- 1Department of Pediatrics, University of Wisconsin School of Medicine and Public Health, Madison, WI, United States
- 2McPherson Eye Research Institute, University of Wisconsin School of Medicine and Public Health, Madison, WI, United States
- 3Department of Ophthalmology and Visual Sciences, University of Wisconsin School of Medicine and Public Health, Madison, WI, United States
- 4Department of Human Oncology, University of Wisconsin School of Medicine and Public Health, Madison, WI, United States
- 5Department of Cell and Regenerative Biology, University of Wisconsin School of Medicine and Public Health, Madison, WI, United States
- 6Department of Biomedical Engineering, University of Wisconsin School of Medicine and Public Health, Madison, WI, United States
Adenosine receptors (AR) are widely expressed in a variety of tissues including the retina and brain. They are involved in adenosine-mediated immune responses underlying the onset and progression of neurodegenerative diseases. The expression of AR has been previously demonstrated in some retinal cells including endothelial cells and retinal pigment epithelial cells, but their expression in the choroid and choroidal cells remains unknown. Caffeine is a widely consumed AR antagonist that can influence inflammation and vascular cell function. It has established roles in the treatment of neonatal sleep apnea, acute migraine, and post lumbar puncture headache as well as the neurodegenerative diseases such as Parkinson and Alzheimer. More recently, AR antagonism with caffeine has been shown to protect preterm infants from ischemic retinopathy and retinal neovascularization. However, whether caffeine impacts the development and progression of ocular age-related diseases including neovascular age-related macular degermation remains unknown. Here, we examined the expression of AR in retinal and choroidal tissues and cells. We showed that antagonism of AR with caffeine or istradefylline decreased sprouting of thoracic aorta and choroid/retinal pigment epithelium explants in ex vivo cultures, consistent with caffeine’s ability to inhibit endothelial cell migration in culture. In vivo studies also demonstrated the efficacy of caffeine in inhibition of choroidal neovascularization and mononuclear phagocyte recruitment to the laser lesion sites. Istradefylline, a specific AR 2A antagonist, also decreased choroidal neovascularization. Collectively, our studies demonstrate an important role for expression of AR in the choroid whose antagonism mitigate choroidal inflammatory and angiogenesis activities.
Introduction
Fenestrated choroidal endothelial cells (ChEC) of the choriocapillaris aid in efficient transport of nutrients to the retinal pigment epithelium (RPE) and photoreceptor cells (Fields et al., 2020). ChEC also respond to pro-angiogenic and pro-survival growth factors including vascular endothelial growth factor (VEGF) with important roles in their survival and function. The choriocapillaris, which lies beneath the RPE, has the highest flow rate in the body (Pemp and Schmetterer, 2008) and plays an important role in vision health. Maintenance of choroidal homeostasis facilitates photoreceptor and outer retinal integrity, and function. The choroidal homeostasis is maintained by a balanced production of pro- and anti- angiogenic and inflammatory factors whose disruption contributes to the pathogenesis of sight-threatening eye diseases including exudative/neovascular age-related macular degeneration (nAMD) (Farnoodian et al., 2017). However, the mechanisms underlying these changes are not well-defined leaving gaps in our understanding of the most effective methods to treat choroidal neovascularization (CNV) in nAMD patients. VEGF is a major ocular proangiogenic factor with important roles in choroidal vascular homeostasis and is the target of current treatment for nAMD, although a significant portion of the patients are non-responsive (Kwak et al., 2000; Campochiaro and Akhlaq, 2020).
Retinal pigment epithelium is a major source of VEGF production with important roles in the health of choroid and retina (Saint-Geniez et al., 2009). Although VEGF expression is essential for RPE maintenance, its increased expression contributes to inflammation, CNV, and vessel leakiness. Anti-VEGF therapies such as bevacizumab (Avastin) and its closely related Fab fragment ranibizumab (Lucentis) are the current standard of care in the treatment of neovascular eye diseases (Hernandez-Zimbron et al., 2018). RPE atrophy is noted in about 20–30% of patients receiving anti-VEGF treatment (Munk et al., 2016). This further demonstrates the essential role VEGF plays in ocular tissue development and homeostasis (Carmeliet et al., 1996; Ferrara et al., 1996). Thus, in patients receiving anti-VEGF therapy for nAMD, the amount of VEGF needs to be reduced to prevent vascular inflammation, excessive permeability, and pathologic neovascularization but not so low to cause RPE atrophy. Clinically, this is challenging to establish and maintain, which may also explain, at least in part, the incomplete response to anti-VEGF noted in 30% of patients with nAMD (Mantel et al., 2020; Maroñas et al., 2020).
Aberrant inflammation and angiogenesis contribute to the pathogenesis of many ocular diseases including nAMD (Potilinski et al., 2021; Zhang and Wong, 2021). One of the several factors that influence angiogenesis is changes in oxygenation. Changes in oxygenation can occur due to disease processes or environment. Stress, trauma, inflammation, and ischemia cause ATP catabolism and subsequent release of adenosine (Riksen et al., 2003; Ryzhov et al., 2007; Ouyang et al., 2013; Bowser et al., 2017; Peleli and Carlstrom, 2017; Santiago et al., 2020). Adenosine mediates its physiologic effects through interactions with receptors denoted A1, A2A, A2B, and A3 (Auchampach, 2007; Ryzhov et al., 2007; Chen et al., 2013; Ouyang et al., 2013). These receptors are expressed on macrophages, endothelial cells, neutrophils, fibroblasts, and other cell types (Hasko et al., 2007). The localization and signaling pathways of adenosine receptors (AR) in various tissues including the retina have been the subject of numerous studies (Santiago et al., 2020). However, the cellular expression and signaling pathways of these receptors in the choroid remains largely unknown. Adenosine signaling through its receptors regulates inflammation and angiogenesis activities (Hasko and Cronstein, 2004; Montesinos et al., 2004; Ouyang et al., 2013). Thus, modulation of AR has been sought for the treatment of several diseases with inflammatory and/or neovascular components such as arthritis, cancer, diabetes, and wound healing (Montesinos et al., 2004; Lopez et al., 2018). There is compelling evidence for the therapeutic potential of AR antagonists in the treatment of ocular diseases characterized by inflammation and neovascularization (Santiago et al., 2020), like nAMD.
Caffeine is a widely consumed AR antagonist that exerts pharmacological effects on various organ systems (Cronstein, 2010; Dervişoğulları et al., 2016; Madeira et al., 2016, 2017; Kolahdouzan and Hamadeh, 2017; Zhang S. et al., 2017; Yoon and Danesh-Meyer, 2019). It is routinely given to preterm infants to treat apnea of prematurity, which also reduces oxygen-induced retinopathy and liver fibrosis (Cronstein, 2010; Zhang S. et al., 2017). The FDA lists caffeine as generally safe for human consumption, with 3–6 mg/kg representing the optimal dose for most people. The consumption of a single cup of coffee (Vural et al., 2014) or a single 200-mg caffeine capsule (Altinkaynak et al., 2016) in healthy subjects transiently decreases the choroidal thickness by 4 h. This decrease was attributed mainly to the vasoconstrictive effect of caffeine, but its chronic consequences on human health and morbidity remains unknown. In a prospective cohort from the Beaver Dam study (average follow-up of 4.8 years), coffee and caffeine consumption were not associated with an increased incidence of early age-related maculopathy, drusen deposits, or pigmentary abnormalities (Tomany et al., 2001). However, coffee consumption has been associated with increased risk for heart disease due to increased cholesterol and blood pressure (Boia et al., 2016). The mechanism by which caffeine mediates its various effects remains largely unknown and is further compounded by research findings that are frequently contradictory. A recent study by Stevens et al. showed increasing coffee consumption was associated with reduced long-term risk of heart failure in participants of the Farmington Heart Study (Stevens et al., 2021).
The protective effects of caffeine on the retina and lens have been the subject of many recent investigations (Yoon and Danesh-Meyer, 2019). Unfortunately, the relationship between caffeine and the eye is complicated by large inter-individual variability. These variables include pharmacological and health effects of caffeine, difficulty estimating dietary exposure to caffeine, and incomplete understanding of the pathological processes in the eye. The relationship between caffeine and the eye is particularly an issue in patients with other diseases associated with aging such as glaucoma and AMD (Santiago et al., 2020). Inflammation contributes to the normal aging, in general, and that of the retina (Steinle et al., 2009), and is a recognized target of the protective action of caffeine (Madeira et al., 2017). Here we addressed major gaps in our understanding of caffeine action in the retinal and choroidal vasculature by examining AR expression and the impact of their antagonism on sprouting angiogenesis and choroidal neovascularization.
Materials and Methods
Ethics Statement, Experimental Animals, and Cell Cultures
All animal experiments were carried out in accordance with and were approved by the Institutional Animal Care and Use Committee of the University of Wisconsin School of Medicine and Public Health. Immorto mice expressing a temperature-sensitive SV40 large T antigen were obtained from Charles River Laboratories (Wilmington, MA). These mice were back-crossed for at least 10 generations to C57BL/6J mice. Using these mice, we have reported the successful isolation and propagation of various ocular cell types including retinal endothelial cells (REC), retinal pericytes (RPC), retinal astrocytes (RAC), retinal pigment epithelium (RPE) cells, choroidal EC (ChEC), choroidal pericytes (ChPC), choroidal melanocytes (ChMC), and microglial cells (Su et al., 2003; Scheef et al., 2005, 2009; Fei et al., 2014; Farnoodian et al., 2015; Lavine et al., 2017). The identity of these cells has been previously confirmed by expression of specific cell markers, and their growth conditions are well detailed. Multiple isolations of these cells are available in the laboratory and were used in experiments described here. Human retinal endothelial cells were obtained from Cell Systems (Kirkland, WA) and ARPE19 cells were obtained from ATCC (Manassas, VA) and cultured under conditions as recommended by the suppliers. Deidentified human donor eyes were purchased from the Lions Eye Bank of Wisconsin (Madison, WI) and were used for the preparation of RNA from retina and choroid, and frozen sections for immune staining.
Cell Viability Assays
To determine potential adverse effects of caffeine on various cells incubated with the different concentrations of caffeine, their viability was assessed after 48 h using an MTS cell viability assay kit (Promega, Madison, WI, United States; G5421) as recommended by the supplier. Various ocular cell types were plated at 1 × 104 cells/well of a 96-well tissue culture plate in 0.1 ml of their corresponding growth medium overnight. The next day, each well received their appropriate medium containing different concentrations of caffeine (0, 100, 200, 400, 800, and 1,600 μM) and incubated for 48 h. The stock solution of caffeine citrate was obtained from our hospital pharmacy (caffeine citrate 20 mg/ml prepared in 5% dextrose: Exela Pharma Sciences, Lenoir, NC, United States). Following incubation, the percent cell viability relative to control (no caffeine; 5% dextrose) was determined as recommended by the supplier. Each group was done in triplicate and repeated with at least two different isolations of desired cell lines.
Scratch Wound Assays
Endothelial cells (4 × 105) were allowed to reach confluence (2–3 days) on a 60-mm dish. One day prior to wounding, the plates were incubated with vehicle, caffeine, or istradefylline at the desired concentrations noted in the figure legends. A wound was inflected to the cell layer with a 1-ml micropipette tip. The plates were rinsed and growth medium containing 5-fluorouracil (100 ng/ml, Sigma, St Louis, MO, United States; F6627) added to rule out the potential contribution of cell proliferation rate differences. The medium also contained the concentrations of vehicle, caffeine, or istradefylline (MedChemExpress, Monmouth Junction, NJ, United States; HY-10888- 10 mM stock in DMSO) as noted in the figure legend. Photographs were taken daily up to 72 h and migration assessed as percent of total distance covered as previously described (Grutzmacher et al., 2010). Each assay was performed in triplicate and repeated with at least two different isolation of cells.
Aortic Ring ex vivo Sprouting Angiogenesis Assays
Periaortic fibro-adipose tissue was removed from the thoracic aortas of 3-week-old male and female C57BL/6j mice as we previously noted (Grutzmacher et al., 2010). Aortas were sectioned into 1-mm-long aortic rings with eight aortic rings per 35-mm dish. The Matrigel (BD Biosciences, San Diego, CA, United States; 356235; 0.5 ml of 10 mg/ml) coated dishes were incubated in a 37°C incubator for 30 min to harden. DMEM with 1% fetal bovine serum (FBS; 2 ml) was added to each dish, and the rings were allowed to attach overnight. The next day, the dishes were fed with fresh medium containing the corresponding vehicle, 200 μM caffeine or 10 μM istradefylline (diluted in 0.3% Tween 80, 10% sucrose in saline). The cultures were fed every other day and at 7 days were photographed using a Nikon microscope equipped with a digital camera. We determined the area of sprouting per mm of tissue using Image J software (NIH).1
Choroid-Retinal Pigment Epithelium Complex ex vivo Sprouting Angiogenesis
Choroid-RPE was dissected from C57BL/6j male and female 3-week-old mice and sectioned into 0.5- to 1-mm pieces as we previously described (Farnoodian et al., 2015). The 10 pieces per eye were placed into 35−mm culture dish coated with 0.5 ml of Matrigel (10 mg/ml; BD Biosciences) and allowed to harden (30 min at 37oC). Endothelial cell growth medium (DMEM containing 10% FBS, 2 mmol/L L−glutamine, 2 mmol/L sodium pyruvate, 20 mmol/L HEPES, 1% non-essential amino acids, 100 μg/ml streptomycin, 100 U/ml penicillin, 55 U/ml heparin, and endothelial growth supplement 100 μg/ml (Sigma, St. Louis, MO, United States; E2759) was then added. After 48 h, the explants were fed every other day with the appropriate vehicle, 400 μM caffeine or 10 μM istradefylline. At 8 days, the explants were fixed with 4% paraformaldehyde and photographed (PFA; Electron Microscopy Sciences, Hatfield, PA, United States; 15710). The area of sprouting was quantified as previously described (Farnoodian et al., 2015).
Laser Induced Choroidal Neovascularization
Male and female pubertal (8-week-old) or mature adult (4-month-old) C57BL/6j mice were given the appropriate dose of vehicle, caffeine, or istradefylline by gavage as delineated below. The day of laser photocoagulation, the mice were anesthetized with ketamine hydrochloride (80 mg/kg) and xylazine (10 mg/kg). To dilate the pupil, Tropicamide (1%) was used. A slit lamp delivery system of an OcuLight GL diode laser (Iridex, Mountain View, CA) located the 9, 12, and 3 o’clock positions of each eye (posterior pole) for laser photocoagulation (75 μm spot size, 0.1 s duration, 120 mW) with a handheld cover slip that served as a contact lens for viewing of the retina. Photocoagulation facilitated rupture of the Bruch’s membrane. To assess neovascularization the choroid-RPE complex was harvested 2 weeks post-laser and fixed in 4% PFA. The complex was incubated with blocking buffer (20% normal goat serum and 5% fetal calf serum in 1 × PBS) for 1 h followed by incubation with anti-ICAM-2 (BD Biosciences; 553326; 1:500 in 1 × PBS with 20% normal goat serum and 20% fetal calf serum) at 4°C overnight. Staining mononuclear phagocytes in choroid-RPE with anti-F4/80 (eBiosciences; 14-4801-82; 1:500 in 1 × PBS) was done as above with choroid-RPE harvested 6 days following laser photocoagulation. Following tissue washing, the complex was incubated with the appropriate secondary antibody and images were captured with a Zeiss microscope (Zeiss, Chester, VA, United States) in digital format. The total area (in μm2) of each individual CNV or mononuclear phagocyte recruitment area was measured using Image J software (National Institute of Mental (NIH; see text footnote 1).
Treatment with caffeine or istradefylline was as follows: 9 days before laser photocoagulation (day 9) male and female C57BL/6j mice were given caffeine citrate 20 mg/kg (in 5% dextrose) or vehicle (5% dextrose) by gavage (10 mice for each group). Subsequent doses of caffeine citrate were 10 mg/kg. Caffeine or vehicle was administered the next 4 days (day 8 to 5). Then, the mice received caffeine or vehicle at days 1 and2 (excluding weekends) prior to laser. Following laser photocoagulation, the mice received caffeine or vehicle by gavage daily excluding weekends for the next 2 weeks. Mice received istradefylline 3 mg/kg (in 0.3% Tween 80, 10% sucrose in saline) or vehicle by gavage using the same dosing times prior and following laser photocoagulation. For regression studies, the mice received caffeine by gavage at 20 mg/kg on day 5 following laser photocoagulation and 10 mg/kg caffeine until choroid-RPE harvest at 2 weeks post-laser photocoagulation. Areas of neovascularization were assessed as described above.
RNA Purification and Real-Time qPCR Analysis
Retinas and choroidal/RPE tissues, as well as various cell types, were lysed and homogenized in Trizol (Invitrogen, San Diego, CA, United States) reagent. Total RNA was extracted using RNeasy mini kit (Qiagen, Maryland, CA, United States) and 1 μg total RNA was used for cDNA synthesis with an RNA to cDNA EcoDry Premix (TaKaRa, Mountain View, CA, United States). For qPCR, cDNA (1 μl each diluted 1:10) was used, and assays were performed in triplicate of three biological replicates using the Mastercycler Realplex (Eppendorf) and TB-Green advantage qPCR Premix (TakaRa). Amplification parameters were as follows: 95°C for 2 min; 40 cycles of amplification (95°C for 15 s and 60°C for 40 s); dissociation curve step (95°C for 15 s, 60°C for 15 s, 95°C for 15 s). We used the primers noted in Table 1. Standard curves were generated from known quantities of each target gene with linearized plasmid DNA. We used 10 times dilution series for each known target, which we amplified using TB-Green qPCR. The linear regression line for DNA (ng) was assessed from relative fluorescent units (RFU) at a threshold fluorescence value (Ct). Gene targets were quantified from cell extracts by comparing the RFU at the Ct to the standard curve and normalized by the simultaneous amplification of RpL13a, a housekeeping gene.
Statistical Analysis
The differences between groups were evaluated with a one-way ANOVA and Dunnett’s multiple comparison test using Prism 8.0 (GraphPad). The comparison between two samples was confirmed with a t-test (paired or unpaired) or non-parametric tests (Mann–Whitney U) depending on whether the data followed a Gaussian distribution as determined by normality tests (D’Agostino and Pearson). Mean ± standard deviation is shown. p < 0.05 was considered significant.
Results
Adenosine Receptor Expression in Various Ocular Cells, Retina, and Choroid/Retinal Pigment Epithelium Tissues
The expression and impact of the various AR in retina and choroid, as well as ocular vascular and non-vascular cells has not been well studied. To gain an appreciation of their expression, we utilized retina or choroid/RPE tissues and various ocular cell types derived from mouse retina and choroid (Figure 1). Adora1 and Adora2b were expressed in choroid/RPE, but their expression was more abundant in the retina, whereas Adora2a expression low in the retina, but was more prominent in the choroid/RPE. Adora2a expression was low in the retina, and Adora1 and Adora2b were expressed in choroid/RPE (Figure 1A). Consistent with the tissue expression patterns, Adora1 expression was mainly noted in microglia, retinal EC, and PC; minimal expression was observed in retinal AC, ChEC, ChPC, ChMC, and RPE cells. The expression of Adora2a was concentrated in RPE cells in contrast to Adora2b, which had more ubiquitous expression (highest in choroidal EC, PC, RPE, and MC). The level of Adora3 was the lowest of all the receptors examined with great variation among all samples (Figure 1B and Supplementary Figure 1). A similar pattern of AR expression was observed in human retina, choroid/RPE, retinal EC, and RPE cells (Figures 1C,D). Thus, AR were expressed to various degrees in all the ocular tissues and cell types examined, with prominent expression of Adora1 in microglia, Adora2a in RPE, and Adora2b in choroidal EC, PC, RPE, and MC, in similar patterns in both mouse and human samples. These results were further confirmed by immune staining of mouse and human eye sections using AR antibodies to A1, A2A and A2B (Supplementary Figure 2).
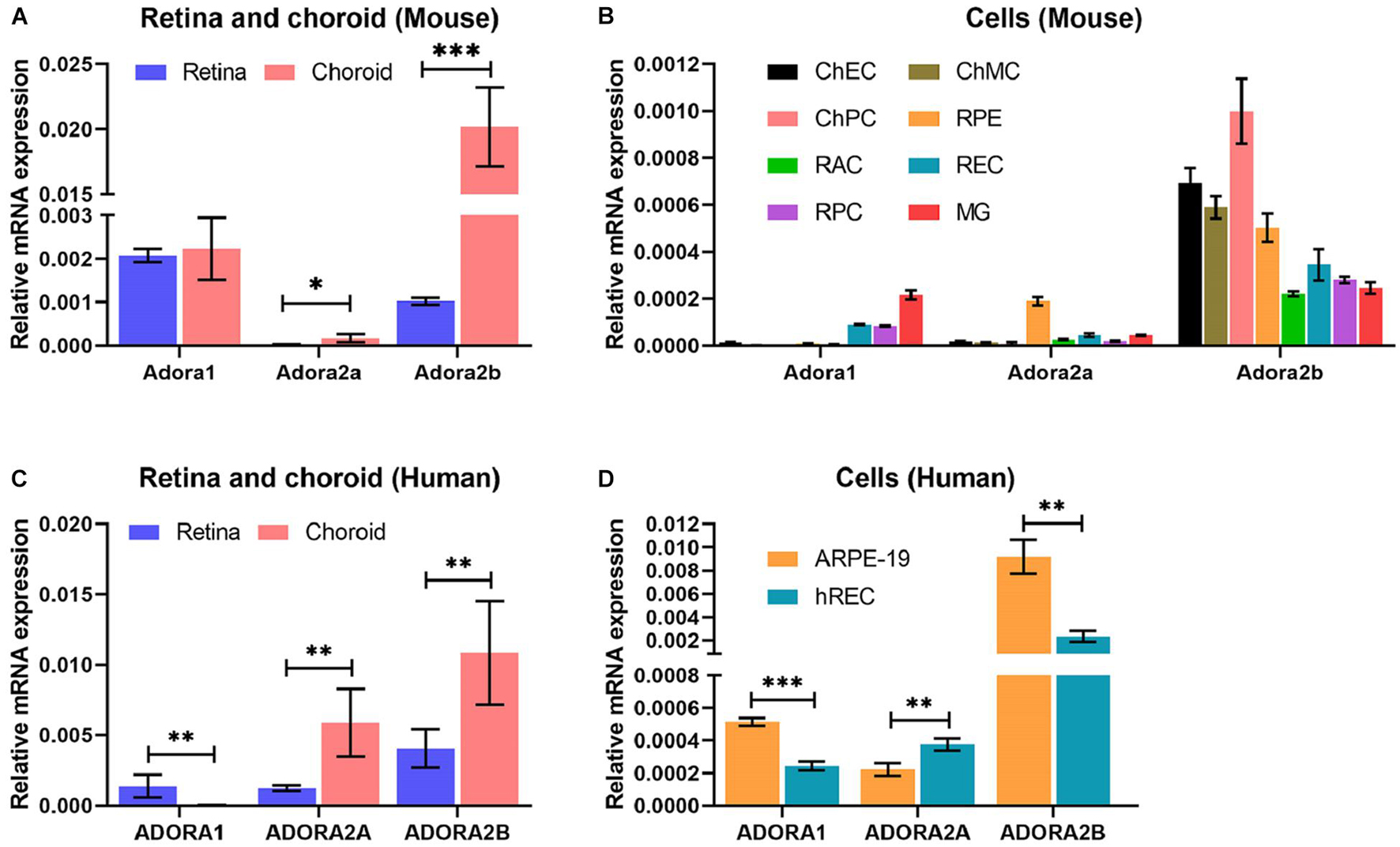
Figure 1. Adenosine receptor expression in ocular cells and tissues. Expression of adenosine receptors (AR), A1 (Adora1), A2A (Adora2a), A2B (Adora2b), and A3 (Adora3) in murine ocular tissues (A) and cells (B) prepared from 2-month-old mice (tissues) and 1-month-old mice (cells) and human ocular tissues (C) and cells (D) were determined by qPCR. These included retinal and choroid/RPE tissues (A) and retinal microglia (MG), retinal astrocytes (RAC), choroidal endothelial cells (ChEC), choroidal pericytes (ChPC), choroidal melanocytes (ChMC), retinal endothelial cells (REC), retinal pericytes (RPC), and retinal pigment epithelium (RPE) cells. Please note highest A1 expression in microglia, A2A expression in RPE cells, and A2B in ChEC, ChPC, ChMC, and RPE cells. The A3 level was very low in all cells examined. The retina and choroid/RPE tissues showed higher A1 and A2B. A similar expression pattern was noted in human tissues (C), and REC and RPE (ARPE19) cells. (*P < 0.05, **P < 0.01, ***P < 0.001).
Caffeine Exhibits Minimal Cytotoxicity in Various Ocular Cell Types
We examined the impact of various caffeine concentrations on viability of ChEC, ChPC, ChMC, RPE cells, and microglial cells. Figure 2 shows that caffeine up to 400 μM had no significant effect on the viability of these cells. We noted a significant increase in the viability of ChEC incubated with caffeine from 200 to 1,600 μM. However, caffeine at 800 μM resulted in a significant decrease in viability of microglial cells. Similarly, caffeine at 1,600 μM significantly reduced the viability of ChPC, ChMC, and microglial cells.
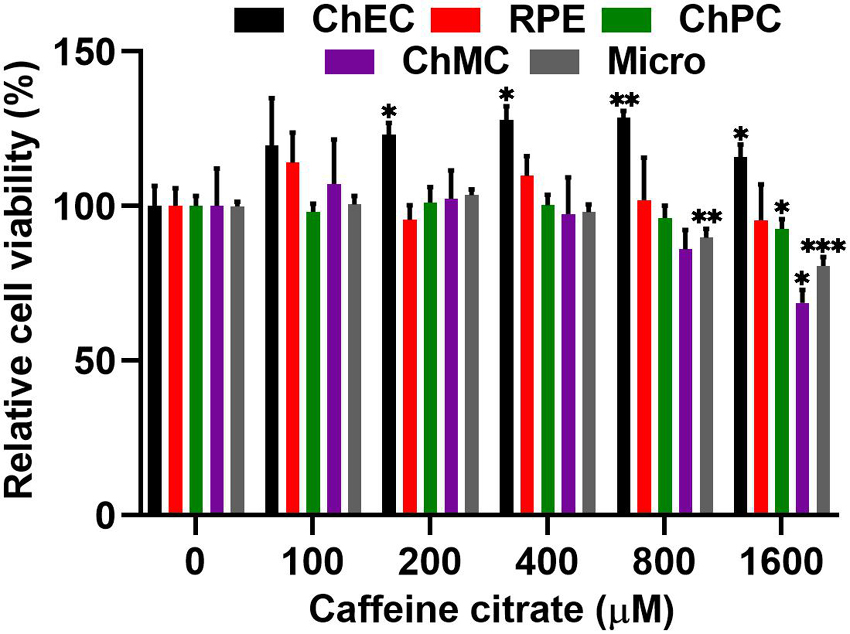
Figure 2. Minimal toxicity was noted in ocular cells incubated with different concentrations of caffeine. Cell viability relative to control cells, was assessed after 48 h of incubation with different concentrations of caffeine as detailed in Methods. Each experiment was done in triplicate and repeated with at least two different isolation of the desired cells (*p < 0.05, **p < 0.01, ***p < 0.001).
Caffeine Inhibits Retinal and Choroidal Endothelial Cell Migration
Optimal EC migration is an important component for the process of normal or pathological angiogenesis. Here, we assessed the impact caffeine, an AR antagonist, and istradefylline an adenosine A2A receptor antagonist, have on migration of retinal and choroidal EC (Figures 3A–C). Caffeine at 400 μM decreased retinal and choroidal EC migration, while 200 μM had no significant effect. Istradefylline did not significantly impact choroidal and retinal EC migration at 1 or 10 μM concentrations (Figures 3D,E).
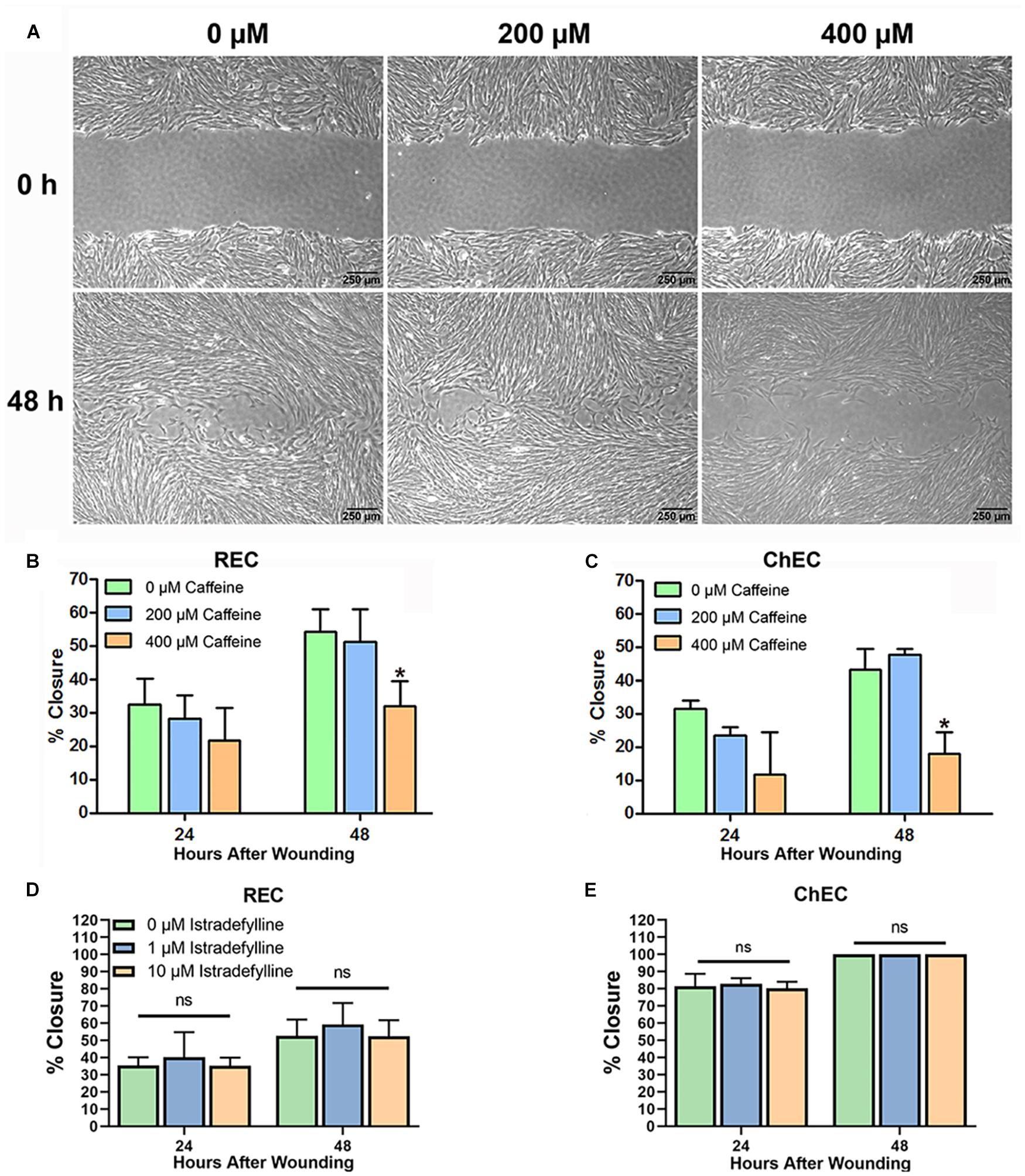
Figure 3. Caffeine mitigates choroidal and retinal endothelial cell migration. Migration of retinal endothelial cells (REC) and choroidal endothelial cells (ChEC) incubated with caffeine was determined using a scratch wound assay and monitored by phase microscopy. The wound images from ChEC are shown in panel (A). The percent wound closure was quantified using ImageJ at 24 and 48 h compared with 0 h [(B) retinal EC; (C) ChEC]. Please note significant wound closure with vehicle while cells are incubated with 400 μM caffeine lagged behind. These caffeine concentrations had no effect on viability after 48 h (Figure 2). Incubation of REC (D) and ChEC (E) with 1 or 10 μM istradefylline did not significantly inhibit their migration. The treatments in panel (E) are identical to those in panel (D). (*P < 0.05).
Ex vivo Choroid/Retinal Pigment Epithelium Explant Sprouting Angiogenesis Is Inhibited by Caffeine
To confirm changes in migration equated with altered sprouting capacity, we next used an ex vivo choroid/RPE sprouting assay. We have found the ex vivo choroid/RPE sprouting assay to be a very reproducible measure of sprouting angiogenesis, since it maintains the local interactions of EC with neighboring pericytes and RPE cells (Shao et al., 2013). We prepared choroid/RPE explants and assessed sprouting of ex vivo choroid/RPE cultures in the presence of vehicle, caffeine (400 μM), or istradefylline (10 μM) (Figure 4). Both caffeine and istradefylline decreased sprouting of choroid-RPE cultures, although to varying extents with caffeine demonstrating a more pronounced decrease in choroid-RPE sprouting. As an alternative method to choroid/RPE cultures to assess sprouting, we also utilized a thoracic aorta-sprouting assay. Thoracic aortas removed from C57BL/6j mice were embedded in Matrigel and incubated with vehicle, caffeine (200 μM), or istradefylline (10 μM) (Figure 5 and Supplementary Figure 3). Both caffeine and istradefylline inhibited sprouting of the embedded aortic rings. Thus, caffeine and istradefylline appear to effectively inhibit sprouting in ex vivo angiogenesis assays.
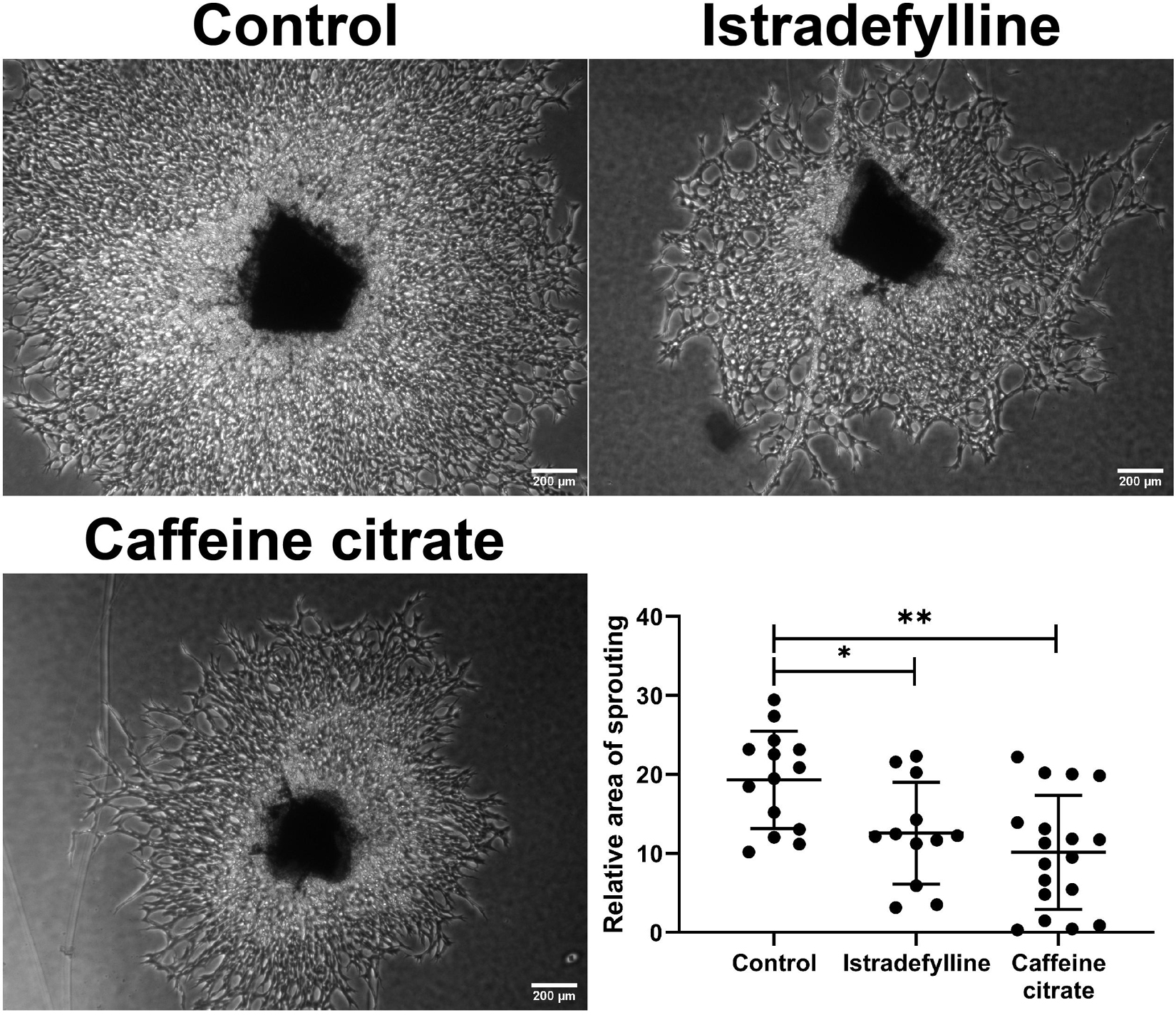
Figure 4. Caffeine mitigates choroid-retinal pigment epithelium (RPE) sprouting in ex vivo culture. Choroid/RPE complex isolated from 4-month-old C57BL/6J mice were grown for 7 days in the presence of vehicle (control), istradefylline (10 μM) or caffeine citrate (400 μM), and photographed. Image J was used to assess sprouting area. Scale bar = 200 μm (∗p < 0.05; ∗∗p < 0.01).
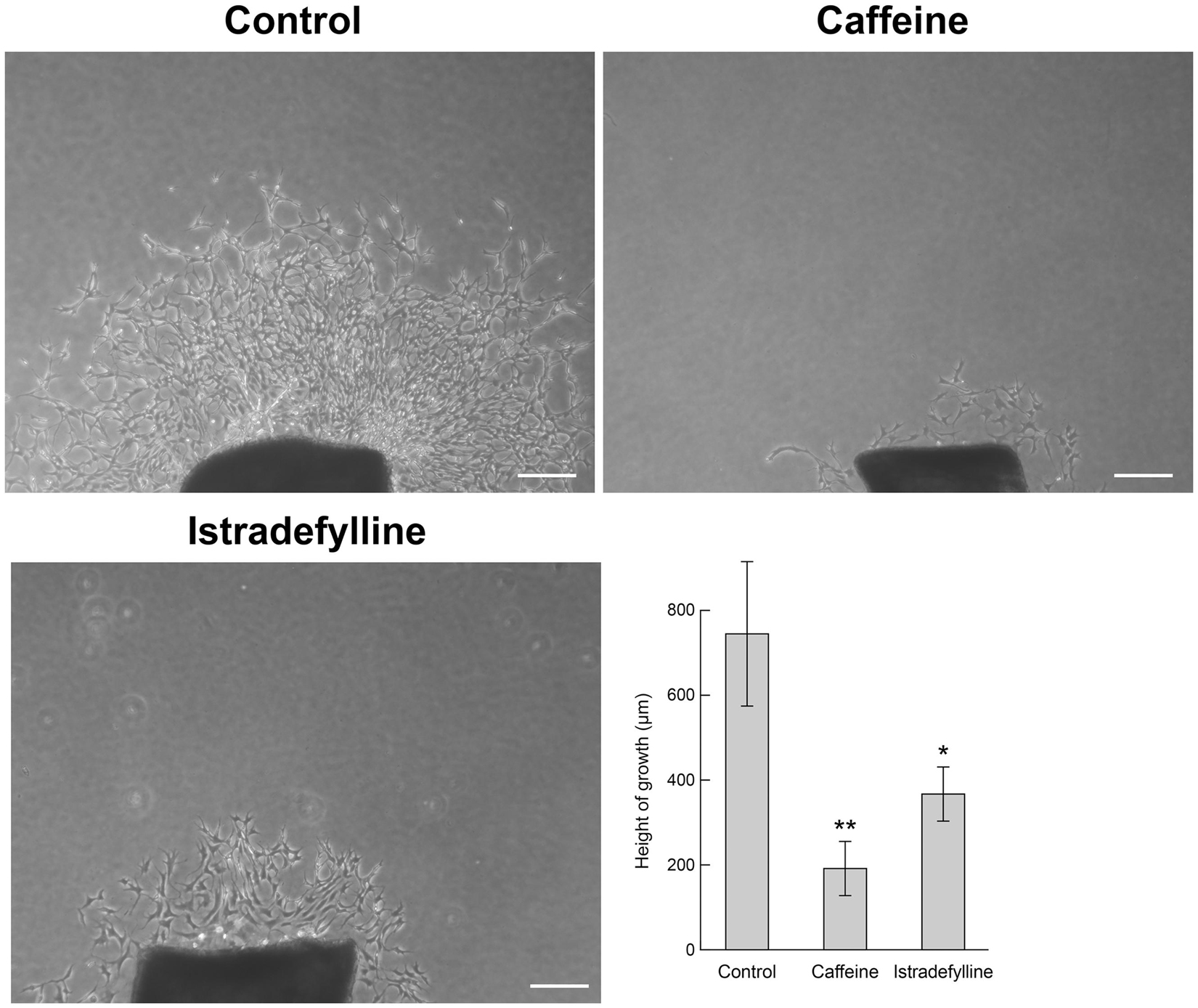
Figure 5. Caffeine and istradefylline mitigate thoracic aortic ring sprouting. Aortic rings were prepared from 3-week-old C57BL/6j mice, embedded in Matrigel in the presence of caffeine (200 μM), istradefylline (selective A2A receptor antagonist; 10 μM) or vehicle. Seven days later, aortic rings were photographed, and the mean area of outgrowths was quantified. Scale bar = 200 μm (∗p < 0.05; ∗∗p < 0.01).
Caffeine Impact on the Expression of Angioinflammatory Mediators of Retinal and Choroidal Endothelial Cells
Next, we assessed whether caffeine modulated expression of genes known to impact angiogenesis and inflammation in choroidal and retinal EC. The choroidal and retinal EC were incubated with caffeine (400 μM) or vehicle (0.5% Dextrose) for 24 h. RNA was isolated and analyzed for expression of various angiogenesis and inflammatory mediators using specific primers (Table 1) by quantitative real time PCR. Figure 6 demonstrates that the expression levels of angiogenesis and inflammatory mediators vary between choroidal and retinal EC, and with caffeine treatment. ChEC expressed lower levels of connective tissue growth factor (Ctgf) than retinal EC and caffeine had a modest, but significant and opposite effect on the level of Ctgf, with expression decreased in retinal EC but increased in ChEC. Compared with retinal EC, ChEC expressed higher levels of pigment epithelium derived factor (Pedf). Caffeine had no significant effect on Pedf expression in ChEC. ChEC expressed similar levels of thrombospondin-1 (Thbs1) as retinal EC. However, Thbs1 level was significantly increased in retinal EC but deceased in ChEC with caffeine treatment. ChEC expressed lower VEGF levels compared with retinal EC; VEGF levels were unaffected by caffeine treatment.
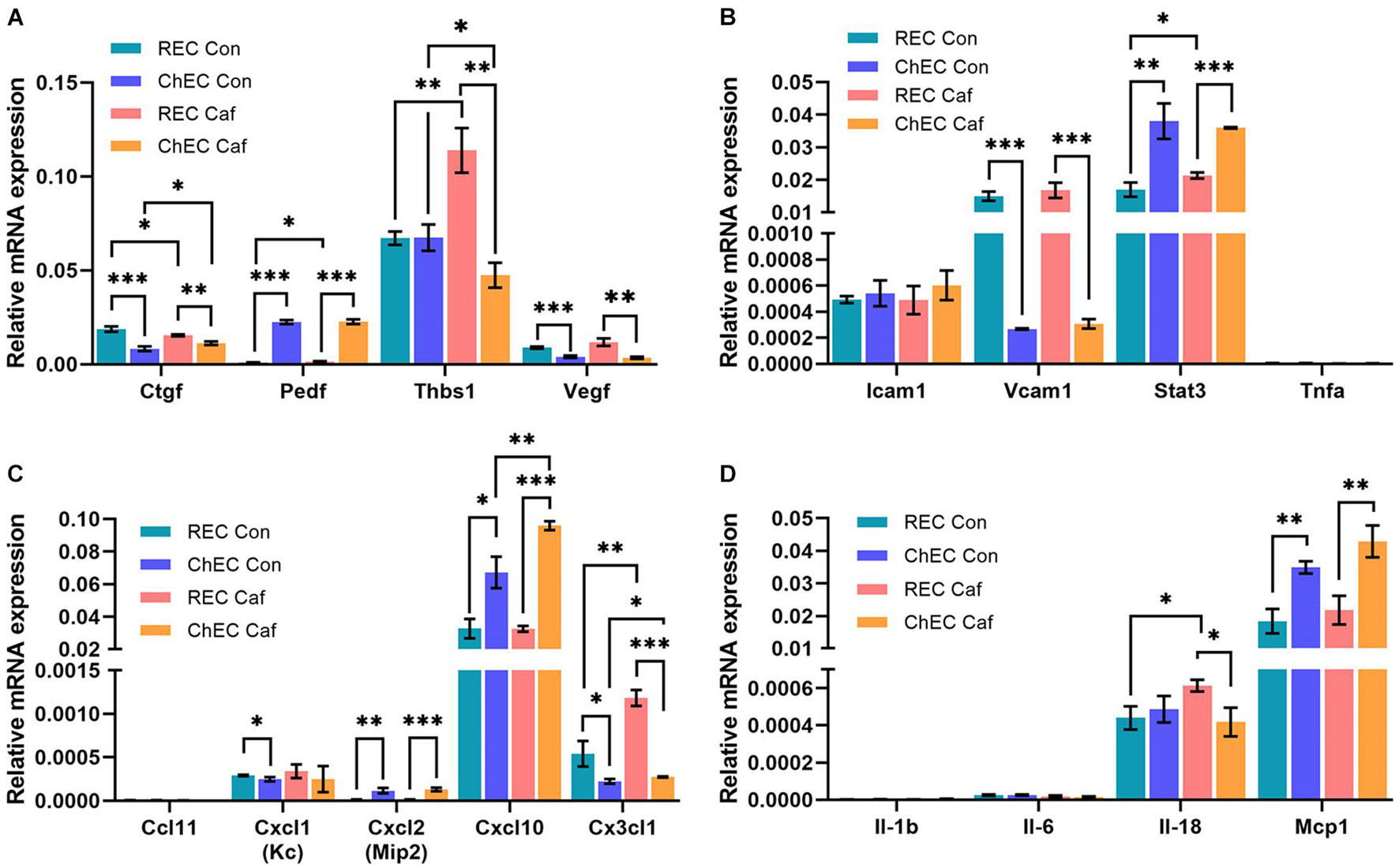
Figure 6. Expression of pro- and anti-angiogenesis and inflammatory mediators in retinal (REC) and choroidal endothelial cells (ChEC) incubated with caffeine. The expression levels of various factors that modulate angiogenesis and inflammation was assessed by qPCR analysis including (A) angiogenesis and inflammatory genes, (B) cell adhesion molecules (C) chemokines, and (D) cytokines. These experiments were done in triplicate and repeated with RNA from two different isolations retinal and choroidal EC (∗p < 0.05; ∗∗p < 0.01; ∗∗∗p < 0.001). RpL13A, 60S ribosomal protein L13a was used as control. Please note a significant increase in thrombospondin-1 (Thbs1) in REC and its decreased expression in ChEC with caffeine. Cxcl10 level increased in ChEC incubated with caffeine. The level of Cx3Cl1 increased in both REC and ChEC. An increase in IL-18 levels was noted in the retina.
ICAM-1, a cell adhesion molecule whose expression is very low on EC, but increases in response to inflammatory mediators, is involved in leukocyte endothelium adhesion and blood-to-tissue transmigration. ChEC and retinal EC showed similar expression of ICAM-1 which was not affected by caffeine. VCAM-1, a cell adhesion molecule that mediates the interaction of endothelial cells and perivascular supporting cells, was expressed at significantly higher levels in retinal EC compared with ChEC, but its expression was not significantly affected by caffeine. Signal transducer and activator of transcription 3 (Stat3) is a transcription factor whose increased expression is associated with enhanced inflammatory conditions. Although Stat3 expression was significantly higher in ChEC compared with retinal EC, its level was not affected by caffeine in ChEC and only modestly, but significantly, increased in retinal EC. Tumor necrosis factor-α (Tnf-α) level was very low in both retinal and choroidal EC with or without caffeine.
We also examined the expression of several cytokines known to impact the inflammatory state of the eye including Ccl11, Cxcl1, Cxcl2, Cxcl10, and Cx3cl1. The level of Ccl11 was undetectable. A similar expression of Cxcl1 was noted in retinal EC and ChEC that was not affected with caffeine treatment. However, its level was modestly, but significantly, lower in ChEC compared with retinal EC. Expression for Cxcl10 was the highest among the chemokines examined; compared with retinal EC, its level was significantly higher in ChEC, especially when incubated with caffeine. Caffeine had no significant effect on Cxcl10 expression in retinal EC. The Cx3cl level was significantly higher in retinal EC compared with ChEC, with levels further increased with caffeine. Caffeine had no effect on Cx3cl1 expression in ChEC. These cells expressed very low levels of IL-1β and IL-6 and were unaffected by caffeine. The level of IL-18 was similar in ChEC and retinal EC. Caffeine treatment caused a modest but significant increase in retinal EC IL-18 level with no effect on ChEC levels. The Mcp1 level was similarly expressed in these cells and significantly increased with caffeine.
Caffeine Mitigates Choroidal Neovascularization in vivo
The choriocapillaris nourishes the outer retina (Lutty et al., 2010). Dysfunction of the choriocapillaris contributes to pathogenesis of AMD and CNV (Biesemeier et al., 2014; Arya et al., 2018; Lutty et al., 2020). Given the ability of caffeine to inhibit migration of ChEC and choroid-RPE explant sprouting, we next determined its impact on CNV following laser photocoagulation-induced rupture of the Bruch’s membrane. Pubertal (2-month-old) or mature (4-month-old) adult C57BL/6j mice received vehicle or caffeine by gavage prior to and following laser photocoagulation-induced rupture of the Bruch’s membrane, as noted in Methods. As a quantitative measure of CNV, ICAM-2 staining was performed 2 weeks later. Caffeine treatment significantly reduced the degree of CNV in both pubertal and mature adult mice (Figures 7A,B).
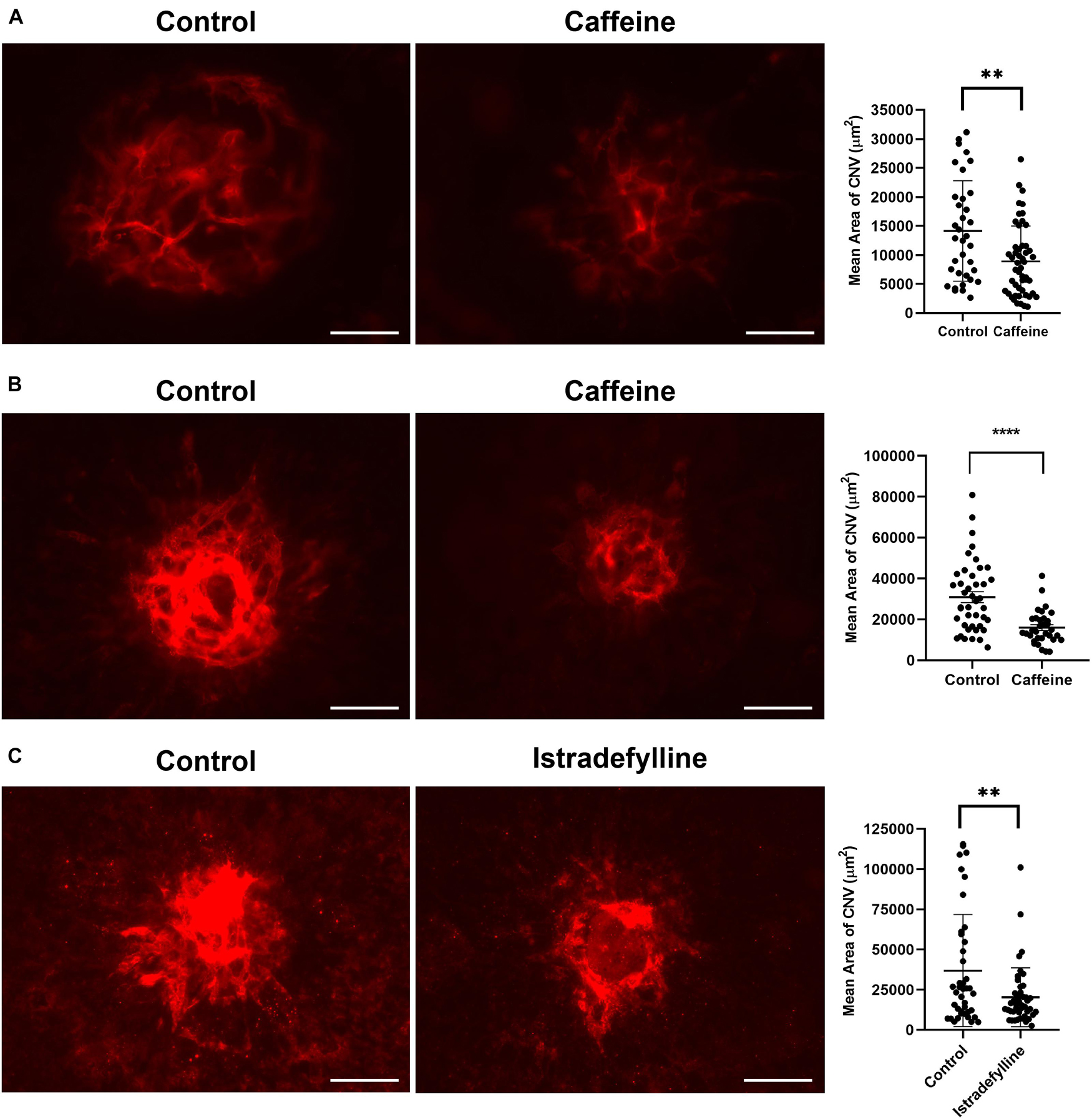
Figure 7. Caffeine mitigates choroidal neovascularization (CNV). The 2-month-old (A) and 4- month-old mice (B) (C57BL/6j male and female) were gavaged with caffeine 20 mg/kg first dose and 10 mg/kg subsequent doses or vehicle starting 5 days prior to laser. Treatments continued until the choroid/RPE was harvested 2 weeks later and stained with anti-ICAM-2. A significant mitigation of choroidal neovascularization (CNV) was noted in mice treated with istradefylline (C). Scale bar = 200 μm (**p < 0.01, ****p < 0.0001; n = 10 mice/group).
Istradefylline inhibited ex vivo sprouting of choroid-RPE and thoracic aortic explants. We assessed whether istradefylline had a similar impact on pubertal mice treated with istradefylline (3 mg/kg) or vehicle by gavage prior to and following laser photocoagulation. Mice that received istradefylline demonstrated decreased area of CNV as noted by ICAM-2 staining (Figure 7C). Thus, our data indicate that antagonism of AR, at least in part through A2A, is an effective means to mitigate CNV.
Next, we determined whether the decrease in CNV with caffeine administration resulted in a decrease in infiltration of mononuclear phagocytes. We have previously examined the temporal distribution of mononuclear phagocytes by staining choroid/RPE flat mounts prepared from mice at different times post laser. As we showed previously, by 3 days after laser, there was a significant accumulation of F4/80+ cells at sites of CNV lesions, which remained the same after 6 days post laser. However, by 14 days after laser, there was more than threefold decrease in the density of F4/80+ cells [(Wang et al., 2012b) and our unpublished data]. To determine the impact of caffeine on density of F4/80+ cells, we harvested choroid/RPE samples 6 days following laser photocoagulation from mice treated with vehicle or caffeine and stained with anti-F4/80. Figure 8 shows a significant decrease in the amounts of F4/80 staining in choroid-RPE samples from caffeine treated mice compared with vehicle-treated mice. Thus, caffeine mitigates the recruitment of mononuclear phagocytes to the laser photocoagulation site and correlates with decreased CNV.
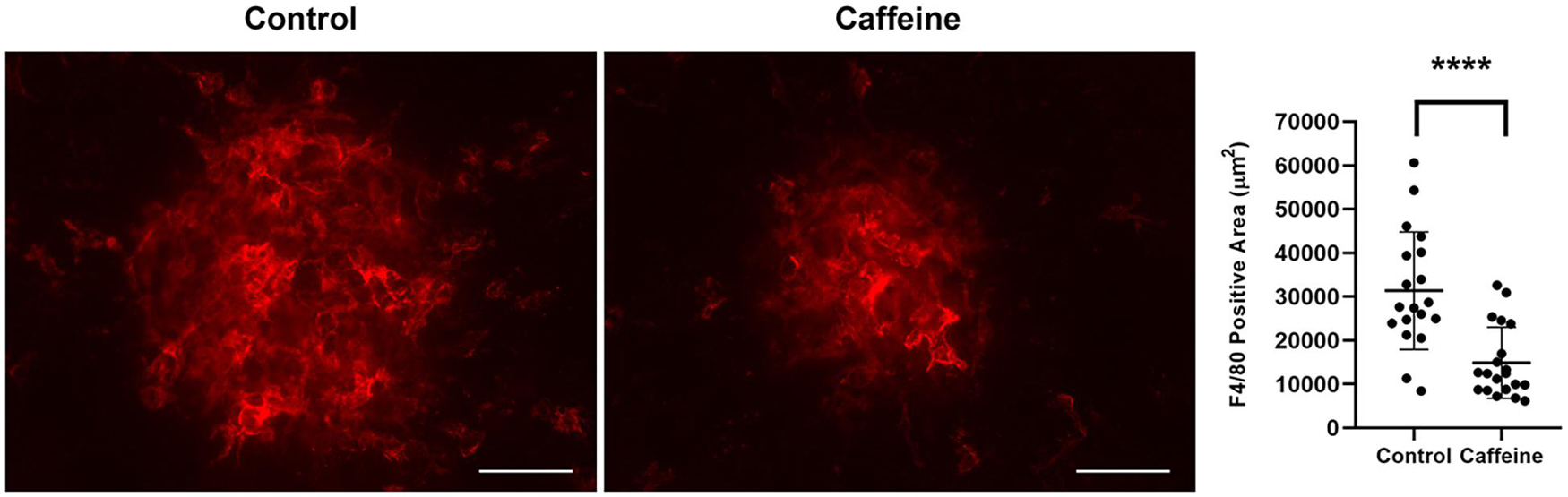
Figure 8. Caffeine treatment reduces macrophage accumulation. Mice (2-month-old C57BL/6j male and female) received caffeine 20 mg/kg first dose and 10 mg/kg subsequent doses or vehicle starting 5 days prior to laser by gavage. Treatments continued until RPE/choroid was harvested 6 days later and stained with anti-F4/80. The degree of macrophage recruitment was assessed as detailed in Methods. Scale bar = 200 μm (****p < 0.0001; n = 10 mice/group).
Caffeine Treatment Impact on Choroidal Neovascularization Regression
CNV begins forming shortly after laser photocoagulation. Here, we asked whether caffeine treatment could decrease the degree of CNV once establishment had begun, that is to promote CNV regression (Figure 9). Caffeine treatment was begun 5 days following laser photocoagulation. Although caffeine treated mice had a lower level of CNV compared with vehicle, this difference did not reach a significant level. Thus, caffeine treatment did not significantly mitigate further CNV formation or enhance CNV regression in these mice.
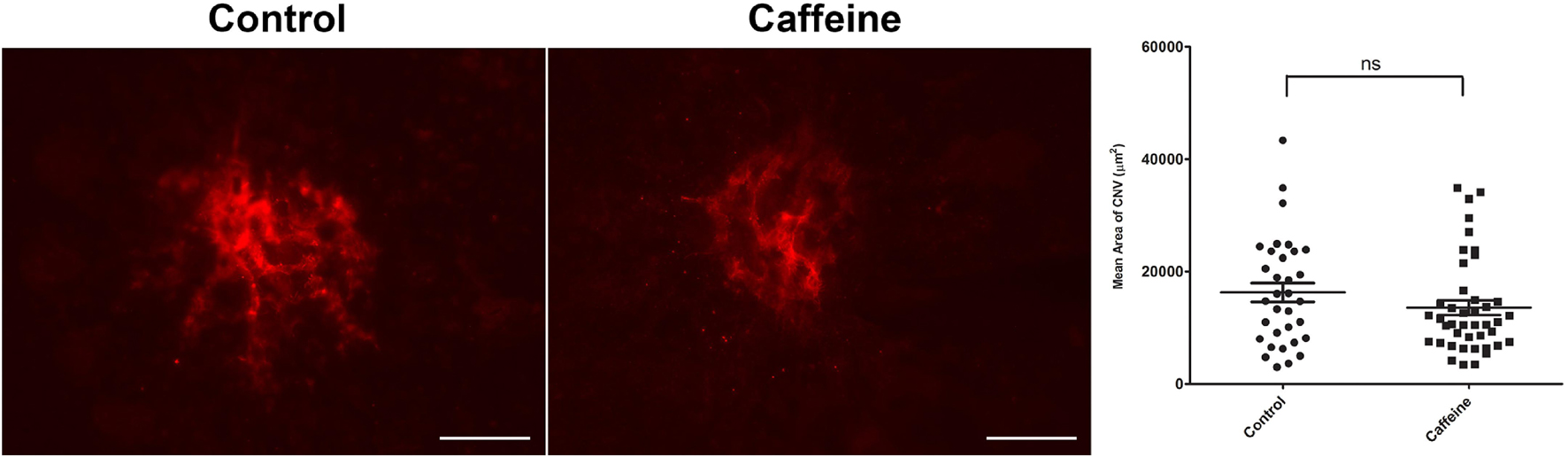
Figure 9. Caffeine is not effective when administered to animals with established choroidal neovascularization (CNV). Mice (2-month-old; C57BL/6j male and female) received caffeine 20 mg/kg first dose and 10 mg/kg subsequent doses or vehicle starting 5 days following laser photocoagulation. Treatments continued until the choroid/RPE was harvested 2 weeks following laser photocoagulation and stained with anti-ICAM-2. Scale bar = 200 μm (ns = not significant; n = 10 mice/group).
The Impact of Laser and Age on Adenosine Receptor Expression in Retina and Choroid/Retinal Pigment Epithelium Tissues
We next addressed whether expression of AR was influenced by laser treatment, and whether chronological age affects AR expression levels. RNA from retina or choroid/RPE samples were prepared from 2-month-old mice and examined for expression of various AR at different times post laser treatment. Figure 10A shows that in the retina, the expression of Adora1 receptor increases significantly a day after laser and stays high even after 1 month. Retinas expressed lower levels of Adora2a and Adora2b whose levels did not change after laser. In contrast, the choroid/RPE samples expressed similar levels of Adora1 receptor and low levels of Adora2a whose expression were not affected at different time post laser (Figure 10B). The expression of Adora2b is nearly 10-fold higher in choroid/RPE compared with the retina, and its expression significantly decreased (Ma and Wong, 2016) after laser in the choroid/RPE, especially after 1 month.
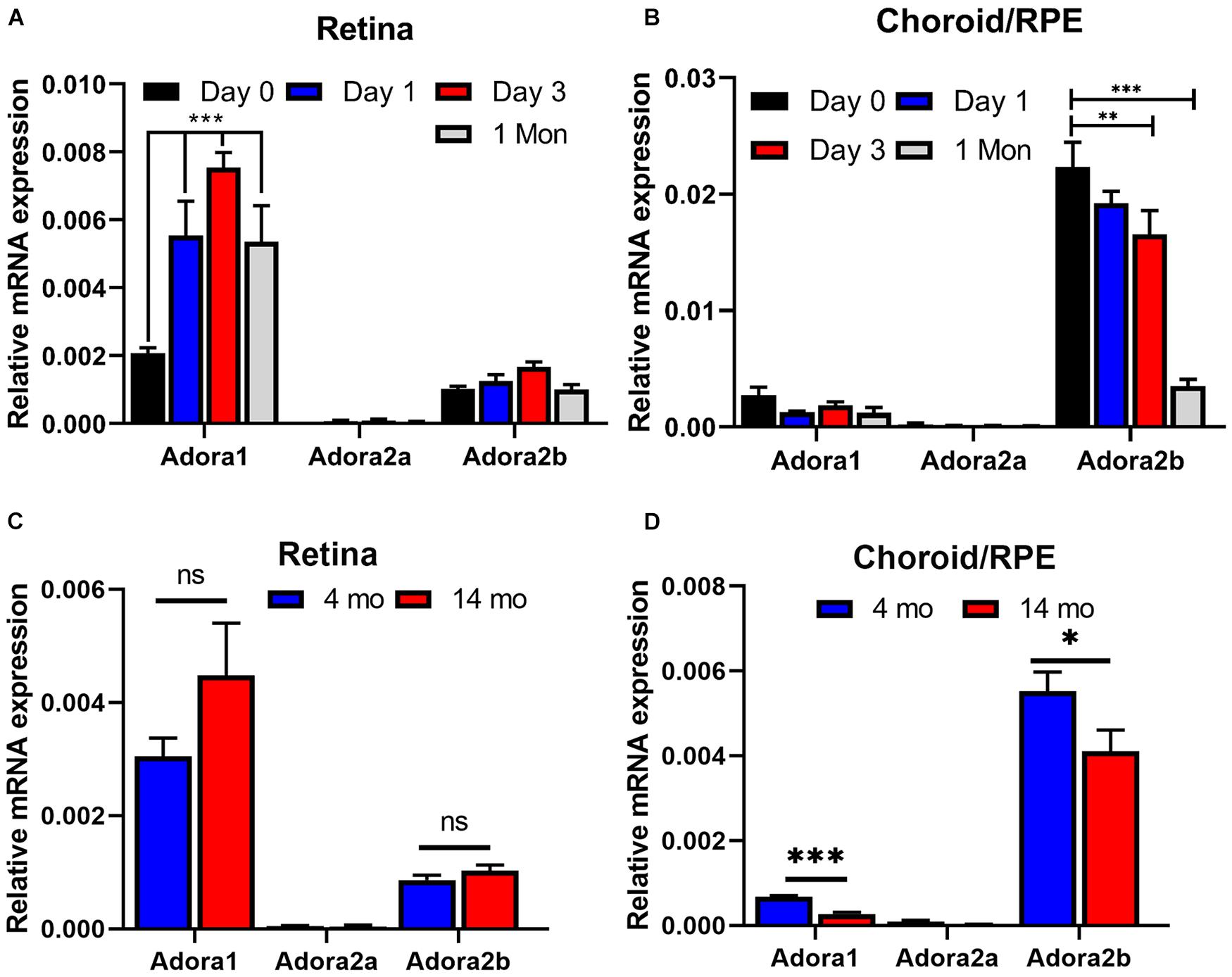
Figure 10. The impact of laser treatment and age on expression of adenosine receptors in retina and choroid. RNA was prepared from retina (A,C) and choroid/RPE (B,D) of mice [2-month-old (A,B), 4-month- and 14-month-old (C,D); C57BL/6j male and female] without laser or 1 day, 3 days, and 1 month post laser. Please note significant up regulation of A1 with laser treatment in the retina, while A2B was decreased in the choroid. Aging minimally affected adenosine receptor (AR) expression in the retina (C). However, the expression of A1 and A2B was significantly decreased with age in choroid RPE (D). (*p < 0.05, **p < 0.01, ***p < 0.001; n = 5 mice/group).
Since AMD pathogenesis and CNV is significantly impacted by aging (Chen et al., 2010; Sachdeva et al., 2014; Edwards et al., 2016; Ma and Wong, 2016), we determined whether the expression of adenosine receptors is affected in young compared with old animals. Retina and choroid/RPE RNA was prepared from 4-month- and 14-month-old mice and the AR levels were assessed by quantitative real time PCR. In the retina of young and old animals, the predominant AR was Adora1, followed by Adora2b (Figure 10C). The level of Adora2a was low, and the expression of these receptors was not significantly different between young and old mice. In the choroid/RPE the predominant receptor was Adora2b, followed by Adora1 and their expression was moderately, but significantly decreased in older animals (Figure 10D). The expression of Adora2a was very low in the choroid/RPE without any effect from aging. Thus, aging has a modest but significant effect on AR expression (Adora1 and Adora2b) in the choroid/RPE.
Caffeine Treatment Does Not Influence Retinal Endothelial Cell or Pericyte Numbers in Developing Retinal Vasculature
The retinal vasculature forms in the mouse after birth with the superficial layer laid down through P7. This is followed by formation of the deep vascular plexus and intermediate layer, which are formed by P21. To assess whether caffeine treatment during retinal vascularization impacts retinal endothelial cell and pericyte numbers, C57BL/6j mice were administered caffeine or vehicle from P1–10 (intraperitoneal doses at P1, 20 mg/kg and P2–10 maintenance dose of 10 mg/kg caffeine or vehicle 5% dextrose). At P21, trypsin digestion of retinal preparations was performed, and the numbers of EC and PC were counted in blinded fashion (Table 2). No significant differences were noted in the number of EC and PC when mice were treated with caffeine compared with vehicle. Thus, caffeine treatment did not impact retinal vascular cell numbers in these studies.
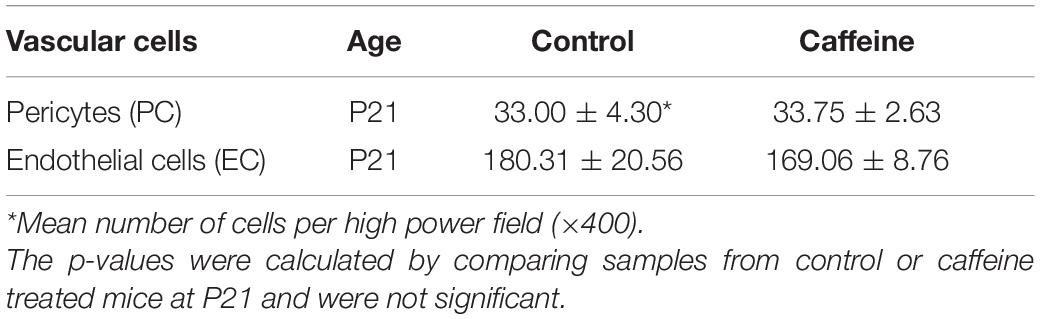
Table 2. Retinal vascular cell density determined in trypsin digest whole mount retina preparations.
Discussion
Age-related macular degeneration is a major cause of vision impairment in the elderly. In its dry form (atrophic AMD) dysfunction and loss of retinal pigment epithelium leads to degeneration of photoreceptors and central vision impairment. The major loss of vision in AMD patients is associated with the wet/exudative form of the disease where growth of abnormal vessels into the retina, and its associated fibrosis and scar formation, lead to vision loss (Farnoodian et al., 2017; Handa et al., 2019). AMD is a multifactorial disease with aging as a major contributing factor. Inflammation is known to contribute to normal aging, and it is identified as an important factor in the pathogenesis of AMD. Adenosine, generated by catabolism of ATP is important in retinal vascular development and neovascularization, and modulation of inflammation and fibrosis (Afzal et al., 2003; Lutty and McLeod, 2003; Hua et al., 2007; Peng et al., 2008; Ryzhov et al., 2008; Zhang J. et al., 2017). The major activity of adenosine is mediated through ARs that are expressed on many cell types including vascular and inflammatory cells (Santiago et al., 2020). Excessive adenosine and AR-mediated signaling under hypoxic conditions contributes to ischemic retinopathy, and thus, are proposed to provide suitable target for therapeutic interventions (Lutty and McLeod, 2003; Zhou et al., 2018). Caffeine, a non-selective adenosine receptor antagonist, has shown effectiveness in mitigating retinal neovascularization (Chen et al., 2017; Zhang S. et al., 2017). In addition, a recent study demonstrated that endothelial expression of A2A is essential for pathological retinal neovascularization during oxygen-induced ischemic retinopathy (Liu et al., 2017). Furthermore, blockade of A2A in microglia diminishes inflammatory responses and protects RPE cell dysfunction and loss of photoreceptor cells in culture (Madeira et al., 2018). However, the contribution of adenosine and AR signaling to pathogenesis AMD, and whether their antagonism by caffeine mitigates CNV, remains unknown. Thus, given the important role of adenosine and its receptors in the regulation of inflammation, neovascularization and fibrosis, and their antagonism by caffeine, we proposed these receptor/ligand signaling axis could play a vital role in the pathogenesis of exudative AMD through modulation of angioinflammatory pathways, and can be mitigated by caffeine.
Adenosine stimulates the proangiogenic properties of retinal EC including proliferation, migration, and capillary morphogenesis. These activities are likely mediated through the A2A and/or A2B AR (Lutty et al., 1998; Afzal et al., 2003; Lutty and McLeod, 2003). The binding affinity to adenosine receptors for adenosine are A1 > A3 > A2A > A2B, and for caffeine are A2A > A1 and A2B > A3 (nM for adenosine and μM for caffeine) (Kolahdouzan and Hamadeh, 2017). The mechanisms by which adenosine or its antagonist affect the angiogenic properties of retinal and choroidal neovascularization related cells remain unknown. We have established cultures of cells from retina and choroid with important roles in neovascularization. The current study demonstrates that these cells express AR namely A1, A2A, A2B, and A3 at varying levels. Although the AR signaling pathways have been previously studied in retinal vascular cells, further delineation is required in CNV-related cells (ChEC, ChPC, melanocytes, RPE cells, and microglia). We determined the impact caffeine has on migration and sprouting angiogenesis of CNV-related cells in vitro and ex vivo, and CNV in vivo. Understanding the response of CNV related cells to adenosine signaling, and its antagonism by caffeine, will allow us to delineate the cell autonomous mechanisms involved. This knowledge will facilitate the development of new therapeutics to circumvent the current incomplete therapy responses to anti-VEGF, and/or use for adjunct therapy.
In this study, choroidal related cells including ChEC, ChPC, ChMC, RPE, and microglial cells were used for all the experiments performed unless otherwise noted. Our laboratory routinely isolates murine choroidal and retinal related cells (Scheef et al., 2009; Fei et al., 2014), which were used here. These cells have been extensively characterized and shown to express all the appropriate cell type specific markers (Scheef et al., 2009; Fei et al., 2014). Using these cells, we showed their AR expression patterns, as well as in whole retina and choroid/RPE tissues. We showed A1 and A2B are predominantly expressed in the retina, while A2A is predominant in choroid/RPE with significant expression of A1 and A2B. A3 levels were very low (near the limit of detection; not shown) and highly variable. Consistent with these expression patterns, we noted microglial cells express predominantly A1 with modest expression in retinal EC and PC. A2A expression was predominant in RPE cells with some expression in other cells from retina and choroid. A2B was more predominant in ChEC, ChPC, ChMC, and RPE cells, with some expression in other cells from retina and choroid. A3 expression was uniformly very low in all the cells from mouse retina and choroid. Given the fact that A2A is generally recognized as a caffeine receptor, which couples to stimulatory G proteins (Gαs/Olf) to activate adenylate cyclase and elevating cellular cAMP levels, we proposed its antagonism in the choroid/RPE could mitigate CNV through its anti-angiogenic and anti-inflammatory activities.
Adenosine stimulates the proangiogenic properties of retinal EC including proliferation, migration, and capillary morphogenesis. These activities were mediated through the A2A and/or A2B AR (Lutty et al., 1998; Afzal et al., 2003; Lutty and McLeod, 2003). The mechanism by which adenosine or its antagonist affects the angiogenic properties of CNV related cells remains unknown. To further confirm changes in the proangiogenic properties of CNV-related cells in response to caffeine, we examined the impact of caffeine and istradefylline on retinal and choroidal EC migration in culture. Although caffeine significantly reduced the migration of retinal and choroidal EC, istradefylline had no significant effect on the migration of these cells. This could be attributed to variation in AR expression patterns in these cells. It is also possible that higher concentrations of istradefylline might be needed. Future experiments will further address these possibilities.
We next used the ex vivo choroid/RPE and aorta explant sprouting assays to further address the impact of AR antagonism on sprouting angiogenesis. The choroid/RPE sprouting assay maintains local interactions of EC with neighboring cells (Shao et al., 2013). Our data demonstrated that decreased sprouting of choroid/RPE explants in the presence of caffeine and istradefylline, occurred at least in part through antagonism of A2A AR. However, the impact of caffeine was more significant than istradefylline, perhaps through antagonism of other AR receptors in addition to the A2A, which is specifically targeted by istradefylline. Similar results were observed in the aortic sprouting assay. Thus, these effects of caffeine on the vasculature sprouting are not specific to retina and choroid.
Given the proposed anti-inflammatory and anti-angiogenesis activity of caffeine, we examined the expression of various genes with pro- and anti-angiogenesis and inflammatory activity that could be impacted by caffeine treatment. We noted a significant impact of caffeine on expression of Ctgf, Pedf, and Thbs1 in choroidal and retinal EC. Caffeine significantly decreased Ctgf expression in retinal EC, while it increased Pedf and Thbs1 expression. In contrast caffeine increased Ctgf expression, but decreased Thbs1 expression in ChEC, without affecting Pedf expression. The Vegf levels were lower in ChEC compared with retinal EC and caffeine had no effect on its expression. The increased Pedf and Thbs1 expression in retinal EC are consistent with the antimigratory effect of caffeine on retinal EC. We previously showed that Thbs1-deficiency results in enhanced proangiogenic activity of retinal EC, but not ChEC. Thbs1-deficient ChEC were less migratory compared with wild type cells (Wang et al., 2006; Fei et al., 2014). Thus, the decreased Thbs1 expression in ChEC is consistent with their reduced migration in the presence of caffeine (Wang et al., 2006; Fei et al., 2014; Falero-Perez et al., 2017; Farnoodian et al., 2018).
Although the expression of ICAM-1 and VCAM-1 were not affected by caffeine treatment, retinal EC expressed significantly higher levels of VCAM-1 compared with ChEC. This could be linked to the importance of EC and PC interactions in the retinal vasculature and development of blood-retina barrier (Garmy-Susini et al., 2005; Wang et al., 2012a). Among the chemokine examined Cxcl10 and Cx3cl1 were expressed at significantly higher levels and their expression was affected by caffeine treatment. The expression of Cxcl10 was significantly higher in ChEC compared with retinal EC, whose level was further increased with caffeine. The level of Cx3cl1 was significantly higher in retinal EC compared with ChEC, which was further increased with caffeine. The interaction of Cx3cl1 with its receptor Cx3cr1 on microglial cells plays an important role in neuroretina homeostasis and function (Raoul et al., 2010; Zhang et al., 2012, 2018). IL-18 was expressed at significantly higher levels than IL-1β, IL-6, and TNF-α in these cells, whose expression modestly, but significantly increased in retinal EC, but decreased in ChEC. The decreased level of IL-18 expression in ChEC with caffeine treatment is consistent with caffeine’s anti-inflammatory and protective activity in the choroid. The MCP-1 was expressed at significantly higher level in ChEC with no effect from caffeine on its expression in these cells. Collectively, caffeine treatment affects the expression of pro- and anti-angiogenic and inflammatory factors of the retina and choroid endothelium, which could contribute to the effect of caffeine in mitigating ocular inflammation and angiogenesis. A recent study identified tyrosinase as a major target of caffeine action in modulating the proliferation and expression of various immunomodulatory cytokines and chemokines in melanomas (Tabolacci et al., 2021). The role of choroidal melanocytes in immunomodulation and pathogenesis of nAMD, and their response to caffeine remain a subject of future investigation.
Caffeine and istradefylline were both efficacious in mitigating CNV, with caffeine being significantly more effective, as demonstrated in ex vivo sprouting assays. Mechanistically, this activity of caffeine was mediated by significantly attenuating the recruitment of inflammatory F4/80 + cells into the laser lesions. To determine how caffeine mediates these inhibitory effects requires further exploration to establish which cell types are involved and how they respond to caffeine. Here, we showed that microglial cells isolated from mouse retina predominantly express the A1 and A2B, the receptors known as potential target of caffeine. We showed laser photocoagulation results in a significant upregulation of A1 receptor expression in the retina but not in the choroid, while A2B level decreases in the choroid, but not in the retina. Although aging had no significant impact on expression of A1 and A2B AR in the retina, their expression significantly decreased in choroid/RPE tissues. The decreased expression of these receptors in the choroid/RPE is consistent with increased inflammation noted with aging (Steinle et al., 2009; Xu et al., 2009; Ma and Wong, 2016). Thus, targeting of retinal microglial cells by caffeine through A1 AR may have significant impact on their phenotype preventing their migration to the sites of laser lesions, dampening the pro-inflammatory and angiogenesis activity in CNV lesions (summarized in Figure 11). Further delineation of AR involved in modulating murine choroidal inflammatory and angiogenesis activity will require conditional deletions of specific receptors in microglia or choroidal cells and assessment of the effect of caffeine in mitigating CNV. Identifying conditions that enhance AR expression with or without caffeine may provide further protection from CNV.
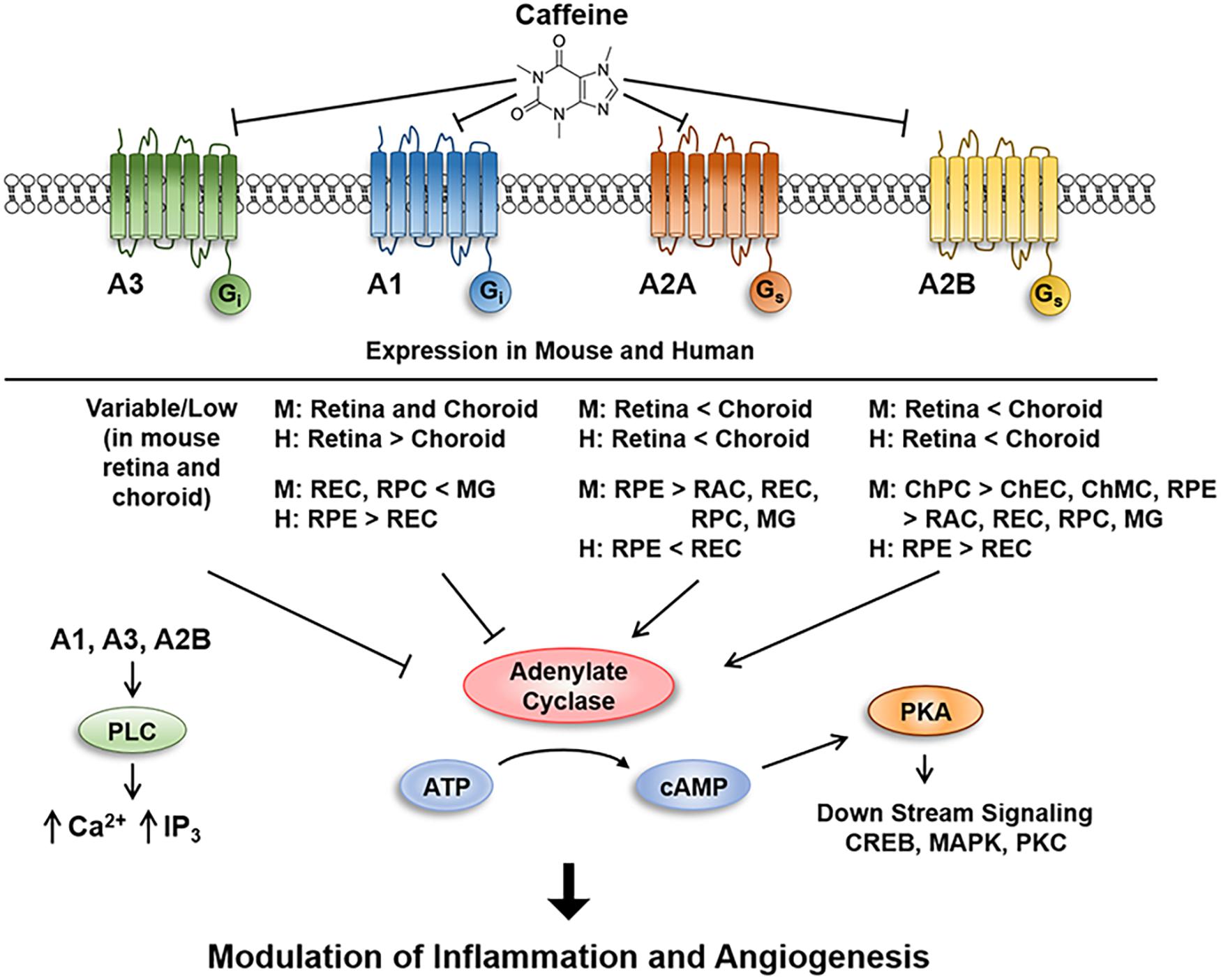
Figure 11. Schematic illustration of caffeine inhibition of signaling through adenosine receptors in retina and choroid. Inhibition of adenosine receptors mitigates sprouting angiogenesis in vitro, ex vivo, and in vivo, in part, by inhibiting the migration of endothelial cells through modulation of pro- and anti-angioinflammatory factors.
Data Availability Statement
The original contributions presented in the study are included in the article/Supplementary Material, further inquiries can be directed to the corresponding author/s.
Ethics Statement
The animal study was reviewed and approved by University of Wisconsin School of Medicine and Public Health Animal Use and Care Committee.
Author Contributions
CS and NS were responsible for the experimental design, conducting experiments, protocol implementation, data analysis and interpretation, and writing and editing the manuscript and for the final approval of the article. Y-SS, IZ, SW, BH, SD, ZG, and DF were responsible for the experiments and data collection. CM and RM helped with the study design and editing of the manuscript. All authors read and approved the final manuscript.
Funding
This work was supported by an unrestricted award from Research to Prevent Blindness to the Department of Ophthalmology and Visual Sciences, Retina Research Foundation, RRF/Daniel M. Albert Chair (P30 EY016665, P30 CA014520, R01 EY026078, and EY030076).
Conflict of Interest
The authors declare that the research was conducted in the absence of any commercial or financial relationships that could be construed as a potential conflict of interest.
Publisher’s Note
All claims expressed in this article are solely those of the authors and do not necessarily represent those of their affiliated organizations, or those of the publisher, the editors and the reviewers. Any product that may be evaluated in this article, or claim that may be made by its manufacturer, is not guaranteed or endorsed by the publisher.
Supplementary Material
The Supplementary Material for this article can be found online at: https://www.frontiersin.org/articles/10.3389/fcell.2021.737426/full#supplementary-material
Footnotes
References
Afzal, A., Shaw, L. C., Caballero, S., Spoerri, P. E., Lewin, A. S., Zeng, D., et al. (2003). Reduction in preretinal neovascularization by ribozymes that cleave the A2B adenosine receptor mRNA. Circ. Res. 93, 500–506. doi: 10.1161/01.res.0000091260.78959.bc
Altinkaynak, H., Ceylan, E., Kartal, B., Keleñ, S., Ekinci, M., and Olcaysu, O. O. (2016). Measurement of choroidal thickness following caffeine intake in healthy subjects. Curr Eye Res. 41, 708–714.
Arya, M., Sabrosa, A. S., Duker, J. S., and Waheed, N. K. (2018). Choriocapillaris changes in dry age-related macular degeneration and geographic atrophy: a review. Eye Vis. (Lond.) 5:22.
Auchampach, J. A. (2007). Adenosine receptors and angiogenesis. Circ. Res. 101, 1075–1077. doi: 10.1161/circresaha.107.165761
Biesemeier, A., Taubitz, T., Julien, S., Yoeruek, E., and Schraermeyer, U. (2014). Choriocapillaris breakdown precedes retinal degeneration in age-related macular degeneration. Neurobiol. Aging 35, 2562–2573. doi: 10.1016/j.neurobiolaging.2014.05.003
Boia, R., Ambrósio, A. F., and Santiago, A. R. (2016). Therapeutic opportunities for caffeine and A2A receptor antagonists in retinal diseases. Ophthalmic Res. 55, 212–218. doi: 10.1159/000443893
Bowser, J. L., Lee, J. W., Yuan, X., and Eltzschig, H. K. (2017). The hypoxia-adenosine link during inflammation. J. Appl. Physiol. (1985) 123, 1303–1320. doi: 10.1152/japplphysiol.00101.2017
Campochiaro, P. A., and Akhlaq, A. (2020). Sustained suppression of VEGF for treatment of retinal/choroidal vascular diseases. Prog. Retin. Eye Res. 83:100921. doi: 10.1016/j.preteyeres.2020.100921
Carmeliet, P., Ferreira, V., Breier, G., Pollefeyt, S., Kieckens, L., Gertsenstein, M., et al. (1996). Abnormal blood vessel development and lethality in embryos lacking a single VEGF allele. Nature 380, 435–439. doi: 10.1038/380435a0
Chen, J. F., Eltzschig, H. K., and Fredholm, B. B. (2013). Adenosine receptors as drug targets–what are the challenges? Nat. Rev. Drug Discov. 12, 265–286. doi: 10.1038/nrd3955
Chen, J. F., Zhang, S., Zhou, R., Lin, Z., Cai, X., Lin, J., et al. (2017). Adenosine receptors and caffeine in retinopathy of prematurity. Mol. Aspects Med. 55, 118–125. doi: 10.1016/j.mam.2017.01.001
Chen, M., Muckersie, E., Forrester, J. V., and Xu, H. (2010). Immune activation in retinal aging: a gene expression study. Invest. Ophthalmol. Vis. Sci. 51, 5888–5896. doi: 10.1167/iovs.09-5103
Cronstein, B. N. (2010). Caffeine, a drug for all seasons. J. Hepatol. 53, 207–208. doi: 10.1016/j.jhep.2010.02.025
Dervişoğulları, M. S., Totan, Y., Yüce, A., and Kulak, A. E. (2016). Acute effects of caffeine on choroidal thickness and ocular pulse amplitude. Cutan. Ocul. Toxicol. 35, 281–286. doi: 10.3109/15569527.2015.1104330
Edwards, M. M., Mcleod, D. S., Bhutto, I. A., Villalonga, M. B., Seddon, J. M., and Lutty, G. A. (2016). Idiopathic preretinal glia in aging and age-related macular degeneration. Exp. Eye Res. 150, 44–61. doi: 10.1016/j.exer.2015.07.016
Falero-Perez, J., Park, S., Sorenson, C. M., and Sheibani, N. (2017). PEDF expression affects retinal endothelial cell proangiogenic properties through alterations in cell adhesive mechanisms. Am. J. Physiol. Cell Physiol. 313, C405–C420.
Farnoodian, M., Kinter, J. B., Yadranji Aghdam, S., Zaitoun, I., Sorenson, C. M., and Sheibani, N. (2015). Expression of pigment epithelium-derived factor and thrombospondin-1 regulate proliferation and migration of retinal pigment epithelial cells. Physiol. Rep. 3:e12266. doi: 10.14814/phy2.12266
Farnoodian, M., Sorenson, C. M., and Sheibani, N. (2018). PEDF expression affects the oxidative and inflammatory state of choroidal endothelial cells. Am. J. Physiol. Cell Physiol. 314, C456–C472.
Farnoodian, M., Wang, S., Dietz, J., Nickells, R. W., Sorenson, C. M., and Sheibani, N. (2017). Negative regulators of angiogenesis: important targets for treatment of exudative AMD. Clin. Sci. (Lond.) 131, 1763–1780. doi: 10.1042/cs20170066
Fei, P., Zaitoun, I., Farnoodian, M., Fisk, D. L., Wang, S., Sorenson, C. M., et al. (2014). Expression of thrombospondin-1 modulates the angioinflammatory phenotype of choroidal endothelial cells. PLoS One 9:e116423. doi: 10.1371/journal.pone.0116423
Ferrara, N., Carver-Moore, K., Chen, H., Dowd, M., Lu, L., O’shea, K. S., et al. (1996). Heterozygous embryonic lethality induced by targeted inactivation of the VEGF gene. Nature 380, 439–442. doi: 10.1038/380439a0
Fields, M. A., Del Priore, L. V., Adelman, R. A., and Rizzolo, L. J. (2020). Interactions of the choroid, Bruch’s membrane, retinal pigment epithelium, and neurosensory retina collaborate to form the outer blood-retinal-barrier. Prog. Retin. Eye Res. 76:100803. doi: 10.1016/j.preteyeres.2019.100803
Garmy-Susini, B., Jin, H., Zhu, Y., Sung, R. J., Hwang, R., and Varner, J. (2005). Integrin alpha4beta1-VCAM-1-mediated adhesion between endothelial and mural cells is required for blood vessel maturation. J. Clin. Invest. 115, 1542–1551. doi: 10.1172/jci23445
Grutzmacher, C., Park, S., Elmergreen, T. L., Tang, Y., Scheef, E. A., Sheibani, N., et al. (2010). Opposing effects of bim and bcl-2 on lung endothelial cell migration. Am. J. Physiol. Lung Cell Mol. Physiol. 299, L607–L620.
Handa, J. T., Bowes Rickman, C., Dick, A. D., Gorin, M. B., Miller, J. W., Toth, C. A., et al. (2019). A systems biology approach towards understanding and treating non-neovascular age-related macular degeneration. Nat. Commun. 10:3347.
Hasko, G., and Cronstein, B. N. (2004). Adenosine: an endogenous regulator of innate immunity. Trends Immunol. 25, 33–39. doi: 10.1016/j.it.2003.11.003
Hasko, G., Pacher, P., Deitch, E. A., and Vizi, E. S. (2007). Shaping of monocyte and macrophage function by adenosine receptors. Pharmacol. Ther. 113, 264–275. doi: 10.1016/j.pharmthera.2006.08.003
Hernandez-Zimbron, L. F., Zamora-Alvarado, R., Ochoa-De La Paz, L., Velez-Montoya, R., Zenteno, E., Gulias-Canizo, R., et al. (2018). Age-related macular degeneration: new paradigms for treatment and management of AMD. Oxid. Med. Cell Longev. 2018:8374647.
Hua, X., Kovarova, M., Chason, K. D., Nguyen, M., Koller, B. H., and Tilley, S. L. (2007). Enhanced mast cell activation in mice deficient in the A2b adenosine receptor. J. Exp. Med. 204, 117–128. doi: 10.1084/jem.20061372
Kolahdouzan, M., and Hamadeh, M. J. (2017). The neuroprotective effects of caffeine in neurodegenerative diseases. CNS Neurosci. Ther. 23, 272–290. doi: 10.1111/cns.12684
Kwak, N., Okamoto, N., Wood, J. M., and Campochiaro, P. A. (2000). VEGF is major stimulator in model of choroidal neovascularization. Invest. Ophthalmol. Vis. Sci. 41, 3158–3164.
Lavine, J. A., Farnoodian, M., Wang, S., Darjatmoko, S. R., Wright, L. S., Gamm, D. M., et al. (2017). beta2-adrenergic receptor antagonism attenuates CNV through inhibition of VEGF and IL-6 expression. Invest. Ophthalmol. Vis. Sci. 58, 299–308. doi: 10.1167/iovs.16-20204
Liu, Z., Yan, S., Wang, J., Xu, Y., Wang, Y., Zhang, S., et al. (2017). Endothelial adenosine A2a receptor-mediated glycolysis is essential for pathological retinal angiogenesis. Nat. Commun. 8:584.
Lopez, C. D., Bekisz, J. M., Corciulo, C., Mediero, A., Coelho, P. G., Witek, L., et al. (2018). Local delivery of adenosine receptor agonists to promote bone regeneration and defect healing. Adv. Drug Deliv. Rev. 146, 240–247. doi: 10.1016/j.addr.2018.06.010
Lutty, G. A., Hasegawa, T., Baba, T., Grebe, R., Bhutto, I., and Mcleod, D. S. (2010). Development of the human choriocapillaris. Eye (Lond.) 24, 408–415. doi: 10.1038/eye.2009.318
Lutty, G. A., Mathews, M. K., Merges, C., and Mcleod, D. S. (1998). Adenosine stimulates canine retinal microvascular endothelial cell migration and tube formation. Curr. Eye Res. 17, 594–607. doi: 10.1080/02713689808951232
Lutty, G. A., and McLeod, D. S. (2003). Retinal vascular development and oxygen-induced retinopathy: a role for adenosine. Prog. Retin. Eye Res. 22, 95–111. doi: 10.1016/s1350-9462(02)00058-7
Lutty, G. A., Mcleod, D. S., Bhutto, I. A., Edwards, M. M., and Seddon, J. M. (2020). Choriocapillaris dropout in early age-related macular degeneration. Exp. Eye Res. 192:107939. doi: 10.1016/j.exer.2020.107939
Ma, W., and Wong, W. T. (2016). Aging changes in retinal microglia and their relevance to age-related retinal disease. Adv. Exp. Med. Biol. 854, 73–78. doi: 10.1007/978-3-319-17121-0_11
Madeira, M. H., Boia, R., Ambrósio, A. F., and Santiago, A. R. (2017). Having a coffee break: the impact of caffeine consumption on microglia-mediated inflammation in neurodegenerative diseases. Mediators Inflamm. 2017:4761081.
Madeira, M. H., Ortin-Martinez, A., Nadal-Nicolas, F., Ambrosio, A. F., Vidal-Sanz, M., Agudo-Barriuso, M., et al. (2016). Caffeine administration prevents retinal neuroinflammation and loss of retinal ganglion cells in an animal model of glaucoma. Sci. Rep. 6:27532.
Madeira, M. H., Rashid, K., Ambrosio, A. F., Santiago, A. R., and Langmann, T. (2018). Blockade of microglial adenosine A2A receptor impacts inflammatory mechanisms, reduces ARPE-19 cell dysfunction and prevents photoreceptor loss in vitro. Sci. Rep. 8:2272.
Mantel, I., Borgo, A., Guidotti, J., Forestier, E., Kirsch, O., Derradji, Y., et al. (2020). Molecular biomarkers of neovascular age-related macular degeneration with incomplete response to anti-vascular endothelial growth factor treatment. Front. Pharmacol. 11:594087. doi: 10.3389/fphar.2020.594087
Maroñas, O., García-Quintanilla, L., Luaces-Rodríguez, A., Fernández-Ferreiro, A., Latorre-Pellicer, A., Abraldes, M. J., et al. (2020). Anti-VEGF treatment and response in age-related macular degeneration: disease’s susceptibility, pharmacogenetics and pharmacokinetics. Curr. Med. Chem. 27, 549–569. doi: 10.2174/0929867326666190711105325
Montesinos, M. C., Shaw, J. P., Yee, H., Shamamian, P., and Cronstein, B. N. (2004). Adenosine A(2A) receptor activation promotes wound neovascularization by stimulating angiogenesis and vasculogenesis. Am. J. Pathol. 164, 1887–1892. doi: 10.1016/s0002-9440(10)63749-2
Munk, M. R., Ceklic, L., Ebneter, A., Huf, W., Wolf, S., and Zinkernagel, M. S. (2016). Macular atrophy in patients with long-term anti-VEGF treatment for neovascular age-related macular degeneration. Acta Ophthalmol. 94, e757–e764.
Ouyang, X., Ghani, A., Malik, A., Wilder, T., Colegio, O. R., Flavell, R. A., et al. (2013). Adenosine is required for sustained inflammasome activation via the A(2)A receptor and the HIF-1alpha pathway. Nat. Commun. 4:2909.
Peleli, M., and Carlstrom, M. (2017). Adenosine signaling in diabetes mellitus and associated cardiovascular and renal complications. Mol. Aspects Med. 55, 62–74. doi: 10.1016/j.mam.2016.12.001
Pemp, B., and Schmetterer, L. (2008). Ocular blood flow in diabetes and age-related macular degeneration. Can. J. Ophthalmol. 43, 295–301. doi: 10.3129/i08-049
Peng, Z., Fernandez, P., Wilder, T., Yee, H., Chiriboga, L., Chan, E. S., et al. (2008). Ecto-5’-nucleotidase (CD73) -mediated extracellular adenosine production plays a critical role in hepatic fibrosis. FASEB J. 22, 2263–2272. doi: 10.1096/fj.07-100685
Potilinski, M. C., Tate, P. S., Lorenc, V. E., and Gallo, J. E. (2021). New insights into oxidative stress and immune mechanisms involved in age-related macular degeneration tackled by novel therapies. Neuropharmacology 188:108513. doi: 10.1016/j.neuropharm.2021.108513
Raoul, W., Auvynet, C., Camelo, S., Guillonneau, X., Feumi, C., Combadiere, C., et al. (2010). CCL2/CCR2 and CX3CL1/CX3CR1 chemokine axes and their possible involvement in age-related macular degeneration. J. Neuroinflammation 7:87.
Riksen, N. P., Rongen, G. A., Blom, H. J., Russel, F. G., Boers, G. H., and Smits, P. (2003). Potential role for adenosine in the pathogenesis of the vascular complications of hyperhomocysteinemia. Cardiovasc. Res. 59, 271–276. doi: 10.1016/s0008-6363(03)00462-0
Ryzhov, S., Mccaleb, J. L., Goldstein, A. E., Biaggioni, I., and Feoktistov, I. (2007). Role of adenosine receptors in the regulation of angiogenic factors and neovascularization in hypoxia. J. Pharmacol. Exp. Ther. 320, 565–572. doi: 10.1124/jpet.106.114850
Ryzhov, S., Zaynagetdinov, R., Goldstein, A. E., Novitskiy, S. V., Blackburn, M. R., Biaggioni, I., et al. (2008). Effect of A2B adenosine receptor gene ablation on adenosine-dependent regulation of proinflammatory cytokines. J. Pharmacol. Exp. Ther. 324, 694–700. doi: 10.1124/jpet.107.131540
Sachdeva, M. M., Cano, M., and Handa, J. T. (2014). Nrf2 signaling is impaired in the aging RPE given an oxidative insult. Exp. Eye Res. 119, 111–114. doi: 10.1016/j.exer.2013.10.024
Saint-Geniez, M., Kurihara, T., Sekiyama, E., Maldonado, A. E., and D’amore, P. A. (2009). An essential role for RPE-derived soluble VEGF in the maintenance of the choriocapillaris. Proc. Natl. Acad. Sci. U.S.A. 106, 18751–18756. doi: 10.1073/pnas.0905010106
Santiago, A. R., Madeira, M. H., Boia, R., Aires, I. D., Rodrigues-Neves, A. C., Santos, P. F., et al. (2020). Keep an eye on adenosine: its role in retinal inflammation. Pharmacol. Ther. 210:107513. doi: 10.1016/j.pharmthera.2020.107513
Scheef, E., Wang, S., Sorenson, C. M., and Sheibani, N. (2005). Isolation and characterization of murine retinal astrocytes. Mol. Vis. 11, 613–624.
Scheef, E. A., Sorenson, C. M., and Sheibani, N. (2009). Attenuation of proliferation and migration of retinal pericytes in the absence of thrombospondin-1. Am. J. Physiol. Cell Physiol. 296, C724–C734.
Shao, Z., Friedlander, M., Hurst, C. G., Cui, Z., Pei, D. T., Evans, L. P., et al. (2013). Choroid sprouting assay: an ex vivo model of microvascular angiogenesis. PLoS One 8:e69552. doi: 10.1371/journal.pone.0069552
Steinle, J. J., Sharma, S., Smith, C. P., and Mcfayden-Ketchum, L. S. (2009). Normal aging involves modulation of specific inflammatory markers in the rat retina and choroid. J. Gerontol. A Biol. Sci. Med. Sci. 64, 325–331.
Stevens, L. M., Linstead, E., Hall, J. L., and Kao, D. P. (2021). Association between coffee intake and incident heart failure risk: a machine learning analysis of the FHS, the ARIC study, and the CHS. Circ. Heart Fail. 14:e006799.
Su, X., Sorenson, C. M., and Sheibani, N. (2003). Isolation and characterization of murine retinal endothelial cells. Mol. Vis. 9, 171–178.
Tabolacci, C., Cordella, M., Rossi, S., Bonaccio, M., Eramo, A., Mischiati, C., et al. (2021). Targeting melanoma-initiating cells by caffeine: in silico and in vitro approaches. Molecules 26:3619. doi: 10.3390/molecules26123619
Tomany, S. C., Klein, R., and Klein, B. E. (2001). The relation of coffee and caffeine to the 5-year incidence of early age-related maculopathy: the Beaver Dam Eye Study. Am. J. Ophthalmol. 132, 271–273. doi: 10.1016/s0002-9394(01)00895-9
Vural, A. D., Kara, N., Sayin, N., Pirhan, D., and Ersan, H. B. (2014). Choroidal thickness changes after a single administration of coffee in healthy subjects. Retina 34, 1223–1228. doi: 10.1097/iae.0000000000000043
Wang, S., Sorenson, C. M., and Sheibani, N. (2012b). Lack of thrombospondin 1 and exacerbation of choroidal neovascularization. Arch. Ophthalmol. 130, 615–620.
Wang, S., Cao, C., Chen, Z., Bankaitis, V., Tzima, E., Sheibani, N., et al. (2012a). Pericytes regulate vascular basement membrane remodeling and govern neutrophil extravasation during inflammation. PLoS One 7:e45499. doi: 10.1371/journal.pone.0045499
Wang, Y., Wang, S., and Sheibani, N. (2006). Enhanced proangiogenic signaling in thrombospondin-1-deficient retinal endothelial cells. Microvasc. Res. 71, 143–151. doi: 10.1016/j.mvr.2006.02.004
Xu, H., Chen, M., and Forrester, J. V. (2009). Para-inflammation in the aging retina. Prog. Retin. Eye Res. 28, 348–368. doi: 10.1016/j.preteyeres.2009.06.001
Yoon, J. J., and Danesh-Meyer, H. V. (2019). Caffeine and the eye. Surv. Ophthalmol. 64, 334–344. doi: 10.1016/j.survophthal.2018.10.005
Zhang, J., Corciulo, C., Liu, H., Wilder, T., Ito, M., and Cronstein, B. (2017). Adenosine A(2a) receptor blockade diminishes Wnt/β-catenin signaling in a murine model of bleomycin-induced dermal fibrosis. Am. J. Pathol. 187, 1935–1944. doi: 10.1016/j.ajpath.2017.05.005
Zhang, M., Xu, G., Liu, W., Ni, Y., and Zhou, W. (2012). Role of fractalkine/CX3CR1 interaction in light-induced photoreceptor degeneration through regulating retinal microglial activation and migration. PLoS One 7:e35446. doi: 10.1371/journal.pone.0035446
Zhang, S., Zhou, R., Li, B., Li, H., Wang, Y., Gu, X., et al. (2017). Caffeine preferentially protects against oxygen-induced retinopathy. FASEB J. 31, 3334–3348. doi: 10.1096/fj.201601285r
Zhang, Y., and Wong, W. T. (2021). Innate immunity in age-related macular degeneration. Adv. Exp. Med. Biol. 1256, 121–141.
Zhang, Y., Zhao, L., Wang, X., Ma, W., Lazere, A., Qian, H. H., et al. (2018). Repopulating retinal microglia restore endogenous organization and function under CX3CL1-CX3CR1 regulation. Sci. Adv. 4:eaa8492.
Keywords: angiogenesis, adenosine receptors, age-related macular degeneration, retinal and choroidal endothelial cells, choroidal melanocytes
Citation: Sorenson CM, Song Y-S, Zaitoun IS, Wang S, Hanna BA, Darjatmoko SR, Gurel Z, Fisk DL, McDowell CM, McAdams RM and Sheibani N (2021) Caffeine Inhibits Choroidal Neovascularization Through Mitigation of Inflammatory and Angiogenesis Activities. Front. Cell Dev. Biol. 9:737426. doi: 10.3389/fcell.2021.737426
Received: 06 July 2021; Accepted: 13 September 2021;
Published: 14 October 2021.
Edited by:
Zi-Bing Jin, Capital Medical University, ChinaReviewed by:
José Carlos Rivera, University of Montreal, CanadaRong Zhou, Wenzhou Medical University, China
Copyright © 2021 Sorenson, Song, Zaitoun, Wang, Hanna, Darjatmoko, Gurel, Fisk, McDowell, McAdams and Sheibani. This is an open-access article distributed under the terms of the Creative Commons Attribution License (CC BY). The use, distribution or reproduction in other forums is permitted, provided the original author(s) and the copyright owner(s) are credited and that the original publication in this journal is cited, in accordance with accepted academic practice. No use, distribution or reproduction is permitted which does not comply with these terms.
*Correspondence: Nader Sheibani, nsheibanikar@wisc.edu