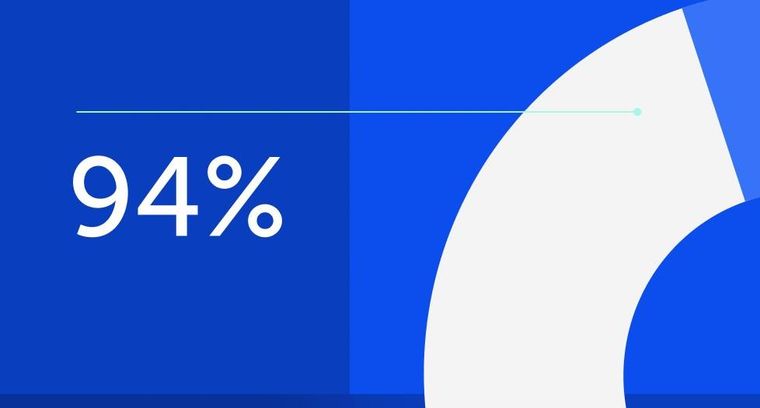
94% of researchers rate our articles as excellent or good
Learn more about the work of our research integrity team to safeguard the quality of each article we publish.
Find out more
REVIEW article
Front. Cell Dev. Biol., 27 July 2021
Sec. Cell Adhesion and Migration
Volume 9 - 2021 | https://doi.org/10.3389/fcell.2021.716842
Chemokine receptors are members of the G protein-coupled receptor superfamily, which together with chemokine ligands form chemokine networks to regulate various cellular functions, immune and physiological processes. These receptors are closely related to cell movement and thus play a vital role in several physiological and pathological processes that require regulation of cell migration. CXCR4, one of the most intensively studied chemokine receptors, is involved in many functions in addition to immune cells recruitment and plays a pivotal role in the pathogenesis of liver disease. Aberrant CXCR4 expression pattern is related to the migration and movement of liver specific cells in liver disease through its cross-talk with a variety of significant cell signaling pathways. An in-depth understanding of CXCR4-mediated signaling pathway and its role in liver disease is critical to identifying potential therapeutic strategies. Current therapeutic strategies for liver disease mainly focus on regulating the key functions of specific cells in the liver, in which the CXCR4 pathway plays a crucial role. Multiple challenges remain to be overcome in order to more effectively target CXCR4 pathway and identify novel combination therapies with existing strategies. This review emphasizes the role of CXCR4 and its important cell signaling pathways in the pathogenesis of liver disease and summarizes the targeted therapeutic studies conducted to date.
Liver disease is a leading cause of illness and death in the world (Wang et al., 2014; Marcellin and Kutala, 2018). In recent years, the incidence of liver disease such as alcoholic liver disease (ALD), non-alcoholic fatty liver disease (NAFLD), viral hepatitis, liver fibrosis and cirrhosis, hepatocellular carcinoma (HCC) and liver failure (LF) has gradually increased (Wang et al., 2014, 2021). Because the molecular mechanism of liver disease is very complicated, there is still no clinically effective treatment for specific pathogenesis. The current academic opinion holds that specific cells in the liver play a significant role in the pathophysiology of liver disease (Poisson et al., 2017; Cai B. et al., 2020). However, how these cells play a role in liver disease, and the specific molecular mechanisms that regulate cellular functions are still not fully elucidated. Therefore, in-depth study of liver disease progression mechanisms and specific cellular functions, as well as the determination of crucial node molecules are important scientific problems to be solved in the field of liver disease research.
Chemokines, also known as chemotactic cytokines, are a large family of small and secreted proteins with molecular weights in the range of 8–12 kDa that are involved in a variety of cellular functions, including inducing target cell chemotaxis (migration), guiding cell movement and mediating immune cell trafficking (Roussos et al., 2011; Kolaczkowska and Kubes, 2013; Griffith et al., 2014; Chen w. et al., 2018; Laufer et al., 2019). Chemokines have become a large family of more than 50 members. Chemokine receptors are 7-transmembrane (7TM) G protein-coupled receptors (GPCRs), which are subdivided into four types (CXCR, CX3CR, XCR, and CCR) according to the class of chemokines they bind (Bussmann and Raz, 2015). Among them, CXC chemokine receptors, including CXCR1 to CXCR7, are one of the largest chemokine families and play important roles in several physiological and pathological processes (Ullah, 2019). They are mainly expressed on immune and inflammatory cells and are also present in non-immune cells such as resident cells within the liver (Zlotnik et al., 2011; Choi et al., 2016). CXC chemokine receptors can be grossly defined as inflammatory, homeostatic or dual-function receptors based on the ligands they bind (Zlotnik and Yoshie, 2012). The major ligands of CXC chemokine receptors are shown in Figure 1.
Figure 1. Classification of CXC chemokine receptors. CXC chemokine receptors are classified according to the ligands they bind, followed by an R (representing receptor) and a number corresponding to the order of discovery. These CXC chemokine ligands are also known as GRO-α (CXCL1), GRO-β (CXCL2), GRO-γ (CXCL3), ENA78 (CXCL5), GCP-2 (CXCL6), NAP-2 (CXCL7), IL-8 (CXCL8), MIG (CXCL9), IP-10 (CXCL10), I-TAC(CXCL11), SDF-1(CXCL12), BCA-1(CXCL13), and SR-PSOX(CXCL16).
C-X-C motif chemokine receptor 4 (CXCR4) is a chemokine receptor that has been intensively studied because of its significant role in cellular functions, immune processes, growth and development, and liver disease (Qin L. et al., 2018; Zheng et al., 2018; Ullah, 2019; Yang et al., 2019). Its role in liver disease may involve the regulation of the migration and movement of hepatocytes, hepatic stellate cells (HSCs), Kupffer cells (KCs), fibroblasts, endothelial cells and circulating immune cells (Chen w. et al., 2018). In the liver environment, CXCR4 is ubiquitously expressed in most liver cells such as HSCs, KCs and liver sinusoidal endothelial cells (LSECs), but also in malignant cells (Hong et al., 2009; Ding et al., 2013; Wang et al., 2018, 2020). CXCR4 can bind to C-X-C motif chemokine 12 (CXCL12), and CXCL12, also known as stromal cell-derived factor-1 (SDF-1), is the only specific endogenous ligand for CXCR4 (Ullah, 2019). CXCL12 (SDF-1) plays an important role in several physiological and pathological processes by binding to CXCR4 and then participating in cell localization, chemotaxis, activation, migration, proliferation and differentiation (Zhuo et al., 2012; Janssens et al., 2018; Daniel et al., 2020). There is mounting evidence that the CXCR4 and its ligand provide potential targets for the treatment of liver disease, tumors, and cardiovascular disease (Zhuo et al., 2012; Döring et al., 2017; Wang et al., 2018). To date, a number of therapeutic studies have been conducted in combination targeting CXCR4 and its ligand (Sung et al., 2018; Zheng et al., 2019). This review aims to provide a comprehensive overview of the role of CXCR4 and its ligand in liver disease, including its potential as a therapeutic target, and summarize the therapeutic studies of combined targeting CXCR4 pathway.
Chemokines (chemotactic cytokines) are a family of small and highly conserved proteins that bind to and signal through cell surface 7TM G protein-coupled receptors, which in turn regulate cell migration and function (Hughes and Nibbs, 2018). The four types of chemokine receptors, based on their expression status and functions executed in healthy and disease states, are further divided into constitutively expressed homeostatic chemokine receptors, inducibly expressed inflammatory chemokine receptors, and dual-type chemokine receptors with both characteristics (Mamazhakypov et al., 2021). In addition to the above four types (CXCR, CX3CR, XCR, and CCR), chemokine receptors also include a group of atypical chemokine receptors (ACKRs), which bind to different families of chemokines, but mainly act as decoy and scavenger receptors (Meyrath et al., 2020). Among these chemokine receptors, CXCR1-7, as important members, have been extensively studied in various organs and systems, both in physiological and pathological conditions. For instance, CXCR1 and CXCR2 are involved in the pathogenesis of inflammation and fibrosis (Kormann et al., 2012; Mattos et al., 2020); CXCR3 plays important roles in angiogenesis and tumors (Quemener et al., 2016); CXCR4 regulates the development of hematopoietic and nervous systems, and modulates different cellular functions, including cell migration, chemotaxis, differentiation, growth, activation, proliferation, survival and apoptosis (Murphy and Heusinkveld, 2018); CXCR5 is closely related to immunomodulation (Zhang et al., 2017); CXCR6 and CXCR7 are mainly involved in the regulation of inflammation and cellular functions (Humpert et al., 2014; Butcher et al., 2016; Chang et al., 2018). Interestingly, CXCL12 can also bind to CXCR7 (also known as atypical chemokine receptor 3, or ACKR3), even with a greater affinity than to CXCR4 (Guo et al., 2015; Liepelt and Tacke, 2016). Notably, among CXCR1-7, CXCR4 is the most intensively studied, and it plays an important role in many pathophysiological processes through different signaling pathways.
Chemokine receptors typically interact with a variety of chemokines to signal, but CXCR4 is an exception, and is specific for the chemokine CXCL12. Together, they constitute CXCR4 pathway that normally play a significant role in the development of multiple systems, but they are also important in disease. CXCR4 signaling is mainly mediated by proteins that interact with receptors, including heterotrimeric G proteins, G protein receptor kinases (GRKs) and β-arrestin adapter proteins (Wang and Knaut, 2014). CXCL12 binding to CXCR7 usually leads to β-arrestin mediated signaling (Daniel et al., 2020). Heterotrimeric G proteins are composed of Gα, Gβ, and Gγ subunits. In the inactive or basal state, the Gα subunit contains guanine nucleotide diphosphate (GDP) (Cojoc et al., 2013). When chemokines stimulate the activation of the receptor CXCR4 and promote interaction between the receptor and the trimeric G-protein α, βγ. This leads to the exchange of GDP for GTP bound to Gα subunits and the dissociation of the Gβγ heterodimers (Mamazhakypov et al., 2021). The dissociated subunits promote downstream signaling through different pathways (Figure 2).
Figure 2. The main signaling pathways and cellular functions of CXCR4. Upon CXCR4 activation, Gα and Gβγ subunits activate different downstream signaling pathways including RhoA/ROCK, cAMP/PKA, PI3K/Akt/mTOR, JAK/STAT, Ras/RAF/ERK1/2, Src, and PLCβ. GRK phosphorylates CXCR4, enabling β-arrestin to bind and internalize CXCR4, which can lead to receptor recycling if receptor phosphorylation is reversed by PP2A or degraded in lysosomes. β-arrestin bound to CXCR4 can also activate MAPK pathways, such as JNK and ERK1/2. These downstream signaling pathways of CXCR4 regulate a variety of cellular functions, such as cell migration, chemotaxis, growth, proliferation, survival and apoptosis. AC, adenylate cyclase; Akt, protein kinase B; ATP, adenosine-5′-triphosphate; cAMP, cyclic adenosine monophosphate; DAG, diacylglycerol; ER, endoplasmic reticulum; IP3, inositol-1,4,5-trisphosphate; ERK1/2, extracellular signal-regulated kinases 1/2; GRK, G protein receptor kinases; JAK, Janus kinase; JNK, c-Jun N-terminal kinase; MAPK, mitogen-activated protein kinase; mTOR, mammalian target of rapamycin; PI3K, phosphoinositide-3-kinase; PIP2, phosphatidylinositol-4,5,-bisphosphate; PIP3, phosphatidylinositol (3,4,5)-trisphosphate; PLCβ, phospholipase Cβ; PKA, protein kinases A; PKC, protein kinases C; PP2A, protein phosphatase 2; RhoA, Ras homolog gene family member A; ROCK, Rho-related PK; STAT, signal transducer and activator of transcription.
The different pathways of GPCRs signaling depend on the coupled Gα subunits, which are divided into four families: Gαs, Gαi, Gαq, and Gα12. Indeed, CXCR4 can signal by coupling to different families of Gα subunits (Wu et al., 2012; Bar-Shavit et al., 2016). CXCR4 coupled to Gαs stimulates adenylate cyclase (AC), whereas CXCR4 bound to Gαi inhibits AC (Rosciglione et al., 2014; Piovan et al., 2018). AC serves as an effector enzyme that catalyzes the conversion of adenosine-5′-triphosphate (ATP) into cyclic adenosine monophosphate (cAMP), thereby activating cAMP-dependent protein kinase (PKA), which further regulates mitogen-activated protein kinase (MAPK) signaling pathway (Wang et al., 2015; Teixidó et al., 2018). In addition, Gαs and Gαi can also change the activity of Src tyrosine kinase, thereby affecting its role in signal integration (Chiou and Zennadi, 2015). Gαq coupled to CXCR4 converts phosphatidylinositol-4,5,-bisphosphate (PIP2) into inositol-1,4,5-trisphosphate (IP3) and diacylglycerol (DAG) by activating phospholipase Cβ (PLCβ). DAG activates a family of protein kinases (PKs), including PKC, which phosphorylates a number of downstream effectors, such as the Ras signaling pathway (Irnaten et al., 2020); while IP3 diffuses to the endoplasmic reticulum (ER) membrane and binds to gated calcium ion (Ca2+) channels, triggering the release of calcium from intracellular storage into the cytoplasm (Dessein et al., 2010). CXCR4 signaling often requires this intracellular calcium mobilization to drive (Engevik et al., 2019). Moreover, CXCR4 coupled to Gα12 further activates Rho-related PK (ROCK) by activating the Ras homolog gene family member A (RhoA), which in turn participates in different cellular functions (Zainal et al., 2018; Figure 2). Activation of phosphoinositide-3-kinase (PI3K) by CXCR4 is predominantly mediated by Gβγ subunits (Teicher and Fricker, 2010). PI3K converts PIP2 to phosphatidylinositol (3,4,5)-trisphosphate (PIP3), triggering a signaling cascade that leads to the activation of the serine/threonine kinase Akt (Akt) and several of its downstream targets, including mammalian target of rapamycin (mTOR) (Qian et al., 2009; Xu et al., 2020). Functionally, in response to CXCR4-mediated signaling, the PI3K/Akt pathway could regulate a variety of cellular activities, including cell migration and survival (Qian et al., 2009; Figure 2).
In addition to signaling via G proteins, activated receptors recruit β-arrestin, which can result in G protein-independent activation of MAPK signaling pathway (Wang and Knaut, 2014). Upon receptor activation, CXCR4 promotes the recruitment of GRKs and other kinases that induce site-specific phosphorylation of the cytoplasmic loops and C-terminus, leading to association with β-arrestin (Pozzobon et al., 2016). β-arrestin mediates receptor desensitization, and targets the receptor for lysosomal degradation following protein internalization and trafficking (Cojoc et al., 2013; Smith and Rajagopal, 2016). Furthermore, β-arrestin also serves as scaffolds for the activation of a variety of signaling molecules, including transcription factors and kinases, such as extracellular signal-regulated kinases 1/2 (ERK1/2) in complex with RAF and MEK1/2 (Figure 2; Mamazhakypov et al., 2021). These pathways, together with the heterotrimeric G proteins-mediated signaling, play important roles in the pathophysiology of diseases, including liver disease (Figure 3).
Figure 3. Regulation of liver disease by CXCR4 and its ligand. (A) In toxin-induced hepatitis, the CXCL12/CXCR4 axis promotes the migration of CD4+ and CD8+ cells to the liver and induces aggregation of inflammation. (B) CXCL12 is dependent on CXCR4 to promote the recruitment of CD4+ T cells in NASH, and the NFκB-CXCR4/7 pathway further promotes inflammation in NASH by forming Mallory-Denk Bodies (MDB). (C) The CXCL12/CXCR4 pathway is involved in the recruitment and homing of immune cells in the liver during viral hepatitis (HBV, HCV, and HEV) infection. In HBV, hepatitis B virus X protein (HBx) facilitates recruitment via CXCL12 signaling, and CXCR4 is highly expressed in CD8+ T cells after PEGylated interferon-α (INF-α) treatment and inversely correlates with HBV DNA loads. (D) The CXCL12/CXCR4 axis promotes migration and homing of mesenchymal stem cells (MSCs), bone marrow mesenchymal stromal stem cells (BMSCs), umbilical cord-derived mesenchymal stem cells (UC-MSCs), adipose-derived stem cells (ADSCs), urine-derived stem cells (USCs) and hematopoietic stem cells to the injured liver to ameliorate liver injury and promote liver repair, proliferation and regeneration. Activation of the PI3K/Akt signaling pathway by CXCR4 in BMSCs could promote cell migration, resulting in better therapeutic effects for liver injury. Hypoxia preconditioning promotes the proliferation and migration of USCs by inducing CXCR4 signaling, leading to recovery from liver injury. (E) CXCL12 activation of CXCR4 promotes hepatic stellate cells (HSCs) differentiation, proliferation, activation and contraction via the MAPK, ERK1/2, PI3K/Akt and calcium-independent pathways, inducing collagen I production with fibrotic effects. CXCR4 HIV promotes liver fibrosis by promoting the phosphorylation of ERK1/2 on activated HSCs and inducing reactive oxygen species (ROS) production in HSCs. The CXCL12/CXCR4 axis induces migration of MSCs and endothelial progenitor cells (EPCs) into fibrotic liver, aggravating and inhibiting liver fibrosis, respectively. (F) The CXCL12/CXCR4 axis promotes HCC cells growth, proliferation, metastasis and invasion, and vascular mimicry formation via activation of heterotrimeric G proteins, MAPK/ERK and PI3K/Akt signaling pathways; and recruits Treg cells to the tumor sites to promote HCC. The CXCL12/CXCR4 axis enhances the recruitment of endothelial cells and EPCs to HCC and promotes tumor neovascularization. CXCR4 is highly expressed on endothelial cells and can be used as a novel vascular marker for vessel sprouting in HCC tissues.
Hepatitis is an inflammation of the liver that can be caused by different types of infectious agents such as toxins or viruses (Wang et al., 2021). If left untreated, hepatitis can lead to serious health problems, including liver damage, liver fibrosis and cirrhosis, liver failure, liver cancer, and even death. Although the pathophysiology of hepatitis has not been fully elucidated, many studies have demonstrated the role of CXCR4 and its ligand in hepatitis. CXCR4 normally interacts with CXCL12 to initiate downstream signaling pathways. CXCR4 then plays a crucial role in regulating signal transduction, maintaining the homeostasis of inflammatory responses, and chemotaxis of inflammatory cells. Importantly, the mechanisms of CXCR4 signaling mediated inflammatory responses may affect the effective chemotactic function of inflammatory cells, such as lymphocytes, neutrophils and monocytes (Tian et al., 2019). These inflammatory cells are chemotactic to the site of inflammation and migrate into the tissues, which in turn participates in the inflammatory response of the tissues (Shi and Pamer, 2011). Indeed, in inflammatory liver disease, most liver-infiltrating lymphocytes express CXCR4, and its intensity is more significantly up-regulated in liver-infiltrating lymphocytes than in peripheral blood lymphocytes (Terada et al., 2003). Here, in Concanavalin A-induced T cell mediated hepatitis, the transmigration of CXCR4+ total CD4+ T cells are enhanced and accumulates in the inflamed liver tissue. This hepatic recruitment of CD4+ T cells population is mainly facilitated by LSECs providing perivascularly expressed CXCL12 through CXCR4 dependent intracellular transport mechanisms (Lutter et al., 2015). Consistently, in hepatitis with alcoholic liver disease, CXCR4 dependent migration of lymphocytes into the tissue is significantly increased in response to treatment with ethanol, resulting in recruitment of CD4+ and CD8+ lymphocytes into liver tissue (Karim et al., 2013). The homing and migration of inflammatory cells to the liver is also critical for the progression of non-alcoholic steatohepatitis (NASH). The pathophysiology of NASH has not been completely elucidated, but it is generally accepted in academia that immune cell recruitment is a crucial factor in initiating and expanding liver inflammation, which contributes to the progression from simple steatosis to NASH (Yu et al., 2019). Here, CXCL12 and CXCR4 protein levels are significantly increased, and CD4+ T cells are hyperresponsive to CXCL12 in NASH liver (Bigorgne et al., 2008; Li et al., 2020). Importantly, CXCL12 promotes the recruitment of CD4+ T cells in NASH and is dependent on CXCR4, which is attributed to the increased affinity of CXCL12 to CXCR4 (Boujedidi et al., 2014). Moreover, in NASH, Mallory-Denk Bodies (MDB) is formed via the NFκB-CXCR4/7 (CXCR4 and CXCR7) pathway, which in turn participates in ongoing inflammation (Liu H. et al., 2014). Notably, AMD3100, as a CXCR4 antagonist inhibits the chemotactic effect of CXCL12 to CD4+ T cells and reduces the number of CD4+ T cells that reach the liver (Boujedidi et al., 2014). Thus, CXCR4 and its ligand offer potential targets for pharmacologic therapies for NASH.
The CXCR4 signaling pathway also plays a vital role in virus-induced hepatitis. Viral hepatitis is one of the most common chronic liver disease, and persistent viral infection could lead to liver fibrosis and cirrhosis, HCC and liver failure (Ringehan et al., 2017). One of the important reasons for the progression of viral hepatitis is immune imbalance, which may be mediated by inflammatory cells. Notably, during chronic hepatitis virus infection, chemokine-chemokine receptor interactions are particularly critical for recruiting T cells to sites of inflammation in the liver (Nishitsuji et al., 2013). Indeed, the CXCL12/CXCR4 pathway plays a crucial role in the recruitment and retention of T cells in the liver during chronic hepatitis C virus (HCV) and hepatitis B virus (HBV) infection (Wald et al., 2004). The expression of CXCR4 is significantly enhanced in HCV and HBV-associated hepatitis tissues compared to normal liver tissues (Hong et al., 2009; Boissonnas et al., 2016; Zhu et al., 2016). Notably, in isolated peripheral blood cells from HBV patients treated with PEGylated interferon-α (IFN-α), CXCR4 is also highly expressed in CD8+ T cells, which is inversely correlated with HBV DNA loads (Liu et al., 2012). Interestingly, recent studies have shown that hepatitis B virus X protein (HBx) is a main factor in the development of HBV-induced disease. HBx increases endoplasmic reticulum (ER) stress-dependent CXCL12 expression and mediates HBV-induced recruitment of immune cells into the liver via CXCL12 signaling (Cho et al., 2014). Furthermore, HBx is involved in the occurrence and development of HBV-related HCC through the CXCL12/CXCR4/β-catenin signaling axis (Wang C. et al., 2017). However, the inhibitory effect of AMD3100 on CXCR4 significantly suppressed CXCL12 signaling-mediated recruitment of immune cells in HBV liver, and significantly disrupted the effect of CXCL12 on the self-renewal capacity of HBx-expressing cancer stem-like cells (CSCs) in HBV-related HCC (Cho et al., 2014; Wang C. et al., 2017). During hepatitis E virus (HEV) infection, CXCR4 expression is increased in immune cells from the periphery in patients. Here, the overall profile of tissue-specific homing receptor CXCR4 expression on the surface of effector/memory peripheral lymphocytes suggests that these cells are targeted to homing specifically to the liver (TrehanPati et al., 2011). Altogether, these data indicate that CXCR4 and its ligand are essential for hepatitis and provide novel ideas for further diagnosis and treatment.
Acute liver injury is the manifestation of sudden hepatic injury and arises from a variety of causes, such as surgical resection, chemical exposure or ischemia/reperfusion (I/R) events. Liver regeneration is critical for acute restoration of liver mass after resection or injury (DeLeve, 2013). Liver regeneration after acute injury is always beneficial and has been intensively studied. Experimental models involving partial hepatectomy or chemical injury have revealed relevant cellular signaling pathways that are used to restore the liver to equivalent mass and function to those prior to injury (Kitto and Henderson, 2020; Michalopoulos and Bhushan, 2020). Notably, the CXCL12/CXCR4 axis has received widespread attention in these signaling pathways. The study found that CXCR4 conditional knock-out mice (i.e., CXCR4f/null mice were crossed with MxCre mice to get MxCre-CXCR4f/null mice; CXCR4 was conditionally deleted after induction of Cre expression by intraperitoneal injection of poly(I)-poly(C) (pIpC) in eight-week-old mice) are susceptible to severe liver injury, with increased mRNA expression of several markers related to liver injury and regeneration in the liver, suggesting that the CXCL12/CXCR4 signaling is essential for liver regeneration and prevention of liver disease progression (Tsuchiya et al., 2012).
Currently, mesenchymal stem cells (MSCs) from different sources are considered to have enormous potential in the treatment of acute liver injury (Deng et al., 2014; Xiu et al., 2020). These cells need to migrate to the injury sites to function, which may be regulated by the CXCL12/CXCR4 signaling axis. Indeed, the CXCL12/CXCR4 axis promotes the migration of MSCs to the injury sites to repair liver injury by differentiating into and fusing with hepatocytes (Hao et al., 2015). Moreover, targeted migration of MSCs modified with CXCR4 to acute failing liver improves liver regeneration (Ma et al., 2014). Similarly, migration and engraftment of MSCs overexpressing CXCR4 into liver grafts improves early liver regeneration of small-for-size liver grafts (Du et al., 2013). However, down-regulation of CXCL12 expression could suppress the directional migration of these MSCs to the injured liver (Lü et al., 2012). The migration of bone marrow mesenchymal stromal/stem cells (BMSCs) is also regulated by the CXCL12/CXCR4 signaling, which is involved in the recruitment of BMSCs to the injured liver, while AMD3100 or anti-CXCR4 antibody can block this migration (Xiao Ling et al., 2016). Interestingly, overexpression of CXCR4 in BMSCs can substantially promote their migration and result in even better therapeutic effects for acute liver injury. This may be attributed to the activation of PI3K/Akt signaling pathway in BMSCs that is downstream of CXCR4 (Xiu et al., 2020). The CXCL12/CXCR4 axis similarly regulates the migration of umbilical cord-derived mesenchymal stem cells (UC-MSCs) to the injured liver. Herein, the pretreatment of UC-MSCs by rapamycin increases CXCR4 expression, enhances the homing and migratory capacity of these cells through the CXCL12/CXCR4 axis and ameliorates liver I/R injury (Zheng et al., 2018). Furthermore, up-regulation of CXCR4 in UC-MSCs induced by serum from rats with acute liver failure also promotes the migration and homing ability of stem cells to the injured liver, which may ultimately be used to treat liver disease (Deng et al., 2014).
The CXCR4 signaling pathway has also been proven to promote the migration and directional distribution of other stem cells at the injury sites (Wu et al., 2015). Urine-derived stem cells (USCs) have strong self-renewal capacity and multi-directional differentiation potential. Hypoxia preconditioning promotes the proliferation, migration and cell fusion of USCs by inducing CXCR4 signaling, leading to liver tissue recovery following injury (Hu et al., 2021). Based on the mechanism of the CXCL12/CXCR4 axis, the systemically transplanted adipose-derived stem cells (ADSCs) home to the injured liver after transplantation can stimulate liver regeneration in hepatectomy and I/R injured model mice (Saito et al., 2014). In addition, bone marrow (BM) and hematopoietic stem cells also participate in liver regeneration and proliferation. CXCL12 is required for effective hematopoietic stem cells mobilization and homing to the liver after hepatectomy (Lehwald et al., 2014). Specifically, hematopoietic stem cells are released from the BM into the peripheral blood, and matrix metalloproteinase 9 (MMP9) contributes to the mobilization of BM cells in the injured liver by up-regulating the expression of CXCR4 on BM cells and attracting BM cells along their CXCL12 gradient (Kawai et al., 2012). Moreover, up-regulation of CXCL12 expression also increases recruitment and mobilization of CXCR7+ BM progenitors of LSECs in the liver and promotes liver regeneration (DeLeve et al., 2016). In summary, accumulating evidence indicates that the CXCR4 signaling pathway plays a vital role in the pathophysiology of liver injury and regeneration, and strategies targeting this pathway may therefore be of therapeutic potential.
Liver fibrosis is the result of a sustained wound-healing response subsequent to chronic liver injury and aims to restore liver integrity after injury caused by different causes (Kamdem et al., 2018). If left untreated, advanced liver fibrosis can lead to cirrhosis, portal hypertension, and eventually HCC and liver failure (Marra and Tacke, 2014; Wang S. et al., 2017). Currently, academic opinion holds that a variety of cells, mainly HSCs, play a vital role in the pathophysiology of liver fibrosis (Higashi et al., 2017). In recent years, with the in-depth study of the mechanism of liver fibrosis, it has been found that CXCR4 and its ligand play a critical role in the pathogenesis of liver fibrosis via the activation and recruitment of various cells (Chen et al., 2014; Zhang et al., 2015). Here, the expression levels of CXCL12 and CXCR4 are significantly elevated in liver fibrosis and cirrhosis (Wald et al., 2004; Saiman et al., 2015; Xiang et al., 2017; Chalin et al., 2019). In response to CXCL12, cells (such as HSCs) expressing CXCR4 can participate in fibrosis and cirrhosis through migration and activation. HSCs are the cellular source of most of the extracellular matrix (ECM), and their activation and migration are the central link of liver fibrosis (Qin L. et al., 2018). Indeed, HSCs express CXCR4 receptor in vitro and in vivo, CXCR4 activation by CXCL12 directly promotes HSCs differentiation, proliferation and activation through the MAPK, ERK1/2 and PI3K/Akt pathways, which has a fibrotic effect (Hong et al., 2009; Chen et al., 2014). Moreover, CXCL12 acting on CXCR4 also promotes the contraction and activation of HSCs in a calcium-independent pathway (Saiman et al., 2013). Interestingly, studies have shown that CXCR4 expression can be induced in activated HSCs during the progression of liver fibrosis (Chow et al., 2016; Yang et al., 2019). The predominance of CXCR4 expression shift angiocrine response of LSECs, and reversely stimulate the proliferation of HSCs (Ding et al., 2013). Subsequently, the binding of CXCL12 to CXCR4 also induces HSCs proliferation and production of collagen I (Chow et al., 2016). In addition, in human immunodeficiency virus (HIV)/HCV co-infected livers, the HIV-1 × 4-envelope protein gp120 promotes the phosphorylation of ERK1/2 by interacting with CXCR4 on activated HSCs and has a pro-fibrogenic effects (Zheng et al., 2012). CXCR4 HIV also regulates the progression of liver fibrosis by inducing reactive oxygen species (ROS) production in HSCs and further promoting the expression of fibrogenesis-related genes (Lin et al., 2011). Given the critical role of HSCs activation in the progression of liver fibrosis, these studies suggest that specific targeting of CXCR4 and its ligand may be beneficial in liver fibrosis.
Currently, several studies have dissected the role of CXCR4 signaling pathway in HSCs and explored therapeutic interventions targeting this pathway in liver fibrosis. In vitro and in vivo studies have found that the inhibition of the CXCL12/CXCR4 biological axis in liver fibrosis can protect against the activation and migration of HSCs, and thus attenuates liver fibrosis (Liu et al., 2016; Qin L. et al., 2018; Sung et al., 2018; Ullah et al., 2019). Therefore, specifically targeting CXCR4 for the treatment of liver fibrosis has become a focus of research. Here, vascular endothelial growth factor (VEGF) siRNAs and CXCR4 antagonist AMD3100 encapsulated in nanoparticles (NPs) targeting CXCR4 can be delivered to fibrotic liver. Upon entry into the liver, VEGF siRNAs decrease VEGF expression, inhibit angiogenesis and normalize the distorted vessels in the fibrotic livers in the carbon tetrachloride (CCl4)-induced mouse model; AMD3100, as a targeting moiety, suppresses the progression of fibrosis by inhibiting the proliferation and activation of HSCs (Liu et al., 2016). Similarly, combined delivery of MEK inhibitor and sorafenib to the liver via CXCR4-targeted NPs prevents activation of ERK in activated HSCs and also has anti-fibrotic effects in the CCl4-induced mouse model (Sung et al., 2018). Furthermore, co-encapsulation of AMD3100 and pirfenidone into CXCR4-targeted combination liposomes for CXCR4 targeting displayed aggressive apoptosis in TGFβ-induced activated HSCs and significantly reduced α-SMA, suggesting a propensity to fibrosis regression (Ullah et al., 2019). But, surprisingly, in the chronic CCl4 model of liver injury, treatment of mice with AMD3100 did not improve hepatic fibrosis, and even aggravated liver fibrosis and inflammation with a specific increase in intrahepatic neutrophils (Saiman et al., 2015). The reason for this contradiction may be related to the targeted delivery method, as well as targeting different cells.
During liver fibrosis, CXCR4 pathway appears to be important for recruiting different cells to the injured liver, which may partly explain contradictory results of this pathway in the process of fibrosis and repair. In a mouse model of CCl4-induced liver fibrosis, the CXCL12/CXCR4 pathway is a critical chemotactic axis regulating the migration of MSCs from the bone marrow to the fibrotic liver, and recruited MSCs play different roles, including aggravating liver fibrosis and attenuating liver injury (Chen et al., 2009; Liu Y. et al., 2015). Notably, corticosterone can inhibit the recruitment and migration of MSCs via down-regulating CXCR4 and CXCR7 expression in MSCs (Zhang et al., 2015). In contrast, transplanted CXCR4-positive expanded endothelial progenitor cells (EPCs), induced by CXCL12 into the rat liver portal tracts, fibrous septa and hepatic sinusoids, effectively promote the remodeling of damaged tissues of liver fibrosis and suppress liver fibrogenesis (Nakamura et al., 2012). Taken together, CXCR4 and its ligand are functionally and mechanistically involved in the progression of liver fibrosis. However, simply blocking profibrotic CXCL12/CXCR4 axis is not sufficient to ameliorate liver fibrosis in vivo. Thus, it is necessary to adopt more cell types, combined with targeted delivery or specific strategies to modulate the CXCL12/CXCR4 signaling to target this pathway in liver fibrosis.
Hepatocellular carcinoma (HCC) is the most common primary malignant tumor of the liver with a high worldwide prevalence and poor prognosis (Hu et al., 2020). Metastasis is the main event leading to death in the vast majority of HCC patients (Ye et al., 2016; Yin et al., 2019). Recent studies have shown that the tumor microenvironment (TME) plays a crucial role in cancer metastasis and development (Ye et al., 2016; Chen x. et al., 2018). During the metastasis and development of HCC, there is neovascularization and the recruitment and migration of related cells in the TME (Shen et al., 2010; Katayama et al., 2019). Here, CXCR4 signaling is the major pathway involved in the above activities in the TME (Wang et al., 2016). Indeed, many studies have found that the expressions of CXCL12 and CXCR4 in peripheral blood of HCC patients are significantly increased, and CXCR4 expression is positively correlated with lymph node metastasis and poor outcome of HCC, affecting the prognosis of HCC patients (Xiang et al., 2009; Neve Polimeno et al., 2014; Toraih et al., 2016; Qin L. F. et al., 2018). Moreover, high levels of CXCL12 are also detected in malignant biopsies of HCC patients. CXCL12 plays a vital role in the recruitment of Treg cells into TME. Increased numbers of Treg cells were shown in peripheral blood as well as in the tumor tissue. In brief, CXCL12 secreted in the TME recruits CD4+CD25+ Treg cells to the tumor sites to contribute to the growth and prosperity of HCC (Shen et al., 2010). Notably, the secretory CXCL12 in turn regulates CXCR4 in endothelial cells, reticular fibers to modulate the TME and regulate neovascularization, which may contribute to the distant metastasis of HCC. Furthermore, increased CXCL12 concentration in the TME activates the CXCL12/CXCR4 axis and enhances the recruitment of EPCs to HCC, which also promotes tumor neovascularization (Wang et al., 2016; Tsai et al., 2020). Neovascularization is known to be one of the major characteristics of HCC. CXCR4 is selectively expressed on a fraction of tumor endothelial cells in HCC tissues, and high levels of CXCR4 tend to develop a sinusoidal vasculature in tumors, which can be used as a novel vascular marker for vessel sprouting in HCC tissues (Meng et al., 2017; Xu et al., 2017). Mechanistically, up-regulated CXCR4 expression on endothelial cells is mediated by the ERK pathway induced by inflammatory cytokines derived from tumor conditioned monocytes/macrophages (Meng et al., 2017). Activated CXCR4/ERK signaling pathway, in turn, promotes HCC metastasis through M2 macrophage polarization (Cai J. et al., 2020). Interestingly, CXCL12 enhances the expression of VE-cadherin, matrix metalloproteinase 2 (MMP2) and laminin5γ2 via CXCR4 in tumor cells (rather than endothelial cells), forming vascular-like channels that promote vascular mimicry (VM) formation and provide blood perfusion for HCC tissues (Yang et al., 2016). In addition, stimulation of the CXCL12/CXCR4 signaling contributes to organ colonization with blood circulating tumor cells in HCC (Tang et al., 2019). Increased CXCR4 expression on tumor cells also leads to invasion, migration and epithelial-mesenchymal transition (EMT) of HCC cells (Gao et al., 2018).
Therefore, the importance of CXCL12/CXCR4 signaling in HCC tumor cells can be foreseen. Indeed, CXCR4 and its ligand CXCL12 initiate cell migration and angiogenesis via activation of the MAPK/ERK and PI3K/Akt signaling pathways, thereby promoting HCC cells growth, proliferation, metastasis and invasion (Yang et al., 2019). Similarly, CXCR7 signaling can also promote angiogenesis as well as HCC cells growth, invasiveness and differentiation by activating MAPK/ERK and Akt signaling pathways (Lin et al., 2014; Xue et al., 2014; Chen et al., 2016). Interestingly, the CXCL12/CXCR4 signaling induces the expression of matrix metalloproteinase 10 (MMP10) in HCC cells through the ERK1/2 pathway, which contributes to angiogenesis, growth and dissemination of HCC, and in turn, HCC cells stably expressing MMP10 have increased CXCR4 expression and migration capacity. This reciprocal crosstalk between the CXCL12/CXCR4 signaling and MMP10 contributes to the metastasis and progression of HCC (García-Irigoyen et al., 2015). Moreover, CXCR4 stimulated by CXCL12 also triggers activation of heterotrimeric G proteins, which regulate the chemotaxis and migration of HCC cells. Specifically, CXCR4-induced signaling pathways, including Gαi, Annexin A2 and Rac, activate actin polymerization to migrate HCC cells (Li et al., 2019). Notably, CXCR4 serves as an important intracellular signal transducer, can relay matrix stiffness signals through ubiquitin domain-containing protein 1 (UBTD1)-mediated YAP signaling pathway to drive HCC progression (Yang et al., 2020). Recent studies have shown that CXCL12 improves cell invasion potential of HCC cells and CXCR4 overexpression is associated with aggressive characteristics and poor prognosis of HCC, while inhibition of CXCR4 activity via CXCR4 knockdown, AMD3100 or neutralizing antibody administration suppresses tumorigenesis of hepatoma cells in vitro and in vivo (Liu H. et al., 2015; Lu et al., 2015). Thus, the CXCL12/CXCR4 signaling has become an attractive target for the diagnosis and treatment of HCC.
The CXCR4 signaling pathway is receiving increasing attention because it is clear that targeting this pathway may be beneficial for HCC. Here, targeting CXCR4 by CRISPR/Cas9 in HCC cells can inhibit invasion, proliferation and migration, reverse EMT, increase chemosensitivity and decrease the malignancy of HCC in vitro and in vivo (Wang X. et al., 2017). In addition, the study found that emodin exerts anti-HCC effects by targeting and down-regulating CXCR4, which is related to its inhibition of CXCL12-induced invasion and migration in HCC cell lines (Man et al., 2013). Another study found that plumbagin restrains HCC angiogenesis, as well as HCC cell proliferation and invasion by inhibiting the CXCL12/CXCR4-CXCR7 axis (Zhong et al., 2019). Notably, inhibition of CXCR7 expression by transfection with CXCR7-short hairpin RNA (shRNA) could significantly inhibit HCC cells and tumor endothelial cells proliferation, invasion, migration and angiogenesis (Zheng et al., 2010; Zhao et al., 2017; Wu et al., 2018). Currently, sorafenib is a multitargeted tyrosine kinase inhibitor approved as a systemic anti-angiogenic agent for advanced HCC, but its clinical application is limited due to moderate therapeutic efficacy and high incidence of acquired resistance resulted from elevated levels of the CXCL12/CXCR4 signaling induced by prolonged sorafenib treatment (Zheng et al., 2019). Thus, targeting down-regulation of CXCR4 expression or intervention in the CXCL12/CXCR4 signaling pathway might overcome sorafenib evasion and resistance (Gao et al., 2015; Zheng et al., 2019). Indeed, formulation of sorafenib in CXCR4-targeted lipid-coated poly (lactic-coglycolic acid) (PLGA) NPs modified with AMD3100 efficiently delivers sorafenib into HCC and human umbilical vein endothelial cells to achieve cytotoxicity and anti-angiogenic effect in vitro and in vivo. This highlights the clinical potential of CXCR4-targeted NPs for delivering sorafenib and overcoming acquired drug resistance in HCC (Gao et al., 2015). Similarly, CXCR4-targeted PEGylated PLGA NPs could co-deliver sorafenib and metapristone (chemopreventive agent targeting SDF-1/CXCR4 axis) into HCC in vitro and in vivo, thereby enhancing cytotoxicity and synergistically suppressing HCC proliferation and resistance (Zheng et al., 2019). Furthermore, the co-delivery of CXCR4-targeted NPs with MEK inhibitor and sorafenib to HCC can increase the feasibility of dual RAF/MEK inhibition to overcome sorafenib treatment evasion in HCC (Chen et al., 2017). Notably, encapsulation of AMD3100 and anti-angiogenic substance VEGF siRNA into lipid-based NPs formulations targeting CXCR4, namely AMD-NPs, could effectively deliver VEGF siRNAs into HCC and down-regulate VEGF expression in vitro and in vivo. Inhibition of CXCR4 by AMD-NPs in combination with either conventional sorafenib treatment or VEGF siRNA induces synergistic anti-angiogenic effects and inhibits local and distant tumor growth in HCC (Liu J. Y. et al., 2015). Targeting CXCR4 with AMD3100 also prevents the polarization toward an immunosuppressive microenvironment after sorafenib treatment, suppresses HCC tumor growth, reduces metastasis and improves survival (Chen et al., 2015). Collectively, targeting the CXCR4 signaling pathway in combination with sorafenib may provide a promising approach for the safe and effective treatment of HCC.
There is strong evidence that CXCR4 and its ligand play a key role in multiple liver diseases such as hepatitis, liver injury and regeneration, liver fibrosis and cirrhosis, as well as in HCC. Specifically, CXCL12 finely regulates signal transduction by activating CXCR4 depending on the internal and external conditions of cells and the pathophysiology of the body, and then participates in the development of liver disease. In addition to its conventional role in mobilizing immune cells to the site of inflammation, the CXCR4 signaling pathway also mediates several cellular functions specific to liver disease, such as promoting the activation and proliferation of HSCs, and the migration and invasion of HCC cells (Table 1). Although not yet fully established, CXCR4 and its ligand would seem to have both beneficial and deleterious effects, depending on the type of cells they target. For instance, the CXCL12/CXCR4 axis induces migration of EPCs, which effectively promotes the remodeling of damaged tissues of liver fibrosis and suppress liver fibrogenesis; however, this axis also produces fibrotic effects by promoting the activation, proliferation and migration of HSCs. Thus, exploiting the pharmacological potential of targeting CXCR4 and its ligand in liver disease requires a better understanding of their divergent actions. The studies outlined in this review article support the view that modulation of CXCR4 and its ligand represents a viable approach in treating liver disease and that combination targeted therapy might become another safe and effective strategy for clinical liver disease treatment (summarized in Table 2). Importantly, although it seems very clear that CXCR4 and its ligand play crucial roles in the pathophysiology of several liver disease, it should be noted that the exact role of targeting different cells needs to be further studied before targeting the CXCL12/CXCR4 signaling to treat these diseases.
SW constructed the major theme and wrote the first draft of the manuscript. SG, YL, and XQ reviewed and revised the manuscript. JL and XL supported the work of the manuscript. All authors have read and approved the final manuscript before submission.
The authors declare that the research was conducted in the absence of any commercial or financial relationships that could be construed as a potential conflict of interest.
All claims expressed in this article are solely those of the authors and do not necessarily represent those of their affiliated organizations, or those of the publisher, the editors and the reviewers. Any product that may be evaluated in this article, or claim that may be made by its manufacturer, is not guaranteed or endorsed by the publisher.
This study was funded by the National Natural Science Foundation of China (Grant Nos. 81270498 and 81970518), the 2016 Annual Leading Talent Introduction and Cultivation Project in Universities (Grant No. gxbjZD2016032), the Nature and Science Fund from Wannan Medical College, China (Grant No. WKS201917), the Science and Technology Research Project of Anhui Province (Grant No. 1604a0802097), and the Natural Science Foundation of Anhui Province (Grant No. 1608085MH178).
Bar-Shavit, R., Maoz, M., Kancharla, A., Nag, J., Agranovich, D., Grisaru-Granovsky, S., et al. (2016). G Protein-coupled receptors in cancer. Int. J. Mol. Sci. 17:1320. doi: 10.3390/ijms17081320
Bigorgne, A. E., Bouchet–Delbos, L., Naveau, S., Dagher, I., Prévot, S., Durand–Gasselin, I., et al. (2008). Obesity-induced lymphocyte hyperresponsiveness to chemokines: a new mechanism of fatty liver inflammation in obese mice. Gastroenterology 134, 1459.e2–1469.e2. doi: 10.1053/j.gastro.2008.02.055
Boissonnas, A., Gadd, V. L., Patel, P. J., Jose, S., Horsfall, L., Powell, E. E., et al. (2016). Altered peripheral blood monocyte phenotype and function in chronic liver disease: implications for hepatic recruitment and systemic inflammation. PLoS One 11:e0157771. doi: 10.1371/journal.pone.0157771
Boujedidi, H., Robert, O., Bignon, A., Cassard-Doulcier, A.-M., Renoud, M.-L., Gary-Gouy, H., et al. (2014). CXCR4 dysfunction in non-alcoholic steatohepatitis in mice and patients. Clin. Sci. 128, 257–267. doi: 10.1042/cs20130833
Bussmann, J., and Raz, E. (2015). Chemokine-guided cell migration and motility in zebrafish development. EMBO J. 34, 1309–1318. doi: 10.15252/embj.201490105
Butcher, M. J., Wu, C.-I., Waseem, T., and Galkina, E. V. (2016). CXCR6 regulates the recruitment of pro-inflammatory IL-17A-producing T cells into atherosclerotic aortas. Int. Immunol. 28, 255–261. doi: 10.1093/intimm/dxv068
Cai, B., Dongiovanni, P., Corey, K. E., Wang, X., Shmarakov, I. O., Zheng, Z., et al. (2020). Macrophage MerTK promotes liver fibrosis in nonalcoholic steatohepatitis. Cell Metab. 31, 406.e7–421.e7. doi: 10.1016/j.cmet.2019.11.013
Cai, J., Zhang, Q., Qian, X., Li, J., Qi, Q., Sun, R., et al. (2020). Extracellular ubiquitin promotes hepatoma metastasis by mediating M2 macrophage polarization via the activation of the CXCR4/ERK signaling pathway. Annals Transl. Med. 8, 929–929. doi: 10.21037/atm-20-1054
Chalin, A., Lefevre, B., Devisme, C., Barget, N., Amiot, L., and Samson, M. (2019). Circulating levels of CXCL11 and CXCL12 are biomarkers of cirrhosis in patients with chronic hepatitis C infection. Cytokine 117, 72–78. doi: 10.1016/j.cyto.2019.02.006
Chang, H.-C., Huang, P.-H., Syu, F.-S., Hsieh, C.-H., Chang, S. L.-Y., Lu, J., et al. (2018). Critical involvement of atypical chemokine receptor CXCR7 in allergic airway inflammation. Immunology 154, 274–284. doi: 10.1111/imm.12881
Chen, W., Zhang, J., Fan, H., and Zhu, J. (2018). Function and therapeutic advances of chemokine and its receptor in nonalcoholic fatty liver disease. Therap. Adv. Gastroenterol. 11:1756284818815184. doi: 10.1177/1756284818815184
Chen, X., Zhang, S., Wang, Z., Wang, F., Cao, X., Wu, Q., et al. (2018). Supervillin promotes epithelial-mesenchymal transition and metastasis of hepatocellular carcinoma in hypoxia via activation of the RhoA/ROCK-ERK/p38 pathway. J. Exp. Clin. Cancer Res. 37:128. doi: 10.1186/s13046-018-0787-2
Chen, Y., Huang, Y., Reiberger, T., Duyverman, A. M., Huang, P., Samuel, R., et al. (2014). Differential effects of sorafenib on liver versus tumor fibrosis mediated by stromal-derived factor 1 alpha/C-X-C receptor type 4 axis and myeloid differentiation antigen-positive myeloid cell infiltration in mice. Hepatology 59, 1435–1447. doi: 10.1002/hep.26790
Chen, Y., Liu, Y.-C., Sung, Y.-C., Ramjiawan, R. R., Lin, T.-T., Chang, C.-C., et al. (2017). Overcoming sorafenib evasion in hepatocellular carcinoma using CXCR4-targeted nanoparticles to co-deliver MEK-inhibitors. Sci. Rep. 7:44123. doi: 10.1038/srep44123
Chen, Y., Ramjiawan, R. R., Reiberger, T., Ng, M. R., Hato, T., Huang, Y., et al. (2015). CXCR4 inhibition in tumor microenvironment facilitates anti-programmed death receptor-1 immunotherapy in sorafenib-treated hepatocellular carcinoma in mice. Hepatology 61, 1591–1602. doi: 10.1002/hep.27665
Chen, Y., Teng, F., Wang, G., and Nie, Z. (2016). Overexpression of CXCR7 induces angiogenic capacity of human hepatocellular carcinoma cells via the AKT signaling pathway. Oncol. Rep. 36, 2275–2281. doi: 10.3892/or.2016.5045
Chen, Y., Xiang, L.-X., Shao, J.-Z., Pan, R.-L., Wang, Y.-X., Dong, X.-J., et al. (2009). Recruitment of endogenous bone marrow mesenchymal stem cells towards injured liver. J. Cell. Mol. Med. 14, 1494–1508. doi: 10.1111/j.1582-4934.2009.00912.x
Chiou, E., and Zennadi, R. (2015). Gαs proteins activate p72Syk and p60-c-Src tyrosine kinases to mediate sickle red blood cell adhesion to endothelium via LW-αvβ3 and CD44–CD44 interactions. Int. J. Biochem. Cell Biol. 65, 40–51. doi: 10.1016/j.biocel.2015.05.013
Cho, H. K., Kim, S. Y., Seong, J. K., and Cheong, J. (2014). Hepatitis B virus X increases immune cell recruitment by induction of chemokine SDF-1. FEBS Lett. 588, 733–739. doi: 10.1016/j.febslet.2014.01.017
Choi, J., Selmi, C., Leung, P. S. C., Kenny, T. P., Roskams, T., and Gershwin, M. E. (2016). Chemokine and chemokine receptors in autoimmunity: the case of primary biliary cholangitis. Expert Rev. Clin. Immunol. 12, 661–672. doi: 10.1586/1744666x.2016.1147956
Chow, L. N., Schreiner, P., Ng, B. Y. Y., Lo, B., Hughes, M. R., Scott, R. W., et al. (2016). Impact of a CXCL12/CXCR4 Antagonist in Bleomycin (BLM) induced pulmonary fibrosis and carbon tetrachloride (CCl4) induced hepatic fibrosis in mice. PLoS One 11:e0151765. doi: 10.1371/journal.pone.0151765
Cojoc, M., Peitzsch, C., Trautmann, F., Polishchuk, L., Telegeev, G. D., and Dubrovska, A. (2013). Emerging targets in cancer management: role of the CXCL12/CXCR4 axis. Onco Targets Ther. 6, 1347–1361. doi: 10.2147/ott.s36109
Daniel, S. K., Seo, Y. D., and Pillarisetty, V. G. (2020). The CXCL12-CXCR4/CXCR7 axis as a mechanism of immune resistance in gastrointestinal malignancies. Semin. Cancer Biol. 65, 176–188. doi: 10.1016/j.semcancer.2019.12.007
DeLeve, L. D. (2013). Liver sinusoidal endothelial cells and liver regeneration. J. Clin. Invest. 123, 1861–1866. doi: 10.1172/jci66025
DeLeve, L. D., Wang, X., and Wang, L. (2016). VEGF-sdf1 recruitment of CXCR7+ bone marrow progenitors of liver sinusoidal endothelial cells promotes rat liver regeneration. Am. J. Physiol.-Gastrointestinal Liver Physiol. 310, G739–G746. doi: 10.1152/ajpgi.00056.2016
Deng, C., Qin, A., Zhao, W., Feng, T., Shi, C., and Liu, T. (2014). Up-regulation of CXCR4 in rat umbilical mesenchymal stem cells induced by serum from rat with acute liver failure promotes stem cells migration to injured liver tissue. Mol. Cell. Biochem. 396, 107–116. doi: 10.1007/s11010-014-2147-7)
Dessein, A.-F., Stechly, L., Jonckheere, N., Dumont, P., Monté, D., Leteurtre, E., et al. (2010). Autocrine induction of invasive and metastatic phenotypes by the MIF-CXCR4 axis in drug-resistant human colon cancer cells. Cancer Res. 70, 4644–4654. doi: 10.1158/0008-5472.can-09-3828
Ding, B.-S., Cao, Z., Lis, R., Nolan, D. J., Guo, P., Simons, M., et al. (2013). Divergent angiocrine signals from vascular niche balance liver regeneration and fibrosis. Nature 505, 97–102. doi: 10.1038/nature12681
Döring, Y., Noels, H., van der Vorst, E. P. C., Neideck, C., Egea, V., Drechsler, M., et al. (2017). Vascular CXCR4 limits atherosclerosis by maintaining arterial integrity. Circulation 136, 388–403. doi: 10.1161/circulationaha.117.027646
Du, Z., Wei, C., Yan, J., Han, B., Zhang, M., Peng, C., et al. (2013). Mesenchymal stem cells overexpressing C-X-C chemokine receptor Type 4 improve early liver regeneration of small-for-size liver grafts. Liver Transpl. 19, 215–225. doi: 10.1002/lt.23577
Engevik, K. A., Hanyu, H., Matthis, A. L., Zhang, T., Frey, M. R., Oshima, Y., et al. (2019). Trefoil factor 2 activation of CXCR4 requires calcium mobilization to drive epithelial repair in gastric organoids. J. Physiol. 597, 2673–2690. doi: 10.1113/jp277259
Gao, D.-Y., Lin, T.-T., Sung, Y.-C., Liu, Y. C., Chiang, W.-H., Chang, C.-C., et al. (2015). CXCR4-targeted lipid-coated PLGA nanoparticles deliver sorafenib and overcome acquired drug resistance in liver cancer. Biomaterials 67, 194–203. doi: 10.1016/j.biomaterials.2015.07.035
Gao, P.-T., Ding, G.-Y., Yang, X., Dong, R.-Z., Hu, B., Zhu, X.-D., et al. (2018). Invasive potential of hepatocellular carcinoma is enhanced by loss of selenium-binding protein 1 and subsequent upregulation of CXCR4. Am. J. Cancer Res. 8, 1040–1049.
García-Irigoyen, O., Latasa, M. U., Carotti, S., Uriarte, I., Elizalde, M., Urtasun, R., et al. (2015). Matrix metalloproteinase 10 contributes to hepatocarcinogenesis in a novel crosstalk with the stromal derived factor 1/C-X-C chemokine receptor 4 axis. Hepatology 62, 166–178. doi: 10.1002/hep.27798
Griffith, J. W., Sokol, C. L., and Luster, A. D. (2014). Chemokines and chemokine receptors: positioning cells for host defense and immunity. Annu. Rev. Immunol. 32, 659–702. doi: 10.1146/annurev-immunol-032713-120145
Guo, F., Wang, Y., Liu, J., Mok, S. C., Xue, F., and Zhang, W. (2015). CXCL12/CXCR4: a symbiotic bridge linking cancer cells and their stromal neighbors in oncogenic communication networks. Oncogene 35, 816–826. doi: 10.1038/onc.2015.139
Hao, N.-B., Li, C.-Z., Lü, M.-H., Tang, B., Wang, S.-M., Wu, Y.-Y., et al. (2015). SDF-1/CXCR4 Axis promotes MSCs to repair liver injury partially through trans-differentiation and fusion with hepatocytes. Stem Cells Int. 2015, 1–10. doi: 10.1155/2015/960387
Higashi, T., Friedman, S. L., and Hoshida, Y. (2017). Hepatic stellate cells as key target in liver fibrosis. Adv. Drug Delivery Rev. 121, 27–42. doi: 10.1016/j.addr.2017.05.007
Hong, F., Tuyama, A., Lee, T. F., Loke, J., Agarwal, R., Cheng, X., et al. (2009). Hepatic stellate cells express functional CXCR4: role in stromal cell-derived factor-1alpha-mediated stellate cell activation. Hepatology 49, 2055–2067. doi: 10.1002/hep.22890
Hu, B., Lin, J. Z., Yang, X. B., and Sang, X. T. (2020). Aberrant lipid metabolism in hepatocellular carcinoma cells as well as immune microenvironment: a review. Cell Prolif. 53:e12772. doi: 10.1111/cpr.12772
Hu, C., He, Y., Liu, D., Zhao, L., Fang, S., Tan, B., et al. (2021). Hypoxia preconditioning promotes the proliferation and migration of human urine-derived stem cells in chronically injured liver of mice by upregulating CXCR4. Stem Cells Dev. 30, 526–536. doi: 10.1089/scd.2021.0008
Hughes, C. E., and Nibbs, R. J. B. (2018). A guide to chemokines and their receptors. FEBS J. 285, 2944–2971. doi: 10.1111/febs.14466
Humpert, M.-L., Pinto, D., Jarrossay, D., and Thelen, M. (2014). CXCR7 influences the migration of B cells during maturation. Eur. J. Immunol. 44, 694–705. doi: 10.1002/eji.201343907
Irnaten, M., Duff, A., Clark, A., and O’Brien, C. (2020). Intra-cellular calcium signaling pathways (PKC, RAS/RAF/MAPK, PI3K) in lamina cribrosa cells in glaucoma. J. Clin. Med. 10:62. doi: 10.3390/jcm10010062
Janssens, R., Struyf, S., and Proost, P. (2018). Pathological roles of the homeostatic chemokine CXCL12. Cytokine Growth Factor Rev. 44, 51–68. doi: 10.1016/j.cytogfr.2018.10.004
Kamdem, S. D., Moyou-Somo, R., Brombacher, F., and Nono, J. K. (2018). Host regulators of liver fibrosis during human schistosomiasis. Front. Immunol. 9:2781. doi: 10.3389/fimmu.2018.02781
Karim, S., Liaskou, E., Hadley, S., Youster, J., Faint, J., Adams, D. H., et al. (2013). An in vitro model of human acute ethanol exposure that incorporates CXCR3- and CXCR4-dependent recruitment of immune cells. Toxicol. Sci. 132, 131–141. doi: 10.1093/toxsci/kfs337
Katayama, Y., Uchino, J., Chihara, Y., Tamiya, N., Kaneko, Y., Yamada, T., et al. (2019). Tumor neovascularization and developments in therapeutics. Cancers (Basel) 11:316. doi: 10.3390/cancers11030316
Kawai, K., Xue, F., Takahara, T., Kudo, H., Yata, Y., Zhang, W., et al. (2012). Matrix Metalloproteinase-9 contributes to the mobilization of bone marrow cells in the injured liver. Cell. Transplant. 21, 453–464. doi: 10.3727/096368911x605367
Kitto, L. J., and Henderson, N. C. (2020). Hepatic stellate cell regulation of liver regeneration and repair. Hepatol. Commun. 5, 358–370. doi: 10.1002/hep4.1628
Kolaczkowska, E., and Kubes, P. (2013). Neutrophil recruitment and function in health and inflammation. Nat. Rev. Immunol. 13, 159–175. doi: 10.1038/nri3399
Kormann, M. S. D., Hector, A., Marcos, V., Mays, L. E., Kappler, M., Illig, T., et al. (2012). CXCR1 and CXCR2 haplotypes synergistically modulate cystic fibrosis lung disease. Eur. Respir. J. 39, 1385–1390. doi: 10.1183/09031936.00130011
Laufer, J. M., Hauser, M. A., Kindinger, I., Purvanov, V., Pauli, A., and Legler, D. F. (2019). Chemokine receptor CCR7 triggers an endomembrane signaling complex for spatial rac activation. Cell Rep. 29, 995.e6–1009.e6. doi: 10.1016/j.celrep.2019.09.031
Lehwald, N., Duhme, C., Wildner, M., Kuhn, S., Fürst, G., Forbes, S. J., et al. (2014). HGF and SDF-1-mediated mobilization of CD133+ BMSC for hepatic regeneration following extensive liver resection. Liver Int. 34, 89–101. doi: 10.1111/liv.12195
Li, H., Wang, Y., Lu, Y., and Li, F. (2019). Annexin A2 interacting with ELMO1 regulates HCC chemotaxis and metastasis. Life Sci. 222, 168–174. doi: 10.1016/j.lfs.2019.03.003
Li, Y., Li, N., Liu, J., and An, X. (2020). Gr-1highLy6G+Myeloid-derived suppressor cells and their role in a murine model of non-alcoholic steatohepatitis. Am. J. Transl. Res. 12, 2827–2842.
Liepelt, A., and Tacke, F. (2016). Stromal cell-derived factor-1 (SDF-1) as a target in liver diseases. Am. J. Physiol.-Gastrointestinal Liver Physiol. 311, G203–G209. doi: 10.1152/ajpgi.00193.2016
Lin, L., Han, M. M., Wang, F., Xu, L. L., Yu, H. X., and Yang, P. Y. (2014). CXCR7 stimulates MAPK signaling to regulate hepatocellular carcinoma progression. Cell Death Dis. 5, e1488–e1488. doi: 10.1038/cddis.2014.392
Lin, W., Wu, G., Li, S., Weinberg, E. M., Kumthip, K., Peng, L. F., et al. (2011). HIV and HCV cooperatively promote hepatic fibrogenesis via induction of reactive oxygen species and NFκB. J. Biol. Chem. 286, 2665–2674. doi: 10.1074/jbc.M110.168286
Liu, C.-H., Chan, K.-M., Chiang, T., Liu, J.-Y., Chern, G.-G., Hsu, F.-F., et al. (2016). Dual-Functional nanoparticles targeting CXCR4 and delivering antiangiogenic siRNA ameliorate liver fibrosis. Mol. Pharm. 13, 2253–2262. doi: 10.1021/acs.molpharmaceut.5b00913
Liu, H., Li, J., Tillman, B., Morgan, T. R., French, B. A., and French, S. W. (2014). TLR3/4 signaling is mediated via the NFκB-CXCR4/7 pathway in human alcoholic hepatitis and non-alcoholic steatohepatitis which formed Mallory–Denk bodies. Exp. Mol. Pathol. 97, 234–240. doi: 10.1016/j.yexmp.2014.07.001
Liu, H., Liu, Y., Liu, W., Zhang, W., and Xu, J. (2015). EZH2-mediated loss of miR-622 determines CXCR4 activation in hepatocellular carcinoma. Nat. Commun. 6:8494. doi: 10.1038/ncomms9494
Liu, J.-Y., Chiang, T., Liu, C.-H., Chern, G.-G., Lin, T.-T., Gao, D.-Y., et al. (2015). Delivery of siRNA Using CXCR4-targeted nanoparticles modulates tumor microenvironment and achieves a potent antitumor response in liver cancer. Mol. Ther. 23, 1772–1782. doi: 10.1038/mt.2015.147
Liu, Y., Yang, X., Jing, Y., Zhang, S., Zong, C., Jiang, J., et al. (2015). Contribution and Mobilization of Mesenchymal Stem Cells in a mouse model of carbon tetrachloride-induced liver fibrosis. Sci. Rep. 5:17762. doi: 10.1038/srep17762
Liu, Y., Hou, F., Ding, P., Ren, Y., Li, S., and Wang, G. (2012). Pegylated interferon α enhances recovery of memory T cells in e antigen positive chronic hepatitis B patients. Virol J. 9:274. doi: 10.1186/1743-422x-9-274
Lu, L., Lu, M., Pei, Y., Chen, J., Qin, L., Zhu, W., et al. (2015). Down-regulation of SDF1-α expression in tumor microenvironment is associated with aspirin-mediated suppression of the pro-metastasis effect of sorafenib in hepatocellular carcinoma. Acta Biochim. Biophys. Sinica 47, 988–996. doi: 10.1093/abbs/gmv112
Lü, M.-H., Li, C.-Z., Hu, C.-J., Fan, Y.-H., Wang, S.-M., Wu, Y.-Y., et al. (2012). microRNA-27b suppresses mouse MSC migration to the liver by targeting SDF-1α in vitro. Biochem. Biophys. Res. Commun. 421, 389–395. doi: 10.1016/j.bbrc.2012.04.027
Lutter, R., Neumann, K., Erben, U., Kruse, N., Wechsung, K., Schumann, M., et al. (2015). Chemokine transfer by liver sinusoidal endothelial cells contributes to the recruitment of CD4+ T cells into the murine liver. PLoS One 10:e0123867. doi: 10.1371/journal.pone.0123867
Ma, H.-C., Shi, X.-L., Ren, H.-Z., Yuan, X.-W., and Ding, Y.-T. (2014). Targeted migration of mesenchymal stem cells modified with CXCR4 to acute failing liver improves liver regeneration. World J. Gastroenterol. 20:14884. doi: 10.3748/wjg.v20.i40.14884
Mamazhakypov, A., Viswanathan, G., Lawrie, A., Schermuly, R. T., and Rajagopal, S. (2021). The role of chemokines and chemokine receptors in pulmonary arterial hypertension. Br. J. Pharmacol. 178, 72–89. doi: 10.1111/bph.14826
Man, K., Manu, K. A., Shanmugam, M. K., Ong, T. H., Subramaniam, A., Siveen, K. S., et al. (2013). Emodin suppresses migration and invasion through the modulation of CXCR4 expression in an orthotopic model of human hepatocellular carcinoma. PLoS One 8:e57015. doi: 10.1371/journal.pone.0057015
Marcellin, P., and Kutala, B. K. (2018). Liver diseases: a major, neglected global public health problem requiring urgent actions and large-scale screening. Liver Int. 38(Suppl. 1), 2–6. doi: 10.1111/liv.13682
Marra, F., and Tacke, F. (2014). Roles for chemokines in liver disease. Gastroenterology 147, 577.e1–594.e1. doi: 10.1053/j.gastro.2014.06.043
Mattos, M. S., Ferrero, M. R., Kraemer, L., Lopes, G. A. O., Reis, D. C., Cassali, G. D., et al. (2020). CXCR1 and CXCR2 inhibition by ladarixin improves neutrophil-dependent airway inflammation in mice. Front. Immunol. 11:566953. doi: 10.3389/fimmu.2020.566953
Meng, Y.-M., Liang, J., Wu, C., Xu, J., Zeng, D.-N., Yu, X.-J., et al. (2017). Monocytes/Macrophages promote vascular CXCR4 expression via the ERK pathway in hepatocellular carcinoma. OncoImmunology 7:e1408745. doi: 10.1080/2162402x.2017.1408745
Meyrath, M., Szpakowska, M., Zeiner, J., Massotte, L., Merz, M. P., Benkel, T., et al. (2020). The atypical chemokine receptor ACKR3/CXCR7 is a broad-spectrum scavenger for opioid peptides. Nat. Commun. 11:3033. doi: 10.1038/s41467-020-16664-0
Michalopoulos, G. K., and Bhushan, B. (2020). Liver regeneration: biological and pathological mechanisms and implications. Nat. Rev. Gastroenterol. Hepatol. 18, 40–55. doi: 10.1038/s41575-020-0342-4
Murphy, P. M., and Heusinkveld, L. (2018). Multisystem multitasking by CXCL12 and its receptors CXCR4 and ACKR3. Cytokine 109, 2–10. doi: 10.1016/j.cyto.2017.12.022
Nakamura, T., Torimura, T., Iwamoto, H., Masuda, H., Naitou, M., Koga, H., et al. (2012). Prevention of liver fibrosis and liver reconstitution of DMN-treated rat liver by transplanted EPCs. Eur. J. Clin. Invest. 42, 717–728. doi: 10.1111/j.1365-2362.2011.02637.x
Neve Polimeno, M., Ierano, C., D’Alterio, C., Simona Losito, N., Napolitano, M., Portella, L., et al. (2014). CXCR4 expression affects overall survival of HCC patients whereas CXCR7 expression does not. Cell. Mol. Immunol. 12, 474–482. doi: 10.1038/cmi.2014.102
Nishitsuji, H., Funami, K., Shimizu, Y., Ujino, S., Sugiyama, K., Seya, T., et al. (2013). Hepatitis C virus infection induces inflammatory cytokines and chemokines mediated by the cross talk between hepatocytes and stellate cells. J. Virol. 87, 8169–8178. doi: 10.1128/jvi.00974-13
Piovan, E., Tosello, V., Amadori, A., and Zanovello, P. (2018). Chemotactic cues for NOTCH1-dependent Leukemia. Front. Immunol. 9:633. doi: 10.3389/fimmu.2018.00633
Poisson, J., Lemoinne, S., Boulanger, C., Durand, F., Moreau, R., Valla, D., et al. (2017). Liver sinusoidal endothelial cells: physiology and role in liver diseases. J. Hepatol. 66, 212–227. doi: 10.1016/j.jhep.2016.07.009
Pozzobon, T., Goldoni, G., Viola, A., and Molon, B. (2016). CXCR4 signaling in health and disease. Immunol. Lett. 177, 6–15. doi: 10.1016/j.imlet.2016.06.006
Qian, C.-N., Furge, K. A., Knol, J., Huang, D., Chen, J., Dykema, K. J., et al. (2009). Activation of the PI3K/AKT pathway induces urothelial carcinoma of the renal pelvis: identification in human tumors and confirmation in animal models. Cancer Res. 69, 8256–8264. doi: 10.1158/0008-5472.can-09-1689
Qin, L., Qin, J., Zhen, X., Yang, Q., and Huang, L. (2018). Curcumin protects against hepatic stellate cells activation and migration by inhibiting the CXCL12/CXCR4 biological axis in liver fibrosis:a study in vitro and in vivo. Biomed. Pharmacother. 101, 599–607. doi: 10.1016/j.biopha.2018.02.091
Qin, L. F., Qin, J. M., Zhang, J. Q., Lv, X. P., Huang, L. Y., and Wang, J. J. (2018). CXCL12 and CXCR4 polymorphisms and expressions in peripheral blood from patients of hepatocellular carcinoma. Future Oncol. 14, 1261–1271. doi: 10.2217/fon-2017-0613
Quemener, C., Baud, J., Boyé, K., Dubrac, A., Billottet, C., Soulet, F., et al. (2016). Dual roles for CXCL4 chemokines and CXCR3 in angiogenesis and invasion of pancreatic cancer. Cancer Res. 76, 6507–6519. doi: 10.1158/0008-5472.can-15-2864
Ringehan, M., McKeating, J. A., and Protzer, U. (2017). Viral hepatitis and liver cancer. Philosop. Trans. R. Soc. B: Biol. Sci. 372:20160274. doi: 10.1098/rstb.2016.0274
Rosciglione, S., Thériault, C., Boily, M.-O., Paquette, M., and Lavoie, C. (2014). Gαs regulates the post-endocytic sorting of G protein-coupled receptors. Nat. Commun. 5:4556. doi: 10.1038/ncomms5556
Roussos, E. T., Condeelis, J. S., and Patsialou, A. (2011). Chemotaxis in cancer. Nat. Rev. Cancer 11, 573–587. doi: 10.1038/nrc3078
Saiman, Y., Agarwal, R., Hickman, D. A., Fausther, M., El-Shamy, A., Dranoff, J. A., et al. (2013). CXCL12 induces hepatic stellate cell contraction through a calcium-independent pathway. Am. J. Physiol.-Gastrointestinal Liver Physiol. 305, G375–G382. doi: 10.1152/ajpgi.00185.2012
Saiman, Y., Jiao, J., Fiel, M. I., Friedman, S. L., Aloman, C., and Bansal, M. B. (2015). Inhibition of the CXCL12/CXCR4 chemokine axis with AMD3100, a CXCR4 small molecule inhibitor, worsens murine hepatic injury. Hepatol. Res. 45, 794–803. doi: 10.1111/hepr.12411
Saito, Y., Shimada, M., Utsunomiya, T., Ikemoto, T., Yamada, S., Morine, Y., et al. (2014). Homing effect of adipose-derived stem cells to the injured liver: the shift of stromal cell-derived factor 1 expressions. J. Hepatobiliary Pancreat. Sci. 21, 873–880. doi: 10.1002/jhbp.147
Shen, X., Li, N., Li, H., Zhang, T., Wang, F., and Li, Q. (2010). Increased prevalence of regulatory T cells in the tumor microenvironment and its correlation with TNM stage of hepatocellular carcinoma. J. Cancer Res. Clin. Oncol. 136, 1745–1754. doi: 10.1007/s00432-010-0833-8
Shi, C., and Pamer, E. G. (2011). Monocyte recruitment during infection and inflammation. Nat. Rev. Immunol. 11, 762–774. doi: 10.1038/nri3070
Smith, J. S., and Rajagopal, S. (2016). The β-Arrestins: multifunctional regulators of G protein-coupled receptors. J. Biol. Chem. 291, 8969–8977. doi: 10.1074/jbc.R115.713313
Sung, Y.-C., Liu, Y.-C., Chao, P.-H., Chang, C.-C., Jin, P.-R., Lin, T.-T., et al. (2018). Combined delivery of sorafenib and a MEK inhibitor using CXCR4-targeted nanoparticles reduces hepatic fibrosis and prevents tumor development. Theranostics 8, 894–905. doi: 10.7150/thno.21168
Tang, Y., Lu, Y., Chen, Y., Luo, L., Cai, L., Peng, B., et al. (2019). Pre-metastatic niche triggers SDF-1/CXCR4 axis and promotes organ colonisation by hepatocellular circulating tumour cells via downregulation of Prrx1. J. Exp. Clin. Cancer Res. 38:473. doi: 10.1186/s13046-019-1475-6
Teicher, B. A., and Fricker, S. P. (2010). CXCL12 (SDF-1)/CXCR4 pathway in cancer. Clin. Cancer Res. 16, 2927–2931. doi: 10.1158/1078-0432.ccr-09-2329
Teixidó, J., Martínez-Moreno, M., Díaz-Martínez, M., and Sevilla-Movilla, S. (2018). The good and bad faces of the CXCR4 chemokine receptor. Int. J. Biochem. Cell Biol. 95, 121–131. doi: 10.1016/j.biocel.2017.12.018
Terada, R., Yamamoto, K., Hakoda, T., Shimada, N., Okano, N., Baba, N., et al. (2003). Stromal cell–derived factor-1 from biliary epithelial cells recruits CXCR4-positive cells: implications for inflammatory liver diseases. Lab. Invest. 83, 665–672. doi: 10.1097/01.lab.0000067498.89585.06
Tian, X., Xie, G., Xiao, H., Ding, F., Bao, W., and Zhang, M. (2019). CXCR4 knockdown prevents inflammatory cytokine expression in macrophages by suppressing activation of MAPK and NF-κB signaling pathways. Cell Biosci. 9:55. doi: 10.1186/s13578-019-0315-x
Toraih, E. A., Fawzy, M. S., El-Falouji, A. I., Hamed, E. O., Nemr, N. A., Hussein, M. H., et al. (2016). Stemness-related transcriptional factors and homing gene expression profiles in hepatic differentiation and cancer. Mol. Med. 22, 653–663. doi: 10.2119/molmed.2016.00096
TrehanPati, N., Sukriti, S., Geffers, R., Hissar, S., Riese, P., Toepfer, T., et al. (2011). Gene expression profiles of T cells from hepatitis E virus infected patients in acute and resolving phase. J. Clin. Immunol. 31, 498–508. doi: 10.1007/s10875-010-9506-2
Tsai, C.-N., Yu, S.-C., Lee, C.-W., Pang, J.-H. S., Wu, C.-H., Lin, S.-E., et al. (2020). SOX4 activates CXCL12 in hepatocellular carcinoma cells to modulate endothelial cell migration and angiogenesis in vivo. Oncogene 39, 4695–4710. doi: 10.1038/s41388-020-1319-z
Tsuchiya, A., Imai, M., Kamimura, H., Takamura, M., Yamagiwa, S., Sugiyama, T., et al. (2012). Increased susceptibility to severe chronic liver damage in CXCR4 conditional knock-out mice. Dig. Dis. Sci. 57, 2892–2900. doi: 10.1007/s10620-012-2239-8
Ullah, A., Wang, K., Wu, P., Oupicky, D., and Sun, M. (2019). CXCR4-targeted liposomal mediated co-delivery of pirfenidone and AMD3100 for the treatment of TGFβ-induced HSC-T6 cells activation. Int. J. Nanomed. 14, 2927–2944. doi: 10.2147/ijn.s171280
Ullah, T. R. (2019). The role of CXCR4 in multiple myeloma: cells’ journey from bone marrow to beyond. J. Bone Oncol. 17:100253. doi: 10.1016/j.jbo.2019.100253
Wald, O., Pappo, O., Safadi, R., Dagan-Berger, M., Beider, K., Wald, H., et al. (2004). Involvement of the CXCL12/CXCR4 pathway in the advanced liver disease that is associated with hepatitis C virus or hepatitis B virus. Eur. J. Immunol. 34, 1164–1174. doi: 10.1002/eji.200324441
Wang, C., Wang, M.-D., Cheng, P., Huang, H., Dong, W., Zhang, W.-W., et al. (2017). Hepatitis B virus X protein promotes the stem-like properties of OV6+ cancer cells in hepatocellular carcinoma. Cell Death Dis. 8, e2560–e2560. doi: 10.1038/cddis.2016.493
Wang, S., Wang, J. Q., and Lv, X. W. (2017). Exosomal miRNAs as biomarkers in the diagnosis of liver disease. Biomark Med. 11, 491–501. doi: 10.2217/bmm-2017-0011
Wang, X., Zhang, W., Ding, Y., Guo, X., Yuan, Y., and Li, D. (2017). CRISPR/Cas9-mediated genome engineering of CXCR4 decreases the malignancy of hepatocellular carcinoma cells in vitro and in vivo. Oncol. Rep. 37, 3565–3571. doi: 10.3892/or.2017.5601
Wang, D., Wang, X., Si, M., Yang, J., Sun, S., Wu, H., et al. (2020). Exosome-encapsulated miRNAs contribute to CXCL12/CXCR4-induced liver metastasis of colorectal cancer by enhancing M2 polarization of macrophages. Cancer Lett. 474, 36–52. doi: 10.1016/j.canlet.2020.01.005
Wang, F. S., Fan, J. G., Zhang, Z., Gao, B., and Wang, H. Y. (2014). The global burden of liver disease: the major impact of China. Hepatology 60, 2099–2108. doi: 10.1002/hep.27406
Wang, J., Huang, Y., Zhang, J., Xing, B., Xuan, W., Wang, H., et al. (2018). High co-expression of the SDF1/CXCR4 axis in hepatocarcinoma cells is regulated by AnnexinA7 in vitro and in vivo. Cell Commun. Signal. 16:22. doi: 10.1186/s12964-018-0234-1
Wang, J., and Knaut, H. (2014). Chemokine signaling in development and disease. Development 141, 4199–4205. doi: 10.1242/dev.101071
Wang, L., Li, X., Zhao, Y., Fang, C., Lian, Y., Gou, W., et al. (2015). Insights into the mechanism of CXCL12-mediated signaling in trophoblast functions and placental angiogenesis. Acta Biochim. Biophys. Sinica 47, 663–672. doi: 10.1093/abbs/gmv064
Wang, S., Gao, S., Zhou, D., Qian, X., Luan, J., and Lv, X. (2021). The role of the CD39–CD73–adenosine pathway in liver disease. J. Cell. Physiol. 236, 851–862. doi: 10.1002/jcp.29932
Wang, Y., Yu, H., Shan, Y., Tao, C., Wu, F., Yu, Z., et al. (2016). EphA1 activation promotes the homing of endothelial progenitor cells to hepatocellular carcinoma for tumor neovascularization through the SDF-1/CXCR4 signaling pathway. J. Exp. Clin. Cancer Res. 35:65. doi: 10.1186/s13046-016-0339-6
Wu, Q., Ji, F.-K., Wang, J.-H., Nan, H., and Liu, D.-L. (2015). Stromal cell-derived factor 1 promoted migration of adipose-derived stem cells to the wounded area in traumatic rats. Biochem. Biophys. Res. Commun. 467, 140–145. doi: 10.1016/j.bbrc.2015.09.097
Wu, Y., Costantino, C. M., Gupta, A., Yewdall, A. W., Dale, B. M., Devi, L. A., et al. (2012). Cannabinoid receptor 2-mediated attenuation of CXCR4-Tropic HIV infection in primary CD4+ T cells. PLoS One 7:e33961. doi: 10.1371/journal.pone.0033961
Wu, Y., Tian, L., Xu, Y., Zhang, M., Xiang, S., Zhao, J., et al. (2018). CXCR7 silencing inhibits the migration and invasion of human tumor endothelial cells derived from hepatocellular carcinoma by suppressing STAT3. Mol. Med. Rep. 18, 1644–1650. doi: 10.3892/mmr.2018.9114
Xiang, Y., Pang, B.-Y., Zhang, Y., Xie, Q.-L., Zhu, Y., Leng, A.-J., et al. (2017). Effect of Yi Guan Jian decoction on differentiation of bone marrow mesenchymalstem cells into hepatocyte-like cells in dimethylnitrosamine-induced liver cirrhosis in mice. Mol. Med. Rep. 15, 613–626. doi: 10.3892/mmr.2016.6083
Xiang, Z., Zeng, Z., Tang, Z., Fan, J., Sun, H., Wu, W., et al. (2009). Increased expression of vascular endothelial growth factor-C and nuclear CXCR4 in hepatocellular carcinoma is correlated with lymph node metastasis and poor outcome. Cancer J. 15, 519–525. doi: 10.1097/ppo.0b013e3181c6aa6b
Xiao Ling, K., Peng, L., Jian Feng, Z., Wei, C., Wei Yan, Y., Nan, S., et al. (2016). Stromal derived Factor-1/CXCR4 axis involved in bone marrow mesenchymal stem cells recruitment to injured liver. Stem Cells Int. 2016, 1–10. doi: 10.1155/2016/8906945
Xiu, G., Li, X., Yin, Y., Li, J., Li, B., Chen, X., et al. (2020). SDF-1/CXCR4 augments the therapeutic effect of bone marrow mesenchymal stem cells in the treatment of lipopolysaccharide-induced liver injury by promoting their migration through PI3K/Akt signaling pathway. Cell Transplant. 29:096368972092999. doi: 10.1177/0963689720929992
Xu, F., Na, L., Li, Y., and Chen, L. (2020). Roles of the PI3K/AKT/mTOR signalling pathways in neurodegenerative diseases and tumours. Cell Biosci. 10:54. doi: 10.1186/s13578-020-00416-0
Xu, J., Liang, J., Meng, Y.-M., Yan, J., Yu, X.-J., Liu, C.-Q., et al. (2017). Vascular CXCR4 expression promotes vessel sprouting and sensitivity to sorafenib treatment in hepatocellular carcinoma. Clin. Cancer Res. 23, 4482–4492. doi: 10.1158/1078-0432.ccr-16-2131
Xue, T.-C., Jia, Q.-A., Bu, Y., Chen, R.-X., Cui, J.-F., Tang, Z.-Y., et al. (2014). CXCR7 correlates with the differentiation of hepatocellular carcinoma and suppresses HNF4α expression through the ERK pathway. Oncol. Rep. 32, 2387–2396. doi: 10.3892/or.2014.3501
Yang, J., Lu, Y., Lin, Y.-Y., Zheng, Z.-Y., Fang, J.-H., He, S., et al. (2016). Vascular mimicry formation is promoted by paracrine TGF-β and SDF1 of cancer-associated fibroblasts and inhibited by miR-101 in hepatocellular carcinoma. Cancer Lett. 383, 18–27. doi: 10.1016/j.canlet.2016.09.012
Yang, J., Zhang, L., Jiang, Z., Ge, C., Zhao, F., Jiang, J., et al. (2019). TCF12 promotes the tumorigenesis and metastasis of hepatocellular carcinoma via upregulation of CXCR4 expression. Theranostics 9, 5810–5827. doi: 10.7150/thno.34973
Yang, N., Chen, T., Wang, L., Liu, R., Niu, Y., Sun, L., et al. (2020). CXCR4 mediates matrix stiffness-induced downregulation of UBTD1 driving hepatocellular carcinoma progression via YAP signaling pathway. Theranostics 10, 5790–5801. doi: 10.7150/thno.44789
Ye, L. Y., Chen, W., Bai, X. L., Xu, X. Y., Zhang, Q., Xia, X. F., et al. (2016). Hypoxia-induced epithelial-to-mesenchymal transition in hepatocellular carcinoma induces an immunosuppressive tumor microenvironment to promote metastasis. Cancer Res. 76, 818–830. doi: 10.1158/0008-5472.can-15-0977
Yin, Z., Dong, C., Jiang, K., Xu, Z., Li, R., Guo, K., et al. (2019). Heterogeneity of cancer-associated fibroblasts and roles in the progression, prognosis, and therapy of hepatocellular carcinoma. J. Hematol. Oncol. 12:101. doi: 10.1186/s13045-019-0782-x
Yu, Y., Liu, Y., An, W., Song, J., Zhang, Y., and Zhao, X. (2019). STING-mediated inflammation in Kupffer cells contributes to progression of nonalcoholic steatohepatitis. J. Clin. Invest. 129, 546–555. doi: 10.1172/JCI121842
Zainal, N. S., Gan, C. P., Lau, B. F., Yee, P. S., Tiong, K. H., Abdul Rahman, Z. A., et al. (2018). Zerumbone targets the CXCR4-RhoA and PI3K-mTOR signaling axis to reduce motility and proliferation of oral cancer cells. Phytomedicine 39, 33–41. doi: 10.1016/j.phymed.2017.12.011
Zhang, S., Lv, C., Yang, X., Han, Z., Zhang, S., Zhang, J., et al. (2015). Corticosterone mediates the inhibitory effect of restraint stress on the migration of mesenchymal stem cell to carbon tetrachloride-induced fibrotic liver by downregulating CXCR4/7 expression. Stem Cells Dev. 24, 587–596. doi: 10.1089/scd.2014.0243
Zhang, X., Huang, W., Chen, X., Lian, Y., Wang, J., Cai, C., et al. (2017). CXCR5-overexpressing mesenchymal stromal cells exhibit enhanced homing and can decrease contact hypersensitivity. Mol. Ther. 25, 1434–1447. doi: 10.1016/j.ymthe.2017.04.004
Zhao, Z.-W., Fan, X.-X., Song, J.-J., Xu, M., Chen, M.-J., Tu, J.-F., et al. (2017). ShRNA knock-down of CXCR7 inhibits tumour invasion and metastasis in hepatocellular carcinoma after transcatheter arterial chemoembolization. J. Cell. Mol. Med. 21, 1989–1999. doi: 10.1111/jcmm.13119
Zheng, J., Li, H., He, L., Huang, Y., Cai, J., Chen, L., et al. (2018). Preconditioning of umbilical cord-derived mesenchymal stem cells by rapamycin increases cell migration and ameliorates liver ischaemia/reperfusion injury in mice via the CXCR4/CXCL12 axis. Cell Prolif. 52:e12546. doi: 10.1111/cpr.12546
Zheng, J. C., Hong, F., Saiman, Y., Si, C., Mosoian, A., and Bansal, M. B. (2012). X4 human immunodeficiency virus Type 1 gp120 promotes human hepatic stellate cell activation and collagen i expression through interactions with CXCR4. PLoS One 7:e33659. doi: 10.1371/journal.pone.0033659
Zheng, K., Li, H.-Y., Su, X.-L., Wang, X.-Y., Tian, T., Li, F., et al. (2010). Chemokine receptor CXCR7 regulates the invasion, angiogenesis and tumor growth of human hepatocellular carcinoma cells. J. Exp. Clin. Cancer Res. 29:31. doi: 10.1186/1756-9966-29-31
Zheng, N., Liu, W., Li, B., Nie, H., Liu, J., Cheng, Y., et al. (2019). Co-delivery of sorafenib and metapristone encapsulated by CXCR4-targeted PLGA-PEG nanoparticles overcomes hepatocellular carcinoma resistance to sorafenib. J. Exp. Clin. Cancer Res. 38:232. doi: 10.1186/s13046-019-1216-x
Zhong, J., Li, J., Wei, J., Huang, D., Huo, L., Zhao, C., et al. (2019). Plumbagin restrains hepatocellular carcinoma angiogenesis by stromal cell-derived factor (SDF-1)/CXCR4-CXCR7 Axis. Med. Sci. Monit. 25, 6110–6119. doi: 10.12659/msm.915782
Zhu, M., Lu, Y., Li, W., Guo, J., Dong, X., Lin, B., et al. (2016). Hepatitis B Virus X protein driven alpha fetoprotein expression to promote malignant behaviors of normal liver cells and hepatoma cells. J. Cancer 7, 935–946. doi: 10.7150/jca.13628
Zhuo, W., Jia, L., Song, N., Lu, X. A., Ding, Y., Wang, X., et al. (2012). The CXCL12-CXCR4 chemokine pathway: a novel axis regulates lymphangiogenesis. Clin. Cancer Res. 18, 5387–5398. doi: 10.1158/1078-0432.ccr-12-0708
Zlotnik, A., Burkhardt, A. M., and Homey, B. (2011). Homeostatic chemokine receptors and organ-specific metastasis. Nat. Rev. Immunol. 11, 597–606. doi: 10.1038/nri3049
Keywords: CXCR4, CXCL12, liver specific cells, stem cells, targeted therapy, liver disease
Citation: Wang S, Gao S, Li Y, Qian X, Luan J and Lv X (2021) Emerging Importance of Chemokine Receptor CXCR4 and Its Ligand in Liver Disease. Front. Cell Dev. Biol. 9:716842. doi: 10.3389/fcell.2021.716842
Received: 29 May 2021; Accepted: 08 July 2021;
Published: 27 July 2021.
Edited by:
Claudia Tanja Mierke, Leipzig University, GermanyReviewed by:
Iain Comerford, University of Adelaide, AustraliaCopyright © 2021 Wang, Gao, Li, Qian, Luan and Lv. This is an open-access article distributed under the terms of the Creative Commons Attribution License (CC BY). The use, distribution or reproduction in other forums is permitted, provided the original author(s) and the copyright owner(s) are credited and that the original publication in this journal is cited, in accordance with accepted academic practice. No use, distribution or reproduction is permitted which does not comply with these terms.
*Correspondence: Jiajie Luan, bHVhbmppYWppZTc1N0AxNjMuY29t; Xiongwen Lv, bHh3MzEyODhAYWxpeXVuLmNvbQ==
Disclaimer: All claims expressed in this article are solely those of the authors and do not necessarily represent those of their affiliated organizations, or those of the publisher, the editors and the reviewers. Any product that may be evaluated in this article or claim that may be made by its manufacturer is not guaranteed or endorsed by the publisher.
Research integrity at Frontiers
Learn more about the work of our research integrity team to safeguard the quality of each article we publish.