- 1Department of Endocrinology, The Second Affiliated Hospital of Fujian Medical University, Quanzhou, China
- 2Department of Endocrinology, The Seventh Affiliated Hospital of Sun Yat-sen University, Shenzhen, China
- 3Centre of Neurological and Metabolic Research, The Second Affiliated Hospital of Fujian Medical University, Quanzhou, China
- 4Diabetes and Metabolism Division, Garvan Institute of Medical Research, Darlinghurst, NSW, Australia
Through the past decade of research, the pathogenic mechanisms underlying metabolic syndrome have been suggested to involve not only the peripheral tissues, but also central metabolic regulation imbalances. The hypothalamus, and the arcuate nucleus in particular, is the control center for metabolic homeostasis and energy balance. Neuropeptide Y neurons are particularly abundantly expressed in the arcuate of the hypothalamus, where the blood-brain barrier is weak, such as to critically integrate peripheral metabolic signals with the brain center. Herein, focusing on metabolic syndrome, this manuscript aims to provide an overview of the regulatory effects of Neuropeptide Y on metabolic syndrome and discuss clinical intervention strategy perspectives for neurometabolic disease.
Introduction
Metabolic syndrome (MS) is a disease characterized by obesity, dyslipidemia, high blood pressure, hyperglycemia, and insulin resistance. The increasing incidence of MS is a result of type 2 diabetes mellitus, cardiovascular disease (CVD), and other risk factors linked to early death (Spahis et al., 2017). MS is a cluster of major health risk factors associated with increased incidence of type 2 diabetes and CVD. Although the definition and criteria for MS vary, all definitions include important risk factors, such as a large circumference, elevated blood pressure, low high-density lipoprotein level, elevated levels of triglycerides, and hyperglycemia (Huang, 2009).
The pathogenic mechanisms underlying MS are complicated. In the past, these have mainly focused on peripheral tissues. However, in recent years, it has been found that central metabolic regulation imbalance may play an essential role. As the body’s metabolic regulation center, the hypothalamus receives peripheral metabolic information, integrates peripheral signals, and regulates energy homeostasis by controlling a series of neuroendocrine functions (Konner et al., 2009; Williams and Elmquist, 2012). In the arcuate (Arc) nucleus of the hypothalamus, there are two major populations of neurons that regulate energy balance. One group expresses orexigenic neuropeptides, including neuropeptide Y (NPY) and Agouti-related peptide (AgRP), while the other expresses anorexigenic proopiomelanocortin (POMC) and cocaine and amphetamine regulated transcripts (CART) (Gropp et al., 2005; Luquet et al., 2005). NPY neurons, located in the Arc of the hypothalamus where the blood-brain barrier is weak, are involved in the central regulation of metabolic homeostasis and energy balance. Therefore, they are the first-line neurons of the brain to integrate peripheral metabolic signals to regulate food intake and energy expenditure. Arc NPY neurons secrete NPY, AgRP, and the neurotransmitter GABA; as such, they are also called NPY-AgRP-GABA neurons. NPY/AgRP neurons are glucose inhibited neurons (Fioramonti et al., 2007), besides, they express insulin receptor (InsR) and leptin receptor (LepR), which can sense peripheral glucose and lipid metabolism signals. Arc NPY neurons project to other metabolic regulation-related nuclei, and thereafter through the autonomic nerve and endocrine system to centrally regulate metabolic balance. Arc NPY neuron response to peripheral metabolic alterations, increase food intake, decrease energy expenditure, and initiate ketogenesis. NPY is closely related to obesity and other metabolic diseases (Koch and Horvath, 2014). Therefore, assessing the metabolic regulation function of Arc NPY neurons and their relationship with the physiological status of metabolic diseases has clinical significance for the prevention and treatment of metabolic-related diseases.
NPY System
Neuropeptide Y was isolated from porcine hypothalamus for the first time in 1982 (Tatemoto et al., 1982). NPY is a 36-amino acid peptide and has 70% homology with peptide YY (PYY) and a 50% homology with pancreatic polypeptide (PP). As such, the three are classified into the same NPY family. NPY is an abundant peptide in the mammalian brain. In the central nervous system, NPY is widely distributed in various areas of the brain, with higher concentrations in the Arc of the hypothalamus, the paraventricular (PVN) nucleus of the hypothalamus, the supraoptic nucleus, the superior nucleus, median eminence, dorsal medial hypothalamic (DMH) nucleus, paraventricular thalamic nucleus, amygdala, hippocampus, nucleus tractus solitarius (NTS), locus coeruleus, nucleus accumbens and cerebral cortex (Morris, 1989). NPY is also expressed in the peripheral sympathetic nervous system and is stored and released together with norepinephrine (Nozdrachev and Masliukov, 2011).
Besides the liver, heart, spleen, kidney, urogenital tract, and vascular endothelial cells also express NPY (Cavadas et al., 2001; Silva et al., 2005). The distribution of NPY receptors is also widespread. NPY binds to receptors to activate specific signaling pathways and produce biological effects. To date, five NPY receptors have been successfully cloned from mammals, namely Y1, Y2, Y4, Y5, and Y6 (Pedragosa-Badia et al., 2013), which all belong to the family of G protein-coupled receptors (Cabrele and Beck-Sickinger, 2000). In the human central and peripheral nervous system, neuropeptide Y receptors are encoded by different genes and have different tissue distribution and intracellular signaling pathways, thereby indicating that the NPY system is involved in a variety of physiological processes and plays different roles in different physiological processes. Y1 receptor is critical to feeding behavior. Studies have shown that selective NPY-Y1 and NPY Y5 receptor agonists strongly promote feeding behaviors (Bo et al., 2016). Furthermore, the increased expression of Y2 and Y4 receptors will produce anorexia effects. Y2 receptor knockout model was found to have weight gain, fat deposition and hyperorexia (Naveilhan et al., 1999). Y4 receptor knockout mice displayed a thin phenotype with lower body weight (Sainsbury et al., 2002).
Interactions Pathways of NPY Neurons
Studies have documented the effect of central NPY on energy homeostasis, alongside the molecular mechanisms underlying these effects, including central and peripheral signaling pathways.
Neural Mechanism of NPY Neurons Regulating Metabolism
Central NPY neurons can integrate peripheral metabolic signals via neural pathways for regulating energy homeostasis (Figure 1).
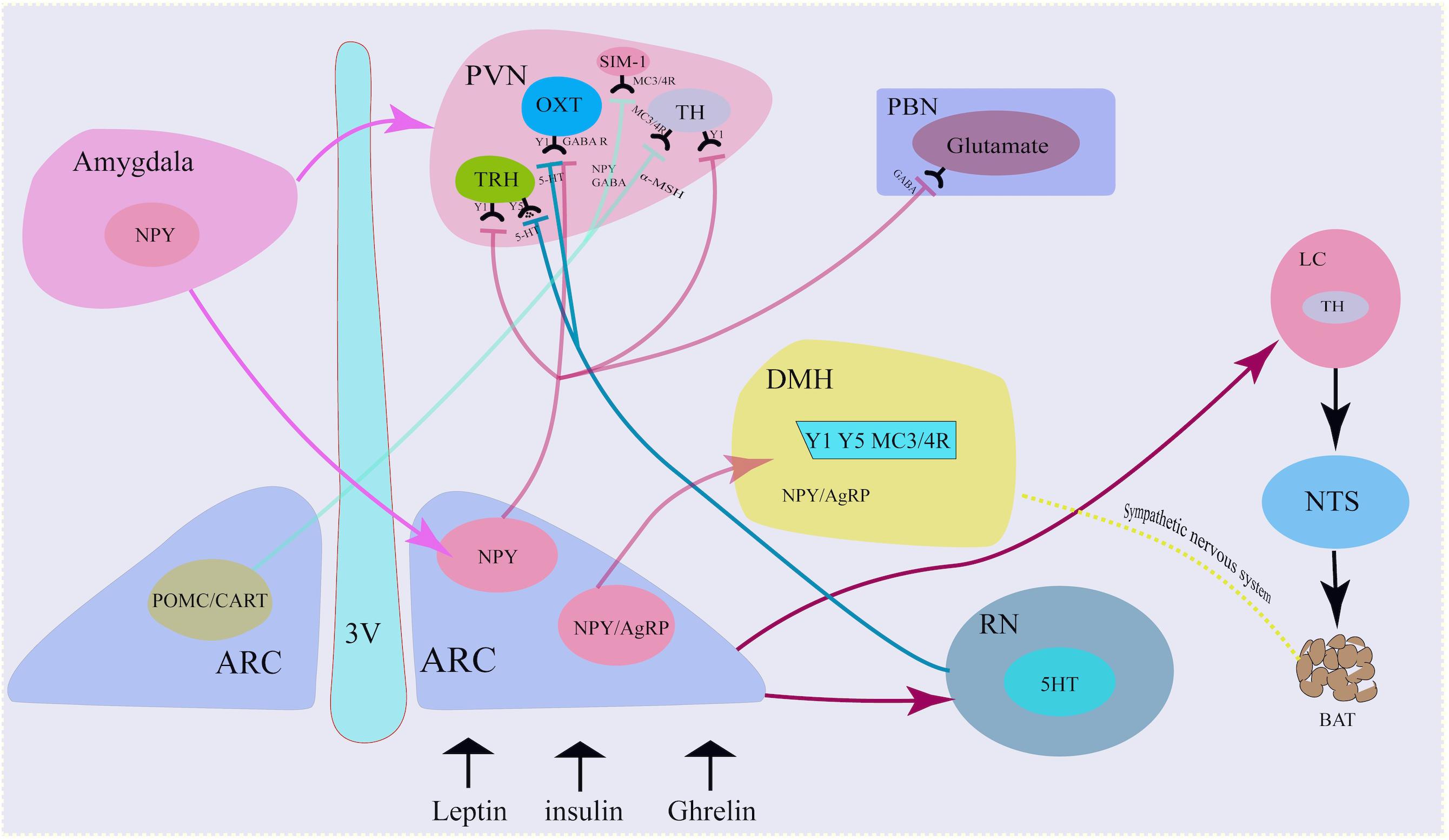
Figure 1. Neural mechanism of NPY neurons. NPY co-locate with neurotransmitters in the sympathetic nervous system. NPY in the Arc projected to the PVN via the activation of Y1 receptors on the TH neurons, TRH neurons and OXT neurons. Similarly, Arc NPY projected to DMH via Y1, Y5, and MC4R receptors. Besides hypothalamus, amygdala also secreted NPY and projected to Arc and PVN. 3 V, third ventricle; ARC, arcuate nucleus; TH, tyrosine hydroxylase; PVN, paraventricular nucleus; NTS, nucleus tractus solitarius; DMH, dorsomedial hypothalamus; LC, locus coeruleus; AgRP, agouti- related peptide; POMC, proopiomelanocortin; CART, cocaine- and amphetamine-regulated transcript; GABA, g-aminobutyric acid; a-MSH, a-melanocyte-stimulating hormone; OXT, oxytocin.
NPY and Central Sympathetic Nervous System
Neuropeptide Y mainly functions as a sympathetic co-transmitter. NPY not only co-locates in neurons with other neurotransmitters (such as norepinephrine) but is also synthesized and released by non-neuronal cells. The traditional neurotransmitter norepinephrine and non-adrenergic transmitter adenosine triphosphate are stored in a large vesicle pool with small dense core vesicles, while NPY is stored in a small vesicle pool with large dense core vesicles, co-localized with norepinephrine (Merten and Beck-Sickinger, 2006). Although multiple neurotransmitters exist in the same vesicle, their release patterns are different due to the varying doses of neurotransmitters at the ends of axons and the activation of sympathetic nerves. For example, studies have shown that although adenosine triphosphate and norepinephrine co-locate in vesicles, the doses of adenosine triphosphate and norepinephrine in each vesicle may be uneven, due to the stimulation intensity of the sympathetic nervous system (Donoso et al., 2004; Ralevic, 2009). Adenosine triphosphate and norepinephrine are mainly released under low-frequency activation of the sympathetic nerve (Todorov et al., 1994), while NPY is released in response to strong, continuous and high-frequency activation of the sympathetic nerve.
Arc NPY Neuron-PVN Neural Pathway
Neuropeptide Y neurons projected to the PVN (Hill, 2012). The PVN is adjacent to the top of the third ventricle and is an important endocrine and metabolic regulation nucleus. Damage to PVN can lead to obesity. Single-minded-1 neuron (SIM-1) in PVN highly expresses melanocortin 3/4 receptor (MC3/4R), which is activated by α-melanocyte-stimulating hormone (α-MSH) and inhibits food intake. MC4R knockout can increase appetite and increase food intake. Activating the projection of PVN to the ventromedial nucleus of the hindbrain (VMH), the vagus nerve complex and locus coeruleus (LC) can also inhibit food intake. PVN neurons secrete corticotropin-releasing factor (CRF) and oxytocin (OXT), both of which inhibit food intake. NPY neuron-PVN is a classic neural pathway that integrate metabolic signals and to convey information from the ARC to other brain areas involved in appetite regulation and energy homeostasis (Simpson et al., 2008; Morton et al., 2014).
Neuropeptide Y/AgRP neurons release NPY to PVN neurons. At the same time, POMC neurons cleave and release α-MSH, an anorexigenic peptide that binds to and activates MC3/4R. α-MSH combines with MC3/4R in PVN neuron and functions to inhibit food intake, while NPY antagonizes the effect of α-MSH and increases food intake (Waterson and Horvath, 2015).
Arcuate NPY neurons project to PVN tyrosine hydroxylase (TH)-expressing neurons, reducing Y1 receptor-mediated TH expression, thereby reducing sympathetic activity and brown adipose tissue (BAT) thermogenesis and modulating energy expenditure. Increased NPY expression in the ARC under fasting, stress, or chronic-overfeeding conditions, acting via PVN Y1 receptors, results in the inhibition of TH tonus and sympathetically innervated BAT thermogenesis by downregulating uncoupling protein 1(UCP1) expression in BAT (Shi et al., 2013).
Arcuate NPY neurons also release NPY to PVN thyrotropin-releasing hormone (TRH) positive neurons under peripheral metabolic signals, such as ghrelin, and bind to Y1/Y5 receptors, inhibit cAMP-PKA signal, reduce TRH gene transcription, and reduce thyroid hormone release. If this process is dysfunctional, this will result in the inhibition of thyroid hormone release, which will indirectly decrease glucagon secretion and impair the function of pancreatic β cells and type 1 diabetes via the autonomic nervous system (Lechan and Fekete, 2006; Vella et al., 2011).
The NPY-PVN OXT projection is involved in the initiation of food intake. NPY neurons release GABA and NPY to OXT-positive neurons in PVN, activate GABAA receptors and NPY-Y1-cAMP-PKA pathways, respectively, thereby inhibiting OXT neurons and reducing the release of oxytocin. This thereafter increases appetite, decreases basal metabolic rate and decreases bone synthesis rate (Atasoy et al., 2012).
Corticotropin-releasing factor neurons in the hypothalamic PVN initiate hypothalamic–pituitary–adrenal axis activity through the release of CRF into the portal system. The recent discovery of neurons expressing CRF receptor type 1 (CRFR1), the primary receptor for CRF, adjacent to CRF neurons within the PVN, suggests that CRF also signals within the hypothalamus to coordinate aspects of the stress response. CRFR1 neurons receive the majority of monosynaptic input from within the hypothalamus, mainly from the PVN itself. Locally, CRFR1 neurons make GABAergic synapses on parvocellular and magnocellular cells within the PVN. CRFR1 neurons resident in the PVN also make long-range glutamatergic synapses in autonomic nuclei such as the nucleus of the solitary tract (Jiang et al., 2018).
Neuropeptide Y neurons project mainly to second-order neurons in the PVN, but also to other encephalic region including the DMH, the lateral hypothalamus(LH) and the ventromedial hypothalamus(VMH) (Waterson and Horvath, 2015), which process the received metabolic information and project to multiple neurocircuits, and play an important role in maintaining energy homeostasis. Deletion of the DMH leads to a reduce of food intake and increase of energy expenditure, a hypophagic lean phenotype (Bellinger and Bernardis, 2002; Rezai-Zadeh et al., 2014). NPY is also expressed in neurons within the DMH. NPY in the DMH serve as an important neuromodulator for maintaining energy homeostasis. Knockdown of NPY expression in the DMH promoted development of brown adipocytes through the sympathetic nervous system, increased energy expenditure, reduced food intake, and thus prevented diet-induced obesity (Chao et al., 2011).
Arc NPY Neuron-Locus Coeruleus-NTS/Raphe Nucleus Projection
In response to fasting, Arc NPY neurons release NPY to the noradrenergic neurons in the locus coeruleus (LC) and PVN, and bind to the Y1 receptor to increase food intake. Arc NPY neurons can also project through the PVN-NTS, inhibit sympathetic preganglionic neurons, down-regulate brown fat UCP-1 expression, reduce body energy expenditure, and down-regulate the basal metabolic rate.
The raphe nucleus (RN) is rich in 5-hydroxytryptamine (5-HT) neurons, which can release 5-HT to NPY neurons via projection, bind to 5-HT1B receptors, and reduce NPY expression. On the other hand, the RN can also release 5-HT to PVN TRH and OXT neurons via its projections, combined with 5-HT2A receptors, and decrease the inhibitory effect of NPY on the electrical activity of PVN neurons, while promoting the release of hormones such as TRH and OXT, resulting in the inhibition of food intake and increase in the basal metabolic rate (Lechan and Fekete, 2006).
Central Amygdala NPY Neurons-Arc NPY Neuron Projection
Neuropeptide Y was not only produced in the hypothalamus, but also in extra-hypothalamic nuclei, such as the amygdala. Ip et al. found that under stress and a high-fat diet, NPY expression in both the central amygdala and Arc increased. Specific NPY overexpression in central amygdala NPY neurons project to the Arc and PVN-NPY neurons, resulting in increased food intake and decreased energy expenditure, and thus the development of obesity, which can be attenuated by ablation of the NPY in the central amygdala (Ip et al., 2019). NPY neurons in the central amygdala control feeding after stress combined with a high-fat diet by diminishing insulin signaling capacity (Ip et al., 2019).
Arc NPY Neuron-PVN-NTS Circuit
Arcuate NPY neurons receive nerve projections from the NTS, and thus integrate the gastrointestinal satiety signal from the vagus nerve to the center through the NTS to regulate feeding homeostasis. NTS’s A2 noradrenergic neurons can sense ghrelin signals, thereafter increasing the level of intracellular dopamine-β-hydroxylase. This enzyme catalyzes dopamine to demethyl norepinephrine (NE), increases neuron projections from these neurons to NPY neurons, and activates NPY P neurons, resulting in increased c-fos protein and neuron firing. This, in turn, causes an increase in food intake and a decrease in the basal metabolic rate. In addition, Arc NPY neurons release NPY to the PVN, through the PVN-NTS projection, indirectly activating medullary GABAergic neurons and NTS neurons, and ultimately inhibiting sympathetic preganglionic neurons, which increases food intake (Wu et al., 2012; Waterson and Horvath, 2015).
Molecular Mechanism of NPY Neurons in Metabolism Regulation
In recent years, the mechanisms underlying the regulation of hypothalamic NPY neurons of glucose and lipid metabolism has been gradually discovered, revealing a series of cell signal pathways and related factors (Figure 2).
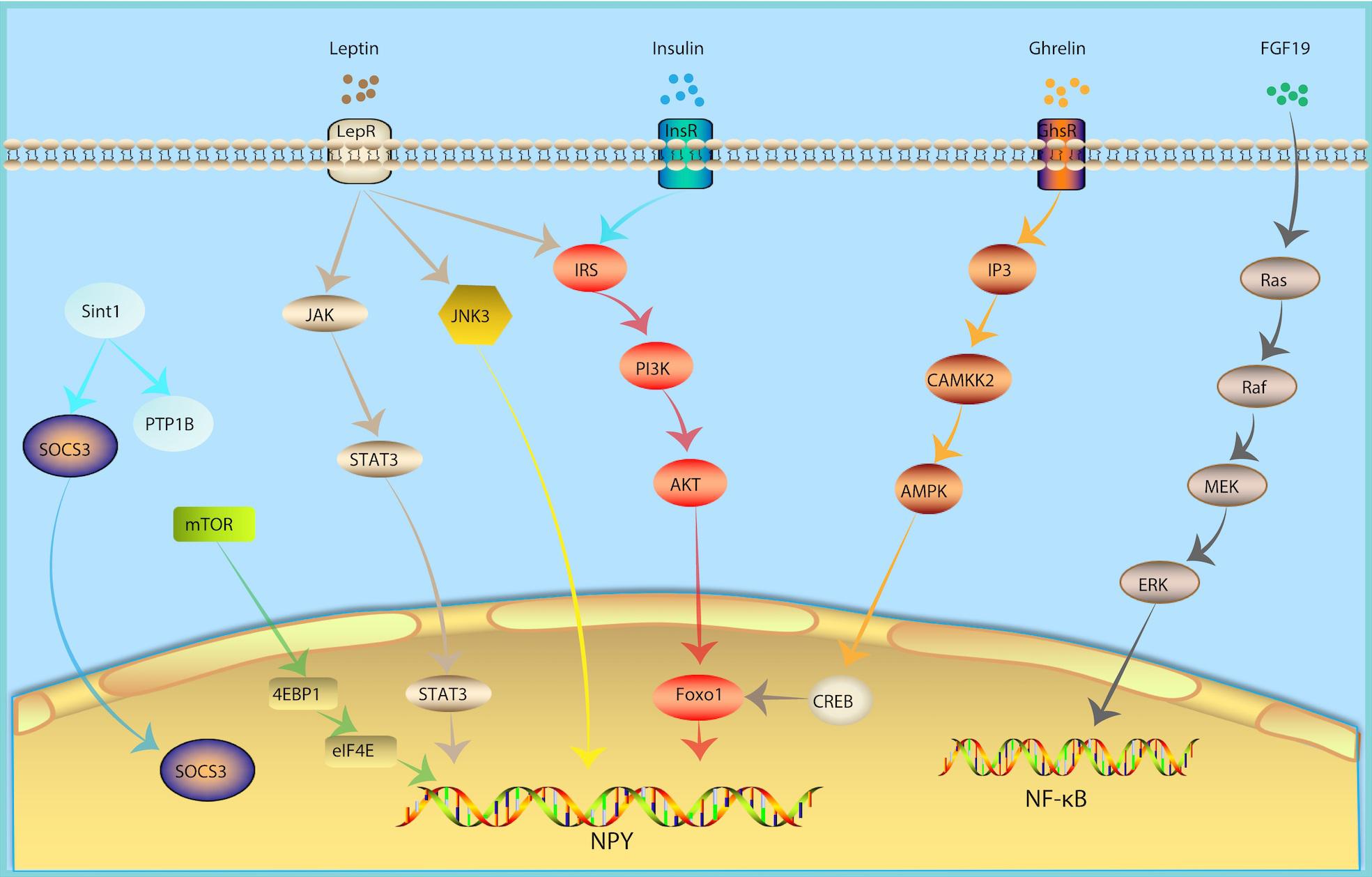
Figure 2. Molecular Mechanism of NPY Neurons in Metabolism Regulation. In response of peripheral metabolic changes, metabolic hormones including leptin, insulin and ghrelin regulated NPY signal via JAK-STAT3, PI3K-AKT-Foxo1, IP3-CAMKK2-AMPK pathway, respectively. FGF19, fibroblast growth factor-19; growth hormone secret agogue receptor (GHSR); InsR, insulin receptor; inositol triphosphate (IP3); JAK, Janus kinase; JNK, Jun NH2-terminal kinase; LepR, leptin receptor; mammalian target of rapamycin (mTOR).
Leptin Signaling
Leptin is one of the metabolic hormones, which is correlated to body fat mass and energy balance (Woods and Seeley, 2000). In response to insufficient energy, levels of leptin are low. In response to excess energy, leptin secretion increases. A decrease in the plasma levels of leptin will lead to an increase in food intake (Weigle et al., 1997). Subsequently, the decline in leptin levels weakens the activation of POMC neurons and activates the NPY signal (Varela and Horvath, 2012b), consequently stimulating feeding behavior. The effects of leptin are mediated by LepR. LepR is expressed in multiple regions of the central nervous system, especially in NPY and POMC neurons in the Arc of hypothalamus (Cowley et al., 2001; Konner et al., 2007; Lin et al., 2010), which can integrate peripheral glucose and lipid metabolism signals. Arc NPY neurons are first-line neurons that sense leptin. NPY neuron-specific LepR knockout mice develop obesity and diabetes (Zhang et al., 2020), which is similar to that of db/db (LepR deficient mice) mice. POMC neurons are also first-line neurons that sense leptin. However, POMC neuron-specific LepR knockout mice do not have this same phenotype (Xu et al., 2018). Leptin knockout can relieve leptin’s inhibition of NPY neurons and cause obesity (Elias et al., 1999) and inhibit NPY neurons by chemogenetic tools which can improve food intake and blood glucose levels in diabetic mice (Zhang et al., 2019). The above evidence indicates that NPY neurons may be the main target of leptin. Leptin binding to the extracellular region of LepR activates Janus kinase (JAK). JAK binds to LepR and is phosphorylated, thereby activating STAT3. Once phosphorylated, STAT3 binds to the promoters of POMC and NPY to increase the expression of POMC and inhibit the expression of NPY (Vaisse et al., 1996; Mesaros et al., 2008; Ernst et al., 2009).
Insulin Signaling
Insulin is an important metabolic hormone for maintaining stable blood glucose and energy homeostasis. Insulin receptors were not only found in peripheral tissues, but also in the central nervous system, especially in NPY and POMC neurons of the Arc of hypothalamus (Cowley et al., 2001; Konner et al., 2007; Lin et al., 2010), which can integrate peripheral glucose and lipid metabolism signals. The insulin signaling pathways converge on PI3K. PI3K activates Akt. The activation of Akt leads to the phosphorylation and inactivation of the POMC expression inhibitor FoxO1, promoting the binding of STAT3 to the POMC promoter, and thereafter up-regulating the expression of POMC and downregulating the expression of NPY and AgRP (Myers and Olson, 2014). Eventually, this reduces food intake and increases insulin sensitivity.
Ghrelin Signaling
Ghrelin can activate the growth hormone secret agogue receptor (GHSR) in hypothalamic Arc NPY neurons, increase the level of inositol triphosphate (IP3) and promote the release of calcium from the endoplasmic reticulum. Calcium and calmodulin together activate CAMKK2, which phosphorylates AMPK’s alpha subunit N-terminal 172 threonine. Next, AMPK further phosphorylates ACC, decreases ACC activity, and reverse the ACC’s inhibitory effect on CPT1 and increases the expression of mitochondrial uncoupling protein 2 (UCP-2). Intracellular CREB is also phosphorylated, and pCREB and FOXO1 can up-regulate NPY/AgRP transcription, ultimately increasing peripheral fatty acid oxidation (Velasco et al., 2016).
NF-κB Signaling
The hypothalamus has the highest density of pro-inflammatory cytokine receptors (Hopkins and Rothwell, 1995). This inflammatory response in the hypothalamic may be the result of plasma cytokines entering the brain, given that some cytokines can cross the blood-brain barrier, including TNFα, IL-6, IL-1α, and IL-1β (Banks and Kastin, 1991; Gutierrez et al., 1993; Banks et al., 1994; Plotkin et al., 1996). These inflammatory cytokines play a role in the pathogenesis of anorexia (Moldawer et al., 1988; Schobitz et al., 1995). A high-fat diet can induce hypothalamus inflammation and damage the neural circuits that control feeding behaviors and energy homeostasis in pregnant and lactating mice, alongside affecting the Arc neurons formation in offspring, and an increase in NPY neurons (Lemes et al., 2018). PI3K, pAkt, and NF-κB levels were negatively correlated with the expression of NPY. Intracerebroventricular infusion of the PI3K inhibitor or NF-κB antisense could attenuate anorexia and reverse the expression of NPY (Hsieh et al., 2014). In addition, the activation of the IKKβ/NF-κB inflammatory pathway plays an important role in the process of POMC neurons loss, and POMC neurons play an important role in preventing obesity (Li et al., 2012; Thaler et al., 2012). Hypothalamic inflammation affects both NPY and POMC and promotes obesity.
mTOR Signaling
Mammalian target of rapamycin (mTOR) is a serine-theonine kinase as a sensor of energy balance, nutrients and oxygen (Roa and Tena-Sempere, 2010). mTOR is expressed in AgRP/NPY neurons in the ARC (Cota et al., 2006). Studies showed that hypothalmamic mTOR signaling plays a role in modulating energy balance responding to nutrient and hommone (Cota et al., 2006). Central administration of hormones, including ghrelin, leptin, thyroid hormone et al., induce phosphorylation of mTOR and activate its downstream targets, serine/threonine ribosomal protein S6 kinase (S6K1) and a ribosomal protein involved in translation S6 in the ARC, thus increase the expression of AgRP and NPY levels, increased food intake and increase body energy expenditure (Cota et al., 2008; Martins et al., 2012; Varela et al., 2012).
c-Jun NH2-Terminal Kinase 3
c-Jun NH2-terminal kinase (JNK) is a member of the MAPK family and an important metabolic stress signaling factor. JNK1, JNK2 and their isomers are widely expressed in various tissues and participate in the regulation of energy balance and insulin resistance. In contrast, JNK3 is expressed in neurons. JNK3 knockout mice showed a continuous high intake of high-fat diets, while they maintained normal food intake habits for normal chow. Hypothalamic LepR-positive NPY neuron-specific JNK3 knockout is a key factor leading to high-fat diet behavior, while LepR-positive POMC neuron-specific JNK3 knockout mice show no such feeding effect. In response to a high-fat diet, the absence of JNK3 signal in Arc NPY neurons will increase the electrical excitability of neurons, which increases food intake and causes obesity, suggesting that the Arc NPY neuron JNK3 signaling pathway plays an important role in maintaining metabolic homeostasis under conditions of metabolic stress such as that of a high-fat diet (Vernia et al., 2016).
Fibroblast Growth Factor 19
Fibroblast growth factor-19 (FGF19) is a hormone-like enterokine which can reduce the blood glucose of insulin resistance model mice (ob/ob mice and high-fat diet mice) and enhance insulin sensitivity. FGF19 can activate FGF receptors in Arc NPY neurons, which in turn activate the Ras-Raf-MEK-ERK1/2 signaling pathway and increases ERK1/2 phosphorylation. In contrast, inhibiting ERK1/2 phosphorylation can reduce blood glucose. The intraventricular injection of FGF19 can increase phosphorylated AKT levels in the liver, quadriceps, and white adipose tissue, suggesting that FGF19 can enhance insulin sensitivity, indicating that it may represent a potential drug for the treatment of type 2 diabetes (Marcelin et al., 2014).
NPY and Metabolic Syndrome
Energy Imbalance
The hypothalamus senses and integrates various signals from the blood and the third ventricle and regulates food intake (Kohno and Yada, 2012; Schneeberger et al., 2014). The arcuate nucleus is located in the ventromedial part of the hypothalamus, close to the median bulge which receives a rich blood supply. Therefore, information from peripheral tissues can easily enter the Arc region. In addition, they also receive the input of neural signals from multiple parts of the central nervous system (Dorr et al., 2015a; Sohn, 2015). The NPY system is a core component of the energy steady-state regulation system. NPY/AgRP neurons and POMC neurons, a group of antagonistic neurons, not only regulate the feeding process, but also participate in the subsequent coordination of the conversion, storage and utilization of carbohydrates and lipids (Varela and Horvath, 2012a). As such, NPY/AgRP neurons are seen as an important bridge for the center to participate in the distribution of peripheral nutrients. NPY/AgRP signaling in adult mice is very important for maintaining normal lipid and glucose homeostasis in peripheral tissues (such as liver, muscle, and pancreas). Depletion of NPY/AgRP neurons in newborn mice will affect the balance of lipid and carbohydrate metabolism. Mice lacking NPY neurons develop obesity and hyperinsulinemia in response to a normal chow diet, but exhibit reduced weight gain and abnormal improvement in glucose tolerance under a high-fat diet (Joly-Amado et al., 2012; Vahatalo et al., 2015), pointing to the role of NPY/AgRP neurons in coordinating nutrient distribution.
Although it is known that AgRP co-localize with NPY neurons in the Arc and AgRP neurons express NPY, there remains a significant sub-population of Arc NPY neurons that do not co-express AgRP (Luquet et al., 2005). A specific deletion of InsRs in AgRP neurons was not found to result in the same metabolic phenotype as NPY specific deletion (Konner et al., 2007; Loh et al., 2017), suggesting that non-AgRP expressing NPY neurons play a more important role in the regulation of energy homeostasis. The difference between non-AgRP NPY neurons and AgRP/NPY co-expression neurons has not yet been completely elucidated. Studies suggest that non-AgRP NPY neurons may be more critical in mediating insulin’s effects on food intake, energy expenditure, and peripheral insulin release, while AgRP/NPY co-expression neurons may be more essential in regulating hepatic glucose production (Sainsbury et al., 1997; Konner et al., 2007; Loh et al., 2017).
Glucose Intolerance
Insulin plays a key role in the short-term and long-term control of blood glucose and energy homeostasis (Saltiel and Kahn, 2001; Plum et al., 2006; Loh et al., 2017). InsRs are also expressed in the hypothalamic nucleus. In POMC/CART neurons, insulin is considered to promote satiety, while in NPY/AgRP neurons, insulin signaling mainly inhibits food intake and increases energy expenditure. Studies have shown that the acute increase in insulin levels by direct administration can reduce the level of NPY in the arcuate nucleus of the hypothalamus (Schwartz et al., 1992; Benoit et al., 2002). In rodent models with an insulin deficiency, such as streptozotocin-induced diabetic rats, the levels of NPY in the hypothalamus were significantly increased, confirming the inhibitory effect of peripheral insulin on central NPY (Gelling et al., 2006). Interestingly, under obesity and insulin resistance, a long-term increase in insulin levels can also lead to an increase in hypothalamic NPY levels, indicating that hypothalamic NPY is resistant to the feedback regulation of insulin elevation. By inhibiting the hypothalamic NPY signaling pathway, insulin secretion will be increased (Konner and Bruning, 2012; Loh et al., 2015), thus participating in the pathogenesis of type 2 diabetes.
Hypertension
In previous studies, it has been proved that NPY is related to various cardiovascular diseases, such as myocardial infarction, heart failure, and cardiac hypertrophy (Thulin and Erlinge, 1995; McDermott and Bell, 2007; Herring et al., 2019). NPY is the most abundant neuropeptide found in the heart, and was the first neuropeptide found in the heart identified in intramural sympathetic nerves closely related to coronary vessels. NPY is a strong vasoconstrictor. It was found that NPY has a positive time-changing and inotropic effect on the heart (Maisel et al., 1989; Millar et al., 1991). In peripheral cardiovascular tissues, NPY is often released together with norepinephrine, especially when sympathetic nerve activity increases. In addition, in the plasma of patients with hypertension, the level of NPY is elevated (Morris et al., 1997; Dorr et al., 2015b). In rats, chronic stress can double plasma NPY levels, increase blood pressure and promote the infiltration of macrophages in the intima, further leading to carotid artery occlusion (Li et al., 2005; Sun et al., 2017). Various lines of evidence indicate that NPY is involved in the pathological process of hypertension. In humans and animal models of hypertension, peripheral and central neuropeptide Y is related to the pathological mechanisms underlying hypertension (Ruohonen et al., 2009; Tan et al., 2018; Liu et al., 2020).
Atherosclerosis
In the cardiovascular system, NPY is expressed in coronary arteries, endothelial cells and cardiomyocytes innervated by sympathetic nerves. Endothelial cells are the barrier between the blood vessel wall and the blood. Endothelial dysfunction can lead to the proliferation and angiogenesis of lipids, inflammatory cells, blood clotting substances, and vascular smooth muscle cells, alongside promoting atherosclerotic plaques. Studies have shown that NPY-Y1, Y2, and Y5 receptors are expressed on endothelial cells, which are involved in the formation of atherosclerotic plagues. Under pathological conditions, NPY induces vasoconstriction and stimulates vascular smooth muscle cell proliferation and hypertrophy via the Y1R. Foam cells and chronic inflammation are critical in atherosclerosis. Studies have shown that the expression of NPY in atherosclerotic plaques significantly increases, and a large number of macrophages aggregate into foam cells and participate in the formation of atherosclerotic plaques via NPY-Y1, Y2 and Y5 receptors. In the process of atherosclerosis, the pathophysiological changes of vascular smooth muscle cells are extremely important. NPY can increase the levels of calcium in the cytoplasm of vascular smooth muscle cells, activate protein kinase C and promote mitosis, thereby leading to atherosclerosis. Hence, the down-regulation of NPY and its receptors can induce anti-atherosclerotic effects. Wu WQ et al. found that physical exercise increased plague collagen and smooth muscle cell content and decreased plaque lipid and macrophage content in apolipoprotein E-deficient mice by decreasing the expression of NPY and NPY Y1 receptors in vasculature, suggesting that the down-regulation of NPY and NPY receptors expression in the aorta is critical to the anti-atherosclerotic effect (Wu et al., 2019; Figure 3).
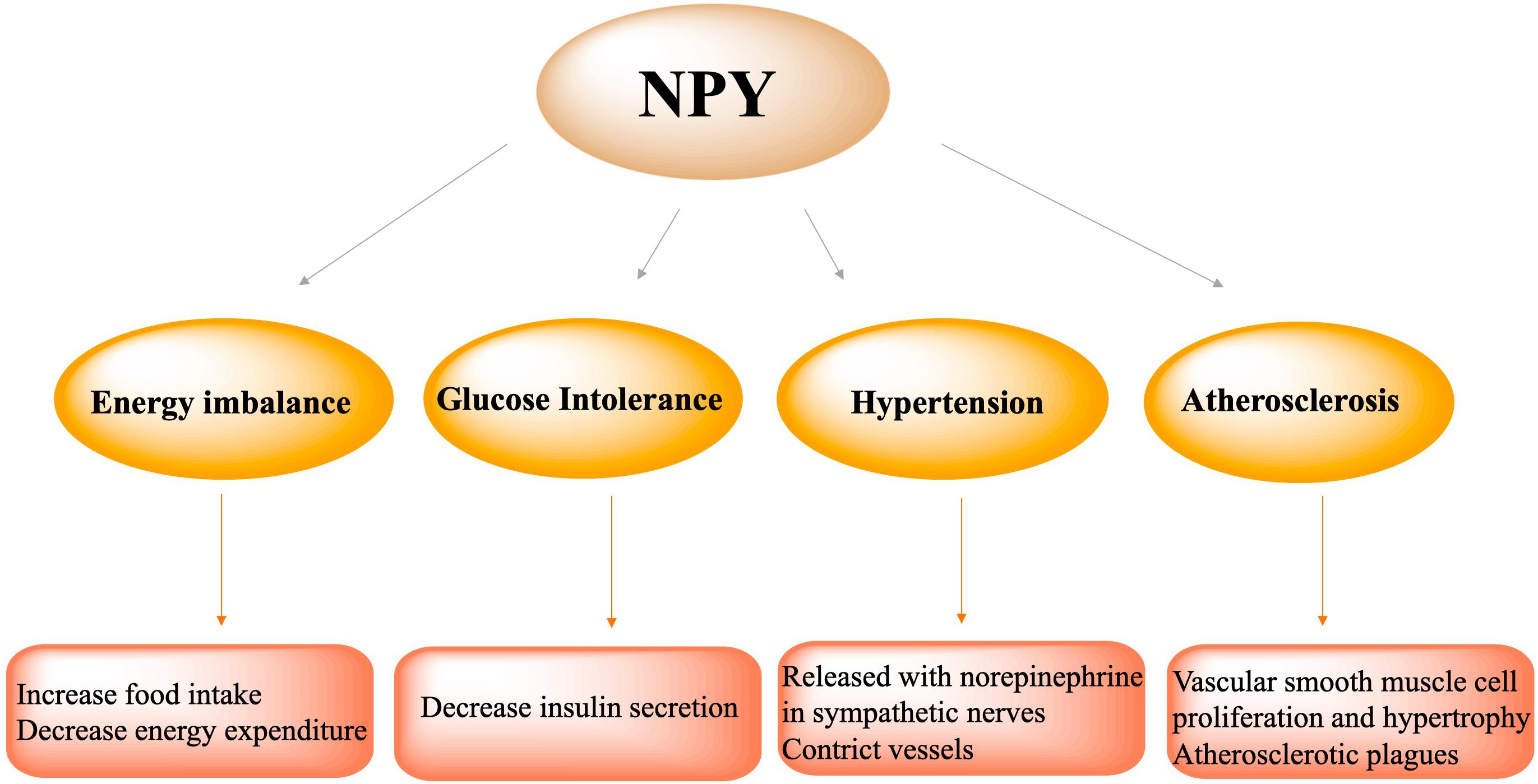
Figure 3. NPY participated in the pathophysiological process of metabolic syndrome, including energy imbalance via increasing food intake and decreasing energy expenditure, glucose intolerance via decreasing insulin secretion, hypertension via NPY release with norepinephrine in sympathetic nerves and vasoconstriction, atherosclerosis via vascular smooth muscle cell proliferation and hypertrophy and formation of atherosclerotic plagues.
Clinical Therapeutic Intervention Strategy
Neuropeptide Y neurons play critical role in metabolic diseases, including obesity, glucose tolerance, hypertension and atherosclerosis. Inhibiting NPY neuronal activity and antagonizing NPY expression may be important potential methods to regulate metabolism. Currently, intervention strategies targeting NPY may include NPY receptor antagonists, anorexigenic hormones (such as leptin) analogs, and NPY gene transcription inhibitors (Figure 4).
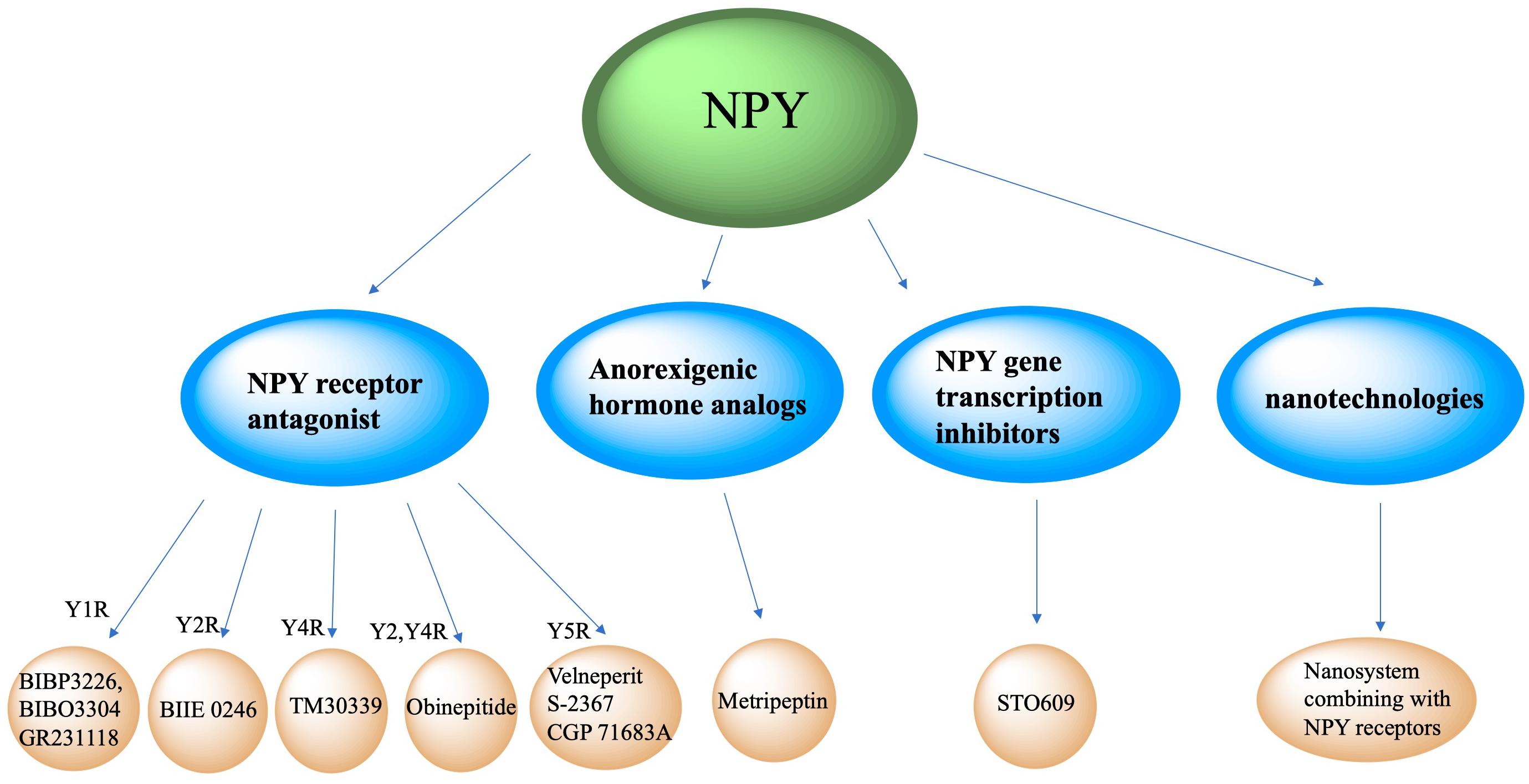
Figure 4. Clinical therapeutic intervention strategy of NPY. Intervention strategies targeting NPY include NPY receptor antagonists, anorexigenic hormones analogs, NPY gene transcription inhibitors and new technologies like nanosystem.
NPY Receptor Antagonist
Neuropeptide Y neurons secrete NPY, which interact with POMC neurons, parabrachial nucleus and paraventricular nucleus neurons, and binds to its Y1-Y5 receptors to increase appetite. Antagonizing NPY receptors and blocking the NPY signaling pathway can attenuate obesity. The Y1 receptor is a 36-amino acid, and its binding affinity to NPY and PYY is much higher than that of PP. A recent published paper mentioned about specific target NPY Y1 receptor antagonism in improving insulin resistance and protects against diet induced obesity (Yan et al., 2021). Selective Y1 receptor antagonists include BIBP3226, BIBO3304 and GR231118. The Y2 receptor consists of 381 amino acids and binds NPY and PYY with a higher affinity than PP, has alongside having a high affinity for C-terminal fragments (Lafferty et al., 2020). BIIE 0246 is a selective antagonist of the Y2 receptor (Ueda et al., 2021). The Y4 receptor consists of 375 amino acids, and has a higher affinity for PP than PYY and NPY (Sun et al., 2017). TM30339, a pancreatic peptide analog, selectively antagonizes the Y4 receptor of NPY. Obinepitide is an analog of pancreatic peptide and peptide tyrosine (PYY3-36) which can selectively antagonize the Y2 and Y4 receptors of NPY, and thus avoids cardiovascular side effects caused by antagonizing the Y1 receptor. The Y5 receptor is a protein consisting of 445 amino acids. NPY, PYY, and PP can all be recognized and bound as ligands. Velneperit S-2367 and CGP 71683A are Y5 receptor selective antagonists. Selectively stimulating or blocking NPY receptors has therapeutic potential in the treatment of obesity and metabolic disorders (Lin et al., 2006).
Anorexigenic Hormone Analogs
Neuropeptide Y neurons express anorexigenic hormone (such as leptin) receptors. When the receptors are activated, the expression of NPY is down-regulated to decrease appetite and food intake. Metripeptin, a leptin analog, can specifically bind to leptin receptors and activate the leptin signaling pathway of NPY neurons, which can significantly reduce appetite and promote fat metabolism (Baykal et al., 2020). The drug was approved for marketing by the US FDA in 2014.
NPY Gene Transcription Inhibitors
This class of drugs inhibit NPY gene transcription in NPY neurons. STO609 can significantly inhibit the intracellular CaMKK2 activity of NPY neurons and reduce the phosphorylation of ACC by CaMKK2, expression of mitochondrial UCP-2, and p-CREB levels, thereby decreasing NPY transcription, decreasing appetite, and increasing basal metabolic rate (Anderson et al., 2008).
New Clinical Therapeutic Intervention Prospect Involving Nanosystem
New technologies, such as nanotechnologies, may also be applied to NPY-related clinical therapeutic intervention strategies. Jiang ZQ et al. conjugated NPY Y1 receptor ligand (Asn6, Pro34)-NPY on the surface of doxorubicin-encapsulated nano Zeolitic imidazole frameworks-90, resulting in a more effective and faster targeted delivery and dual responsive release of DOX, significantly improving therapeutic efficacy in tumors and survival rates (Jiang et al., 2019). Another study created Y1 receptor ligand (NPY)-modified bubbles, finding that, compared with modified bubbles without NPY targeting, NPY-modified bubbles group showed a high tumor suppression effect and a prolonged survival time in Y1 receptor-overexpressed breast cancer treatments (Qian et al., 2020). Although nanosystems combining with NPY receptors are currently focused on tumor treatments, we believe nanotechnologies combined with NPY receptor ligands may play a role in neuroendocrine disease treatment.
Conclusion
The universality and diversity of the distribution of NPY and its receptors enable the NPY system to participate in the regulation of multiple physiological processes, especially in the regulation of energy metabolism balance, alongside integrating information with peripheral hormones such as insulin, leptin, and the sympathetic nervous system. A number of complex regulatory circuits are used to precisely regulate energy metabolism. When the balance is disrupted, or the hypothalamus is affected by inflammatory factors, metabolic disorders will emerge, alongside manifestations of abnormal lipid metabolism, in the form of obesity, dyslipidemia and abnormal glucose metabolism such as high blood glucose and insulin resistance development. The NPY system plays an important role in the pathological mechanisms underlying MS, in which multiple receptors and metabolic pathways can be used as potential targets for drug development. Therefore, further research is warranted to thoroughly clarify the processes and interactions of various types of regulatory mechanisms in the NPY system.
Therefore, NPY and its receptors provide a significant prospect for the clinical intervention strategy of MS and drug development in the future. The intervention targeting NPY receptor with pharmaceuticals showed benefits to metabolic syndrome, however, the strategy is non-specific, and will cause other non-specific side effects, which should be paid attention to.
Therefore, it is necessary to select more precise and individualized intervention methods, precise treatment, and individualized treatment; specifically determine the indications of each strategy. Besides, together with the pharmaceuticals treatment of MS, exercice can reduce NPY and improve NPY-related target treatments, suggesting that encouraging patients to do exercise is quite important for treatment too.
Besides, nanosystems combined with NPY receptor ligands or new molecular biology therapy, which can achieve more precise targeted therapy might represent a potential method in the context of neuroendocrine disease treatment.
Author Contributions
YH contributed in the literature search and manuscript writing. XL made the figures and the manuscript writing. SL contributed in the study design and manuscript writing. All the authors read and approved the final manuscript.
Funding
The authors thank the support from Postdoc Fund of the Second Affiliated Hospital of Fujian Medical University (2020BSH02) and Miaopu Fund of the Second Affiliated Hospital of Fujian Medical University (2012MP45). Science and Technology Bureau of Quanzhou (2002CT003), Natural Science Foundation of Fujian Province (2020J01221) and the Key Young Talents Health Training Project of Fujian Province (2020GGA057).
Conflict of Interest
The authors declare that the research was conducted in the absence of any commercial or financial relationships that could be construed as a potential conflict of interest.
Glossary
Arc, arcuate nucleus; BAT, brown adipose tissue; BDNF, brain-derived neurotrophic factor; CVD, cardiovascular disease; CART, cocaine and amphetamine regulated transcripts; CRF, corticotropin-releasing factor; CRFR1, corticotropin-releasing factor receptor type 1; DMH, dorsal medial hypothalamic; FGF19, fibroblast growth factor-19; Gpr17, G protein-coupled receptor 17; 5-HT, 5-hydroxytryptamine; InsR, insulin receptor; JAK, Janus kinase; JNK, Jun NH2-terminal kinase; LepR, leptin receptor; LC, locus coeruleus; NE, norepinephrine; MS, metabolic syndrome; MC3/4R, melanocortin 3/4 receptor; α-MSH, α-melanocyte-stimulating hormone; NPY, neuropeptide Y; NTS, nucleus tractus solitarius; OXT, oxytocin; POMC, proopiomelanocortin; PYY, peptide YY; PP, pancreatic polypeptide; PVN, paraventricular; POA, preoptic area; RN, raphe nucleus; SIM-1, single-minded-1 neuron; TH, tyrosine hydroxylase; TRH, thyrotropin-releasing hormone; TrkB, tropomyosin receptor kinase B; UCP1, uncoupling protein 1; VMH, ventromedial nucleus of the hindbrain.
References
Anderson, K. A., Ribar, T. J., Lin, F., Noeldner, P. K., Green, M. F., Muehlbauer, M. J., et al. (2008). Hypothalamic CaMKK2 contributes to the regulation of energy balance. Cell Metab. 7, 377–388. doi: 10.1016/j.cmet.2008.02.011
Atasoy, D., Betley, J. N., Su, H. H., and Sternson, S. M. (2012). Deconstruction of a neural circuit for hunger. Nature 488, 172–177. doi: 10.1038/nature11270
Banks, W. A., and Kastin, A. J. (1991). Blood to brain transport of interleukin links the immune and central nervous systems. Life Sci. 48, L117–L121.
Banks, W. A., Kastin, A. J., and Gutierrez, E. G. (1994). Penetration of interleukin-6 across the murine blood-brain barrier. Neurosci. Lett. 179, 53–56. doi: 10.1016/0304-3940(94)90933-4
Baykal, A. P., Parks, E. J., Shamburek, R., Syed-Abdul, M. M., Chacko, S., Cochran, E., et al. (2020). Leptin decreases de novo lipogenesis in patients with lipodystrophy. JCI Insight 5:e137180.
Bellinger, L. L., and Bernardis, L. L. (2002). The dorsomedial hypothalamic nucleus and its role in ingestive behavior and body weight regulation: lessons learned from lesioning studies. Physiol. Behav. 76, 431–442. doi: 10.1016/s0031-9384(02)00756-4
Benoit, S. C., Air, E. L., Coolen, L. M., Strauss, R., Jackman, A., Clegg, D. J., et al. (2002). The catabolic action of insulin in the brain is mediated by melanocortins. J. Neurosci. 22, 9048–9052. doi: 10.1523/jneurosci.22-20-09048.2002
Bo, E., Farinetti, A., Marraudino, M., Sterchele, D., Eva, C., Gotti, S., et al. (2016). Adult exposure to tributyltin affects hypothalamic neuropeptide Y, Y1 receptor distribution, and circulating leptin in mice. Andrology 4, 723–734. doi: 10.1111/andr.12222
Cabrele, C., and Beck-Sickinger, A. G. (2000). Molecular characterization of the ligand-receptor interaction of the neuropeptide Y family. J. Pept. Sci. 6, 97–122. doi: 10.1002/(sici)1099-1387(200003)6:3<97::aid-psc236>3.0.co;2-e
Cavadas, C., Silva, A. P., Mosimann, F., Cotrim, M. D., Ribeiro, C. A., Brunner, H. R., et al. (2001). NPY regulates catecholamine secretion from human adrenal chromaffin cells. J. Clin. Endocrinol. Metab. 86, 5956–5963. doi: 10.1210/jcem.86.12.8091
Chao, P. T., Yang, L., Aja, S., Moran, T. H., and Bi, S. (2011). Knockdown of NPY expression in the dorsomedial hypothalamus promotes development of brown adipocytes and prevents diet-induced obesity. Cell Metab. 13, 573–583. doi: 10.1016/j.cmet.2011.02.019
Cota, D., Matter, E. K., Woods, S. C., and Seeley, R. J. (2008). The role of hypothalamic mammalian target of rapamycin complex 1 signaling in diet-induced obesity. J. Neurosci. 28, 7202–7208. doi: 10.1523/jneurosci.1389-08.2008
Cota, D., Proulx, K., Smith, K. A., Kozma, S. C., Thomas, G., Woods, S. C., et al. (2006). Hypothalamic mTOR signaling regulates food intake. Science 312, 927–930. doi: 10.1126/science.1124147
Cowley, M. A., Smart, J. L., Rubinstein, M., Cerdan, M. G., Diano, S., Horvath, T. L., et al. (2001). Leptin activates anorexigenic POMC neurons through a neural network in the arcuate nucleus. Nature 411, 480–484. doi: 10.1038/35078085
Donoso, M. V., Miranda, R., Irarrazaval, M. J., and Huidobro-Toro, J. P. (2004). Neuropeptide Y is released from human mammary and radial vascular biopsies and is a functional modulator of sympathetic cotransmission. J. Vasc. Res. 41, 387–399. doi: 10.1159/000080900
Dorr, O., Ewen, S., Liebetrau, C., Mollmann, H., Gaede, L., Linz, D., et al. (2015a). Neuropeptide Y as an indicator of successful alterations in sympathetic nervous activity after renal sympathetic denervation. Clin. Res. Cardiol. 104, 1064–1071. doi: 10.1007/s00392-015-0874-8
Dorr, O., Liebetrau, C., Mollmann, H., Mahfoud, F., Ewen, S., Gaede, L., et al. (2015b). Beneficial effects of renal sympathetic denervation on cardiovascular inflammation and remodeling in essential hypertension. Clin. Res. Cardiol. 104, 175–184. doi: 10.1007/s00392-014-0773-4
Elias, C. F., Aschkenasi, C., Lee, C., Kelly, J., Ahima, R. S., Bjorbaek, C., et al. (1999). Leptin differentially regulates NPY and POMC neurons projecting to the lateral hypothalamic area. Neuron 23, 775–786. doi: 10.1016/s0896-6273(01)80035-0
Ernst, M. B., Wunderlich, C. M., Hess, S., Paehler, M., Mesaros, A., Koralov, S. B., et al. (2009). Enhanced Stat3 activation in POMC neurons provokes negative feedback inhibition of leptin and insulin signaling in obesity. J. Neurosci. 29, 11582–11593. doi: 10.1523/jneurosci.5712-08.2009
Fioramonti, X., Contie, S., Song, Z., Routh, V. H., Lorsignol, A., and Penicaud, L. (2007). Characterization of glucosensing neuron subpopulations in the arcuate nucleus: integration in neuropeptide Y and pro-opio melanocortin networks? Diabetes 56, 1219–1227. doi: 10.2337/db06-0567
Gelling, R. W., Morton, G. J., Morrison, C. D., Niswender, K. D., Myers, M. G. Jr., Rhodes, C. J., et al. (2006). Insulin action in the brain contributes to glucose lowering during insulin treatment of diabetes. Cell Metab. 3, 67–73. doi: 10.1016/j.cmet.2005.11.013
Gropp, E., Shanabrough, M., Borok, E., Xu, A. W., Janoschek, R., Buch, T., et al. (2005). Agouti-related peptide-expressing neurons are mandatory for feeding. Nat. Neurosci. 8, 1289–1291. doi: 10.1038/nn1548
Gutierrez, E. G., Banks, W. A., and Kastin, A. J. (1993). Murine tumor necrosis factor alpha is transported from blood to brain in the mouse. J. Neuroimmunol. 47, 169–176. doi: 10.1016/0165-5728(93)90027-v
Herring, N., Tapoulal, N., Kalla, M., Ye, X., Borysova, L., Lee, R., et al. (2019). Neuropeptide-Y causes coronary microvascular constriction and is associated with reduced ejection fraction following ST-elevation myocardial infarction. Eur. Heart J. 40, 1920–1929. doi: 10.1093/eurheartj/ehz115
Hill, J. W. (2012). PVN pathways controlling energy homeostasis. Indian J. Endocrinol. Metab. 16, S627–S636.
Hopkins, S. J., and Rothwell, N. J. (1995). Cytokines and the nervous system. I: expression and recognition. Trends Neurosci. 18, 83–88. doi: 10.1016/0166-2236(95)80029-2
Hsieh, Y. S., Chen, P. N., Yu, C. H., and Kuo, D. Y. (2014). Central dopamine action modulates neuropeptide-controlled appetite via the hypothalamic PI3K/NF-kappaB-dependent mechanism. Genes Brain Behav. 13, 784–793. doi: 10.1111/gbb.12174
Huang, P. L. (2009). A comprehensive definition for metabolic syndrome. Dis. Model. Mech. 2, 231–237. doi: 10.1242/dmm.001180
Ip, C. K., Zhang, L., Farzi, A., Qi, Y., Clarke, I., Reed, F., et al. (2019). Amygdala NPY circuits promote the development of accelerated obesity under chronic stress conditions. Cell Metab. 30, 111–128.e6.
Jiang, Z., Rajamanickam, S., and Justice, N. J. (2018). Local Corticotropin-releasing factor signaling in the hypothalamic paraventricular nucleus. J. Neurosci. 38, 1874–1890. doi: 10.1523/jneurosci.1492-17.2017
Jiang, Z., Wang, Y., Sun, L., Yuan, B., Tian, Y., Xiang, L., et al. (2019). Dual ATP and pH responsive ZIF-90 nanosystem with favorable biocompatibility and facile post-modification improves therapeutic outcomes of triple negative breast cancer in vivo. Biomaterials 197, 41–50. doi: 10.1016/j.biomaterials.2019.01.001
Joly-Amado, A., Denis, R. G., Castel, J., Lacombe, A., Cansell, C., Rouch, C., et al. (2012). Hypothalamic AgRP-neurons control peripheral substrate utilization and nutrient partitioning. EMBO J. 31, 4276–4288. doi: 10.1038/emboj.2012.250
Koch, M., and Horvath, T. L. (2014). Molecular and cellular regulation of hypothalamic melanocortin neurons controlling food intake and energy metabolism. Mol. Psychiatry 19, 752–761. doi: 10.1038/mp.2014.30
Kohno, D., and Yada, T. (2012). Arcuate NPY neurons sense and integrate peripheral metabolic signals to control feeding. Neuropeptides 46, 315–319. doi: 10.1016/j.npep.2012.09.004
Konner, A. C., and Bruning, J. C. (2012). Selective insulin and leptin resistance in metabolic disorders. Cell Metab. 16, 144–152. doi: 10.1016/j.cmet.2012.07.004
Konner, A. C., Janoschek, R., Plum, L., Jordan, S. D., Rother, E., Ma, X., et al. (2007). Insulin action in AgRP-expressing neurons is required for suppression of hepatic glucose production. Cell Metab. 5, 438–449. doi: 10.1016/j.cmet.2007.05.004
Konner, A. C., Klockener, T., and Bruning, J. C. (2009). Control of energy homeostasis by insulin and leptin: targeting the arcuate nucleus and beyond. Physiol. Behav. 97, 632–638. doi: 10.1016/j.physbeh.2009.03.027
Lafferty, R. A., Tanday, N., Flatt, P. R., and Irwin, N. (2020). Generation and characterisation of C-terminally stabilised PYY molecules with potential in vivo NPYR2 activity. Metabolism 111:154339. doi: 10.1016/j.metabol.2020.154339
Lechan, R. M., and Fekete, C. (2006). The TRH neuron: a hypothalamic integrator of energy metabolism. Prog. Brain Res. 153, 209–235. doi: 10.1016/s0079-6123(06)53012-2
Lemes, S. F., de Souza, A. C. P., Payolla, T. B., Versutti, M. D., de Fatima da Silva Ramalho, A., Mendes-da-Silva, C., et al. (2018). Maternal consumption of high-fat diet in mice alters hypothalamic notch pathway, NPY cell population and food intake in offspring. Neuroscience 371, 1–15. doi: 10.1016/j.neuroscience.2017.11.043
Li, J., Tang, Y., and Cai, D. (2012). IKKbeta/NF-kappaB disrupts adult hypothalamic neural stem cells to mediate a neurodegenerative mechanism of dietary obesity and pre-diabetes. Nat. Cell Biol. 14, 999–1012. doi: 10.1038/ncb2562
Li, L., Jönsson-Rylander, A. C., Abe, K., and Zukowska, Z. (2005). Chronic stress induces rapid occlusion of angioplasty-injured rat carotid artery by activating neuropeptide Y and its Y1 receptors. Arterioscler. Thromb. Vasc. Biol. 25, 2075–2080. doi: 10.1161/01.atv.0000179601.19888.19
Lin, E. J., Sainsbury, A., Lee, N. J., Boey, D., Couzens, M., Enriquez, R., et al. (2006). Combined deletion of Y1, Y2, and Y4 receptors prevents hypothalamic neuropeptide Y overexpression-induced hyperinsulinemia despite persistence of hyperphagia and obesity. Endocrinology 147, 5094–5101. doi: 10.1210/en.2006-0097
Lin, H. V., Plum, L., Ono, H., Gutierrez-Juarez, R., Shanabrough, M., Borok, E., et al. (2010). Divergent regulation of energy expenditure and hepatic glucose production by insulin receptor in agouti-related protein and POMC neurons. Diabetes 59, 337–346. doi: 10.2337/db09-1303
Liu, Y., Zhao, S. Y., Feng, Y., Sun, J., Lu, X. L., Yan, Q. X., et al. (2020). Contribution of baroreflex afferent pathway to NPY-mediated regulation of blood pressure in rats. Neurosci. Bull. 36, 396–406. doi: 10.1007/s12264-019-00438-w
Loh, K., Herzog, H., and Shi, Y. C. (2015). Regulation of energy homeostasis by the NPY system. Trends Endocrinol. Metab. 26, 125–135. doi: 10.1016/j.tem.2015.01.003
Loh, K., Zhang, L., Brandon, A., Wang, Q., Begg, D., Qi, Y., et al. (2017). Insulin controls food intake and energy balance via NPY neurons. Mol. Metab. 6, 574–584. doi: 10.1016/j.molmet.2017.03.013
Luquet, S., Perez, F. A., Hnasko, T. S., and Palmiter, R. D. (2005). NPY/AgRP neurons are essential for feeding in adult mice but can be ablated in neonates. Science 310, 683–685. doi: 10.1126/science.1115524
Maisel, A. S., Scott, N. A., Motulsky, H. J., Michel, M. C., Boublik, J. H., Rivier, J. E., et al. (1989). Elevation of plasma neuropeptide Y levels in congestive heart failure. Am. J. Med. 86, 43–48. doi: 10.1016/0002-9343(89)90228-3
Marcelin, G., Jo, Y. H., Li, X., Schwartz, G. J., Zhang, Y., Dun, N. J., et al. (2014). Central action of FGF19 reduces hypothalamic AGRP/NPY neuron activity and improves glucose metabolism. Mol. Metab. 3, 19–28. doi: 10.1016/j.molmet.2013.10.002
Martins, L., Fernandez-Mallo, D., Novelle, M. G., Vazquez, M. J., Tena-Sempere, M., Nogueiras, R., et al. (2012). Hypothalamic mTOR signaling mediates the orexigenic action of ghrelin. PLoS One 7:e46923. doi: 10.1371/journal.pone.0046923
McDermott, B. J., and Bell, D. (2007). NPY and cardiac diseases. Curr. Top. Med. Chem. 7, 1692–1703. doi: 10.2174/156802607782340939
Merten, N., and Beck-Sickinger, A. G. (2006). Molecular ligand-receptor interaction of the NPY/PP peptide family. EXS 95, 35–62. doi: 10.1007/3-7643-7417-9_3
Mesaros, A., Koralov, S. B., Rother, E., Wunderlich, F. T., Ernst, M. B., Barsh, G. S., et al. (2008). Activation of Stat3 signaling in AgRP neurons promotes locomotor activity. Cell Metab. 7, 236–248. doi: 10.1016/j.cmet.2008.01.007
Millar, B. C., Weis, T., Piper, H. M., Weber, M., Borchard, U., McDermott, B. J., et al. (1991). Positive and negative contractile effects of neuropeptide Y on ventricular cardiomyocytes. Am. J. Physiol. 261, H1727–H1733.
Moldawer, L. L., Andersson, C., Gelin, J., and Lundholm, K. G. (1988). Regulation of food intake and hepatic protein synthesis by recombinant-derived cytokines. Am. J. Physiol. 254, G450–G456.
Morris, B. J. (1989). Neuronal localisation of neuropeptide Y gene expression in rat brain. J. Comp. Neurol. 290, 358–368. doi: 10.1002/cne.902900305
Morris, M. J., Cox, H. S., Lambert, G. W., Kaye, D. M., Jennings, G. L., Meredith, I. T., et al. (1997). Region-specific neuropeptide Y overflows at rest and during sympathetic activation in humans. Hypertension 29, 137–143. doi: 10.1161/01.hyp.29.1.137
Morton, G. J., Meek, T. H., and Schwartz, M. W. (2014). Neurobiology of food intake in health and disease. Nat. Rev. Neurosci. 15, 367–378.
Myers, M. G. Jr., and Olson, D. P. (2014). SnapShot: neural pathways that control feeding. Cell Metab. 19, 732–732.e1.
Naveilhan, P., Hassani, H., Canals, J. M., Ekstrand, A. J., Larefalk, A., Chhajlani, V., et al. (1999). Normal feeding behavior, body weight and leptin response require the neuropeptide Y Y2 receptor. Nat. Med. 5, 1188–1193. doi: 10.1038/13514
Nozdrachev, A. D., and Masliukov, P. M. (2011). [Neuropeptide Y and autonomic nervous system]. Zh. Evol. Biokhim. Fiziol. 47, 105–112.
Pedragosa-Badia, X., Stichel, J., and Beck-Sickinger, A. G. (2013). Neuropeptide Y receptors: how to get subtype selectivity. Front. Endocrinol. 4:5. doi: 10.3389/fendo.2013.00005
Plotkin, S. R., Banks, W. A., and Kastin, A. J. (1996). Comparison of saturable transport and extracellular pathways in the passage of interleukin-1 alpha across the blood-brain barrier. J. Neuroimmunol. 67, 41–47. doi: 10.1016/s0165-5728(96)00036-7
Plum, L., Belgardt, B. F., and Bruning, J. C. (2006). Central insulin action in energy and glucose homeostasis. J. Clin. Invest. 116, 1761–1766. doi: 10.1172/jci29063
Qian, X., Wang, Y., Xu, Y., Ma, L., Xue, N., Jiang, Z., et al. (2020). Active targeting nano-scale bubbles enhanced ultrasound cavitation chemotherapy in Y1 receptor-overexpressed breast cancer. J. Mater. Chem. B 8, 6837–6844. doi: 10.1039/d0tb00556h
Ralevic, V. (2009). Purines as neurotransmitters and neuromodulators in blood vessels. Curr. Vasc. Pharmacol. 7, 3–14. doi: 10.2174/157016109787354123
Rezai-Zadeh, K., Yu, S., Jiang, Y., Laque, A., Schwartzenburg, C., Morrison, C. D., et al. (2014). Leptin receptor neurons in the dorsomedial hypothalamus are key regulators of energy expenditure and body weight, but not food intake. Mol. Metab. 3, 681–693. doi: 10.1016/j.molmet.2014.07.008
Roa, J., and Tena-Sempere, M. (2010). Energy balance and puberty onset: emerging role of central mTOR signaling. Trends Endocrinol. Metab. 21, 519–528. doi: 10.1016/j.tem.2010.05.003
Ruohonen, S. T., Savontaus, E., Rinne, P., Rosmaninho-Salgado, J., Cavadas, C., Ruskoaho, H., et al. (2009). Stress-induced hypertension and increased sympathetic activity in mice overexpressing neuropeptide Y in noradrenergic neurons. Neuroendocrinology 89, 351–360. doi: 10.1159/000188602
Sainsbury, A., Rohner-Jeanrenaud, F., Cusin, I., Zakrzewska, K. E., Halban, P. A., Gaillard, R. C., et al. (1997). Chronic central neuropeptide Y infusion in normal rats: status of the hypothalamo-pituitary-adrenal axis, and vagal mediation of hyperinsulinaemia. Diabetologia 40, 1269–1277. doi: 10.1007/s001250050820
Sainsbury, A., Schwarzer, C., Couzens, M., Jenkins, A., Oakes, S. R., Ormandy, C. J., et al. (2002). Y4 receptor knockout rescues fertility in ob/ob mice. Genes Dev. 16, 1077–1088. doi: 10.1101/gad.979102
Saltiel, A. R., and Kahn, C. R. (2001). Insulin signalling and the regulation of glucose and lipid metabolism. Nature 414, 799–806. doi: 10.1038/414799a
Schneeberger, M., Gomis, R., and Claret, M. (2014). Hypothalamic and brainstem neuronal circuits controlling homeostatic energy balance. J. Endocrinol. 220, T25–T46.
Schobitz, B., Pezeshki, G., Pohl, T., Hemmann, U., Heinrich, P. C., Holsboer, F., et al. (1995). Soluble interleukin-6 (IL-6) receptor augments central effects of IL-6 in vivo. FASEB J. 9, 659–664. doi: 10.1096/fasebj.9.8.7768358
Schwartz, M. W., Sipols, A. J., Marks, J. L., Sanacora, G., White, J. D., Scheurink, A., et al. (1992). Inhibition of hypothalamic neuropeptide Y gene expression by insulin. Endocrinology 130, 3608–3616. doi: 10.1210/endo.130.6.1597158
Shi, Y. C., Lau, J., Lin, Z., Zhang, H., Zhai, L., Sperk, G., et al. (2013). Arcuate NPY controls sympathetic output and BAT function via a relay of tyrosine hydroxylase neurons in the PVN. Cell Metab. 17, 236–248. doi: 10.1016/j.cmet.2013.01.006
Silva, A. P., Kaufmann, J. E., Vivancos, C., Fakan, S., Cavadas, C., Shaw, P., et al. (2005). Neuropeptide Y expression, localization and cellular transducing effects in HUVEC. Biol. Cell 97, 457–467. doi: 10.1042/bc20040102
Simpson, K. A., Martin, N. M., and Bloom, S. R. (2008). Hypothalamic regulation of appetite. Expert Rev. Endocrinol. Metab. 3, 577–592.
Sohn, J. W. (2015). Network of hypothalamic neurons that control appetite. BMB Rep. 48, 229–233. doi: 10.5483/bmbrep.2015.48.4.272
Spahis, S., Borys, J. M., and Levy, E. (2017). Metabolic syndrome as a multifaceted risk factor for oxidative stress. Antioxid. Redox Signal. 26, 445–461. doi: 10.1089/ars.2016.6756
Sun, W. W., Zhu, P., Shi, Y. C., Zhang, C. L., Huang, X. F., Liang, S. Y., et al. (2017). Current views on neuropeptide Y and diabetes-related atherosclerosis. Diab. Vasc. Dis. Res. 14, 277–284. doi: 10.1177/1479164117704380
Tan, C. M. J., Green, P., Tapoulal, N., Lewandowski, A. J., Leeson, P., and Herring, N. (2018). The role of neuropeptide Y in cardiovascular health and disease. Front. Physiol. 9:1281. doi: 10.3389/fphys.2018.01281
Tatemoto, K., Carlquist, M., and Mutt, V. (1982). Neuropeptide Y–a novel brain peptide with structural similarities to peptide YY and pancreatic polypeptide. Nature 296, 659–660. doi: 10.1038/296659a0
Thaler, J. P., Yi, C. X., Schur, E. A., Guyenet, S. J., Hwang, B. H., Dietrich, M. O., et al. (2012). Obesity is associated with hypothalamic injury in rodents and humans. J. Clin. Invest. 122, 153–162.
Todorov, L. D., Bjur, R. A., and Westfall, D. P. (1994). Temporal dissociation of the release of the sympathetic co-transmitters ATP and noradrenaline. Clin. Exp. Pharmacol. Physiol. 21, 931–932. doi: 10.1111/j.1440-1681.1994.tb02469.x
Ueda, D., Yonemochi, N., Kamata, T., Shibasaki, M., Kamei, J., Waddington, J. L., et al. (2021). Increase in neuropeptide Y activity impairs social behaviour in association with glutamatergic dysregulation in diabetic mice. Br. J. Pharmacol. 178, 726–740. doi: 10.1111/bph.15326
Vahatalo, L. H., Ruohonen, S. T., Makela, S., Kovalainen, M., Huotari, A., Makela, K. A., et al. (2015). Neuropeptide Y in the noradrenergic neurones induces obesity and inhibits sympathetic tone in mice. Acta Physiol. 213, 902–919. doi: 10.1111/apha.12436
Vaisse, C., Halaas, J. L., Horvath, C. M., Darnell, J. E. Jr., Stoffel, M., and Friedman, J. M. (1996). Leptin activation of Stat3 in the hypothalamus of wild-type and ob/ob mice but not db/db mice. Nat. Genet. 14, 95–97. doi: 10.1038/ng0996-95
Varela, L., and Horvath, T. L. (2012a). AgRP neurons: a switch between peripheral carbohydrate and lipid utilization. EMBO J. 31, 4252–4254. doi: 10.1038/emboj.2012.287
Varela, L., and Horvath, T. L. (2012b). Leptin and insulin pathways in POMC and AgRP neurons that modulate energy balance and glucose homeostasis. EMBO Rep. 13, 1079–1086. doi: 10.1038/embor.2012.174
Varela, L., Martinez-Sanchez, N., Gallego, R., Vazquez, M. J., Roa, J., Gandara, M., et al. (2012). Hypothalamic mTOR pathway mediates thyroid hormone-induced hyperphagia in hyperthyroidism. J. Pathol. 227, 209–222. doi: 10.1002/path.3984
Velasco, C., Libran-Perez, M., Otero-Rodino, C., Lopez-Patino, M. A., Miguez, J. M., Cerda-Reverter, J. M., et al. (2016). Ghrelin modulates hypothalamic fatty acid-sensing and control of food intake in rainbow trout. J. Endocrinol. 228, 25–37. doi: 10.1530/joe-15-0391
Vella, K. R., Ramadoss, P., Lam, F. S., Harris, J. C., Ye, F. D., Same, P. D., et al. (2011). NPY and MC4R signaling regulate thyroid hormone levels during fasting through both central and peripheral pathways. Cell Metab. 14, 780–790. doi: 10.1016/j.cmet.2011.10.009
Vernia, S., Morel, C., Madara, J. C., Cavanagh-Kyros, J., Barrett, T., Chase, K., et al. (2016). Excitatory transmission onto AgRP neurons is regulated by cJun NH2-terminal kinase 3 in response to metabolic stress. Elife 5:e10031.
Waterson, M. J., and Horvath, T. L. (2015). Neuronal regulation of energy homeostasis: beyond the hypothalamus and feeding. Cell Metab. 22, 962–970. doi: 10.1016/j.cmet.2015.09.026
Weigle, D. S., Duell, P. B., Connor, W. E., Steiner, R. A., Soules, M. R., and Kuijper, J. L. (1997). Effect of fasting, refeeding, and dietary fat restriction on plasma leptin levels. J. Clin. Endocrinol. Metab. 82, 561–565. doi: 10.1210/jc.82.2.561
Williams, K. W., and Elmquist, J. K. (2012). From neuroanatomy to behavior: central integration of peripheral signals regulating feeding behavior. Nat. Neurosci. 15, 1350–1355. doi: 10.1038/nn.3217
Woods, S. C., and Seeley, R. J. (2000). Adiposity signals and the control of energy homeostasis. Nutrition 16, 894–902. doi: 10.1016/s0899-9007(00)00454-8
Wu, Q., Clark, M. S., and Palmiter, R. D. (2012). Deciphering a neuronal circuit that mediates appetite. Nature 483, 594–597. doi: 10.1038/nature10899
Wu, W. Q., Peng, S., Wan, X. Q., Lin, S., Li, L. Y., and Song, Z. Y. (2019). Physical exercise inhibits atherosclerosis development by regulating the expression of neuropeptide Y in apolipoprotein E-deficient mice. Life Sci. 237:116896. doi: 10.1016/j.lfs.2019.116896
Xu, J., Bartolome, C. L., Low, C. S., Yi, X., Chien, C. H., Wang, P., et al. (2018). Genetic identification of leptin neural circuits in energy and glucose homeostases. Nature 556, 505–509. doi: 10.1038/s41586-018-0049-7
Yan, C., Zeng, T., Lee, K., Nobis, M., Loh, K., Gou, L., et al. (2021). Peripheral-specific Y1 receptor antagonism increases thermogenesis and protects against diet-induced obesity. Nat. Commun. 12:2622.
Zhang, L., Hernandez-Sanchez, D., and Herzog, H. (2019). Regulation of Feeding-related behaviors by arcuate neuropeptide Y neurons. Endocrinology 160, 1411–1420.
Keywords: neuropeptide Y, metabolic syndrome, arcuate nucleus, clinical intervention strategy perspective, hypothalamus
Citation: Huang Y, Lin X and Lin S (2021) Neuropeptide Y and Metabolism Syndrome: An Update on Perspectives of Clinical Therapeutic Intervention Strategies. Front. Cell Dev. Biol. 9:695623. doi: 10.3389/fcell.2021.695623
Received: 15 April 2021; Accepted: 21 June 2021;
Published: 09 July 2021.
Edited by:
Zhi-Gang Zhang, Shanghai Jiao Tong University, ChinaReviewed by:
Daisuke Kohno, Gunma University, JapanNadia Saderi, Autonomous University of San Luis Potosí, Mexico
Copyright © 2021 Huang, Lin and Lin. This is an open-access article distributed under the terms of the Creative Commons Attribution License (CC BY). The use, distribution or reproduction in other forums is permitted, provided the original author(s) and the copyright owner(s) are credited and that the original publication in this journal is cited, in accordance with accepted academic practice. No use, distribution or reproduction is permitted which does not comply with these terms.
*Correspondence: Xia-Hong Lin, bGlueGlhaG9uZ2RyQGZqbXUuZWR1LmNu; Shu Lin, c2h1bGluMTk1NkAxMjYuY29t