- 1School of Biosciences, University of Sheffield, Sheffield, United Kingdom
- 2The Centre for Environment, Fisheries and Aquaculture Science, Weymouth, United Kingdom
- 3Behavioural Biology, Centre for Behaviour and Neuroscience, University of Groningen, Groningen, Netherlands
Costs of reproduction shape the life-history evolution of investment in current and future reproduction and thereby aging. Androgens have been proposed to regulate the physiology governing these investments. Furthermore, androgens are hypothesized to play a central role in carotenoid-dependent sexual signaling, regulating how much carotenoids are diverted to ornamentation and away from somatic maintenance, increasing oxidative stress, and accelerating aging. We investigated these relationships in male three-spined stickleback in which we elevated 11-ketotestosterone and supplied vitamin E, an antioxidant, in a 2 × 2 design. Androgen elevation shortened the time stickleback maintained reproductive activities. We suspect that this effect is caused by 11-ketotestosterone stimulating investment in current reproduction, but we detected no evidence for this in our measurements of reproductive effort: nest building, body composition, and breeding coloration. Carotenoid-dependent coloration was even slightly decreased by 11-ketotestosterone elevation and was left unaffected by vitamin E. Red coloration correlated with life expectancy and reproductive capacity in a quadratic manner, suggesting overinvestment of the individuals exhibiting the reddest bellies. In contrast, blue iris color showed a negative relationship with survival, suggesting physiological costs of producing this aspect of nuptial coloration. In conclusion, our results support the hypothesis that androgens regulate investment in current versus future reproduction, yet the precise mechanisms remain elusive. The quadratic relationships between sexual signal expression and aspects of quality have wider consequences for how we view sexual selection on ornamentation and its relationship with aging.
Introduction
Organisms evolve to optimize allocation of resources between different physiological processes to maximize fitness. Such resource-based trade-offs are central to life-history theory (Stearns, 1989) and have been adopted throughout biology, including the biology of aging (Maklakov and Chapman, 2019). Questions concerning, for example, the optimal arrival time at breeding sites (Kokko, 1999), litter size (Dijkstra et al., 1990; Sikes et al., 1998), foraging effort (Abrams, 1991), dietary restriction (McCracken et al., 2020a), and prey choice (Rutten et al., 2006) can all be explained in a framework of fitness costs and benefits. A central or arguably ultimate cost in life history and the biology of aging is the cost of reproduction (Reznick et al., 2000; Lemaître et al., 2015). If there are no costs of producing offspring, why not simply produce more offspring to increase fitness?
The most direct test of these “costs of reproduction” is to increase reproductive effort experimentally and measure the long-term fitness consequences for the parents. In birds, this approach, by manipulating clutch or brood size, has been used many times. Yet, despite there being some undisputed demonstrations (Daan et al., 1990; Tinbergen and Daan, 1990) of costs of reproduction, a recent meta-analysis of 29 studies (Santos and Nakagawa, 2012) found that parental effort was associated with survival only in males, but not in females, with notably small overall effect sizes. Possibly, the fitness costs of parental effort are not traded off exclusively against future survival but also to future reproduction. Alternatively, the costs of an increased brood size are mainly paid by the offspring. This notion is supported by the finding that, in general, animals will not increase parental effort to such a degree that it fully compensates for the extra provisioning and care required by the offspring added, reducing offspring quality (Dijkstra et al., 1990; Simons et al., 2011). Indeed, the combined effects of brood enlargement studies on current reproduction, future reproduction, parental survival, and offspring fitness can reduce the total sum of fitness gained (Smith et al., 1989; Dijkstra et al., 1990; Tinbergen and Daan, 1990). Thus, whether the relationship between survival and investment in reproduction is causal and central in shaping the aging phenotype of animals remains less clear than current theory predicts.
In Drosophila, more suitable for artificial selection experiments than vertebrates, a genetic relationship between reproduction and survival has been demonstrated (Flatt, 2011). Surprisingly, however, there are multiple examples of long-lived mutants or experimental manipulations in Drosophila and Caenorhabditis elegans that extend lifespan without reduced or even increased fecundity (Flatt, 2011; McCracken et al., 2020b; Yamamoto et al., 2020; Lind et al., 2021). Note that these observations are made in the laboratory environment, and thus might not occur in the wild (Briga and Verhulst, 2015). Costs of reproduction therefore remain plausible but may be difficult to demonstrate or detect for different reasons. Physiological costs of reproduction may be context specific (Simons et al., 2014a), could be difficult to measure, or might be compensated or temporally dynamic.
Costs are also central to sexual signaling theory (Kotiaho, 2001; Számadó, 2011), and the cost of sexual traits for acquiring fertilizations should be viewed as part of the cost of reproduction (Höglund et al., 1998). According to the handicap hypothesis, mate choice for traits signaling male quality is only evolutionary stable when the trait bears costs, precluding cheating. Behavioral punishment (Számadó, 2011), energetic investment (Grafen, 1990; Candolin, 1999), mechanistic constraints (Emlen et al., 2012), and specific resource investment (Simons et al., 2014b) are mechanisms mediating the fitness cost of sexual signals. This diversity in the nature of costs may hamper detection of costs of sexual signals (Svensson and Wong, 2011), also because they may not be apparent in a laboratory setting where behavioral punishment is not possible, or because food is supplied ad libitum.
In general, all investment into reproduction, sexual signaling, and otherwise should be viewed as investment into current reproduction (Höglund et al., 1998), which is predicted to trade off with somatic maintenance and repair. It is this central trade-off that is persistently viewed as central in the biology of aging field, and forms the basis of the disposable soma theory of aging (Kirkwood, 2002). Investing in reproduction at the cost of somatic maintenance is usually optimal, because extrinsic mortality (mortality that cannot be fully intrinsically controlled) is almost never zero and investment into the soma is lost at death by an extrinsic cause (Williams, 1957). Any physiological investment that increases reproductive success is thus expected to reduce future reproduction either via accelerated mortality or via reproductive senescence.
Given this connection between any physiological cost paid to enhance current reproductive success at the expense of future reproductive success, we may expect physiological regulators balancing these investment decisions. Hormones, and in particular sex hormones, have gathered considerable attention in this regard (Hau, 2007; Regan et al., 2019; Bell, 2020; Garratt et al., 2020; Sugrue et al., 2021). In sexual signaling studies, testosterone specifically has attracted considerable attention for several reasons. First, the immunocompetence handicap hypothesis postulates that testosterone both suppresses the immune system and enhances expression of sexual traits and behavior (Folstad and Karter, 1992; Sheldon and Verhulst, 1996). Yet, evidence for direct immunosuppressive effects of testosterone is limited (Roberts et al., 2004), but see Newhouse and Vernasco (2020). In contrast, however, the reverse does hold, immune activation suppresses plasma testosterone, suggesting that testosterone plays a role in the trade-off between reproduction and somatic maintenance (Boonekamp et al., 2008). Second, testosterone has been shown to elevate carotenoid-based coloration (Blas et al., 2006; Kurtz et al., 2007; Perez-Rodriguez et al., 2008; Khalil et al., 2020), thereby possibly mediating the trade-off between current reproductive effort and oxidative stress (Schantz et al., 1999), induced by allocating carotenoids, an antioxidant [but see Koch et al. (2018) and Koch and Hill (2018)], away from maintenance towards sexual signaling (Peters, 2007; Svensson and Wong, 2011; Simons et al., 2012a). The link between oxidative stress and carotenoid signaling has gained further support by the increases in coloration observed when antioxidants are supplemented, such as vitamin E in stickleback (Gasterosteus aculeatus) (Pike et al., 2007a) and in gulls (Larus michahellis) (Pérez et al., 2008), but see Karu et al. (2008) and Giraudeau et al. (2013). A trade-off concerning oxidative stress can also link testosterone to immune suppression, given that higher levels of oxidative stress are hypothesized to negatively affect immunity (Kurtz et al., 2007; Peters, 2007; Costantini and Møller, 2009), but see Casagrande et al. (2012) and Newhouse and Vernasco (2020). Third, testosterone has also been suggested to increase metabolic rate but evidence is mixed (Buttemer et al., 2008; Holtmann et al., 2017), yet could possibly enhance food intake and thereby carotenoid intake or growth of bodily extremities used as ornaments (Mougeot et al., 2004).
These multiple relationships between testosterone, physiology, and sexual signaling hamper the interpretation of negative findings on the trade-off between current and future reproductive success as costs may come about in physiological aspects other than those under study. Moreover, which and how physiology is altered by elevated testosterone may vary over time or cost may come to expression only later in life. Long-term experiments of the physiological consequences of elevated testosterone are therefore required.
In several of such longer-term studies, testosterone has been shown to induce costs. Experimental elevation of testosterone in adult male brown-headed cowbirds (Molothrus ater) showed reduced return rates, and this has been explained by experiencing higher rates of aggression, because testosterone-implanted individuals also showed more signs of injury likely incurred during fighting (Dufty, 1989). Male testosterone-implanted mountain spiny lizards (Sceloporus jarrovi) also show reduced survival, but this effect is negated by food supplementation, suggesting an energetic cost (Marler and Moore, 1991). Experimental elevation in males of another lizard species (Psammodromus algirus) also reduced survival and increased ectoparasitic infestation (Salvador et al., 1996). In birds, survival of dark-eyed juncos (Junco hyemalis) (Reed et al., 2006), red grouse (Lagopus lagopus scoticus) (Moss et al., 1994; Mougeot et al., 2005; Redpath et al., 2006), and red-legged partridges (Alectoris rufa) (Alonso-Alvarez et al., 2020) was also lowered in individuals in which testosterone was experimentally elevated. Return rates of testosterone-implanted greater prairie-chicken cocks (Tympanunchus cupido) males were also lower although not significantly so (Augustine et al., 2011).
These studies suggest that testosterone elevation indeed has long-term survival costs. However, in several of these studies, elevations of testosterone were in the pharmacological range and/or were maintained after the breeding season in which the hormone is not elevated. So, to what extent elevated exposure to testosterone reduces survival or future reproduction under more natural conditions is not clear. In addition, effects on reproductive senescence and mechanistic links to carotenoid-dependent sexual signal expression were not directly investigated in these studies. Here, we test whether (11-keto)-testosterone (the most biologically active androgen in most teleost fish) modulates the trade-off between current and future reproduction in three-spined stickleback. Reproductive behaviors and sexual coloration are absent in castrated stickleback, but can readily be restored by 11-ketoandrostenedione, which is rapidly converted to 11-ketotestosterone (Borg and Mayer, 1995). Male sticklebacks produce nests from algae and plant material, glued together with “spiggin,” produced in their kidneys in response to 11-ketotestosterone (Jakobsson et al., 1996; Jakobsson et al., 1999). Using elaborate courtship, males attract gravid females to their nest to spawn and care for the offspring, and they repeat this nesting cycle multiple times in a single breeding season (Wootton and Robert, 1984). During their breeding season, stickleback exhibit a carotenoid-dependent trait, their reddish belly (Wedekind et al., 1998), which is subject to female choice (Künzler and Bakker, 2001; Pike et al., 2007b) and shows senescence (a decrease in functioning attributed to aging) within one breeding season (Kim et al., 2016). Males with redder bellies were previously found to have longer lifespans, and carotenoid supplementation extends lifespan and the time reproductive effort can be maintained (Pike et al., 2007b). Stickleback populations can either inhabit fresh water throughout the year or migrate to sea and back to breed in spring (anadromous populations).
The subjects of this study are wild-caught individuals on migration from sea to their breeding grounds. This population has been reported to be annual (van Mullem and van der Vlugt, 1964) (including more contemporary information from the Dutch Water Board). Size distribution data from caught stickleback in fishways, estuaries (including fish included in this study), and at the freshwater breeding grounds at the start of the breeding season showed a single peak in the distribution. Notably returning fish to sea also indicated a single peak of juvenile fish on their return migration (personal communication with and reports from the Dutch Water Board). Size density distributions are the most practical indication of the age distribution of populations of small fish. All indications from the limited data (from peer-reviewed sources and ecological reports) available on this population are therefore that the ecology of this population is an anadromous population that has an annual breeding lifecycle in freshwater. Hence, reproductive activities during a single breeding season likely determine lifetime reproductive effort. We hypothesize that 11-ketotestosterone elevation increases investment in current reproduction, e.g., nest building and sexual coloration, at the cost of maintaining the soma and thereby future reproduction.
Methods
Animals
Anadromous three-spined stickleback were caught using a lift net at the locks of Noordpolderzijl, the Netherlands (53°25′56″N, 6°34′ 59″E). Small leaks of fresh water through the locks attract stickleback into the estuary when they start migration toward fresh water early spring. Fish were transported to the laboratory (<25 km away) by car in aerated buckets filled with water from the estuary. In our aquarium facility, fresh water was added to adjust the fish to fresh water conditions across several days. Groups of fish were housed together in large glass aquaria (>60 × 30 × 30 cm, L × H × W).
Setup
At the start of the experiment, individual males (N = 237) were housed in individual plastic tanks (27.5 × 17.5 × 17 cm, L × H × W, Ferplast geolarge), covered with a see-through plastic lid, containing a plastic plant (in the front of the tank, Tetra Plantastics Ambulia Limnophila heterophylla, 11–15 cm) and a pressure air (provided via connected tubing by a Resun LP-100 air-pump)-operated filter (at the back of the tank, Europet Bernina). One side adjacent to another tank was blinded with white adhesive plastic that precluded any visual contact between the fish. The tanks were set in eight vertically connected steel cabinets each containing six rows of shelves. Treatments were distributed across the cabinets balanced evenly for row and column and fish started the experiment distributed across 5 days balanced for treatment to divide the time required for husbandry and measurements. The room was air-conditioned to keep water temperatures at 18°C. Lighting, LD 16:8, corresponding to the daylength at the height of summer in the Netherlands, started when males were put in individual tanks, and was provided by fluorescent tubes (OSRAM Cool White, L40W/640SA) placed on the ceiling in front of the cabinets.
Fish were fed every morning with defrosted red bloodworms, (Chironomus, 3F Frozen Fish Food) in portions of ±0.25 g using a plastic pipette. If after 15 min of the first portion an individual fish had finished all the provided food, it received another portion, to achieve near ad libitum feeding without detrimental effects of overfeeding on water quality. At the end of each day (at least 1 h after the first feeding round), excess food was removed from each individual tank using a plastic syringe. To stimulate sexual behavior, gravid females in a plastic see-through jar were shown to individual males each day for 5 min. Each week, all water of each individual tank was changed, excluding the water retained in the small filter compartment.
Males were provided with 400 threads of green polyester threads (0.840 g of ±6-cm-long threads) (Barber, 2001; Rushbrook et al., 2008) placed behind the artificial plant and a petri dish (placed in the back of the aquarium) filled with white aquarium sand as nesting material. Each week, the fish received new nesting material and had to rebuild their nest. Nests were examined each day, and if completed (judged by the presence of a tunnel in the nest) and if all material was used in the nest, a portion of extra green polyester threads (0.150 g) was added to the aquarium behind the artificial plant. This protocol allowed us to calculate a metric of nesting intensity as a composite of nest material used and speed of nest completion. This metric was expressed as the amount of days nest material was added minus the days it took to complete the nest.
The ability to build nests we used as a proxy of reproductive capacity given that without a nest a male stickleback cannot produce offspring, when sneaking of fertilizations that sometimes happen in specific populations is ignored. Our experimental protocol therefore did not assess paternal care at any point. We purposefully studied the consequences of androgens on the reproductive phase in which males display and attract females, as we were interested in trade-offs with carotenoid-dependent signaling. The photoperiod was changed to a short photoperiod (LD 8:16) after 160 days, when less than 10% of the individuals were showing nest building behavior. At that point, nest material provision and female stimulation ceased and individuals were subsequently only monitored for survival.
Injections and Circulating 11 kt Concentrations
After 1 week of acclimatization, individual males received two intraperitoneal injections (using 0.3-ml syringes, Becton Dickinson, Micro-Fine) of molten cocoa butter (14 μl/g fish body mass). The cocoa butter was injected at a temperature of 37°C (and solidified quickly in the fish kept in water of 18°C) and was loaded with 11-keto-androstenedione (in suspension, 4 mg/ml, based on a pilot study described below; Sigma Aldrich) and/or with vitamin E (dissolved, 226 mg/ml, α-tocopherol, Sigma Aldrich) or with nothing. With these combinations, we created a balanced 2 × 2 design of 11-keto-androstenedione and vitamin E. Our rationale for choosing vitamin E as a supplement was to study the suggested androgen-regulated relationship between carotenoid-dependent signaling and oxidative stress (Peters, 2007). To this end, we wanted to supplement an antioxidant, rather than pigmentary carotenoids with disputed antioxidant potential (Simons et al., 2012b), and vitamin E has previously been shown to increase the red belly of the stickleback (Pike et al., 2007a). In fish, 11-keto-androstenedione is rapidly converted to 11-ketotestosterone and similar methods have been used in three-spined stickleback previously to elevate 11-ketotestosterone concentrations (Páll et al., 2002; Kurtz et al., 2007), the main androgen in most male teleost fish (Borg et al., 1993; Borg, 1994; Borg and Mayer, 1995).
Prior to the main experiment, but in the same spring, we carried out a pilot experiment to determine the appropriate dose of 11-keto-androstenedione. We injected, as described above, concentrations of 1, 4, and 8 mg/ml 11-keto-androstenedione and subsequently obtained plasma 5 days later. 11-Ketotestosterone levels in plasma were determined by radioimmunoassay (RIA) and were found to be elevated strongly with the 4 mg/ml dose, without any apparent further increase at the highest dose [0 vs. 4, χ(1)2 = 7.4, p = 0.007; 4 vs. 8, χ(1)2 = 0.62, p = 0.43; Figure 1].
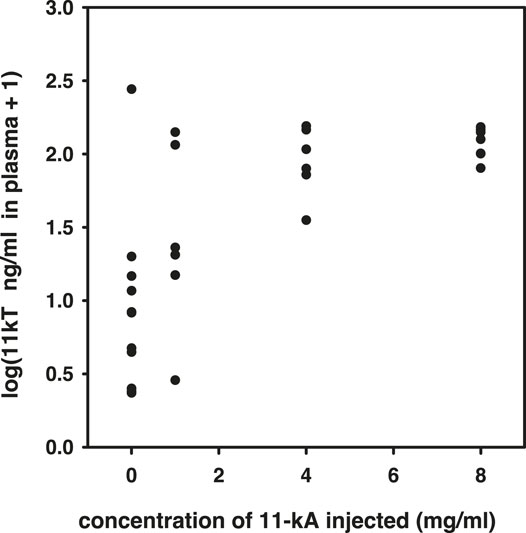
FIGURE 1. Results of the pilot experiment to determine resulting plasma concentrations of 11-ketotestosterone after treatment with different dosages of 11-keto-androstenedione.
At 2, 3, 4, and 5 weeks after the injections, random subsets of individuals of the main experiment were sacrificed for blood collection balanced for treatment. The fish were killed by a blow to the head, the tail was cut just posterior of the anus, and blood was collected from the caudal vein using heparinized glass capillaries. Blood was kept on ice until plasma was obtained via centrifugation (850 RCF for 7 min). Hematocrit of the individual samples was measured from the centrifuged capillaries with a digital caliper (Mitutoyo, to the nearest 10 μm). Plasma was stored in −80°C prior to analyses.
Individual fish were weighed prior to blood taking and, after blood taking, the mass of the testes, liver, kidney, and spleen were determined. 11-Keto testosterone levels were determined via radioimmunoassay (RIA) as previously described (Sebire et al., 2007). After thawing, the plasma samples were centrifuged at 13,000 rpm for 3 min at 4°C and then 5 μl was transferred into a 1.5-ml Eppendorf tube. Distilled water (100 μl) and ethyl acetate (1 ml) were added to the tube and vortexed for a few seconds. The samples were again centrifuged (13,000 rpm for 3 min at 4°C) and the bottom of the tube was subsequently placed briefly into liquid nitrogen. The organic phase was separated from the frozen aqueous phase into a glass tube. This extraction was repeated a second time. The ethyl acetate extracts were dried under a nitrogen stream at 45°C and redissolved in 500 μl of RIA buffer prior to analysis by RIA.
Within the actual experiment, 11-keto-androstenedione treatment increased 11-ketotestosterone levels but relatively mildly [F(1,64) = 5.97, p = 0.017; Figure 3]. Note that these samples were taken later in the season (relative to the time of injection) than in the pilot study (Figure 1), which may explain the lower elevation measured. However, we did not detect an effect of time at which plasma was collected in this dataset [F(1,61) = 0.18, p = 0.91] which suggests that the mild elevation in 11-ketotestosterone our treatment induced was maintained for at least 5 weeks, yet levels prior to 2 weeks were probably higher, judging from the elevation measured in the pilot study. Furthermore, we only detected a significant effect of our treatment if we took natural variation of testis size into account, which covaried positively with 11-ketotestosterone plasma levels [F(1,64) = 9.20, p = 0.004; Figure 2]. Compared to the population variance in 11-ketotestosterone, independent of variance attributable to testis size, our experimental treatment with 11-keto-androstenedione resulted in an elevation of 0.63 standard deviation of 11-ketotestosterone. So, the 11-ketotestosterone level of a given fish in the 11-keto-androstenedione-treated group was elevated beyond its own endogenous production as determined by its testis size. This rationale is in line with the lack of feedback of circulating 11-ketotestosterone concentrations on its production in this species, implicating that experimental elevation of the hormone does not impact on its endogenous production. For example, removal of one testis halves 11-ketotestosterone levels (Hellqvist et al., 2002). No effects of vitamin E treatment on 11-ketotestosterone levels were detected (p > 0.77). Variation in 11-ketotestosterone did not correlate with maximum or average blue or red breeding coloration prior to sacrificing (p > 0.64 and p > 0.10, respectively).
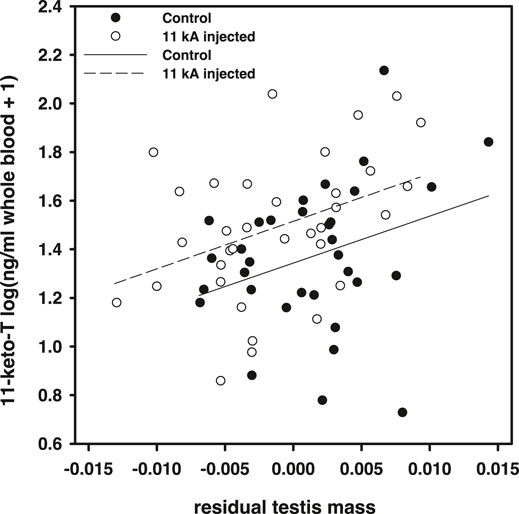
FIGURE 2. 11-ketotestosterone plasma concentrations in the main experiment regressed against residual testis mass (against body mass). Open dots and dashed regression line, 11-keto-androstenedione treated individuals; closed dots and solid line, controls.
Breeding Coloration
Weekly measurements of coloration were made using digital photography (Sony α-200) with fixed camera setting and in a controlled lighting environment. Fish were placed in a small glass container with a piece of foam at the back to restrain the fish to the front of the glass with its body side. The glass was fitted within a holder to allow it to be placed in a fully darkened box slightly tilted to avoid reflections in the glass. The camera was attached to this setup and a lightproof fabric was wrapped around the camera to avoid any outside light entering the box. A white-LED ring light (Sony HVL-RLAM) was placed on the lens of the camera and lit the box.
As digital cameras do not respond linearly to light, and hence are biased in measuring properties of light reflectance (e.g., color) (Stevens et al., 2007; Pike, 2011), we calibrated (Stigell et al., 2007) our camera with a large set of color patches (Munsell Glossy Edition, with known reflectance from the Joensuu Spectral Database) under the same lighting and fixed camera settings. Such an approach allows for an accurate representation of reflectance spectra from RGB values extracted from digital pictures (Stigell et al., 2007; Simons et al., 2012c; Simons et al., 2016). Fish were extracted from the pictures automatically using thresholding, cluster analysis, and alpha shapes in Matlab. The reddest and bluish part (consisting largely of blue iris coloration) of the individual fish were selected using image segmentation via thresholding of chroma from the simulated spectra per pixel (Figure 3). If all pixels fell below this threshold, this data point was excluded from the analysis, which happened in 8% of the cases within the analyses of blue coloration and in 0% of the cases where we analyzed red pigmentation. From these selected patches, we calculated an average simulated spectra to estimate the chroma of the blue (summed reflectance between 420 and 540 nm divided by total reflectance between 420 and 740 nm) and red (summed reflectance between 620 and 740 nm divided by total reflectance between 420 and 740 nm) breeding coloration for each individual fish. The red and blue coloration, with the latter relatively understudied, of three-spined stickleback determine sexual attractiveness and are associated with aspects of quality (Milinski and Bakker, 1990; Künzler and Bakker, 2001; Rush et al., 2003; Pike et al., 2007b; Flamarique et al., 2013).
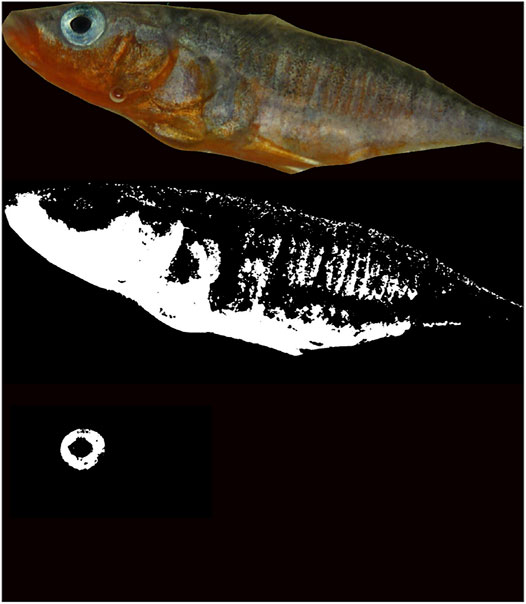
FIGURE 3. Example of the automatic selection and thresholding used to obtain red and blue chroma of the nuptial coloration of the stickleback. Top shows the individual stickleback extracted from the picture, the middle part shows the selected pixels (in white) above the red chroma threshold, and the bottom part shows the extraction of the blue iris, also using chroma thresholding.
Statistical Analyses
All individual measures on the fish were analyzed with general linear models or mixed models. If multiple measures from a fish were included, a random intercept for each fish was included in the model to correct for pseudo-replication. Note, the time variable (“week”) in the experiment was included as a categorical variable in the mixed models, as ignoring or misestimating random slopes in mixed models leads to a large inflation of type I error (Schielzeth and Forstmeier, 2009). Interpretation of patterns over time were based on least square means and their associated standard errors as provided in the figures. Model selection was based on backward selection of a model containing the interactions of the two treatment variables with the week of the experiment and mass at the start of the experiment. Breeding coloration was solely analyzed in fish that were still breeding (buildings nests), because breeding coloration fades quickly after the termination of reproductive activities. Reproductive senescence, as measured by the period that reproductive activities (i.e., nest building) were maintained, was analyzed using time to event Cox proportional hazard models (“coxph”) (Therneau and Grambsch, 2000). These models used right-hand censoring for the sacrificed animals, those that died an accidental death, or those that were still building nests when the experiment was terminated (21 weeks after the injections). All analyses were performed in SAS JMP 7.0 and R. Sample sizes differ between the analyses that span across the breeding season, due to a decline in the number of eligible fish (i.e., surviving and in breeding state). Hence, degrees of freedom of each analysis provide information on the underlying sample size. No violations of the assumption of a Gaussian distribution were detected in the dependent variables and residuals of the parametric models.
Results
Reproductive and Mortality Senescence
11-Keto-androstenedione treatment accelerated reproductive senescence. The period that individuals maintained their breeding activities (week of last complete nest minus week of first complete nest) was shorter after androgen treatment (logHR = 0.35 ± 0.16, p = 0.034, Figure 4), whereas the interaction with the main effect of vitamin E treatment was not significant (p > 0.22) and therefore removed from the model. This effect did not arise from 11-keto-androstenedione-treated animals starting with nest building sooner (rank test, W = 6,985.5, p = 0.45). A small part of this effect can be attributed to lower survival in the 11-keto-androstenedione treated fish. When analyzed across all data available for this study, there is a small decrease in survival that is far from significant (logHR = 0.05 ± 0.17, p = 0.75; Figure 5). Yet, when we analyzed data of the breeding season only, or up to the point of which we are sure 11-ketotestosterone is elevated (the last point at which animals were sacrificed for this purpose) the reduced survival in the 11-keto-androstenedione group these effects were stronger, but remained non-significant (logHR = 0.24 ± 0.25, p = 0.33, logHR = 0.53 ± 0.38, p = 0.16, respectively). No effects of vitamin E were detected in any of these models (p > 0.24).
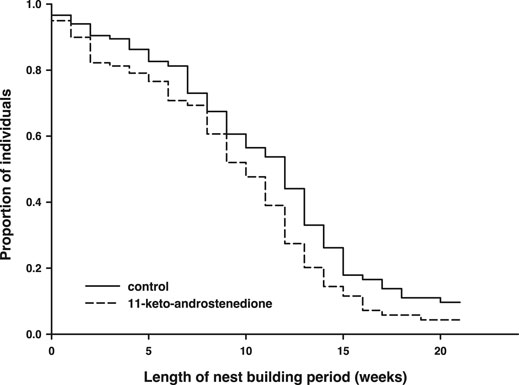
FIGURE 4. Period (in weeks) during which reproductive activities could be maintained was reduced in individuals in which 11-ketotestosterone was elevated (dashed line) compared to controls (closed line).
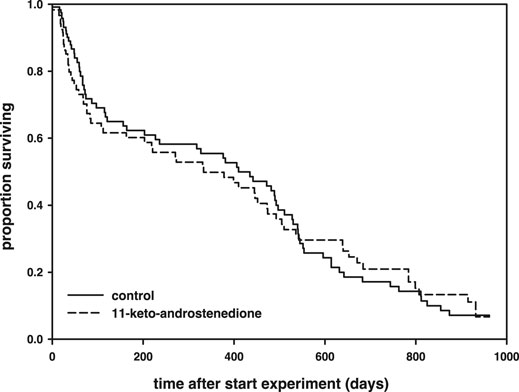
FIGURE 5. Survival (in days after the start of the experiment) plotted across the whole follow-up period, separated for 11-keto-androstenedione-treated individuals (dashed) and controls (solid). The breeding season from which data on coloration and nest building are presented lasted for 160 days of long photoperiod after the start of the experiment.
Body Composition
Individual mass at sacrificing covaried positively with all four organ masses (p < 0.0002) and was therefore included in the models testing for treatment effects. 11-Keto-androstenedione treatment and vitamin E treatment did not interact for any of the organ masses (p > 0.14) and the week at which the individual was killed did not contribute significantly either (p > 0.08). Therefore, we tested the effect of 11-keto-androstenedione and vitamin E treatment separately on the masses of the testes, liver, kidney, and spleen, and also on mass at sacrificing with mass at the start of treatment included as covariate (Table 1). No effects of 11-keto-androstenedione treatment were detected. Vitamin E treatment reduced mass-specific liver and spleen mass, and also mass change from mass at sacrificing (Table 1). Note that most of these effects are likely driven by mass loss compared to mass at injection (mass at injection was balanced but slightly higher in vitamin E treated fish, estimate: 0.013 ± 0.11, p = 0.23). When mass at sacrificing was removed from the models, all associations with vitamin E and organ masses were reduced to non-significant trends, p > 0.07.
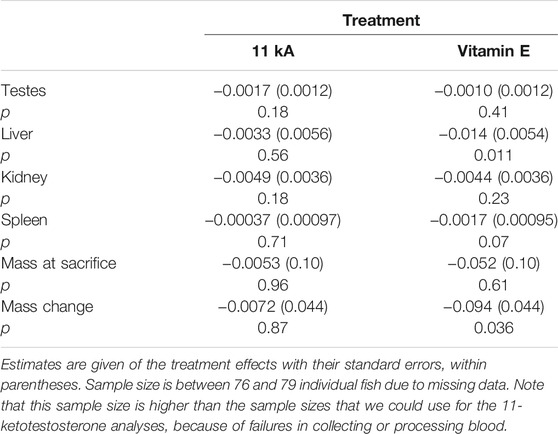
TABLE 1. Effects of treatment with 11-keto-androstenedione (11 kA) or vitamin E on organ mass (including mass at sacrificing as covariate) and mass at sacrificing and mass change (including mass at injection as covariate).
Coloration
Red coloration increased during the first part of the breeding season and declined at the end [week: F(21,1663) = 14.8, p < 0.0001]. This increase was lower in the androgen-treated fish, resulting in lower red coloration during the middle of the breeding season, but this effect did not reach statistical significance [11 kA × week: F(21,1663) = 1.46, p = 0.08; Figure 6]. In the models, we also included mass at the start of the experiment, which covaried positively with the intensity of the red breeding coloration [estimate: 0.010 ± 0.003, F(1,220.2) = 10.90, p = 0.001]. In these models, we did not detect any effects or interactions with vitamin E treatment (p > 0.70).
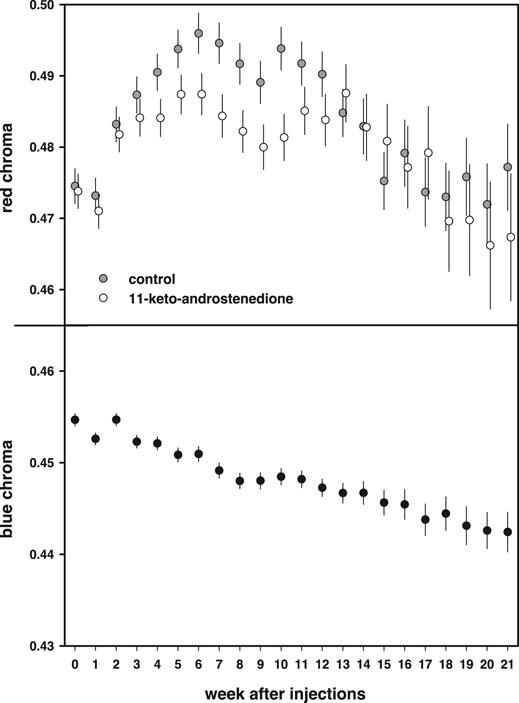
FIGURE 6. Breeding coloration plotted against the weeks after injections. Red chroma of the belly is plotted in the top panel and first increases during the breeding season to subsequently decline after an optimum. 11-Keto-androstenedione-treated animals have slightly less concentrated coloration of their bellies before and after the period of the optimum. The bottom panel shows blue iris coloration, which steadily declines during the breeding season irrespective of treatment.
Blue coloration decreased during the breeding season [F(21,1536) = 13.8, p < 0.0001; Figure 6] but no effects or interactions with either 11-keto-androstenedione or vitamin E treatment were detected (p > 0.72) and no relationship with mass was detected [estimate: 0.0017 ± 0.001, F(1,220.8) = 2.64, p = 0.11].
Nest Building Behavior
Nesting intensity, the amount of times extra material was added minus the time needed to complete a nest, increased at the start of the breeding season and gradually declined towards the end of the season [week: F(21,1609) = 6.18, p < 0.0001, Figure 7]. 11-Keto-androstenedione-treated individuals showed reduced nesting intensity at the beginning and end of the breeding season [week × 11 kA: F(21,1609) = 1.60, p = 0.042; Figure 7]. No interactions with vitamin E treatment were detected (p > 0.47) and mass was positively related to nesting intensity [estimate = 0.94 ± 0.25, F(1,203.8) = 13.6, p = 0.0003].
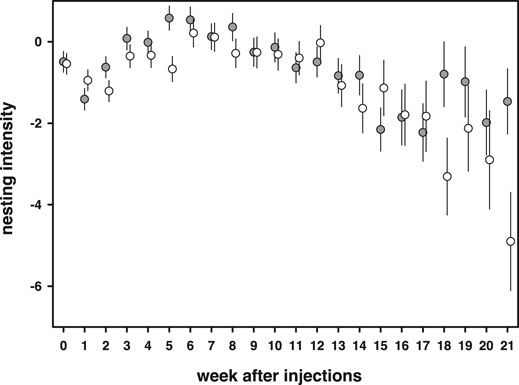
FIGURE 7. Nesting intensity [amount of days extra material was added minus the time needed to complete a nest (days)] first increased during the breeding season and then declined. 11-Keto-androstenedione (open dots)-treated individuals showed in general reduced nesting intensity during the start of the breeding season.
Relationships Between the Intensity of Breeding Coloration With Reproductive Senescence and Lifespan
Average and maximum chroma (which correlated strongly, r = 0.94, N = 155, p < 0.0001) achieved during the breeding season correlated positively with the time individuals could maintain their nesting activity (average: r = 0.17, p = 0.036; maximum r = 0.29, p = 0.0002, N = 155; Figure 8). For maximum blue coloration, we also detected a trend of a positive relationship (r = 0.15, p = 0.06, N = 155, but not for average r = −0.12, p = 0.12, N = 155). Note that from these analyses, we excluded individuals that were sacrificed for the 11-ketotestosterone analyses, because these animals are censored with respect to the averaged and maximum chroma they could have achieved. Because quadratic relationships with sexual signal expression and longevity have been reported previously, we also tested for quadratic effects and detected a quadratic effect in the relationship between maximum red chroma and breeding period [F(1,152) = 7.73, p = 0.006; Figure 8, quadratic relationships with max blue chroma were not significant p > 0.9]. Note that this quadratic relationship with breeding period (and also lifespan below) and maximum chroma cannot be attributable to regression to the mean. The maximum is predicted to increase with the number of sampling points, related to breeding period (and lifespan) in our setup, and in this respect, the linear slope of maximum chroma may be biased upward, rather than downward.
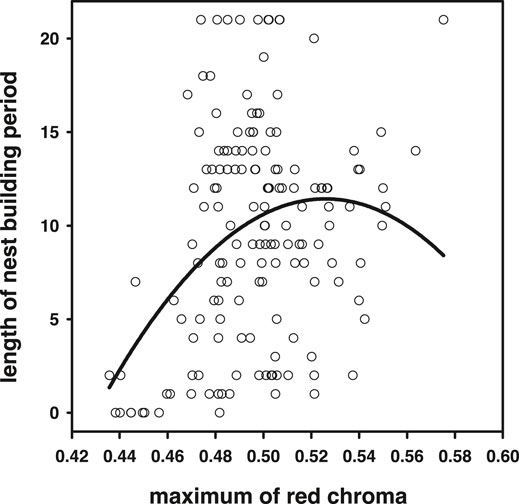
FIGURE 8. Higher maximum red chroma achieved during the breeding season signaled higher reproductive capacity. However, the significance of the quadratic term suggests that in the reddest individuals, this relationship levels off or may even become negative.
Lifespan was also positively related to average [logHR: −3.29 ± 4.06, χ(1)2 = 0.67, p = 0.42] and maximum red chroma [logHR: −9.20 ± 4.00, χ(1)2 = 5.50, p = 0.022]. For maximum red chroma, we also detected a quadratic relationship (logHR estimates: linear: −290 ± 90.7, p = 0.001; quadratic 279 ± 89.8, p < 0.002). Higher maximum blue chroma tended to be associated with lower survival (logHR: 16.64 ± 10.90, p = 0.13). When maximum blue chroma was added to the quadratic maximum red chroma model, both the linear term and the quadratic term of red chroma remained significant (p < 0.008), and maximum blue chroma was significantly positively related to mortality hazard (logHR: 27.7 ± 12.3, p = 0.024). Note that maximum red chroma and maximum blue chroma only loosely correlate (r = 0.35, p < 0.001) (Rush et al., 2003); multicollinearity biasing the model estimates is therefore unlikely.
Discussion
Current and Future Reproduction
11-Ketotestosterone elevation decreased our proxy for future reproductive success, the length that reproductive activities are maintained. This suggests that androgens are involved in the regulation of the trade-off between current and future reproduction. However, we do not find any evidence for positive effects on our proxies for current reproduction after 11-ketotestosterone elevation. On the contrary, if anything, red breeding coloration and nesting vigor are lowered in the 11-ketotestosterone elevated animals. Negative consequences of our treatment because of high pharmacological dosing are unlikely because our treatment resulted in only a mild increase in 11-ketotestosterone. Therefore, investment induced by 11-ketotestosterone in other aspects of physiology that we did not measure may have generated the costs that resulted in the reduction in future reproductive success we observed. These costs could be related to various aspects of reproduction such as sperm production, spiggin production, and sexual behavior. It is probably unlikely that these costs involve direct increased metabolic demand, because mass and body composition were left unaffected by 11-ketotestosterone elevation. Alternatively, there might be direct costs of higher levels of 11-ketotestosterone imposed by unknown physiological constraints, thereby reducing future reproduction.
Androgens, Vitamin E, and Carotenoid-Dependent Coloration
The hypothesized involvement of androgens in the trade-offs concerning carotenoid-dependent coloration (Alonso-Alvarez et al., 2007; Peters, 2007) is not supported by our findings. Elevation of 11-ketotestosterone did not increase red breeding coloration, but rather decreased it. In addition, variation in 11-ketotestosterone levels was not related to breeding coloration, in line with an earlier smaller study (Wright et al., 2016). Furthermore, another study on stickleback red breeding coloration and 11-ketotestosterone (Kurtz et al., 2007) found that if individuals had spent 4 (higher 11-ketotestosterone level) compared to 6 weeks (lower 11-ketotestosterone levels) in breeding conditions prior to measurement of circulating 11-ketotestosterone levels, a correlation with breeding coloration was not apparent. Our results and previous ones might thus suggest that variation in 11-ketotestosterone levels in full breeding condition does not determine investment in breeding coloration but may still regulate a suite of other behaviors or aspects of physiology related to current reproduction.
Our results also do not support the mechanistic explanation for the hypothesized trade-off between oxidative stress and carotenoid allocation to sexual coloration. Vitamin E treatment did not increase coloration. This is contrary to an earlier report in stickleback showing that breeding coloration increased under a diet of a combination of vitamin C and E (Pike et al., 2007a). Similarly, the reduction in time that 11-keto-androstenedione-treated fish could maintain their reproductive activities is unlikely to be attributable to oxidative stress costs, because we detected no interactions with the vitamin E treatment. These conclusions all assume that our methodology of injecting vitamin E resulted in elevated levels of vitamin E in our fish. No pellets were lost by fish and were observed in all fish that were sacrificed and died a natural death. We must assume that vitamin E was therefore available to the fish for uptake. However, we prioritized analyzing the plasma samples for 11-ketotestosterone analyses as they were too small to also measure vitamin E. Treatment with vitamin E led to slightly lower body and organ mass, which could indicate a loss rather than a gain in body condition. Our conclusions are therefore dependent on the only partially supported assumption that vitamin E injection led to increased bioavailable vitamin E.
Senescence of Nuptial Coloration and Associations With Survival
Variation in red breeding coloration was positively related to longevity and the time nesting activities could be maintained. This is in concordance with an earlier study on sticklebacks of a smaller sample size (N = 32) that investigated the relationship between redness and longevity (Pike et al., 2007b). Sexual ornament expression is in general found to be positively related to survival (Jennions et al., 2001), yet examples for carotenoid-dependent coloration are relatively scarce and dominated by studies on birds (Hill, 1991; Hõrak et al., 2001; Figuerola and Carlos Senar, 2007; Simons et al., 2012d; Simons et al., 2016; Cantarero et al., 2019). Interestingly, we detected a quadratic relationship of redness with survival and the length that breeding can be maintained, suggesting that, at a certain point, carotenoid-dependent breeding coloration does not signal quality but may be related to reduced survival and breeding capacity. It is possible that this is a common pattern for carotenoid-dependent signals or even sexual signals in general. Serins (Serinus serinus) with intermediate carotenoid-derived brightness have higher survival (Figuerola and Carlos Senar, 2007), and in the zebra finch (Taeniopygia guttata), we detected a similar quadratic relationship of their carotenoid-based bill coloration and longevity, when controlling for terminal declines (Simons et al., 2012d; Simons et al., 2016). Together, these findings indicate that the reddest mates potentially overinvest in their ornament to attract females. Choice for these males (Milinski and Bakker, 1990; Künzler and Bakker, 2001; Pike et al., 2007b) might still provide the direct benefits of producing sexy sons, or high signaling males might signal different aspects of quality, perhaps related more to current rather than future reproduction. For example, redness of the stickleback belly is related to functional fertility (Pike et al., 2010) and unexpectedly higher somatic and germline damage was detected in males with the most red signals (Kim and Velando, 2020).
Compared to the carotenoid-based red belly of the stickleback, their iridescent blue iris has been studied less, but has been suggested to be a decisive feature during female mate choice (Rush et al., 2003; Flamarique et al., 2013). Males may maintain a red belly for the purpose of enhancing blue iris contrast (Rush et al., 2003; Flamarique et al., 2013). Surprisingly we find that chroma of the blue iris reduces over the breeding season and that red breeding coloration is not at its maximum when the blue iris is. There is thus a mismatch between these two signals that does not result in the highest possible contrast, which would be perceived as most attractive to females. Moreover, the maximum intensity of the blue iris was negatively related to survival, which can suggest that there are costs to maintain iris color. The iridescent blue iris of the stickleback is formed by endogenously produced pigments (Frischknecht, 1993). The associated costs or detailed information on its physiology is currently lacking and warrant future study.
Conclusion
11-Ketotestosterone elevation reduced future reproductive success. Although we did not detect benefits to current reproduction, it is plausible that 11-ketotestosterone regulates aspects of the trade-off between current and future reproduction. It remains to be determined what these aspects are. We did not detect any evidence for the proposed regulatory role of androgens in the carotenoid trade-off between somatic maintenance and sexual signaling. We did find, however, that carotenoid-dependent coloration signals reproductive capacity and longevity. In the reddest individuals, however, this relationship is diminished and even turns negative. These quadratic relationships between sexual signal expression and aspects of quality (Candolin, 1999; Simons et al., 2016), not unique to the stickleback, have important consequences for how we view sexual selection on ornamentation in general.
Data Availability Statement
The raw data supporting the conclusion of this article will be made available by the authors, without undue reservation.
Ethics Statement
The animal study was reviewed and approved by the DEC University of Groningen.
Author Contributions
MJPS conducted the experiments, analyzed the data, and wrote the manuscript. MS conducted laboratory analyses. SV and TG supervised the project.
Funding
MJPS was funded by a Toptalent grant from the Netherlands Organization of Scientific Research (NWO) and is currently funded by a Sir Henry Dale Fellowship (Wellcome and Royal Society; 216405/Z/19/Z) and an Academy of Medical Sciences Springboard Award (the Wellcome Trust, the Government Department of Business, Energy and Industrial Strategy (BEIS), the British Heart Foundation, and Diabetes UK).
Conflict of Interest
The authors declare that the research was conducted in the absence of any commercial or financial relationships that could be construed as a potential conflict of interest.
Publisher’s Note
All claims expressed in this article are solely those of the authors and do not necessarily represent those of their affiliated organizations, or those of the publisher, the editors, and the reviewers. Any product that may be evaluated in this article, or claim that may be made by its manufacturer, is not guaranteed or endorsed by the publisher.
Acknowledgments
Rüdiger Schultz and Wytske van Dijk (Utrecht University) are thanked for carrying out the RIA analyses for the pilot experiment. Ditlev Simons is thanked for support in building the experimental setup.
References
Abrams, P. A. (1991). The Fitness Costs of Senescence: The Evolutionary Importance of Events in Early Adult Life. Evol. Ecol. 5, 343–360. doi:10.1007/bf02214152
Alonso-Alvarez, C., Bertrand, S., Faivre, B., Chastel, O., and Sorci, G. (2007). Testosterone and Oxidative Stress: The Oxidation Handicap Hypothesis. Proc. R. Soc. B. 274, 819–825. doi:10.1098/rspb.2006.3764
Alonso-Alvarez, C., Cantarero, A., Romero-Haro, A. Á., Chastel, O., and Pérez-Rodríguez, L. (2020). Life-Long Testosterone and Antiandrogen Treatments Affect the Survival and Reproduction of Captive Male Red-Legged Partridges (Alectoris rufa). Behav. Ecol. Sociobiol. 74, 98. doi:10.1007/s00265-020-02878-1
Augustine, Jacqueline, K., Millspaugh, Joshua, J., Sandercock, , and Brett, K. (2011). “Chapter Fourteen. Testosterone Mediates Mating Success in Greater Prairie-Chickens,” in Ecology, Conservation, and Management of Grouse: Published for the Cooper Ornithological Society. Editors K. S. Brett, Kathy Martin, and Gernot Segelbacher. (Berkeley: University of California Press), 195–208. doi:10.1525/9780520950573-016
Barber, I. (2001). Nests as Ornaments: Revealing Construction by Male Sticklebacks. Behav. Ecol. 12, 390–396. doi:10.1093/beheco/12.4.390
Bell, A. M. (2020). Individual Variation and the Challenge Hypothesis. Horm. Behav. 123, 104549. doi:10.1016/j.yhbeh.2019.06.013
Blas, J., Perez-Rodriguez, L., Bortolotti, G. R., Vinuela, J., and Marchant, T. A. (2006). Testosterone Increases Bioavailability of Carotenoids: Insights into the Honesty of Sexual Signaling. Proc. Natl. Acad. Sci. 103, 18633–18637. doi:10.1073/pnas.0609189103
Boonekamp, J. J., Ros, A. H. F., and Verhulst, S. (2008). Immune Activation Suppresses Plasma Testosterone Level: A Meta-Analysis. Biol. Lett. 4, 741–744. doi:10.1098/rsbl.2008.0347
Borg, B. (1994). Androgens in Teleost Fishes. Comp. Biochem. Physiol. C: Pharmacol. Toxicol. Endocrinol. 109, 219–245. doi:10.1016/0742-8413(94)00063-g
Borg, B., Antonopoulou, E., Andersson, E., Carlberg, T., and Mayer, I. (1993). Effectiveness of Several Androgens in Stimulating Kidney Hypertrophy, a Secondary Sexual Character, in Castrated Male Three-Spined Sticklebacks, Gasterosteus aculeatus. Can. J. Zool. 71, 2327–2329. doi:10.1139/z93-326
Borg, B., and Mayer, I. (1995). Androgens and Behaviour in the Three-Spined Stickleback. Behaviour 132, 1025–1035. doi:10.1163/156853995x00432
Briga, M., and Verhulst, S. (2015). What Can Long-Lived Mutants Tell Us about Mechanisms Causing Aging and Lifespan Variation in Natural Environments? Exp. Gerontol. 71, 21–26. doi:10.1016/j.exger.2015.09.002
Buttemer, W. A., Warne, S., Bech, C., and Astheimer, L. B. (2008). Testosterone Effects on Avian Basal Metabolic Rate and Aerobic Performance: Facts and Artefacts. Comp. Biochem. Physiol. A Mol. Integr. Physiol. 150, 204–210. doi:10.1016/j.cbpa.2006.06.047
Candolin, U. (1999). The Relationship between Signal Quality and Physical Condition: Is Sexual Signalling Honest in the Three-Spined Stickleback? Anim. Behav. 58, 1261–1267. doi:10.1006/anbe.1999.1259
Cantarero, A., Pérez-Rodríguez, L., Romero-Haro, A. Á., Chastel, O., and Alonso-Alvarez, C. (2019). Carotenoid-based Coloration Predicts Both Longevity and Lifetime Fecundity in Male Birds, but Testosterone Disrupts Signal Reliability. PLoS One 14, e0221436. doi:10.1371/journal.pone.0221436
Casagrande, S., Costantini, D., Dell’Omo, G., Tagliavini, J., and Groothuis, T. G. G. (2012). Differential Effects of Testosterone Metabolites Oestradiol and Dihydrotestosterone on Oxidative Stress and Carotenoid-Dependent Colour Expression in a Bird. Behav. Ecol. Sociobiol. 66, 1319–1331. doi:10.1007/s00265-012-1387-3
Costantini, D., and Møller, A. P. (2009). Does Immune Response Cause Oxidative Stress in Birds? A Meta-Analysis. Comp. Biochem. Physiol. A Mol. Integr. Physiol. 153, 339–344. doi:10.1016/j.cbpa.2009.03.010
Daan, S., Dijkstra, C., and Tinbergen, J. M. (1990). Family Planning in the Kestrel (Falco tinnunculus): The Ultimate Control of Covariation of Laying Date and Clutch Size. Behaviour 114, 83–116. doi:10.1163/156853990x00077
Dijkstra, C., Bult, A., Bijlsma, S., Daan, S., Meijer, T., and Zijlstra, M. (1990). Brood Size Manipulations in the Kestrel (Falco tinnunculus): Effects on Offspring and Parent Survival. J. Anim. Ecol. 59, 269. doi:10.2307/5172
Dufty, A. M. (1989). Testosterone and Survival: A Cost of Aggressiveness. Horm. Behav. 23, 185–193. doi:10.1016/0018-506x(89)90059-7
Emlen, D. J., Warren, I. A., Johns, A., Dworkin, I., and Lavine, L. C. (2012). A Mechanism of Extreme Growth and Reliable Signaling in Sexually Selected Ornaments and Weapons. Science 337, 860–864. doi:10.1126/science.1224286
Figuerola, J., and Carlos Senar, J. (2007). Serins with Intermediate Brightness Have a Higher Survival in the Wild. Oikos 116, 636–641. doi:10.1111/j.0030-1299.2007.14719.x
Flamarique, I. N., Bergstrom, C., Cheng, C. L., and Reimchen, T. E. (2013). Role of the Iridescent Eye in Stickleback Female Mate Choice. J. Exp. Biol. 216, 2806–2812. doi:10.1242/jeb.084889
Flatt, T. (2011). Survival Costs of Reproduction in Drosophila. Exp. Gerontol. 46, 369–375. doi:10.1016/j.exger.2010.10.008
Folstad, I., and Karter, A. J. (1992). Parasites, Bright Males, and the Immunocompetence Handicap. The Am. Naturalist 139, 603–622. doi:10.1086/285346
Frischknecht, M. (1993). The Breeding Colouration of Male Three-Spined Sticklebacks (Gasterosteus aculeatus) as an Indicator of Energy Investment in Vigour. Evol. Ecol. 7, 439–450. doi:10.1007/bf01237640
Garratt, M., Try, H., Smiley, K. O., Grattan, D. R., and Brooks, R. C. (2020). Mating in the Absence of Fertilization Promotes a Growth-Reproduction versus Lifespan Trade-Off in Female Mice. Proc. Natl. Acad. Sci. U.S.A. 117, 15748–15754. doi:10.1073/pnas.2003159117
Giraudeau, M., Sweazea, K., Butler, M. W., and McGraw, K. J. (2013). Effects of Carotenoid and Vitamin E Supplementation on Oxidative Stress and Plumage Coloration in House Finches (Haemorhous mexicanus). Comp. Biochem. Physiol. A Mol. Integr. Physiol. 166, 406–413. doi:10.1016/j.cbpa.2013.07.014
Grafen, A. (1990). Biological Signals as Handicaps. J. Theor. Biol. 144, 517–546. doi:10.1016/s0022-5193(05)80088-8
Hau, M. (2007). Regulation of Male Traits by Testosterone: Implications for the Evolution of Vertebrate Life Histories. BioEssays 29, 133–144. doi:10.1002/bies.20524
Hellqvist, A., Mayer, I., and Borg, B. (2002). Effects of Hemi-Castration on Plasma Steroid Levels in Two Teleost Fishes; the Three-Spined Stickleback, Gasterosteus aculeatus, and the Atlantic salmon, Salmo salar. Fish. Physiol. Biochem. 26, 107–110. doi:10.1023/A:1025435625551
Hill, G. E. (1991). Plumage Coloration Is a Sexually Selected Indicator of Male Quality. Nature 350, 337–339. doi:10.1038/350337a0
Höglund, J., Sheldon, B. C., and Hoglund, J. (1998). The Cost of Reproduction and Sexual Selection. Oikos 83, 478, doi:10.2307/3546675
Holtmann, B., Lagisz, M., and Nakagawa, S. (2017). Metabolic Rates, and Not Hormone Levels, are a Likely Mediator of Between‐individual Differences in Behaviour: A Meta‐Analysis. Funct. Ecol. 31, 685–696. doi:10.1111/1365-2435.12779
Hõrak, P., Ots, I., Vellau, H., Spottiswoode, C., and Pape Møller, A. (2001). Carotenoid-based Plumage Coloration Reflects Hemoparasite Infection and Local Survival in Breeding Great Tits. Oecologia 126, 166–173. doi:10.1007/s004420000513
Jakobsson, S., Borg, B., Haux, C., and Hyllner, S. J. (1999). An 11-ketotestosterone Induced Kidney-Secreted Protein: the Nest Building Glue from Male Three-Spined Stickleback, Gasterosteus aculeatus. Fish. Physiol. Biochem. 20, 79–85. doi:10.1023/a:1007776016610
Jakobsson, S., Mayer, I., Schulz, R. W., Blankenstein, M. A., and Borg, B. (1996). Specific Binding of 11-ketotestosterone in an Androgen Target Organ, the Kidney of the Male Three-Spined Stickleback, Gasterosteus aculeatus. Fish. Physiol. Biochem. 15, 459–467. doi:10.1007/bf01874920
Jennions, M. D., Moller, A. P., and Petrie, M. (2001). Sexually Selected Traits and Adult Survival: a Meta-Analysis. Q. Rev. Biol. 76, 3–36. doi:10.1086/393743
Karu, U., Saks, L., and Hõrak, P. (2008). Carotenoid-based Plumage Coloration Is Not Affected by Vitamin E Supplementation in Male Greenfinches. Ecol. Res. 23, 931–935. doi:10.1007/s11284-007-0457-x
Khalil, S., Welklin, J. F., McGraw, K. J., Boersma, J., Schwabl, H., Webster, M. S., et al. (2020). Testosterone Regulates CYP2J19-Linked Carotenoid Signal Expression in Male Red-Backed Fairywrens (Malurus melanocephalus). Proc. Biol. Sci. 287, 20201687. doi:10.1098/rspb.2020.1687
Kim, S. Y., Metcalfe, N. B., and Velando, A. (2016). A Benign Juvenile Environment Reduces the Strength of Antagonistic Pleiotropy and Genetic Variation in the Rate of Senescence. J. Anim. Ecol. 85, 705–714. doi:10.1111/1365-2656.12468
Kim, S. Y., and Velando, A. (2020). Attractive Male Sticklebacks Carry More Oxidative DNA Damage in the Soma and Germline. J. Evol. Biol. 33, 121–126. doi:10.1111/jeb.13552
Kirkwood, T. B. L. (2002). Evolution of Ageing. Mech. Ageing Dev. 123, 737–745. doi:10.1016/s0047-6374(01)00419-5
Koch, R. E., Kavazis, A. N., Hasselquist, D., Hood, W. R., Zhang, Y., Toomey, M. B., et al. (2018). No Evidence that Carotenoid Pigments Boost Either Immune or Antioxidant Defenses in a Songbird. Nat. Commun. 9, 491–497. doi:10.1038/s41467-018-02974-x
Koch, R. E., and Hill, G. E. (2018). Do Carotenoid‐Based Ornaments Entail Resource Trade‐offs? an Evaluation of Theory and Data. Funct. Ecol. 32, 1908–1920. doi:10.1111/1365-2435.13122
Kokko, H. (1999). Competition for Early Arrival in Migratory Birds. J. Anim. Ecol. 68, 940–950. doi:10.1046/j.1365-2656.1999.00343.x
Kotiaho, J. S. (2001). Costs of Sexual Traits: A Mismatch Between Theoretical Considerations and Empirical Evidence. Biol. Rev. 76, 365–376. doi:10.1017/s1464793101005711
Künzler, R., and Bakker, T. C. M. (2001). Female Preferences for Single and Combined Traits in Computer Animated Stickleback Males. Behav. Ecol. 12, 681–685. doi:10.1093/beheco/12.6.681
Kurtz, J., Kalbe, M., Langefors, Å, Mayer, I., Milinski, M., and Hasselquist, D. (2007). An Experimental Test of the Immunocompetence Handicap Hypothesis in a Teleost Fish: 11‐Ketotestosterone Suppresses Innate Immunity in Three‐Spined Sticklebacks. Am. Nat. 170, 509–519. doi:10.1086/521316
Lemaître, J. F., Berger, V., Bonenfant, C., Douhard, M., Gamelon, M., Plard, F., et al. (2015). Early-late Life Trade-Offs and the Evolution of Ageing in the Wild. Proc. R. Soc. B Biol. Sci. 282, 20150209. doi:10.1098/rspb.2015.0209
Lind, M. I., Carlsson, H., Duxbury, E. M. L., Ivimey-Cook, E., and Maklakov, A. A. (2021). Cost-free Lifespan Extension via Optimization of Gene Expression in Adulthood Aligns with the Developmental Theory of Ageing. Proc. R. Soc. B Biol. Sci. 288, 20201728. doi:10.1098/rspb.2020.1728
Maklakov, A. A., and Chapman, T. (2019). Evolution of Ageing as a Tangle of Trade-Offs: Energy versus Function. Proc. Biol. Sci. 286, 20191604. doi:10.1098/rspb.2019.1604
Marler, C. A., and Moore, M. C. (1991). Supplementary Feeding Compensates for Testosterone-Induced Costs of Aggression in Male Mountain Spiny Lizards, Sceloporus Jarrovi. Anim. Behav. 42, 209–219. doi:10.1016/s0003-3472(05)80552-4
McCracken, A. W., Adams, G., Hartshorne, L., Tatar, M., and Simons, M. J. P. (2020). The Hidden Costs of Dietary Restriction: Implications for its Evolutionary and Mechanistic Origins. Sci. Adv. 6, eaay3047. doi:10.1126/sciadv.aay3047
McCracken, A. W., Buckle, E., and Simons, M. J. P. (2020). The Relationship between Longevity and Diet is Genotype Dependent and Sensitive to Desiccation in Drosophila melanogaster. J. Exp. Biol. 223, jeb230185. doi:10.1242/jeb.230185
Milinski, M., and Bakker, T. C. M. (1990). Female Sticklebacks Use Male Coloration in Mate Choice and Hence Avoid Parasitized Males. Nature 344, 330–333. doi:10.1038/344330a0
Moss, R., Parr, R., and Lambin, X. (1994). Effects of Testosterone on Breeding Density, Breeding success and Survival of Red Grouse. Proc. R. Soc. Lond. B 258, 175–180. doi:10.1098/rspb.1994.0159
Mougeot, F., Irvine, J. R., Seivwright, L., Redpath, S. M., and Piertney, S. (2004). Testosterone, Immunocompetence, and Honest Sexual Signaling in Male Red Grouse. Behav. Ecol. 15, 930–937. doi:10.1093/beheco/arh087
Mougeot, F., Piertney, S. B., Leckie, F., Evans, S., Moss, R., Redpath, S. M., et al. (2005). Experimentally Increased Aggressiveness Reduces Population Kin Structure and Subsequent Recruitment in Red Grouse Lagopus Lagopus Scoticus. J. Anim. Ecol. 74, 488–497. doi:10.1111/j.1365-2656.2005.00947.x
Newhouse, D. J., and Vernasco, B. J. (2020). Developing a Transcriptomic Framework for Testing Testosterone-Mediated Handicap Hypotheses. Gen. Comp. Endocrinol. 298, 113577. doi:10.1016/j.ygcen.2020.113577
Páll, M. K., Mayer, I., and Borg, B. (2002). Androgen and Behavior in the Male Three-Spined Stickleback, Gasterosteus aculeatus I. - Changes in 11-ketotestosterone Levels during the Nesting Cycle. Horm. Behav. 41, 377–383. doi:10.1006/hbeh.2002.1777
Pérez, C., Lores, M., and Velando, A. (2008). Availability of Nonpigmentary Antioxidant Affects Red Coloration in Gulls. Behav. Ecol. 19, 967–973. doi:10.1093/beheco/arn053
Perez-Rodriguez, L., Mougeot, F., Alonso-Alvarez, C., Blas, J., Viñuela, J., and Bortolotti, G. R. (2008). Cell-mediated Immune Activation Rapidly Decreases Plasma Carotenoids but Does Not Affect Oxidative Stress in Red-Legged Partridges (Alectoris rufa). J. Exp. Biol. 211, 2155–2161. doi:10.1242/jeb.017178
Peters, A. (2007). Testosterone and Carotenoids: An Integrated View of Trade-Offs between Immunity and Sexual Signalling. BioEssays 29, 427–430. doi:10.1002/bies.20563
Pike, T. W., Blount, J. D., Bjerkeng, B., Lindström, J., and Metcalfe, N. B. (2007). Carotenoids, Oxidative Stress and Female Mating Preference for Longer Lived Males. Proc. R. Soc. B. 274, 1591–1596. doi:10.1098/rspb.2007.0317
Pike, T. W., Blount, J. D., Lindström, J., and Metcalfe, N. B. (2007). Availability of Non-Carotenoid Antioxidants Affects the Expression of a Carotenoid-Based Sexual Ornament. Biol. Lett. 3, 353–356. doi:10.1098/rsbl.2007.0072
Pike, T. W., Blount, J. D., Lindström, J., and Metcalfe, N. B. (2010). Dietary Carotenoid Availability, Sexual Signalling and Functional Fertility in Sticklebacks. Biol. Lett. 6, 191–193. doi:10.1098/rsbl.2009.0815
Pike, T. W. (2011). Using Digital Cameras to Investigate Animal Colouration: Estimating Sensor Sensitivity Functions. Behav. Ecol. Sociobiol. 65, 849–858. doi:10.1007/s00265-010-1097-7
Redpath, S. M., Mougeot, F., Leckie, F. M., and Evans, S. A. (2006). The Effects of Autumn Testosterone on Survival and Productivity in Red Grouse, Lagopus Lagopus Scoticus. Anim. Behav. 71, 1297–1305. doi:10.1016/j.anbehav.2005.08.012
Reed, W. L., Clark, M. E., Parker, P. G., Raouf, S. A., Arguedas, N., Monk, D. S., et al. (2006). Physiological Effects on Demography: A Long‐Term Experimental Study of Testosterone's Effects on Fitness. Am. Nat. 167, 667–683. doi:10.1086/503054
Regan, J. C., Froy, H., Walling, C. A., Moatt, J. P., and Nussey, D. H. (2019). Dietary Restriction and Insulin‐like Signalling Pathways as Adaptive Plasticity: A Synthesis and Re‐evaluation. Funct. Ecol. 34, 107–128. doi:10.1111/1365-2435.13418
Reznick, D., Nunney, L., and Tessier, A. (2000). Big Houses, Big Cars, Superfleas and the Costs of Reproduction. Trends Ecol. Evol. 15, 421–425. doi:10.1016/s0169-5347(00)01941-8
Roberts, M. L., Buchanan, K. L., and Evans, M. R. (2004). Testing the Immunocompetence Handicap Hypothesis: A Review of the Evidence. Anim. Behav. 68, 227–239. doi:10.1016/j.anbehav.2004.05.001
Rush, V., Abney, M., McKinnon, J., and Sargent, R. C. (2003). Reflectance Spectra from Free-Swimming Sticklebacks (Gasterosteus): Social Context and Eye-Jaw Contrast. Behaviour 140, 1003–1019. doi:10.1163/156853903322589614
Rushbrook, B. J., Dingemanse, N. J., and Barber, I. (2008). Repeatability in Nest Construction by Male Three-Spined Sticklebacks. Anim. Behav. 75, 547–553. doi:10.1016/j.anbehav.2007.06.011
Rutten, A. L., Oosterbeek, K., Ens, B. J., and Verhulst, S. (2006). Optimal Foraging on Perilous Prey: Risk of Bill Damage Reduces Optimal Prey Size in Oystercatchers. Behav. Ecol. 17, 297–302. doi:10.1093/beheco/arj029
Salvador, A., Veiga, J. P., Martin, J., Lopez, P., Abelenda, M., and Puertac, M. (1996). The Cost of Producing a Sexual Signal: Testosterone Increases the Susceptibility of Male Lizards to Ectoparasitic Infestation. Behav. Ecol. 7, 145–150. doi:10.1093/beheco/7.2.145
Santos, E. S. A., and Nakagawa, S. (2012). The Costs of Parental Care: A Meta-Analysis of the Trade-Off between Parental Effort and Survival in Birds. J. Evol. Biol. 25, 1911–1917. doi:10.1111/j.1420-9101.2012.02569.x
Schantz, T. v., Bensch, S., Grahn, M., Hasselquist, D., and Wittzell, H. (1999). Good Genes, Oxidative Stress and Condition-Dependent Sexual Signals. Proc. R. Soc. Lond. B 266, 1–12. doi:10.1098/rspb.1999.0597
Schielzeth, H., and Forstmeier, W. (2009). Conclusions Beyond Support: Overconfident Estimates in Mixed Models. Behav. Ecol. 20, 416–420. doi:10.1093/beheco/arn145
Sebire, M., Katsiadaki, I., and Scott, A. P. (2007). Non-Invasive Measurement of 11-ketotestosterone, Cortisol and Androstenedione in Male Three-Spined Stickleback (Gasterosteus aculeatus). Gen. Comp. Endocrinol. 152, 30–38. doi:10.1016/j.ygcen.2007.02.009
Sheldon, B. C., and Verhulst, S. (1996). Ecological Immunology: Costly Parasite Defences and Trade-Offs in Evolutionary Ecology. Trends Ecol. Evol. 11, 317–321. doi:10.1016/0169-5347(96)10039-2
Sikes, R. S., Ylönen, H., and Ylonen, H. (1998). Considerations of Optimal Litter Size in Mammals. Oikos 83, 452. doi:10.2307/3546673
Simons, M. J., Briga, M., Koetsier, E., Folkertsma, R., Wubs, M. D., Dijkstra, C., et al. (2012). Bill Redness Is Positively Associated with Reproduction and Survival in Male and Female Zebra Finches. PLoS One 7, e40721. doi:10.1371/journal.pone.0040721
Simons, M. J., Briga, M., and Verhulst, S. (2016). Stabilizing Survival Selection on Presenescent Expression of a Sexual Ornament Followed by a Terminal Decline. J. Evol. Biol. 29, 1368–1378. doi:10.1111/jeb.12877
Simons, M. J., Cohen, A. A., and Verhulst, S. (2012). What Does Carotenoid-dependent Coloration Tell? Plasma Carotenoid Level Signals Immunocompetence and Oxidative Stress State in Birds-A Meta-Analysis. PLoS One 7, e43088. doi:10.1371/journal.pone.0043088
Simons, M. J., Cohen, A. A., and Verhulst, S. (2012). What Does Carotenoid-dependent Coloration Tell? Plasma Carotenoid Level Signals Immunocompetence and Oxidative Stress State in Birds-A Meta-Analysis. PLoS One 7, e43088. doi:10.1371/journal.pone.0043088
Simons, M. J., Maia, R., Leenknegt, B., and Verhulst, S. (2014). Carotenoid-dependent Signals and the Evolution of Plasma Carotenoid Levels in Birds. Am. Nat. 184, 741–751. doi:10.1086/678402
Simons, M. J. P., Briga, M., Koetsier, E., Folkertsma, R., Wubs, M. D., Dijkstra, C., et al. (2012). Bill Redness is Positively Associated with Reproduction and Survival in Male and Female Zebra Finches. PLoS One 7, e40721. doi:10.1371/journal.pone.0040721
Simons, M. J. P., Briga, M., Leenknegt, B., and Verhulst, S. (2014). Context-dependent Effects of Carotenoid Supplementation on Reproduction in Zebra Finches. Behav. Ecol. 25. doi:10.1093/beheco/aru062
Simons, M. J. P., Reimert, I., van der Vinne, V., Hambly, C., Vaanholt, L. M., Speakman, J. R., et al. (2011). Ambient Temperature Shapes Reproductive Output During Pregnancy and Lactation in the Common Vole (Microtus arvalis): A Test of the Heat Dissipation Limit Theory. J. Exp. Biol. 214, 38–49. doi:10.1242/jeb.044230
Smith, H. G., Kallander, H., and Nilsson, J.-A. (1989). The Trade-off Between Offspring Number and Quality in the Great Tit Parus major. J. Anim. Ecol. 58, 383–401. doi:10.2307/4837
Stearns, S. C. (1989). Trade-offs in Life-History Evolution. Funct. Ecol. 3, 259–268. doi:10.2307/2389364
Stevens, M., Párraga, C. A., Cuthill, I. C., Partridge, J. C., and Troscianko, T. S. (2007). Using Digital Photography to Study Animal Coloration. Biol. J. Linn. Soc. 90, 211–237. doi:10.1111/j.1095-8312.2007.00725.x
Stigell, P., Miyata, K., and Hauta-Kasari, M. (2007). Wiener Estimation Method in Estimating of Spectral Reflectance from RGB Images. Pattern Recognit. Image Anal. 17, 233–242. doi:10.1134/s1054661807020101
Sugrue, V. J., Zoller, J. A., Narayan, P., Lu, A. T., Ortega-Recalde, O. J., Grant, M. J., et al. (2021). Castration Delays Epigenetic Aging and Feminizes DNA Methylation at Androgen-Regulated Loci. Elife 10, e64932. doi:10.7554/eLife.64932
Svensson, P. A., and Wong, B. B. M. (2011). Carotenoid-based Signals in Behavioural Ecology: A Review. Behaviour 148, 131–189. doi:10.1163/000579510x548673
Számadó, S. (2011). The Cost of Honesty and the Fallacy of the Handicap Principle. Anim. Behav. 81, 3–10. doi:10.1016/j.anbehav.2010.08.022
Therneau, T. M., and Grambsch, P. M. (2000). Modeling Survival Data: Extending the Cox Model. Germany: Springer New York. doi:10.1007/978-1-4757-3294-8
Tinbergen, J. M., and Daan, S. (1990). Family Planning in the Great Tit (Parus Major): Optimal Clutch Size as Integration of Parent and Offspring Fitness. Behaviour 114, 161–190. doi:10.1163/156853990x00103
van Mullem, P. J., and van der Vlugt, J. C. (1964). On the Age, Growth and Migration of the Anadromous Stickleback Gasterosteus aculeatus L. Investigated in Mixed Populations. Arch. Néerl Zool 16, 111–139. doi:10.1163/036551664x00031
Wedekind, C., Meyer, P., Frischknecht, M., Niggli, U. A., and Pfander, H. (1998). Different Carotenoids and Potential Information Content of Red Coloration of Male Tree-Spined Sticklebacks. J. Chem. Ecol. 24, 787–801. doi:10.1023/a:1022365315836
Williams, G. C. (1957). Pleiotropy, Natural Selection, and the Evolution of Senescence. Evolution 11, 398. doi:10.2307/2406060
Wright, D. S., Yong, L., Pierotti, M. E., and Mckinnon, J. S. (2016). Male Red Throat Coloration, Pelvic Spine Coloration, and Courtship Behaviours in Threespine Stickleback. Evol. Ecol. Res. 17 (3), 407–418.
Keywords: aging, fecundity, carotenoid, stickleback, signaling, sexual hormone, sexual selection
Citation: Simons MJP, Sebire M, Verhulst S and Groothuis TGG (2021) Androgen Elevation Accelerates Reproductive Senescence in Three-Spined Stickleback. Front. Cell Dev. Biol. 9:752352. doi: 10.3389/fcell.2021.752352
Received: 02 August 2021; Accepted: 23 November 2021;
Published: 17 December 2021.
Edited by:
Alan A. Cohen, Université de Sherbrooke, CanadaReviewed by:
Jeffrey McKinnon, East Carolina University, United StatesOwen Jones, University of Southern Denmark, Denmark
Copyright © 2021 Simons, Sebire, Verhulst and Groothuis. This is an open-access article distributed under the terms of the Creative Commons Attribution License (CC BY). The use, distribution or reproduction in other forums is permitted, provided the original author(s) and the copyright owner(s) are credited and that the original publication in this journal is cited, in accordance with accepted academic practice. No use, distribution or reproduction is permitted which does not comply with these terms.
*Correspondence: Mirre J. P. Simons, bS5zaW1vbnNAc2hlZmZpZWxkLmFjLnVr