- 1Department of Pathology, Case Western Reserve University, Cleveland, OH, United States
- 2The Case Comprehensive Cancer Center, Case Western Reserve University, Cleveland, OH, United States
- 3Department of Biochemistry, School of Medicine, Case Western Reserve University, Cleveland, OH, United States
Nuclear factor-kappaB (NF-κB) is a pleiotropic, evolutionarily conserved transcription factor family that plays a central role in regulating immune responses, inflammation, cell survival, and apoptosis. Great strides have been made in the past three decades to understand the role of NF-κB in physiological and pathological conditions. Carcinogenesis is associated with constitutive activation of NF-κB that promotes tumor cell proliferation, angiogenesis, and apoptosis evasion. NF-κB is ubiquitously expressed, however, its activity is under tight regulation by inhibitors of the pathway and through multiple posttranslational modifications. O-GlcNAcylation is a dynamic posttranslational modification that controls NF-κB-dependent transactivation. O-GlcNAcylation acts as a nutrient-dependent rheostat of cellular signaling. Increased uptake of glucose and glutamine by cancer cells enhances NF-κB O-GlcNAcylation. Growing evidence indicates that O-GlcNAcylation of NF-κB is a key molecular mechanism that regulates cancer cell proliferation, survival and metastasis and acts as link between inflammation and cancer. In this review, we are attempting to summarize the current understanding of the cohesive role of NF-κB O-GlcNAcylation in inflammation and cancer.
Introduction
The nuclear factor kappaB (NF-κB) family is a highly conserved group of master transcription factors critical in regulating cellular functions including cell survival, proliferation, differentiation, apoptosis, and immune response. NF-κB is composed of five subunits: p65 (RelA), RelB, c-Rel (Rel), p50/p105 (NF-κB1), and p52/p100 (NF-κB2) which exists as homo and heterodimers bound to the of inhibitor of kappaB (IκB) proteins (Hayden and Ghosh, 2011). NF-κB exists as preformed proteins, and its functional regulation primarily occurs through stimulus-dependent posttranslational modifications (PTMs) including phosphorylation, methylation, ubiquitination, acetylation, nitrosylation, sumoylation, and O-linked β-N-acetylglucosamine glycosylation (O-GlcNAcylation). Extensive crosstalk between PTMs (e.g., phosphorylation/O-GlcNAcylation) also regulates the function of NF-κB (Liu et al., 2004; Wang et al., 2007, 2008; Hart et al., 2011).
O-GlcNAcylation has garnered considerable interest in physiology and pathology since its discovery (Torres and Hart, 1984). O-GlcNAcylation is a posttranslational enzymatic addition of an N-acetylglucosamine (GlcNAc) to the oxygen atom of a serine, threonine, or tyrosine residue on intracellular proteins through a β-O-glycosidic linkage. In contrast to the abundance of kinases and phosphatases controlling phosphorylation (Ardito et al., 2017), O-GlcNAc cycling is controlled solely by the reciprocal activities of O-GlcNAc transferase (OGT) and O-GlcNAcase (OGA), which adds and removes O-GlcNAc from proteins, respectively. Ogt deficiency results in embryonic lethality (Shafi et al., 2000) and Oga deficiency affects embryonic development leading to neonatal lethality (Yang et al., 2012). Conditional deletion of Ogt showed the requirement of O-GlcNAcylation in T cell and fibroblast survival (O’Donnell et al., 2004) while conditional deletion of Oga in hematopoietic stem cells showed that O-GlcNAcylation cycling is essential in early thymocyte development (Abramowitz et al., 2019). Increased O-GlcNAcylation also blocks RANKL-induced p65 phosphorylation and suppresses osteoclastogenesis (Takeuchi et al., 2016). In addition to development, O-GlcNAcylation regulates a plethora of cellular functions, including cell survival, apoptosis, cell cycle, cell proliferation, stress response, and immune response, by controlling transcription, translation, and protein stability. A large number of O-GlcNAcylated proteins are involved in transcription regulation, including transcription factors such as NF-κB (Hart et al., 2007; Li et al., 2019). Exposure to high glucose or glucosamine induces NF-κB O-GlcNAcylation and increases NF-κB-dependent gene expression (James et al., 2002). NF-κB O-GlcNAcylation occurs via direct binding to OGT (Golks et al., 2007). Multiple sites of NF-κB p65 O-GlcNAcylation were later identified to have specific functional roles (Yang et al., 2008; Allison et al., 2012; Ma et al., 2017). We discovered that, unlike p65 with multiple O-GlcNAcylation sites, NF-κB c-Rel has a single O-GlcNAcylation site at Ser-350. Approximately 5% of c-Rel is O-GlcNAcylated under physiological glucose conditions (5 mM), while 25% of c-Rel is O-GlcNAcylated under hyperglycemic conditions (30 mM) (Ramakrishnan et al., 2013). The stoichiometry of p65 O-GlcNAcylation has yet to be defined. O-GlcNAcylation also modifies other proteins within the NF-κB pathway. Ser-733 O-GlcNAcylation of IKK2 is essential for its catalytic activity (Kawauchi et al., 2009). Similarly, TAB3 and TAB1 are O-GlcNAcylated at Ser-408, and Ser-395, respectively (Pathak et al., 2012; Tao et al., 2016; Figure 1).
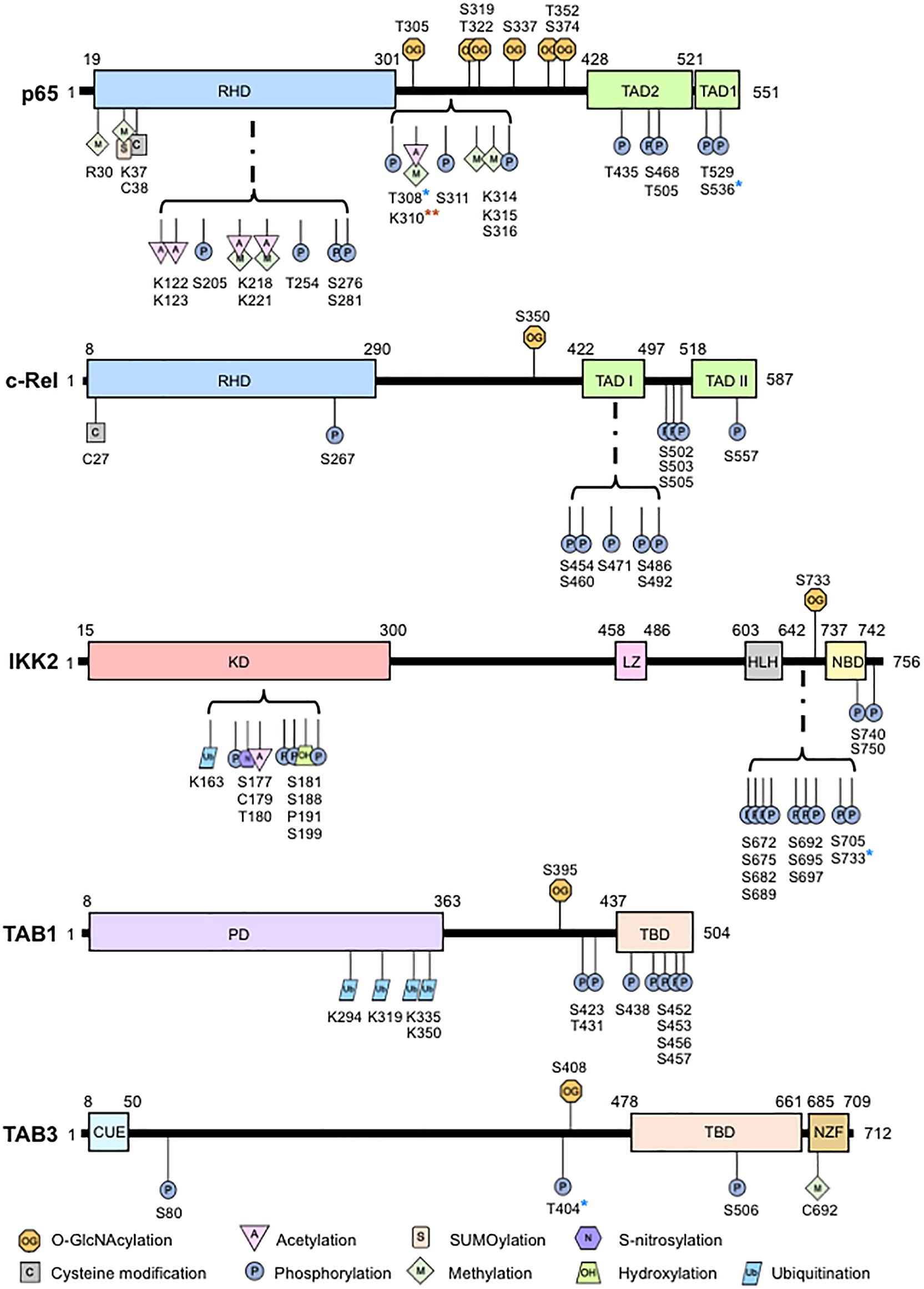
Figure 1. Overview of O-GlcNAcylation sites and other posttranslational modifications in the nuclear factor-kappaB (NF-κB) pathway proteins. Shown are the principle structural motifs and posttranslational modification sites of p65, c-Rel, IKK2, TAB1, and TAB3. RHD, rel homology domain; TAD, transactivation domain; KD, kinase domain; LZ, leucine zipper; HLH: helix-loop-helix domain, NBD, Nemo-binding domain; PD, pseudophosphatase domain, TBD, TAK1 binding domain; CUE, coupling of ubiquitin conjugation to endoplasmic reticulum degradation; NZF, Npl4 zinc finger. *Activity has been shown to have crosstalk with O-GlcNAcylation; **Site specific acetylation but not methylation at this site has been shown to be related to O-GlcNAcylation.
O-GlcNAcylation is regulated by nutrient availability. Influxes of glucose and its metabolic products activate the hexosamine biosynthetic pathway (HBP) and enhance O-GlcNAcylation (Hanover et al., 2010; Chiaradonna et al., 2018). Cancer cells favor glycolysis and lactate fermentation for their survival and proliferation, a biological phenomenon called the Warburg effect (Liberti and Locasale, 2016). Cancer cells also consume excess glutamine to sustain protein synthesis (Rajagopalan and DeBerardinis, 2011). An increase of total protein O-GlcNAcylation due to the influx of glucose and glucosamine is a typical characteristic in cancer cells (Ma and Vosseller, 2014; Ferrer et al., 2016). Constitutively overactive NF-κB is also common in both solid and hematological cancers (Karin et al., 2002; Imbert and Peyron, 2017), with altered NF-κB expression associated with worse patient outcomes (Wu et al., 2015). In turn, enhanced NF-κB O-GlcNAcylation alters the homeostatic regulation of NF-κB-dependent transcription in cancer cells. This review summarizes the current knowledge on NF-κB O-GlcNAcylation as a molecular mechanism that connects inflammation and cancer, and discusses its potential therapeutic implications and future perspectives.
Nuclear Factor-Kappab O-Glcnacylation in Solid Cancer
Elevated global protein O-GlcNAcylation is a common finding in several solid cancers including breast (Caldwell et al., 2010; Krześlak et al., 2012), prostate (Lynch et al., 2012), colorectal (Mi et al., 2011), and liver (Zhu et al., 2012). These cancers are also associated with dysregulated NF-κB function, which contributes to tumorigenesis (Xia et al., 2014). There have been many studies separately identifying the importance of NF-κB signaling and O-GlcNAcylation in tumor development, however, few have linked them together directly. Suppression of IKK2 significantly decreases tumor development in colitis-associated colon cancer (Greten et al., 2004). Separately, inhibition of bacterial (He et al., 2020) and murine (Yang et al., 2015) colonic O-GlcNAcylation is protective against colitis development possibly due to the disruption of p65 activation, which may also involve IKK2 O-GlcNAcylation. NF-κB signaling (Meylan et al., 2009; Bassères et al., 2010) and global O-GlcNAcylation (Taparra et al., 2018) are both required for the development of KRAS-induced lung cancer in mouse models. NF-κB activity facilitates the cell cycle transition from the G1 to S phase through antagonizing p53 and upregulating cyclin D1 gene expression (Chen et al., 2001) which is correlated with decreased O-GlcNAcylation (Wang et al., 2010; Drougat et al., 2012). Interestingly, there are specific instances in which NF-κB acts as a tumor suppressor. NF-κB inhibition in hepatocytes via IKK2-targeted deletion promotes hepatocarcinogenesis (Maeda et al., 2005). Separately, enhanced O-GlcNAcylation is seen in patients who suffered from tumor recurrence following liver transplantation. Taken together, this indicates that enhanced O-GlcNAcylation may inhibit NF-κB activation in hepatocytes to promote hepatocarcinogenesis. More research must be completed to specifically learn the tissue specific activating or inhibitory roles of NF-κB O-GlcNAcylation.
The existence of enhanced NF-κB activation and increased O-GlcNAcylation in several cancers beg the question: is site specific NF-κB O-GlcNAcylation involved in cancer pathogenesis? Several studies justify this possibility (Table 1). Enhancement of p65 O-GlcNAcylation is associated with the upregulation of CXCR4 expression in metastasized cervical cancer cells in the lung (Ali et al., 2017) suggesting that enhanced p65 O-GlcNAcylation during cancer may increase the metastatic potential of tumor cells. Furthermore, enhanced O-GlcNAcylation upregulates expression of matrix-metalloproteinases via increased p65 nuclear translocation resulting in an NF-κB O-GlcNAcylation-dependent increase in cholangiocarcinoma cell migration and invasion (Phoomak et al., 2016). Enhanced p65 nuclear translocation can be correlated with the decreased interaction between Thr-352 O-GlcNAcylated p65 and its physiological repressor, IκBα (Yang et al., 2008). O-GlcNAcylation at Thr-352, but not Thr-322, is required for p65 transcriptional activity (Yang et al., 2008), but O-GlcNAcylation at both sites is required for the anchorage of pancreatic ductal adenocarcinoma cells in vitro (Ma et al., 2013; Figure 1). Thus, enhanced site-specific p65 O-GlcNAcylation may contribute to the ability of tumor cells to invade and metastasize: a key hallmark of cancer. It is unclear whether or not O-GlcNAcylated p65 regulates gene expression differentially across various tissue types, and if it causes altered nuclear translocation, differential dimerization with other proteins, modulation of p65-DNA binding, or a combination of several functions.
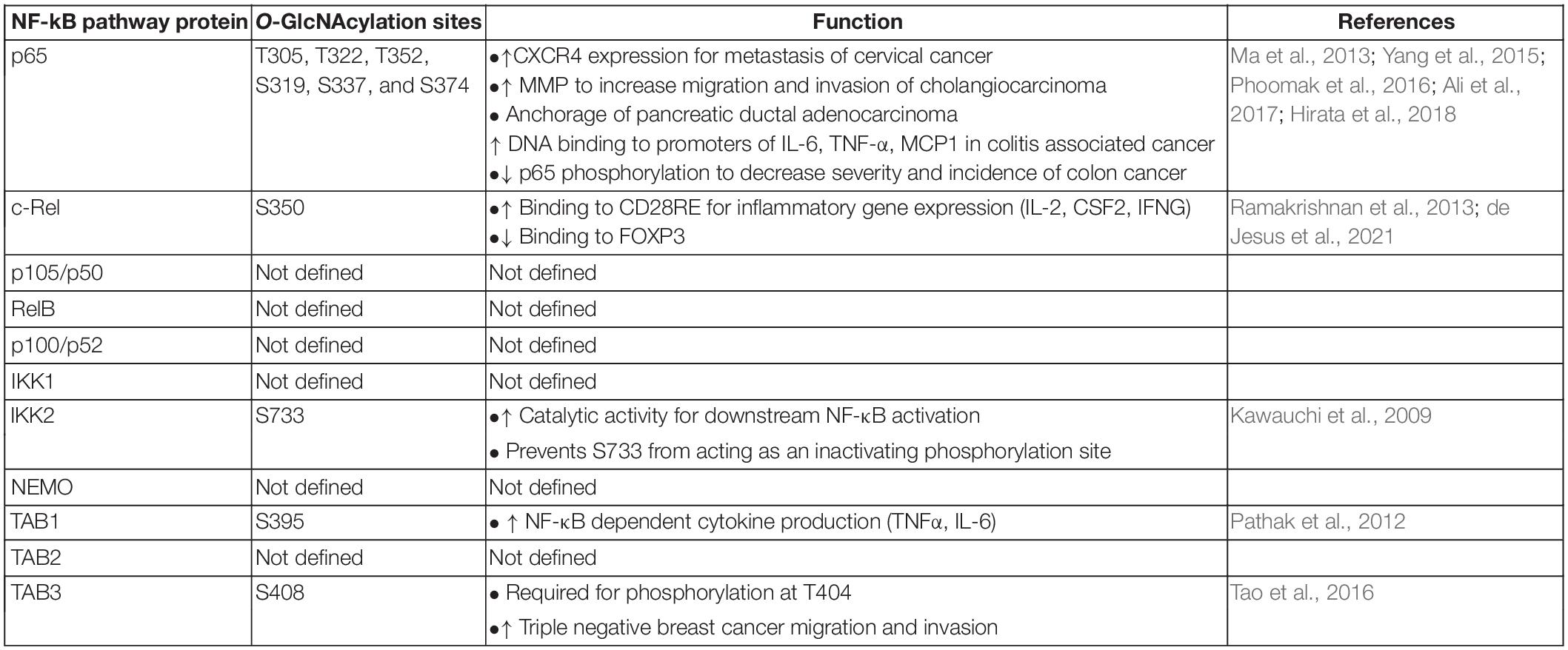
Table 1. List of known O-GlcNAcylation sites and functions in the nuclear factor-kappaB (NF-κB) pathway proteins.
Aside from p65, O-GlcNAcylation of other NF-κB signaling proteins can also be involved in solid tumor pathogenesis. TAB3 O-GlcNAcylation promotes triple negative breast cancer cell migration and invasion, and is correlated with worse patient outcomes (Tao et al., 2016). Enhanced O-GlcNAcylation of IKK2 induces NF-κB signaling (Kawauchi et al., 2009), and increased IKK2 expression is essential for cell viability in prostate cancer (Pflueger et al., 2011). This suggests that O-GlcNAcylation may regulate IKK2 function to create a cell survival advantage. More research is needed to specifically address the mechanistic role of O-GlcNAcylation of NF-κB pathway proteins in carcinogenesis and cancer progression.
Nuclear Factor-Kappab O-Glcnacylation in Hematological Cancers
The role of NF-κB O-GlcNAcylation in hematological cancers is largely unknown. Enhanced O-GlcNAcylation promotes multiple myeloma cell survival by increasing proteasome biogenesis and function to contribute to proteasomal inhibitor drug resistance (Sekine et al., 2018; Hashimoto et al., 2020). Heightened proteasome function may possibly result in an increase of IκBα degradation and subsequently enhance NF-κB activation in multiple myeloma, as seen in pancreatic cancer cells (Ma et al., 2013). Thus, elevated O-GlcNAcylation may worsen multiple myeloma patient outcomes through enhanced NF-κB activation causing diminished treatment efficacy. The reported function of global O-GlcNAcylation in leukemia is mixed. Enhanced O-GlcNAcylation is associated with chronic lymphocytic (Shi et al., 2010) and pre-B acute lymphocytic (Zhang et al., 2017) leukemia pathogenesis, and is accompanied with constitutive NF-κB activity due to the persistent activation of IκB kinases (Kordes et al., 2000; Takaesu et al., 2000; Bichi et al., 2002). Enhanced O-GlcNAcylation (Asthana et al., 2018) and aberrant NF-κB activity (Guzman et al., 2001) also occur in acute myeloid leukemia, and pharmacological inhibition of O-GlcNAcylation is able to induce the differentiation and apoptosis of AML cells (Asthana et al., 2018), However, contrasting studies have shown that enhanced O-GlcNAcylation via an increase of OGT protein expression via hypomethylation of its 5’ UTR promoter region using dronabinol also induces acute myeloid leukemic cell apoptosis and releases the differentiation blockage of acute myeloid leukemic blasts (Kampa-Schittenhelm et al., 2020). This contrasting data can potentially be explained due to the usage of a mouse xenograft model versus human patient samples with specific molecular mutations. Dronabinol also acts as an unselective epigenetic modifier, and while its effect on OGT was identified as a key regulator in instigating leukemia cell apoptosis and differentiation, it is possible that cross talk or contribution from other proteins is occurring. It is also notable that neither of these reports identified specific O-GlcNAcylated proteins that regulate this phenomenon seen in AML cells. Additional studies on NF-κB-specific O-GlcNAcylation in hematological cancers are necessary.
Of note, there are also studies on site-specific O-GlcNAcylation in proteins that interact with NF-κB. It has been reported that NF-κB can be positively and negatively regulated by STAT5. The binding of NF-κB p65 to the IL-6 promoter is enhanced by STAT5 interaction in myeloid leukemic M1 cells (Kawashima et al., 2001). Interestingly, STAT5 O-GlcNAcylation was found to enhance its tyrosine phosphorylation to promote neoplastic myeloid transformation (Freund et al., 2017). However, STAT5 also competes with NF-κB for binding to target genes in B cell acute lymphoblastic leukemia (Katerndahl et al., 2017). How these interactions directly modulate STAT5-dependent NF-κB function remains to be determined.
Crosstalk Between O-Glcnacylation and Other Posttranslational Modifications of Nuclear Factor-Kappab
The complexity and the diverse functional roles of O-GlcNAcylation, and its crosstalk with other PTMs of NF-κB [reviewed elsewhere (Perkins, 2006; Christian et al., 2016)], are immense. O-GlcNAcylation can act independently, directly compete with phosphorylation sites or modulate adjacent PTMs by altering the structure and/or binding properties of the protein. O-GlcNAcylation and phosphorylation often occur at the same or adjacent hydroxyl moieties. There has yet to be evidence to show competition between O-GlcNAcylation and phosphorylation at the same site in the five NF-κB subunits. Preliminary evidence suggests that p65 Thr-308 phosphorylation may impair Thr-305 O-GlcNAcylation (Ma et al., 2017). A similar crosstalk may also occur between phosphorylation sites at Ser-311 and Ser-316 phosphorylation of p65 and adjacent O-GlcNAcylation sites at Thr-305, Ser-319 or Ser-322 due to their proximity. O-GlcNAcylation can also negatively regulate p65 phosphorylation. Enhanced global O-GlcNAcylation following PUGNAc treatment, which also increases p65-specific O-GlcNAcylation, inhibits Ser-536 phosphorylation and NF-κB activation (Xing et al., 2011). In contrast, enhanced O-GlcNAcylation by OGT overexpression positively increases IKK-mediated Ser-536 phosphorylation of p65 contributing to NF-κB activation (Ma et al., 2017). Thus, it appears that an enhanced global O-GlcNAcylated state attained by OGA inhibition and an increase in O-GlcNAcylation cycling achieved by OGT overexpression yield contrasting outcomes, suggesting the need for careful choice in experimental methodology while studying O-GlcNAcylation. p65 O-GlcNAcylation at Thr-305 and Ser-319 has also been shown to enhance p65 acetylation at Lys-310 by p300 (Allison et al., 2012; Ma et al., 2017). OGT is essential for p300-mediated p65 Lys-310 acetylation (Allison et al., 2012), possibly through increasing p300 expression (Ma et al., 2017). p65 Lys-310 can also undergo methylation (Lu and Stark, 2015) although no studies have linked O-GlcNAcylation and methylation at this site (Figure 1). Much remains unknown on the mechanisms involved in this interplay, which may involve a plausible preferential recognition of O-GlcNAcylated p65 by p300 and formation of a p65, p300, and OGT complex.
Nominal information exists on the crosstalk between O-GlcNAcylation and other PTMs on other NF-κB pathway proteins (Figure 1). TAB3 O-GlcNAcylation at Ser-408 is required for its phosphorylation at Thr-404, which subsequently activates downstream TAK1 and NF-κB signaling. In contrast, the relationship between O-GlcNAcylation and phosphorylation in IKK2 appears to be competitive. Induction of O-GlcNAcylation of IKK2 at Ser-733 through glucose exposure, commonly found in oncogenic cells undergoing increased glycolysis, prevents the residue from functioning as an inactivating phosphorylation site thus creating a positive feedback loop to induce NF-κB activity (Kawauchi et al., 2009). Since IKK2 activity enhances cell viability in prostate cancer (Pflueger et al., 2011), O-GlcNAcylation of IKK2 may promote prostate cancer cell survival. Taken together, it appears that O-GlcNAcylation of upstream regulators of NF-κB pathway drives the NF-κB overactivity commonly seen in cancer.
Proinflammatory Role of Nuclear Factor-Kappab O-Glcnacylation in Cancer
While the pathogenesis of cancer is varied, a common principle that underlies many tumorigenic processes is chronic inflammation. NF-κB is an important molecular link between inflammation and cancer. In hepatitis-associated neoplastic transformation, NF-κB activation enhances TNF-α, which in turn enhances NF-κB-dependent anti-apoptotic gene expression in a feed-forward loop (Pikarsky et al., 2004), and IKK2 is required for epithelial growth during colitis-associated cancer (Greten et al., 2004). There have been several reports suggesting the role of NF-κB O-GlcNAcylation in inflammation-induced cancer. Hyperglycemia-induced O-GlcNAcylation of p65 (Dela Justina et al., 2017) and c-Rel (Ramakrishnan et al., 2013) augments the production of inflammatory cytokines. Thr-322 O-GlcNAcylation of p65 has been proposed to control constitutive NF-κB activity in states of hyperglycemia (Yang et al., 2008). OGT-mediated O-GlcNAcylation of p65 and IKK1 promotes TNF-α and nitric oxide production in rat pancreatic acinar cells and progresses acute pancreatitis (Zhang et al., 2015). p65 O-GlcNAcylation at Thr-322 and Thr-352 also enhances DNA binding to the promoters of IL-6, TNF-α, and MCP1 which contributes to the development of colitis and colitis-associated cancer (Yang et al., 2015). Similar to p65, c-Rel Ser-350 O-GlcNAcylation may also contribute to an inflammatory state under hyperglycemic conditions via the expression of TCR-induced, CD28RE-dependent genes such as IL-2, CSF2, and IFNG (Ramakrishnan et al., 2013). Thus, c-Rel O-GlcNAcylation may contribute to inflammation-induced cancer or cancer-associated inflammation, both of which involve malignant cells utilizing excess glucose. Interestingly, c-Rel O-GlcNAcylation suppresses FOXP3 expression needed for the development of immunosuppressive Treg cells (de Jesus et al., 2021). Thus, c-Rel O-GlcNAcylation may also impart antitumor effects, as compromised Treg cell function may allow for improved cancer cell elimination by cytotoxic T cells. Further linking proinflammatory functions of NF-κB, O-GlcNAcylation and cancer, Ser-395 O-GlcNAcylation of TAB1 is required for NF-κB activation and expression of IL-6 and TNF-α (Pathak et al., 2012). Taken together, it appears that O-GlcNAcylation-dependent NF-κB functions may predominantly create a proinflammatory state to promote cancer development.
Mechanistic knowledge on the regulation of NF-κB by O-GlcNAcylation is limited. p65 O-GlcNAcylation decreases the binding of p65 to the inhibitor, IκBα, which increases p65 nuclear translocation and transactivation of proinflammatory genes (Yang et al., 2008). Whether c-Rel O-GlcNAcylation exerts a similar effect on IκBα is unknown. The mechanistic role of c-Rel O-GlcNAcylation has been implicated in the regulation of both protein-DNA and protein-protein interactions. O-GlcNAcylation regulates c-Rel-DNA binding and transcriptional activity both positively (Hwang et al., 2013; Ramakrishnan et al., 2013) and negatively (de Jesus et al., 2021). In addition, enhanced c-Rel O-GlcNAcylation following proinflammatory LPS treatment promotes interaction of c-Rel with p50 in microglia cells (Hwang et al., 2013) and O-GlcNAcylated c-Rel exists in complex with p65 in lymphocytes (Ramakrishnan et al., 2013). This indicates the intriguing possibility of a cell type specific O-GlcNAcylation-dependent NF-κB dimerization, which is an interesting future research direction.
Anti-Inflammatory Role of Nuclear Factor-Kappab O-Glcnacylation in Cancer
A number of studies have found that NF-κB O-GlcNAcylation has an anti-inflammatory role. p65 O-GlcNAcylation was found to protect against inflammatory stress by attenuating NF-κB signaling in smooth muscle cells (Xing et al., 2011) and primary cultured cardiomyocytes (Zou et al., 2009). These findings were supported by the observations that glucosamine treatment improved cardiac function and attenuated inflammation following trauma hemorrhage or arterial damage and suppressed LPS-induced inflammation by reducing the amount of c-Rel O-GlcNAcylation and decreasing the production of inflammatory cytokines (Hwang et al., 2013). This countered many previous reports, which stated that increased concentrations of glucosamine would augment O-GlcNAcylation through the influx of the HBP pathway. While it is possible that the amount of O-GlcNAcylation enhancement after glucosamine treatment is indeed protein specific, it was later reported that the ability of glucosamine to impact c-Rel O-GlcNAcylation was dependent on glucose concentration in the culture conditions (Hwang et al., 2017). As these findings were in non-tumorous cells, it is possible that the context in which NF-κB is O-GlcNAcylated dictates its proinflammatory or anti-inflammatory capabilities. However, a later study showed that enhanced O-GlcNAcylation leads to a decrease in incidence and severity of inflammation-mediated colon carcinogenesis by suppressing NF-κB p65 phosphorylation and activation (Hirata et al., 2018). This directly opposes the results from Yang et al. (2015), which stated that enhanced p65 O-GlcNAcylation induces an inflammatory state and promotes colitis-associated cancer. These contrasting data may potentially be explained through their usage of differing mouse models. Yang et al., utilized an Oga+/– mouse model to enhance O-GlcNAcylation while Hirata et al., implemented an Ogt-transgenic mouse. OGA has been reported to have histone acetylation activity (Toleman et al., 2004; Hayakawa et al., 2013) and partial deficiency of this protein may thus inadvertently decrease histone acetylation. OGT also interacts with multiple proteins, and its overexpression can lead to non-specific O-GlcNAcylation of cellular proteins. As such, these studies suggest and intricate relationship of glucose, glucosamine, inflammation, and warrants comprehensive studies on the specific role of O-GlcNAcylation in the context of cancer to derive mechanistic insights.
Therapeutic Implications and Future Perspectives
Usage of NF-κB inhibitors to improve cancer therapy has long been discussed. Bortezomib, a first-in-class proteasome inhibitor with pan-NF-κB inhibitory function, has shown strong antitumor activity in multiple myeloma (Field-Smith et al., 2006) and AML (Attar et al., 2008; Colado et al., 2008; Howard et al., 2013), as well as in solid tumors such as preclinical models of breast (Teicher et al., 1999) and prostate (Adams et al., 1999) cancer. NF-κB inhibition using a non-degradable IκB super repressor (Duffey et al., 1999; Cusack et al., 2000; Arlt et al., 2002) or parthenolide (Sohma et al., 2011; Carlisi et al., 2017; Darwish et al., 2019) have been successful in various models. However, severe adverse effects have significantly hampered the clinical use of pan-NF-κB inhibitors due to the multifarious biological roles of NF-κB. This indicates a need for less toxic but still potent NF-κB inhibitors. Targeting specific PTMs of NF-κB could thus prove to be an ideal approach to target aberrant NF-κB functions in cancer while still maintaining its beneficial functions. Hence, the implications of an NF-κB-specific O-GlcNAcylation inhibitor are extremely promising with the potential to allow for optimal drug translation, but remains as an unmet need. As discussed previously, multiple O-GlcNAcylation sites have been identified in p65, with Thr-352 specifically required for its transcriptional activity (Yang et al., 2008). Inhibition of p65 O-GlcNAcylation at Thr-352 would likely dampen NF-κB overactivity and curtail the overexpression of p65-dependent genes, particularly those involved in tumor metastasis. However, the function of p65 O-GlcNAcylation has been found to both increase p65 DNA binding, as well as inhibit NF-κB activation. Taking into consideration NF-κB’s ability to act as both a tumor promoter and suppressor – as a word of caution - it is possible that enhanced p65 O-GlcNAcylation may upregulate or downregulate genes controlling cell proliferation or inflammation in a context dependent manner. c-Rel is only O-GlcNAcylated at Ser-350 (Ramakrishnan et al., 2013). c-Rel activity has been positively correlated with worse outcomes in hematological and solid tumors (Hunter et al., 2016). We have shown that O-GlcNAc modification of Ser-350 positively and negatively regulates c-Rel-dependent transcription (Ramakrishnan et al., 2013; de Jesus et al., 2021), and thus pharmacological regulation of Ser-350 in c-Rel could have significant benefits in both cancer and autoimmune disorders.
Current known roles of NF-κB O-GlcNAcylation are far from complete. Existing knowledge provides only partial insight on the role of O-GlcNAcylation in controlling p65 and c-Rel functions. Among the large number of upstream proteins regulating the NF-κB pathway, only three (IKK2, TAB1, and TAB3) have had their specific O-GlcNAcylation sites mapped (Table 1). Thus, identification of O-GlcNAcylation sites and function in other NF-κB subunits, (p105/p50, p100/p52, and RelB), and other key proteins regulating the canonical and non-canonical NF-κB pathways is an immediate need to be addressed in the field. Mechanistic knowledge gained through studying the regulation of NF-κB family of proteins by O-GlcNAcylation will have broad implications in learning how O-GlcNAcylation may control the functions of thousands of downstream proteins involved in carcinogenesis. Understanding the role of site-specific O-GlcNAcylation is essential in developing NF-κB PTM-specific cancer therapeutics that may exhibit relatively lower side effects than targeting total NF-κB proteins. Such novel therapeutics targeting O-GlcNAcylated NF-κB may position them as independent agents or as complements to current therapeutic regimens.
Author Contributions
PR conceptualized the idea. AL wrote the manuscript. PR and AL edited, contributed to the article, and approved the submitted version.
Funding
PR was supported by NIH/NCI grant R21 CA246194 and NIH/NIAID grants R01AI116730 and R21AI144264. AL was supported by the National Institute of Health NIH/NEI T32 pre-doctoral training grant T32EY007157.
Conflict of Interest
The authors declare that the research was conducted in the absence of any commercial or financial relationships that could be construed as a potential conflict of interest.
Publisher’s Note
All claims expressed in this article are solely those of the authors and do not necessarily represent those of their affiliated organizations, or those of the publisher, the editors and the reviewers. Any product that may be evaluated in this article, or claim that may be made by its manufacturer, is not guaranteed or endorsed by the publisher.
References
Abramowitz, L. K., Harly, C., Das, A., Bhandoola, A., and Hanover, J. A. (2019). Blocked O-GlcNAc cycling disrupts mouse hematopoeitic stem cell maintenance and early T cell development. Sci. Rep. 9:12569. doi: 10.1038/s41598-019-48991-8
Adams, J., Palombella, V. J., Sausville, E. A., Johnson, J., Destree, A., Lazarus, D. D., et al. (1999). Proteasome inhibitors: a novel class of potent and effective antitumor agents. Cancer Res. 59, 2615–2622.
Ali, A., Kim, S. H., Kim, M. J., Choi, M. Y., Kang, S. S., Cho, G. J., et al. (2017). O-GlcNAcylation of NF-κB promotes lung metastasis of cervical cancer cells via upregulation of CXCR4 expression. Mol. Cells 40, 476–484. doi: 10.14348/molcells.2017.2309
Allison, D. F., Wamsley, J. J., Kumar, M., Li, D., Gray, L. G., Hart, G. W., et al. (2012). Modification of RelA by O-linked N-acetylglucosamine links glucose metabolism to NF-κB acetylation and transcription. Proc. Natl. Acad. Sci. U.S.A. 109, 16888–16893. doi: 10.1073/pnas.1208468109
Ardito, F., Giuliani, M., Perrone, D., Troiano, G., and Lo Muzio, L. (2017). The crucial role of protein phosphorylation in cell signalingand its use as targeted therapy (Review). Int. J. Mol. Med. 40, 271–280. doi: 10.3892/ijmm.2017.3036
Arlt, A., Vorndamm, J., Müerköster, S., Yu, H., Schmidt, W. E., Fölsch, U. R., et al. (2002). Autocrine production of interleukin 1β confers constitutive nuclear factor κB activity and chemoresistance in pancreatic carcinoma cell lines. Cancer Res. 62, 910–916.
Asthana, A., Ramakrishnan, P., Vicioso, Y., Zhang, K., and Parameswaran, R. (2018). Hexosamine biosynthetic pathway inhibition leads to AML cell differentiation and cell death. Mol. Cancer Ther. 17, 2226–2237. doi: 10.1158/1535-7163.MCT-18-0426
Attar, E. C., DeAngelo, D. J., Supko, J. G., D’Amato, F., Zahrieh, D., Sirulnik, A., et al. (2008). Phase I and pharmacokinetic study of bortezomib in combination with idarubicinand cytarabine in patients with acute myelogenous leukemia. Clin. Cancer Res. 14, 1446–1454. doi: 10.1158/1078-0432.CCR-07-4626
Bassères, D. S., Ebbs, A., Levantini, E., and Baldwin, A. S. (2010). Requirement of the NF-κB subunit p65/RelA for K-Ras-induced lung tumorigenesis. Cancer Res. 70, 3537–3546. doi: 10.1158/0008-5472.CAN-09-4290
Bichi, R., Shinton, S. A., Martin, E. S., Koval, A., Calin, G. A., Cesari, R., et al. (2002). Human chronic lymphocytic leukemia modeled in mouse by targeted TCL1 expression. Proc. Natl. Acad. Sci. U.S.A. 99, 6955–6960. doi: 10.1073/pnas.102181599
Caldwell, S. A., Jackson, S. R., Shahriari, K. S., Lynch, T. P., Sethi, G., Walker, S., et al. (2010). Nutrient sensor O-GlcNAc transferase regulates breast cancer tumorigenesis through targeting of the oncogenic transcription factor FoxM1. Oncogene 29, 2831–2842. doi: 10.1038/onc.2010.41
Carlisi, D., De Blasio, A., Drago-Ferrante, R., Di Fiore, R., Buttitta, G., Morreale, M., et al. (2017). Parthenolide prevents resistance of MDA-MB231 cells to doxorubicin and mitoxantrone: the role of Nrf2. Cell Death Discov. 3:17078. doi: 10.1038/cddiscovery.2017.78
Chen, F., Castranova, V., and Shi, X. (2001). New insights into the role of nuclear factor-κB in cell growth regulation. Am. J. Pathol. 159, 387–397. doi: 10.1016/S0002-9440(10)61708-7
Chiaradonna, F., Ricciardiello, F., and Palorini, R. (2018). The nutrient-sensing hexosamine biosynthetic pathway as the hub of cancer metabolic rewiring. Cells 7:53. doi: 10.3390/cells7060053
Christian, F., Smith, E., and Carmody, R. (2016). The regulation of NF-κB subunits by phosphorylation. Cells 5:12. doi: 10.3390/cells5010012
Colado, E., Álvarez-Fernández, S., Maiso, P., Martín-Sánchez, J., Vidriales, M. B., Garayoa, M., et al. (2008). The effect of the proteasome inhibitor bortezomib on acute myeloid leukemia cells and drug resistance associated with the CD34+ immature phenotype. Haematologica 93, 57–66. doi: 10.3324/haematol.11666
Cusack, J. C., Liu, R., and Baldwin, A. S. (2000). Inducible chemoresistance to 7-ethyl-10-[4-(1-piperidino)-1-piperidino]- carbonyloxycamptothecin (CPT-11) in colorectal cancer cells and a xenograft model is overcome by inhibition of nuclear factor-κB activation. Cancer Res. 60, 2323–2330.
Darwish, N. H. E., Sudha, T., Godugu, K., Bharali, D. J., Elbaz, O., Abd El-Ghaffar, H. A., et al. (2019). Novel targeted nano-parthenolide molecule against NF-kB in acute myeloid leukemia. Molecules 24:2103. doi: 10.3390/molecules24112103
de Jesus, T. J., Tomalka, J. A., Centore, J. T., Staback Rodriguez, F. D., Agarwal, R. A., Liu, A. R., et al. (2021). Negative regulation of FOXP3 expression by c-Rel O-GlcNAcylation. Glycobiology 31, 812–826. doi: 10.1093/glycob/cwab001
Dela Justina, V., Gonçalves, J. S., de Freitas, R. A., Fonseca, A. D., Volpato, G. T., Tostes, R. C., et al. (2017). Increased O-linked N-acetylglucosamine modification of NF-K B and augmented cytokine production in the placentas from hyperglycemic rats. Inflammation 40, 1773–1781. doi: 10.1007/s10753-017-0620-7
Drougat, L., Olivier-Van Stichelen, S., Mortuaire, M., Foulquier, F., Lacoste, A. S., Michalski, J. C., et al. (2012). Characterization of O-GlcNAc cycling and proteomic identification of differentially O-GlcNAcylated proteins during G1/S transition. Biochim. Biophys. Acta Gen. Subj. 1820, 1839–1848. doi: 10.1016/j.bbagen.2012.08.024
Duffey, D. C., Chen, Z., Dong, G., Ondrey, F. G., Wolf, J. S., Brown, K., et al. (1999). Expression of a dominant-negative mutant inhibitor-κBα of nuclear factor-κB in human head and neck squamous cell carcinoma inhibits survival, proinflammatory cytokine expression, and tumor growth in vivo. Cancer Res. 59, 3468–3474.
Ferrer, C. M., Sodi, V. L., and Reginato, M. J. (2016). O-GlcNAcylation in cancer biology: linking metabolism and signaling. J. Mol. Biol. 428, 3282–3294. doi: 10.1016/j.jmb.2016.05.028
Field-Smith, A., Morgan, G. J., and Davies, F. E. (2006). Bortezomib (VelcadeTM) in the treatment of multiple myeloma. Ther. Clin. Risk Manag. 2, 271–279. doi: 10.2147/tcrm.2006.2.3.271
Freund, P., Kerenyi, M. A., Hager, M., Wagner, T., Wingelhofer, B., Pham, H. T. T., et al. (2017). O-GlcNAcylation of STAT5 controls tyrosine phosphorylation and oncogenic transcription in STAT5-dependent malignancies. Leukemia 31, 2132–2142. doi: 10.1038/leu.2017.4
Golks, A., Tran, T. T., Goetschy, J. F., and Guerini, D. (2007). Requirement for O-linked N -acetylglucosaminyltransferase in lymphocytes activation. EMBO J. 26, 4368–4379. doi: 10.1038/sj.emboj.7601845
Greten, F. R., Eckmann, L., Greten, T. F., Park, J. M., Li, Z. W., Egan, L. J., et al. (2004). IKKβ links inflammation and tumorigenesis in a mouse model of colitis-associated cancer. Cell 118, 285–296. doi: 10.1016/j.cell.2004.07.013
Guzman, M. L., Neering, S. J., Upchurch, D., Grimes, B., Howard, D. S., Rizzieri, D. A., et al. (2001). Nuclear factor-κB is constitutively activated in primitive human acute myelogenous leukemia cells. Blood 98, 2301–2307. doi: 10.1182/blood.V98.8.2301
Hanover, J. A., Krause, M. W., and Love, D. C. (2010). The hexosamine signaling pathway: O-GlcNAc cycling in feast or famine. Biochim. Biophys. Acta Gen. Subj. 1800, 80–95. doi: 10.1016/j.bbagen.2009.07.017
Hart, G. W., Housley, M. P., and Slawson, C. (2007). Cycling of O-linked β-N-acetylglucosamine on nucleocytoplasmic proteins. Nature 446, 1017–1022. doi: 10.1038/nature05815
Hart, G. W., Slawson, C., Ramirez-Correa, G., and Lagerlof, O. (2011). Cross Talk between O-GlcNAcylation and phosphorylation: roles in signaling, transcription, and chronic disease. Annu. Rev. Biochem. 80, 825–858. doi: 10.1146/annurev-biochem-060608-102511
Hashimoto, E., Okuno, S., Hirayama, S., Arata, Y., Goto, T., Kosako, H., et al. (2020). Enhanced O-GlcNAcylation mediates cytoprotection under proteasome impairment by promoting proteasome turnover in cancer cells. iScience 23:101299. doi: 10.1016/j.isci.2020.101299
Hayakawa, K., Hirosawa, M., Tabei, Y., Arai, D., Tanaka, S., Murakami, N., et al. (2013). Epigenetic switching by the metabolism-sensing factors in the generation of orexin neurons from mouse embryonic stem cells. J. Biol. Chem. 288, 17099–17110. doi: 10.1074/jbc.M113.455899
Hayden, M. S., and Ghosh, S. (2011). NF-κB in immunobiology. Cell Res. 21, 223–244. doi: 10.1038/cr.2011.13
He, X., Gao, J., Peng, L., Hu, T., Wan, Y., Zhou, M., et al. (2020). Bacterial O-GlcNAcase genes abundance decreases in ulcerative colitis patients and its administration ameliorates colitis in mice. Gut 70, 1872–1883. doi: 10.1136/gutjnl-2020-322468
Hirata, Y., Nakagawa, T., Moriwaki, K., Koubayashi, E., Kakimoto, K., Takeuchi, T., et al. (2018). Augmented O-GlcNAcylation alleviates inflammation-mediated colon carcinogenesis via suppression of acute inflammation. J. Clin. Biochem. Nutr. 62, 221–229. doi: 10.3164/jcbn.17-106
Howard, D. S., Liesveld, J., Phillips, G. L., Hayslip, J., Weiss, H., Jordan, C. T., et al. (2013). A phase I study using bortezomib with weekly idarubicin for treatment of elderly patients with acute myeloid leukemia. Leuk. Res. 37, 1502–1508. doi: 10.1016/j.leukres.2013.09.003
Hunter, J. E., Leslie, J., and Perkins, N. D. (2016). C-Rel and its many roles in cancer: an old story with new twists. Br. J. Cancer 114, 1–6. doi: 10.1038/bjc.2015.410
Hwang, J. S., Kwon, M. Y., Kim, K. H., Lee, Y., Lyoo, I. K., Kim, J. E., et al. (2017). Lipopolysaccharide (LPS)-stimulated iNOS induction is increased by glucosamine under normal glucose conditions but is inhibited by glucosamine under high glucose conditions in macrophage cells. J. Biol. Chem. 292, 1724–1736. doi: 10.1074/jbc.M116.737940
Hwang, S., Hwang, J., Kim, S., and Han, I.-O. (2013). O-GlcNAcylation and p50/p105 binding of c-Rel are dynamically regulated by LPS and glucosamine in BV2 microglia cells. Br. J. Pharmacol. 169, 1551–1560.
Imbert, V., and Peyron, J. F. (2017). NF-κB in hematological malignancies. Biomedicines 5:27. doi: 10.3390/biomedicines5020027
James, L. R., Tang, D., Ingram, A., Ly, H., Thai, K., Cai, L., et al. (2002). Flux through the hexosamine pathway is a determinant of nuclear factor κB-dependent promoter activation. Diabetes 51, 1146–1156. doi: 10.2337/diabetes.51.4.1146
Kampa-Schittenhelm, K. M., Haverkamp, T., Bonin, M., Tsintari, V., Bühring, H. J., Haeusser, L., et al. (2020). Epigenetic activation of O-linked β-N-acetylglucosamine transferase overrides the differentiation blockage in acute leukemia. EBioMedicine 54:102678. doi: 10.1016/j.ebiom.2020.102678
Karin, M., Cao, Y., Greten, F. R., and Li, Z. W. (2002). NF-κB in cancer: from innocent bystander to major culprit. Nat. Rev. Cancer 2, 301–310. doi: 10.1038/nrc780
Katerndahl, C. D. S., Heltemes-Harris, L. M., Willette, M. J. L., Henzler, C. M., Frietze, S., Yang, R., et al. (2017). Antagonism of B cell enhancer networks by STAT5 drives leukemia and poor patient survival. Nat. Immunol. 18, 694–704. doi: 10.1038/ni.3716
Kawashima, T., Murata, K., Akira, S., Tonozuka, Y., Minoshima, Y., Feng, S., et al. (2001). STAT5 induces macrophage differentiation of M1 leukemia cells through activation of IL-6 production mediated by NF-κB p65. J. Immunol. 167, 3652–3660. doi: 10.4049/jimmunol.167.7.3652
Kawauchi, K., Araki, K., Tobiume, K., and Tanaka, N. (2009). Loss of p53 enhances catalytic activity of IKKβ through O-linked β-N-acetyl glucosamine modification. Proc. Natl. Acad. Sci. U.S.A. 106, 3431–3436. doi: 10.1073/pnas.0813210106
Kordes, U., Krappmann, D., Heissmeyer, V., Ludwig, W. D., and Scheidereit, C. (2000). Transcription factor NF-κB is constitutively activated in acute lymphoblastic leukemia cells. Leukemia 14, 399–402. doi: 10.1038/sj.leu.2401705
Krześlak, A., Forma, E., Bernaciak, M., Romanowicz, H., and Bryś, M. (2012). Gene expression of O-GlcNAc cycling enzymes in human breast cancers. Clin. Exp. Med. 12, 61–65. doi: 10.1007/s10238-011-0138-5
Li, Y., Xie, M., Men, L., and Du, J. (2019). O-GlcNAcylation in immunity and inflammation: an intricate system (Review). Int. J. Mol. Med. 44, 363–374. doi: 10.3892/ijmm.2019.4238
Liberti, M. V., and Locasale, J. W. (2016). The Warburg effect: how does it benefit cancer cells? Trends Biochem. Sci. 41, 211–218. doi: 10.1016/j.tibs.2015.12.001
Liu, F., Iqbal, K., Grundke-Iqbal, I., Hart, G. W., and Gong, C. X. (2004). O-GlcNAcylation regulates phosphorylation of tau: a mechanism involved in Alzheimer’s disease. Proc. Natl. Acad. Sci. U.S.A. 101, 10804–10849. doi: 10.1073/pnas.0400348101
Lu, T., and Stark, G. R. (2015). NF-κB: regulation by methylation. Cancer Res. 75, 3692–3695. doi: 10.1158/0008-5472.CAN-15-1022
Lynch, T. P., Ferrer, C. M., Jackson, S. R. E., Shahriari, K. S., Vosseller, K., and Reginato, M. J. (2012). Critical role of O-linked β-N-acetylglucosamine transferase in prostate cancer invasion, angiogenesis, and metastasis. J. Biol. Chem. 287, 11070–11081. doi: 10.1074/jbc.M111.302547
Ma, Z., Chalkley, R. J., and Vosseller, K. (2017). Hyper-O-GlcNAcylation activates nuclear factor κ-light-chainenhancer of activated B cells (NF-κB) signaling through interplay with phosphorylation and acetylation. J. Biol. Chem. 292, 9150–9163. doi: 10.1074/jbc.M116.766568
Ma, Z., Vocadlo, D. J., and Vosseller, K. (2013). Hyper-O-GlcNAcylation is anti-apoptotic and maintains constitutive NF-κB activity in pancreatic cancer cells. J. Biol. Chem. 288, 15121–15130. doi: 10.1074/jbc.M113.470047
Ma, Z., and Vosseller, K. (2014). Cancer metabolism and elevated O-GlcNAc in oncogenic signaling. J. Biol. Chem. 289, 34457–34465. doi: 10.1074/jbc.R114.577718
Maeda, S., Kamata, H., Luo, J. L., Leffert, H., and Karin, M. (2005). IKKβ couples hepatocyte death to cytokine-driven compensatory proliferation that promotes chemical hepatocarcinogenesis. Cell 121, 977–990. doi: 10.1016/j.cell.2005.04.014
Meylan, E., Dooley, A. L., Feldser, D. M., Shen, L., Turk, E., Ouyang, C., et al. (2009). Requirement for NF-kappaB signalling in a mouse model of lung adenocarcinoma. Nature 462, 104–107. doi: 10.1038/nature08462
Mi, W., Gu, Y., Han, C., Liu, H., Fan, Q., Zhang, X., et al. (2011). O-GlcNAcylation is a novel regulator of lung and colon cancer malignancy. Biochim. Biophys. Acta Mol. Basis Dis. 1812, 514–519. doi: 10.1016/j.bbadis.2011.01.009
O’Donnell, N., Zachara, N. E., Hart, G. W., and Marth, J. D. (2004). Ogt -dependent X-chromosome-linked protein glycosylation is a requisite modification in somatic cell function and embryo viability. Mol. Cell. Biol. 24, 1680–1690. doi: 10.1128/mcb.24.4.1680-1690.2004
Pathak, S., Borodkin, V. S., Albarbarawi, O., Campbell, D. G., Ibrahim, A., and Van Aalten, D. M. F. (2012). O-GlcNAcylation of TAB1 modulates TAK1-mediated cytokine release. EMBO J. 31, 1394–1404. doi: 10.1038/emboj.2012.8
Perkins, N. D. (2006). Post-translational modifications regulating the activity and function of the nuclear factor kappa B pathway. Oncogene 25, 6717–6730. doi: 10.1038/sj.onc.1209937
Pflueger, D., Terry, S., Sboner, A., Habegger, L., Esgueva, R., Lin, P. C., et al. (2011). Discovery of non-ETS gene fusions in human prostate cancer using next-generation RNA sequencing. Genome Res. 21, 56–67. doi: 10.1101/gr.110684.110
Phoomak, C., Vaeteewoottacharn, K., Sawanyawisuth, K., Seubwai, W., Wongkham, C., Silsirivanit, A., et al. (2016). Mechanistic insights of O-GlcNAcylation that promote progression of cholangiocarcinoma cells via nuclear translocation of NF-κB. Sci. Rep. 6:27853. doi: 10.1038/srep27853
Pikarsky, E., Porat, R. M., Stein, I., Abramovitch, R., Amit, S., Kasem, S., et al. (2004). NF-κB functions as a tumour promoter in inflammation-associated cancer. Nature 431, 461–466. doi: 10.1038/nature02924
Rajagopalan, K. N., and DeBerardinis, R. J. (2011). Role of glutamine in cancer: therapeutic and imaging implications. J. Nucl. Med. 52, 1005–1008. doi: 10.2967/jnumed.110.084244
Ramakrishnan, P., Clark, P. M., Mason, D. E., Peters, E. C., Hsieh-wilson, L. C., Baltimore, D., et al. (2013). Activation of the transcriptional function of the NF-kB protein c-Rel by O-GlcNAc glycosylation. Sci. Signal. 6:ra75. doi: 10.1126/scisignal.2004097
Sekine, H., Okazaki, K., Kato, K., Alam, M. M., Shima, H., Katsuoka, F., et al. (2018). O -GlcNAcylation signal mediates proteasome inhibitor resistance in cancer cells by stabilizing NRF1. Mol. Cell. Biol. 38:e00252-18. doi: 10.1128/mcb.00252-18
Shafi, R., Iyer, S. P. N., Ellies, L. G., O’Donnell, N., Marek, K. W., Chui, D., et al. (2000). The O-GlcNAc transferase gene resides on the X chromosome and is essential for embryonic stem cell viability and mouse ontogeny. Proc. Natl. Acad. Sci. U.S.A. 97, 5735–5739. doi: 10.1073/pnas.100471497
Shi, Y., Tomic, J., Wen, F., Shaha, S., Bahlo, A., Harrison, R., et al. (2010). Aberrant O-GlcNAcylation characterizes chronic lymphocytic leukemia. Leukemia 24, 1588–1598. doi: 10.1038/leu.2010.152
Sohma, I., Fujiwara, Y., Sugita, Y., Yoshioka, A., Shirakawa, M., Moon, J. H., et al. (2011). Parthenolide, an NF-κB inhibitor, suppresses tumor growth and enhances response to chemotherapy in gastric cancer. Cancer Genomics Proteomics 8, 39–47.
Takaesu, G., Kishida, S., Hiyama, A., Yamaguchi, K., Shibuya, H., Irie, K., et al. (2000). TAB2, a novel adaptor protein, mediates activation of TAK1 MAPKKK by linking TAK1 to TRAF6 in the IL-1 signal transduction pathway. Mol. Cell 5, 649–658. doi: 10.1016/S1097-2765(00)80244-0
Takeuchi, T., Nagasaka, M., Shimizu, M., Tamura, M., and Arata, Y. (2016). N-acetylglucosamine suppresses osteoclastogenesis in part through the promotion of O-GlcNAcylation. Bone Rep. 3, 15–21. doi: 10.1016/j.bonr.2016.02.001
Tao, T., He, Z., Shao, Z., and Lu, H. (2016). TAB3 O-GlcNAcylation promotes metastasis of triple negative breast cancer. Oncotarget 7, 22807–22818. doi: 10.18632/oncotarget.8182
Taparra, K., Wang, H., Malek, R., Lafargue, A., Barbhuiya, M. A., Wang, X., et al. (2018). O-GlcNAcylation is required for mutant KRAS-induced lung tumorigenesis. J. Clin. Invest. 128, 4924–4937. doi: 10.1172/JCI94844
Teicher, B. A., Ara, G., Herbst, R., Palombella, V. J., and Adams, J. (1999). The proteasome inhibitor PS-341 in cancer therapy. Clin. Cancer Res. 5, 2638–2645. doi: 10.1158/1078-0432.CCR-14-2549
Toleman, C., Paterson, A. J., Whisenhunt, T. R., and Kudlow, J. E. (2004). Characterization of the histone acetyltransferase (HAT) domain of a bifunctional protein with activable O-GlcNAcase and HAT activities. J. Biol. Chem. 279, 53665–53673. doi: 10.1074/jbc.M410406200
Torres, C. R., and Hart, G. W. (1984). Topography and polypeptide distribution of terminal N-acetylglucosamine residues on the surfaces of intact lymphocytes. Evidence for O-linked GlcNAc. J. Biol. Chem. 259, 3308–3317. doi: 10.1016/s0021-9258(17)43295-9
Wang, Z., Gucek, M., and Hart, G. W. (2008). Cross-talk between GlcNAcylation and phosphorylation: site-specific phosphorylation dynamics in response to globally elevated O-GlcNAc. Proc. Natl. Acad. Sci. U.S.A. 105, 13793–13798. doi: 10.1073/pnas.0806216105
Wang, Z., Pandey, A., and Hart, G. W. (2007). Dynamic interplay between O-linked N-acetylglucosaminylation and glycogen synthase kinase-3-dependent phosphorylation. Mol. Cell. Proteomics 6, 1365–1379. doi: 10.1074/mcp.M600453-MCP200
Wang, Z., Udeshi, N. D., Slawson, C., Compton, P. D., Sakabe, K., Cheung, W. D., et al. (2010). Extensive crosstalk between O-GlcNAcylation and phosphorylation regulates cytokinesis. Sci. Signal. 3:ra2. doi: 10.1126/scisignal.2000526
Wu, D., Wu, P., Zhao, L., Huang, L., Zhang, Z., Zhao, S., et al. (2015). NF-κB expression and outcomes in solid tumors: a systematic review and meta-analysis. Medicine 94:e1687. doi: 10.1097/MD.0000000000001687
Xia, Y., Shen, S., and Verma, I. M. (2014). NF-κB, an active player in human cancers. Cancer Immunol. Res. 2, 823–830. doi: 10.1158/2326-6066.CIR-14-0112
Xing, D., Gong, K., Feng, W., Nozell, S. E., Chen, Y. F., Chatham, J. C., et al. (2011). O-GlcNAc modification of nfκb p65 inhibits tnf-α-induced inflammatory mediator expression in rat aortic smooth muscle cells. PLoS One 6:e24021. doi: 10.1371/journal.pone.0024021
Yang, W. H., Park, S. Y., Nam, H. W., Kim, D. H., Kang, J. G., Kang, E. S., et al. (2008). NFκB activation is associated with its O-GlcNAcylation state under hyperglycemic conditions. Proc. Natl. Acad. Sci. U.S.A. 105, 17345–17350. doi: 10.1073/pnas.0806198105
Yang, Y. R., Kim, D. H., Seo, Y. K., Park, D., Jang, H. J., Choi, S. Y., et al. (2015). Elevated O-GlcNAcylation promotes colonic inflammation and tumorigenesis by modulating NF-κB signaling. Oncotarget 6, 12529–12542. doi: 10.18632/oncotarget.3725
Yang, Y. R., Song, M., Lee, H., Jeon, Y., Choi, E. J., Jang, H. J., et al. (2012). O-GlcNAcase is essential for embryonic development and maintenance of genomic stability. Aging Cell 11, 439–448. doi: 10.1111/j.1474-9726.2012.00801.x
Zhang, B., Zhou, P., Li, X., Shi, Q., Li, D., and Ju, X. (2017). Bitterness in sugar: O-GlcNAcylation aggravates pre-B acute lymphocytic leukemia through glycolysis via the PI3K/Akt/c-Myc pathway. Am. J. Cancer Res. 7, 1337–1349.
Zhang, D., Cai, Y., Chen, M., Gao, L., Shen, Y., and Huang, Z. (2015). OGT-mediated O-GlcNAcylation promotes NF-κB activation and inflammation in acute pancreatitis. Inflamm. Res. 64, 943–952. doi: 10.1007/s00011-015-0877-y
Zhu, Q., Zhou, L., Yang, Z., Lai, M., Xie, H., Wu, L., et al. (2012). O-GlcNAcylation plays a role in tumor recurrence of hepatocellular carcinoma following liver transplantation. Med. Oncol. 29, 985–993. doi: 10.1007/s12032-011-9912-1
Zou, L., Yang, S., Champattanachai, V., Hu, S., Chaudry, I. H., Marchase, R. B., et al. (2009). Glucosamine improves cardiac function following trauma-hemorrhage by increased protein O-GlcNAcylation and attenuation of NF-κB signaling. Am. J. Physiol. Heart Circ. Physiol. 296, H515–H523. doi: 10.1152/ajpheart.01025.2008
Keywords: inflammation, cancer, posttranslational modifications (PTMs), O-GlcNAcylation, NF-kappaB (NF-κB), leukemia, hexosamine biosynthetic pathway
Citation: Liu AR and Ramakrishnan P (2021) Regulation of Nuclear Factor-kappaB Function by O-GlcNAcylation in Inflammation and Cancer. Front. Cell Dev. Biol. 9:751761. doi: 10.3389/fcell.2021.751761
Received: 02 August 2021; Accepted: 23 September 2021;
Published: 15 October 2021.
Edited by:
Soumen Basak, National Institute of Immunology (NII), IndiaReviewed by:
Srinivasa-Gopalan Sampathkumar, National Institute of Immunology (NII), IndiaMichael May, University of Pennsylvania, United States
Copyright © 2021 Liu and Ramakrishnan. This is an open-access article distributed under the terms of the Creative Commons Attribution License (CC BY). The use, distribution or reproduction in other forums is permitted, provided the original author(s) and the copyright owner(s) are credited and that the original publication in this journal is cited, in accordance with accepted academic practice. No use, distribution or reproduction is permitted which does not comply with these terms.
*Correspondence: Parameswaran Ramakrishnan, pxr150@case.edu