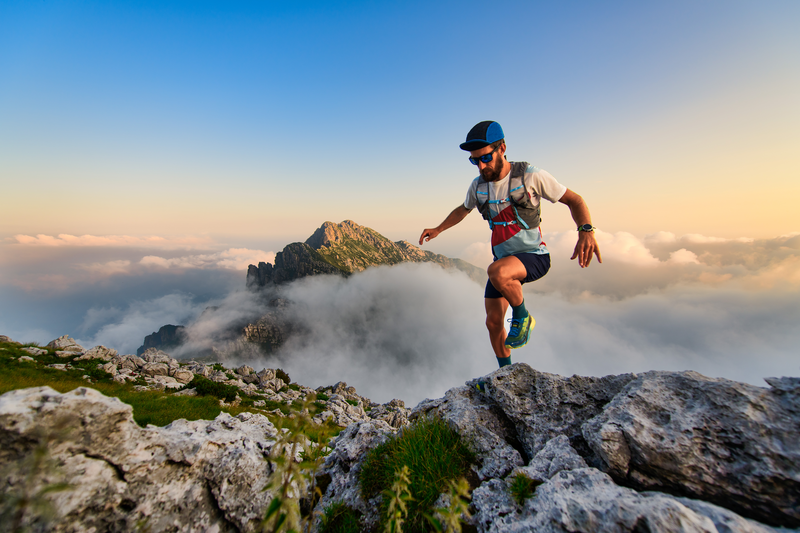
94% of researchers rate our articles as excellent or good
Learn more about the work of our research integrity team to safeguard the quality of each article we publish.
Find out more
MINI REVIEW article
Front. Cell Dev. Biol. , 25 October 2021
Sec. Stem Cell Research
Volume 9 - 2021 | https://doi.org/10.3389/fcell.2021.727640
This article is part of the Research Topic Double Side Blade: Niche in Stem Cell Potency and Potential Application View all 11 articles
The enrichment of cancer-associated fibroblast (CAFs) in a tumor microenvironment (TME) cultivates a pro-tumorigenic niche via aberrant paracrine signaling and matrix remodeling. A favorable niche is critical to the maintenance of cancer stem cells (CSCs), a population of cells that are characterized by their enhanced ability to self-renew, metastasis, and develop therapy resistance. Mounting evidence illustrates the interplay between CAF and cancer cells expedites malignant progression. Therefore, targeting the key cellular components and factors in the niche may promote a more efficacious treatment. In this study, we discuss how CAF orchestrates a niche that enhances CSC features and the potential therapeutic implication.
Tumors are illustrated as “wounds that do not heal” due to the enrichment of fibroblasts and immune cells at the tumor site, which highly mimics that of an inflammatory response of a non-neoplastic tissue (Dvorak, 1986). Indeed, cancer-associated fibroblast (CAF) constitutes the bulk of the stromal component in solid tumors. Cancer cells hijack the normal physiological function of activated fibroblast functions during wound recovery to fuel malignant development. Given cancer stemness accelerates disease progression and impinges therapy, understanding how CAFs cultivate a niche that bestows cancer stem cell (CSC) features in cancer cells may facilitate novel therapeutic intervention.
Cancer-associated fibroblast, also known as tumor-associated fibroblast, is generally regarded as fibroblasts that exist within or enveloping the tumor (Öhlund et al., 2014). The presence of CAFs throughout tumor development, from the incipient stage to advanced stages, alludes to CAF participation in tumor initiation and progression (Erez et al., 2010). Compared with non-tumor fibroblast (NF) or peri-tumor fibroblast, CAFs are located more proximal to the neoplastic region and exhibit a greater activation marker, namely actin smooth muscle (αSMA) and fibroblast activation protein (FAP) (Qin et al., 2016, 2019). However, compiling studies indicate CAF displays vast heterogeneity within a tumor with distinct functions, underscoring the importance of identifying the function of how each subtype contributes to malignancy (Öhlund et al., 2017; Costa et al., 2018; Lambrechts et al., 2018). A myriad of elements may affect CAF subtypes, for instance, proximity to the tumor. CAF subsets were distinguished based on their expression of αSMA and IL6 with high-αSMA localized nearer to the tumor and high-IL6 further, indicating juxtacrine and paracrine interaction between cancer cells and fibroblast may stimulate the CAF to differentiate to subtypes with distinct functions (Öhlund et al., 2017). Aside from molecular markers, the secretome of CAF and NF can be discerned as the former secretes a greater abundance of ligands that promote stemness properties of HCC (Jiang et al., 2017; Álvarez-Teijeiro et al., 2018). CAF plays an extensive role in shaping the extracellular matrix (ECM) of the tissues, allowing it to exert its oncogenic influence by modulating the physical properties of the TME. In addition to promoting cancer progression, fibroblasts are shown to be educated by neoplastic cells to their benefits, depicting the dynamic cellular network in the TME (Figure 1).
Figure 1. CAF and cancer cells in a dynamic TME. CAF secretes chemokines that can potentiate stemness and metastasis properties of cancer cells via distinct pathways. In turn, cancer cells have demonstrated the capacity to direct CAF to fuel their malignant properties. Immune cells, such as T cells, macrophages, and neutrophils, are orchestrated by CAF-cancer cell interaction to promote tumor progression. ANXA1, Annexin A1; CLCF1, cardiotrophin-like cytokine factor 1; COMP, cartilage oligomeric matrix protein; CXCL6, C-X-C motif chemokine ligand 6; CXCL12, C-X-C motif chemokine ligand 12; CCL2, CC-chemokine ligand 2; CCL5, CC-chemokine ligand 5; CCL7, CC-chemokine ligand 7; CCL16, CC-chemokine ligand 16; FGF5, fibroblast growth factor 5; HA, hyaluronan; Hh, hedgehog ligand; HMGB1, high-mobility group box protein 1; HGF, hepatocyte growth factor; IGFI, insulin-like growth factor 1; IGFII, insulin-like growth factor 2; IL6, interleukin 6; IL8, interleukin 8; MMP2; matrix metalloproteinase-2; MMP9, matrix metalloproteinase-9; POSTN, periostin; PGE2, prostaglandin E2; SHH, sonic hedgehog ligand; TGF-β, transforming growth factor-β; TGF-β1, transforming growth factor-β 1; TGF-β2, transforming growth factor-β 2; TGF-β3, transforming growth factor-β 3; WNT3a, Wnt Family Member 3A. The figure is created with BioRender.com.
Cancer stem cells are generally deemed as a rare population of cancer cells that share similar features to normal stem cells, including the ability to self-renew and differentiate into lineages that comprise the tumor bulk, thus regarded as tumorigenic, as opposed to non-CSC cancer cells (Reya et al., 2001; Clarke et al., 2006). Common CSC markers include surface markers, such as CD24, CD44, CD90, CD133, EpCAM, LGR5, and aldehyde dehydrogenase (ALDH) ALDH activity (summarized in Walcher et al., 2020). Moreover, expressions of stemness genes, such as OCT4, SOX2, and NANOG, are often deployed to measure cancer stemness. Functional surrogate to assess cancer stemness properties includes in vitro sphere formation and in vivo limiting dilution in which cancer cells are injected at low doses into animal models. Cancer cells with greater stemness are generally considered to have augmented metastasis ability due to their enhanced ability to reconstitute a tumor at the secondary site, and the acquisition of EMT is coupled with elevated stem cell properties, including stem cell markers and tumorigenicity, signifying EMT mimics features of CSC (Mani et al., 2008; Baccelli and Trumpp, 2012; Oskarsson et al., 2014). Emerging evidence illustrates that stemness properties of cancer cells are dependent on their niche (Plaks et al., 2015; Batlle and Clevers, 2017). Factors derived from niche may promote plasticity of non-CSC cancer cells into CSC, whereas depletion of factors may reduce the CSC population, thereby implicating niche factors as potential therapeutic targets (Batlle and Clevers, 2017). Given the CAF fosters the niche by enriching pro-tumor factors and remodeling the matrix of the TME, understanding how a niche facilitates cancer initiation and progression will allow us to contrive therapy targeting the key cellular components and factors sustaining cancer stemness.
Accumulating research illuminates the cancer cells instigate pro-tumor CAF to foster a pro-favorable niche. Transforming growth factor-β3 (TGF-β3) derived from HNSCC cells can activate CAF to secrete POSTN, leading to greater metastasis ability of the neoplastic cells (Qin et al., 2016). Prostate cancer cells can recruit marrow-derived mesenchymal stem cells (MSCs) and activate them into CAF by TGF-β1 (Barcellos-de-Souza et al., 2016). Of significance, MSC-derived CAF can recruit and induce monocytes into M2 macrophages, illustrating the CAF capacity to govern the TME. Cardiotrophin-like cytokine factor (CLCF1) derived from CAF stimulates the production of C-X-C motif ligand 6 (CXCL6) and TGF-β, which not only escalate stemness properties of cancer cells but also stimulate CLCF1 expression in the CAF, thereby fostering a positive feedback loop that expedites malignancy (Song et al., 2021). Furthermore, CXCL6 and TGF-β can enhance infiltration and development of pro-tumor N2-neutrophils (Song et al., 2021), corroborating CAF capacity to foster an immunosuppressive TME. Sonic hedgehog (SHH) ligand derived from CD24+CD49fhibreast CSCs constructs a pro-tumorigenic TME by activating CAF (Valenti et al., 2017). Cancer cells-derived hedgehog (Hh) instigates the production of pro-tumor paracrine factors and ECM constituents in CAF (Cazet et al., 2018). Autocrine and colorectal cancer cells-derived paracrine signaling of IL34 facilitate the conversion of normal fibroblast into CAF that adopts a pro-tumor secretome that includes stemness-promoting factors, such as Netrin-1 and FGF2 protein (Giulianelli et al., 2019; Sung et al., 2019). The coculture of HNSCC cells and CAF stimulate CAF to generate WNT3A, leading to increased cancer stemness (Le et al., 2019).
Exosomal miRNAs are gaining interest as a mediator between cancer cell-fibroblast crosstalk. Activation of focal adhesion kinase (FAK) signaling by cancer cell-derived miRNA stimulates the production of CAF-derived exosomes that facilitate the spheroid formation and metastasis (Wu et al., 2020). Tumor-derived exosomal miR-1247-3p triggers the activation of fibroblast by fueling NF-κB signaling consequently stimulates the CAF to secrete a greater abundance of IL6 and IL8, instigating the cancer cells to an EMT phenotype with enhanced lung metastases (Fang et al., 2018). Exosomal miR-9-5p elevates IL6 production in CAF, leading to enhanced spheroid-forming ability (Zhang et al., 2020). In summary, targeting the aforementioned factors or exosomes may disrupt the ability of cancer cells to assemble a pro-tumor niche.
The TGF-β family is shown to play pivotal roles in allowing cancer cells to colonize secondary sites. TGF-β3 rooted from cancer cells fortifies a favorable niche to colonize a foreign site by stimulating fibroblast-derived POSTN, which is crucial for initiating breast cancer cell colonization at the lung by maintaining CSC properties via the Wnt signaling (Malanchi et al., 2011). In accordance, HNSCC cancer cells secrete TGF-β3 that enhances POSTN secretion from CAF, resulting in the augmented migratory phenotype of cancer cells (Qin et al., 2016). Upon stimulation by TGF-β, CAF confers colorectal cancer cells-enhanced tumor-initiating capacity and reduced tumor latency (Calon et al., 2015). Tumor organoids with elevated TGF-β profoundly enhanced ability to form liver metastases by orchestrating a pro-tumor stroma niche (Calon et al., 2015). Colorectal cancer (CRC) fueled by driver mutations in the Lgr5 + stem cells is accompanied by enrichment of CAF-derived TGF-β that suppresses the tumor-killing T cell populations, thus leading to greater metastases to the liver (Tauriello et al., 2018), highlighting the interplay between CAF, immune cells, and cancer cells. Conversely, the TGF-β-signaling inhibitor significantly dampened the tumor volume and liver metastases of the colon.
In a tumor, fibroblast-secreted factors and cytokines, which serve for tissue recovery, are seized by cancer cells. Compiling studies have depicted the critical roles of CAF-derived factors in maintaining the CSC features of cancer cells by promoting stemness pathways (Table 1). HGF is reported to enhance cancer stemness by potentiating the frequency of CD44+, CD47+, and CD90+ HCC CSC and spheroid formation, stemness and EMT gene expressions (Lau et al., 2016; Ding et al., 2018; Yan et al., 2018). Mechanistically, HGF exerts its oncogenic influence via distinct mechanisms in a tissue-dependent manner. HGF augments ERK/FRA1/HEY1, STAT3/TWIST1, and YAP/HIF-1α in HCC, gastric cancer, and pancreatic cancer, respectively (Lau et al., 2016; Ding et al., 2018; Yan et al., 2018). CAF-derived IL6 is demonstrated to fuel stemness as evident in increased spheroid formation, stemness genes markers via STAT3 signaling (Xiong et al., 2018; Zhang et al., 2020). CAF paracrine signaling regulates the AKT pathway via insulin-like growth factor 2 (IGFII), thereby promoting NANOG expression, enhanced tumorigenicity in vivo, and ALDH activity (Chen et al., 2014). The AKT signaling is also mediated by cartilage oligomeric matrix protein (COMP) to fuel EMT and spheroid formation (Li et al., 2018). CC-chemokine ligand 2, 5, 7, and 16 (CCL2, CCL5, CCL7, and CXCL16) potentiate EMT via the Hh and TGF-β signaling (Liu et al., 2016). Serglycin (SRGN), a proteoglycan secreted from cancer cells and CAFs, renders chemoresistance and EMT features via a CD44-dependent NF-κB activation (Guo et al., 2017). The binding of CAF-derived periostin (POSTN) to protein tyrosine kinase 7 (PTK7) drives the β-catenin signaling, leading to increased expression of CSC markers and stemness genes (Yu et al., 2018). Annexin A1 (AnxA1) secreted from CAF potentiates stemness and EMT genes expressions, and spheroid formation (Geary et al., 2014). Prostaglandin E2 (PGE2) produced from fibroblasts drives the expansion of tumor-initiating cells by inducing YAP signaling (Roulis et al., 2020). The cellular crosstalk within the TME was displayed as CAFs recruit tumor-associated macrophages (TAMs) via CXCL12 secretion and induces the M2-phenotype TAMs, subsequently promotes stemness and EMT signature genes (Li et al., 2019). Alteration in specific genes may dictate the impact of CAF on cancer cells. Androgen receptor (AR)-depleted CAF enhances the production of IFN-γ and M-CSF, leading to increased cancer stemness (Liao et al., 2017). Notch1-depleted CAFs augment CD271+ melanoma CSC frequency (Du et al., 2019) and metastasis to the lung (Shao et al., 2016). Together, blocking CAF-derived factors or a downstream signaling cascade may be a therapeutic revenue to diminish cancer stemness.
A fundamental function of activated fibroblast is its participation in ECM homeostasis by synthesizing ECM constituents, including collagen and fibronectin, and producing ECM-degrading proteases to aid communication and trafficking of inflammatory cells (Kalluri, 2016). While the impact of matrix stiffness remains controversial as studies indicate both softer matrix (Tan et al., 2014; Ng et al., 2021) and stiffer matrix (Pang et al., 2016; Tao et al., 2021) can instigate cancer stemness, the role of CAF in mediating the stiffness of the ECM illustrates its ability to affect stemness. Indeed, collagen deposited by CAF functions as a mechano signal to escalate stem cell markers and spheroid formation ability (Cazet et al., 2018). Additionally, hyaluronan (HA), a major constituent of the ECM that boosts CSC self-renewal and EMT (summarized in Chanmee et al., 2015), is shown to be highly produced by CAF (Affo et al., 2021). Given CAFs are a source of ECM-degrading proteases, namely matrix metalloproteinases (MMPs), they can affect CSC properties by remodeling the ECM. Studies demonstrated IL6 derived from prostate cancer cells elicits pro-tumor FAP+CAF (Giannoni et al., 2010). Consequently, these FAP+ CAF secretes MMP2 and MMP9 to potentiate EMT and stemness genes. MMP3 that is predominantly produced by fibroblast (Witty et al., 1995) is demonstrated to promote stem cell population by enhancing canonical Wnt signaling, potentially leading to hyperplastic growth of normal tissues (Kessenbrock et al., 2013), depicting fibroblast can contribute to tumor initiation via ECM remodeling. Future research can focus on ablating the effect of CAF on the ECM, either by targeting ECM constitutes or MMPs, to attenuate the CAF-mediated oncogenic effect.
The augmented cancer stemness conferred by CAF may endow cancer resistance to conventional therapy. Patients with breast cancer, who are resistant to chemotherapy, show a greater abundance of CAF marked with a cluster of differentiation 10 (CD10) and G protein-coupled receptor 77 (GRP77) cell surface proteins (Su et al., 2018). CD10+GRP77+ CAFs promote sphere formation, the proportion of ALDH+ and CD44+CD24– breast CSCs, and chemoresistance properties of cancer cells by secreting IL6 and IL8. A similar phenomenon was observed in HNSCC as CAFs undergoing autophagy secrete greater levels of IL6 and IL8 to confer chemoresistance (New et al., 2017). Increased NANOG and SOX2 stemness gene expression in cancer cells treated with CAF-conditioned media may lead to greater cisplatin resistance (Peltanova et al., 2021). CAF-derived TGF-β1 drives self-renewal and gemcitabine resistance by upregulation of activating transcription factor 4 (ATF4) via the SMAD2/3 pathway (Wei et al., 2021). SRGN facilitates cisplatin resistance via inducing NANOG (Guo et al., 2017). In line, CAF-rooted TGF-β2 can elicit stemness and chemoresistance by enhancing GLI Family Zinc Finger 2 (GLI2) (Tang et al., 2018). CAF-derived exosomes enhance the stemness properties of CD133+ colorectal CSCs, leading to greater chemoresistance to fluorouracil and oxaliplatin (Hu et al., 2015). Additionally, exosomal miR-92a-3p derived from colorectal CAF targets anti-tumor F-box/WD repeat-containing protein 7 (FBXW7) and a modulator of apoptosis 1 (MOAP1), thereby endowing chemoresistance to cancer cells (Hu et al., 2019). In line, exosomal miRNA was demonstrated to confer chemoresistance in prostate cancer (Shan et al., 2020) and head and neck cancer (Qin et al., 2019). CAF-derived exosomal long non-coding RNA (lncRNA) H19 was exhibited to enable stemness expression by sponging miR-141 that targets β-catenin (Ren et al., 2018).
Another mechanism that CAF mediates chemoresistance resides in its ability to remodel the ECM. Studies have demonstrated HA upregulates NANOG/STAT signaling, leading to increased expression of multidrug transporter (MDR1) that contributes to chemoresistance (Bourguignon et al., 2008). In accordance, HA-CD44 interaction mediates stemness signaling that governs miRNA regulation of genes involved in chemoresistance in breast cancer cells (Bourguignon et al., 2009) and HNSCC cells (Bourguignon et al., 2012a, b). HA mediates CD44v3highALDH1highHNSCC CSC via an epigenetic alteration to promote cisplatin chemoresistance (Bourguignon et al., 2016). The ability of CAF to mediate matrix stiffness via MMPs may be an area of interest. Given matrix stiffness regulates stemness and chemoresistance (Ng et al., 2021); how a CAF-mediated ECM fosters a niche that endows chemoresistance warrants further investigations.
State-of-the-art single-cell transcriptomic profiling of intrahepatic cholangiocarcinoma (ICC) revealed six distinct fibroblast populations (Zhang et al., 2020). Vascular CAFs (vCAF), the most abundant population, were found to secrete IL6 to promote self-renewal of cancer cells. Of note, the characterization of the function of other CAF subtypes identified such as matrix CAFs, which expressed high ECM signature genes, would be an interesting area of research. Lineage tracing coupled with single-cell RNA sequencing (scRNA-seq) in sophisticated animal models categorized CAF into myofibroblast (myCAF) and inflammatory and growth factor-enriched CAF (iCAF) populations that modulate tumor cell proliferation via distinct mechanisms; the former has a high level of hyaluronan synthase 2 (Has2) that can enhance pro-tumor HA; the latter secretes HGF (Affo et al., 2021). scRNA-seq identified prostaglandin-endoperoxide synthase 2 (Ptgs2)-expressing fibroblasts that expand tumor-initiating stem cells via YAP signaling, whereas ablation of Ptgs2 diminished the occurrence of the small intestine and colon cancers (Roulis et al., 2020). Capturing cellular diversity at single-cell resolution ignites exciting research questions as follows: how cellular and non-cellular components of the TME govern CAF identity? How each subtype contributes to disease progression? How CAFs differentiate or undifferentiate into different subtypes?
As aforementioned (Table 1), cancer-promoting bioactive molecules derived from stromal cells may be targeted using specific neutralizing antibodies, particularly in combination with chemotherapy. However, the promising preclinical results have yet to yield optimistic results in clinical settings. A Phase 2 trial (NCT00433446) examining siltuximab (CNTO328), an anti-IL6 antibody, showed siltuximab did not have clinical benefit for patients with advanced prostate cancer (Dorff et al., 2010). Similarly, the addition of siltuximab (CNTO328) to mitoxantrone/prednisone regimen did not yield improved clinical (NCT00385827) outcomes compared to mitoxantrone/prednisone alone in treating advanced prostate cancer (Fizazi et al., 2012). The Phase 2, single-arm, clinical trial (NCT00992186) explored the efficacy of carlumab (CNTO 888), antibody targeting (CCL2), in patients with metastatic castration-resistant prostate cancer (CRPC), who had undergone docetaxel treatment (Pienta et al., 2013). However, none of the patients treated with carlumab showed partial or complete remission. One key possibility is that, while the CCL2 level was depleted upon carlumab administration, the level rebounded rapidly within a week, thus suggesting carlumab cannot suppress CCL2 for the clinically meaningful duration (Pienta et al., 2013). Nonetheless, the addition of siltuximab to bortezomib-melphalan-prednisone (VMP) demonstrated marginal clinical benefits in multiple myeloma by statistically improving partial response rate (San-Miguel et al., 2014). Together, these imply more precise stratification of patients and usage of biomarkers may result in better clinical outcomes. Of note, several clinical trials are exploring the clinical utility of neutralizing antibodies that target HGF (NCT04368507), MET (NCT04077099), and IL6R (NCT03999749). Aside from neutralizing antibodies, a combination of inhibitors-targeting receptors, including Osimertinib (an EGFR inhibitor) and Savolitinib (MET inhibitors), are being tested in clinical trials (NCT03778229). Notably, MP0250, a drug candidate targeting HGF and VEGF, which have met the safety requirement and demonstrated clinical efficacy, is being tested in combination with existing drugs to treat multiple myeloma (NCT03136653). Given the TME is often accompanied by an upsurge of cytokines and chemokines, targeting multiple factors may promote treatment efficacy.
The ability of CAF to endow stemness of cancer cells makes it an intriguing therapy. CAF is shown to be more positively correlated with gene sets associated with poor prognosis compared with epithelial cancer cells, immune cells, and endothelial cells (Calon et al., 2015), substantiating targeting CAF as a potential therapeutic avenue. Studies have demonstrated that αSMA expression represents a marker for worse prognosis in colorectal cancer, indicating myofibroblast abundance is crucial to disease prediction (Tsujino et al., 2007). In accordance, studies conducted in tongue cancer and oral cancer depicted similar results (Vered et al., 2010; Marsh et al., 2011).
However, the heterogeneity of CAF necessitates precise identification of more specific markers as tumor-restraining CAF exists. Genetic deletion of αSMA in mouse models enhances the progression of pancreatic ductal adenocarcinoma (PDAC) as evidenced by a lower survival rate in αSMA-depleted mice (Ozdemir et al., 2014). Interestingly, the myofibroblast-depleted tumor demonstrated enhanced spheroid-forming ability, indicating a greater proportion of CSC. Indeed, identification of CAF subtypes shows they promote or suppress tumor progression in a tissue-dependent manner (Galbo et al., 2021), corroborating the need for further investigation into more CAF-specific markers. Recent work has illuminated the potential therapeutic benefit of targeting tumor-promoting fibroblasts. Administration of GRP77 neutralizing antibody-targeting CAF depletes CAF-secreted IL6 and IL8, thereby abolishing a stem cell-supporting niche and sensitizing breast cancer cells to doxycycline, leading to significant shrinkage of the tumor volume (Su et al., 2018), highlighting the therapeutic potential of targeting precise tumor-promoting CAF.
Reeducating pro-tumor CAF into a quiescent state or even anti-tumor CAF is a tempting strategy. Vitamin D metabolite 1α, 25-dihydroxyvitamin D3 [1,25(OH)2D3] is shown to deplete the oncogenic influence of stromal fibroblast to the cancer cells, while 1,25(OH)2D3-treated stromal fibroblast displayed a gene signature that favors a clinical outcome (Ferrer-Mayorga et al., 2017). VDR activation of the stromal fibroblast using calcipotriol, a vitamin D analog, diminished expression of genes involved in growth factors and cytokines, for instance, the IL6 and POSTN, thereby suppressing a tumor-promoting secretome (Sherman et al., 2014). Additionally, the combination treatment of calcipotriol and gemcitabine markedly prolonged survival in preclinical models. Moreover, high vitamin D receptor (VDR) expression in stromal cells predicts favorable survival (Ferrer-Mayorga et al., 2017). All-trans retinoic acid (ATRA), also known as tretinoin, a vitamin A metabolite, renders pancreatic stellate cells into a quiescent state, which, in turn, secreted a greater level of secreted frizzled-related protein 4 (sFRP4) that negatively modulates Wnt signaling of cancer cells in a paracrine manner (Froeling et al., 2011). Indeed, ATRA has passed the safety in Phase I clinical trial (NCT03307148) and will proceed to Phase II (NCT04241276) as encouraging therapeutic responses were observed (Kocher et al., 2020). Given vitamins are essential for healthy tissue and their toxicity is relatively lower compared to chemotherapy, repurposing vitamin analogs to rewire activated fibroblast into a quiescent state may present a viable therapeutic strategy that can be translated into the clinical settings.
Another strategy to rewire the population of fibroblasts is by reprogramming the fibroblast using growth factors as exemplified in the plasticity of CAF found in PDAC. While IL1 induces fibroblast into having an inflammatory phenotype categorized by an elevated cytokines production, TGF-β antagonizes an IL1-induced phenotype and stimulates the fibroblast to adopt a myofibroblastic phenotype with less tumorigenesis, particularly reduced expression of factors promoting cancer stemness such as Il6 and Cxcl12 (Biffi et al., 2019). Together, this rationalizes the option to rewire tumor-promoting into tumor-restraining fibroblast. To account for the ability of the cancer cell to instigate tumor-promoting CAFs, inhibitors may be deployed to circumvent cancer cell-mediated activation of CAF. Breast cancer cells activate CAF via the hedgehog signaling to cultivate a stem cell niche by ECM remodeling and FGF5 secretion that promote docetaxel chemoresistance (Cazet et al., 2018). As such, targeting the crosstalk via smoothened inhibitors (SMOi), a hedgehog-signaling inhibitor, sensitizes triple-negative breast cancer (TNBC) to docetaxel. Concomitantly, inhibition of hedgehogs abrogates the activated stromal cells, thereby augmenting the efficacy of chemotherapy in treating pancreatic cancer (Olive et al., 2009). However, either genetic depletion or pharmacological inhibition of SHH, a ligand that activates pancreatic CAFs, resulted in less stromal composition but also a more aggressive tumor (Rhim et al., 2014), suggesting treatment should be tailored based on tissue and treatment.
Advances in single-cell RNA sequencing may not only aid the characterization of cell types based on their molecular profiles and, subsequently, functions but also have the potential to be used to identify new biomarkers for patient stratification and tailor-personalized medicine (Dominguez et al., 2020). Harnessing single-cell RNA-sequencing to profile CAF at the molecular level, future studies can address outstanding questions, including the origin and development of specific CAF subtypes, identification of biomarkers corresponding to each subtype, how each CAF subtype interacts with its niche, and amenable therapeutic opportunities to tackle tumor-promoting CAF. A more holistic approach investigating the CAF molecular profile, for instance, epitranscriptomic and epigenetics (Delaunay and Frye, 2019; Song et al., 2020), may unravel novel insights into how CAF shuttles between cell types. Recent studies have unmasked that the altered epigenetics profile between CAF and NF allows the former to generate greater levels of WNT5A that confers malignancy to the neoplastic cells (Maeda et al., 2020). A critical unmet knowledge gap in our understanding of CAF function is if juxtracrine signaling between CAF and cancer cells affects cancer stemness.
Given the TME is a dynamic region with various cell types actively contributing to tumor progression, merely focusing on targeting a single aspect seems unlikely to yield any long-term therapeutic benefit. For example, targeting niche factors alone may not be sufficient to eradicate the tumor due to the inclination of cancer cells to evolve into a niche-independent malignancy as the disease progresses (Fujii et al., 2016). Therefore, comprehensive characterization of cell types and their respective functions in the TME may pave the way for a multimodal approach to improve cancer treatment.
JL wrote the review article. SM edited the review article and provided funding support. Both authors contributed to the article and approved the submitted version.
This project was supported in part by grants from the Research Grants Council of Hong Kong—Theme-Based Research Scheme (T12-704/16-R), Collaborative Research Fund (C7026-18G), and the Health and Medical Research Fund from Food and Health Bureau of the Hong Kong Government (06172546).
The authors declare that the research was conducted in the absence of any commercial or financial relationships that could be construed as a potential conflict of interest.
All claims expressed in this article are solely those of the authors and do not necessarily represent those of their affiliated organizations, or those of the publisher, the editors and the reviewers. Any product that may be evaluated in this article, or claim that may be made by its manufacturer, is not guaranteed or endorsed by the publisher.
Affo, S., Nair, A., Brundu, F., Ravichandra, A., Bhattacharjee, S., Matsuda, M., et al. (2021). Promotion of cholangiocarcinoma growth by diverse cancer-associated fibroblast subpopulations. Cancer Cell 39, 866–882.e11. doi: 10.1016/j.ccell.2021.03.012
Álvarez-Teijeiro, S., Garcia-Inclan, C., Villaronga, M. A., Casado, P., Hermida-Prado, F., Granda-Diaz, R., et al. (2018). Factors secreted by cancer-associated fibroblasts that sustain cancer stem properties in head and neck squamous carcinoma cells as potential therapeutic targets. Cancers 10:334. doi: 10.3390/cancers10090334
Baccelli, I., and Trumpp, A. (2012). The evolving concept of cancer and metastasis stem cells. J. Cell Biol. 198, 281–293. doi: 10.1083/jcb.201202014
Barcellos-de-Souza, P., Comito, G., Pons-Segura, C., Taddei, M. L., Gori, V., Becherucci, V., et al. (2016). Mesenchymal stem cells are recruited and activated into carcinoma-associated fibroblasts by prostate cancer microenvironment-derived TGF-beta1. Stem Cells 34, 2536–2547. doi: 10.1002/stem.2412
Batlle, E., and Clevers, H. (2017). Cancer stem cells revisited. Nat. Med. 23, 1124–1134. doi: 10.1038/nm.4409
Biffi, G., Oni, T. E., Spielman, B., Hao, Y., Elyada, E., Park, Y., et al. (2019). IL1-induced JAK/STAT signaling Is antagonized by TGFbeta to shape CAF heterogeneity in pancreatic ductal adenocarcinoma. Cancer Discov. 9, 282–301. doi: 10.1158/2159-8290.CD-18-0710
Bourguignon, L. Y., Peyrollier, K., Xia, W., and Gilad, E. (2008). Hyaluronan-CD44 interaction activates stem cell marker Nanog, Stat-3-mediated MDR1 gene expression, and ankyrin-regulated multidrug efflux in breast and ovarian tumor cells. J. Biol. Chem. 283, 17635–17651. doi: 10.1074/jbc.M800109200
Bourguignon, L. Y., Spevak, C. C., Wong, G., Xia, W., and Gilad, E. (2009). Hyaluronan-CD44 interaction with protein kinase C(epsilon) promotes oncogenic signaling by the stem cell marker Nanog and the Production of microRNA-21, leading to down-regulation of the tumor suppressor protein PDCD4, anti-apoptosis, and chemotherapy resistance in breast tumor cells. J. Biol. Chem. 284, 26533–26546. doi: 10.1074/jbc.M109.027466
Bourguignon, L. Y., Earle, C., Wong, G., Spevak, C. C., and Krueger, K. (2012a). Stem cell marker (Nanog) and Stat-3 signaling promote MicroRNA-21 expression and chemoresistance in hyaluronan/CD44-activated head and neck squamous cell carcinoma cells. Oncogene 31, 149–160. doi: 10.1038/onc.2011.222
Bourguignon, L. Y., Wong, G., Earle, C., and Chen, L. (2012b). Hyaluronan-CD44v3 interaction with Oct4-Sox2-Nanog promotes miR-302 expression leading to self-renewal, clonal formation, and cisplatin resistance in cancer stem cells from head and neck squamous cell carcinoma. J. Biol. Chem. 287, 32800–32824. doi: 10.1074/jbc.M111.308528
Bourguignon, L. Y., Wong, G., and Shiina, M. (2016). Up-regulation of histone methyltransferase, DOT1L, by matrix hyaluronan promotes microRNA-10 expression leading to tumor cell invasion and chemoresistance in cancer stem cells from head and neck squamous cell carcinoma. J. Biol. Chem. 291, 10571–10585. doi: 10.1074/jbc.M115.700021
Calon, A., Lonardo, E., Berenguer-Llergo, A., Espinet, E., Hernando-Momblona, X., Iglesias, M., et al. (2015). Stromal gene expression defines poor-prognosis subtypes in colorectal cancer. Nat. Genet. 47, 320–329. doi: 10.1038/ng.3225
Cazet, A. S., Hui, M. N., Elsworth, B. L., Wu, S. Z., Roden, D., Chan, C. L., et al. (2018). Targeting stromal remodeling and cancer stem cell plasticity overcomes chemoresistance in triple negative breast cancer. Nat. Commun. 9:2897. doi: 10.1038/s41467-018-05220-6
Chanmee, T., Ontong, P., Kimata, K., and Itano, N. (2015). Key roles of hyaluronan and its CD44 receptor in the stemness and survival of cancer stem cells. Front. Oncol. 5:180. doi: 10.3389/fonc.2015.00180
Chen, W. J., Ho, C. C., Chang, Y. L., Chen, H. Y., Lin, C. A., Ling, T. Y., et al. (2014). Cancer-associated fibroblasts regulate the plasticity of lung cancer stemness via paracrine signalling. Nat. Commun. 5:3472. doi: 10.1038/ncomms4472
Clarke, M. F., Dick, J. E., Dirks, P. B., Eaves, C. J., Jamieson, C. H., Jones, D. L., et al. (2006). Cancer stem cells - perspectives on current status and future directions: AACR workshop on cancer stem cells. Cancer Res. 66, 9339–9344. doi: 10.1158/0008-5472.CAN-06-3126
Costa, A., Kieffer, Y., Scholer-Dahirel, A., Pelon, F., Bourachot, B., Cardon, M., et al. (2018). Fibroblast heterogeneity and immunosuppressive environment in human breast cancer. Cancer Cell 33, 463–479.e10. doi: 10.1016/j.ccell.2018.01.011
Delaunay, S., and Frye, M. (2019). RNA modifications regulating cell fate in cancer. Nat. Cell Biol. 21, 552–559. doi: 10.1038/s41556-019-0319-0
Ding, X., Ji, J., Jiang, J., Cai, Q., Wang, C., Shi, M., et al. (2018). HGF-mediated crosstalk between cancer-associated fibroblasts and MET-unamplified gastric cancer cells activates coordinated tumorigenesis and metastasis. Cell Death Dis. 9:867. doi: 10.1038/s41419-018-0922-1
Dominguez, C. X., Muller, S., Keerthivasan, S., Koeppen, H., Hung, J., Gierke, S., et al. (2020). Single-cell RNA sequencing reveals stromal evolution into LRRC15(+) myofibroblasts as a determinant of patient response to cancer immunotherapy. Cancer Discov. 10, 232–253. doi: 10.1158/2159-8290.CD-19-0644
Donnarumma, E., Fiore, D., Nappa, M., Roscigno, G., Adamo, A., Iaboni, M., et al. (2017). Cancer-associated fibroblasts release exosomal microRNAs that dictate an aggressive phenotype in breast cancer. Oncotarget 8, 19592–19608. doi: 10.18632/oncotarget.14752
Dorff, T. B., Goldman, B., Pinski, J. K., Mack, P. C., Lara, P. N. Jr., Van Veldhuizen, P. J. Jr., et al. (2010). Clinical and correlative results of SWOG S0354: a phase II trial of CNTO328 (siltuximab), a monoclonal antibody against interleukin-6, in chemotherapy-pretreated patients with castration-resistant prostate cancer. Clin. Cancer Res. 16, 3028–3034. doi: 10.1158/1078-0432.CCR-09-3122
Du, Y., Shao, H., Moller, M., Prokupets, R., Tse, Y. T., and Liu, Z. J. (2019). Intracellular Notch1 signaling in cancer-associated fibroblasts dictates the plasticity and stemness of melanoma stem/initiating cells. Stem Cells 37, 865–875. doi: 10.1002/stem.3013
Dvorak, H. F. (1986). Tumors: wounds that do not heal. Similarities between tumor stroma generation and wound healing. N. Engl. J. Med. 315, 1650–1659. doi: 10.1056/NEJM198612253152606
Erez, N., Truitt, M., Olson, P., Arron, S. T., and Hanahan, D. (2010). Cancer-associated fibroblasts are activated in incipient neoplasia to orchestrate tumor-promoting inflammation in an NF-kappaB-dependent manner. Cancer Cell 17, 135–147. doi: 10.1016/j.ccr.2009.12.041
Fang, T., Lv, H., Lv, G., Li, T., Wang, C., Han, Q., et al. (2018). Tumor-derived exosomal miR-1247-3p induces cancer-associated fibroblast activation to foster lung metastasis of liver cancer. Nat. Commun. 9:191. doi: 10.1038/s41467-017-02583-0
Ferrer-Mayorga, G., Gomez-Lopez, G., Barbachano, A., Fernandez-Barral, A., Pena, C., Pisano, D. G., et al. (2017). Vitamin D receptor expression and associated gene signature in tumour stromal fibroblasts predict clinical outcome in colorectal cancer. Gut 66, 1449–1462. doi: 10.1136/gutjnl-2015-310977
Fizazi, K., De Bono, J. S., Flechon, A., Heidenreich, A., Voog, E., Davis, N. B., et al. (2012). Randomised phase II study of siltuximab (CNTO 328), an anti-IL6 monoclonal antibody, in combination with mitoxantrone/prednisone versus mitoxantrone/prednisone alone in metastatic castration-resistant prostate cancer. Eur. J. Cancer 48, 85–93. doi: 10.1016/j.ejca.2011.10.014
Franze, E., Di Grazia, A., Sica, G. S., Biancone, L., Laudisi, F., and Monteleone, G. (2020). Interleukin-34 enhances the tumor promoting function of colorectal cancer-associated fibroblasts. Cancers 12:3537. doi: 10.3390/cancers12123537
Froeling, F. E., Feig, C., Chelala, C., Dobson, R., Mein, C. E., Tuveson, D. A., et al. (2011). Retinoic acid-induced pancreatic stellate cell quiescence reduces paracrine Wnt-beta-catenin signaling to slow tumor progression. Gastroenterology 141, 1486–1497. doi: 10.1053/j.gastro.2011.06.047
Fujii, M., Shimokawa, M., Date, S., Takano, A., Matano, M., Nanki, K., et al. (2016). A colorectal tumor organoid library demonstrates progressive loss of niche factor requirements during tumorigenesis. Cell Stem Cell 18, 827–838. doi: 10.1016/j.stem.2016.04.003
Galbo et al., P. M. Jr., Zang, X., and Zheng, D. (2021). Molecular features of cancer-associated fibroblast subtypes and their implication on cancer pathogenesis, prognosis, and immunotherapy resistance. Clin. Cancer Res. 1, 2636–2647. doi: 10.1158/1078-0432.CCR-20-4226
Geary, L. A., Nash, K. A., Adisetiyo, H., Liang, M., Liao, C. P., Jeong, J. H., et al. (2014). CAF-secreted annexin A1 induces prostate cancer cells to gain stem cell-like features. Mol. Cancer Res. 12, 607–621. doi: 10.1158/1541-7786.MCR-13-0469
Giannoni, E., Bianchini, F., Masieri, L., Serni, S., Torre, E., Calorini, L., et al. (2010). Reciprocal activation of prostate cancer cells and cancer-associated fibroblasts stimulates epithelial-mesenchymal transition and cancer stemness. Cancer Res. 70, 6945–6956. doi: 10.1158/0008-5472.CAN-10-0785
Giulianelli, S., Riggio, M., Guillardoy, T., Perez Pinero, C., Gorostiaga, M. A., Sequeira, G., et al. (2019). FGF2 induces breast cancer growth through ligand-independent activation and recruitment of ERalpha and PRBDelta4 isoform to MYC regulatory sequences. Int. J. Cancer 145, 1874–1888. doi: 10.1002/ijc.32252
Guo, J. Y., Hsu, H. S., Tyan, S. W., Li, F. Y., Shew, J. Y., Lee, W. H., et al. (2017). Serglycin in tumor microenvironment promotes non-small cell lung cancer aggressiveness in a CD44-dependent manner. Oncogene 36, 2457–2471. doi: 10.1038/onc.2016.404
Hu, J. L., Wang, W., Lan, X. L., Zeng, Z. C., Liang, Y. S., Yan, Y. R., et al. (2019). CAFs secreted exosomes promote metastasis and chemotherapy resistance by enhancing cell stemness and epithelial-mesenchymal transition in colorectal cancer. Mol. Cancer 18:91. doi: 10.1186/s12943-019-1019-x
Hu, Y., Yan, C., Mu, L., Huang, K., Li, X., Tao, D., et al. (2015). Fibroblast-derived exosomes contribute to chemoresistance through priming cancer stem cells in colorectal cancer. PLoS One 10:e0125625. doi: 10.1371/journal.pone.0125625
Jiang, J., Ye, F., Yang, X., Zong, C., Gao, L., Yang, Y., et al. (2017). Peri-tumor associated fibroblasts promote intrahepatic metastasis of hepatocellular carcinoma by recruiting cancer stem cells. Cancer Lett. 404, 19–28. doi: 10.1016/j.canlet.2017.07.006
Kalluri, R. (2016). The biology and function of fibroblasts in cancer. Nat. Rev. Cancer 16, 582–598. doi: 10.1038/nrc.2016.73
Kessenbrock, K., Dijkgraaf, G. J., Lawson, D. A., Littlepage, L. E., Shahi, P., Pieper, U., et al. (2013). A role for matrix metalloproteinases in regulating mammary stem cell function via the Wnt signaling pathway. Cell Stem Cell 13, 300–313. doi: 10.1016/j.stem.2013.06.005
Kocher, H. M., Basu, B., Froeling, F. E. M., Sarker, D., Slater, S., Carlin, D., et al. (2020). Phase I clinical trial repurposing all-trans retinoic acid as a stromal targeting agent for pancreatic cancer. Nat. Commun. 11:4841. doi: 10.1038/s41467-020-18636-w
Lambrechts, D., Wauters, E., Boeckx, B., Aibar, S., Nittner, D., Burton, O., et al. (2018). Phenotype molding of stromal cells in the lung tumor microenvironment. Nat. Med. 24, 1277–1289. doi: 10.1038/s41591-018-0096-5
Lau, E. Y., Lo, J., Cheng, B. Y., Ma, M. K., Lee, J. M., Ng, J. K., et al. (2016). Cancer-associated fibroblasts regulate tumor-initiating cell plasticity in hepatocellular carcinoma through c-Met/FRA1/HEY1 signaling. Cell Rep. 15, 1175–1189. doi: 10.1016/j.celrep.2016.04.019
Le, P. N., Keysar, S. B., Miller, B., Eagles, J. R., Chimed, T. S., Reisinger, J., et al. (2019). Wnt signaling dynamics in head and neck squamous cell cancer tumor-stroma interactions. Mol. Carcinog. 58, 398–410. doi: 10.1002/mc.22937
Li, Q., Wang, C., Wang, Y., Sun, L., Liu, Z., Wang, L., et al. (2018). HSCs-derived COMP drives hepatocellular carcinoma progression by activating MEK/ERK and PI3K/AKT signaling pathways. J. Exp. Clin. Cancer Res. 37:231. doi: 10.1186/s13046-018-0908-y
Li, X., Bu, W., Meng, L., Liu, X., Wang, S., Jiang, L., et al. (2019). CXCL12/CXCR4 pathway orchestrates CSC-like properties by CAF recruited tumor associated macrophage in OSCC. Exp. Cell Res. 378, 131–138. doi: 10.1016/j.yexcr.2019.03.013
Liao, C. P., Chen, L. Y., Luethy, A., Kim, Y., Kani, K., MacLeod, A. R., et al. (2017). Androgen receptor in cancer-associated fibroblasts influences stemness in cancer cells. Endocr. Relat. Cancer 24, 157–170. doi: 10.1530/ERC-16-0138
Liu, J., Chen, S., Wang, W., Ning, B. F., Chen, F., Shen, W., et al. (2016). Cancer-associated fibroblasts promote hepatocellular carcinoma metastasis through chemokine-activated hedgehog and TGF-beta pathways. Cancer Lett. 379, 49–59. doi: 10.1016/j.canlet.2016.05.022
Maeda, M., Takeshima, H., Iida, N., Hattori, N., Yamashita, S., Moro, H., et al. (2020). Cancer cell niche factors secreted from cancer-associated fibroblast by loss of H3K27me3. Gut 69, 243–251. doi: 10.1136/gutjnl-2018-317645
Malanchi, I., Santamaria-Martinez, A., Susanto, E., Peng, H., Lehr, H. A., Delaloye, J. F., et al. (2011). Interactions between cancer stem cells and their niche govern metastatic colonization. Nature 481, 85–89. doi: 10.1038/nature10694
Mani, S. A., Guo, W., Liao, M. J., Eaton, E. N., Ayyanan, A., Zhou, A. Y., et al. (2008). The epithelial-mesenchymal transition generates cells with properties of stem cells. Cell 133, 704–715. doi: 10.1016/j.cell.2008.03.027
Marsh, D., Suchak, K., Moutasim, K. A., Vallath, S., Hopper, C., Jerjes, W., et al. (2011). Stromal features are predictive of disease mortality in oral cancer patients. J. Pathol. 223, 470–481. doi: 10.1002/path.2830
New, J., Arnold, L., Ananth, M., Alvi, S., Thornton, M., Werner, L., et al. (2017). Secretory autophagy in cancer-associated fibroblasts promotes head and neck cancer progression and offers a novel therapeutic target. Cancer Res. 77, 6679–6691. doi: 10.1158/0008-5472.CAN-17-1077
Ng, K. Y., Shea, Q. T., Wong, T. L., Luk, S. T., Tong, M., Lo, C. M., et al. (2021). Chemotherapy-enriched THBS2-deficient cancer stem cells drive hepatocarcinogenesis through matrix softness induced histone H3 modifications. Adv. Sci. 8:2002483. doi: 10.1002/advs.202002483
Öhlund, D., Elyada, E., and Tuveson, D. (2014). Fibroblast heterogeneity in the cancer wound. J. Exp. Med. 211, 1503–1523. doi: 10.1084/jem.20140692
Öhlund, D., Handly-Santana, A., Biffi, G., Elyada, E., Almeida, A. S., Ponz-Sarvise, M., et al. (2017). Distinct populations of inflammatory fibroblasts and myofibroblasts in pancreatic cancer. J. Exp. Med. 214, 579–596. doi: 10.1084/jem.20162024
Olive, K. P., Jacobetz, M. A., Davidson, C. J., Gopinathan, A., McIntyre, D., Honess, D., et al. (2009). Inhibition of Hedgehog signaling enhances delivery of chemotherapy in a mouse model of pancreatic cancer. Science 324, 1457–1461. doi: 10.1126/science.1171362
Oskarsson, T., Batlle, E., and Massague, J. (2014). Metastatic stem cells: sources, niches, and vital pathways. Cell Stem Cell 14, 306–321. doi: 10.1016/j.stem.2014.02.002
Ozdemir, B. C., Pentcheva-Hoang, T., Carstens, J. L., Zheng, X., Wu, C. C., Simpson, T. R., et al. (2014). Depletion of carcinoma-associated fibroblasts and fibrosis induces immunosuppression and accelerates pancreas cancer with reduced survival. Cancer Cell 25, 719–734. doi: 10.1016/j.ccr.2014.04.005
Pang, M. F., Siedlik, M. J., Han, S., Stallings-Mann, M., Radisky, D. C., and Nelson, C. M. (2016). Tissue stiffness and hypoxia modulate the integrin-linked kinase ILK to control breast cancer stem-like cells. Cancer Res. 76, 5277–5287. doi: 10.1158/0008-5472.CAN-16-0579
Peltanova, B., Liskova, M., Gumulec, J., Raudenska, M., Polanska, H. H., Vaculovic, T., et al. (2021). Sensitivity to cisplatin in head and neck cancer cells is significantly affected by patient-derived cancer-associated fibroblasts. Int. J. Mol. Sci. 22:1912. doi: 10.3390/ijms22041912
Pienta, K. J., Machiels, J. P., Schrijvers, D., Alekseev, B., Shkolnik, M., Crabb, S. J., et al. (2013). Phase 2 study of carlumab (CNTO 888), a human monoclonal antibody against CC-chemokine ligand 2 (CCL2), in metastatic castration-resistant prostate cancer. Invest. New Drugs 31, 760–768. doi: 10.1007/s10637-012-9869-8
Plaks, V., Kong, N., and Werb, Z. (2015). The cancer stem cell niche: how essential is the niche in regulating stemness of tumor cells? Cell Stem Cell 16, 225–238. doi: 10.1016/j.stem.2015.02.015
Qin, X., Guo, H., Wang, X., Zhu, X., Yan, M., Wang, X., et al. (2019). Exosomal miR-196a derived from cancer-associated fibroblasts confers cisplatin resistance in head and neck cancer through targeting CDKN1B and ING5. Genome Biol. 20:12. doi: 10.1186/s13059-018-1604-0
Qin, X., Yan, M., Zhang, J., Wang, X., Shen, Z., Lv, Z., et al. (2016). TGFbeta3-mediated induction of Periostin facilitates head and neck cancer growth and is associated with metastasis. Sci. Rep. 6:20587. doi: 10.1038/srep20587
Ren, J., Ding, L., Zhang, D., Shi, G., Xu, Q., Shen, S., et al. (2018). Carcinoma-associated fibroblasts promote the stemness and chemoresistance of colorectal cancer by transferring exosomal lncRNA H19. Theranostics 8, 3932–3948. doi: 10.7150/thno.25541
Reya, T., Morrison, S. J., Clarke, M. F., and Weissman, I. L. (2001). Stem cells, cancer, and cancer stem cells. Nature 414, 105–111. doi: 10.1038/35102167
Rhim, A. D., Oberstein, P. E., Thomas, D. H., Mirek, E. T., Palermo, C. F., Sastra, S. A., et al. (2014). Stromal elements act to restrain, rather than support, pancreatic ductal adenocarcinoma. Cancer Cell 25, 735–747. doi: 10.1016/j.ccr.2014.04.021
Roulis, M., Kaklamanos, A., Schernthanner, M., Bielecki, P., Zhao, J., Kaffe, E., et al. (2020). Paracrine orchestration of intestinal tumorigenesis by a mesenchymal niche. Nature 580, 524–529. doi: 10.1038/s41586-020-2166-3
San-Miguel, J., Blade, J., Shpilberg, O., Grosicki, S., Maloisel, F., Min, C. K., et al. (2014). Phase 2 randomized study of bortezomib-melphalan-prednisone with or without siltuximab (anti-IL6) in multiple myeloma. Blood 123, 4136–4142. doi: 10.1182/blood-2013-12-546374
Shan, G., Gu, J., Zhou, D., Li, L., Cheng, W., Wang, Y., et al. (2020). Cancer-associated fibroblast-secreted exosomal miR-423-5p promotes chemotherapy resistance in prostate cancer by targeting GREM2 through the TGF-beta signaling pathway. Exp. Mol. Med. 52, 1809–1822. doi: 10.1038/s12276-020-0431-z
Shao, H., Cai, L., Moller, M., Issac, B., Zhang, L., Owyong, M., et al. (2016). Notch1-WISP-1 axis determines the regulatory role of mesenchymal stem cell-derived stromal fibroblasts in melanoma metastasis. Oncotarget 7, 79262–79273. doi: 10.18632/oncotarget.13021
Sherman, M. H., Yu, R. T., Engle, D. D., Ding, N., Atkins, A. R., Tiriac, H., et al. (2014). Vitamin D receptor-mediated stromal reprogramming suppresses pancreatitis and enhances pancreatic cancer therapy. Cell 159, 80–93. doi: 10.1016/j.cell.2014.08.007
Song, H., Liu, D., Dong, S., Zeng, L., Wu, Z., Zhao, P., et al. (2020). Epitranscriptomics and epiproteomics in cancer drug resistance: therapeutic implications. Signal Transduct. Target. Ther. 5:193. doi: 10.1038/s41392-020-00300-w
Song, M., He, J., Pan, Q. Z., Yang, J., Zhao, J., Zhang, Y. J., et al. (2021). Cancer-associated fibroblast-mediated cellular crosstalk supports hepatocellular carcinoma progression. Hepatology 73, 1717–1735. doi: 10.1002/hep.31792
Su, S., Chen, J., Yao, H., Liu, J., Yu, S., Lao, L., et al. (2018). CD10(+)GPR77(+) cancer-associated fibroblasts promote cancer formation and chemoresistance by sustaining cancer stemness. Cell 172, 841–856.e16. doi: 10.1016/j.cell.2018.01.009
Sun, L., Wang, Y., Wang, L., Yao, B., Chen, T., Li, Q., et al. (2019). Resolvin D1 prevents epithelial-mesenchymal transition and reduces the stemness features of hepatocellular carcinoma by inhibiting paracrine of cancer-associated fibroblast-derived COMP. J. Exp. Clin. Cancer Res. 38, 170. doi: 10.1186/s13046-019-1163-6
Sung, P. J., Rama, N., Imbach, J., Fiore, S., Ducarouge, B., Neves, D., et al. (2019). Cancer-associated fibroblasts produce netrin-1 to control cancer cell plasticity. Cancer Res. 79, 3651–3661. doi: 10.1158/0008-5472.CAN-18-2952
Tan, Y., Tajik, A., Chen, J., Jia, Q., Chowdhury, F., Wang, L., et al. (2014). Matrix softness regulates plasticity of tumour-repopulating cells via H3K9 demethylation and Sox2 expression. Nat. Commun. 5:4619. doi: 10.1038/ncomms5619
Tang, Y. A., Chen, Y. F., Bao, Y., Mahara, S., Yatim, S., Oguz, G., et al. (2018). Hypoxic tumor microenvironment activates GLI2 via HIF-1alpha and TGF-beta2 to promote chemoresistance in colorectal cancer. Proc. Natl. Acad. Sci. U. S. A. 115, E5990–E5999. doi: 10.1073/pnas.1801348115
Tao, B., Song, Y., Wu, Y., Yang, X., Peng, T., Peng, L., et al. (2021). Matrix stiffness promotes glioma cell stemness by activating BCL9L/Wnt/beta-catenin signaling. Aging 13, 5284–5296. doi: 10.18632/aging.202449
Tauriello, D. V. F., Palomo-Ponce, S., Stork, D., Berenguer-Llergo, A., Badia-Ramentol, J., Iglesias, M., et al. (2018). TGFbeta drives immune evasion in genetically reconstituted colon cancer metastasis. Nature 554, 538–543. doi: 10.1038/nature25492
Tsujino, T., Seshimo, I., Yamamoto, H., Ngan, C. Y., Ezumi, K., Takemasa, I., et al. (2007). Stromal myofibroblasts predict disease recurrence for colorectal cancer. Clin. Cancer Res. 13, 2082–2090. doi: 10.1158/1078-0432.CCR-06-2191
Valenti, G., Quinn, H. M., Heynen, G., Lan, L., Holland, J. D., Vogel, R., et al. (2017). Cancer stem cells regulate cancer-associated fibroblasts via activation of hedgehog signaling in mammary gland tumors. Cancer Res. 77, 2134–2147. doi: 10.1158/0008-5472.CAN-15-3490
Vered, M., Dobriyan, A., Dayan, D., Yahalom, R., Talmi, Y. P., Bedrin, L., et al. (2010). Tumor-host histopathologic variables, stromal myofibroblasts and risk score, are significantly associated with recurrent disease in tongue cancer. Cancer Sci. 101, 274–280. doi: 10.1111/j.1349-7006.2009.01357.x
Walcher, L., Kistenmacher, A. K., Suo, H., Kitte, R., Dluczek, S., Strauss, A., et al. (2020). Cancer stem cells- origins and biomarkers: perspectives for targeted personalized therapies. Front. Immunol. 11:1280. doi: 10.3389/fimmu.2020.01280
Wei, L., Lin, Q., Lu, Y., Li, G., Huang, L., Fu, Z., et al. (2021). Cancer-associated fibroblasts-mediated ATF4 expression promotes malignancy and gemcitabine resistance in pancreatic cancer via the TGF-beta1/SMAD2/3 pathway and ABCC1 transactivation. Cell Death Dis. 12:334. doi: 10.1038/s41419-021-03574-2
Witty, J. P., Wright, J. H., and Matrisian, L. M. (1995). Matrix metalloproteinases are expressed during ductal and alveolar mammary morphogenesis, and misregulation of stromelysin-1 in transgenic mice induces unscheduled alveolar development. Mol. Biol. Cell 6, 1287–1303. doi: 10.1091/mbc.6.10.1287
Wu, H. J., Hao, M., Yeo, S. K., and Guan, J. L. (2020). FAK signaling in cancer-associated fibroblasts promotes breast cancer cell migration and metastasis by exosomal miRNAs-mediated intercellular communication. Oncogene 39, 2539–2549. doi: 10.1038/s41388-020-1162-2
Xiong, S., Wang, R., Chen, Q., Luo, J., Wang, J., Zhao, Z., et al. (2018). Cancer-associated fibroblasts promote stem cell-like properties of hepatocellular carcinoma cells through IL6/STAT3/Notch signaling. Am. J. Cancer Res. 8, 302–316.
Yan, B., Jiang, Z., Cheng, L., Chen, K., Zhou, C., Sun, L., et al. (2018). Paracrine HGF/c-MET enhances the stem cell-like potential and glycolysis of pancreatic cancer cells via activation of YAP/HIF-1alpha. Exp. Cell Res. 371, 63–71. doi: 10.1016/j.yexcr.2018.07.041
Yu, B., Wu, K., Wang, X., Zhang, J., Wang, L., Jiang, Y., et al. (2018). Periostin secreted by cancer-associated fibroblasts promotes cancer stemness in head and neck cancer by activating protein tyrosine kinase 7. Cell Death Dis. 9:1082. doi: 10.1038/s41419-018-1116-6
Zhang, M., Yang, H., Wan, L., Wang, Z., Wang, H., Ge, C., et al. (2020). Single-cell transcriptomic architecture and intercellular crosstalk of human intrahepatic cholangiocarcinoma. J. Hepatol. 73, 1118–1130. doi: 10.1016/j.jhep.2020.05.039
Keywords: inflammation, targeted therapy, tumor microenvironment, cancer associated fibroblast, cancer stem cell, cancer stemness
Citation: Loh JJ and Ma S (2021) The Role of Cancer-Associated Fibroblast as a Dynamic Player in Mediating Cancer Stemness in the Tumor Microenvironment. Front. Cell Dev. Biol. 9:727640. doi: 10.3389/fcell.2021.727640
Received: 19 June 2021; Accepted: 24 September 2021;
Published: 25 October 2021.
Edited by:
Takahiko Hara, Tokyo Metropolitan Institute of Medical Science, JapanReviewed by:
Borhane Guezguez, German Cancer Research Center (DKFZ), GermanyCopyright © 2021 Loh and Ma. This is an open-access article distributed under the terms of the Creative Commons Attribution License (CC BY). The use, distribution or reproduction in other forums is permitted, provided the original author(s) and the copyright owner(s) are credited and that the original publication in this journal is cited, in accordance with accepted academic practice. No use, distribution or reproduction is permitted which does not comply with these terms.
*Correspondence: Stephanie Ma, c3RlZm1hQGhrdS5oaw==
Disclaimer: All claims expressed in this article are solely those of the authors and do not necessarily represent those of their affiliated organizations, or those of the publisher, the editors and the reviewers. Any product that may be evaluated in this article or claim that may be made by its manufacturer is not guaranteed or endorsed by the publisher.
Research integrity at Frontiers
Learn more about the work of our research integrity team to safeguard the quality of each article we publish.