- 1School of Public Health, Shandong First Medical University and Shandong Academy of Medical Sciences, Taian, China
- 2Department of Clinical Laboratory, The Second Hospital of Shandong University, Jinan, China
- 3Department of Hematology, Jining First People’s Hospital, Jining, China
- 4Department of Neurosurgery, Qilu Hospital of Shandong University and Institute of Brain and Brain-Inspired Science, Shandong University, Jinan, China
- 5Key Laboratory of Brain Function Remodeling, Qilu Hospital of Shandong University, Jinan, China
Long non-coding RNAs (lncRNAs) are a type of non-coding RNAs that act as molecular fingerprints and modulators of many pathophysiological processes, particularly in cancer. Specifically, lncRNAs can be involved in the pathogenesis and progression of brain tumors, affecting stemness/differentiation, replication, invasion, survival, DNA damage response, and chromatin dynamics. Furthermore, the aberrations in the expressions of these transcripts can promote treatment resistance, leading to tumor recurrence. The development of next-generation sequencing technologies and the creation of lncRNA-specific microarrays have boosted the study of lncRNA etiology. Cerebrospinal fluid (CSF) directly mirrors the biological fluid of biochemical processes in the brain. It can be enriched for small molecules, peptides, or proteins released by the neurons of the central nervous system (CNS) or immune cells. Therefore, strategies that identify and target CSF lncRNAs may be attractive as early diagnostic and therapeutic options. In this review, we have reviewed the studies on CSF lncRNAs in the context of brain tumor pathogenesis and progression and discuss their potential as biomarkers and therapeutic targets.
Background
Brain tumors refer to the primary intracranial tumors as well as the metastatic tumors in the brain with a primary lesion. They account for 1.8% of newly diagnosed cancers and 2.3% of cancer-related deaths worldwide (Gerlinger et al., 2012; Xie et al., 2014; Hodges et al., 2017; Siegel et al., 2017). Patients often suffer from symptoms due to increased intracranial pressure (headache, nausea, and vomiting) and neurological dysfunction (seizures, hemiplegia, aphasia, and cognitive deficits) (Cahill et al., 2012; Hadidchi et al., 2019). The natural disease progression in these patients is characterized by a progressive neurological loss and a rapid decline in the quality of the patients’ lives.
While considerable advancements in fundamental scientific research and clinical practice have shed light on the brain tumor pathophysiology in the past decades, challenges remain in precise and early diagnosis (Sawaya et al., 1998; Wang and Bettegowda, 2017; Nevel et al., 2018). For instance, computed tomography (CT) (Lebanony et al., 2009) and magnetic resonance imaging (MRI) are reliable in determining the spatial and structural characteristics for brain tumors; however, these non-invasive approaches can hardly determine the pathological classification and malignant degree of tumors (Cao et al., 2017; Huang et al., 2019); Surgical biopsy can overcome these limitations by extracting brain tissue samples, while it is much more invasive, expensive, and risky. Added to the difficulties associated with accessing the nature of brain tumors, there are currently limited therapeutic options available nowadays, including (Galldiks et al., 2017; Laudicella et al., 2021) resection surgeries, chemotherapy, radiotherapy, and electric field therapy (Uddin et al., 2020; López Vázquez et al., 2021). Unfortunately, total removal of the target lesion cannot always be achieved while minimizing the risk of any postoperative functional decline attributable to the surgical procedure itself (Martinez-Rios et al., 2016; Duffau, 2017). Certain brain malignancies are also prone to drive tumor progression and resist chemo- and radiotherapy. Therefore, the outcome of patients with brain tumors is still miserable. The 5- and 10-year survival rates for central nervous system (CNS) tumors are about 36% and 31%, respectively. For the patients with newly diagnosed glioblastoma, the most malignant form of brain tumor, the median survival duration does not exceed 2 years (Martinez-Rios et al., 2016; Duffau, 2017; Jeon et al., 2021; Rankin-Turner et al., 2021; Reddy et al., 2021).
In this scenario, it is essential to develop new methodologies to determine the diagnostic and prognostic parameters for various brain tumors and guide the individualized therapy (Horbinski et al., 2019; Kristensen et al., 2019). Non-coding RNAs (ncRNAs), especially long non-coding RNAs (lncRNAs), have emerged as a novel family of master regulators, because they are widely involved in the development and progression of various CNS disorders, including brain tumors (Kang et al., 2011; Hawrylycz et al., 2012; Chen and Qin, 2015; Hanan et al., 2017). Also, ncRNA-based tumor liquid biopsy has been demonstrated recently at the preclinical level, which detects tumor-specific ncRNA in a less invasive manner from certain body fluids, including serum, urine, and cerebrospinal fluid (CSF). Compared to serum and urine, brain tumor associated ncRNAs are much enriched in the CSF. Therefore, CSF has been considered a promising candidate for brain liquid biopsy. In fact, dysregulation of CSF lncRNAs has been demonstrated in multiple tumors and non-tumor nervous system disorders, including Alzheimer’s disease (Zhuang et al., 2020), cerebral vasospasm secondary to subarachnoid haemorrhage (Pan et al., 2020), and cerebral ischemia-reperfusion injury (Zhang Y. et al., 2020). In this review, we focus on the regulatory mechanism of CSF lncRNAs in the pathophysiology of brain tumors and discuss its potential application as diagnostic markers and therapeutic targets.
Functions and Characteristics of Cerebrospinal Fluid
The main limitation of blood biopsy for brain tumors is the low serum levels of tumor specific biomarkers, mainly caused by the brain blood barrier (BBB), which is formed by brain microvascular endothelial cells sealed by tight junctions (Banks, 2019). The BBB maintains the independent circulation of CSF and microenvironment homeostasis for the brain tissue (Sweeney et al., 2019). However, the BBB also brings significant challenges to diagnosis and treat brain tumors (Jeon et al., 2021; Pottoo et al., 2021). For instance, most tumor-specific antigens are confined within this barrier, and their systematic detection can be problematic. In addition, the poor BBB penetration naturally hinders the delivery of therapeutic drugs (such as chemotherapeutic drugs, targeted therapeutic drugs, and monoclonal antibodies) into the brain (Seo et al., 2020; Zhang et al., 2021). Although researchers have been developing strategies to modulate BBB permeability, most approaches are difficult to apply in the clinical setting (Sprowls et al., 2019; Pandit et al., 2020). Under these circumstances, CSF biopsy and CSF-based therapeutics have been gradually recognized as an alternative approach to overcome these obstacles.
The CSF is an ultrafiltrate of plasma surrounding the brain and spinal cord (Parnetti et al., 2019). The majority of CSF is produced by the choroid plexus, circulates through the ventricles, the cisterns, and the subarachnoid space to be absorbed into the blood by the arachnoid villi (Johanson et al., 2008). The CSF provides biological and mechanical support to the brain, transports nutrients, signaling molecules, and debris, and regulates brain immunity (Orešković, 2015; Tumani et al., 2017; Attier-Zmudka et al., 2019). Therefore, homeostasis in the production, circulation, and absorption of the CSF is critical for brain function (Proulx, 2021). Analogously, abnormal CSF mirrors the dysregulation of brain in various neurological diseases (Figure 1), mainly because CSF is more closely associated with small molecules, peptides, or proteins released from the brain tissues (Johanson et al., 2008; Sakka et al., 2011). Since CSF directly mirrors the biochemical processes in the brain, CSF components are widely used to identify pathogen invasion or diagnosis of neurological diseases (Figure 1). Lundborg et al. (2010) reported that glial cell line-derived neurotrophic factor (GDNF) was increased in the CSF of patients with long-term pain but decreased in the blood. Tumor-specific biomarkers are also enriched in the CSF. For instance, the levels of miR-10b and miR-21 are found significantly increased in the CSF of patients with glioblastoma and brain metastasis of breast and lung cancer, compared with tumors in remission and a variety of non-neoplastic conditions (Teplyuk et al., 2012). Similarly, significantly higher CSF levels of miR-21, miR-19b, and miR-92a were identified in primary CNS lymphoma (PCNSL) (Zajdel et al., 2019). Recently, it was reported that immune cell profiling of the CSF enabled the characterization of the brain metastasis microenvironment (Rubio-Perez et al., 2021).
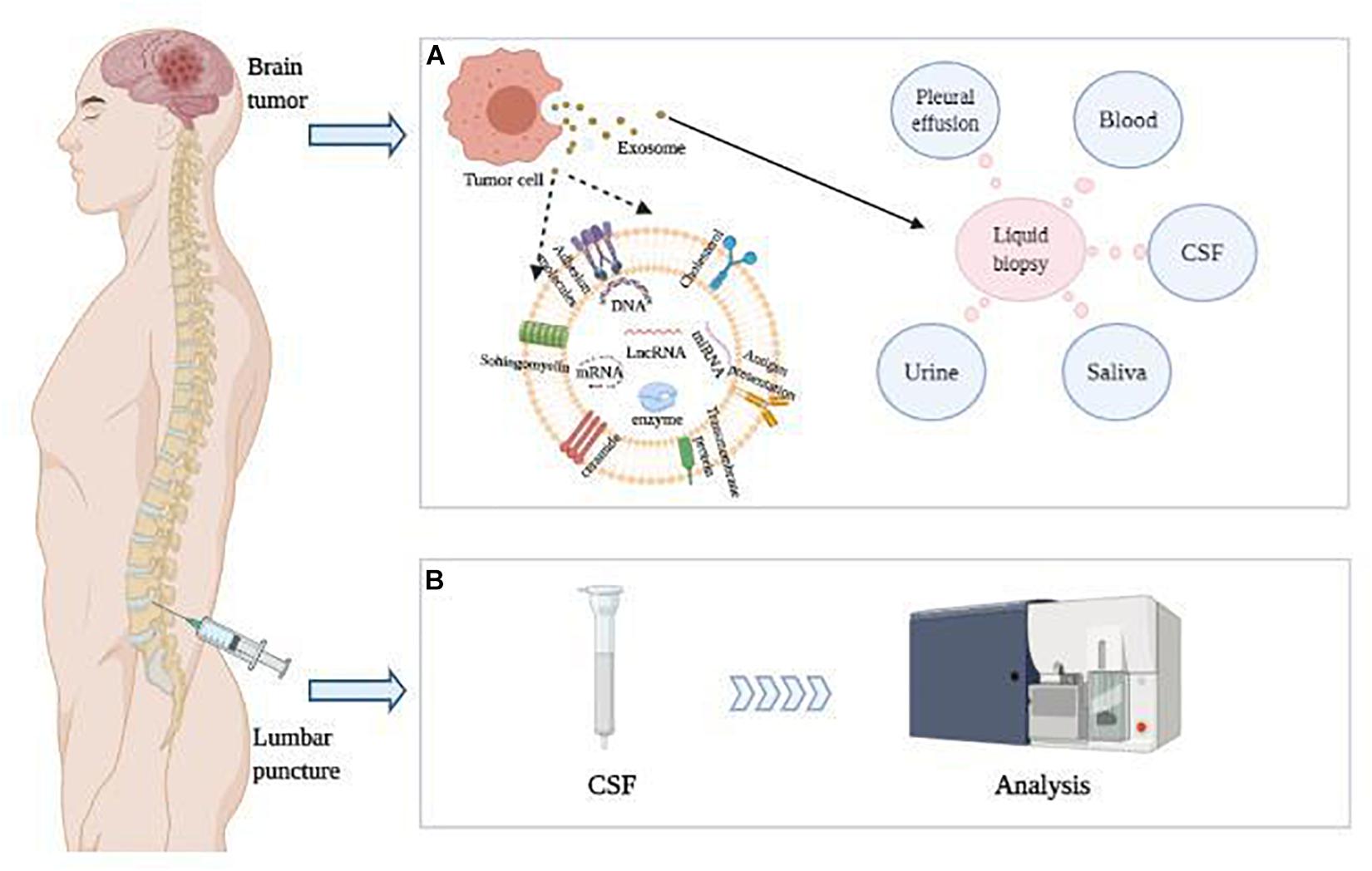
Figure 1. LncRNA in CSF were detected as diagnostic and prognostic indicators of brain tumors. (A) Brain tumor cells release exosomes containing nucleic acids, which can be further detected in various body fluids. (B) CSF was obtained by lumbar puncture, and CSF lncRNAs packaged in exosomes were detected as diagnostic and prognostic indicators of brain tumors.
Long Non-Coding RNA in the Cerebrospinal Fluid
LncRNAs, of which length ≥ 200 bp, exhibit a wide range of regulatory activities based on their subcellular localization, including gene transcriptional regulation and mRNA splicing in the nucleus, as well as mRNA stability and protein function modulation in the cytoplasm (Figure 2; Ramanathan et al., 2019; Yao et al., 2019). Dysregulation of lncRNAs has been demonstrated to contribute to cancer development and progression via abnormal epigenetic alterations in oncogene regulation pathways., including abnormal DNA methylation or histone changes at their gene promoters. More specifically, emerging data suggest that lncRNAs comprise a network of epigenetic modulators by creating platforms for chromatin-remodeling complexes and transcription factors capable of modulating the transcriptional state of lncRNA-controlled genomic loci (Romani et al., 2018; Chen et al., 2021).
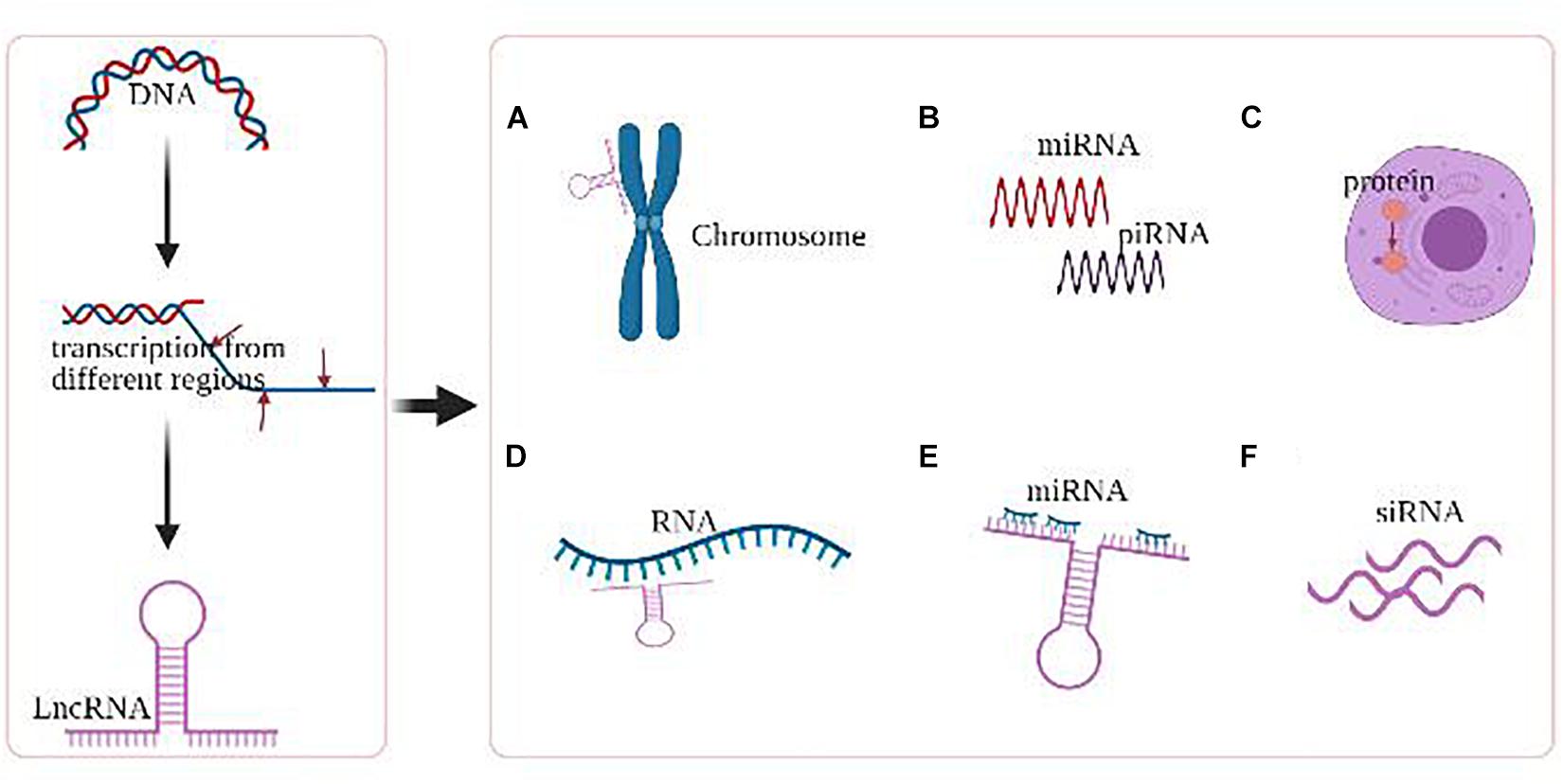
Figure 2. Major epigenetic regulations of lncRNA. (A) LncRNAs mediate chromosomal remodeling. (B) LncRNAs act as small RNA (miRNA, piRNA) precursor. (C) LncRNAs alter cellular localization of proteins. (D) LncRNAs involve in the transcriptional regulation of genes. (E) LncRNAs act as miRNA sponge. (F) LncRNAs produce endogenous siRNAs with the involvement of the dicer enzyme.
Like the other ncRNAs, lncRNAs are selectively packaged, secreted, and transferred between cells by exosomes, which are small bilipid layer enclosed extracellular nano-vesicles with various physiological and pathophysiological functions (Sullivan et al., 2017; Raposo and Stahl, 2019). With exosomes as vectors, lncRNAs are able to cross the BBB and readily accessible in CSF, making it an ideal diagnostic and therapeutic candidate for multiple diseases in the CNS, such as neurodegenerative disorders, stroke, multiple sclerosis, and brain tumors (Cheng et al., 2020; Rastogi et al., 2021). For instance, lncRNAs MALAT1 and SNHG4 are downregulated in the CSF samples of patients with Alzheimer’s disease and acute cerebral infarction, respectively (Zhang S. et al., 2020; Zhuang et al., 2020). lncRNA HIF1-AS3 transcriptomic downregulation in human choroid plexus tissue and abnormal PAI-1 level in CSF were observed in patients with progressive multiple sclerosis (Rodríguez-Lorenzo et al., 2020). Intriguingly, Pan et al. (2020) established a CSF lncRNA-based signature (ZFAS1, MALAT1, LINC00261, and LINC01619) to predictive cerebral vasospasm in patients with subarachnoid hemorrhage.
Abundant evidence has revealed that CSF lncRNAs were also extensively expressed in different brain tumors and involved in tumorigenesis, tumor progression, invasion, angiogenesis, and metastasis. In 2017, Ma et al. (2017) firstly observed that lncRNA HOTAIR derived from glioma cells promotes angiogenesis by regulating the endothelial VEGF expression. Similar to Ma’s observation, Bian et al. (2019) found that exosomal lncRNA-ATB triggered astrocytes to facilitate the glioma invasion. Moreover, Zhang et al. demonstrated that that lncRNA SBF2-AS1 was upregulated in chemotherapy-resistant glioblastoma (GBM), and overexpression of SBF2-AS1 led to the promotion of chemotherapy resistance, which was regulated by transcription factor ZEB1. ZEB1 directly binds to the SBF2-AS1 promoter region to regulate SBF2-AS1 level and affected temozolomide (TMZ) resistance in GBM cells (Zhang Z. et al., 2019). Recently, Li D. et al. (2021) demonstrated that the expression of CSF lncRNA-CCRR was evidently up-regulated in breast cancer metastasis patients, especially in patients with brain metastasis, which provides a direct piece of evidence to demonstrate the dysregulation of CSF lncRNA in brain cancers. On the other hand, Wang M. et al. (2020) found that exosomal LGALS9 in glioblastoma CSF suppressed dendritic cell antigen presentation and cytotoxic T-cell immunity, while blocking the secretion of exosomal LGALS9 could regain sustained tumor antigen-presenting activity of dendritic cells and long-lasting antitumor immunity. Together, CSF lncRNAs have exhibited the significance as promising biomarkers and potential targets for various brain tumors.
Long Non-Coding RNAs Implications in Various Brain Tumors
Glioma
As the most prevalent form of primary brain tumors, glioma develops from neural glial cells, mainly star-shaped astrocytes. Based on the classical WHO tumor classification, glioma can be subgrouped into Grade 1–4 (Louis et al., 2016). Despite highly variable histological and genetic characteristics, glioma is notorious for its rapid proliferation, extensive invasion, genetic heterogeneity, and therapeutic resistance. Even given the multiple discipline therapeutics, patients with glioma suffer from the dismal outcomes. Emerging evidence suggests that lncRNAs play a vital role in mediating glioma initiation and progression. Here, we highlighted the current research focusing on the implications of lncRNA on glioblastoma and LGG, respectively (Figure 3).
Glioblastoma
Glioblastoma (GBM, WHO Grade 4) is the most devastating type of primary brain cancer, accounting for more than half of all primary CNS tumors (Ostrom et al., 2019). In 2016, WHO classified glioblastoma into IDH (isocitrate dehydrogenase) wild-type, IDH mutant, and NOS groups (Louis et al., 2016). In the recently updated WHO classification, glioblastoma is further defined as IDH wild-type adult-type diffuse gliomas (Grade 4), with the iconic molecular profiling including TERT promoter mutation, gain of chromosome 7 and loss of chromosome 10, and EGFR amplification (Louis et al., 2021). Glioblastoma diffusely and rapidly grows to infiltrate the white matter tract and eloquent cortex, making it extremely difficult to achieve early diagnosis and maximal safety resection. Despite the optimal therapeutic approaches combined with surgical resection, targeted radiotherapy, high-dose chemotherapy as well as novel electric field treatment, the median overall survival (OS) of glioblastoma is still less than 21 months, and the 5-year survival rate is between 1–19% (Alexander and Cloughesy, 2017).
In 2019, Yang et al. (2019) reported that glioma stem cell (GSC)-derived lncRNA MALAT1 was transferred to surrounding microglia through exosomal secretion, thereby regulating the inflammatory response through the miR-1295p/HMGB1 axis to affect the secretion of IL-6, IL-8, and TNF-α. This study indicated that exosomal lncRNA played a significant role in maintaining immunosuppressive microenvironment for glioma survival and invasiveness (Yang et al., 2019). Han et al. (2016) demonstrated that lncRNA MALAT1 also mediated anti-glioma effect by suppressing the activation of extracellular regulated protein kinases/mitogen-activated protein kinase (ERK/MAPK) signaling pathway and expression of matrix metalloproteinase 2 (MMP2). Similarly, lncRNA SNHG12, a sponge of miR-129-5p, endows the glioblastoma cells with TMZ resistance by upregulating MAPK1 and activating the MAPK/ERK pathway. Clinically, SNHG12 overexpression was associated with the poor survival of patients treated with TMZ (Lu et al., 2020). Moreover, Tian et al. (2019) found that patients with glioblastoma having high expression of LncRNA AGAP2-AS1 had shorter overall survival time than those with low expression of AGAP2-AS1. The loss-of-function studies showed that downregulation of AGAP2-AS1 depressed cell proliferation, migration, and invasion, and promoted cell apoptosis in glioblastoma. Therefore, AGAP2-AS1 might serve as an oncogenic lncRNA and prognostic biomarker in glioblastoma (Tian et al., 2019). Han et al. (2020) reported that MIR22HG was a critical inducer of the Wnt/β-catenin signaling pathway for glioblastoma, and a specific small-molecule inhibitor, AC1L6JTK, could cause the inhibition of tumor growth in vivo. Interestingly, MIR22HG was also enlisted in an immune-related lncRNA signature associated with glioblastoma prognosis, indicating its pathophysiological complexity in this malignancy (Li X. et al., 2021). Together, abundant evidence has revealed that lncRNAs play critical roles in multiple aspects of glioblastoma biology.
Low Grade Glioma
In the recently updated WHO classification, the term “anaplastic” was not routinely included; therefore, familiar names like “anaplastic astrocytoma” and “anaplastic oligodendroglioma” were abandoned. Under this circumstance, LGG is now designated as WHO grade 2 astrocytoma and oligodendroglioma, accounting for approximately 5–10% of all CNS tumors (Ostrom et al., 2016). The classical low grade astrocytoma is IDH-mutant, with alternations of ATRX, TP53, and CDKN2A/B. Chromosome 1p19q codeletion is the gold standard characteristics to distinguish oligodendroglioma apart from astrocytoma, along with the alternations of TERT promoter, CIC, FUBP1, and NOTCH1 (Louis et al., 2021). Compared to glioblastoma, LGG has highly variable clinical behavior (Brat et al., 2015). Patients with certain subtype of low grade glioma can survival for decades, while some others progress to secondary glioblastoma within months (Gusyatiner and Hegi, 2018). To improve the diagnostic accuracy and optimize treatment selection, numerous attempts have been made, from the classical histologic characterization to novel molecular profiling, including lncRNA. Nevertheless, considerable controversies remain nowadays. Meanwhile, maximum safety resection might not always be feasible due to the diffuse invasion nature of LGG, and radiotherapy and chemotherapy are alternatively recommended (Franz et al., 2013, 2014).
Growing evidence demonstrates the regulatory role of lncRNA in LGG biology. For example, PR-lncRNA refers to the lncRNAs regulated by P53. In vitro experiments of glioma tissues and cell lines revealed that PR-lncRNA expression was negatively correlated with SOX1, SOX2, and SOX9 stem cell factors. Knockdown of SOX abolished the role of PR-lncRNA silencing in glioma cell activity, indicating that the expression and function of PR-lncRNA were significantly altered in LGG formation (Torres-Bayona et al., 2018). LncRNA H19 can enhance tumorigenesis by directly inducing the c-Myc oncogene (Barsyte-Lovejoy et al., 2006). The expression level of H19 was positively correlated with glioma malignancy (Xiao et al., 2020), and H19 could affect the immune infiltration level of glioma through changes in copy number (Yao et al., 2015). As a sponge for miR-675, H19 can regulate the proliferation and migration of glioma cells by producing miR-675 to inhibit the expression of CDK6 (Shi et al., 2014; Li et al., 2016). Moreover, lncRNA LINC00174 is extensively associated with a variety of cancers, including glioblastoma and LGG. In particular, LINC00174 facilitates glycolysis and tumor growth by regulating the miR-152-3p/SLC2A1 axis and regulates the miR-138-5p/SOX9 axis to promote chemotherapeutic resistance to temozolomide in glioma (Shi et al., 2019; Li B. et al., 2020). Therefore, lncRNAs have the potential for diagnostic and prognostic biomarkers in patients with LGG.
Pituitary Adenoma
Pituitary adenomas are mostly located in the anterior lobe of the pituitary gland, which are usually slow-growing and benign. The symptoms of pituitary adenoma may include headache, optic nerve compression, and endocrine changes, including menopause, gigantism, acromegaly, and Cushing’s disease (Bronstein et al., 2011; Holmes, 2016). In 2018, Zhu et al. (2018) suggested that osteoclast differentiation in bone-invasive pituitary adenomas was directly induced by TNFα, which was further regulated by lncRNA SNHG24. Wu et al. (2018) reported that lncRNA H19 inhibited the phosphorylation of 4E-BP1 by preventing the binding of 4E-BP1 to Raptor. In contrast, the overexpression of H19 significantly inhibited the growth of pituitary tumor cells through cell membrane transport. Zhang et al. further demonstrated that the prognosis of patients with prolactinoma was closely related to the expression level of exosomal H19. In addition, the use of cabergoline could enhance the expression of H19 to exert a synergistic therapeutic effect with exosomal H19, which implies the potential of exosomal H19 in the diagnosis and treatment of pituitary tumors (Zhang Y. et al., 2019). Recently, Mao et al. (2020) reported that lncRNA SNHG6 induced the epithelial-mesenchymal transition (EMT) in pituitary adenomas by inhibiting miR-944. Simultaneously, SNHG6/miR-944/RAB11A axis regulated pituitary adenoma proliferation and invasive behavior (Mao et al., 2020).
Meningioma
Meningiomas are the most common benign intracranial tumors arising from the arachnoid cells in the meninges, accounting for 38.3% of all CNS tumors and 54.5% of non-malignant CNS tumors (Louis et al., 2016; Ostrom et al., 2020). The overall survival for patients with benign meningiomas is good, whereas the 5-year survival rate for patients with atypical or malignant meningiomas (WHO grade 2 and 3) is less than 60% (Rohringer et al., 1989). Chromosomal abnormalities at 14q32 are commonly implicated in meningioma pathogenesis and progression (Simon et al., 1995; Weber et al., 1997; Martínez-Glez et al., 2010). MEG3, an imprinted gene located at 14q32, can encode non-coding RNAs with antiproliferative functions (Ghafouri-Fard and Taheri, 2019). Ding et al. (2020) confirmed that lncRNA MEG3 mediated the invasive behavior of meningioma cells through the miR-29c/AKAP12 axis. The upregulation in miR-29c levels can eliminate the adverse effects caused by MEG3 expression on the cell cycle, migration, invasion, and proliferation of meningioma cells (Ding et al., 2020). Zhang et al. (2010) also demonstrated that MEG3 mRNA was highly expressed in normal arachnoid cells but was lost in human meningioma cells, and there was a strong association between the loss of MEG3 expression and tumor grade. Additional evidence has shown that MEG3 could induce the expression of P53, which is a tumor suppressor gene in humans; that is, MEG3 overexpression could reduce the proliferation and metastasis of gastric cancer cells (Wei and Wang, 2017). Alternatively, lncRNA SNHG1 is also associated with meningioma progression. Zhao et al. (2019) reported that SNHG1 was overexpressed in meningioma cell lines, of which deficiency restrained cell growth and accelerated apoptosis. Further mechanism experiments demonstrated that SNHG1/miR-556-5p/TCF12 feedback loop promotes proliferation and inhibits apoptosis in meningiomas through the Wnt signaling pathway (Zhang Y. et al., 2020).
Medulloblastoma
Medulloblastoma is the most common pediatric malignant brain tumor in clinical practice and accounts for 9.2% of pediatric brain tumors (Louis et al., 2007; Millard and De Braganca, 2016; Ostrom et al., 2017). It usually occurs in the lower cerebellar hilum and then metastasizes through CSF circulation (Dufour et al., 2012; Amirjamshidi, 2017). Therefore, CSF cytology has long been one of the routine tests for medulloblastoma. New WHO CNS tumor classification has altered the subgroups of medulloblastomas to mirror new knowledge of their clinical and biological heterogeneity, including 4 principal molecular groups: WNT-activated, sonic hedgehog (SHH)-activated, group 3, and group 4 (Pietsch et al., 2014). SHH is the most common in infants and adults, while other subtypes are common in children (Northcott et al., 2012a, 2017; Taylor et al., 2012). These classifications provide targets for personalized therapy, some of which are currently being tested clinically.
Exploring on the novel molecular biomarkers in medulloblastoma initiation and progression has drawn extensive attention (Ramaswamy and Taylor, 2017). Li B. et al. (2019) reported that lncRNA TP73-AS1 and EIF5A2 were upregulated in medulloblastoma, while miR-494-3p was downregulated. They identify that ELF5A2 is a direct target of miR-494-3p, and knockdown of TP73-AS1 inhibits the proliferation, invasion, and migration of medulloblastoma and promotes cell apoptosis. These findings suggest that lncRNA TP73-AS1 is involved in the development of medulloblastoma as a pro-oncogene (Li B. et al., 2019). Gao et al. (2018a) found that lncRNA LOXL1-AS1 promoted the proliferation and metastasis of medulloblastoma by activating the PI3K/AKT pathway, providing evidence that knockdown of LncRNA LOXL1-AS1 might be a potential therapeutic strategy against medulloblastoma. Moreover, Zhang J. et al. (2020) identified that the expression of lncRNA HOTAIR was higher in medulloblastoma tissues and cell lines than normal samples, which promoted tumor growth, migration, invasion, and EMT by negatively regulating miR-1 and miR-206.
Primary Central Nervous System Lymphoma
PCNSL refers to the aggressive non-Hodgkin lymphoma in the brain without systemic involvement (Olson et al., 2002; van der Sanden et al., 2002). The most common type of PCNSL is diffuse large B-cell lymphoma (DLBCL), accounting for approximately 2–3% of brain tumors (Langner-Lemercier et al., 2016; Swerdlow et al., 2016; Fox et al., 2019). PCNSL poses an extraordinary challenge to oncologists and neurosurgeons because the impermeability of the BBB hinders the delivery of common chemotherapeutic drugs to the brain (Hanjin et al., 2018). Therefore, intrathecal chemotherapy (injection of chemotherapeutic agents into the CSF via lumbar puncture and delivery of chemotherapeutic agents to the CNS via the cerebrospinal circulation) is reasonable for this malignancy (Cortelazzo et al., 2017).
Recent studies have demonstrated that lncRNAs were widely involved in the biological mechanism of DLBCL by regulating of vital downstream factors through “sponge” intracellular molecules (Huang et al., 2020). For example, lncRNA MALAT1 acts as a ceRNA sponge for miR-195 to stimulate DLBCL cell proliferation and immune escape by activating the immune checkpoint molecules PD-L1 (Wang et al., 2019). LncRNA SNHG14 can act as a ceRNA sponge for miR-5590-3p to upregulate the downstream protein zinc finger E-box binding homeobox 1 (ZEB1). Meanwhile, ZEB1 inversely promotes immune escape of DLBCL cells by transcriptionally activating SNHG14 and PD-L1 (Zhao et al., 2019). SNHG12, another member of the lncRNA SNHG family, can also promote the tumorigenesis of DLCBL by stimulating miR-195 spongiosis (Chen et al., 2020).
Brain Metastases
Brain metastases refer to the malignant tumors metastasizing to the brain, which are the most common intracranial tumors in adults. Brain metastases may occur up to 10 times more frequently than primary brain tumors (Schouten et al., 2002; Barnholtz-Sloan et al., 2004; Tominaga et al., 2015). Primarily, brain metastases are located in the cerebral hemispheres (80%); the rest were found in the cerebellum and brainstem (Eichler and Loeffler, 2007; Suh et al., 2020). Common cancers causing brain metastases include lung cancer, melanoma, breast cancer, and renal cell carcinoma (Xie et al., 2014). Primary lung cancer is the most common cancer source for brain metastases (Gould, 2018). However, the mechanism underlying the metastatic procedure remains poorly understood. In the cases with multiple metastases, surgery alone usually cannot achieve local control (Mahajan et al., 2017; Churilla et al., 2019). Although whole-brain radiation therapy is acceptable, it is associated with significant cognitive decline (Chang et al., 2009). Similarly, the efficiency of systemic chemotherapy is quite limited, mainly because of the drug resistance and poor penetration through the BBB.
Shen et al. (2015) reported that lncRNA MALAT1 promoted brain metastasis by inducing epithelial-mesenchymal transition in lung cancer, while silencing MALAT1 inhibited highly invasive metastasis cancer cell migration and metastasis by inducing EMT. Furthermore, lncRNA lnc-BM is believed a prognostic indicator for intracranial metastasis patients with breast cancer. Elevated lnc-BM expression promotes STAT3-dependent ICAM1 and CCL2, which mediates communication between breast cancer cells and microenvironment immune cells (Wang et al., 2017). Although the differences in lncRNA expression profiles between primary and metastatic cancer are still controversial, this points to the possibility of distinguishing certain types of metastatic brain tumor with CSF biopsy (Tahira et al., 2011). For instance, Li and colleagues observed the upregulation of lncRNA-CCRR in CSF of metastatic brain tumor from breast cancer.
The Potential of Cerebrospinal Fluid Long Non-Coding RNAs in the Diagnosis and Treatment of Brain Tumors
Cerebrospinal Fluid Long Non-coding RNAs as Brain Tumors Diagnostic and Prognostic Biomarkers
Clinical diagnosis of brain tumors depends on the evaluation of symptoms and signs, neuroimaging (such as CT, MRI, and PET-CT), and pathological examination of tissues as the gold standard (Di Lullo and Kriegstein, 2017). In this era of precision medicine, non-invasive neuroimaging can hardly provide the necessary molecular profiling of brain tumors or prognostic information. On the other hand, serial samples of brain tumors are difficult to obtain, and, therefore, tracking tumor progression is complex. Moreover, surgical biopsy of the brain tissue is challenging because of the tumor location and hemorrhagic risk. Alternatively, brain tumor biomarkers acquired from the body fluid, including circulating tumor cells, cell-free DNA, and exosomal ncRNAs, have drawn extensive attention to overcome these limitations (Mattox et al., 2019; Le Rhun et al., 2020).
A cancer biomarker can be a substance naturally produced by a tumor or the body’s unique reaction to the presence of diseases (Ariston Gabriel et al., 2020; Simonato et al., 2021). Regarding brain tumors, the presence of BBB hinders the transport of nucleic acids and proteins and dramatically decreases the concentration of tumor biomarkers in the peripheral blood of patients (Antonetti et al., 2021). In contrast, CSF is more closely associated with brain tissue than serum and can be enriched for tumor specific biomarkers (Killer, 2013; Parnetti et al., 2019; Gaetani et al., 2020). LncRNAs in the CSF, therefore, are supposed to be potential candidates as sensitive and accurate early diagnostic and prognostic tools for various brain tumors (Latowska et al., 2020).
An increasing number of studies have evidenced the key roles of lncRNAs in regulating cell proliferation, apoptosis, GSC self-renewal, differentiation, and response to hypoxic stress of different brain tumors. A previous study by Jing et al. (2016) examined the expression of lncRNA CRNDE in 164 gliomas and neighboring non-tumor tissues. Overexpression of CRNDE was correlated with a higher WHO grade, recurrence, and tumor volume expansion in tumor tissues; therefore, elevated expression of this lncRNA may be considered a new prognostic marker in glioma. Similarly, lncRNA HOTAIR has been widely discussed regarding glioma biology. For instance, Suppressing HOTAIR expression inhibits glioma cell proliferation, migration, and invasion, which involves the PI3K/AKT signaling pathway (Ke et al., 2015). Therefore, this lncRNA could be considered a novel prognostic and diagnostic biomarker for glioblastoma (Tan et al., 2018). Furthermore, the expression of lncRNA miR210HG was substantially upregulated in tumor tissue than adjacent normal tissue. Patients with glioma exhibited substantially higher serum miR210HG level than healthy controls, indicating this lncRNA is a potential diagnostic biomarker for glioma (Min et al., 2016). Considering the complicated biological effects and interactions of lncRNAs, the multiple-lncRNA signatures could be better diagnostic and prognostic indicators rather than single lncRNAs. Zhang et al. (2012) suggested that several lncRNAs could be used to distinguish between the stage and type of glioma. This study indicated that approximately 129 lncRNAs were differentially expressed between gliomas and normal brain tissue, demonstrating the capacity of lncRNAs in tumor stratification. Serial studies focused on medulloblastoma have also shown that a variety of lncRNAs, including CCAT1, CRNDE, Linc-NeD125, and PVT1, were associated with tumor progression (Northcott et al., 2012b; Song et al., 2016; Laneve et al., 2017; Gao et al., 2018b). Among these lncRNAs, Linc-NeD125 was overexpressed in medulloblastoma tissues compared to normal brain tissues; further studies showed that its ectopic expression promoted cell proliferation, migration, and invasion of medulloblastoma cells in vitro (Laneve et al., 2017). Interestingly, in a recent report from Li and colleagues, tissue expression and CSF expressions of lncRNA CCRR were both evidently upregulated in breast cancer patients with brain metastases. However, the upregulation of serum lncRNA level was not documented, indicating that CSF lncRNAs might be better biomarkers for intracranial tumors (Li D. et al., 2021). Together, the facts mentioned above indicate that CSF lncRNAs have great potential in diagnosing and predicting brain tumors and provide new approaches for the individualized treatment of patients. More information on lncRNAs as biomarkers for brain tumors is summarized in Table 1.
Although CSF lncRNAs is difficult to be used as a routine screening nowadays, it has exhibited several advantages. First, serial CSF lncRNAs can be acquired by lumber puncture, to monitor the progression in a micro-invasive and dynamic way. Second, CSF lncRNAs are directly secreted and confined within the CNS to eliminate the systemic factors, which helps us to understand the biology and pathophysiology of brain tumors. Third, multiple lncRNA microarray would further improve diagnostic accuracy such as sensitivity and specificity. Fourth, the lowest MRI resolution ranges in the order of millimeters, whereas the dimensions of the tumor cell are in micrometers. Such disparity in scale may lead to delay in diagnosis, which can be compromised by CSF lncRNA biopsy. Finally, lncRNA can be combined with the current neuroimage, rather than replace it. For instance, Wu et al. (2021) observed that lncRNA CASC19 promoted glioma progression by modulating the miR-454-3p/RAB5A axis, which was associated with unfavorable MRI features. Similarly, lncRNA SAMMSON overexpression help distinguishing patients with glioblastoma from diffuse neurosarcoidosis, which shares quite similar radiological features (Xie et al., 2019).
Cerebrospinal Fluid Long Non-coding RNA as Brain Tumor Therapeutic Agents
Although conventional strategies for brain tumor treatment have been shown to be promising, it remains a considerable challenge to improve the outcomes of patients. For instance, even given the multidiscipline approaches combined with surgical resection, targeted radiotherapy, high-dose chemotherapy, and novel electric field treatment, the median overall survival for glioblastoma is still less than 21 months (Tan et al., 2020). One of the major obstacles is the poor penetration of BBB. Many attempts have been made to deliver drugs efficiently through BBB (Allhenn et al., 2012), including the intrathecal administration (drug injection to the lumbar arachnoid space) and intraventricular administration (drug injection or infusion into the lateral ventricles of the brain, Calias et al., 2014). Kim et al. (2016) evaluated the efficiency of different anti-miR delivery strategies, including intratumoral, intrathecal, and intraventricular routes, in an orthotopic model of GBM. Intraventricular injection of anti-Let-7 resulted in a significant reduction in target gene expression in the whole tumor, indicating a promising approach for ncRNA therapy in brain tumors (Kim et al., 2016). More recently, Donovan et al. (2020) demonstrated that administration of chimeric antigen receptor T (CAR-T) cells into the CSF could be a highly effective therapy for multiple metastatic mouse models of medulloblastoma and PFA ependymoma. Yang et al. (2019) also reported that intrathecal injection of umbilical cord blood mesenchymal stem cells could improve the pain through lncRNA H19/microRNA-29a-3p/FOS axis. These studies suggest that CSF delivery is practical and promising approach to fight against brain tumors. Specifically, intrathecal and intraventricular administration of lncRNAs packaged by exosomes can be evaluated as novel therapeutics for various brain tumors. For instance, Lai et al. (2014) created a sensitive extracellular vesicle system with high stability and BBB permeability.
Generally, lncRNAs participate in the multiple aspects of tumor biology, such as proliferation, invasion, angiogenesis, treatment resistance, stemness maintenance, and immune suppression. Many ongoing studies, therefore, are designed to identify the lncRNAs with potential anti-tumor characteristics, as summarized in Table 2. For instance, TMZ-based chemotherapy is the fundamental treatment for patients with glioma, especially for malignant glioma. Several lncRNAs have been found to be involved in chemoresistance to TMZ in glioma cells, including lncRNA HOTAIR, H19, and MALAT1 (Jiang et al., 2016; Zhang L. et al., 2020). Recently, Lv et al. (2020) demonstrated that high expression of lncRNA DLEU1 predicted a poor prognosis. Furthermore, silencing lncRNA DLEU1 suppressed TMZ-activated autophagy and promoted the sensitivity of glioma cells to TMZ by triggering apoptosis (Lv et al., 2020). Considering the complicated epigenetic effects and interactions of lncRNAs, single lncRNA can be involved in different tumor biological functions. LncRNA LINC00174 is extensively associated with a variety of cancers, including glioblastoma and LGGs. In particular, LINC00174 facilitates glycolysis and tumor growth by regulating the miR-152-3p/SLC2A1 axis and regulates the miR-138-5p/SOX9 axis to promote chemotherapeutic resistance to temozolomide in glioma (Shi et al., 2019; Li B. et al., 2020). It also acts as an oncogene in glioblastoma via promoting proliferative phenotype (Wang Z. et al., 2020). Besides glioma, lncRNAs can be therapeutic targets in other brain cancers. For instance, knockdown of oncogenic lncRNA CRNDE inhibited tumor development in medulloblastoma cell lines, significantly decreased cell proliferation, and increased apoptosis (Song et al., 2016). Similarly, Xing et al. (2018) indicated that lncRNA LINC00460 promoted MMP-9 expression through targeting miR-539, acting as an oncogenic RNA in the meningioma malignancy and accelerating the proliferation and metastasis of meningioma. In conclusion, these studies suggest CSF lncRNAs have shown great potential as a therapeutic target, although further effort is needed before the clinical application.
Conclusion and Prospects
Brain tumors directly threaten the cognition, behavior, and neurologic functions of human beings. While considerable advancements in fundamental scientific research and clinical practice have shed light on brain tumor pathophysiology in the past decades, challenges remain in precise and early diagnosis. LncRNAs exert critical regulatory efforts in the development and progression of different brain tumors, including glioma, meningioma, pituitary adenoma, medulloblastoma, PCNSL, and brain metastasis. Compared to the other ncRNAs, such as miRNAs and circRNAs, the regulatory mechanisms of lncRNAs seems to be more complicated. For instance, miRNAs are small ncRNAs consisting of approximately 21–25 nucleotides, which act as regulators of gene expression by complementary binding of the 3′ untranslated regions (UTR) of targeted mRNAs, thus reducing the mRNA stability or modulating gene translation. On the contrary, lncRNA might exert epigenetic functions in a more comprehensive and complex manner (as shown in Figure 2), including transcriptional regulation of genes, acting as small RNA precursors and miRNA sponges, protein localization alternation, and production of endogenous siRNAs. Also, the interactions between these ncRNAs have been described, which construct a regulatory network of brain cancer. CSF biopsy represents a novel approach to monitor the pathophysiology of brain tumors in an efficient, mini-invasive, and continuous manner. Moreover, intrathecal or intraventricular administration has been demonstrated to deliver multiple drugs and therapeutic agents efficiently through BBB. As we reviewed, CSF lncRNAs provide great promises for clinical applications, including the diagnosis and treatment of brain tumors.
Though promising, several challenges remain to be addressed. First, the trace amount of lncRNAs in the CSF brings considerable difficulties to detection and diagnosis. Nowadays, high-throughput RNA-seq technology develops rapidly, making it possible to simultaneously detect multiple tumor-specific lncRNAs to balance the sensitivity and accuracy of early diagnostics. In fact, numerous bioinformatics-based lncRNA signatures have been established with diagnostic and predictive potential. Second, the bioactivity of certain lncRNA needs to be fully elucidated due to its epigenetic effort before the clinical application. Third, directly targeting CSF lncRNAs is challenging, or even useless, because these lncRNAs are dominantly released to the CSF by tumor cells as biomarkers. To exert maximal therapeutics effort, intrathecal or intraventricular administration of therapeutic agents, such as small interfering RNA (siRNA), antisense oligonucleotide (ASO), small molecule inhibitors, or even exosome-sealed ncRNAs, should be considered to reduce the expression of tissue lncRNAs or inhibit their functions within the tumor microenvironment. Finally, CSF lncRNAs cannot be the only answer for the diagnostics and treatment of brain tumors. Synergy between lncRNAs and other oncogenic regulators has been primarily documented (Parasramka et al., 2017; Wu et al., 2020). With progressively better understanding of lncRNA regulatory mechanisms, we believe CSF lncRNAs combined with others wild become increasingly valuable agents in diagnosing and treating various brain tumors.
Author Contributions
KX and SX conceived the structure of the manuscript and revised the manuscript. KX, XJ, and AAG designed and drafted the manuscript. XJ, YW, XL, and SX discussed and revised the manuscript. All authors read and approved the final manuscript.
Funding
This paper was funded by the National Natural Science Foundation of China (81502164), the Taishan Scholarship Young Expert Program (tsqn201909174), and the Department of Science and Technology of Shandong Province (2016GSF201055).
Conflict of Interest
The authors declare that the research was conducted in the absence of any commercial or financial relationships that could be construed as a potential conflict of interest.
Publisher’s Note
All claims expressed in this article are solely those of the authors and do not necessarily represent those of their affiliated organizations, or those of the publisher, the editors and the reviewers. Any product that may be evaluated in this article, or claim that may be made by its manufacturer, is not guaranteed or endorsed by the publisher.
Acknowledgments
The figures were created with BioRender.com, and we appreciate it.
Abbreviations
lncRNA, Long non-coding RNA; CSF, Cerebrospinal fluid; CT, Computed tomography; MRI, Magnetic resonance imaging; PET, Positron emission tomography; GBM, Glioblastoma; BBB, Blood-brain barrier; CNS, Central nervous system; miRNA, microRNA; EMT, Epithelial-to-mesenchymal transition; IDH, Isocitrate dehydrogenase, HGG: High-grade glioma; EGFR, Epidermal growth factor receptor; TERT, Telomerase reverse transcriptase; ATRX, A-thalassemiamental retardation syndrome X; GSC, Glioma stem cell; LGG, Low-grade glioma; LPS, Lipopolysaccharide; MMP, Matrix metalloproteinase; TMZ, Temozolomide; PCNSL, primary CNS lymphoma; DLBCL, Diffuse large B-cell lymphoma; ZEB1, Zinc finger E-box binding homeobox 1; UTR Untranslated regions; siRNA, Small interfering RNA; ASO, Antisense oligonucleotide; ncRNA, non-coding RNA; WHO, World health organization.
References
Alexander, B. M., and Cloughesy, T. F. (2017). Adult glioblastoma. J. Clin. Oncol. 35, 2402–2409. doi: 10.1200/JCO.2017.73.0119
Allhenn, D., Boushehri, M. A., and Lamprecht, A. (2012). Drug delivery strategies for the treatment of malignant gliomas. Int. J. Pharm. 436, 299–310. doi: 10.1016/j.ijpharm.2012.06.025
Amirjamshidi, A. (2017). A case of early extraneural medulloblastoma metastases in a young adult. Asian J. Neurosurg. 12:349. doi: 10.4103/1793-5482.175647
Antonetti, D. A., Silva, P. S., and Stitt, A. W. (2021). Current understanding of the molecular and cellular pathology of diabetic retinopathy. Nat. Rev. Endocrinol. 17, 195–206. doi: 10.1038/s41574-020-00451-4
Ariston Gabriel, A. N., Wang, F., Jiao, Q., Yvette, U., Yang, X., Al-Ameri, S. A., et al. (2020). The involvement of exosomes in the diagnosis and treatment of pancreatic cancer. Mol. Cancer 19:132. doi: 10.1186/s12943-020-01245-y
Attier-Zmudka, J., Sérot, J. M., Valluy, J., Saffarini, M., Macaret, A. S., Diouf, M., et al. (2019). Decreased cerebrospinal fluid flow is associated with cognitive deficit in elderly patients. Front. Aging Neurosci. 11:87. doi: 10.3389/fnagi.2019.00087
Banks, W. A. (2019). The blood-brain barrier as an endocrine tissue. Nat. Rev. Endocrinol. 15, 444–455. doi: 10.1038/s41574-019-0213-7
Barnholtz-Sloan, J. S., Sloan, A. E., Davis, F. G., Vigneau, F. D., Lai, P., and Sawaya, R. E. (2004). Incidence proportions of brain metastases in patients diagnosed (1973 to 2001) in the Metropolitan Detroit Cancer Surveillance System. J. Clin. Oncol. 22, 2865–2872. doi: 10.1200/JCO.2004.12.149
Barsyte-Lovejoy, D., Lau, S. K., Boutros, P. C., Khosravi, F., Jurisica, I., Andrulis, I. L., et al. (2006). The c-Myc oncogene directly induces the H19 noncoding RNA by allele-specific binding to potentiate tumorigenesis. Cancer Res. 66, 5330–5337. doi: 10.1158/0008-5472.CAN-06-0037
Bian, E. B., Chen, E. F., Xu, Y. D., Yang, Z. H., Tang, F., Ma, C. C., et al. (2019). Exosomal lncRNA-ATB activates astrocytes that promote glioma cell invasion. Int. J. Oncol. 54, 713–721. doi: 10.3892/ijo.2018.4644
Brat, D. J., Verhaak, R. G., Aldape, K. D., Yung, W. K., Salama, S. R., Cooper, L. A., et al. (2015). Comprehensive, integrative genomic analysis of diffuse lower-grade gliomas. N. Engl. J. Med. 372, 2481–2498. doi: 10.1056/NEJMoa1402121
Bronstein, M. D., Paraiba, D. B., and Jallad, R. S. (2011). Management of pituitary tumors in pregnancy. Nat. Rev. Endocrinol. 7, 301–310. doi: 10.1038/nrendo.2011.38
Cahill, J., Lobiondo-Wood, G., Bergstrom, N., and Armstrong, T. (2012). Brain tumor symptoms as antecedents to uncertainty: an integrative review. J. Nurs. Scholarsh. 44, 145–155. doi: 10.1111/j.1547-5069.2012.01445.x
Cai, T., Liu, Y., and Xiao, J. (2018). Long noncoding RNA MALAT1 knockdown reverses chemoresistance to temozolomide via promoting microRNA-101 in glioblastoma. Cancer Med. 7, 1404–1415. doi: 10.1002/cam4.1384
Calias, P., Banks, W. A., Begley, D., Scarpa, M., and Dickson, P. (2014). Intrathecal delivery of protein therapeutics to the brain: a critical reassessment. Pharmacol. Ther. 144, 114–122. doi: 10.1016/j.pharmthera.2014.05.009
Cao, Y., Tseng, C. L., Balter, J. M., Teng, F., Parmar, H. A., and Sahgal, A. (2017). MR-guided radiation therapy: transformative technology and its role in the central nervous system. Neuro Oncol. 19, ii16–ii29. doi: 10.1093/neuonc/nox006
Chang, E. L., Wefel, J. S., Hess, K. R., Allen, P. K., Lang, F. F., Kornguth, D. G., et al. (2009). Neurocognition in patients with brain metastases treated with radiosurgery or radiosurgery plus whole-brain irradiation: a randomised controlled trial. Lancet Oncol. 10, 1037–1044. doi: 10.1016/S1470-2045(09)70263-3
Chen, L. Y., Zhang, X. M., Han, B. Q., and Dai, H. B. (2020). Long noncoding RNA SNHG12 indicates the prognosis and accelerates tumorigenesis of diffuse large B-Cell lymphoma through sponging microR-195. Onco Targets Ther. 13, 5563–5574. doi: 10.2147/OTT.S249429
Chen, S., Li, W., and Guo, A. (2019). LOXL1-AS1 predicts poor prognosis and promotes cell proliferation, migration, and invasion in osteosarcoma. Biosci. Rep. 39:BSR20190447. doi: 10.1042/BSR20190447
Chen, W., and Qin, C. (2015). General hallmarks of microRNAs in brain evolution and development. RNA Biol. 12, 701–708. doi: 10.1080/15476286.2015.1048954
Chen, X., Guo, G., Lu, Y., Wang, S., Zhang, Y., and Huang, Q. (2021). Mechanisms and functions of long non-coding RNAs in glioma (Review). Oncol. Rep. 45:9. doi: 10.3892/or.2021.7960
Cheng, J., Meng, J., Zhu, L., and Peng, Y. (2020). Exosomal noncoding RNAs in Glioma: biological functions and potential clinical applications. Mol. Cancer 19:66. doi: 10.1186/s12943-020-01189-3
Chunharojrith, P., Nakayama, Y., Jiang, X., Kery, R. E., Ma, J., De La Hoz Ulloa, C. S., et al. (2015). Tumor suppression by MEG3 lncRNA in a human pituitary tumor derived cell line. Mol. Cell. Endocrinol. 416, 27–35. doi: 10.1016/j.mce.2015.08.018
Churilla, T. M., Chowdhury, I. H., Handorf, E., Collette, L., Collette, S., Dong, Y., et al. (2019). Comparison of local control of brain metastases with stereotactic radiosurgery vs surgical resection: a secondary analysis of a randomized clinical trial. JAMA Oncol. 5, 243–247. doi: 10.1001/jamaoncol.2018.4610
Cortelazzo, S., Ferreri, A., Hoelzer, D., and Ponzoni, M. (2017). Lymphoblastic lymphoma. Crit. Rev. Oncol. Hematol. 113, 304–317. doi: 10.1016/j.critrevonc.2017.03.020
D’Angelo, D., Mussnich, P., Sepe, R., Raia, M., Del Vecchio, L., Cappabianca, P., et al. (2019). RPSAP52 lncRNA is overexpressed in pituitary tumors and promotes cell proliferation by acting as miRNA sponge for HMGA proteins. J. Mol. Med. (Berl.) 97, 1019–1032. doi: 10.1007/s00109-019-01789-7
Di Lullo, E., and Kriegstein, A. R. (2017). The use of brain organoids to investigate neural development and disease. Nat. Rev. Neurosci. 18, 573–584. doi: 10.1038/nrn.2017.107
Ding, C., Yi, X., Xu, J., Huang, Z., Bu, X., Wang, D., et al. (2020). Long non-coding RNA MEG3 modifies cell-cycle, migration, invasion, and proliferation through AKAP12 by sponging miR-29c in meningioma cells. Front. Oncol. 10:537763. doi: 10.3389/fonc.2020.537763
Donovan, L. K., Delaidelli, A., Joseph, S. K., Bielamowicz, K., Fousek, K., Holgado, B. L., et al. (2020). Locoregional delivery of CAR T cells to the cerebrospinal fluid for treatment of metastatic medulloblastoma and ependymoma. Nat. Med. 26, 720–731. doi: 10.1038/s41591-020-0827-2
Duffau, H. (2017). A two-level model of interindividual anatomo-functional variability of the brain and its implications for neurosurgery. Cortex 86, 303–313. doi: 10.1016/j.cortex.2015.12.009
Dufour, C., Beaugrand, A., Pizer, B., Micheli, J., Aubelle, M. S., Fourcade, A., et al. (2012). Metastatic medulloblastoma in childhood: chang’s classification revisited. Int. J. Surg. Oncol. 2012:245385. doi: 10.1155/2012/245385
Eichler, A. F., and Loeffler, J. S. (2007). Multidisciplinary management of brain metastases. Oncologist 12, 884–898. doi: 10.1634/theoncologist.12-7-884
Fox, C. P., Phillips, E. H., Smith, J., Linton, K., Gallop-Evans, E., Hemmaway, C., et al. (2019). Guidelines for the diagnosis and management of primary central nervous system diffuse large B-cell lymphoma. Br. J. Haematol. 184, 348–363. doi: 10.1111/bjh.15661
Franz, D. N., Belousova, E., Sparagana, S., Bebin, E. M., Frost, M., Kuperman, R., et al. (2014). Everolimus for subependymal giant cell astrocytoma in patients with tuberous sclerosis complex: 2-year open-label extension of the randomised EXIST-1 study. Lancet Oncol. 15, 1513–1520. doi: 10.1016/S1470-2045(14)70489-9
Franz, D. N., Belousova, E., Sparagana, S., Bebin, E. M., Frost, M., Kuperman, R., et al. (2013). Efficacy and safety of everolimus for subependymal giant cell astrocytomas associated with tuberous sclerosis complex (EXIST-1): a multicentre, randomised, placebo-controlled phase 3 trial. Lancet 381, 125–132. doi: 10.1016/S0140-6736(12)61134-9
Fu, D., Zhang, Y., and Cui, H. (2018). Long noncoding RNA CCAT2 is activated by E2F1 and exerts oncogenic properties by interacting with PTTG1 in pituitary adenomas. Am. J. Cancer Res. 8, 245–255.
Gaetani, L., Paolini Paoletti, F., Bellomo, G., Mancini, A., Simoni, S., Di Filippo, M., et al. (2020). CSF and blood biomarkers in neuroinflammatory and neurodegenerative diseases: implications for treatment. Trends Pharmacol. Sci. 41, 1023–1037. doi: 10.1016/j.tips.2020.09.011
Galldiks, N., Albert, N. L., Sommerauer, M., Grosu, A. L., Ganswindt, U., Law, I., et al. (2017). PET imaging in patients with meningioma-report of the RANO/PET Group. Neuro Oncol. 19, 1576–1587. doi: 10.1093/neuonc/nox112
Gao, R., Zhang, R., Zhang, C., Liang, Y., and Tang, W. (2018a). LncRNA LOXL1-AS1 promotes the proliferation and metastasis of medulloblastoma by activating the PI3K/AKT pathway. Anal. Cell. Pathol. (Amst.) 2018:9275685. doi: 10.1155/2018/9275685
Gao, R., Zhang, R., Zhang, C., Zhao, L., and Zhang, Y. (2018b). Long noncoding RNA CCAT1 promotes cell proliferation and metastasis in human medulloblastoma via MAPK pathway. Tumori 104, 43–50. doi: 10.5301/tj.5000662
Gerlinger, M., Rowan, A. J., Horswell, S., Math, M., Larkin, J., Endesfelder, D., et al. (2012). Intratumor heterogeneity and branched evolution revealed by multiregion sequencing. N. Engl. J. Med. 366, 883–892. doi: 10.1056/NEJMoa1113205
Ghafouri-Fard, S., and Taheri, M. (2019). Maternally expressed gene 3 (MEG3): a tumor suppressor long non coding RNA. Biomed. Pharmacother. 118:109129. doi: 10.1016/j.biopha.2019.109129
Gould, J. (2018). Breaking down the epidemiology of brain cancer. Nature 561, S40–S41. doi: 10.1038/d41586-018-06704-7
Gusyatiner, O., and Hegi, M. E. (2018). Glioma epigenetics: from subclassification to novel treatment options. Semin. Cancer Biol. 51, 50–58. doi: 10.1016/j.semcancer.2017.11.010
Hadidchi, S., Surento, W., Lerner, A., Liu, C. J., Gibbs, W. N., Kim, P. E., et al. (2019). Headache and Brain Tumor. Neuroimaging Clin. N. Am. 29, 291–300. doi: 10.1016/j.nic.2019.01.008
Han, M., Wang, S., Fritah, S., Wang, X., Zhou, W., Yang, N., et al. (2019). Interfering with long non-coding RNA MIR22HG processing inhibits glioblastoma progression through suppression of Wnt/β-catenin signalling. Brain 143, 512–530. doi: 10.1093/brain/awz406
Han, M., Wang, S., Fritah, S., Wang, X., Zhou, W., Yang, N., et al. (2020). Interfering with long non-coding RNA MIR22HG processing inhibits glioblastoma progression through suppression of Wnt/β-catenin signalling. Brain 143, 512–530.
Han, Y., Wu, Z., Wu, T., Huang, Y., Cheng, Z., Li, X., et al. (2016). Tumor-suppressive function of long noncoding RNA MALAT1 in glioma cells by downregulation of MMP2 and inactivation of ERK/MAPK signaling. Cell Death Dis. 7:e2123. doi: 10.1038/cddis.2015.407
Hanan, M., Soreq, H., and Kadener, S. (2017). CircRNAs in the brain. RNA Biol. 14, 1028–1034. doi: 10.1080/15476286.2016.1255398
Hanjin, C., Tao, L., Pengfei, L., Ali, Y., Huajun, Z., Jiekun, L., et al. (2018). Altered long noncoding RNA and messenger RNA expression in experimental intracerebral hemorrhage - a preliminary study. Cell. Physiol. Biochem. 45, 1284–1301. doi: 10.1159/000487464
Hawrylycz, M. J., Lein, E. S., Guillozet-Bongaarts, A. L., Shen, E. H., Ng, L., Miller, J. A., et al. (2012). An anatomically comprehensive atlas of the adult human brain transcriptome. Nature 489, 391–399. doi: 10.1038/nature11405
Hodges, T. R., Ott, M., Xiu, J., Gatalica, Z., Swensen, J., Zhou, S., et al. (2017). Mutational burden, immune checkpoint expression, and mismatch repair in glioma: implications for immune checkpoint immunotherapy. Neuro Oncol. 19, 1047–1057. doi: 10.1093/neuonc/nox026
Holmes, D. (2016). Pituitary gland: sex difference in comorbidity burden associated with nonfunctioning pituitary adenomas. Nat. Rev. Endocrinol. 12:374. doi: 10.1038/nrendo.2016.82
Horbinski, C., Ligon, K. L., Brastianos, P., Huse, J. T., Venere, M., Chang, S., et al. (2019). The medical necessity of advanced molecular testing in the diagnosis and treatment of brain tumor patients. Neuro Oncol. 21, 1498–1508. doi: 10.1093/neuonc/noz119
Huang, R. Y., Bi, W. L., Griffith, B., Kaufmann, T. J., La Fougère, C., Schmidt, N. O., et al. (2019). Imaging and diagnostic advances for intracranial meningiomas. Neuro Oncol. 21, i44–i61. doi: 10.1093/neuonc/noy143
Huang, T., Cai, M., Chen, C., Ling, C., Zhang, B., Zheng, W., et al. (2021). LINC01116 boosts the progression of pituitary adenoma via regulating miR-744-5p/HOXB8 pathway. Mol. Cell. Endocrinol. 536:111350. doi: 10.1016/j.mce.2021.111350
Huang, X., Qian, W., and Ye, X. (2020). Long noncoding RNAs in diffuse large B-cell lymphoma: current advances and perspectives. Onco Targets Ther. 13, 4295–4303. doi: 10.2147/OTT.S253330
Jeon, M. T., Kim, K. S., Kim, E. S., Lee, S., Kim, J., Hoe, H. S., et al. (2021). Emerging pathogenic role of peripheral blood factors following BBB disruption in neurodegenerative disease. Ageing Res. Rev. 68:101333. doi: 10.1016/j.arr.2021.101333
Jiang, P., Wang, P., Sun, X., Yuan, Z., Zhan, R., Ma, X., et al. (2016). Knockdown of long noncoding RNA H19 sensitizes human glioma cells to temozolomide therapy. Onco Targets Ther. 9, 3501–3509. doi: 10.2147/OTT.S96278
Jing, S. Y., Lu, Y. Y., Yang, J. K., Deng, W. Y., Zhou, Q., and Jiao, B. H. (2016). Expression of long non-coding RNA CRNDE in glioma and its correlation with tumor progression and patient survival. Eur. Rev. Med. Pharmacol. Sci. 20, 3992–3996.
Johanson, C. E., Duncan, J. A. III, Klinge, P. M., Brinker, T., Stopa, E. G., and Silverberg, G. D. (2008). Multiplicity of cerebrospinal fluid functions: new challenges in health and disease. Cerebrospinal Fluid Res. 5:10. doi: 10.1186/1743-8454-5-10
Kang, H. J., Kawasawa, Y. I., Cheng, F., Zhu, Y., Xu, X., Li, M., et al. (2011). Spatio-temporal transcriptome of the human brain. Nature 478, 483–489. doi: 10.1038/nature10523
Katsushima, K., Lee, B., Kunhiraman, H., Zhong, C., Murad, R., Yin, J., et al. (2021). The long noncoding RNA lnc-HLX-2-7 is oncogenic in Group 3 medulloblastomas. Neuro Oncol. 23, 572–585. doi: 10.1093/neuonc/noaa235
Ke, J., Yao, Y. L., Zheng, J., Wang, P., Liu, Y. H., Ma, J., et al. (2015). Knockdown of long non-coding RNA HOTAIR inhibits malignant biological behaviors of human glioma cells via modulation of miR-326. Oncotarget 6, 21934–21949. doi: 10.18632/oncotarget.4290
Killer, H. E. (2013). Production and circulation of cerebrospinal fluid with respect to the subarachnoid space of the optic nerve. J. Glaucoma 22(Suppl. 5), S8–S10. doi: 10.1097/IJG.0b013e318293498b
Kim, D. G., Kim, K. H., Seo, Y. J., Yang, H., Marcusson, E. G., Son, E., et al. (2016). Anti-miR delivery strategies to bypass the blood-brain barrier in glioblastoma therapy. Oncotarget 7, 29400–29411. doi: 10.18632/oncotarget.8837
Kraus, T. F., Greiner, A., Guibourt, V., Lisec, K., and Kretzschmar, H. A. (2015). Identification of stably expressed lncRNAs as valid endogenous controls for profiling of human glioma. J. Cancer 6, 111–119. doi: 10.7150/jca.10867
Kristensen, B. W., Priesterbach-Ackley, L. P., Petersen, J. K., and Wesseling, P. (2019). Molecular pathology of tumors of the central nervous system. Ann. Oncol. 30, 1265–1278. doi: 10.1093/annonc/mdz164
Lai, C. P., Mardini, O., Ericsson, M., Prabhakar, S., Maguire, C., Chen, J. W., et al. (2014). Dynamic biodistribution of extracellular vesicles in vivo using a multimodal imaging reporter. ACS Nano 8, 483–494. doi: 10.1021/nn404945r
Laneve, P., Po, A., Favia, A., Legnini, I., Alfano, V., Rea, J., et al. (2017). The long noncoding RNA linc-NeD125 controls the expression of medulloblastoma driver genes by microRNA sponge activity. Oncotarget 8, 31003–31015. doi: 10.18632/oncotarget.16049
Langner-Lemercier, S., Houillier, C., Soussain, C., Ghesquières, H., Chinot, O., Taillandier, L., et al. (2016). Primary CNS lymphoma at first relapse/progression: characteristics, management, and outcome of 256 patients from the French LOC network. Neuro Oncol. 18, 1297–1303. doi: 10.1093/neuonc/now033
Latowska, J., Grabowska, A., Zarȩbska, Ż, Kuczyński, K., Kuczyńska, B., and Rolle, K. (2020). Non-coding RNAs in brain tumors, the contribution of lncRNAs, circRNAs, and snoRNAs to cancer development-their diagnostic and therapeutic potential. Int. J. Mol. Sci. 21:7001. doi: 10.3390/ijms21197001
Laudicella, R., Quartuccio, N., Argiroffi, G., Alongi, P., Baratto, L., Califaretti, E., et al. (2021). Unconventional non-amino acidic PET radiotracers for molecular imaging in gliomas. Eur. J. Nucl. Med. Mol. Imaging doi: 10.1007/s00259-021-05352-w
Le Rhun, E., Seoane, J., Salzet, M., Soffietti, R., and Weller, M. (2020). Liquid biopsies for diagnosing and monitoring primary tumors of the central nervous system. Cancer Lett. 480, 24–28. doi: 10.1016/j.canlet.2020.03.021
Lebanony, D., Benjamin, H., Gilad, S., Ezagouri, M., Dov, A., Ashkenazi, K., et al. (2009). Diagnostic assay based on hsa-miR-205 expression distinguishes squamous from nonsquamous non-small-cell lung carcinoma. J. Clin. Oncol. 27, 2030–2037. doi: 10.1200/JCO.2008.19.4134
Li, B., Shen, M., Yao, H., Chen, X., and Xiao, Z. (2019). Long noncoding RNA TP73-AS1 modulates medulloblastoma progression in vitro and in vivo by sponging miR-494-3p and targeting EIF5A2. Onco Targets Ther. 12, 9873–9885. doi: 10.2147/OTT.S228305
Li, B., Zhao, H., Song, J., Wang, F., and Chen, M. (2020). LINC00174 down-regulation decreases chemoresistance to temozolomide in human glioma cells by regulating miR-138-5p/SOX9 axis. Hum. Cell 33, 159–174. doi: 10.1007/s13577-019-00281-1
Li, D., Li, L., Chen, X., Zhou, C., Hao, B., and Cao, Y. (2021). Dysregulation of lncRNA-CCRR contributes to brain metastasis of breast cancer by intercellular coupling via regulating connexin 43 expression. J. Cell. Mol. Med. 25, 4826–4834. doi: 10.1111/jcmm.16455
Li, J., Qian, Y., Zhang, C., Wang, W., Qiao, Y., Song, H., et al. (2021). LncRNA LINC00473 is involved in the progression of invasive pituitary adenoma by upregulating KMT5A via ceRNA-mediated miR-502-3p evasion. Cell Death Dis. 12:580. doi: 10.1038/s41419-021-03861-y
Li, J., Zhang, Q., Ge, P., Zeng, C., Lin, F., Wang, W., et al. (2020). FAM225B Is a Prognostic lncRNA for Patients with Recurrent Glioblastoma. Dis. Markers 2020:8888085. doi: 10.1155/2020/8888085
Li, T., Ren, J., Ma, J., Wu, J., Zhang, R., Yuan, H., et al. (2019). LINC00702/miR-4652-3p/ZEB1 axis promotes the progression of malignant meningioma through activating Wnt/β-catenin pathway. Biomed. Pharmacother. 113:108718. doi: 10.1016/j.biopha.2019.108718
Li, W., Jiang, P., Sun, X., Xu, S., Ma, X., and Zhan, R. (2016). Suppressing H19 modulates tumorigenicity and stemness in U251 and U87MG glioma cells. Cell. Mol. Neurobiol. 36, 1219–1227. doi: 10.1007/s10571-015-0320-5
Li, X., Sun, L., Wang, X., Wang, N., Xu, K., Jiang, X., et al. (2021). A five immune-related lncRNA signature as a prognostic target for glioblastoma. Front. Mol. Biosci. 8:632837. doi: 10.3389/fmolb.2021.632837
Liu, Q., Yu, W., Zhu, S., Cheng, K., Xu, H., Lv, Y., et al. (2018). Long noncoding RNA GAS5 regulates the proliferation, migration, and invasion of glioma cells by negatively regulating miR-18a-5p. J. Cell. Physiol. 234, 757–768. doi: 10.1002/jcp.26889
López Vázquez, M., Du, W., Kanaya, N., Kitamura, Y., and Shah, K. (2021). Next-Generation immunotherapies for brain metastatic cancers. Trends Cancer 7, 809–822. doi: 10.1016/j.trecan.2021.02.003
Louis, D. N., Ohgaki, H., Wiestler, O. D., Cavenee, W. K., Burger, P. C., Jouvet, A., et al. (2007). The 2007 WHO classification of tumours of the central nervous system. Acta Neuropathol. 114, 97–109. doi: 10.1007/s00401-007-0243-4
Louis, D. N., Perry, A., Reifenberger, G., Von Deimling, A., Figarella-Branger, D., Cavenee, W. K., et al. (2016). The 2016 world health organization classification of tumors of the central nervous system: a summary. Acta Neuropathol. 131, 803–820. doi: 10.1007/s00401-016-1545-1
Louis, D. N., Perry, A., Wesseling, P., Brat, D. J., Cree, I. A., Figarella-Branger, D., et al. (2021). The 2021 WHO classification of tumors of the central nervous system: a summary. Neuro Oncol. 23, 1231–1251. doi: 10.1093/neuonc/noab106
Lu, C., Wei, Y., Wang, X., Zhang, Z., Yin, J., Li, W., et al. (2020). DNA-methylation-mediated activating of lncRNA SNHG12 promotes temozolomide resistance in glioblastoma. Mol. Cancer 19:28. doi: 10.1186/s12943-020-1137-5
Lundborg, C., Hahn-Zoric, M., Biber, B., and Hansson, E. (2010). Glial cell line-derived neurotrophic factor is increased in cerebrospinal fluid but decreased in blood during long-term pain. J. Neuroimmunol. 220, 108–113. doi: 10.1016/j.jneuroim.2010.01.007
Lv, Q. L., Wang, L. C., Li, D. C., Lin, Q. X., Shen, X. L., Liu, H. Y., et al. (2020). Knockdown lncRNA DLEU1 inhibits gliomas progression and promotes temozolomide chemosensitivity by regulating autophagy. Front. Pharmacol. 11:560543. doi: 10.3389/fphar.2020.560543
Ma, X., Li, Z., Li, T., Zhu, L., Li, Z., and Tian, N. (2017). Long non-coding RNA HOTAIR enhances angiogenesis by induction of VEGFA expression in glioma cells and transmission to endothelial cells via glioma cell derived-extracellular vesicles. Am. J. Transl. Res. 9, 5012–5021.
Mahajan, A., Ahmed, S., Mcaleer, M. F., Weinberg, J. S., Li, J., Brown, P., et al. (2017). Post-operative stereotactic radiosurgery versus observation for completely resected brain metastases: a single-centre, randomised, controlled, phase 3 trial. Lancet Oncol. 18, 1040–1048. doi: 10.1016/S1470-2045(17)30414-X
Mao, D., Jie, Y., and Lv, Y. (2020). LncRNA SNHG6 induces epithelial-mesenchymal transition of pituitary adenoma via suppressing MiR-944. Cancer Biother. Radiopharm. doi: 10.1089/cbr.2020.3587
Martínez-Glez, V., Alvarez, L., Franco-Hernández, C., Torres-Martin, M., De Campos, J. M., Isla, A., et al. (2010). Genomic deletions at 1p and 14q are associated with an abnormal cDNA microarray gene expression pattern in meningiomas but not in schwannomas. Cancer Genet. Cytogenet. 196, 1–6. doi: 10.1016/j.cancergencyto.2009.08.003
Martinez-Rios, C., Mcandrews, M. P., Logan, W., Krings, T., Lee, D., and Widjaja, E. (2016). MRI in the evaluation of localization-related epilepsy. J. Magn. Reson. Imaging 44, 12–22. doi: 10.1002/jmri.25269
Mattox, A. K., Yan, H., and Bettegowda, C. (2019). The potential of cerebrospinal fluid-based liquid biopsy approaches in CNS tumors. Neuro Oncol. 21, 1509–1518. doi: 10.1093/neuonc/noz156
Mazor, G., Levin, L., Picard, D., Ahmadov, U., Carén, H., Borkhardt, A., et al. (2019). The lncRNA TP73-AS1 is linked to aggressiveness in glioblastoma and promotes temozolomide resistance in glioblastoma cancer stem cells. Cell Death Dis. 10:246. doi: 10.1038/s41419-019-1477-5
Mei, J.-C., Yan, G., and Mei, S.-Q. (2020). Diagnostic and prognostic potentials of long noncoding RNA ELF3-AS1 in glioma patients. Dis. Markers 2020:8871746. doi: 10.1155/2020/8871746
Millard, N. E., and De Braganca, K. C. (2016). Medulloblastoma. J. Child Neurol. 31, 1341–1353. doi: 10.1177/0883073815600866
Min, W., Dai, D., Wang, J., Zhang, D., Zhang, Y., Han, G., et al. (2016). Long noncoding RNA miR210HG as a potential biomarker for the diagnosis of glioma. PLoS One 11:e0160451. doi: 10.1371/journal.pone.0160451
Nevel, K. S., Wilcox, J. A., Robell, L. J., and Umemura, Y. (2018). The utility of liquid biopsy in central nervous system malignancies. Curr. Oncol. Rep. 20:60. doi: 10.1007/s11912-018-0706-x
Northcott, P. A., Buchhalter, I., Morrissy, A. S., Hovestadt, V., Weischenfeldt, J., Ehrenberger, T., et al. (2017). The whole-genome landscape of medulloblastoma subtypes. Nature 547, 311–317. doi: 10.1038/nature22973
Northcott, P. A., Jones, D. T., Kool, M., Robinson, G. W., Gilbertson, R. J., Cho, Y. J., et al. (2012a). Medulloblastomics: the end of the beginning. Nat. Rev. Cancer 12, 818–834. doi: 10.1038/nrc3410
Northcott, P. A., Shih, D. J., Peacock, J., Garzia, L., Morrissy, A. S., Zichner, T., et al. (2012b). Subgroup-specific structural variation across 1,000 medulloblastoma genomes. Nature 488, 49–56.
Olson, J. E., Janney, C. A., Rao, R. D., Cerhan, J. R., Kurtin, P. J., Schiff, D., et al. (2002). The continuing increase in the incidence of primary central nervous system non-Hodgkin lymphoma: a surveillance, epidemiology, and end results analysis. Cancer 95, 1504–1510. doi: 10.1002/cncr.10851
Orešković, D. (2015). The controversy on choroid plexus function in cerebrospinal fluid production in humans: how long different views could be neglected? Croat. Med. J. 56, 306–310. doi: 10.3325/cmj.2015.56.306
Ostrom, Q. T., Cioffi, G., Gittleman, H., Patil, N., Waite, K., Kruchko, C., et al. (2019). CBTRUS statistical report: primary brain and other central nervous system tumors diagnosed in the United States in 2012-2016. Neuro Oncol. 21, v1–v100. doi: 10.1093/neuonc/noz150
Ostrom, Q. T., Gittleman, H., Liao, P., Vecchione-Koval, T., Wolinsky, Y., Kruchko, C., et al. (2017). CBTRUS Statistical Report: primary brain and other central nervous system tumors diagnosed in the United States in 2010-2014. Neuro Oncol. 19, v1–v88. doi: 10.1093/neuonc/nox158
Ostrom, Q. T., Gittleman, H., Xu, J., Kromer, C., Wolinsky, Y., Kruchko, C., et al. (2016). CBTRUS statistical report: primary brain and other central nervous system tumors diagnosed in the United States in 2009-2013. Neuro Oncol. 18, v1–v75. doi: 10.1093/neuonc/now207
Ostrom, Q. T., Patil, N., Cioffi, G., Waite, K., Kruchko, C., and Barnholtz-Sloan, J. S. (2020). CBTRUS statistical report: primary brain and other central nervous system tumors diagnosed in the United States in 2013-2017. Neuro Oncol. 22, iv1–iv96. doi: 10.1093/neuonc/noaa200
Pan, C. Y., Tian, M., Zhang, L. L., Tian, D., Wang, L. Y., Sun, Y. J., et al. (2020). lncRNA signature for predicting cerebral vasospasm in patients with SAH: implications for precision neurosurgery. Mol. Ther. Nucleic Acids 21, 983–990. doi: 10.1016/j.omtn.2020.07.028
Pandit, R., Chen, L., and Götz, J. (2020). The blood-brain barrier: physiology and strategies for drug delivery. Adv. Drug Deliv. Rev. 165-166, 1–14. doi: 10.1016/j.addr.2019.11.009
Parasramka, M., Yan, I. K., Wang, X., Nguyen, P., Matsuda, A., Maji, S., et al. (2017). BAP1 dependent expression of long non-coding RNA NEAT-1 contributes to sensitivity to gemcitabine in cholangiocarcinoma. Mol. Cancer 16:22. doi: 10.1186/s12943-017-0587-x
Parnetti, L., Gaetani, L., Eusebi, P., Paciotti, S., Hansson, O., El-Agnaf, O., et al. (2019). CSF and blood biomarkers for Parkinson’s disease. Lancet Neurol. 18, 573–586. doi: 10.1016/S1474-4422(19)30024-9
Pietsch, T., Wohlers, I., Goschzik, T., Dreschmann, V., Denkhaus, D., Dörner, E., et al. (2014). Supratentorial ependymomas of childhood carry C11orf95-RELA fusions leading to pathological activation of the NF-κB signaling pathway. Acta Neuropathol. 127, 609–611. doi: 10.1007/s00401-014-1264-4
Pottoo, F. H., Javed, M. N., Rahman, J. U., Abu-Izneid, T., and Khan, F. A. (2021). Targeted delivery of miRNA based therapeuticals in the clinical management of Glioblastoma Multiforme. Semin. Cancer Biol. 69, 391–398. doi: 10.1016/j.semcancer.2020.04.001
Proulx, S. T. (2021). Cerebrospinal fluid outflow: a review of the historical and contemporary evidence for arachnoid villi, perineural routes, and dural lymphatics. Cell. Mol. Life Sci. 78, 2429–2457. doi: 10.1007/s00018-020-03706-5
Ramanathan, M., Porter, D. F., and Khavari, P. A. (2019). Methods to study RNA-protein interactions. Nat. Methods 16, 225–234. doi: 10.1038/s41592-019-0330-1
Ramaswamy, V., and Taylor, M. D. (2017). Medulloblastoma: from myth to molecular. J. Clin. Oncol. 35, 2355–2363. doi: 10.1200/JCO.2017.72.7842
Rankin-Turner, S., Vader, P., O’driscoll, L., Giebel, B., Heaney, L. M., and Davies, O. G. (2021). A call for the standardised reporting of factors affecting the exogenous loading of extracellular vesicles with therapeutic cargos. Adv. Drug Deliv. Rev. 173, 479–491. doi: 10.1016/j.addr.2021.04.012
Raposo, G., and Stahl, P. D. (2019). Extracellular vesicles: a new communication paradigm? Nat. Rev. Mol. Cell Biol. 20, 509–510. doi: 10.1038/s41580-019-0158-7
Rastogi, S., Sharma, V., Bharti, P. S., Rani, K., Modi, G. P., Nikolajeff, F., et al. (2021). The evolving landscape of exosomes in neurodegenerative diseases: exosomes characteristics and a promising role in early diagnosis. Int. J. Mol. Sci. 22:440. doi: 10.3390/ijms22010440
Reddy, S., Tatiparti, K., Sau, S., and Iyer, A. K. (2021). Recent advances in nano delivery systems for blood-brain barrier (BBB) penetration and targeting of brain tumors. Drug Discov. Today 26, 1944–1952. doi: 10.1016/j.drudis.2021.04.008
Rodríguez-Lorenzo, S., Ferreira Francisco, D. M., Vos, R., Van Het Hof, B., Rijnsburger, M., Schroten, H., et al. (2020). Altered secretory and neuroprotective function of the choroid plexus in progressive multiple sclerosis. Acta Neuropathol. Commun. 8:35. doi: 10.1186/s40478-020-00903-y
Rohringer, M., Sutherland, G. R., Louw, D. F., and Sima, A. A. (1989). Incidence and clinicopathological features of meningioma. J. Neurosurg. 71, 665–672. doi: 10.3171/jns.1989.71.5.0665
Romani, M., Pistillo, M. P., and Banelli, B. (2018). Epigenetic targeting of glioblastoma. Front. Oncol. 8:448. doi: 10.3389/fonc.2018.00448
Rubio-Perez, C., Planas-Rigol, E., Trincado, J. L., Bonfill-Teixidor, E., Arias, A., Marchese, D., et al. (2021). Immune cell profiling of the cerebrospinal fluid enables the characterization of the brain metastasis microenvironment. Nat. Commun. 12:1503. doi: 10.1038/s41467-021-21789-x
Sakka, L., Coll, G., and Chazal, J. (2011). Anatomy and physiology of cerebrospinal fluid. Eur. Ann. Otorhinolaryngol. Head Neck Dis. 128, 309–316. doi: 10.1016/j.anorl.2011.03.002
Sawaya, R., Hammoud, M., Schoppa, D., Hess, K. R., Wu, S. Z., Shi, W. M., et al. (1998). Neurosurgical outcomes in a modern series of 400 craniotomies for treatment of parenchymal tumors. Neurosurgery 42, 1044–1055; discussion 1055-1046. doi: 10.1097/00006123-199805000-00054
Schouten, L. J., Rutten, J., Huveneers, H. A., and Twijnstra, A. (2002). Incidence of brain metastases in a cohort of patients with carcinoma of the breast, colon, kidney, and lung and melanoma. Cancer 94, 2698–2705. doi: 10.1002/cncr.10541
Seo, S., Kim, H., Sung, J. H., Choi, N., Lee, K., and Kim, H. N. (2020). Microphysiological systems for recapitulating physiology and function of blood-brain barrier. Biomaterials 232:119732. doi: 10.1016/j.biomaterials.2019.119732
Shang, C., Tang, W., Pan, C., Hu, X., and Hong, Y. (2018). Long non-coding RNA TUSC7 inhibits temozolomide resistance by targeting miR-10a in glioblastoma. Cancer Chemother. Pharmacol. 81, 671–678. doi: 10.1007/s00280-018-3522-y
Shen, L., Chen, L., Wang, Y., Jiang, X., Xia, H., and Zhuang, Z. (2015). Long noncoding RNA MALAT1 promotes brain metastasis by inducing epithelial-mesenchymal transition in lung cancer. J. Neurooncol. 121, 101–108. doi: 10.1007/s11060-014-1613-0
Shi, J., Zhang, Y., Qin, B., Wang, Y., and Zhu, X. (2019). Long non-coding RNA LINC00174 promotes glycolysis and tumor progression by regulating miR-152-3p/SLC2A1 axis in glioma. J. Exp. Clin. Cancer Res. 38:395. doi: 10.1186/s13046-019-1390-x
Shi, Y., Wang, Y., Luan, W., Wang, P., Tao, T., Zhang, J., et al. (2014). Long non-coding RNA H19 promotes glioma cell invasion by deriving miR-675. PLoS One 9:e86295. doi: 10.1371/journal.pone.0086295
Siegel, R. L., Miller, K. D., and Jemal, A. (2017). Cancer statistics, 2017. CA Cancer J. Clin. 67, 7–30. doi: 10.3322/caac.21387
Simon, M., Von Deimling, A., Larson, J. J., Wellenreuther, R., Kaskel, P., Waha, A., et al. (1995). Allelic losses on chromosomes 14, 10, and 1 in atypical and malignant meningiomas: a genetic model of meningioma progression. Cancer Res. 55, 4696–4701.
Simonato, M., Agoston, D. V., Brooks-Kayal, A., Dulla, C., Fureman, B., Henshall, D. C., et al. (2021). Identification of clinically relevant biomarkers of epileptogenesis - a strategic roadmap. Nat. Rev. Neurol. 17, 231–242. doi: 10.1038/s41582-021-00461-4
Song, H., Han, L., Gao, Q., and Sun, Y. (2016). Long non-coding RNA CRNDE promotes tumor growth in medulloblastoma. Eur. Rev. Med. Pharmacol. Sci. 20, 2588–2597.
Sprowls, S. A., Arsiwala, T. A., Bumgarner, J. R., Shah, N., Lateef, S. S., Kielkowski, B. N., et al. (2019). Improving CNS delivery to brain metastases by blood-tumor barrier disruption. Trends Cancer 5, 495–505. doi: 10.1016/j.trecan.2019.06.003
Suh, J. H., Kotecha, R., Chao, S. T., Ahluwalia, M. S., Sahgal, A., and Chang, E. L. (2020). Current approaches to the management of brain metastases. Nat. Rev. Clin. Oncol. 17, 279–299. doi: 10.1038/s41571-019-0320-3
Sullivan, R., Maresh, G., Zhang, X., Salomon, C., Hooper, J., Margolin, D., et al. (2017). The emerging roles of extracellular vesicles as communication vehicles within the tumor microenvironment and beyond. Front. Endocrinol. (Lausanne) 8:194. doi: 10.3389/fendo.2017.00194
Sweeney, M. D., Zhao, Z., Montagne, A., Nelson, A. R., and Zlokovic, B. V. (2019). Blood-Brain barrier: from physiology to disease and back. Physiol. Rev. 99, 21–78. doi: 10.1152/physrev.00050.2017
Swerdlow, S. H., Campo, E., Pileri, S. A., Harris, N. L., Stein, H., Siebert, R., et al. (2016). The 2016 revision of the World Health Organization classification of lymphoid neoplasms. Blood 127, 2375–2390. doi: 10.1182/blood-2016-01-643569
Tahira, A. C., Kubrusly, M. S., Faria, M. F., Dazzani, B., Fonseca, R. S., Maracaja-Coutinho, V., et al. (2011). Long noncoding intronic RNAs are differentially expressed in primary and metastatic pancreatic cancer. Mol. Cancer 10:141. doi: 10.1186/1476-4598-10-141
Tan, A. C., Ashley, D. M., López, G. Y., Malinzak, M., Friedman, H. S., and Khasraw, M. (2020). Management of glioblastoma: state of the art and future directions. CA Cancer J. Clin. 70, 299–312. doi: 10.3322/caac.21613
Tan, S. K., Pastori, C., Penas, C., Komotar, R. J., Ivan, M. E., Wahlestedt, C., et al. (2018). Serum long noncoding RNA HOTAIR as a novel diagnostic and prognostic biomarker in glioblastoma multiforme. Mol. Cancer 17:74. doi: 10.1186/s12943-018-0822-0
Taylor, M. D., Northcott, P. A., Korshunov, A., Remke, M., Cho, Y. J., Clifford, S. C., et al. (2012). Molecular subgroups of medulloblastoma: the current consensus. Acta Neuropathol. 123, 465–472. doi: 10.1007/s00401-011-0922-z
Teplyuk, N. M., Mollenhauer, B., Gabriely, G., Giese, A., Kim, E., Smolsky, M., et al. (2012). MicroRNAs in cerebrospinal fluid identify glioblastoma and metastatic brain cancers and reflect disease activity. Neuro Oncol. 14, 689–700. doi: 10.1093/neuonc/nos074
Tian, Y., Zheng, Y., and Dong, X. (2019). AGAP2-AS1 serves as an oncogenic lncRNA and prognostic biomarker in glioblastoma multiforme. J. Cell. Biochem. 120, 9056–9062. doi: 10.1002/jcb.28180
Tominaga, N., Kosaka, N., Ono, M., Katsuda, T., Yoshioka, Y., Tamura, K., et al. (2015). Brain metastatic cancer cells release microRNA-181c-containing extracellular vesicles capable of destructing blood-brain barrier. Nat. Commun. 6:6716. doi: 10.1038/ncomms7716
Torres-Bayona, S., Aldaz, P., Auzmendi-Iriarte, J., Saenz-Antoñanzas, A., Garcia, I., Arrazola, M., et al. (2018). PR-LncRNA signature regulates glioma cell activity through expression of SOX factors. Sci. Rep. 8:12746. doi: 10.1038/s41598-018-30836-5
Tumani, H., Huss, A., and Bachhuber, F. (2017). The cerebrospinal fluid and barriers - anatomic and physiologic considerations. Handb. Clin. Neurol. 146, 21–32. doi: 10.1016/B978-0-12-804279-3.00002-2
Uddin, M. S., Mamun, A. A., Alghamdi, B. S., Tewari, D., Jeandet, P., Sarwar, M. S., et al. (2020). Epigenetics of glioblastoma multiforme: from molecular mechanisms to therapeutic approaches. Semin. Cancer Biol. doi: 10.1016/j.semcancer.2020.12.015
van der Sanden, G. A., Schouten, L. J., Van Dijck, J. A., Van Andel, J. P., Van Der Maazen, R. W., and Coebergh, J. W. (2002). Primary central nervous system lymphomas: incidence and survival in the Southern and Eastern Netherlands. Cancer 94, 1548–1556. doi: 10.1002/cncr.10357
Wang, J., and Bettegowda, C. (2017). Applications of DNA-Based liquid biopsy for central nervous system neoplasms. J. Mol. Diagn. 19, 24–34. doi: 10.1016/j.jmoldx.2016.08.007
Wang, M., Cai, Y., Peng, Y., Xu, B., Hui, W., and Jiang, Y. (2020). Exosomal LGALS9 in the cerebrospinal fluid of glioblastoma patients suppressed dendritic cell antigen presentation and cytotoxic T-cell immunity. Cell Death Dis. 11:896. doi: 10.1038/s41419-020-03042-3
Wang, Q. M., Lian, G. Y., Song, Y., Huang, Y. F., and Gong, Y. (2019). LncRNA MALAT1 promotes tumorigenesis and immune escape of diffuse large B cell lymphoma by sponging miR-195. Life Sci. 231:116335. doi: 10.1016/j.lfs.2019.03.040
Wang, S., Liang, K., Hu, Q., Li, P., Song, J., Yang, Y., et al. (2017). JAK2-binding long noncoding RNA promotes breast cancer brain metastasis. J. Clin. Invest. 127, 4498–4515. doi: 10.1172/JCI91553
Wang, Z., Wang, Q., Bao, Z., Guo, L., Chen, H., Lv, T., et al. (2020). LINC00174 is a favorable prognostic biomarker in glioblastoma via promoting proliferative phenotype. Cancer Biomark 28, 421–427. doi: 10.3233/CBM-191026
Weber, R. G., Boström, J., Wolter, M., Baudis, M., Collins, V. P., Reifenberger, G., et al. (1997). Analysis of genomic alterations in benign, atypical, and anaplastic meningiomas: toward a genetic model of meningioma progression. Proc. Natl. Acad. Sci. U.S.A. 94, 14719–14724. doi: 10.1073/pnas.94.26.14719
Wei, G. H., and Wang, X. (2017). lncRNA MEG3 inhibit proliferation and metastasis of gastric cancer via p53 signaling pathway. Eur. Rev. Med. Pharmacol. Sci. 21, 3850–3856.
Wu, J., Ma, C., Tang, X., Shi, Y., Liu, Z., Chai, X., et al. (2020). The regulation and interaction of PVT1 and miR181a-5p contributes to the repression of SP1 expression by the combination of XJD decoction and cisplatin in human lung cancer cells. Biomed. Pharmacother. 121:109632. doi: 10.1016/j.biopha.2019.109632
Wu, Y. J., Yang, Q. S., Chen, H., Wang, J. T., Wang, W. B., and Zhou, L. (2021). Long non-coding RNA CASC19 promotes glioma progression by modulating the miR-454-3p/RAB5A axis and is associated with unfavorable MRI features. Oncol. Rep. 45, 728–737. doi: 10.3892/or.2020.7876
Wu, Y., Liang, S., Xu, B., Zhang, R., Zhu, M., Zhou, W., et al. (2017). Long noncoding RNA eosinophil granule ontogeny transcript inhibits cell proliferation and migration and promotes cell apoptosis in human glioma. Exp. Ther. Med. 14, 3817–3823. doi: 10.3892/etm.2017.4949
Wu, Z. R., Yan, L., Liu, Y. T., Cao, L., Guo, Y. H., Zhang, Y., et al. (2018). Inhibition of mTORC1 by lncRNA H19 via disrupting 4E-BP1/Raptor interaction in pituitary tumours. Nat. Commun. 9:4624. doi: 10.1038/s41467-018-06853-3
Xiao, Y., Zhu, Z., Li, J., Yao, J., Jiang, H., Ran, R., et al. (2020). Expression and prognostic value of long non-coding RNA H19 in glioma via integrated bioinformatics analyses. Aging (Albany NY) 12, 3407–3430. doi: 10.18632/aging.102819
Xie, J., Wang, X., Liu, S., Chen, C., Jiang, F., Mao, K., et al. (2019). LncRNA SAMMSON overexpression distinguished glioblastoma patients from patients with diffuse neurosarcoidosis. Neuroreport 30, 817–821. doi: 10.1097/WNR.0000000000001278
Xie, T., Cho, Y. B., Wang, K., Huang, D., Hong, H. K., Choi, Y. L., et al. (2014). Patterns of somatic alterations between matched primary and metastatic colorectal tumors characterized by whole-genome sequencing. Genomics 104, 234–241. doi: 10.1016/j.ygeno.2014.07.012
Xing, H., Wang, S., Li, Q., Ma, Y., and Sun, P. (2018). Long noncoding RNA LINC00460 targets miR-539/MMP-9 to promote meningioma progression and metastasis. Biomed. Pharmacother. 105, 677–682. doi: 10.1016/j.biopha.2018.06.005
Yang, B., Wei, Z. Y., Wang, B. Q., Yang, H. C., Wang, J. Y., and Bu, X. Y. (2018). Down-regulation of the long noncoding RNA-HOX transcript antisense intergenic RNA inhibits the occurrence and progression of glioma. J. Cell. Biochem. 119, 2278–2287. doi: 10.1002/jcb.26390
Yang, J., Sun, G., Hu, Y., Yang, J., Shi, Y., Liu, H., et al. (2019). Extracellular vesicle lncRNA metastasis-associated lung adenocarcinoma transcript 1 released from glioma stem cells modulates the inflammatory response of microglia after lipopolysaccharide stimulation through regulating miR-129-5p/High mobility group Box-1 Protein Axis. Front. Immunol. 10:3161. doi: 10.3389/fimmu.2019.03161
Yao, R. W., Wang, Y., and Chen, L. L. (2019). Cellular functions of long noncoding RNAs. Nat. Cell Biol. 21, 542–551. doi: 10.1038/s41556-019-0311-8
Yao, Y., Ma, J., Xue, Y., Wang, P., Li, Z., Liu, J., et al. (2015). Knockdown of long non-coding RNA XIST exerts tumor-suppressive functions in human glioblastoma stem cells by up-regulating miR-152. Cancer Lett. 359, 75–86. doi: 10.1016/j.canlet.2014.12.051
Yu, G., Li, C., Xie, W., Wang, Z., Gao, H., Cao, L., et al. (2017). Long non-coding RNA C5orf66-AS1 is downregulated in pituitary null cell adenomas and is associated with their invasiveness. Oncol. Rep. 38, 1140–1148. doi: 10.3892/or.2017.5739
Zajdel, M., Rymkiewicz, G., Sromek, M., Cieslikowska, M., Swoboda, P., Kulinczak, M., et al. (2019). Tumor and cerebrospinal fluid microRNAs in primary central nervous system lymphomas. Cancers (Basel) 11:1647. doi: 10.3390/cancers11111647
Zhang, J., Li, N., Fu, J., and Zhou, W. (2020). Long noncoding RNA HOTAIR promotes medulloblastoma growth, migration and invasion by sponging miR-1/miR-206 and targeting YY1. Biomed. Pharmacother. 124:109887. doi: 10.1016/j.biopha.2020.109887
Zhang, L., He, A., Chen, B., Bi, J., Chen, J., Guo, D., et al. (2020). A HOTAIR regulatory element modulates glioma cell sensitivity to temozolomide through long-range regulation of multiple target genes. Genome Res. 30, 155–163. doi: 10.1101/gr.251058.119
Zhang, S., Sun, W. C., Liang, Z. D., Yin, X. R., Ji, Z. R., Chen, X. H., et al. (2020). LncRNA SNHG4 attenuates inflammatory responses by sponging miR-449c-5p and up-regulating STAT6 in microglial during cerebral ischemia-reperfusion injury. Drug Des. Devel. Ther. 14, 3683–3695. doi: 10.2147/DDDT.S245445
Zhang, X., Gejman, R., Mahta, A., Zhong, Y., Rice, K. A., Zhou, Y., et al. (2010). Maternally expressed gene 3, an imprinted noncoding RNA gene, is associated with meningioma pathogenesis and progression. Cancer Res. 70, 2350–2358. doi: 10.1158/0008-5472.CAN-09-3885
Zhang, X., Sun, S., Pu, J. K. S., Tsang, A. C. O., Lee, D., Man, V. O. Y., et al. (2012). Long non-coding RNA expression profiles predict clinical phenotypes in glioma. Neurobiol. Dis. 48, 1–8. doi: 10.1016/j.nbd.2012.06.004
Zhang, X., Zhou, J., Gu, Z., Zhang, H., Gong, Q., and Luo, K. (2021). Advances in nanomedicines for diagnosis of central nervous system disorders. Biomaterials 269:120492. doi: 10.1016/j.biomaterials.2020.120492
Zhang, Y., Li, Y., Wang, J., and Lei, P. (2018a). Long non-coding RNA ferritin heavy polypeptide 1 pseudogene 3 controls glioma cell proliferation and apoptosis via regulation of the microRNA-224-5p/tumor protein D52 axis. Mol. Med. Rep. 18, 4239–4246. doi: 10.3892/mmr.2018.9491
Zhang, Y., Liu, Y. T., Tang, H., Xie, W. Q., Yao, H., Gu, W. T., et al. (2019). Exosome-transmitted lncRNA H19 inhibits the growth of pituitary adenoma. J. Clin. Endocrinol. Metab. 104, 6345–6356. doi: 10.1210/jc.2019-00536
Zhang, Y., Wang, T., Wang, S., Xiong, Y., Zhang, R., Zhang, X., et al. (2018b). Nkx2-2as suppression contributes to the pathogenesis of sonic hedgehog medulloblastoma. Cancer Res. 78, 962–973. doi: 10.1158/0008-5472.CAN-17-1631
Zhang, Y., Yu, R., Li, Q., Li, Y., Xuan, T., Cao, S., et al. (2020). SNHG1/miR-556-5p/TCF12 feedback loop enhances the tumorigenesis of meningioma through Wnt signaling pathway. J. Cell. Biochem. 121, 1880–1889. doi: 10.1002/jcb.29423
Zhang, Z., Yin, J., Lu, C., Wei, Y., Zeng, A., and You, Y. (2019). Exosomal transfer of long non-coding RNA SBF2-AS1 enhances chemoresistance to temozolomide in glioblastoma. J. Exp. Clin. Cancer Res. 38:166. doi: 10.1186/s13046-019-1139-6
Zhao, L., Liu, Y., Zhang, J., Liu, Y., and Qi, Q. (2019). LncRNA SNHG14/miR-5590-3p/ZEB1 positive feedback loop promoted diffuse large B cell lymphoma progression and immune evasion through regulating PD-1/PD-L1 checkpoint. Cell Death Dis. 10:731. doi: 10.1038/s41419-019-1886-5
Zhu, H. B., Li, B., Guo, J., Miao, Y. Z., Shen, Y. T., Zhang, Y. Z., et al. (2021). LncRNA MEG8 promotes TNF-α expression by sponging miR-454-3p in bone-invasive pituitary adenomas. Aging (Albany NY) 13, 14342–14354. doi: 10.18632/aging.203048
Zhu, H., Guo, J., Shen, Y., Dong, W., Gao, H., Miao, Y., et al. (2018). Functions and mechanisms of tumor necrosis factor-α and noncoding RNAs in bone-invasive pituitary adenomas. Clin. Cancer Res. 24, 5757–5766. doi: 10.1158/1078-0432.CCR-18-0472
Keywords: lncRNA, CSF, brain tumor, biomarker, treatment, diagnostic
Citation: Xu K, Jiang X, Ariston Gabriel AN, Li X, Wang Y and Xu S (2021) Evolving Landscape of Long Non-coding RNAs in Cerebrospinal Fluid: A Key Role From Diagnosis to Therapy in Brain Tumors. Front. Cell Dev. Biol. 9:737670. doi: 10.3389/fcell.2021.737670
Received: 07 July 2021; Accepted: 31 August 2021;
Published: 07 October 2021.
Edited by:
Yuanyuan Lu, Fourth Military Medical University, ChinaReviewed by:
Pei Wang, Second Military Medical University, ChinaLifang Ma, Shanghai Jiao Tong University, China
Xiaomin Niu, Shanghai Jiao Tong University, China
Qingqiong Luo, Shanghai Jiao Tong University, China
Copyright © 2021 Xu, Jiang, Ariston Gabriel, Li, Wang and Xu. This is an open-access article distributed under the terms of the Creative Commons Attribution License (CC BY). The use, distribution or reproduction in other forums is permitted, provided the original author(s) and the copyright owner(s) are credited and that the original publication in this journal is cited, in accordance with accepted academic practice. No use, distribution or reproduction is permitted which does not comply with these terms.
*Correspondence: Shuo Xu, eHVzaHVvQHNkdS5lZHUuY24=
†These authors have contributed equally to this work