc Degeneration with Co-transfection by Adeno-Associated Virus-Mediated SOX9 and Osteogenic Protein-1 Double Genes In Vivo. Int. J. Mol. Med. 32 (5), 1063–1068. doi:10.3892/ijmm.2013.1497
Richardson, S. M., Kalamegam, G., Pushparaj, P. N., Matta, C., Memic, A., Khademhosseini, A., et al. (2016). Mesenchymal Stem Cells in Regenerative Medicine: Focus on Articular Cartilage and Intervertebral Disc Regeneration. Methods 99, 69–80. doi:10.1016/j.ymeth.2015.09.015
Risbud, M. V., Albert, T. J., Guttapalli, A., Vresilovic, E. J., Hillibrand, A. S., Vaccaro, A. R., et al. (2004a). Differentiation of Mesenchymal Stem Cells towards a Nucleus Pulposus-like Phenotype In Vitro: Implications for Cell-Based Transplantation Therapy. Spine 29 (23), 2627–2632. doi:10.1097/01.brs.0000146462.92171.7f
Risbud, M. V., and Shapiro, I. M. (2014). Role of Cytokines in Intervertebral Disc Degeneration: Pain and Disc Content. Nat. Rev. Rheumatol. 10 (1), 44–56. doi:10.1038/nrrheum.2013.160
Risbud, M. V., Shapiro, I. M., Vaccaro, A. R., and Albert, T. J. (2004b). Stem Cell Regeneration of the Nucleus Pulposus. Spine J. 4 (6 Suppl. l), S348–S353. doi:10.1016/j.spinee.2004.07.031
Rustenburg, C. M. E., Emanuel, K. S., Peeters, M., Lems, W. F., Vergroesen, P.-P. A., and Smit, T. H. (2018). Osteoarthritis and Intervertebral Disc Degeneration: Quite Different, Quite Similar. JOR Spine 1 (4), e1033. doi:10.1002/jsp2.1033
Ryu, J.-S., Jeong, E.-J., Kim, J.-Y., Park, S. J., Ju, W. S., Kim, C.-H., et al. (2020). Application of Mesenchymal Stem Cells in Inflammatory and Fibrotic Diseases. Ijms 21 (21), 8366. doi:10.3390/ijms21218366
Saint-Pol, J., Gosselet, F., Duban-Deweer, S., Pottiez, G., and Karamanos, Y. (2020). Targeting and Crossing the Blood-Brain Barrier with Extracellular Vesicles. Cells 9 (4), 851. doi:10.3390/cells9040851
Sakai, D., and Andersson, G. B. J. (2015). Stem Cell Therapy for Intervertebral Disc Regeneration: Obstacles and Solutions. Nat. Rev. Rheumatol. 11 (4), 243–256. doi:10.1038/nrrheum.2015.13
Sakai, D., and Grad, S. (2015). Advancing the Cellular and Molecular Therapy for Intervertebral Disc Disease. Adv. Drug Deliv. Rev. 84, 159–171. doi:10.1016/j.addr.2014.06.009
Sakai, D., Mochida, J., Iwashina, T., Watanabe, T., Nakai, T., Ando, K., et al. (2005). Differentiation of Mesenchymal Stem Cells Transplanted to a Rabbit Degenerative Disc Model. Spine 30 (21), 2379–2387. doi:10.1097/01.brs.0000184365.28481.e3
Schiel, J. E., and Hage, D. S. (2009). Kinetic Studies of Biological Interactions by Affinity Chromatography. J. Sep. Sci. 32 (10), 1507–1522. doi:10.1002/jssc.200800685
Schorey, J. S., and Bhatnagar, S. (2008). Exosome Function: from Tumor Immunology to Pathogen Biology. Traffic 9 (6), 871–881. doi:10.1111/j.1600-0854.2008.00734.x
Shi, J., Zhao, Y.-C., Niu, Z.-F., Fan, H.-J., Hou, S.-K., Guo, X.-Q., et al. (2021). Mesenchymal Stem Cell-Derived Small Extracellular Vesicles in the Treatment of Human Diseases: Progress and prospect. Wjsc 13 (1), 49–63. doi:10.4252/wjsc.v13.i1.49
Sidhom, K., Obi, P. O., and Saleem, A. (2020). A Review of Exosomal Isolation Methods: Is Size Exclusion Chromatography the Best Option? Ijms 21 (18), 6466. doi:10.3390/ijms21186466
Sinkemani, A., Wang, F., Xie, Z., Chen, L., Zhang, C., and Wu, X. (2020). Nucleus Pulposus Cell Conditioned Medium Promotes Mesenchymal Stem Cell Differentiation into Nucleus Pulposus-like Cells under Hypoxic Conditions. Stem Cell Int. 2020, 1–24. doi:10.1155/2020/8882549
Sokolova, V., Ludwig, A.-K., Hornung, S., Rotan, O., Horn, P. A., Epple, M., et al. (2011). Characterisation of Exosomes Derived from Human Cells by Nanoparticle Tracking Analysis and Scanning Electron Microscopy. Colloids Surf. B: Biointerfaces 87 (1), 146–150. doi:10.1016/j.colsurfb.2011.05.013
Song, Y., Li, S., Geng, W., Luo, R., Liu, W., Tu, J., et al. (2018). Sirtuin 3-dependent Mitochondrial Redox Homeostasis Protects against AGEs-Induced Intervertebral Disc Degeneration. Redox Biol. 19, 339–353. doi:10.1016/j.redox.2018.09.006
Steck, E., Bertram, H., Abel, R., Chen, B., Winter, A., and Richter, W. (2005). Induction of Intervertebral Disc-like Cells from Adult Mesenchymal Stem Cells. Stem Cells 23 (3), 403–411. doi:10.1634/stemcells.2004-0107
Stoyanov, J., Gantenbein-Ritter, B., Gantenbein-Ritter, B., Bertolo, A., Aebli, N., Baur, M., et al. (2011). Role of Hypoxia and Growth and Differentiation Factor-5 on Differentiation of Human Mesenchymal Stem Cells towards Intervertebral Nucleus Pulposus-like Cells. eCM 21, 533–547. doi:10.22203/ecm.v021a40
Sun, S.-J., Wei, R., Li, F., Liao, S.-Y., and Tse, H.-F. (2021a). Mesenchymal Stromal Cell-Derived Exosomes in Cardiac Regeneration and Repair. Stem Cel Rep. 16 (7), 1662–1673. doi:10.1016/j.stemcr.2021.05.003
Sun, Y., Leung, V. Y., and Cheung, K. M. (2019). Clinical Trials of Intervertebral Disc Regeneration: Current Status and Future Developments. Int. Orthopaedics (Sicot) 43 (4), 1003–1010. doi:10.1007/s00264-018-4245-8
Sun, Y., Zhang, W., and Li, X. (2021b). Induced Pluripotent Stem Cell-Derived Mesenchymal Stem Cells Deliver Exogenous miR-105-5p via Small Extracellular Vesicles to Rejuvenate Senescent Nucleus Pulposus Cells and Attenuate Intervertebral Disc Degeneration. Stem Cel Res Ther 12 (1), 286. doi:10.1186/s13287-021-02362-1
Tauro, B. J., Greening, D. W., Mathias, R. A., Ji, H., Mathivanan, S., Scott, A. M., et al. (2012). Comparison of Ultracentrifugation, Density Gradient Separation, and Immunoaffinity Capture Methods for Isolating Human colon Cancer Cell Line LIM1863-Derived Exosomes. Methods 56 (2), 293–304. doi:10.1016/j.ymeth.2012.01.002
Théry, C., Amigorena, S., Raposo, G., and Clayton, A. (2006). Isolation and Characterization of Exosomes from Cell Culture Supernatants and Biological Fluids. Curr. Protoc. Cel Biol. 30, Unit 3 22. doi:10.1002/0471143030.cb0322s30
Travascio, F., Elmasry, S., and Asfour, S. (2014). Modeling the Role of IGF-1 on Extracellular Matrix Biosynthesis and Cellularity in Intervertebral Disc. J. Biomech. 47 (10), 2269–2276. doi:10.1016/j.jbiomech.2014.04.046
Vadalà, G., Russo, F., Ambrosio, L., Papalia, R., and Denaro, V. (2016). Mesenchymal Stem Cells for Intervertebral Disc Regeneration. J. Biol. Regul. Homeost Agents 30 (4 Suppl. 1), 173–179.
van den Akker, G. G. H., Surtel, D. A. M., Cremers, A., Richardson, S. M., Hoyland, J. A., van Rhijn, L. W., et al. (2016). Novel Immortal Cell Lines Support Cellular Heterogeneity in the Human Annulus Fibrosus. PLoS One 11 (1), e0144497. doi:10.1371/journal.pone.0144497
van Middelkoop, M., Rubinstein, S. M., Kuijpers, T., Verhagen, A. P., Ostelo, R., Koes, B. W., et al. (2011). A Systematic Review on the Effectiveness of Physical and Rehabilitation Interventions for Chronic Non-specific Low Back Pain. Eur. Spine J. 20 (1), 19–39. doi:10.1007/s00586-010-1518-3
Veyel, D., Sokolowska, E. M., Moreno, J. C., Kierszniowska, S., Cichon, J., Wojciechowska, I., et al. (2018). PROMIS, Global Analysis of PROtein-Metabolite Interactions Using Size Separation in Arabidopsis thaliana. J. Biol. Chem. 293 (32), 12440–12453. doi:10.1074/jbc.RA118.003351
Vlassov, A. V., Magdaleno, S., Setterquist, R., and Conrad, R. (2012). Exosomes: Current Knowledge of Their Composition, Biological Functions, and Diagnostic and Therapeutic Potentials. Biochim. Biophys. Acta (Bba) - Gen. Subjects 1820 (7), 940–948. doi:10.1016/j.bbagen.2012.03.017
Vo, N. V., Hartman, R. A., Yurube, T., Jacobs, L. J., Sowa, G. A., and Kang, J. D. (2013). Expression and Regulation of Metalloproteinases and Their Inhibitors in Intervertebral Disc Aging and Degeneration. Spine J. 13 (3), 331–341. doi:10.1016/j.spinee.2012.02.027
Wallach, C. J., Sobajima, S., Watanabe, Y., Kim, J. S., Georgescu, H. I., Robbins, P., et al. (2003). Gene Transfer of the Catabolic Inhibitor TIMP-1 Increases Measured Proteoglycans in Cells from Degenerated Human Intervertebral Discs. Spine 28 (20), 2331–2337. doi:10.1097/01.BRS.0000085303.67942.94
Wang, B., Tan, Z., and Guan, F. (2019a). Tumor-Derived Exosomes Mediate the Instability of Cadherins and Promote Tumor Progression. Ijms 20 (15), 3652. doi:10.3390/ijms20153652
Wang, S.-z., Chang, Q., Lu, J., and Wang, C. (2015). Growth Factors and Platelet-Rich Plasma: Promising Biological Strategies for Early Intervertebral Disc Degeneration. Int. Orthopaedics (Sicot) 39 (5), 927–934. doi:10.1007/s00264-014-2664-8
Wang, S.-Z., Rui, Y.-F., Tan, Q., and Wang, C. (2013). Enhancing Intervertebral Disc Repair and Regeneration through Biology: Platelet-Rich Plasma as an Alternative Strategy. Arthritis Res. Ther. 15 (5), 220. doi:10.1186/ar4353
Wang, X., He, L., Huang, X., Zhang, S., Cao, W., Che, F., et al. (2021). Recent Progress of Exosomes in Multiple Myeloma: Pathogenesis, Diagnosis, Prognosis and Therapeutic Strategies. Cancers 13 (7), 1635. doi:10.3390/cancers13071635
Wang, X., Liang, T., Qiu, J., Qiu, X., Gao, B., Gao, W., et al. (2019b). Melatonin Reverses the Loss of Stemness Induced by TNF-α in Human Bone Marrow Mesenchymal Stem Cells through Upregulation of YAP Expression. Stem Cell Int. 2019, 1–16. doi:10.1155/2019/6568394
Wei, H., Qian, X., Xie, F., and Cui, D. (2021). Isolation of Exosomes from Serum of Patients with Lung Cancer: a Comparison of the Ultra-high Speed Centrifugation and Precipitation Methods. Ann. Transl Med. 9 (10), 882. doi:10.21037/atm-21-2075
Wen, T., Wang, H., Li, Y., Lin, Y., Zhao, S., Liu, J., et al. (2021). Bone Mesenchymal Stem Cell-Derived Extracellular Vesicles Promote the Repair of Intervertebral Disc Degeneration by Transferring microRNA-199a. Cell Cycle 20 (3), 256–270. doi:10.1080/15384101.2020.1863682
Wu, A., Dong, W., Liu, S., Cheung, J. P. Y., Kwan, K. Y. H., Zeng, X., et al. (2019). The Prevalence and Years Lived with Disability Caused by Low Back Pain in China, 1990 to 2016: Findings from the Global burden of Disease Study 2016. Pain 160 (1), 237–245. doi:10.1097/j.pain.0000000000001396
Wu, H., Wang, Q., Zhong, H., Li, L., Zhang, Q., Huang, Q., et al. (2020). Differentially Expressed microRNAs in Exosomes of Patients with Breast Cancer Revealed by Next-generation S-equencing. Oncol. Rep. 43 (1), 240–250. doi:10.3892/or.2019.7401
Xia, C., Zeng, Z., Fang, B., Tao, M., Gu, C., Zheng, L., et al. (2019). Mesenchymal Stem Cell-Derived Exosomes Ameliorate Intervertebral Disc Degeneration via Anti-oxidant and Anti-inflammatory Effects. Free Radic. Biol. Med. 143, 1–15. doi:10.1016/j.freeradbiomed.2019.07.026
Xie, L., Chen, Z., Liu, M., Huang, W., Zou, F., Ma, X., et al. (2020). MSC-derived Exosomes Protect Vertebral Endplate Chondrocytes against Apoptosis and Calcification via the miR-31-5p/ATF6 Axis. Mol. Ther. - Nucleic Acids 22, 601–614. doi:10.1016/j.omtn.2020.09.026
Xu, Y., Shen, L., Li, F., Yang, J., Wan, X., and Ouyang, M. (2019). microRNA‐16‐5p‐containing Exosomes Derived from Bone Marrow‐derived Mesenchymal Stem Cells Inhibit Proliferation, Migration, and Invasion, while Promoting Apoptosis of Colorectal Cancer Cells by Downregulating ITGA2. J. Cel Physiol 234 (11), 21380–21394. doi:10.1002/jcp.28747
Xunian, Z., and Kalluri, R. (2020). Biology and Therapeutic Potential of Mesenchymal Stem Cell‐derived Exosomes. Cancer Sci. 111 (9), 3100–3110. doi:10.1111/cas.14563
Yaghoubi, Y., Movassaghpour, A., Zamani, M., Talebi, M., Mehdizadeh, A., and Yousefi, M. (2019). Human Umbilical Cord Mesenchymal Stem Cells Derived-Exosomes in Diseases Treatment. Life Sci. 233, 116733. doi:10.1016/j.lfs.2019.116733
Yang, H., Cao, C., Wu, C., Yuan, C., Gu, Q., Shi, Q., et al. (2015). TGF-βl Suppresses Inflammation in Cell Therapy for Intervertebral Disc Degeneration. Sci. Rep. 5, 13254. doi:10.1038/srep13254
Yang, X.-X., Sun, C., Wang, L., and Guo, X.-L. (2019). New Insight into Isolation, Identification Techniques and Medical Applications of Exosomes. J. Controlled Release 308, 119–129. doi:10.1016/j.jconrel.2019.07.021
Yao, W., Mai, X., Luo, C., Ai, F., and Chen, Q. (2011). A Cross-Sectional Survey of Nonspecific Low Back Pain Among 2083 Schoolchildren in China. Spine 36 (22), 1885–1890. doi:10.1097/BRS.0b013e3181faadea
Yu, B., Zhang, X., and Li, X. (2014). Exosomes Derived from Mesenchymal Stem Cells. Ijms 15 (3), 4142–4157. doi:10.3390/ijms15034142
Yu, L.-L., Zhu, J., Liu, J.-X., Jiang, F., Ni, W.-K., Qu, L.-S., et al. (2018). A Comparison of Traditional and Novel Methods for the Separation of Exosomes from Human Samples. Biomed. Res. Int. 2018, 1–9. doi:10.1155/2018/3634563
Yuan, Q., Wang, X., Liu, L., Cai, Y., Zhao, X., Ma, H., et al. (2020). Exosomes Derived from Human Placental Mesenchymal Stromal Cells Carrying AntagomiR-4450 Alleviate Intervertebral Disc Degeneration through Upregulation of ZNF121. Stem Cell Dev. 29 (16), 1038–1058. doi:10.1089/scd.2020.0083
Zeng, X., Lin, J., Wu, H., Yu, J., Tu, M., Cheang, L. H., et al. (2020). Effect of Conditioned Medium from Human Umbilical Cord-Derived Mesenchymal Stromal Cells on Rejuvenation of Nucleus Pulposus Derived Stem/Progenitor Cells from Degenerated Intervertebral Disc. Ijsc 13 (2), 257–267. doi:10.15283/ijsc20027
Zhang, C., Gan, X., Liang, R., and Jian, J. (2020a). Exosomes Derived from Epigallocatechin Gallate-Treated Cardiomyocytes Attenuated Acute Myocardial Infarction by Modulating MicroRNA-30a. Front. Pharmacol. 11, 126. doi:10.3389/fphar.2020.00126
Zhang, C., Gullbrand, S. E., Schaer, T. P., Boorman, S., Elliott, D. M., Chen, W., et al. (2021). Combined Hydrogel and Mesenchymal Stem Cell Therapy for Moderate-Severity Disc Degeneration in Goats. Tissue Eng. A 27 (1-2), 117–128. doi:10.1089/ten.TEA.2020.0103
Zhang, J., Zhang, J., Zhang, Y., Liu, W., Ni, W., Huang, X., et al. (2020b). Mesenchymal Stem Cells‐derived Exosomes Ameliorate Intervertebral Disc Degeneration through Inhibiting Pyroptosis. J. Cel. Mol. Med. 24 (20), 11742–11754. doi:10.1111/jcmm.15784
Zhang, X., Wang, S., Ding, X., Guo, J., and Tian, Z. (2020c). Potential Methods for Improving the Efficacy of Mesenchymal Stem Cells in the Treatment of Inflammatory Bowel Diseases. Scand. J. Immunol. 92 (3), e12897. doi:10.1111/sji.12897
Zhang, Y., Markova, D., Im, H.-J., Hu, W., Thonar, E. J.-M. A., He, T.-C., et al. (2009). Primary Bovine Intervertebral Disc Cells Transduced with Adenovirus Overexpressing 12 BMPs and Sox9 Maintain Appropriate Phenotype. Am. J. Phys. Med. Rehabil. 88 (6), 455–463. doi:10.1097/PHM.0b013e3181a5f0aa
Zhang, Z., Lin, J., Nisar, M., Chen, T., Xu, T., Zheng, G., et al. (2019). The Sirt1/P53 Axis in Diabetic Intervertebral Disc Degeneration Pathogenesis and Therapeutics. Oxidative Med. Cell Longevity 2019, 1–21. doi:10.1155/2019/7959573
Zhang, Z., Wang, C., Lin, J., Jin, H., Wang, K., Yan, Y., et al. (2018). Therapeutic Potential of Naringin for Intervertebral Disc Degeneration: Involvement of Autophagy against Oxidative Stress-Induced Apoptosis in Nucleus Pulposus Cells. Am. J. Chin. Med. 46, 1561–1580. doi:10.1142/S0192415X18500805
Zhu, G., Yang, X., Peng, C., Yu, L., and Hao, Y. (2020a). Exosomal miR-532-5p from Bone Marrow Mesenchymal Stem Cells Reduce Intervertebral Disc Degeneration by Targeting RASSF5. Exp. Cel Res. 393 (2), 112109. doi:10.1016/j.yexcr.2020.112109
Zhu, L., Shi, Y., Liu, L., Wang, H., Shen, P., and Yang, H. (2020b). Mesenchymal Stem Cells-Derived Exosomes Ameliorate Nucleus Pulposus Cells Apoptosis via Delivering miR-142-3p: Therapeutic Potential for Intervertebral Disc Degenerative Diseases. Cell Cycle 19 (14), 1727–1739. doi:10.1080/15384101.2020.1769301
Zhu, M., Hua, T., Ouyang, T., Qian, H., and Yu, B. (2021). Applications of Mesenchymal Stem Cells in Liver Fibrosis: Novel Strategies, Mechanisms, and Clinical Practice. Stem Cell Int. 2021, 1–17. doi:10.1155/2021/6546780
Keywords: exosomes, extracellular vesicles (EVs), mesenchymal stem cell (MSC), IVD degeneration (IVDD), microRNA
Citation: Lu L, Xu A, Gao F, Tian C, Wang H, Zhang J, Xie Y, Liu P, Liu S, Yang C, Ye Z and Wu X (2022) Mesenchymal Stem Cell-Derived Exosomes as a Novel Strategy for the Treatment of Intervertebral Disc Degeneration. Front. Cell Dev. Biol. 9:770510. doi: 10.3389/fcell.2021.770510
Received: 03 September 2021; Accepted: 31 December 2021;
Published: 24 January 2022.
Edited by:
Bo Gao, Air Force Military Medical University, ChinaReviewed by:
Xu Sun, Nanjing Drum Tower Hospital, ChinaJun Zou, Soochow University, China
Baoshan Xu, Tianjin Hospital, China
Copyright © 2022 Lu, Xu, Gao, Tian, Wang, Zhang, Xie, Liu, Liu, Yang, Ye and Wu. This is an open-access article distributed under the terms of the Creative Commons Attribution License (CC BY). The use, distribution or reproduction in other forums is permitted, provided the original author(s) and the copyright owner(s) are credited and that the original publication in this journal is cited, in accordance with accepted academic practice. No use, distribution or reproduction is permitted which does not comply with these terms.
*Correspondence: Zhewei Ye, eWV6aGV3ZWlAaHVzdC5lZHUuY24=; Xinghuo Wu, d3V4aW5naHVvQDE2My5jb20=
†These authors have contributed equally to this work