- 1State Key Laboratory of Pharmaceutical Biotechnology, Division of Sports Medicine and Adult Reconstructive Surgery, Department of Orthopedic Surgery, Nanjing Drum Tower Hospital, The Affiliated Hospital of Nanjing University Medical School, Nanjing, China
- 2Laboratory for Bone and Joint Diseases, RIKEN Center for Integrative Medical Science (IMS, RIKEN), Tokyo, Japan
Knee osteoarthritis (KOA) is the most common form of joint degeneration with increasing prevalence and incidence in recent decades. KOA is a molecular disorder characterized by the interplay of numerous molecules, a considerable number of which can be detected in body fluids, including synovial fluid, urine, and blood. However, the current diagnosis and treatment of KOA mainly rely on clinical and imaging manifestations, neglecting its molecular pathophysiology. The mismatch between participants’ molecular characteristics and drug therapeutic mechanisms might explain the failure of some disease-modifying drugs in clinical trials. Hence, according to the temporal alteration of representative molecules, we propose a novel molecular classification of KOA divided into pre-KOA, early KOA, progressive KOA, and end-stage KOA. Then, progressive KOA is furtherly divided into four subtypes as cartilage degradation-driven, bone remodeling-driven, inflammation-driven, and pain-driven subtype, based on the major pathophysiology in patient clusters. Multiple clinical findings of representatively investigated molecules in recent years will be reviewed and categorized. This molecular classification allows for the prediction of high-risk KOA individuals, the diagnosis of early KOA patients, the assessment of therapeutic efficacy, and in particular, the selection of homogenous patients who may benefit most from the appropriate therapeutic agents.
Introduction
Osteoarthritis (OA) is a common and disabling condition globally, within which knee OA (KOA) accounts for a large proportion and manifests several symptoms that weaken the quality of life, such as pain, stiffness, dysfunction, and even deformity (Martel-Pelletier et al., 2016). Combined with imaging methods, diagnosis for KOA is made on the basis of symptom assessment and a brief physical examination (Hunter and Bierma-Zeinstra, 2019). Plain radiograph is widely used to assess the Kellgren-Lawrence (KL) composite score, and magnetic resonance imaging (MRI) is usually performed to assess the cartilage, synovium and subchondral bone lesions. However, these symptomatic, physical and imaging methods are insensitive to reflect early pathophysiology (Menashe et al., 2012). Besides, current treatment of KOA highly relies on the identification of clinical information and, consequently, the evaluation of therapeutic efficacy is largely based on clinical, and frequently rater-dependent, outcomes (Figure 1A).
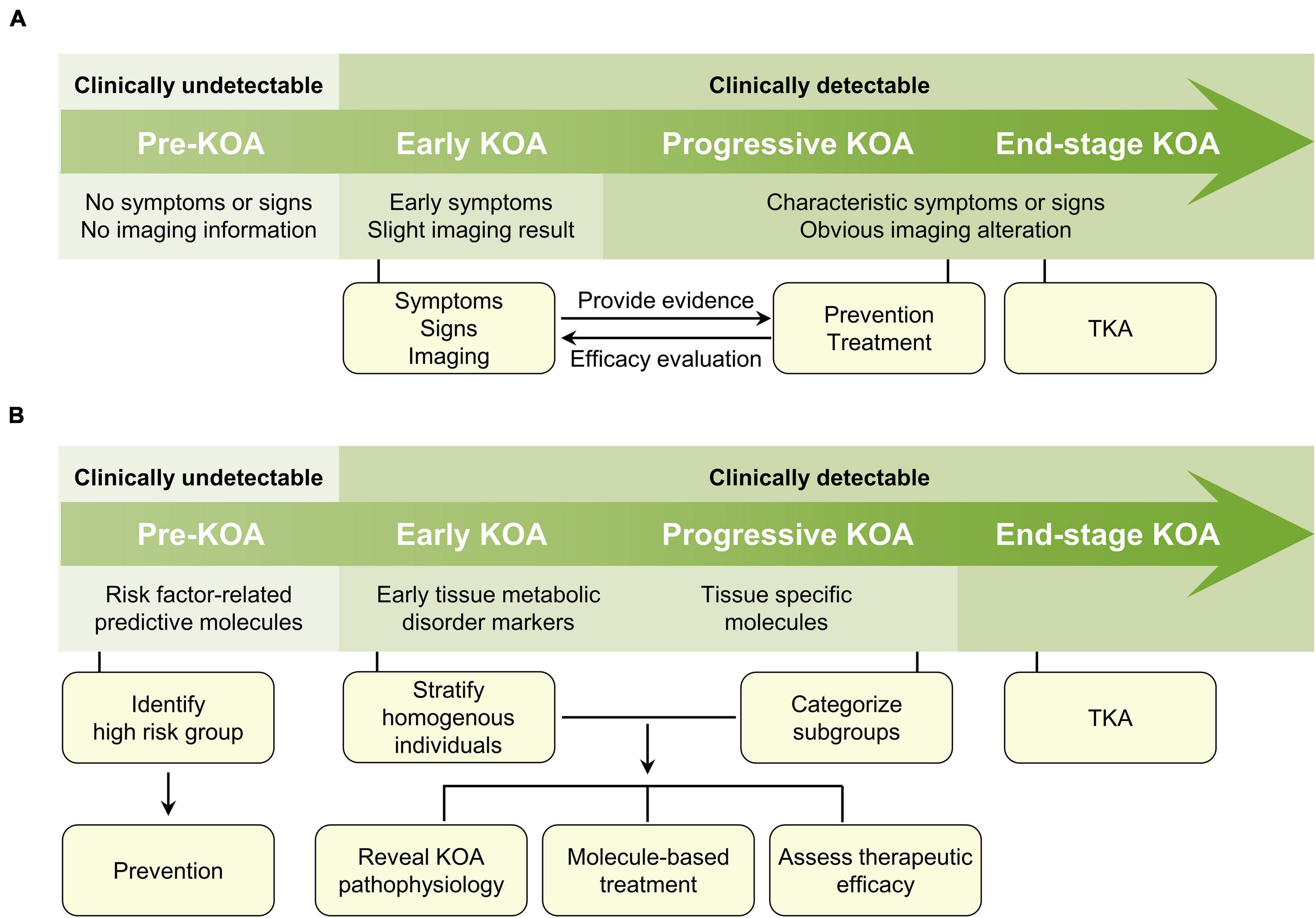
Figure 1. Clinical information-based and molecule-based diagnosis and treatment algorithm of KOA. (A) In the clinical information-based KOA diagnosis and treatment algorithm, there is no symptoms, signs, and imaging information for pre-KOA. In early KOA, early symptoms and mild imaging results can be acquired. In progressive and late-stage KOA, the diagnosis is comparatively easy due to obvious clinical manifestations. Treatment decision making principally depends on clinical appearances and in turn, the evaluation of therapeutic efficacy is largely based on clinical outcomes. (B) In the molecule-based KOA diagnosis and treatment algorithm, the detection of KOA risk factors-related molecules in body fluids provides opportunity for the prediction of high-risk KOA individuals, and subsequently the preventive strategies. Although clinical information in early and progressive stage are acquirable, disease corresponding molecules permit the stratification of homogenous individuals and the classification of KOA into several subtypes. The molecular classification of KOA may help to reveal the pathogenesis of KOA, explore the molecule-based treatment, and further assess the therapeutic efficacy of treatment. For end-stage KOA, TKA might still be the best option. KOA, knee osteoarthritis; TKA, total knee arthroplasty.
Among the drugs applied clinically, some of them show acceptable outcomes in pain relief and joint function improvement, whereas, a fair portion of them with unclear results (Sharma, 2021). Clinical guidelines regarding the use of some pharmaceutics are usually inconsistent (Nguyen et al., 2016). This divergence may be due to the differences between clinical trials, in which the biologically heterogeneous subjects are included. In addition to the completed clinical trials, the inclusion criteria for ongoing clinical trials starting from 2016 to 2021 to explore the effects of a certain drug on KOA did not consider the molecular characteristics of participants (Table 1). Hence, a critical research gap in the matching of drug mechanisms with patients’ molecular features still exists and needs to be filled.
Indeed, patients with KOA exhibit early molecular and structural changes before the disease shows any clinical manifestations (Goldring and Goldring, 2010). Recently, the Osteoarthritis Research Society International (OARSI) endorsed a new definition of OA, emphasizing the molecular derangement as the primary disorder followed by anatomic, and/or physiologic disarrays (Kraus et al., 2015), which highlights a molecular characterization of the pathological mechanisms responsible for KOA. One of the most imperative contributors of the diagnostic work-up of KOA toward a molecular based identification is the research on molecules acting as biomarkers. Indeed, a large amount of KOA-related molecules are detectable in body fluids (Akul et al., 2019), reflecting KOA pathogenesis. Biochemical analysis of body fluids in hospitals and clinics is frequently employed for effective disease diagnosis as they contain numerous valuable disease information (Sung et al., 2021), which provides the prospect of diagnosing and evaluating KOA at the molecular level.
Herein, according to the temporal alteration of representative molecules in body fluids, we propose a novel molecular classification of KOA (i.e., pre-KOA, early KOA, progressive KOA, and end-stage KOA), with a focus on its role in KOA prediction, diagnosis, and treatment efficacy evaluation. This classification criteria may allow for a molecule-based diagnosis and treatment algorithm (Figure 1B), for instance, facilitating the enrollment of biologically homogeneous patients in clinical trials and potentiate the therapeutic efficacy of disease-modifying drugs in a specific patient cluster.
KOA: A Molecular Disorder
Traditionally, KOA has been considered as a disorder of articular cartilage. However, the current view is that KOA is a whole-joint disease, or even a systemic disorder since it could be affected by various local and systemic risk factors. The major pathogenesis in KOA contains the molecular crosstalk between articular cartilage, synovium, subchondral bone, meniscus, tendon, muscle and infrapatellar fat pad (IFP) (Loeser et al., 2012; Fan et al., 2021). For instance, the accumulation of M1 macrophage in synovium is responsible for the secretion of proinflammatory cytokines, which facilitates the formation of inflammation microenvironment and aggravates cartilage degradation and synovitis (Robinson et al., 2016; Zhang et al., 2020). A variety of cytokines and adipokines secreted by IFP also participate the local inflammation and contribute to the development of KOA (Zeng et al., 2020). In turn, the debris released from degenerated cartilage can also boost the inflammatory response within the joint (Lambert et al., 2019). Such cross-linked molecular dysregulation is the basis of the visibly pathological events, making a strong case to outline its profile in KOA.
From a practical point of view, less invasive or non-invasive methods to obtain the molecular spectrum will have greater clinical significance. Interestingly, along with intense molecular crosstalk, the opportunity to assess molecular changes is presented by the molecular carrying medium, in which synovial fluid (SF), blood, and urine are included (Sung et al., 2021). SF is a gold standard fluid to identify molecules for KOA due to its intimate relationship with various joint tissues and important role in transmitting and receiving molecular signal in the joint cavity (Ingale et al., 2021). Blood and urine are easily withdrawn and reserve a cluster of molecules reflecting KOA pathogenesis (Attur et al., 2011; Bihlet et al., 2019). Given the widespread use of humoral tests, it would be anticipated to characterize the molecular profile of KOA patients by analyzing SF, blood, and urine.
Pre-KOA
Although the identification of early KOA has gained widespread popularity in the past decade (Madry et al., 2016), there is growing awareness of the necessity to identify the pre-stage of KOA, where the molecular and cellular processes have kicked into action due to the presence of risk factors but without structural changes. To describe the long transition from healthy to clinically assessable early KOA, Ryd et al. defined pre-KOA as “A knee exhibiting one or many risk factors without pain, normal standing radiographs, no structural changes on arthroscopy or standard MRI, that is, before early KOA can be diagnosed” (Ryd et al., 2015). At this symptom- and imaging-free stage, the corresponding molecules of risk factors warrant special attention in the predictive work-up and in the preventive decision making. In turn, risk factors-related molecules may become a proxy for evaluating the efficacy of preventive interventions.
Obesity is a well-established risk factor for KOA. Lifetime risk of KOA rises with increasing body mass index, and longitudinal study shows that two-thirds of obese adults will develop symptomatic OA (Wluka et al., 2013). Despite the excessive joint loading by weight, increasing data shows that adipokines secreted by white adipose tissue indicate the start of KOA before the emergence of clinical manifestations (Abella et al., 2017). As the precursor of adipokines, leptin has been shown to be related to KOA initiation, and a serum elevation of 5 μg/L is associated with a 30% increased risk of structural KOA in obese participants (Karvonen-Gutierrez et al., 2012; Kroon et al., 2019). Similarly, serum resistin starts to increase even 5 years before the onset of radiographic KOA (Van Spil et al., 2012). There is consistent data showing that both leptin and resistin are responsible for activating innate immune responses and stimulating the expression of inflammatory cytokines, which ultimately result in cartilage and bone metabolic disorder (Conde et al., 2010; Acquarone et al., 2019; Xie and Chen, 2019). Accordingly, based on increased adipokine level, in designated cohorts of asymptomatic individuals with high risk for KOA, weight control to reduce adipose tissue mass should be suggested. Leptin and resistin serum level monitoring may help to assess the volatility risk of KOA. Actually, the reduction of 5% body weight within a 20-week period in overweight KOA patients helps them to experience symptomatic relief (Christensen et al., 2007), but the data on decreasing the initial risk of KOA is lacking.
Previous injury is a predominant joint-level risk factor for KOA initiation. Among the injuries, direct articular cartilage damage, anterior cruciate ligament (ACL) injury, and meniscal tear have been reported to be closely associated with KOA development (Vina and Kwoh, 2018). Both ACL injury and meniscal tear can disrupt the stability of knee, alongside with the disturbance of biomechanics and uneven distribution of mechanical load in cartilage (Englund et al., 2012; Wang et al., 2020). Simultaneously, some biomarkers are produced in response to mechanical stimuli (Chu et al., 2018). Among the candidate molecules for mirroring knee injury, cartilage oligomeric matrix protein (COMP) and C-telopeptide of type II collagen (CTX-II) are the most promising. COMP is a pentameric glycoprotein, highly expressed in hyaline cartilage, and plays a vital role in maintaining chondrocyte proliferation and ECM network integrity (Posey et al., 2018). CTX-II is a well-established biomarker for collagen II (Col-II) breakdown (Lattermann et al., 2016). In a community-based cohort study, the highest quartile level of serum COMP at baseline was correlated with the increased risk of radiographic KOA over 20 years (Kluzek et al., 2015). After running 200 km, COMP in the runners’ serum increased threefold, indicating that COMP could be a sensitive indicator of cartilage damage (Kim et al., 2007). After acute ACL injury, COMP concentration in SF is twofold increased within 6 weeks after injury and remains elevated in 5-year follow-up (Struglics et al., 2018). Similarly, the level of CTX-II in SF is significantly increased immediately after ACL injury (Lattermann et al., 2016). Therefore, given that post-traumatic KOA begins at the time of injury (Lattermann et al., 2016), the combination of injury history and elevated COMP and/or CTX-II levels might be a sensitive and effective index in predicting injury-related pre-KOA.
Female sex is another major causal risk factor of KOA, with a prevalence 1.2–2.8 times higher than males (Hunter and Bierma-Zeinstra, 2019; Sasaki et al., 2020). Therefore, interest is growing in the relationship between sex hormones and KOA. In a study enrolling 842 women, Sowers et al. (2006) found that the concentrations of estradiol and its metabolite, 2-hydroxyestrone, in the lowest tertile were strongly associated with higher KOA prevalence and incidence. Considering the protective role of estrogen in cartilage and bone homeostasis, estrogen-related drugs may be favorable for postmenopausal patients against KOA onset, but further preclinical and clinical studies are needed to confirm this (Xiao et al., 2016).
Although KOA is not considered as an autoimmune disease, activated molecular and cellular processes may stimulate maladaptive repair responses, which often include pro-inflammatory pathways of innate immunity and subsequent production of autoantibodies (AAbs) (Leslie et al., 2001; Geurts et al., 2018). Recently, Camacho-Encina et al. (2019) found that the serum level of methionine adenosyltransferase two beta autoantibody (MAT2β-AAb) increased as early as 8 years before the incidence of radiographic KOA. They further verified that the addition of MAT2β-AAb improved the efficiency of clinical prognostic model to identify high risk KOA individuals (Camacho-Encina et al., 2019). This could be applicable in KOA prediction. In addition, although preliminary data suggest that dietary supplementation of the product of MAT2β, S-adenosylmethionine (SAMe), can relieve KOA pain (Kim et al., 2009), further studies are needed to determine whether SAMe has a preventive effect on pre-KOA, especially in people with relatively high serum MAT2β-AAb levels.
Taken together, these predictive molecules will help to discriminate individuals with high risk of KOA initiation (pre-KOA) and may suggest the molecular based interventions to prevent KOA occurrence.
Early KOA
Luyten et al. (2012) proposed the definition and classification of early KOA, in which physical examination and imaging findings were included. In detail, early KOA should fulfill the following three criteria: (i) knee pain; (ii) KL grade < 2; (iii) cartilage lesions by arthroscopy or MRI, or meniscal or subchondral lesions by MRI (Luyten et al., 2012). Early KOA is thought to be a complicated phase, with limited and sporadic signs or symptoms, usually without early radiographs in most cases. Among this condition, molecules in body fluids can objectively provide useful diagnostic and prognostic information by mirroring the disease relevant biological activity (Mobasheri et al., 2017).
The molecular disorder is a consequence of disturbed gene expression landscape, which is fine tuned by small, non-coding RNAs named microRNAs (miRNAs). By inhibiting the function of protein-coding transcripts, miRNAs alter multiple aspects of cell structure and function, including chondrocytes phenotype (Swingler et al., 2019). Dysregulation of the miRNAs system, driving the disturbances of molecular composition, has been shown as an early detectable mechanism underlies KOA.
Among the miRNAs in early KOA, miR-140 and miR-210 are probably the most representative. Increasing evidence shows that miR-140 is capable for maintaining both cartilage and bone formation and homeostasis (Luo et al., 2018; Swingler et al., 2019). Also, it mediates the inhibition of IL1-induced proteinases (Si et al., 2019). However, the expression levels of miR-140 in SF is significantly reduced in early KOA patients compared with healthy individuals (Si et al., 2016). Furthermore, the SF levels of miR-140 were negatively correlated with the KL grades (Si et al., 2016), suggesting that the protective effect of miR-140 continued to be lost. miR-210 has been recognized as a major hypoxia-induced miRNA that contributes to the induction of angiogenesis (Bavelloni et al., 2017). Xie et al. found that miR-210 was significantly upregulated in patients with early-stage and late-stage KOA compared with healthy subjects (Xie et al., 2019), suggesting that the beginning of an increase in miR-210 level was an indicator of early KOA. These findings allow for early molecular diagnosis of KOA by detecting miRNAs. Other differentially expressed miRNAs, such as miR-19, miR-122, miR-146a, miR-186, miR-210, miR-223 and miR-486, are potential biomarkers of early KOA and still warrant further studies (Kong et al., 2017; Xie et al., 2019; Rousseau et al., 2020). Additionally, the development of disease-modifying drugs, especially miRNA therapy, might be attributed to a well-developed miRNA spectrum in early-KOA.
Another highly promising SF biomarker of early KOA is IL-17. By inducing de novo gene transcription or stabilizing target mRNA transcripts, IL-17 upregulates inflammatory gene expression, including IL-1β and TNFα, two major players of KOA pathophysiology (Kapoor et al., 2011; Amatya et al., 2017). The level of IL-17 in SF of KOA patients was significantly higher than that of healthy individuals, but there was no statistically significant difference between KOA groups based on KL grades (Liu et al., 2015). In addition, IL-17 level tended to decrease as the severity of KOA increased. This dynamic signature of changes in IL-17 level may herald that the start of IL-17 elevation indicates the onset of KOA. These characteristics represent the potential efficacy of IL-17 in providing early biological fingerprint of KOA, allowing for early diagnosis and identification of the cluster with inflammation-based KOA onset. Accordingly, the therapeutic effect of IL-17 inhibitors, such as the widely used secukinumab (McInnes et al., 2020), may be strengthened in selected clusters of early KOA individuals with high IL-17 levels, which has not been tested in clinical trials yet.
In addition to IL-17, IL-15 is also detected and viewed as a biomarker for early KOA (Scanzello et al., 2009). Produced by several cell types within the knee, including fibroblasts and macrophages, IL-15 has been shown to stimulate matrix metalloproteinase (MMPs) production, specifically MMP1 and MMP9 (Constantinescu et al., 2001; Waldmann et al., 2001). It was observed that the level of IL-15 in SF was significantly increased in early KOA and decreased in late KOA (Scanzello et al., 2009). Moreover, serum IL-15 levels were significantly higher in KOA patients compared with healthy individuals, but there was no significant correlation with KL grads (Sun et al., 2013). These findings suggest a potential role for IL-15 in the diagnosis of early KOA. Further studies focused on evaluating the utility of IL-15 in clinical practice and delineating molecular pathways responsible for IL-15 secretion are essential.
The identification of a comprehensive molecular profile for early KOA, including miRNAs, IL-17, IL-15, and future identified molecules, provides insight into the pathogenesis of KOA initiation. This strategy could promote the pathological mechanism-based diagnosis and facilitate the molecule-based treatment of early KOA.
Progressive KOA
Knee osteoarthritis is a heterogeneously progressive disease with different clinical phenotypes that eventually leading to a common final pathway of joint destruction (Castañeda et al., 2014). Persistent pathological factors contribute to the development of KOA from an early preventive stage toward an advanced, probably irreversible stage. Unlike accelerated KOA, which initiate and progress into advanced stage within 4 years (Driban et al., 2019), typical KOA is a slow-progressing disease, a process that usually takes decades. Whereas, the heterogeneous progression trajectories of KOA patients present as an obstacle in the development of disease-modifying drugs and the design of clinical trials (Halilaj et al., 2018). Furthermore, the current diagnosis and treatment strategies for KOA are still “one rule applies to all patients” (Yuan et al., 2020), highlighting the necessity for a more accurate molecular classification to provide evidence for targeted therapies. Hence, considering the characteristics of patients with KOA and the classification criteria for better clinical decision making (Dell’Isola and Steultjens, 2018), we classified progressive KOA into four subtypes based on the identified representative molecular profiles: cartilage degradation-driven, bone remodeling-driven, inflammation-driven, and pain-driven subtype (Figure 2). The pathogenesis-based first treatment options for different subgroups are also suggested (Table 2), but more solid data is indispensable to examine the correspondence between the altered molecules and therapeutic drugs.
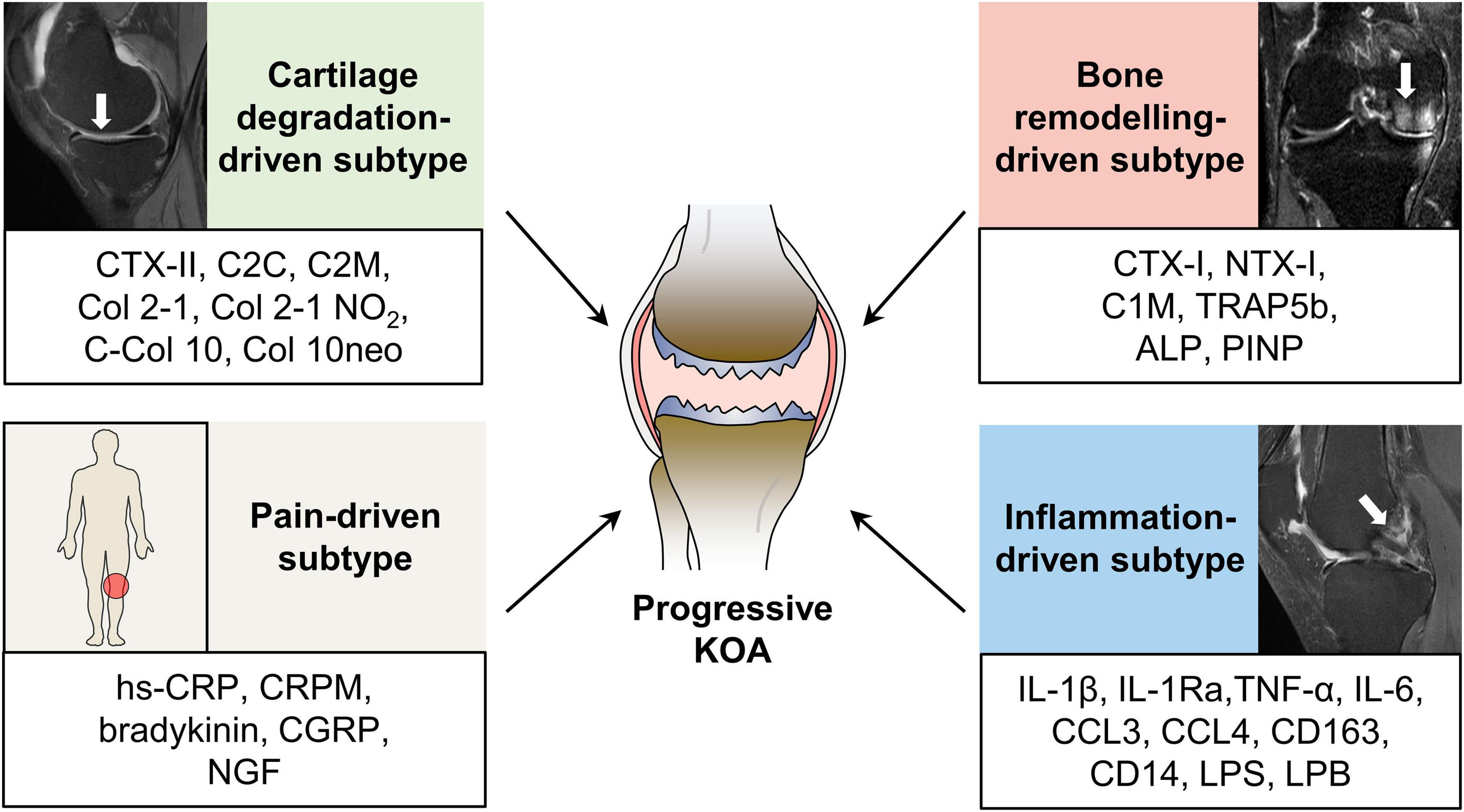
Figure 2. Molecular subtypes of progressive KOA. Increasing evidence suggests that progressive KOA patients fall into several subtypes based on the identified molecular profiles, including cartilage degradation-driven subtype, bone remodeling-driven subtype, inflammation-driven subtype, and pain-driven subtype. Representative molecules are listed in the box bellow the MRI manifestation of each subtype. ALP, alkaline phosphatase; CCL3, CC-chemokine ligand 3; CCL4, CC-chemokine ligand 4; Col 2-1 NO2, the nitrated form of Col 2-1; C-Col 10, C-terminus of collagen X; Col 10neo, a neoepitope of collagen 10; CGRP, calcitonin gene-related peptide; CRPM, the fragment of C-reactive protein; CTX-I, C-telopeptide of Col-I; CTX-II, C-telopeptide fragments of Col-II; C2C, the cleavage neoepitope of collagen II; C1M, the product of collagen I degraded by matrix metalloproteinases; C2M, the fragments of collagen II degraded by matrix metalloproteinases; hs-CRP, high sensitive C-reactive protein; IL-1β, interleukin 1β; IL-1Ra, IL-1 receptor antagonist; IL-6, interleukin 6; KOA, knee osteoarthritis; LPS, lipopolysaccharide; LPB, LPS binding protein; MRI, magnetic resonance imaging; NGF, nerve growth factor; NTX-I, N-telopeptide of Collagen I; PINP, N-terminal collagen type I extension propeptide; TNFα, tumor necrosis factor α; TRAP5b, tartrate resistant acid phosphatase 5b.
Cartilage Degradation-Driven Subtype
As the most typical phenotype of KOA lesions, cartilage loss is a dynamic alteration arising from an imbalance between its anabolism and catabolism, leading to changes in the properties of cartilage materials and increases its susceptibility to disruption by physical forces (Hunter and Bierma-Zeinstra, 2019). Detection of debris released from degraded cartilage provides the possibility of assessing cartilage loss during the progression of KOA.
Among the degradation of cartilage components, the most paradigmatic example derives from the disruption of Col-II because it is a major structural and highly specific molecule within cartilage (Martel-Pelletier et al., 2016). Numerous studies have shown that the urinary CTX-II was highly associated with the severity of KOA, mirroring the ongoing Col-II digestion by MMPs and cathepsins (Charni-Ben Tabassi et al., 2008; Xin et al., 2017; Sofat et al., 2019). Besides, CTX-II is an independent risk indicator of total knee arthroplasty (TKA) (Garnero et al., 2020), suggesting potential cartilage-targeting therapy attenuating KOA progression may delay TKA. Of particular interest, the magnitude of change in urinary CTX-II is responsive to the efficacy of conventional treatment measures, such as the chondroprotective glucosamine (Nagaoka et al., 2019). In this scenario, the assessment of CTX-II in urine might allow for the identification of patients who may benefit most from chondroprotective treatments, and CTX-II monitoring may help to evaluate the treatment efficacy dynamically. Notably, urinary CTX-II levels in women have been found significantly higher than in men (van Spil et al., 2013; Bihlet et al., 2019), highlighting its gender differences that need to be critically considered in clinical settings.
Other promising molecules reflecting the extent of Col-II degradation have been extensively explored as progressive KOA biomarkers. The fragments of Col-II degraded by MMPs (C2M) in serum have shown positive association with knee structural changes assessed by KL grades (Siebuhr et al., 2014). In a study enrolling 600 cases, Kraus et al. investigated that the cleavage neoepitope of Col-II (C2C) in urine was remarkably increased in established radiographic KOA individuals, and its time-integrated concentration on 24-month follow-up was predictive for KOA progression (Kraus et al., 2017a). Although detected in different body fluids, both C2M and C2C are suitable for the identification of Col-II degeneration.
After the denaturation of the triple helix of Col-II, Coll 2-1 is released and detectable in urine, as well as its nitrated form, Coll 2-1 NO2, which results from the nitration of aromatic amino acids by peroxynitrite under oxidative condition in KOA chondrocytes (Bolduc et al., 2019). In progressive KOA patients, both Coll 2-1 and Coll 2-1 NO2 are correlated with the severity of KOA, and their one-year changes are positively associated with joint space narrowing (Deberg et al., 2005), indicating Col-II degeneration and oxidative stress simultaneously happened in KOA pathophysiology. More importantly, the laboratory environment, sampling condition and circadian rhythm have no impact on the measurement of Coll 2-1 (Hick et al., 2019), ensuring Coll 2-1 as a reproducible and credible biomarker for evaluating Col-II degradation. Its responsiveness to treatment, such as intra-articular injection of hyaluronic acid (Henrotin et al., 2013), also warrants its use as an indicator for monitoring therapeutic efficacy.
Another promising strategy for evaluating cartilage degradation is the measurement of molecules reflecting the abnormally enhanced chondrocyte hypertrophy during cartilage destruction (Rim et al., 2020). Type X collagen (Col-X) is a major marker used to detect hypertrophic chondrocyte. In a cluster of 271 KOA patients stratified by KL grade, the serum levels of the C-terminus of Col-X (C-Col 10) were positively correlated with cartilage degeneration (He et al., 2014). In a recent investigation, the higher levels of a neoepitope of Col-X (Col 10neo), 479GIATKG, in urine were associated with greater KL scores (He et al., 2019), indicating the ongoing hypertrophic process of chondrocytes during KOA progression. Further validation of C-Col 10 and Col 10neo in large-scale clinical trials is needed with the purpose of their application in clinical practice.
Thus, in progressive KOA, molecules mirroring cartilage degenerative and chondrocyte hypertrophic process help to select cartilage degradation-driven subtype patients, who may benefit most from chondroprotective interventions.
Bone Remodeling-Driven Subtype
Bone remodeling is a result of the coupling of osteoblastic bone formation and osteoclastic bone resorption (Hu et al., 2021). For mechanically unstable joints, the subchondral bone may exhibit bone bruise, known as bone marrow edema on MRI, which has been shown to be a potent risk factor for disease structural and symptomatic progression in patients with KOA (Felson et al., 2003). Intriguingly, mounting evidence suggests that bone resorption activation mainly occurs in early-stage KOA, whereas, bone formation activation is the major characteristic of late-stage KOA (Funck-Brentano and Cohen-Solal, 2011). Although subchondral bone abnormality is not presented in all KOA individuals, it is indeed the earliest pathological change in a fraction of patients (Hu et al., 2021). Therefore, the identification of molecular profiles in progressive KOA would help to stratify patients to the bone remodeling-driven subtype and provide evidence for the application of pathogenesis-based pharmaceutical medications.
Type I collagen (Col-I) is the most abundant protein in bone, accounting for 90% of total bone protein (Eastell and Szulc, 2017). Attaching to bone with a sealing zone, osteoclasts secrete acid to dissolve the bone mineral (Martel-Pelletier et al., 2016). After that, osteoclasts release enzymes (e.g., cathepsin K) to digest proteins and release the fragments, such as C-telopeptide of Col-I (CTX-I) and N-telopeptide of Col-I (NTX-I), which are indicative of osteoclast activity (Eastell and Szulc, 2017). Several clinical trials with more than 1,000 participants have shown that both urinary CTX-I and serum NTX-I were positively correlated with the symptomatic and radiographic severity of KOA (Kraus et al., 2017b; Bihlet et al., 2019), indicating the activation of bone absorption. In parallel, C1M, the product of Col-I degraded by MMPs, is also suggestive for KOA progression (Siebuhr et al., 2014). Remarkably, monitoring the levels of CTX-I and NTX-I allows for assessing the therapeutic efficacy of targeting bone absorption treatment, as they are significantly reduced in a dose-dependent manner after the treatment of a selective cathepsin K inhibitor, MIV-711 (Lindström et al., 2018). Tartrate resistant acid phosphatase 5b (TRAP5b, also known as ACP5) is one of the osteoclasts produced enzymes. It is fairly specific to bone and its level is responsible for reflecting the number of osteoclasts (Lv et al., 2015). Serum levels of TRAP5b have been shown associated with the severity of knee symptoms in KOA individuals (Nwosu et al., 2017), providing evidence for the aggregation of osteoclasts during bone remodeling. Based on this, KOA patients with obviously active bone absorption may benefit most from the antiresorptive agents, such as bisphosphonates, cathepsin K inhibitor and calcitonin.
Osteoblasts express the highest concentration of collagen during their proliferative phase and of bone alkaline phosphatase (ALP) during matrix maturation (Stein and Lian, 1993). N-terminal collagen type I extension propeptide (PINP), primarily originates from bone, is derived from the post-translational cleavage of type I procollagen and has been well established as a bone formation biomarker (Eastell and Szulc, 2017). Increasing evidence supports that KOA progression, especially the osteophytosis progression, was preceded and accompanied by the enhanced bone formation as assessed by the values of serum PINP (Kumm et al., 2013). Besides, Park et al. (2020) demonstrated that serum ALP activity was independently and positively correlated with KOA severity by including 3,060 participants. The elevation of serum PINP and ALP might be useful in the assessment of abnormal bone formation, such as subchondral bone sclerosis and osteophyte formation, in progressive KOA. In such cases, antiresorptive treatment may be inappropriate and may aggravate the abnormal bone formation. Unfortunately, therapeutic agents targeting the abnormal activation of bone formation has been scarcely investigated.
Several clinical trials have been focused on evaluating the potential therapeutic efficacy of anti-absorption agents on KOA, regrettably, with scarce data on the beneficial effects. For instance, intravenous zoledronic acid, a classical antiresorptive agent, did not show a significant improvement of pain score and the reduction of cartilage volume loss in KOA patients, even in those with bone marrow lesions (Aitken et al., 2018; Cai et al., 2020). The most likely reason for the failure may be that their inclusion criteria were based only on clinical and radiographic diagnosis, which is heterogeneous in molecular level. Further molecular based stratification may help to enroll the biologically homogeneous KOA patients with active bone remodeling and facilitate the highest potential success of the remodeling targeting agents.
Inflammation-Driven Subtype
Accumulating evidence supports that the inflammation in KOA is chronic, comparatively low-grade, and primarily mediated by the innate immune system (Robinson et al., 2016). Clinically, many patients with KOA have symptoms of joint inflammation, such as pain, morning stiffness, and warmth (Sellam and Berenbaum, 2010). Hence, therapeutic strategies targeting the low-grade inflammation may be able to halt KOA progression. However, disappointing results have been described in several tested anti-inflammatory therapeutics (McAlindon et al., 2017; Deyle et al., 2020). Given the heterogeneity of KOA, revealing the molecular characteristics of inflammation during disease progression might help to recruit homogenous patients for clinical trials testing anti-inflammatory agents.
Highlighting the role of inflammation, much interest has been expressed in identifying secreted inflammatory cytokines in the pathophysiology of KOA progression. The most widely studied biomarkers are IL-1β and TNF-α, two major players of KOA inflammation. Increasing evidence shows that both the serum levels of IL-1β and TNF-α are highly associated with the symptomatic and radiographic progression of KOA (Attur et al., 2011, 2020; Larsson et al., 2015). Their effects can generally be described as the inhibition of cartilage anabolism, activation of cartilage catabolism, and perpetuation of inflammatory responses by inducing the production of other proinflammatory cytokines, such as IL-6 (Livshits et al., 2009; Kapoor et al., 2011). Despite the extensively available non-steroidal anti-inflammatory drugs (NSAIDs) and steroids showing no solid data of restoring the joint damage, anti-IL-1β and anti-TNF-α therapies in several clinical trials represent promising therapeutic efficacy as determined by the relief of pain (Grunke and Schulze-Koops, 2006; Wang et al., 2017). Whereas, the neglection of matching molecular profile with therapeutic mechanisms might be the reason of a recent failure attempting to target IL-1β (Fleischmann et al., 2019). Intriguingly, the plasmatic levels of IL-1 receptor antagonist (IL-1Ra), a natural inhibitor of IL-1, has been shown to be independently associated with the progression of symptomatic KOA (Attur et al., 2015). IL-1Ra possesses anti-inflammatory properties by competitively binding to IL-1 receptors with no signaling transduction effects. The elevation of IL-1Ra may indicate the burden of tissue exposure to inflammation and the endogenous attempt to antagonize the overproduced IL-1β. Techniques delivering IL-1Ra into joint cavity without altering its biological activity may provide new insight for KOA-modifying strategies (Agarwal et al., 2016).
Synovitis is highly associated with KOA progression. Indeed, growing evidence supports the role of immune cells, particularly macrophages, in KOA pathophysiology (Zhang et al., 2020). Some molecules reflecting the infiltration and accumulation of macrophages within the synovium have been proposed as biomarkers of KOA progression (Zhao et al., 2015). The levels of plasma chemokines, such as CCL3 and CCL4, are associated with KOA severity, indicating the infiltration of macrophages and the progression of synovitis in KOA (Zhao et al., 2015). Consequently, the SF levels of CD163 and CD14, two soluble markers for macrophages, are positively associated with the abundance of activated macrophages in synovium (Daghestani et al., 2015), allowing for the timely assessment of macrophage-mediated synovitis progression. The validation of these macrophage-related molecules is needed in view of their application in the development of macrophage targeting therapy.
In addition to local inflammation of the joint, systemic inflammation may also have a vital role in KOA pathogenesis (Huang and Kraus, 2016). For example, obesity is known as an important risk factor of KOA progression possibly not only by the increased mechanical load on the knee joint, but also by the perturbation of the intestinal microbiota and the harvest of persistent and low-grade inflammatory response (Berenbaum et al., 2013; Cox et al., 2015). Weight loss can alleviate KOA symptoms by the substantial reduction of systemic levels of C-reactive protein (CRP) and IL-6, two well-established biomarkers of KOA progression (Beavers et al., 2015). In this context, the assessment of weight loss may represent the decrease of systemic inflammation. Interestingly, lipopolysaccharide (LPS; also known as endotoxin) and LPS binding protein (LBP) are positively associated with the quantity of activated macrophages in knee joint (Huang et al., 2016). In line with this, their levels are also associated with clinical manifestations including total Western Ontario and McMaster University Osteoarthritis Index (WOMAC) scores and self-reported knee pain (Huang et al., 2016).
Identifying the molecular profiles, including the local and systemic inflammatory cytokines, would help reveal the major inflammatory mechanisms and stratify KOA patients with different molecular characteristics. This strategy could facilitate the diagnosis of inflammation-driven subtype of KOA and the development of molecular based treatment strategies.
Pain-Driven Subtype
Pain is a major driver of health service use and clinical decision making of KOA (Neogi, 2013). However, the origin and mechanisms of pain remain enigmatic. At present, KOA pain is mainly controlled by NSAIDs and analgesics, with unsustainable pain relief and substantial adverse effects (O’Neil et al., 2012). The presence and severity of pain have been shown due to bone marrow lesions and synovitis, and in turn, a change in pain within a person relates to a change in synovitis or in the number or size of bone marrow lesions (Yusuf et al., 2011; Zhang et al., 2011), which may indicate inflammation or bone remodeling-related molecular mechanisms of pain. Therapeutic strategies might vary between patients depending on their underlying disease mechanisms.
Numerous works focused on the anti-inflammatory strategies that might be capable of alleviating KOA pain. Among the inflammatory molecules related to KOA pain, CRP is extensively and long-term used in reflecting the inflammatory response. Jin et al. included 32 studies in their meta-analysis and found that serum high sensitive CRP (hs-CRP) levels were significantly correlated with knee pain and physical function decline, but not with radiographic changes (Jin et al., 2015), indicating that hs-CRP could reflect KOA symptoms progression. Among KOA patients, elevated CRP levels were associated with heightened pain sensitivity (Lee et al., 2011). Of particular interest, the trends of CRP concentrations in serum and SF were consistent and responsive to KOA treatments (Rondanelli et al., 2019). Accordingly, CRP levels may contribute to the selection of patients with inflammatory pain and could monitor the analgesic effect of anti-inflammatory strategies. After degradation by MMPs, the fragment of CRP (CRPM) can be detected in body fluids. CRPM is related to the central pain sensitization and the risk of symptomatic KOA progression (Arendt-Nielsen et al., 2014). However, the levels of CRP and CRPM may be subject to a variety of pathophysiology disturbances, which reduced their specificity to KOA. Future work should address how to improve the specificity of CRP to KOA pain.
Bradykinin is another promising peptide involved in KOA inflammatory pain by exciting and sensitizing sensory nerve fibers (Wang et al., 2005). As a vasodilator and inflammatory nonapeptide, bradykinin is generated in synovium, and its level in SF is related to KOA progression (Bellucci et al., 2013). In KOA patients, intra-articular injection of a specific bradykinin B2 receptor antagonist showed a long-lasting analgesic effect (Meini and Maggi, 2008). Such molecular based analgesic treatment provides an exemplary therapeutic algorithm for KOA pain management.
Recent studies found that several neuronal factors related to bone remodeling played an important role in the innervation of sensory nerves (Martel-Pelletier et al., 2016). During bone absorption, osteoclasts secret H+ to induce an acidic microenvironment and then activate the acid-sensing receptor transient receptor potential vanilloid 1, which is responsible for the transcriptional activation of calcitonin gene-related peptide (CGRP), a well-established pain responder (Yoneda et al., 2015). The levels of CGRP have shown to be positively associated with KL grades and the WOMAC pain scores (Dong et al., 2015), suggesting its role in reflecting KOA progression. However, the humanized monoclonal antibody of CGRP, galcanezumab, failed to reduce KOA pain (Jin et al., 2018), which might be a result of the heterogeneity of CGRP levels in their participants. Preosteoclasts can produce nerve growth factor (NGF), which is a key driver of subchondral nerve innervation (Hu et al., 2021). Binding to its high affinity receptor TrKA, NGF excites TrKA+ sensory neurons, leading to the hypersensitivity and hyperexcitability of nociceptors, which is one of the most fundamental mechanisms of clinical pain (Schmelz et al., 2019; Malfait et al., 2020). In the serum and SF of KOA patients, the levels of NGF and TrKA showed a stage-dependent increase in KOA (Montagnoli et al., 2017), indicating their role in mirroring KOA progression. Several studies utilized NGF-neutralizing monoclonal antibodies showed imperative analgesic effects, but with adverse events of unclear etiology (Jayabalan and Schnitzer, 2017; Miller et al., 2017). Further studies should focus on the mechanisms of the side effects produced by NGF blockade therapy.
Collectively, further exploration of the molecular characteristics of KOA pain may allow for the identification of patients belonging to the pain-driven subtype. For these patients, analgesia will be the first treatment option. According to the major molecular mechanisms, the development of specific drugs for pain management will be an excellent approach.
End-Stage KOA
End-stage KOA has been viewed as a knee with considerable pain and functional limitations, accompanied by structural damage and/or other complications, such as flexion contractures and joint laxity, that prohibit the normal use of a joint (Driban et al., 2016). Generally, end-stage KOA is characterized as KL grade 4 on radiography (Guermazi et al., 2015). For patients at this stage, TKA surgery should be considered if medical interventions failed to improve persistent debilitating symptom (Martel-Pelletier et al., 2016). Although it is easy to diagnose end-stage KOA by symptoms and imaging methods, the value of some molecules in predicting end-stage KOA and evaluating the prognosis of TKA cannot be underestimated.
As aforementioned, KOA is an inflammatory disease with persistent and low-grade inflammation. Among the indicators from blood test, neutrophil-lymphocyte ratio (NLR) has become a useful, economical and simple tool for reflecting inflammation. In a study enrolling 176 KOA patients, blood NLR was significantly higher in the severe (KL grade 4) group (2.18 ± 1.04) than in the mild to moderate group (1.79 ± 0.8), suggesting NLR as an indicator of end-stage KOA (Taşoğlu et al., 2016). Further analysis revealed that NLR ≥ 2.1 was an independent predictor of severe KOA, with a specificity of 77% and a sensitivity of 50% (Taşoğlu et al., 2016). Although these findings are provocative, further longitudinal studies are needed, given that a single blood sample does not provide a stable assessment of NLR.
To explore the relationship between serum miRNA levels and the occurrence of severe KOA, Beyer et al. identified differentially expressed miRNAs in a population-based cohort including 816 individuals (Beyer et al., 2015). They found that let-7e emerged as the most promising predictor of severe KOA necessitating arthroplasty, and that let-7e levels were negatively correlated with the frequency of surgical knee replacement in end-stage KOA (Beyer et al., 2015). This finding may bring light to a new method for the prognostic evaluation of TKA. Further exploration to validate the role of let-7e in different applications, such as KOA pathological mechanism, and disease activity, is warranted to assess its incremental value.
Future Directions
Given the emerging evidence demonstrating biomarkers for KOA (Mobasheri et al., 2017; van Spil and Szilagyi, 2020), it is conceivable that molecular diagnostic and therapeutic algorithm could be well developed. Based on the representative molecules, classifying KOA patients into different stages and subtypes should logically have an important effect on clinical decision making by presenting the ongoing pathological processes. We clearly recognize that the KOA subtypes are not necessarily mutually exclusive, and sometimes overlap, for example, patients with subchondral bone lesions usually suffer pain (Moisio et al., 2009). However, this does not diminish the clinical significance of molecular classification. A combination of treatments targeting different mechanisms may be effective. Before this, the effect of specific therapies targeting molecular characteristics firstly warrant further validation in longitudinal studies.
In the past decade, MRI has been rapidly evolved due to technical advances, and its application in clinical research has provided sufficient evidence regarding the feature of disease (Link et al., 2003; Li and Majumdar, 2013; Roemer et al., 2020). According to the predominant structural alterations under MRI, KOA has been previously stratified as five different phenotypes (Roemer et al., 2018). However, more accurate information about the pathological characteristics of each phenotype would help to promote the development of disease-modifying KOA drugs. In the future, the combination of clinical parameters, MRI, and molecular information would form a comprehensive diagnosis and treatment algorithm: clinical and imaging characteristics will be used for the initial screening of KOA subtypes, and molecular characteristics will play a dominant role in predicting high risk individuals and determining the drug selection.
In addition, in order to prevent the influence of other diseases, population with similar baseline conditions should be preferred in clinical trials evaluating the KOA molecules. Future studies should also focus on verifying the efficacy and threshold values of a single molecule, or a panel of molecules, in clinical settings, and exploring new molecules to improve the molecular classification to promote its clinical application.
Conclusion
In KOA, different interlinked molecules cause and sustain the pathogenesis, as early as before the clinical and radiographic manifestations are available. As such, based on the representatively investigated molecules, we proposed the novel KOA molecular classification (Figure 3), which offers the possibility to: (i) predict patients at high risk of KOA initiation; (ii) select patients in early and progressive stage, when disease-modifying drugs have the best chance of a successful outcome; (iii) stratify biologically homogenous patients, who may benefit most from therapeutic agents in clinical trials and clinical settings; (iv) provide monitoring biomarkers for the assessment of treatment efficacy; and (v) offer molecular evidence for the development of disease-modifying drugs. Although this molecular KOA classification is merely a simple concept that needs to be further refined, its impact on preclinical and clinical studies is increasing because of the growing need to match molecular mechanisms with treatment strategies.
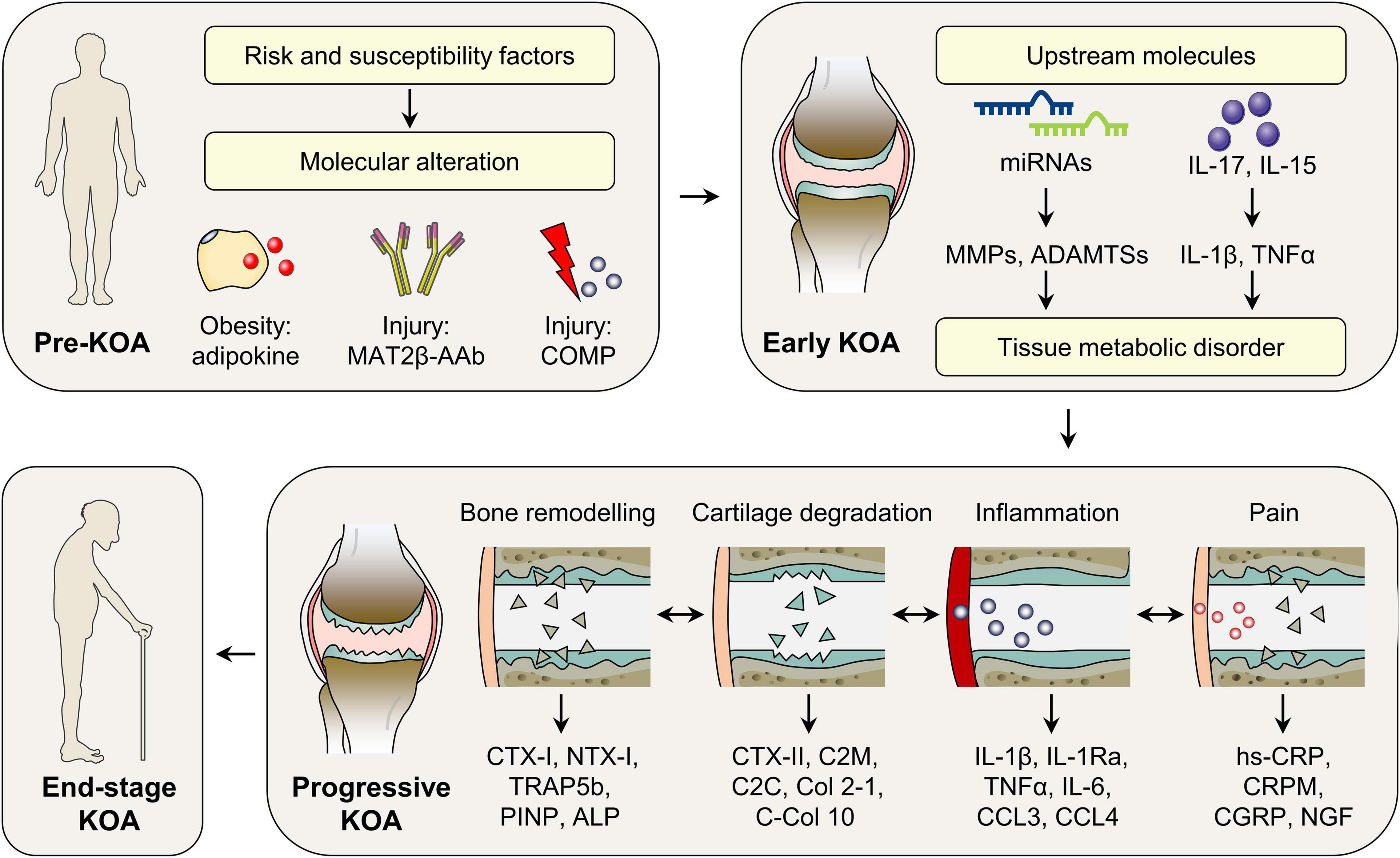
Figure 3. Molecular classification of KOA. According to the temporal alteration of representative molecules during disease initiation and progression, KOA could be classified into pre-KOA, early KOA, progressive KOA, and end-stage KOA. In pre-KOA, risk factors-related molecules, such as adipokines, COMP, and MAT2β-AAb, can be detected in body fluids, which may provide evidence for KOA prediction. In early KOA, molecules can reflect the ongoing pathogenesis, when symptoms and imaging information are ambiguous. Based on the major pathophysiology in patient clusters, progressive KOA is further classified into four subtypes, i.e., cartilage degradation-driven subtype, bone remodeling driven subtype, inflammation-driven subtype, and pain-driven subtype, suggesting different treatment options in future clinical setting. ADAMTSs, a disintegrin and metalloproteinase with thrombospondin motifs; ALP, alkaline phosphatase; CCL3, CC-chemokine ligand 3; CCL4, CC-chemokine ligand 4; C-Col 10, C-terminus of collagen X; CGRP, calcitonin gene-related peptide; COMP, cartilage oligomeric matrix protein; CRPM, the fragment of CRP; CTX-I, C-telopeptide of Col-I; CTX-II, C-telopeptide fragments of Col-II; C2C, the cleavage neoepitope of collagen II; C2M, the fragments of collagen II degraded by matrix metalloproteinases; hs-CRP, high sensitive CRP; IL-1β, interleukin 1β; IL-1Ra, IL-1 receptor antagonist; IL-6, interleukin 6; IL-15, interleukin 15; IL-17, interleukin 17; KOA, knee osteoarthritis; MAT2β-AAb, methionine adenosyltransferase two beta autoantibody; miRNAs, microRNAs; MMPs, matrix metalloproteinases; NGF, nerve growth factor; NTX-I, N-telopeptide of Collagen I; PINP, N-terminal collagen type I extension propeptide; TNFα, tumor necrosis factor α; TRAP5b, tartrate resistant acid phosphatase 5b.
Search Strategy and Selection Criteria
We searched titles and abstracts in PubMed, using the search term “osteoarthritis” in combination with, but not limited to, “knee,” “biomarker,” “diagnosis,” “stage,” “progression,” “classification,” “body fluid,” “synovial fluid,” “blood,” “serum,” “urine,” “pathophysiology,” “subtype,” “phenotype,” and “clinical trial.” We mostly selected publications from the past 10 years, although commonly referenced and important older publications were not excluded. English articles presenting data on soluble biomarkers in body fluids (synovial fluid, blood, and urine) of human KOA were included.
Author Contributions
All authors listed have made a substantial, direct and intellectual contribution to the work, and approved it for publication.
Funding
This work was supported by National Key R&D Program of China (2018YFC1105904), Key Program of NSFC (81730067), National Science Foundation of China (81772335, 81941009, and 81802196), Natural Science Foundation of Jiangsu Province, China (BK20180127), Jiangsu Provincial Key Medical Talent Foundation, Six Talent Peaks Project of Jiangsu Province (WSW-079).
Conflict of Interest
The authors declare that the research was conducted in the absence of any commercial or financial relationships that could be construed as a potential conflict of interest.
Publisher’s Note
All claims expressed in this article are solely those of the authors and do not necessarily represent those of their affiliated organizations, or those of the publisher, the editors and the reviewers. Any product that may be evaluated in this article, or claim that may be made by its manufacturer, is not guaranteed or endorsed by the publisher.
References
Abella, V., Scotece, M., Conde, J., Pino, J., Gonzalez-Gay, M. A., Gómez-Reino, J. J., et al. (2017). Leptin in the interplay of inflammation, metabolism and immune system disorders. Nat. Rev. Rheumatol. 13, 100–109. doi: 10.1038/nrrheum.2016.209
Acquarone, E., Monacelli, F., Borghi, R., Nencioni, A., and Odetti, P. (2019). Resistin: a reappraisal. Mech. Ageing Dev. 178, 46–63. doi: 10.1016/j.mad.2019.01.004
Agarwal, R., Volkmer, T. M., Wang, P., Lee, L. A., Wang, Q., and García, A. J. (2016). Synthesis of self-assembled IL-1Ra-presenting nanoparticles for the treatment of osteoarthritis. J. Biomed. Mater. Res. A 104, 595–599. doi: 10.1002/jbm.a.35601
Aitken, D., Laslett, L. L., Cai, G., Hill, C., March, L., Wluka, A. E., et al. (2018). A protocol for a multicentre, randomised, double-blind, placebo-controlled trial to compare the effect of annual infusions of zoledronic acid to placebo on knee structural change and knee pain over 24 months in knee osteoarthritis patients – ZAP2. BMC Musculoskelet. Disord. 19:217. doi: 10.1186/s12891-018-2143-2
Akul, M., Santul, B., Daniel, H., Monte, H., Ravindra, K., and Sadanand, F. (2019). Advances in molecular biomarker for early diagnosis of osteoarthritis. Biomol. Concepts 10, 111–119. doi: 10.1515/bmc-2019-0014
Amatya, N., Garg, A. V., and Gaffen, S. L. (2017). IL-17 signaling: the Yin and the Yang. Trends Immunol. 38, 310–322. doi: 10.1016/j.it.2017.01.006
Arendt-Nielsen, L., Eskehave, T. N., Egsgaard, L. L., Petersen, K. K., Graven-Nielsen, T., Hoeck, H. C., et al. (2014). Association between experimental pain biomarkers and serologic markers in patients with different degrees of painful knee osteoarthritis. Arthritis Rheumatol. 66, 3317–3326. doi: 10.1002/art.38856
Attur, M., Belitskaya-Lévy, I., Oh, C., Krasnokutsky, S., Greenberg, J., Samuels, J., et al. (2011). Increased interleukin-1β gene expression in peripheral blood leukocytes is associated with increased pain and predicts risk for progression of symptomatic knee osteoarthritis. Arthritis Rheum. 63, 1908–1917. doi: 10.1002/art.30360
Attur, M., Krasnokutsky, S., Zhou, H., Samuels, J., Chang, G., Bencardino, J., et al. (2020). The combination of an inflammatory peripheral blood gene expression and imaging biomarkers enhance prediction of radiographic progression in knee osteoarthritis. Arthritis Res. Ther. 21:208.
Attur, M., Statnikov, A., Samuels, J., Li, Z., Alekseyenko, A. V., Greenberg, J. D., et al. (2015). Plasma levels of interleukin-1 receptor antagonist (IL1Ra) predict radiographic progression of symptomatic knee osteoarthritis. Osteoarthritis Cartilage 23, 1915–1924. doi: 10.1016/j.joca.2015.08.006
Bannuru, R. R., Natov, N. S., Dasi, U. R., Schmid, C. H., and McAlindon, T. E. (2011). Therapeutic trajectory following intra-articular hyaluronic acid injection in knee osteoarthritis – meta-analysis. Osteoarthritis Cartilage 19, 611–619. doi: 10.1016/j.joca.2010.09.014
Bannuru, R. R., Osani, M. C., Vaysbrot, E. E., Arden, N. K., Bennell, K., Bierma-Zeinstra, S. M. A., et al. (2019). OARSI guidelines for the non-surgical management of knee, hip, and polyarticular osteoarthritis. Osteoarthritis Cartilage 27, 1578–1589. doi: 10.1016/j.joca.2019.06.011
Bavelloni, A., Ramazzotti, G., Poli, A., Piazzi, M., Focaccia, E., Blalock, W., et al. (2017). MiRNA-210: a current overview. Anticancer Res. 37, 6511–6521.
Beavers, K. M., Beavers, D. P., Newman, J. J., Anderson, A. M., Loeser, R. F. Jr., Nicklas, B. J., et al. (2015). Effects of total and regional fat loss on plasma CRP and IL-6 in overweight and obese, older adults with knee osteoarthritis. Osteoarthritis Cartilage 23, 249–256. doi: 10.1016/j.joca.2014.11.005
Bellucci, F., Meini, S., Cucchi, P., Catalani, C., Nizzardo, A., Riva, A., et al. (2013). Synovial fluid levels of bradykinin correlate with biochemical markers for cartilage degradation and inflammation in knee osteoarthritis. Osteoarthritis Cartilage 21, 1774–1780. doi: 10.1016/j.joca.2013.08.014
Berenbaum, F., Blanco, F. J., Guermazi, A., Miki, K., Yamabe, T., Viktrup, L., et al. (2020). Subcutaneous tanezumab for osteoarthritis of the hip or knee: efficacy and safety results from a 24-week randomised phase III study with a 24-week follow-up period. Ann. Rheum. Dis. 79, 800–810. doi: 10.1136/annrheumdis-2019-216296
Berenbaum, F., Eymard, F., and Houard, X. (2013). Osteoarthritis, inflammation and obesity. Curr. Opin. Rheumatol. 25, 114–118. doi: 10.1097/bor.0b013e32835a9414
Beyer, C., Zampetaki, A., Lin, N.-Y., Kleyer, A., Perricone, C., Iagnocco, A., et al. (2015). Signature of circulating microRNAs in osteoarthritis. Ann. Rheum. Dis. 74:e18.
Bihlet, A. R., Byrjalsen, I., Bay-Jensen, A.-C., Andersen, J. R., Christiansen, C., Riis, B. J., et al. (2019). Associations between biomarkers of bone and cartilage turnover, gender, pain categories and radiographic severity in knee osteoarthritis. Arthritis Res. Ther. 21:203.
Bolduc, J. A., Collins, J. A., and Loeser, R. F. (2019). Reactive oxygen species, aging and articular cartilage homeostasis. Free Radic. Biol. Med. 132, 73–82. doi: 10.1016/j.freeradbiomed.2018.08.038
Cai, G., Aitken, D., Laslett, L. L., Pelletier, J.-P., Martel-Pelletier, J., Hill, C., et al. (2020). Effect of intravenous zoledronic acid on tibiofemoral cartilage volume among patients with knee osteoarthritis with bone marrow lesions: a randomized clinical trial. JAMA 323, 1456–1466. doi: 10.1001/jama.2020.2938
Camacho-Encina, M., Balboa-Barreiro, V., Rego-Perez, I., Picchi, F., VanDuin, J., Qiu, J., et al. (2019). Discovery of an autoantibody signature for the early diagnosis of knee osteoarthritis: data from the Osteoarthritis Initiative. Ann. Rheum. Dis. 78, 1699–1705. doi: 10.1136/annrheumdis-2019-215325
Castañeda, S., Roman-Blas, J. A., Largo, R., and Herrero-Beaumont, G. (2014). Osteoarthritis: a progressive disease with changing phenotypes. Rheumatology 53, 1–3. doi: 10.1093/rheumatology/ket247
Charni-Ben Tabassi, N., Desmarais, S., Bay-Jensen, A. C., Delaissé, J. M., Percival, M. D., and Garnero, P. (2008). The type II collagen fragments Helix-II and CTX-II reveal different enzymatic pathways of human cartilage collagen degradation. Osteoarthritis Cartilage 16, 1183–1191. doi: 10.1016/j.joca.2008.02.008
Christensen, R., Bartels, E. M., Astrup, A., and Bliddal, H. (2007). Effect of weight reduction in obese patients diagnosed with knee osteoarthritis: a systematic review and meta-analysis. Ann. Rheum. Dis. 66, 433–439. doi: 10.1136/ard.2006.065904
Chu, C. R., Sheth, S., Erhart-Hledik, J. C., Do, B., Titchenal, M. R., and Andriacchi, T. P. (2018). Mechanically stimulated biomarkers signal cartilage changes over 5 years consistent with disease progression in medial knee osteoarthritis patients. J. Orthop. Res. 36, 891–897.
Conaghan, P. G., Bowes, M. A., Kingsbury, S. R., Brett, A., Guillard, G., Rizoska, B., et al. (2019). Disease-modifying effects of a novel cathepsin K inhibitor in osteoarthritis. Ann. Intern. Med. 172, 86–95. doi: 10.7326/m19-0675
Conde, J., Scotece, M., Gómez, R., Gómez-Reino, J. J., Lago, F., and Gualillo, O. (2010). At the crossroad between immunity and metabolism: focus on leptin. Expert Rev. Clin. Immunol. 6, 801–808. doi: 10.1586/eci.10.48
Constantinescu, C. S., Grygar, C., Kappos, L., and Leppert, D. (2001). Interleukin 15 stimulates production of matrix metalloproteinase-9 and tissue inhibitor of metalloproteinase-1 by human peripheral blood mononuclear cells. Cytokine 13, 244–247. doi: 10.1006/cyto.2000.0818
Cox, A. J., West, N. P., and Cripps, A. W. (2015). Obesity, inflammation, and the gut microbiota. Lancet Diabetes Endocrinol. 3, 207–215. doi: 10.1016/b978-0-12-821039-0.00003-4
Daghestani, H. N., Pieper, C. F., and Kraus, V. B. (2015). Soluble macrophage biomarkers indicate inflammatory phenotypes in patients with knee osteoarthritis. Arthritis Rheumatol. 67, 956–965. doi: 10.1002/art.39006
Deberg, M. A., Labasse, A. H., Collette, J., Seidel, L., Reginster, J. Y., and Henrotin, Y. E. (2005). One-year increase of Coll 2-1, a new marker of type II collagen degradation, in urine is highly predictive of radiological OA progression. Osteoarthritis Cartilage 13, 1059–1065. doi: 10.1016/j.joca.2005.06.014
Dell’Isola, A., and Steultjens, M. (2018). Classification of patients with knee osteoarthritis in clinical phenotypes: data from the osteoarthritis initiative. PLoS One 13:e0191045. doi: 10.1371/journal.pone.0191045
Deyle, G. D., Allen, C. S., Allison, S. C., Gill, N. W., Hando, B. R., Petersen, E. J., et al. (2020). Physical therapy versus glucocorticoid injection for osteoarthritis of the knee. N. Engl. J. Med. 382, 1420–1429.
Dong, T., Chang, H., Zhang, F., Chen, W., Zhu, Y., Wu, T., et al. (2015). Calcitonin gene-related peptide can be selected as a predictive biomarker on progression and prognosis of knee osteoarthritis. Int. Orthop. 39, 1237–1243. doi: 10.1007/s00264-015-2744-4
Driban, J. B., Davis, J. E., Lu, B., Price, L. L., Ward, R. J., MacKay, J. W., et al. (2019). Accelerated knee osteoarthritis is characterized by destabilizing meniscal tears and preradiographic structural disease burden. Arthritis Rheumatol. 71, 1089–1100. doi: 10.1002/art.40826
Driban, J. B., Price, L. L., Lynch, J., Nevitt, M., Lo, G. H., Eaton, C. B., et al. (2016). Defining and evaluating a novel outcome measure representing end-stage knee osteoarthritis: data from the Osteoarthritis Initiative. Clin. Rheumatol. 35, 2523–2530. doi: 10.1007/s10067-016-3299-5
Eastell, R., and Szulc, P. (2017). Use of bone turnover markers in postmenopausal osteoporosis. Lancet Diabetes Endocrinol. 5, 908–923. doi: 10.1016/s2213-8587(17)30184-5
Englund, M., Roemer, F. W., Hayashi, D., Crema, M. D., and Guermazi, A. (2012). Meniscus pathology, osteoarthritis and the treatment controversy. Nat. Rev. Rheumatol. 8, 412–419. doi: 10.1038/nrrheum.2012.69
Fan, X., Wu, X., Crawford, R., Xiao, Y., and Prasadam, I. (2021). Macro, micro, and molecular. Changes of the osteochondral interface in osteoarthritis development. Front. Cell Dev. Biol. 9:659654. doi: 10.3389/fcell.2021.659654
Felson, D. T., McLaughlin, S., Goggins, J., LaValley, M. P., Gale, M. E., Totterman, S., et al. (2003). Bone marrow edema and its relation to progression of knee osteoarthritis. Ann. Intern. Med. 139(5 Pt 1), 330–336. doi: 10.7326/0003-4819-139-5_part_1-200309020-00008
Fleischmann, R. M., Bliddal, H., Blanco, F. J., Schnitzer, T. J., Peterfy, C., Chen, S., et al. (2019). A phase II trial of lutikizumab, an anti–interleukin-1α/β dual variable domain immunoglobulin, in knee osteoarthritis patients with synovitis. Arthritis Rheumatol. 71, 1056–1069. doi: 10.1002/art.40840
Fransen, M., Agaliotis, M., Nairn, L., Votrubec, M., Bridgett, L., Su, S., et al. (2015). Glucosamine and chondroitin for knee osteoarthritis: a double-blind randomised placebo-controlled clinical trial evaluating single and combination regimens. Ann. Rheum. Dis. 74, 851–858. doi: 10.1136/annrheumdis-2013-203954
Funck-Brentano, T., and Cohen-Solal, M. (2011). Crosstalk between cartilage and bone: when bone cytokines matter. Cytokine Growth Fact. Rev. 22, 91–97. doi: 10.1016/j.cytogfr.2011.04.003
Garnero, P., Sornay-Rendu, E., and Chapurlat, R. (2020). The cartilage degradation marker, urinary CTX-II, is associated with the risk of incident total joint replacement in postmenopausal women. A 18 year evaluation of the OFELY prospective cohort. Osteoarthritis Cartilage 28, 468–474. doi: 10.1016/j.joca.2019.12.012
Geurts, J., Jurić, D., Müller, M., Schären, S., and Netzer, C. (2018). Novel ex vivo human osteochondral explant model of knee and spine osteoarthritis enables assessment of inflammatory and drug treatment responses. Int. J. Mol. Sci. 19:1314. doi: 10.3390/ijms19051314
Goldring, M. B., and Goldring, S. R. (2010). Articular cartilage and subchondral bone in the pathogenesis of osteoarthritis. Ann. N. Y. Acad. Sci. 1192, 230–237. doi: 10.1111/j.1749-6632.2009.05240.x
Grunke, M., and Schulze-Koops, H. (2006). Successful treatment of inflammatory knee osteoarthritis with tumour necrosis factor blockade. Ann. Rheum. Dis. 65, 555–556. doi: 10.1136/ard.2006.053272
Guermazi, A., Hayashi, D., Roemer, F., Felson, D. T., Wang, K., Lynch, J., et al. (2015). Severe radiographic knee osteoarthritis–does Kellgren and Lawrence grade 4 represent end stage disease?–the MOST study. Osteoarthritis Cartilage 23, 1499–1505. doi: 10.1016/j.joca.2015.04.018
Halilaj, E., Le, Y., Hicks, J. L., Hastie, T. J., and Delp, S. L. (2018). Modeling and predicting osteoarthritis progression: data from the osteoarthritis initiative. Osteoarthritis Cartilage 26, 1643–1650. doi: 10.1016/j.joca.2018.08.003
He, Y., Manon-Jensen, T., Arendt-Nielsen, L., Petersen, K. K., Christiansen, T., Samuels, J., et al. (2019). Potential diagnostic value of a type X collagen neo-epitope biomarker for knee osteoarthritis. Osteoarthritis Cartilage 27, 611–620. doi: 10.1016/j.joca.2019.01.001
He, Y., Siebuhr, A. S., Brandt-Hansen, N. U., Wang, J., Su, D., Zheng, Q., et al. (2014). Type X collagen levels are elevated in serum from human osteoarthritis patients and associated with biomarkers of cartilage degradation and inflammation. BMC Musculoskelet. Disord. 15:309. doi: 10.1186/1471-2474-15-309
Henrotin, Y., Chevalier, X., Deberg, M., Balblanc, J. C., Richette, P., Mulleman, D., et al. (2013). Early decrease of serum biomarkers of type II collagen degradation (Coll2-1) and joint inflammation (Coll2-1 NO2) by hyaluronic acid intra-articular injections in patients with knee osteoarthritis: a research study part of the Biovisco study. J. Orthop. Res. 31, 901–907. doi: 10.1002/jor.22297
Hick, A.-C., Fonck, M., Costes, B., Cobraiville, E., Pirson, S., Garcia, L., et al. (2019). Serum levels of Coll2-1, a specific biomarker of cartilage degradation, are not affected by sampling conditions, circadian rhythm, and seasonality. Cartilage 1947603519878489. doi: 10.1177/1947603519878489 [Epub ahead of print].
Hochberg, M. C., Martel-Pelletier, J., Monfort, J., Möller, I., Castillo, J. R., Arden, N., et al. (2016). Combined chondroitin sulfate and glucosamine for painful knee osteoarthritis: a multicentre, randomised, double-blind, non-inferiority trial versus celecoxib. Ann. Rheum. Dis. 75, 37–44. doi: 10.1136/annrheumdis-2014-206792
Hu, W., Chen, Y., Dou, C., and Dong, S. (2021). Microenvironment in subchondral bone: predominant regulator for the treatment of osteoarthritis. Ann. Rheum. Dis. 80, 413–422. doi: 10.1136/annrheumdis-2020-218089
Huang, Z., and Kraus, V. B. (2016). Does lipopolysaccharide-mediated inflammation have a role in OA? Nat. Rev. Rheumatol. 12, 123–129. doi: 10.1038/nrrheum.2015.158
Huang, Z. Y., Stabler, T., Pei, F. X., and Kraus, V. B. (2016). Both systemic and local lipopolysaccharide (LPS) burden are associated with knee OA severity and inflammation. Osteoarthritis Cartilage 24, 1769–1775. doi: 10.1016/j.joca.2016.05.008
Ingale, D., Kulkarni, P., Electricwala, A., Moghe, A., Kamyab, S., Jagtap, S., et al. (2021). Synovium-synovial fluid axis in osteoarthritis pathology: a key regulator of the cartilage degradation process. Genes (Basel) 12:989. doi: 10.3390/genes12070989
Jayabalan, P., and Schnitzer, T. J. (2017). Tanezumab in the treatment of chronic musculoskeletal conditions. Expert Opin. Biol. Ther. 17, 245–254. doi: 10.1080/14712598.2017.1271873
Jin, X., Beguerie, J. R., Zhang, W., Blizzard, L., Otahal, P., Jones, G., et al. (2015). Circulating C reactive protein in osteoarthritis: a systematic review and meta-analysis. Ann. Rheum. Dis. 74, 703–710. doi: 10.1136/annrheumdis-2013-204494
Jin, Y., Smith, C., Monteith, D., Brown, R., Camporeale, A., McNearney, T. A., et al. (2018). CGRP blockade by galcanezumab was not associated with reductions in signs and symptoms of knee osteoarthritis in a randomized clinical trial. Osteoarthritis Cartilage 26, 1609–1618. doi: 10.1016/j.joca.2018.08.019
Jotanovic, Z., Mihelic, R., Sestan, B., and Dembic, Z. (2012). Role of interleukin-1 inhibitors in osteoarthritis. Drugs Aging 29, 343–358. doi: 10.2165/11599350-000000000-00000
Kapoor, M., Martel-Pelletier, J., Lajeunesse, D., Pelletier, J.-P., and Fahmi, H. (2011). Role of proinflammatory cytokines in the pathophysiology of osteoarthritis. Nat. Rev. Rheumatol. 7, 33–42. doi: 10.1038/nrrheum.2010.196
Karsdal, M. A., Byrjalsen, I., Alexandersen, P., Bihlet, A., Andersen, J. R., Riis, B. J., et al. (2015). Treatment of symptomatic knee osteoarthritis with oral salmon calcitonin: results from two phase 3 trials. Osteoarthritis Cartilage 23, 532–543. doi: 10.1016/j.joca.2014.12.019
Karvonen-Gutierrez, C. A., Sowers, M. R., and Heeringa, S. G. (2012). Sex dimorphism in the association of cardiometabolic characteristics and osteophytes-defined radiographic knee osteoarthritis among obese and non-obese adults: NHANES III. Osteoarthritis Cartilage 20, 614–621. doi: 10.1016/j.joca.2012.02.644
Kim, H. J., Lee, Y. H., and Kim, C. K. (2007). Biomarkers of muscle and cartilage damage and inflammation during a 200 km run. Eur. J. Appl. Physiol. 99, 443–447. doi: 10.1007/s00421-006-0362-y
Kim, J., Lee, E. Y., Koh, E.-M., Cha, H.-S., Yoo, B., Lee, C. K., et al. (2009). Comparative clinical trial of S-adenosylmethionine versus nabumetone for the treatment of knee osteoarthritis: an 8-week, multicenter, randomized, double-blind, double-dummy, phase IV study in Korean patients. Clin. Ther. 31, 2860–2872. doi: 10.1016/j.clinthera.2009.12.016
Kluzek, S., Bay-Jensen, A.-C., Judge, A., Karsdal, M. A., Shorthose, M., Spector, T., et al. (2015). Serum cartilage oligomeric matrix protein and development of radiographic and painful knee osteoarthritis. A community-based cohort of middle-aged women. Biomarkers 20, 557–564. doi: 10.3109/1354750x.2015.1105498
Kolasinski, S. L., Neogi, T., Hochberg, M. C., Oatis, C., Guyatt, G., Block, J., et al. (2020). 2019 American College of Rheumatology/Arthritis Foundation Guideline for the Management of osteoarthritis of the hand, hip, and knee. Arthritis Care Res. 72, 149–162. doi: 10.1002/acr.24131
Kong, R., Gao, J., Si, Y., and Zhao, D. (2017). Combination of circulating miR-19b-3p, miR-122-5p and miR-486-5p expressions correlates with risk and disease severity of knee osteoarthritis. Am. J. Transl. Res. 9, 2852–2864.
Kraus, V. B., Blanco, F. J., Englund, M., Karsdal, M. A., and Lohmander, L. S. (2015). Call for standardized definitions of osteoarthritis and risk stratification for clinical trials and clinical use. Osteoarthritis Cartilage 23, 1233–1241. doi: 10.1016/j.joca.2015.03.036
Kraus, V. B., Collins, J. E., Hargrove, D., Losina, E., Nevitt, M., Katz, J. N., et al. (2017a). Predictive validity of biochemical biomarkers in knee osteoarthritis: data from the FNIH OA Biomarkers Consortium. Ann. Rheum. Dis. 76, 186–195. doi: 10.1136/annrheumdis-2016-209252
Kraus, V. B., Hargrove, D. E., Hunter, D. J., Renner, J. B., and Jordan, J. M. (2017b). Establishment of reference intervals for osteoarthritis-related soluble biomarkers: the FNIH/OARSI OA Biomarkers Consortium. Ann. Rheum. Dis. 76, 179–185. doi: 10.1136/annrheumdis-2016-209253
Kroon, F. P. B., Veenbrink, A. I., de Mutsert, R., Visser, A. W., van Dijk, K. W., le Cessie, S., et al. (2019). The role of leptin and adiponectin as mediators in the relationship between adiposity and hand and knee osteoarthritis. Osteoarthritis Cartilage 27, 1761–1767. doi: 10.1016/j.joca.2019.08.003
Kumm, J., Tamm, A., Lintrop, M., and Tamm, A. (2013). Diagnostic and prognostic value of bone biomarkers in progressive knee osteoarthritis: a 6-year follow-up study in middle-aged subjects. Osteoarthritis Cartilage 21, 815–822. doi: 10.1016/j.joca.2013.03.008
Lambert, C., Borderie, D., Dubuc, J. E., Rannou, F., and Henrotin, Y. (2019). Type II collagen peptide Coll2-1 is an actor of synovitis. Osteoarthritis Cartilage 27, 1680–1691. doi: 10.1016/j.joca.2019.07.009
Larsson, S., Englund, M., Struglics, A., and Lohmander, L. S. (2015). Interleukin-6 and tumor necrosis factor alpha in synovial fluid are associated with progression of radiographic knee osteoarthritis in subjects with previous meniscectomy. Osteoarthritis Cartilage 23, 1906–1914. doi: 10.1016/j.joca.2015.05.035
Lattermann, C., Jacobs, C. A., Proffitt Bunnell, M., Huston, L. J., Gammon, L. G., Johnson, D. L., et al. (2016). A multicenter study of early anti-inflammatory treatment in patients with acute anterior cruciate ligament tear. Am. J. Sports Med. 45, 325–333. doi: 10.1177/0363546516666818
Lee, Y. C., Lu, B., Bathon, J. M., Haythornthwaite, J. A., Smith, M. T., Page, G. G., et al. (2011). Pain sensitivity and pain reactivity in osteoarthritis. Arthritis Care Res. 63, 320–327.
Leslie, D., Lipsky, P., and Notkins, A. L. (2001). Autoantibodies as predictors of disease. J. Clin. Invest. 108, 1417–1422. doi: 10.1172/jci14452
Li, X., and Majumdar, S. (2013). Quantitative MRI of articular cartilage and its clinical applications. J. Magn. Reson. Imaging 38, 991–1008. doi: 10.1002/jmri.24313
Lindström, E., Rizoska, B., Henderson, I., Terelius, Y., Jerling, M., Edenius, C., et al. (2018). Nonclinical and clinical pharmacological characterization of the potent and selective cathepsin K inhibitor MIV-711. J. Transl. Med. 16:125.
Link, T. M., Steinbach, L. S., Ghosh, S., Ries, M., Lu, Y., Lane, N., et al. (2003). Osteoarthritis: MR imaging findings in different stages of disease and correlation with clinical findings. Radiology 226, 373–381. doi: 10.1148/radiol.2262012190
Liu, Y., Peng, H., Meng, Z., and Wei, M. (2015). Correlation of IL-17 level in synovia and severity of knee osteoarthritis. Med. Sci. Monit. 21, 1732–1736. doi: 10.12659/msm.893771
Livshits, G., Zhai, G., Hart, D. J., Kato, B. S., Wang, H., Williams, F. M. K., et al. (2009). Interleukin-6 is a significant predictor of radiographic knee osteoarthritis: the Chingford Study. Arthritis Rheum. 60, 2037–2045. doi: 10.1002/art.24598
Loeser, R. F., Goldring, S. R., Scanzello, C. R., and Goldring, M. B. (2012). Osteoarthritis: a disease of the joint as an organ. Arthritis Rheum. 64, 1697–1707. doi: 10.1002/art.34453
Lugo, J. P., Saiyed, Z. M., and Lane, N. E. (2016). Efficacy and tolerability of an undenatured type II collagen supplement in modulating knee osteoarthritis symptoms: a multicenter randomized, double-blind, placebo-controlled study. Nutr. J. 15:14.
Luo, W., Liu, L., Yang, L., Dong, Y., Liu, T., Wei, X., et al. (2018). The vitamin D receptor regulates miR-140-5p and targets the MAPK pathway in bone development. Metabolism 85, 139–150. doi: 10.1016/j.metabol.2018.03.018
Luyten, F. P., Denti, M., Filardo, G., Kon, E., and Engebretsen, L. (2012). Definition and classification of early osteoarthritis of the knee. Knee Surg. Sports Traumatol. Arthrosc. 20, 401–406. doi: 10.1007/s00167-011-1743-2
Lv, Y., Wang, G., Xu, W., Tao, P., Lv, X., and Wang, Y. (2015). Tartrate-resistant acid phosphatase 5b is a marker of osteoclast number and volume in RAW 264.7 cells treated with receptor-activated nuclear κB ligand. Exp. Ther. Med. 9, 143–146. doi: 10.3892/etm.2014.2071
Madry, H., Kon, E., Condello, V., Peretti, G. M., Steinwachs, M., Seil, R., et al. (2016). Early osteoarthritis of the knee. Knee Surg. Sports Traumatol. Arthrosc. 24, 1753–1762.
Malfait, A.-M., Miller, R. E., and Block, J. A. (2020). Targeting neurotrophic factors: novel approaches to musculoskeletal pain. Pharmacol. Ther. 211:107553. doi: 10.1016/j.pharmthera.2020.107553
Martel-Pelletier, J., Barr, A. J., Cicuttini, F. M., Conaghan, P. G., Cooper, C., Goldring, M. B., et al. (2016). Osteoarthritis. Nat. Rev. Dis. Primers 2:16072.
McAlindon, T. E., LaValley, M. P., Harvey, W. F., Price, L. L., Driban, J. B., Zhang, M., et al. (2017). Effect of intra-articular triamcinolone vs saline on knee cartilage volume and pain in patients with knee osteoarthritis: a randomized clinical trial. JAMA 317, 1967–1975. doi: 10.1001/jama.2017.5283
McInnes, I. B., Behrens, F., Mease, P. J., Kavanaugh, A., Ritchlin, C., Nash, P., et al. (2020). Secukinumab versus adalimumab for treatment of active psoriatic arthritis (EXCEED): a double-blind, parallel-group, randomised, active-controlled, phase 3b trial. Lancet 395, 1496–1505.
Meini, S., and Maggi, C. A. (2008). Knee osteoarthritis: a role for bradykinin? Inflamm. Res. 57, 351–361.
Menashe, L., Hirko, K., Losina, E., Kloppenburg, M., Zhang, W., Li, L., et al. (2012). The diagnostic performance of MRI in osteoarthritis: a systematic review and meta-analysis. Osteoarthritis Cartilage 20, 13–21.
Miller, R. E., Block, J. A., and Malfait, A.-M. (2017). Nerve growth factor blockade for the management of osteoarthritis pain: what can we learn from clinical trials and preclinical models? Curr. Opin. Rheumatol. 29, 110–118.
Mobasheri, A., Bay-Jensen, A. C., van Spil, W. E., Larkin, J., and Levesque, M. C. (2017). Osteoarthritis year in review 2016: biomarkers (biochemical markers). Osteoarthritis Cartilage 25, 199–208.
Moisio, K., Eckstein, F., Chmiel, J. S., Guermazi, A., Prasad, P., Almagor, O., et al. (2009). Denuded subchondral bone and knee pain in persons with knee osteoarthritis. Arthritis Rheum. 60, 3703–3710.
Montagnoli, C., Tiribuzi, R., Crispoltoni, L., Pistilli, A., Stabile, A. M., Manfreda, F., et al. (2017). β-NGF and β-NGF receptor upregulation in blood and synovial fluid in osteoarthritis. Biol. Chem. 398, 1045–1054.
Nagaoka, I., Tsuruta, A., and Yoshimura, M. (2019). Chondroprotective action of glucosamine, a chitosan monomer, on the joint health of athletes. Int. J. Biol. Macromol. 132, 795–800.
Neogi, T. (2013). The epidemiology and impact of pain in osteoarthritis. Osteoarthritis Cartilage 21, 1145–1153.
Nguyen, C., Lefèvre-Colau, M.-M., Poiraudeau, S., and Rannou, F. (2016). Evidence and recommendations for use of intra-articular injections for knee osteoarthritis. Ann. Phys. Rehabil. Med. 59, 184–189.
Nwosu, L. N., Allen, M., Wyatt, L., Huebner, J. L., Chapman, V., Walsh, D. A., et al. (2017). Pain prediction by serum biomarkers of bone turnover in people with knee osteoarthritis: an observational study of TRAcP5b and cathepsin K in OA. Osteoarthritis Cartilage 25, 858–865.
O’Neil, C. K., Hanlon, J. T., and Marcum, Z. A. (2012). Adverse effects of analgesics commonly used by older adults with osteoarthritis: focus on non-opioid and opioid analgesics. Am. J. Geriatr. Pharmacother. 10, 331–342.
Park, H.-M., Lee, J.-H., and Lee, Y.-J. (2020). Positive association of serum alkaline phosphatase level with severe knee osteoarthritis: a nationwide population-based study. Diagnostics (Basel) 10:1016. doi: 10.3390/diagnostics10121016
Posey, K. L., Coustry, F., and Hecht, J. T. (2018). Cartilage oligomeric matrix protein: COMPopathies and beyond. Matrix Biol. 7, 161–173. doi: 10.1016/j.matbio.2018.02.023
Rim, Y. A., Nam, Y., and Ju, J. H. (2020). The role of chondrocyte hypertrophy and senescence in osteoarthritis initiation and progression. Int. J. Mol. Sci. 21:2358. doi: 10.3390/ijms21072358
Robinson, W. H., Lepus, C. M., Wang, Q., Raghu, H., Mao, R., Lindstrom, T. M., et al. (2016). Low-grade inflammation as a key mediator of the pathogenesis of osteoarthritis. Nat. Rev. Rheumatol. 12, 580–592. doi: 10.1038/nrrheum.2016.136
Roemer, F. W., Demehri, S., Omoumi, P., Link, T. M., Kijowski, R., Saarakkala, S., et al. (2020). State of the art: imaging of osteoarthritis-revisited 2020. Radiology 296, 5–21. doi: 10.1148/radiol.2020192498
Roemer, F. W., Kwoh, C. K., Hayashi, D., Felson, D. T., and Guermazi, A. (2018). The role of radiography and MRI for eligibility assessment in DMOAD trials of knee OA. Nat. Rev. Rheumatol. 14, 372–380. doi: 10.1038/s41584-018-0010-z
Rondanelli, M., Braschi, V., Gasparri, C., Nichetti, M., Faliva, M. A., Peroni, G., et al. (2019). Effectiveness of non-animal chondroitin sulfate supplementation in the treatment of moderate knee osteoarthritis in a group of overweight subjects: a randomized, double-blind, placebo-controlled pilot study. Nutrients 11:2027. doi: 10.3390/nu11092027
Rousseau, J.-C., Millet, M., Croset, M., Sornay-Rendu, E., Borel, O., and Chapurlat, R. (2020). Association of circulating microRNAs with prevalent and incident knee osteoarthritis in women: the OFELY study. Arthritis Res. Ther. 22:2. doi: 10.1186/s13075-019-2086-5
Ryd, L., Brittberg, M., Eriksson, K., Jurvelin, J. S., Lindahl, A., Marlovits, S., et al. (2015). Pre-osteoarthritis: definition and diagnosis of an elusive clinical entity. Cartilage 6, 156–165. doi: 10.1177/1947603515586048
Sagar, D. R., Ashraf, S., Xu, L., Burston, J. J., Menhinick, M. R., Poulter, C. L., et al. (2014). Osteoprotegerin reduces the development of pain behaviour and joint pathology in a model of osteoarthritis. Ann. Rheum. Dis. 73, 1558–1565. doi: 10.1136/annrheumdis-2013-203260
Sasaki, E., Ota, S., Chiba, D., Kimura, Y., Sasaki, S., Yamamoto, Y., et al. (2020). Early knee osteoarthritis prevalence is highest among middle-aged adult females with obesity based on new set of diagnostic criteria from a large sample cohort study in the Japanese general population. Knee Surg. Sports Traumatol. Arthrosc. 28, 984–994. doi: 10.1007/s00167-019-05614-z
Scanzello, C. R., Umoh, E., Pessler, F., Diaz-Torne, C., Miles, T., DiCarlo, E., et al. (2009). Local cytokine profiles in knee osteoarthritis: elevated synovial fluid interleukin-15 differentiates early from end-stage disease. Osteoarthritis Cartilage 17, 1040–1048. doi: 10.1016/j.joca.2009.02.011
Schmelz, M., Mantyh, P., Malfait, A.-M., Farrar, J., Yaksh, T., Tive, L., et al. (2019). Nerve growth factor antibody for the treatment of osteoarthritis pain and chronic low-back pain: mechanism of action in the context of efficacy and safety. Pain 160, 2210–2220. doi: 10.1097/j.pain.0000000000001625
Sellam, J., and Berenbaum, F. (2010). The role of synovitis in pathophysiology and clinical symptoms of osteoarthritis. Nat. Rev. Rheumatol. 6, 625–635. doi: 10.1038/nrrheum.2010.159
Sharma, L. (2021). Osteoarthritis of the knee. N. Engl. J. Med. 384, 51–59. doi: 10.1056/NEJMcp1903768
Si, H., Zeng, Y., Zhou, Z., Pei, F., Lu, Y., Cheng, J., et al. (2016). Expression of miRNA-140 in chondrocytes and synovial fluid of knee joints in patients with osteoarthritis. Chin. Med. Sci. J. 31, 207–212. doi: 10.1016/S1001-9294(17)30002-0
Si, H.-B., Yang, T.-M., Li, L., Tian, M., Zhou, L., Li, D.-P., et al. (2019). miR-140 attenuates the progression of early-stage osteoarthritis by retarding chondrocyte senescence. Mol. Ther. Nucleic Acids 19, 15–30. doi: 10.1016/j.omtn.2019.10.032
Siebuhr, A. S., Petersen, K. K., Arendt-Nielsen, L., Egsgaard, L. L., Eskehave, T., Christiansen, C., et al. (2014). Identification and characterisation of osteoarthritis patients with inflammation derived tissue turnover. Osteoarthritis Cartilage 22, 44–50. doi: 10.1016/j.joca.2013.10.020
Sofat, N., Ejindu, V., Heron, C., Harrison, A., Koushesh, S., Assi, L., et al. (2019). Biomarkers in painful symptomatic knee OA demonstrate that MRI assessed joint damage and type II collagen degradation products are linked to disease progression. Front. Neurosci. 13:1016. doi: 10.3389/fnins.2019.01016
Sowers, M. R., McConnell, D., Jannausch, M., Buyuktur, A. G., Hochberg, M., and Jamadar, D. A. (2006). Estradiol and its metabolites and their association with knee osteoarthritis. Arthritis Rheum. 54, 2481–2487. doi: 10.1002/art.22005
Stein, G. S., and Lian, J. B. (1993). Molecular mechanisms mediating proliferation/differentiation interrelationships during progressive development of the osteoblast phenotype. Endocr. Rev. 14, 424–442. doi: 10.1210/edrv-14-4-424
Struglics, A., Larsson, S., Pramhed, A., Frobell, R., and Swärd, P. (2018). Changes in synovial fluid and serum concentrations of cartilage oligomeric matrix protein over 5 years after anterior cruciate ligament rupture: an exploratory analysis in the KANON trial. Osteoarthritis Cartilage 26, 1351–1358. doi: 10.1016/j.joca.2018.06.005
Sun, J.-M., Sun, L.-Z., Liu, J., Su, B. H., and Shi, L. (2013). Serum interleukin-15 levels are associated with severity of pain in patients with knee osteoarthritis. Dis. Markers 35, 203–206. doi: 10.1155/2013/176278
Sung, W.-H., Tsao, Y.-T., Shen, C.-J., Tsai, C.-Y., and Cheng, C.-M. (2021). Small-volume detection: platform developments for clinically-relevant applications. J. Nanobiotechnol. 19:114.
Swingler, T. E., Niu, L., Smith, P., Paddy, P., Le, L., Barter, M. J., et al. (2019). The function of microRNAs in cartilage and osteoarthritis. Clin. Exp. Rheumatol. 37(Suppl. 120), 40–47.
Taşoğlu, Ö, Bölük, H., and Şahin Onat, Ş, Taşoğlu, Ý, and Özgirgin, N. (2016). Is blood neutrophil-lymphocyte ratio an independent predictor of knee osteoarthritis severity? Clin. Rheumatol. 35, 1579–1583.
van Spil, W. E., Drossaers-Bakker, K. W., and Lafeber, F. P. J. G. (2013). Associations of CTX-II with biochemical markers of bone turnover raise questions on its tissue origin: data from CHECK, a cohort study of early osteoarthritis. Ann. Rheum. Dis. 72, 29–36. doi: 10.1136/annrheumdis-2011-201177
van Spil, W. E., and Szilagyi, I. A. (2020). Osteoarthritis year in review 2019: biomarkers (biochemical markers). Osteoarthritis Cartilage 28, 296–315. doi: 10.1016/j.joca.2019.11.007
Van Spil, W. E., Welsing, P. M. J., Kloppenburg, M., Bierma-Zeinstra, S. M., Bijlsma, J. W. J., Mastbergen, S. C., et al. (2012). Cross-sectional and predictive associations between plasma adipokines and radiographic signs of early-stage knee osteoarthritis: data from CHECK. Osteoarthritis Cartilage 20, 1278–1285. doi: 10.1016/j.joca.2012.08.001
Vina, E. R., and Kwoh, C. K. (2018). Epidemiology of osteoarthritis: literature update. Curr. Opin. Rheumatol. 30, 160–167. doi: 10.1097/BOR.0000000000000479
Waldmann, T. A., Dubois, S., and Tagaya, Y. (2001). Contrasting roles of IL-2 and IL-15 in the life and death of lymphocytes: implications for immunotherapy. Immunity 14, 105–110. doi: 10.1016/S1074-7613(09)00091-0
Wang, H., Kohno, T., Amaya, F., Brenner, G. J., Ito, N., Allchorne, A., et al. (2005). Bradykinin produces pain hypersensitivity by potentiating spinal cord glutamatergic synaptic transmission. J. Neurosci. 25, 7986–7992. doi: 10.1523/JNEUROSCI.2393-05.2005
Wang, L.-J., Zeng, N., Yan, Z.-P., Li, J.-T., and Ni, G.-X. (2020). Post-traumatic osteoarthritis following ACL injury. Arthritis Res. Ther. 22:57. doi: 10.1186/s13075-020-02156-5
Wang, S. X., Abramson, S. B., Attur, M., Karsdal, M. A., Preston, R. A., Lozada, C. J., et al. (2017). Safety, tolerability, and pharmacodynamics of an anti-interleukin-1α/β dual variable domain immunoglobulin in patients with osteoarthritis of the knee: a randomized phase 1 study. Osteoarthritis Cartilage 25, 1952–1961. doi: 10.1016/j.joca.2017.09.007
Wluka, A. E., Lombard, C. B., and Cicuttini, F. M. (2013). Tackling obesity in knee osteoarthritis. Nat. Rev. Rheumatol. 9, 225–235. doi: 10.1038/nrrheum.2012.224
Xiao, Y.-P., Tian, F.-M., Dai, M.-W., Wang, W.-Y., Shao, L.-T., and Zhang, L. (2016). Are estrogen-related drugs new alternatives for the management of osteoarthritis? Arthritis Res. Ther. 18:151.
Xie, C., and Chen, Q. (2019). Adipokines: new therapeutic target for osteoarthritis? Curr. Rheumatol. Rep. 21:71. doi: 10.1007/s11926-019-0868-z
Xie, W., Su, W., Xia, H., Wang, Z., Su, C., and Su, B. (2019). Synovial fluid microRNA-210 as a potential biomarker for early prediction of osteoarthritis. Biomed Res. Int. 2019:7165406. doi: 10.1155/2019/7165406
Xin, L., Wu, Z., Qu, Q., Wang, R., Tang, J., and Chen, L. (2017). Comparative study of CTX-II, Zn2+, and Ca2+ from the urine for knee osteoarthritis patients and healthy individuals. Medicine 96:e7593. doi: 10.1097/MD.0000000000007593
Yoneda, T., Hiasa, M., Nagata, Y., Okui, T., and White, F. (2015). Contribution of acidic extracellular microenvironment of cancer-colonized bone to bone pain. Biochim. Biophys. Acta 1848, 2677–2684. doi: 10.1016/j.bbamem.2015.02.004
Yuan, C., Pan, Z., Zhao, K., Li, J., Sheng, Z., Yao, X., et al. (2020). Classification of four distinct osteoarthritis subtypes with a knee joint tissue transcriptome atlas. Bone Res. 8:38. doi: 10.1038/s41413-020-00109-x
Yusuf, E., Kortekaas, M. C., Watt, I., Huizinga, T. W. J., and Kloppenburg, M. (2011). Do knee abnormalities visualised on MRI explain knee pain in knee osteoarthritis? A systematic review. Ann. Rheum. Dis. 70, 60–67. doi: 10.1136/ard.2010.131904
Zeng, N., Yan, Z.-P., Chen, X.-Y., and Ni, G.-X. (2020). Infrapatellar fat pad and knee osteoarthritis. Aging Dis. 11, 1317–1328. doi: 10.14336/AD.2019.1116
Zhang, H., Cai, D., and Bai, X. (2020). Macrophages regulate the progression of osteoarthritis. Osteoarthritis Cartilage 28, 555–561. doi: 10.1016/j.joca.2020.01.007
Zhang, Y., Nevitt, M., Niu, J., Lewis, C., Torner, J., Guermazi, A., et al. (2011). Fluctuation of knee pain and changes in bone marrow lesions, effusions, and synovitis on magnetic resonance imaging. Arthritis Rheum. 63, 691–699. doi: 10.1002/art.30148
Keywords: knee osteoarthritis, molecular classification, pathophysiology, body fluids, subtypes
Citation: Lv Z, Yang YX, Li J, Fei Y, Guo H, Sun Z, Lu J, Xu X, Jiang Q, Ikegawa S and Shi D (2021) Molecular Classification of Knee Osteoarthritis. Front. Cell Dev. Biol. 9:725568. doi: 10.3389/fcell.2021.725568
Received: 15 June 2021; Accepted: 09 August 2021;
Published: 27 August 2021.
Edited by:
Yi Zhang, Central South University, ChinaReviewed by:
Ren Xu, Xiamen University, ChinaHongwei Ouyang, Zhejiang University, China
Elisa Belluzzi, University of Padua, Italy
Cale Jacobs, University of Kentucky, United States
Copyright © 2021 Lv, Yang, Li, Fei, Guo, Sun, Lu, Xu, Jiang, Ikegawa and Shi. This is an open-access article distributed under the terms of the Creative Commons Attribution License (CC BY). The use, distribution or reproduction in other forums is permitted, provided the original author(s) and the copyright owner(s) are credited and that the original publication in this journal is cited, in accordance with accepted academic practice. No use, distribution or reproduction is permitted which does not comply with these terms.
*Correspondence: Dongquan Shi, shidongquan@nju.edu.cn