- Division of Cancer Sciences, Faculty of Biology, Medicine and Health, Manchester Academic Health Science Centre, University of Manchester, Manchester, United Kingdom
The Notch signalling pathway is a highly conserved developmental signalling pathway, with vital roles in determining cell fate during embryonic development and tissue homeostasis. Aberrant Notch signalling has been implicated in many disease pathologies, including cancer. In this review, we will outline the mechanism and regulation of the Notch signalling pathway. We will also outline the role Notch signalling plays in normal mammary gland development and how Notch signalling is implicated in breast cancer tumorigenesis and progression. We will cover how Notch signalling controls several different hallmarks of cancer within epithelial cells with sections focussed on its roles in proliferation, apoptosis, invasion, and metastasis. We will provide evidence for Notch signalling in the breast cancer stem cell phenotype, which also has implications for therapy resistance and disease relapse in breast cancer patients. Finally, we will summarise the developments in therapeutic targeting of Notch signalling, and the pros and cons of this approach for the treatment of breast cancer.
Introduction
At the turn of millennium, there was growing interest in the role Notch signalling played in tissue homeostasis and the aetiology of human diseases. Over the previous decade, all four Notch homologues had been identified in mammals, along with the five Notch ligands. The generation of genetic knockouts in mice had demonstrated the importance of Notch signalling in embryonic development and the aetiology of several human genetic disorders, including Alagille syndrome. There was also growing evidence that aberrant Notch signalling was linked to several different cancers, in particular certain leukaemias. Amongst solid cancers, breast cancer was of particular interest. Integration of the Mouse Mammary Tumour Virus (MMTV) into the Notch1 or Notch4 loci, leading to the expression of an activated form of the respective Notch proteins, had been shown to disrupt mammary gland development and cause tumour development. However, it was unclear whether Notch signalling played a role in the normal development of mammary gland or in the aetiology of breast cancer in humans. Work since, has provided conclusive evidence for both.
Notch Signalling
At first glance, the Notch signalling pathway is a simple one, with a relatively small number of core signalling components compared to other vital developmental pathways, and lacking in any second messengers, phosphorylation, or amplification steps (Bray, 2006, 2016; Hori et al., 2013; Figure 1). Signalling through the pathway is typically thought to be initiated by physical association between a Notch receptor expressed on the surface of the signal-receiving cell, and a Notch ligand expressed on the surface of the signal-sending cell. However, there is clear evidence that signalling through the pathway can also be initiated in a ligand independent-manner following Deltex-mediated endocytosis localising the Notch protein to the outer surface of the multivesicular body (Steinbuck and Winandy, 2018).
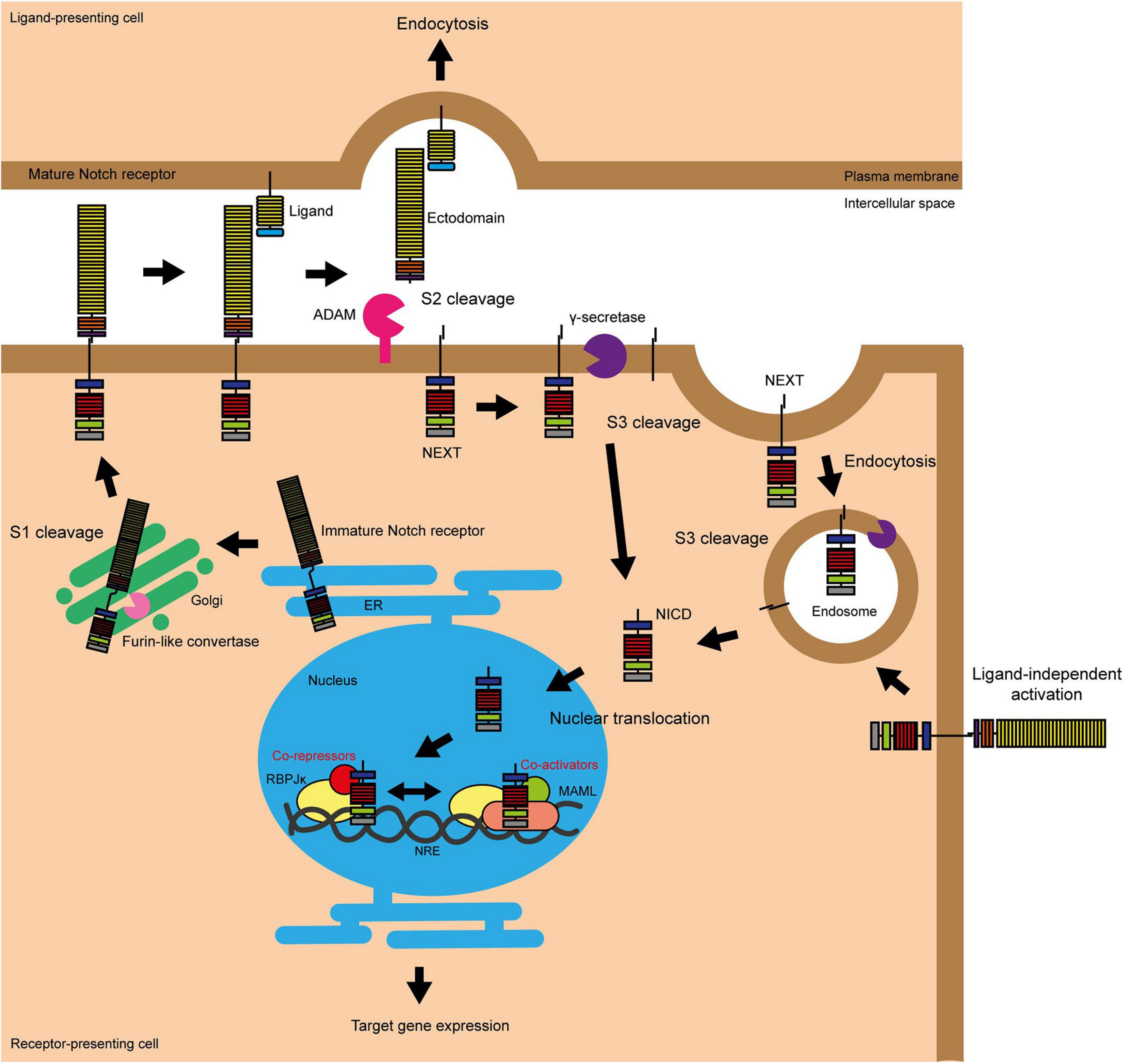
Figure 1. Activation of Notch signalling. The Notch pathway can be activated in two ways, either by interacting with a ligand on an adjacent cell or following endocytosis driven by Deltex. S1 cleavage occurs in the Golgi and mediates the production of the mature Notch heterodimer which is presented on the surface of the cell. Ligand binding stimulates S2 cleavage, which causes the release of the Notch ectodomain and subsequent endocytosis by the ligand-presenting cell. S2 cleavage provides the substrate for γ-secretase, which carries out the final S3 cleavage and releases NICD into the cytoplasm where it can translocate into the nucleus to activate target gene transcription (Fortini, 2009; Aster et al., 2017). The endocytosis of the Notch protein to the multivesicular body driven by Deltex also provides a substrate for γ-secretase and thus target gene transcription (Steinbuck and Winandy, 2018).
There are four Notch receptors (Notch 1–4). As these receptors are produced in the endoplasmic reticulum (ER), they undergo vital post-translational modification and processing steps in the Golgi. This includes proteolytic cleavage by Furin-like convertase and O-glycosylation by protein O-fucosyltransferase 1 (POFUT1), protein O-glucosyltransferase 1 (POGLUT1) and the Fringe proteins. Furin processing of the Notch receptor is known as S1 cleavage, and results in the presentation of the receptor at the plasma membrane as a heterodimer, with the two fragments linked by non-covalent Ca2+ salt bridge interactions (Logeat et al., 1998; Rand et al., 2000). O-Glycosylation alters the folding of the Notch protein, increasing its stability and presentation at the cell surface, and changing its interaction with the five Notch ligands, Delta-like 1, 3, and 4 (DLL1, DLL3, and DLL4) and Jagged 1 and 2 (JAG1 and JAG2) (Harvey and Haltiwanger, 2018). Fringe modification of the O-glycosylation chains favours binding by the DLL ligands.
Notch receptor-ligand binding triggers endocytosis of the ligand by the ligand-presenting cell (Parks et al., 2000). This induces a mechanical force across the receptor which causes the unfolding of the NRR domain, exposing the S2 site to proteolytic cleavage by disintegrin and metalloprotease (ADAM) proteases (Brou et al., 2000; Nichols et al., 2007). ADAM protease activity causes the release of the Notch ectodomain, leaving the activated and membrane bound form of Notch known as NEXT (Notch extracellular truncation) (Andersson et al., 2011). The Notch ectodomain is endocytosed by the ligand-presenting cell (Hori et al., 2013). NEXT is the substrate for γ-secretase, a complex comprising presenilin, nicastrin, presenilin enhancer 2 (PEN2) and anterior pharynx-defective 1 (APH1), which carries out the third and final Notch proteolytic cleavage (S3) to release NICD into the cytoplasm (Schroeter et al., 1998; De Strooper et al., 1999; Bray, 2006). S3 cleavage by γ-secretase can occur at the plasma membrane or within endosomes as part of NEXT endosomal trafficking (Vaccari et al., 2008; Andersson et al., 2011). Once in the cytoplasm, NICD is transported to the nucleus via importin-α proteins, where it is able to induce target gene transcription (Huenniger et al., 2010).
As well as the ligand-activated Notch signalling pathway outlined above, evidence shows that the core Notch pathway can be activated in a ligand-independent manner through the activity of the E3 ubiquitin ligase Deltex (DTX). In this scenario, full length Notch is trafficked into the cell through endocytosis. DTX functions to stabilise Notch in the endocytic compartment via ubiquitination, and assists in the delivery of the receptor to the limiting membrane of the multivesicular body. Here the receptor undergoes S3 cleavage and NICD is released into the cytoplasm (Shimizu et al., 2014; Steinbuck and Winandy, 2018). There are also suggestions that Notch/DLL and Notch/JAG signalling are distinct, with Notch/DLL signalling favouring the classical lateral inhibition patterning causing cells within a sheet to adopt two different fates in a salt and pepper pattern and Notch/JAG signalling favouring lateral induction causing a group of neighbouring cells to adopt the same fate (Bocci et al., 2020).
In the absence of NICD, Notch target gene expression is repressed by the transcription factor RBPJκ (also known as CBF1) and its co-repressors (Jarriault et al., 1995). These co-repressors, such as RBPJκ-interacting and tubulin-associated (RITA) and silencing mediator for retinoid or thyroid hormone receptors (SMRT)/histone deacetylase (HDAC) 1-associated repressor protein (known as SHARP), compete for NICD binding, as well as actively silencing target gene transcription (Kao et al., 1998; Oswald et al., 2005; Wacker et al., 2011). In the presence of NICD, the co-repressors are displaced and a transcriptional activator complex is formed containing NICD, RBPJκ, and various co-activators including the Mastermind-like (MAML) proteins (Wu et al., 2000; Nam et al., 2006; Wilson and Kovall, 2006). The transcriptional activator complex binds to Notch regulatory elements (NREs) located in gene enhancer elements, resulting in Notch target gene expression (Aster et al., 2017). This is the traditional “switch” model of Notch target gene regulation; however more recent studies have suggested a more dynamic role for RBPJκ than previously thought, involving the movement of the whole transcriptional activator/repressor complex on and off the NRE (Kao et al., 1998; Castel et al., 2013; Bray, 2016).
The classical Notch target genes are the hairy and enhancer of split-related genes; belonging to the HES and HEY families. Hes/Hey proteins are basic helix-loop-helix (bHLH) transcription factors which play key roles during embryonic development as transcriptional repressors. Other canonical Notch target genes include the transcription factors c-Myc, GATA2/3 and Snail; cell cycle regulators E2F, cyclin D1/3, and p21; immune components interleukin 2 receptor subunit alpha [IL2RA (CD25)], pre-T cell receptor α (pTa) and NFκB2; developmental homeobox (HOX) A genes; the matrix metalloprotease ADAM19, and the receptor tyrosine kinase platelet-derived growth factor receptor beta (PDGFRβ) (Oswald et al., 1998; Deftos et al., 2000; Rangarajan et al., 2001; Ronchini and Capobianco, 2001; Reizis and Leder, 2002; Robert-Moreno et al., 2005; Maillard et al., 2006; Palomero et al., 2006; Weerkamp et al., 2006; Weng et al., 2006; Amsen et al., 2007; Fang et al., 2007; Jin et al., 2008; Sahlgren et al., 2008; Borggrefe and Oswald, 2009; Joshi et al., 2009; Bivik et al., 2016). Finally, like many key signalling pathways, Notch is involved in crosstalk with other notable signalling networks in the regulation of development, inflammation and cell function. This is particularly important to consider in the context of Notch signalling in oncogenesis and the design of Notch-targeting therapeutic approaches. For example, Notch interacts with the Wnt, NFκB, TGFβ, HIF1α, YAP/TAZ, EGFR and Akt signalling pathways (Andersson et al., 2011; Bray, 2016; Fazio and Ricciardiello, 2016; Siebel and Lendahl, 2017; Totaro et al., 2018).
Mammary Stem Cells, Progenitors and Lineage Determination
A small subpopulation of mammary epithelial cells can re-populate a full functional mammary gland in a cleared mammary fat pad (Kordon and Smith, 1998; Figure 2). These mammary gland-reconstituting cells contain multi/bipotent mammary stem cells (MaSCs) and unipotent mammary epithelial progenitors (Slepicka et al., 2020). MaSCs are primarily active during the embryonic stage as foetal mammary stem cells (fMaSCs). Most post-natal mammary gland development originates from unipotent lineage-committed progenitors (luminal and myoepithelial progenitor cells) located in the basal epithelium (Inman et al., 2015). Cells in the basal layer generally do not express ER(α), however, luminal cells are a mixed population of both ER+ (oestrogen sensitive) and ER- (oestrogen non-responsive) cells (Clarke et al., 1997; Russo et al., 1999; Watson and Khaled, 2020).
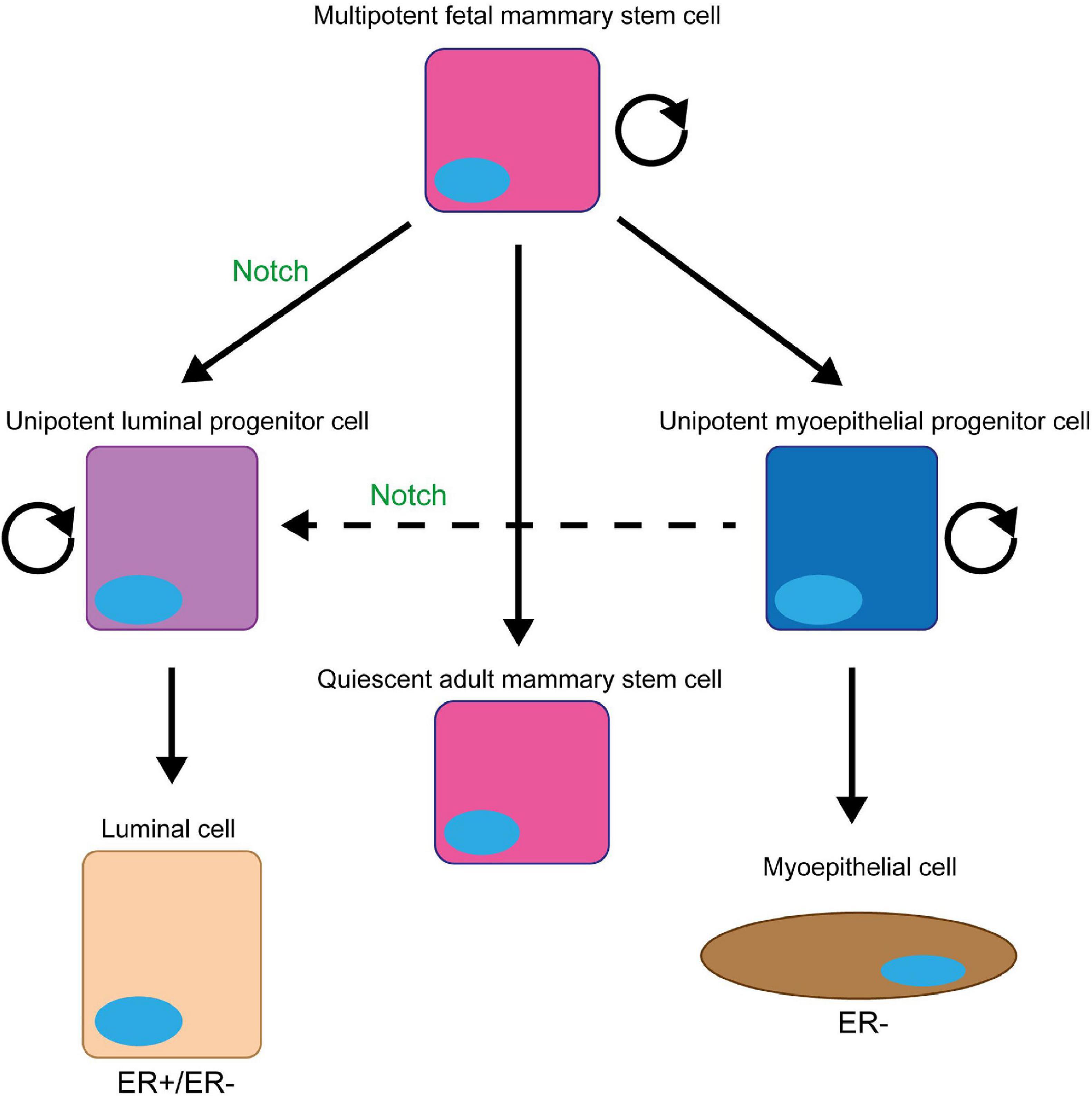
Figure 2. Notch signalling promotes and maintains the luminal progenitor cell fate in the mammary gland. The initial stem cell found within the mammary gland (fMaSC) is multipotent and can form both luminal and myoepithelial cells. However, by late embryogenesis, the gland contains two unipotent progenitor cells that form and maintain the luminal and myoepithelial cell layers of the ductal structures within the gland during puberty and adult life and a quiescent multipotent adult MaSC that is only reactivated upon injury (Woodward et al., 2005; Watson and Khaled, 2020). Notch signalling promotes the differentiation of the foetal MaSCs into the unipotent luminal progenitor cell and prevents this cell differentiating into mature luminal epithelial cells to maintain the population (Dontu et al., 2004; Buono et al., 2006; Bouras et al., 2008; Raouf et al., 2008; Lafkas et al., 2013; Šale et al., 2013; Rodilla et al., 2015; Zhang Y. et al., 2016; Lilja et al., 2018). Upon ablation of the luminal epithelial cells in the adult mammary gland, Notch signalling can also promote the conversion of unipotent myoepithelial progenitor cells into unipotent luminal progenitors to repopulate the luminal lineage (Centonze et al., 2020).
Notch signalling is critical in MaSC and mammary progenitor cell function. Early studies focused on Notch4 signalling suggested that it is upregulated in MaSCs and important for their self-renewal (Dontu et al., 2004; Chakrabarti et al., 2018). However, these studies are not supported by other reports in the literature. Firstly, the original description of the Notch4 knockout mouse failed to identify a mammary gland phenotype (Krebs et al., 2000), suggesting that there isn’t a significant role for Notch4 in MaSCs in vivo. Secondly, more recent work looking at the function of the Notch pathway inhibitor Numb in the mammary gland demonstrated that it segregates asymmetrically during MaSC division to the daughter cell with more stem-like characteristics (Santoro et al., 2016), arguing that Notch signalling is blocked in the MaSC. Interestingly, Notch signalling does play an indirect role in maintaining MaSCs within the growing mammary ducts. DLL1 expressed in MaSCs found within the cap cell layer of terminal end buds, the outer layer of cells at growing the tip of mammary duct, activates Notch signalling in adjacent macrophages. This induces the expression of several Wnt proteins which signal back to the MaSCs within the terminal end bud to maintain the stem cell fate (Dontu et al., 2004; Chakrabarti et al., 2018).
On the other hand, there is abundant evidence that Notch signalling plays a significant role in driving MaSCs toward the unipotent luminal progenitor fate. Notch1–3 are more highly expressed in luminal cells, whilst the Notch pathway inhibitors Numb and Numb-like are found in myoepithelial cells (Bouras et al., 2008; Raouf et al., 2008; Raafat et al., 2011; Zhang Y. et al., 2016). Functional studies using knockout and transgenic mouse models and primary human cells have confirmed that Notch signalling controls the luminal vs. myoepithelial lineage balance (Smith et al., 1995; Dontu et al., 2004; Kiaris et al., 2004; Buono et al., 2006; Hu et al., 2006; Bouras et al., 2008; Raouf et al., 2008; Yalcin-Ozuysal et al., 2010; Santoro et al., 2016; Zhang Y. et al., 2016; Onoyama et al., 2020). In the absence of Notch signalling, there is an accumulation of myoepithelial cells, whilst increased Notch signalling leads to an expansion of the of the luminal lineage (Smith et al., 1995; Kiaris et al., 2004; Buono et al., 2006; Hu et al., 2006; Bouras et al., 2008; Yalcin-Ozuysal et al., 2010; Zhang Y. et al., 2016; Onoyama et al., 2020). Lineage tracing studies have found that Notch signalling drives MaSCs into the unipotent luminal progenitor fate by late embryogenesis (Lafkas et al., 2013; Šale et al., 2013; Rodilla et al., 2015; Lilja et al., 2018). However, recent experiments have shown that following ablation of luminal cells, Notch signalling is reactivated in unipotent myoepithelial progenitors to drive the regeneration of luminal cells (Centonze et al., 2020). Interestingly, the functional studies have also shown that Notch signalling not only drives MaSCs toward the luminal progenitor cell fate but also maintains cells in this fate preventing their terminal differentiation (Dontu et al., 2004; Buono et al., 2006; Bouras et al., 2008; Raouf et al., 2008; Zhang Y. et al., 2016). Maintaining this proliferative cell fate could explain why tumour development is seen in transgenic and knockout mouse models where Notch signalling is activated in the mammary gland (Smith et al., 1995; Kiaris et al., 2004; Hu et al., 2006).
Although Notch1, 2, and 3 are all expressed in luminal epithelial cells, Notch3 is the most highly expressed (Raafat et al., 2011). It also appears to be the most important for the decision to adopt the luminal progenitor fate, as the only reports of a phenotype seen when ablating signalling through an individual Notch receptor come from papers reporting the Notch3 knockout in mice (Xiong et al., 2020) and Notch3 knockdown in primary human breast epithelial cells (Dontu et al., 2004; Buono et al., 2006; Bouras et al., 2008; Raouf et al., 2008; Zhang Y. et al., 2016). In contrast, expressing an activated form of Notch 1, 3, or 4 seems to be sufficient to drive tumour formation (Smith et al., 1995; Kiaris et al., 2004; Hu et al., 2006; Bouras et al., 2008; Zhang Y. et al., 2016; Onoyama et al., 2020).
Notch in Breast Cancer
Notch signalling is aberrantly activated in breast cancer, with increased NICD accumulation and target gene expression detected in a range of breast cancer cell lines and primary samples (Weijzen et al., 2002; Stylianou et al., 2006; Mittal et al., 2009). Overexpression of Notch receptors and ligands have been reported in breast tumours, and is correlated with poorer patient prognosis (Reedijk et al., 2005). Aberrant Notch signalling has also been extensively linked to the triple negative breast cancer (TNBC) subtype; Notch receptor overexpression is correlated with the aggressive, metastatic and therapy resistance phenotype characteristic of TNBC (Zhong et al., 2016; Giuli et al., 2019). Notch4 is particularly associated with TNBC. One study found that Notch4 was expressed in 55.6% of TNBC samples compared to 25.5% of ER+ samples (Wang J.W. et al., 2018a).
Data suggests that deregulation of Notch signalling is an early event in breast cancer tumorigenesis, with accumulation of NICD and increased Hey1 expression detected in a broad range of subtypes, including ductal carcinoma in situ and epithelial hyperplasia (Stylianou et al., 2006; Mittal et al., 2009; Zardawi et al., 2010). This implies that aberrant Notch signalling plays a causative role in breast tumour initiation. In contrast to haematological malignancies, aberrant activation of Notch signalling in the breast is primarily induced through means other than Notch receptor or ligand mutation, although some mutations have been identified. Activating mutations within and surrounding the PEST domain of Notch1, 2, and 3; mutations disrupting the NRR and heterodimerisation domains; and focal amplifications have been identified in patient tumours and patient-derived xenograft (PDX) models, notably with enrichment in TNBCs (Wang et al., 2015). These mutations result in increased nuclear accumulation of NICD and upregulated target gene expression. In particular, Notch4 mutation and overexpression is correlated with metastatic and poor prognosis TNBC, implicating Notch4 in BCSC activity and chemoresistance (Giuli et al., 2019). Loss of Numb is a frequent cause of aberrant Notch signalling in breast cancer (Stylianou et al., 2006). Pece et al. (2004) found that Numb protein was completely lost or reduced in ∼50% of all breast cancers analysed, through ubiquitination and proteasomal degradation. Numb levels and tumour grade were inversely correlated, which was corroborated by another study that identified Numb loss as a determinant in aggressive and poor prognosis tumours. Collectively, these studies emphasise the importance of Numb as a tumour suppressor in the breast (Colaluca et al., 2008).
Increased Notch activation is sufficient to induce mammary gland tumour formation in vivo (Smith et al., 1995; Kiaris et al., 2004; Hu et al., 2006). Moreover, in vitro, overexpression of NICD1/4 or RBPJκ-VP16 (which activates RBPJκ-dependent Notch target gene expression in the absence of upstream stimulation) is sufficient to transform mammary epithelial cells (Imatani and Callahan, 2000; Stylianou et al., 2006). Notch co-operation with other pro-tumorigenic signalling pathways, including other developmental pathways, growth factor signalling, pro-inflammatory cytokines, oncogenic kinase pathways and transcription factors, compounds its role in breast tumour initiation and the cancer cell phenotype (Guo et al., 2011). Notch-Wnt crosstalk in particular has been implicated in breast tumour initiation. For example, Ayyanan et al. (2006) demonstrated that Wnt-induced primary mammary epithelial cell transformation was dependent on upregulated Notch activity via increased expression of DLL ligands. Conversely, inhibition of Notch signalling has consistently been shown to reduce or abolish breast tumour development and/or progression (Lee et al., 2008a; Efferson et al., 2010; Castro et al., 2015; Choy et al., 2017). More detail on the potential of Notch therapeutic targeting in cancer will be given later in this review.
Cell Proliferation
Signalling from the Notch1, 3, and 4 receptors promotes cell proliferation, both directly through target gene expression and indirectly through activation of downstream signalling pathways (Figure 3). Importantly for the consideration of therapeutic targeting, inhibition of Notch signalling suppresses breast cancer cell proliferation and tumour growth, while ectopic activation of Notch signalling increases proliferation rate (Sun et al., 2005; Yamaguchi et al., 2008; Nagamatsu et al., 2014; Castro et al., 2015; Pei and Wang, 2015; Zhang Q. et al., 2016; Choy et al., 2017; Kong et al., 2018; Rui et al., 2018; Tu et al., 2019). The Hes/Hey canonical Notch target genes are both pro- and anti-proliferative, with Hes1 inhibiting cell cycle progression by suppressing E2F1 expression and Hes6 upregulating E2F1 expression to promote cell cycle progression (Hartman et al., 2009; Strom et al., 2009). In fact, several Notch target genes are cell cycle regulators, meaning that aberrant Notch signalling significantly deregulates cell cycle progression. A few cyclins are upregulated by Notch signalling, and Cyclin D1 is a direct target of JAG1-Notch1/3 signalling in triple negative breast cancer cells (Reedijk, 2012). Inhibition of JAG1 expression in MDA-MB-231 cells is sufficient to reduce cell cycle progression, while JAG1 and cyclin D1 expression are positively correlated in basal breast cancers (Cohen et al., 2010). The proto-oncogene c-Myc is an important direct RBPJκ-dependent Notch target gene. Ablation of c-Myc in MMTV/NICD1 mice can prevent tumour formation (Klinakis et al., 2006; Aster et al., 2017). Crosstalk with signalling molecules such as Ras and Wnt also mediate the role of Notch in breast cancer cell proliferation, with studies detecting concomitant suppression of these pathways in response to Notch inhibition (Mittal et al., 2014; Lai et al., 2018).
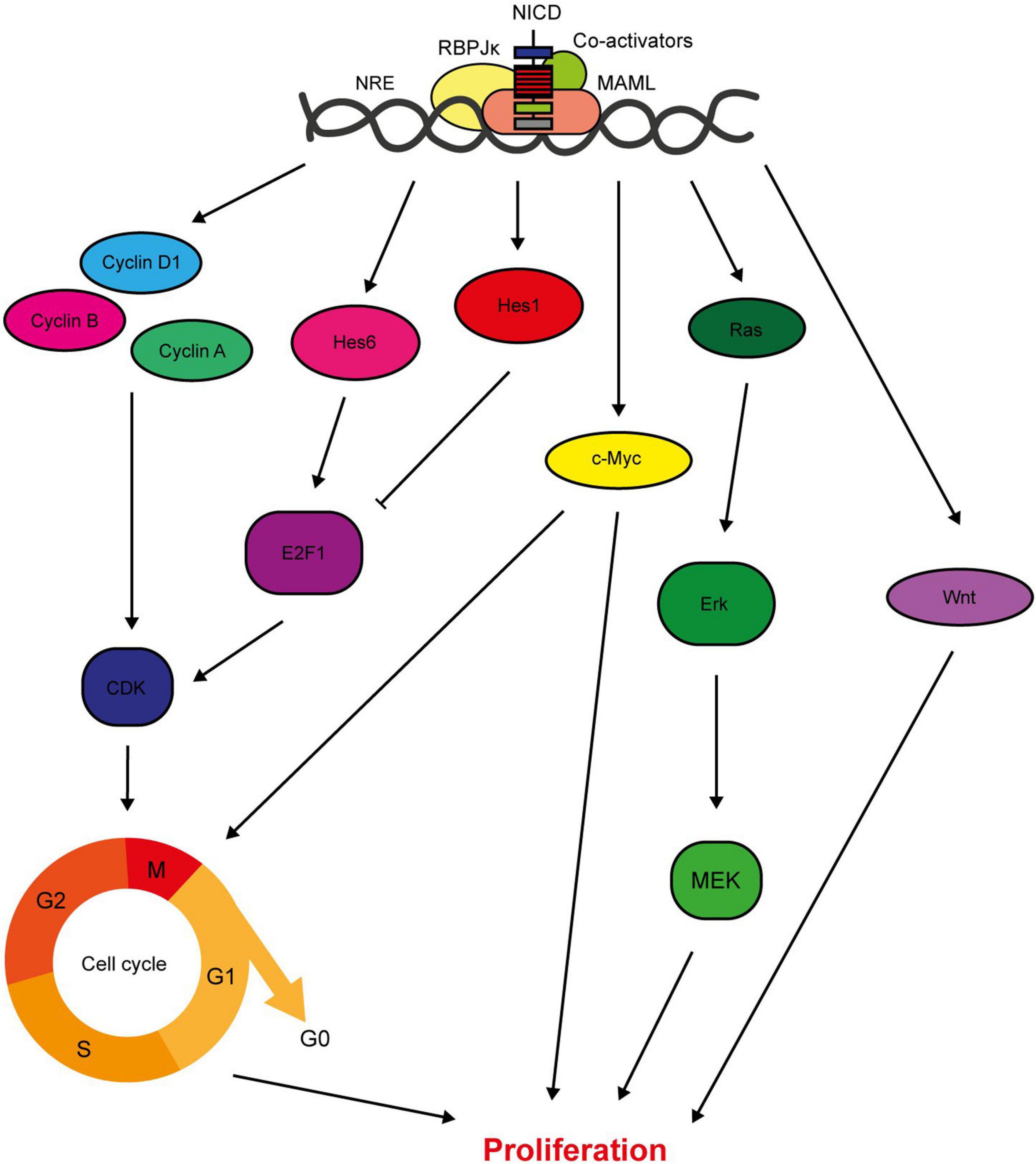
Figure 3. Notch regulates breast cancer cell proliferation. Notch signalling has several direct target genes implicated in cell cycle regulation. These include cyclins A, B and D1, and Hes/Hey family members (Rizzo et al., 2008; Cohen et al., 2010). While most factors downstream of Notch increase the proliferative rate of the cell, Hes1 downregulates E2F1 expression which inhibits cell cycle progression (Hartman et al., 2009; Strom et al., 2009). Notch also activates key oncogenic signalling pathways with pleiotropic effects on cellular function including proliferation, such as c-Myc, Ras and Wnt (Mittal et al., 2009; Aster et al., 2017; Lai et al., 2018).
Cell Survival
Notch1/3/4 signalling is anti-apoptotic in the breast, and hence promotes breast cancer cell survival (Figure 4). Previous work in our lab has shown that activation of Notch signalling in non-transformed breast epithelial cells inhibits drug-induced apoptosis. Conversely, inhibition of Notch in breast cancer cells is sufficient to re-sensitise the cells to apoptosis. This was determined to be through Notch-induced activation of Akt, via an unknown autocrine signalling factor, and a downstream apoptosis signal regulating kinase 1 (ASK1)/c-Jun N-terminal kinase (JNK)/p53 signalling axis (Stylianou et al., 2006; Meurette et al., 2009). This mechanism was independent of PTEN, an important negative regulator of Akt activation which is downregulated by Notch in other cancer types (Palomero et al., 2007). This work intertwines Notch, Akt and p53 in anti-apoptotic signalling. This supports previous findings by researchers such as Mungamuri et al. (2006) who showed that treatment of Notch-activated MCF-7 cells with a PI3K or mTOR inhibitor sensitised the cells to cytotoxic drug-induced apoptosis, which was accompanied by activation of a p53-specific reporter. Mechanistically, it was determined that Notch1-induced pro-survival signalling was mediated by mTOR-dependent PI3K/Akt inhibition of p53 (Mungamuri et al., 2006).
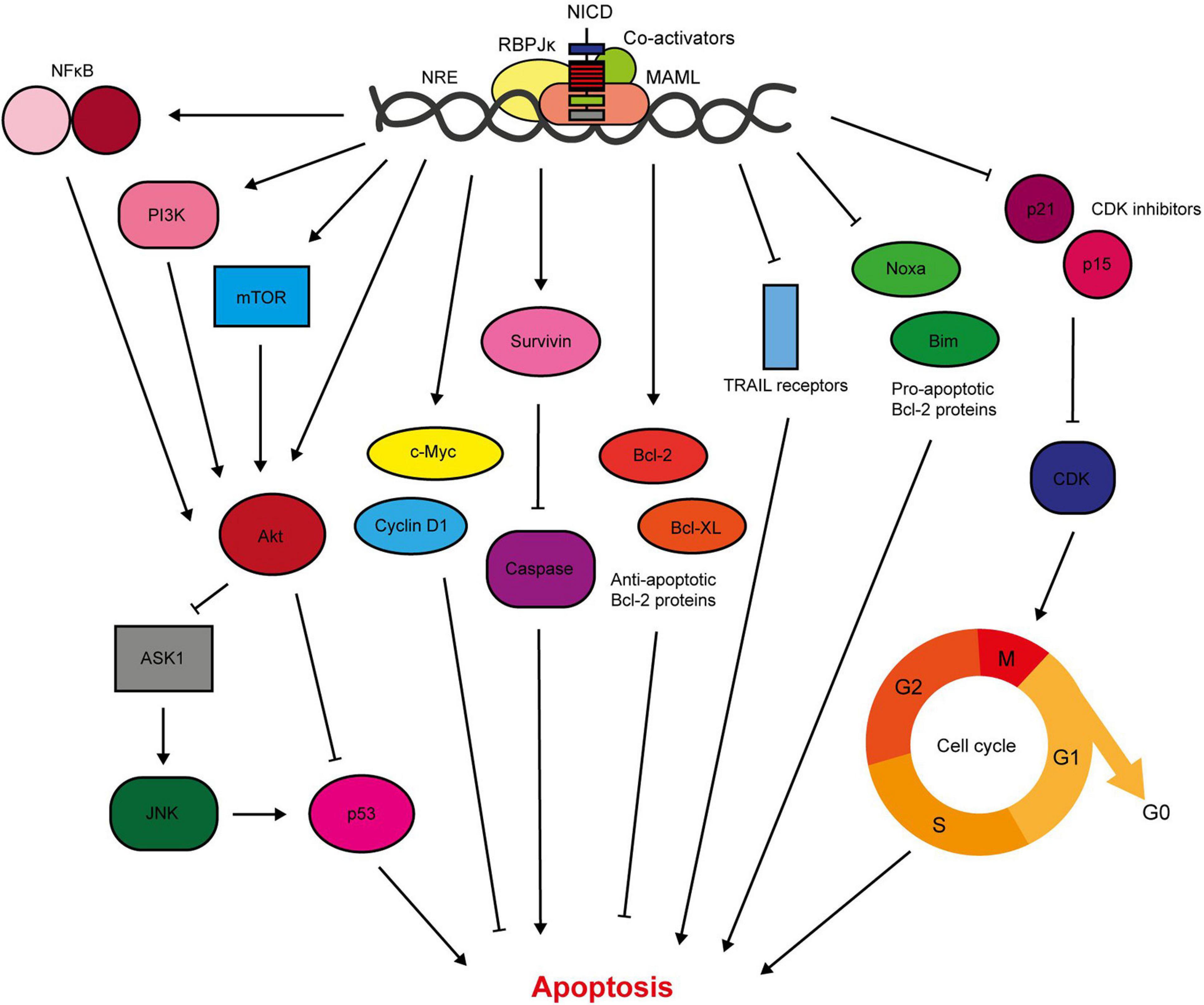
Figure 4. Notch signalling inhibits breast cancer cell apoptosis. Notch activates pro-survival Akt signalling through NFκB, PI3K, and mTOR signalling (Mungamuri et al., 2006; Osipo et al., 2008a; Efferson et al., 2010; Zang et al., 2010; Zhu et al., 2013; Li L. et al., 2014b; Hossain et al., 2018). Our lab have shown that Notch also stimulates Akt through a secreted factor, which triggers stabilisation of p53 through ASK1/JNK signalling (Meurette et al., 2009). The Notch target gene c-Myc is anti-apoptotic, and there is significant evidence demonstrating upregulation of survivin in response to Notch activation (Klinakis et al., 2006; Lee et al., 2008a, b). Survivin prevents apoptosis through indirect and direct caspase inhibition (Garg et al., 2016). Of the Bcl-2 family members, Notch upregulates the anti-apoptotic members including Bcl-2 and Bcl-XL, while downregulating pro-apoptotic members such as Bim and Noxa (Portanova et al., 2013; Sales-Dias et al., 2019). Active Notch signalling reduces the sensitivity of TNBC cells to TRAIL-induced apoptosis (Portanova et al., 2013). Notch regulation of cell cycle regulators, including cyclin D1, p21 and p15, also contributes to apoptosis resistance (Cohen et al., 2010; Sales-Dias et al., 2019).
Furthermore, Notch can activate pro-survival Akt signalling through NFκB in breast cancer cells. Cytoplasmic Notch and phosphorylated Akt (pAkt) correlate with nuclear NFκB in TNBC tumour samples, while mechanistic work in triple negative cell lines demonstrated JAG1-Notch1 (but RBPJκ-independent) stimulation of Akt via mTOR and IκB kinase (IKK) α. Combination treatment of a GSI with either an Akt inhibitor or an IKK inhibitor significantly inhibited TNBC PDX-derived mammosphere growth (Zhu et al., 2013; Hossain et al., 2018). This corroborates data from the Liu lab which showed that Notch1-induced proliferation and reduction in apoptosis was accompanied by NFκB activation and target gene expression in triple negative MDA-MB-231 cells (Li L. et al., 2014b).
Additionally, Notch upregulates anti-apoptotic proteins such as survivin and Bcl2 (Lee et al., 2008b; Sales-Dias et al., 2019). Lee et al. (2008a) showed that GSI treatment reduced survivin expression in triple negative breast cancer cell lines (but not ER+ cell lines), which was sufficient to induce apoptosis, prevent colony formation in soft agar and inhibit xenograft tumour growth and lung metastasis in mice. Portanova et al. (2013) also demonstrated sensitisation of breast cancer cell lines to tumour necrosis-factor related apoptosis-inducing ligand (TRAIL)-induced apoptosis by GSI treatment. Interestingly this effect was found to be more potent in the triple negative MDA-MB-231 cell line compared to ER+ MCF-7 cells. TNBC is the most common breast cancer subtype that develops in BRCA1 mutation carriers (Mavaddat et al., 2012). In a high throughput sequencing screen of BRCA1-deficient murine mammary tumours, Notch1 was identified as an oncogenic driver. Notch1 suppressed DNA damage and mitotic catastrophe (and therefore lethality) caused by BRCA1 deficiency. Notch1 signalling restored the S/G2 and G2/M cell cycle checkpoints, likely through an ATR/CHK1 signalling pathway (Miao et al., 2020).
EMT, Invasion and Metastasis
Notch signalling is implicated in a broad range of processes required for breast cancer cell metastasis including survival of hypoxia, angiogenesis, EMT, local tissue invasion, survival in the circulation, chemoresistance and colonisation of secondary sites (Zhang et al., 2019).
Notch signalling promotes breast epithelial cell EMT (Leong et al., 2007). Inhibition of Notch signalling in TNBC cells reverses the characteristic epithelial to mesenchymal cobblestone to spindle cell morphology and associated marker switch, as well as reducing invasion and migration (Shao et al., 2015; Zhang J. et al., 2016). Mechanistically, Notch induces EMT through activation of Slug and Snail; transcriptional repressor proteins that downregulate E-cadherin expression (Martin et al., 2005; Leong et al., 2007; Zhang et al., 2010; Shao et al., 2015; Figure 5). JAG1, Notch1 and Slug expression correlate in patient tumour samples. Notch4 inhibition also reduces the number and size of MDA-MB-231 xenograft tumour metastases in vivo, which is accompanied by restoration of E-cadherin expression, inactivation of β-catenin and downregulation of Slug (Leong et al., 2007). Interesting recent work suggests that differences between Notch/DLL and Notch/JAG signalling may induce different patterns of EMT within a cancer, with Notch/DLL signalling favouring the induction of EMT in individual cells and Notch/Jag signalling favouring EMT in clusters of cells (Bocci et al., 2019, 2020). The latter may go on to form circulating tumour cell clusters.
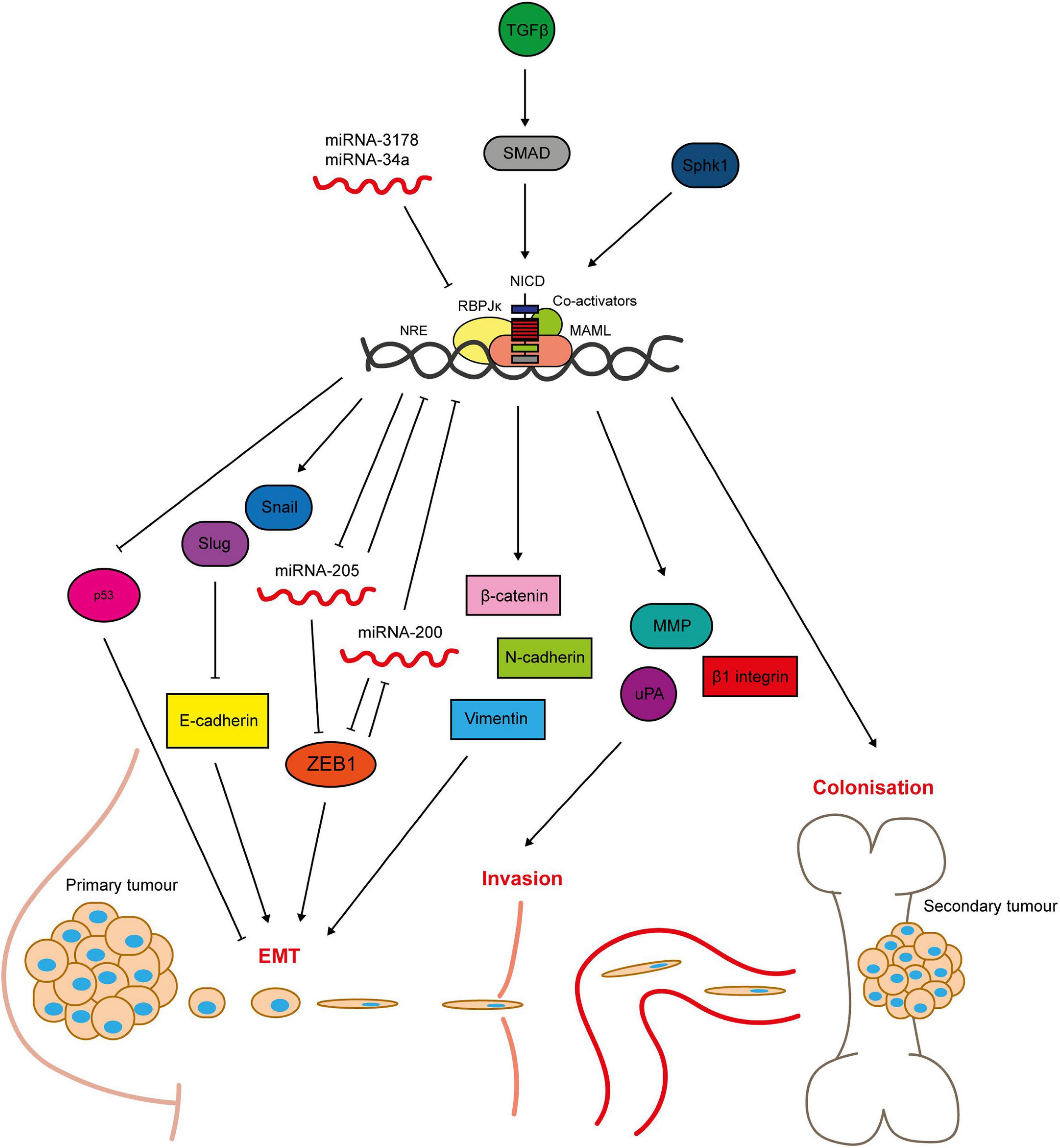
Figure 5. Notch signalling regulates breast cancer cell metastasis. Notch-mediated metastasis is induced by factors such as TGFβ and Sphk1 (Zavadil et al., 2004; Zhang et al., 2010; Sethi et al., 2011; Wang S. et al., 2018b). Notch activates key regulators of EMT including the transcriptional repressors Slug and Snail, that mediate loss of cell-cell contacts through inhibition of E-cadherin expression (Leong et al., 2007; Sahlgren et al., 2008; Zhang et al., 2010; Du et al., 2012; Shao et al., 2015; Kong et al., 2018). The mesenchymal markers ZEB1, β-catenin, N-cadherin and vimentin are upregulated by Notch signalling (Bolós et al., 2013; Lai et al., 2018). ZEB1 is activated through complex bi-directional signalling involving micro-RNAs (Brabletz et al., 2011). Micro-RNAs negatively regulate Notch signalling, and their loss is sufficient to induce EMT in breast epithelial cells (Du et al., 2012; Chao et al., 2014; Kong et al., 2018). Notch is also implicated in tissue invasion, as it upregulates matrix-degrading enzymes including MMP2 and 9 and urokinase-type plasminogen activator (uPA), as well as β1-integrin (Shimizu et al., 2011; Lai et al., 2018). Anti-apoptotic Notch signalling (see Figure 4) enables the cells to survive in the blood stream and travel to secondary sites. Notch signalling between the cancer cells and cells in the bone microenvironment facilitates colonisation and growth at the metastatic site (Sethi et al., 2011).
Hypoxia-induced breast epithelial cell EMT appears to be dependent on Notch signalling, with one study finding that hypoxia downregulated E-cadherin in MCF10A cells only when Notch signalling was aberrantly activated by immobilised JAG1 (Sahlgren et al., 2008). Another study found that Snail expression and E-cadherin downregulation induced by hypoxic treatment of breast cancer cells was abrogated by GSI treatment or dominant negative MAML expression. Hypoxic breast cancer cells had increased invasive and migratory capability in Boyden chamber and scratch wound assay respectively, which was reversed by GSI treatment (Chen et al., 2010). Notch1 has been implicated specifically in triple negative breast cancer EMT. For example, Notch1 is a downstream target of miRNA-3178, an inhibitory miRNA downregulated in TNBC (but not non-TNBC subtypes), that plays a role in EMT through regulation of Snail (Kong et al., 2018). Micro-RNAs are a recurring theme in Notch-mediated EMT, with miRNAs acting both upstream and downstream of the pathway (Du et al., 2012; Chao et al., 2014; Figure 5). Notch is also implicated in bi-directional crosstalk with the mesenchymal marker zinc finger E-box binding homeobox 1 (ZEB1). Knockdown of ZEB1 in breast cancer cells inhibits Notch activity, including downregulation of JAG1, MAML2/3 and HEY1 expression, via de-repression of miRNA-200 expression. In primary TNBC samples high ZEB1 expression was correlated with upregulated JAG1 and Notch activity in invasive tumour regions (Brabletz et al., 2011).
The role of Notch signalling in EMT corresponds to its promotion of invasive and metastatic phenotypes. Activation of Notch signalling in non-invasive breast cancer cells promotes cell invasion and migration, while inhibition of Notch in invasive cells reduces their invasive and migratory capacity (Bolós et al., 2013; Castro et al., 2015; Lai et al., 2018; Leontovich et al., 2018). Moreover, Notch signalling is correlated with metastasis in vivo (Kontomanolis et al., 2014). In a single cell gene expression analysis, NOTCH4, NOTCH3 and JAG1 were upregulated in metastatic breast cancer cells compared to primary tumour cells isolated from TNBC patient-derived xenograft (PDX) models (Lawson et al., 2015). JAG1-induced Notch signalling is also important in breast cancer cell colonisation of the bone metastatic niche (Zhang et al., 2010). High JAG1 expression is correlated with bone-tropic metastatic breast cancer cell lines and samples from patient bone metastasised tumours. It was shown that JAG1 is upregulated in the cancer cells by SMAD-dependent TGFβ signalling (Figure 5), and activates Notch signalling in osteoblasts within the bone microenvironment. Importantly, pharmacological inhibition of Notch signalling was sufficient to reduce breast cancer bone metastasis and osteolysis in vivo, implying that targeting Notch signalling may be a suitable therapeutic approach for inhibiting breast cancer metastasis (Sethi et al., 2011).
A Role in Breast Cancer Therapy Resistance
Notch signalling is induced by breast cancer chemotherapy, and is upregulated in therapy-resistant tumour cells (Bhola et al., 2016; Xiao et al., 2019). Activation of Notch signalling is sufficient to induce chemotherapy resistance, while inhibition of Notch signalling re-sensitises resistant cells to conventional therapy (Li X.J. et al., 2012a; García-Heredia et al., 2016; Xiao et al., 2019). Combining Notch inhibitors with conventional chemotherapies has an additive effect, increasing treatment efficacy both in vitro and in vivo (Qiu et al., 2013; Rustighi et al., 2014; Li et al., 2015; Zhou et al., 2017). Furthermore, the failure of inhibitors of key pro-oncogenic signalling pathways in clinical trials has been partially attributed to Notch signalling. For example, investigation of TNBC PI3K/mTOR inhibitor resistance found that PI3K/mTOR or TORC1/2 treatment enriched for BCSCs with upregulated Notch1 expression. GSI Notch blockade prevented this BCSC enrichment (Bhola et al., 2016). In addition, Diluvio et al. (2018) demonstrated that GSI treatment sensitised EGFR tyrosine kinase inhibitor (TKI) resistant TNBC cells to gefitinib.
Notch signalling is also implicated in breast cancer cell resistance to radiotherapy. Radiation induces BCSCs and Notch activity in vivo (Lagadec et al., 2013; Kim et al., 2016), which confers radioresistance in TNBC (Lee et al., 2019). GSI treatment prevents radiation-induced BCSC enrichment (Lagadec et al., 2013). Mechanistically, Notch has been found to mediate triple negative/basal-like breast cancer radioresistance through BCSC enrichment downstream of tribbles homolog 3 (TRIB3), and in parallel with STAT1 (Boelens et al., 2014; Lee et al., 2019). It also mediates radiation-induced EMT as part of an IL-6/JAK/STAT3 signalling axis (Kim et al., 2016).
There is significant evidence to suggest that Notch signalling plays a role in ER+ breast cancer endocrine therapy resistance (Acar et al., 2016). Notch signalling is upregulated in endocrine therapy resistant ER+ breast cancer cell lines (Magnani et al., 2013). In particular, Notch4 activity has been found to be increased in resistant ER+ cell lines and tamoxifen and fulvestrant-treated PDX models. Activation of JAG1/Notch4 signalling was sufficient to induce endocrine therapy resistance in MCF-7 cells, and tamoxifen resistance could be predicted for in ER+ breast cancer patients using a Notch4/HES/Hey gene signature. This Notch4-induced resistance was accompanied by an enrichment for BCSCs. GSI treatment inhibited endocrine therapy-induced BCSC activity and re-implantation tumour formation in breast cancer PDX models and cell lines (Simões et al., 2015). Furthermore, evidence suggests that Notch signalling functions in a paracrine signalling mechanism between bulk ER+ tumour cells and ER- BCSCs (Harrison et al., 2013).
Finally, Notch signalling has been connected to trastuzumab and lapatinib resistance in HER2+ breast cancer. Notch signalling is upregulated following trastuzumab or lapatinib treatment and HER2 positive cells have lower Notch transcriptional activity than HER2 negative cells (Osipo et al., 2008b; Abravanel et al., 2015). This is controlled by several mechanisms, including through HES1 and NRARP, but also through protein kinase C (PKC) α, which acts downstream of HER2 to restrict the availability of JAG1 for receptor binding (Abravanel et al., 2015). Interestingly, PKCα/Notch4 crosstalk has also been identified in endocrine therapy resistant ER+ breast cancers (Yun et al., 2013). Regardless, trastuzumab inhibits HER2, so trastuzumab treatment releases the block on Notch activation, enabling the cells to survive. Importantly, combining trastuzumab with a GSI potentiates the HER2-targeting treatment, and restores sensitivity to resistant cells (Osipo et al., 2008b; Pandya et al., 2016). Trastuzumab/GSI combination treatment also prevented breast tumour recurrence post-treatment insensitive orthotopic breast tumour xenografts (Pandya et al., 2011).
Breast Cancer Stem Cells
Notch-conferred therapy resistance is often accompanied by enrichment for breast cancer stem cells (BCSCs). BCSCs are defined as a subpopulation of cancer cells within the breast tumour, capable of both self-renewal and differentiation. They are purportedly responsible for tumour initiation, intratumoral heterogeneity and disease recurrence, and are more resistant to therapy than the rest of the tumour cell population. This puts them in particular focus for the development of novel breast cancer therapies, as ablation of BCSCs would result in tumour regression and eliminate risk of recurrence (Figure 6).
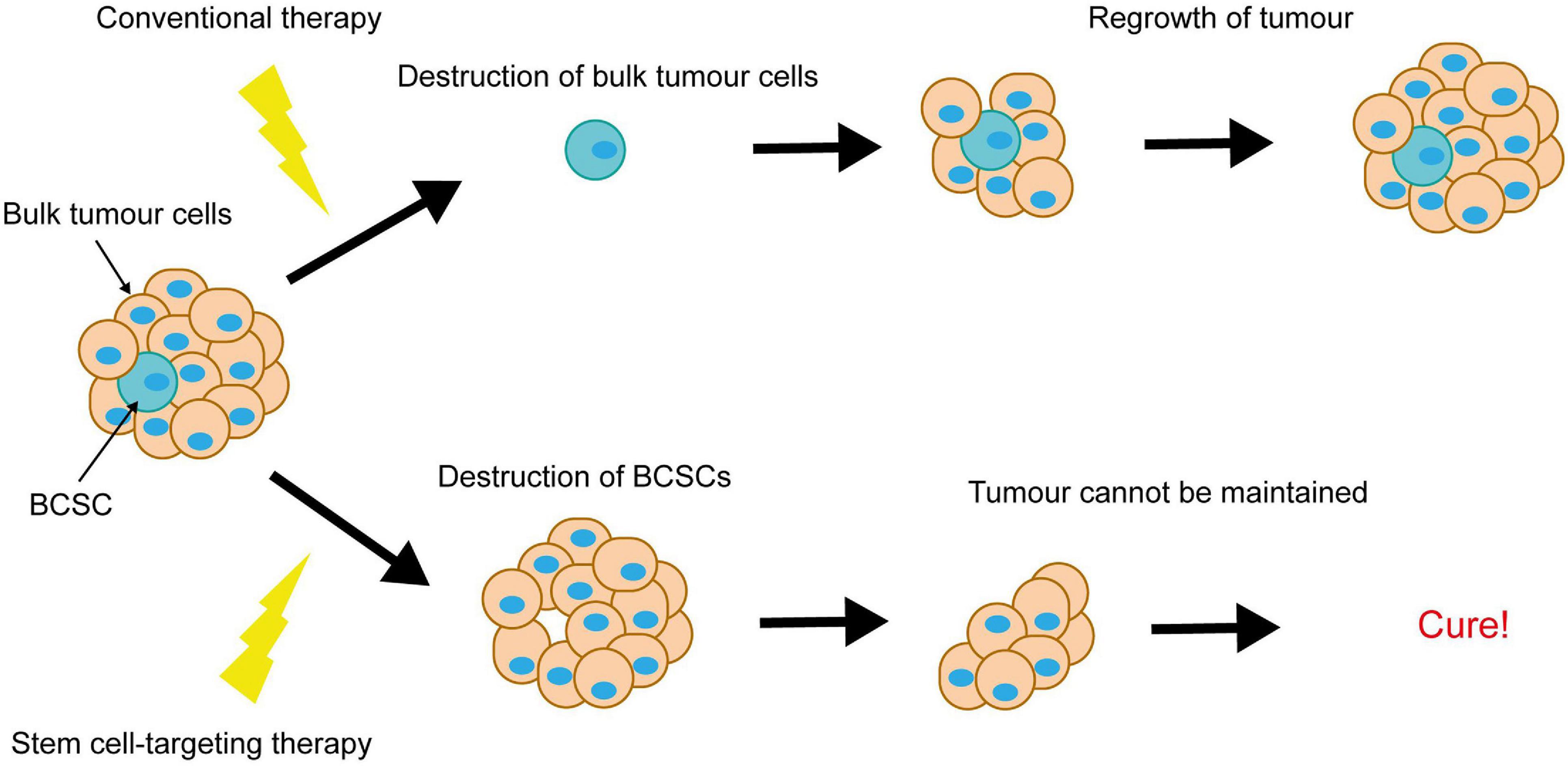
Figure 6. Elimination of breast cancer stem cells is key to achieving complete tumour regression. Conventional therapies destroy the bulk tumour cells, causing tumour regression, however resistant BCSCs survive and re-populate the tumour. Elimination of the BCSCs (even without immediate destruction of the bulk tumour cells) could induce complete tumour regression, as the tumour cells die off and are not replaced.
Notch signalling is implicated in BCSC self-renewal. Notch1 expression is positively correlated with ALDH positivity in breast tumour samples, and downregulation of Notch signalling in ALDH+ cells inhibits growth and induces apoptosis (Suman et al., 2013; Zhong et al., 2016). Breast cancer cells with high Notch activity are also more stem cell-enriched, and activation of Notch with DSL peptide increases mammosphere self-renewal in patient-derived samples (Dontu et al., 2004; D’Angelo et al., 2015; Mamaeva et al., 2016).
In line with its connection to endocrine therapy resistance, Notch4 appears to be the most important of the Notch receptors in breast cancer stem cells (Harrison et al., 2010). Notch4 expression is detected in secondary mammospheres, the basal layer in mammary gland tissue samples and is restricted to the terminal end bud (TEB) regions of mammary ducts (Dontu et al., 2004; Harrison et al., 2010). Notch4 blocking antibody treatment reduces primary mammosphere forming efficiency (MFE) and abolishes secondary mammosphere formation (Dontu et al., 2004; Farnie et al., 2007). Notch3 is also involved, with inhibition or ablation of Notch3 sufficient to reduce breast cancer cell mammosphere formation and self-renewal, and BCSC marker expression (Sansone et al., 2007a, b). Mechanistically, Notch signalling may enhance the expansion of BCSCs and/or progenitors through downstream cyclin D1 activity (Ling et al., 2010; Ling and Jolicoeur, 2013). Numerous factors have been identified upstream of Notch-induced BCSC activity including JNK, Ras, Pin1, HIF, cyclooxygenase 2 (COX2), syndecan-1 and BMP-4 (Mittal et al., 2014; Rustighi et al., 2014; Ibrahim et al., 2017; Xie et al., 2017; Yan et al., 2018; Choi et al., 2019). Notch/ZEB1 signalling has recently been implicated in the critical interaction between BCSCs and the tumour microenvironment. JAG1 expressed on endothelial cells in the tumour microenvironment activates Notch1 in adjacent BCSCs, resulting in ZEB1 induction and increased stemness. A positive feedback loop is formed as ZEB1 upregulates endothelial JAG1 via VEGFA (Jiang et al., 2020).
Evidence collected in these studies suggest that targeting Notch signalling as a part of breast cancer therapy may enable us to home in on BCSCs within the tumour cell population. In an exciting study, Mamaeva et al. (2016) developed mesoporous silica nanoparticles (MSNs, functionalised with glucose moieties) designed to specifically target breast cancer cells and BCSCs with γ-secretase inhibitors. They found that these DAPT loaded MSNs reduced the BCSC pool both in vitro and in vivo (Mamaeva et al., 2016). A note of caution however, lies in the heterogeneity of BCSCs and the implication on treatment efficacy. For example, one study identified two tumour initiating cell subsets within the BCSC population and demonstrated active Notch1 signalling in the more proliferative, invasive and metastatic subpopulation (CD44+/CD24low) but not the other (CD44+/CD24–). Concordantly, GSI treatment reduced mammosphere formation and tumour growth from CD44+/CD24low cells but not CD44+/CD24– cells (Azzam et al., 2013). These data warn that BCSC heterogeneity may limit the efficacy of GSI’s in breast cancer therapy. Despite this concern, studies have shown that Notch inhibitors can still successfully reduce the overall CD44high/CD24low/– subpopulation, and that this has phenotypic effect in mammosphere and re-implantation assays, particularly in combination with other agents (Qiu et al., 2013; Mittal et al., 2014; D’Angelo et al., 2015). Importantly, GSI treatment and Notch antibody blockade have been used to inhibit breast cancer cell secondary re-implantation tumour development, alone or in combination with docetaxel (Qiu et al., 2013; D’Angelo et al., 2015).
Crosstalk With ER and HER2 Signalling
A significant factor when considering the therapeutic value of targeting Notch signalling in the different subtypes of breast cancer, is the pathway’s crosstalk with ER and HER2 signalling. There is a clear correlation between aberrant Notch signalling and the triple negative phenotype, and multiple studies have identified roles for Notch signalling in TNBC that are not recapitulated in ER+ or HER2+ breast cancer (Lee et al., 2008a; Yamaguchi et al., 2008; Giuli et al., 2019). Notch/ER/HER2 crosstalk is also key in Notch-mediated resistance to endocrine and HER2-targeting therapy.
The Miele group have shown that Notch transcriptional activity is highest in ER- breast cancer cells, where inhibition of Notch signalling is effective in inducing cancer cell death in vitro. In contrast, in ER+ breast cancer cells, oestrogen inhibits Notch activity. This means that Notch activity is induced by endocrine therapy in the ER+ subtype, contributing to therapy resistance. Combining tamoxifen with a GSI resulted in significantly enhanced ER+ xenograft tumour regression compared to monotherapy, suggesting that combining Notch inhibitors with endocrine therapy may be a promising therapeutic strategy for ER+ breast cancer (Rizzo et al., 2008). Supporting this, mutation of ER in breast cancer stem cells induces Notch4 activity (Gelsomino et al., 2018). Similarly, HER2 suppresses Notch signalling in HER2+ breast cancer cells (Osipo et al., 2008b; Ju et al., 2013). HER2 regulates the activity of γ-secretase via ERK, and the nuclear translocation of NICD via Akt, in independent mechanisms (Ju et al., 2013).
Collectively, these studies suggest that in the absence of the growth-promoting pathways induced by ER and HER2, whether it be in the context of TNBC or cancers treated with anti-ER or HER2 therapies, Notch acts as a compensatory growth-promoting stimulus, enabling the cells to survive in the absence of these pathways (Figure 7).
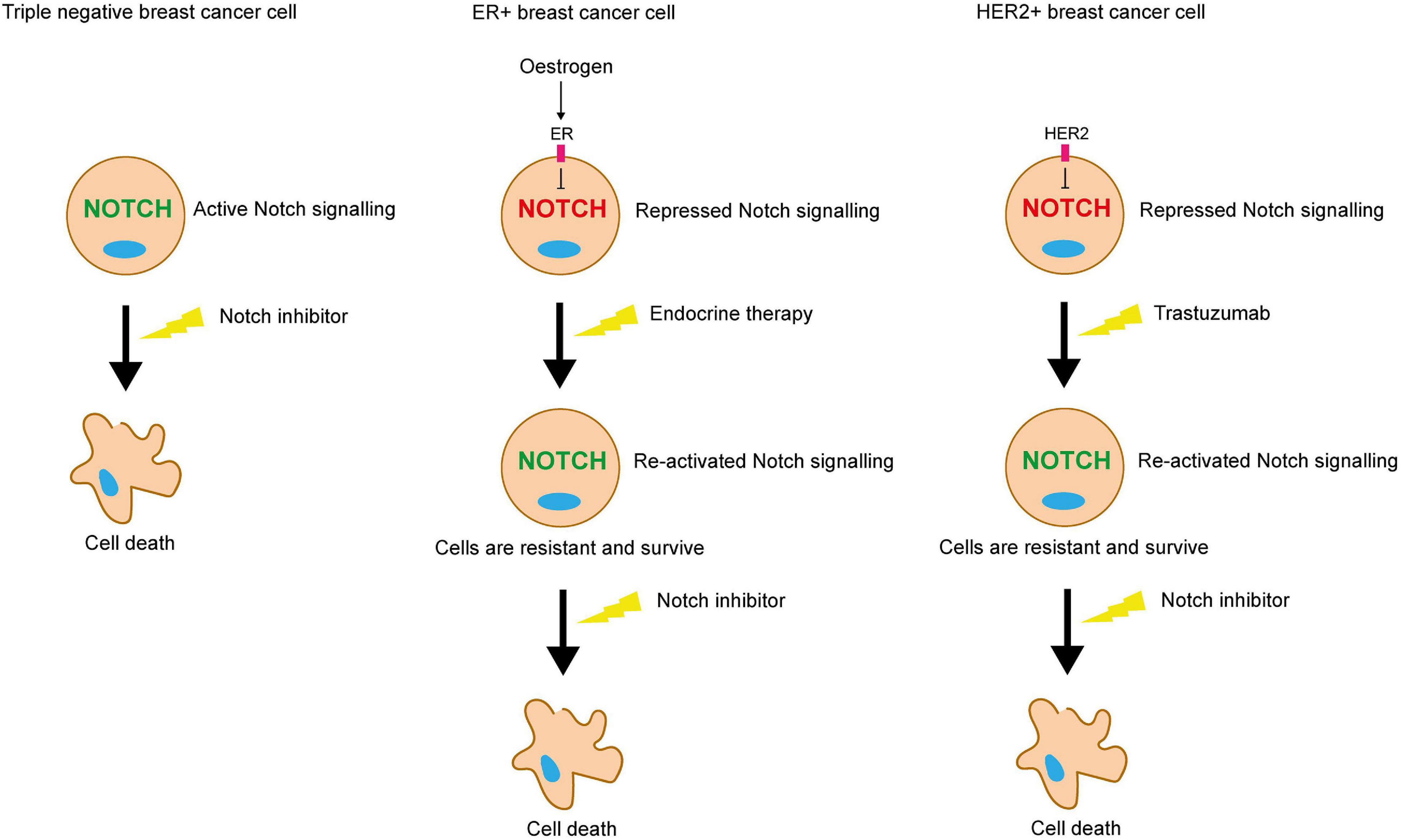
Figure 7. Notch inhibition may be a viable strategy for targeting therapy resistant breast cancer cells. ER and HER2 signalling inhibits Notch in ER+ and HER2+ breast cancer cells respectively. Endocrine or trastuzumab treatment inhibit these pathways, releasing the blockade on Notch signalling. Pro-survival Notch activity enables the cells to survive the targeted treatments. Notch inhibitors could be used in combination to sensitise these resistant cells to the targeted treatment. Triple negative breast cancer cells lack the ER and HER2 receptors, meaning that they are unaffected by endocrine therapy or trastuzumab, but are sensitive to Notch inhibitors.
Conversely, several studies contradict these findings and provide evidence that ER signalling promotes Notch signalling (Calaf and Roy, 2008; Kumar et al., 2019). Imperfect oestrogen response elements (EREs) have been detected in the Notch1 and JAG1 promoters, which translated to oestrogen-induced expression and increased signalling activity (Soares et al., 2004). Moreover, adding further complexity and implying the presence of regulatory feedback loops, Notch signalling can transactivate lower levels of the ER signalling pathway in the absence of oestrogen (Hao et al., 2010). RBPJκ binding sites have also been identified in the ERα promoter, suggesting that Notch can upregulate ERα expression (Dou et al., 2017). Likewise, Notch1 induces HER2 transcription in a RBPJκ-dependent mechanism (Chen et al., 1997).
Targeting Notch in Breast Cancer Therapy
Due to its multiple roles in breast tumorigenesis and therapy resistance, the Notch signalling pathway is an attractive therapeutic target. Many studies have shown that pharmacological Notch inhibition is effective both in vitro and in in vivo mouse models at inhibiting tumour growth, inducing tumour regression, preventing metastasis, targeting BCSCs and sensitising breast cancer cells to conventional therapies.
Many strategies for targeting the Notch signalling pathway have been developed and examples of currently available inhibitors are summarised in Figure 8. However, there are several issues that must be considered before these inhibitors are used clinically, beyond the obvious need to select patients with Notch-responsive tumours. These issues may explain why many clinical trials involving the use of Notch inhibitors have been put on hold or terminated due to toxicity or failure to reach trial endpoints, despite showing promise in pre-clinical studies (Table 1; Mollen et al., 2018).
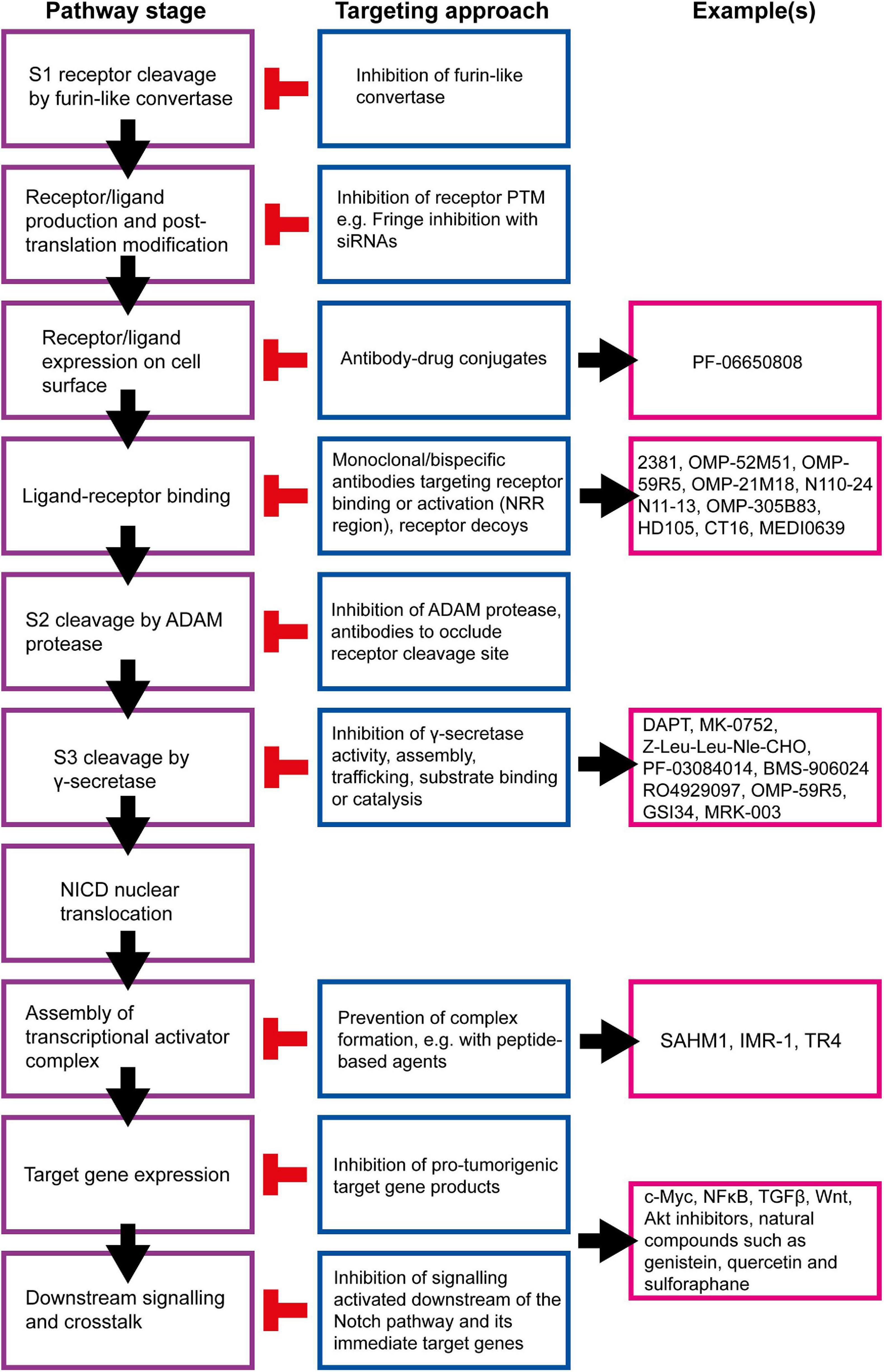
Figure 8. Summary of targetable points of the Notch pathway. The Notch signalling pathway can be inhibited at almost all stages, and a number of strategies are being developed to target these steps for therapeutic purposes (Andersson and Lendahl, 2014). γ-secretase inhibitors (GSIs) are the most well-established Notch inhibitors. Most competitively inhibit presenilin in the γ-secretase complex, and are hence pan Notch inhibitors that prevent all signalling events downstream of the Notch receptor regardless of receptor isoform or activating ligand (Krishna et al., 2019). The γ-secretase complex can also be targeted with monoclonal antibodies raised against presenilin or nicastrin (Hayashi et al., 2012; Takagi-Niidome et al., 2013; Zhang et al., 2014). Other pan Notch inhibitors include those that target the NICD/RBPJκ/MAML transcriptional activator complex. SAHM1 is a synthetic hydrocarbon-stapled α-helical peptide designed to mimic a portion of the N terminus of MAML. It competitively binds NICD/RBPJκ, preventing MAML binding (Moellering et al., 2009). Ligand-receptor binding is a popular target for current Notch inhibitor development. This can be achieved through receptor decoys, monoclonal antibodies, bispecific antibodies and antibody-drug conjugates (Noguera-Troise et al., 2006; Li L. et al., 2008; Hoey et al., 2009; Wu et al., 2010; Sharma et al., 2012; Li D. et al., 2014; Kangsamaksin et al., 2015; ClinicalTrials.gov., 2016, 2019a; Hidalgo et al., 2016; Lee et al., 2016; Cubillo Gracian et al., 2017; Hu et al., 2017; Lamy et al., 2017; Zheng et al., 2017; Hughes et al., 2018; Giuli et al., 2019; Jimeno et al., 2019; Smith et al., 2019; Rosen et al., 2020). Various natural compounds (and their derivatives) have been found to inhibit Notch signalling (Kawahara et al., 2009; Kallifatidis et al., 2011; Li Y. et al., 2012b; Pan et al., 2012; Xia et al., 2012; Nwaeburu et al., 2016; Sha et al., 2016; Mori et al., 2017; Castro et al., 2019; Li et al., 2020). These hold potential to be adapted and appropriated into cancer therapy.
Firstly, Notch signalling is a ubiquitous and essential developmental signalling pathway. It functions in normal tissue homeostasis throughout the body, meaning that systemic inhibition could have potentially harmful effects in healthy organs and mammary gland tissue. For example, long term γ-secretase inhibition has been shown to cause significant histopathologic changes in the gastrointestinal (GI) tract, including intestinal goblet cell metaplasia, apoptosis of small intestinal crypt epithelial cells, villous stunting, epithelial vacuolation and accumulation of intraluminal mucous (Milano et al., 2004). Another study found that long term anti-DLL4 antibody treatment in mice and rats caused highly significant histopathological defects in multiple organs including the liver and thymus. Most detrimentally, was the formation of ulcerating subcutaneous tumours with features of vascular neoplasms. Rarer necrotic lesions were also identified in the heart and lungs (Yan et al., 2010).
Secondly, targetable proteins within the Notch pathway are shared with other pathways important in normal cellular function. For instance, γ-secretase cleaves many other substrates including low density lipoprotein (LDL) receptor-related protein, E-cadherin, ErbB-4 and amyloid-β protein precursor (AβPP) (Haapasalo and Kovacs, 2011).
Thirdly, particularly in the case of cancer therapy, Notch functions as a tumour suppressor in certain contexts. This means that patients treated with systemic pan Notch inhibitors may be in danger of secondary tumour development, an unacceptable risk. Studies have found evidence for tumour suppressive Notch in a number of tissues, but this is most well characterised in the skin (Nicolas et al., 2003). Loss of function Notch1 mutations have been identified in squamous cell carcinoma, where they occur early on in tumorigenesis (Agrawal et al., 2011; Wang et al., 2011; South et al., 2014). The clinical significance of this was demonstrated in a phase III trial of the GSI semagacestat for Alzheimer’s disease. In addition to failing to slow disease progression, semagacestat increased the risk of skin cancer in the treated patient cohort (Extance, 2010). It may also be the case that Notch has contrasting roles within the same tissue, dependent on factors such as receptor isoform, the strength of activation signal and the presence or absence of regulators (Nowell and Radtke, 2017). Within breast cancer, the role of Notch2 has been controversial, with some studies finding that it has a tumour suppressive role, in direct contrast to the Notch1 and 4 isoforms (Parr et al., 2004; O’Neill et al., 2007). Similarly, Notch3 has been found to inhibit breast cancer cell EMT (Zhang X. et al., 2016; Lin et al., 2018; Wen et al., 2018).
There are a number of strategies that have been suggested for overcoming the gastrointestinal side effects associated with pan Notch inhibition. These include minimal dosing and intermittent administration to reduce the length of continuous treatment periods, which has shown some success (Krop et al., 2012). Z-Leu-Leu-Nle-CHO can be used at lower doses than conventional GSIs, as it is also anti-tumorigenic through negative regulation of the proteasome (Han et al., 2009). Moreover, nanoparticles could be utilised to specifically direct GSIs to the tumour, avoiding damage to healthy tissue (Mamaeva et al., 2016).
GSIs could be combined with conventional chemotherapy and targeted treatments. This has the dual benefit of minimising of the dose of each individual drug required (reducing toxicity), and enhancing overall treatment efficacy. Notch inhibition alone is unlikely to be sufficient to induce tumour regression, but shows promise in combination therapy (Meurette et al., 2009; Brennan and Clarke, 2013; Proia et al., 2015). In clinical trials, GSIs have been combined with conventional chemotherapeutics and endocrine therapy, as well as radiotherapy to help improve radiosensitivity and reach metastatic cells in hard to access areas (Table 1; Albain et al., 2010; ClinicalTrials.gov., 2016, 2019a,b; Lamy et al., 2017).
An alternative strategy to minimise the risk of side effects is to target specific Notch receptors or ligands. Monoclonal antibodies have been developed that bind to specific Notch receptor/ligand isoforms to inhibit receptor-ligand interaction, prevent processing by ADAM proteases, or induce inactivation via a conformational change of the receptor structure (Giuli et al., 2019; see Figure 8 for examples). These have shown promising results in pre-clinical studies, but have suffered end point failure in clinical trials (Noguera-Troise et al., 2006; Li L. et al., 2008; Hoey et al., 2009; Wu et al., 2010; Sharma et al., 2012; Li D. et al., 2014; ClinicalTrials.gov., 2016, 2019a; Hidalgo et al., 2016; Cubillo Gracian et al., 2017; Lamy et al., 2017; Zheng et al., 2017; Hughes et al., 2018; Giuli et al., 2019; Smith et al., 2019). Bispecific antibodies and antibody-drug conjugates may be able to improve on the limited success of monoclonal antibodies. Bispecific antibodies can be used to target other oncogenic signalling pathways simultaneously, which has been proven to be more efficacious than administering two separate monoclonal antibodies (Lee et al., 2016; Hu et al., 2017; Jimeno et al., 2019). Antibody-drug conjugates are designed to target cancer cells with potent cytotoxic drugs while minimising damage to surrounding healthy cells and tissues, reducing side effect risk and severity. For example, PF-06650808 is a novel anti-Notch3-auristatin conjugate that has demonstrated manageable toxicity and signs of anti-tumour activity in breast cancer patients. The anti-Notch3 component binds the agent to Notch3-expressing tumour cells, where it is internalised and trafficked to vesicles containing proteolytic enzymes. These enzymes cleave the linker connecting the two components, releasing the auristatin-based payload into the cytoplasm where it induces cell cycle arrest and apoptosis (Rosen et al., 2020).
Recent research has begun investigating whether Notch signalling could be harnessed in immunotherapy-based cancer treatment. Notch is important in lineage determination in the haematopoietic system where it helps to direct the differentiation of CD4+ T helper cells into TH1 and TH2 subsets. The TH1 response is generally considered to be anti-tumorigenic, meaning that if Notch could be very specifically and carefully activated to induce the TH1 response, then immune cell anti-cancer activity could be increased (Nowell and Radtke, 2017). For instance, Kondo et al. (2017) generated induced stem cell memory T (iTSCM) cells with potent anti-tumour activity from activated CD4+ and CD8+ T cells by co-culture with stromal cells expressing DLL1. In these circumstances, systemically inhibiting Notch signalling would be a hindrance rather than a help.
Discussion
Over the last 20 years, it has become abundantly clear that Notch signalling plays an important role in both the development of the mammary gland and the aetiology of breast cancer. Within the normal mammary gland, Notch signalling is important in driving multipotent foetal MaSCs into the unipotent luminal progenitor cell fate and in maintaining the progenitor fate through puberty and adult life. In breast cancer, elevated Notch signalling is seen in all cancers but it is particularly associated with TNBC and cancers that show therapy resistance where elevated Notch signalling is associated with poor prognosis.
Given the role Notch signalling plays in lineage commitment within the normal mammary gland as well as in the self-renewal of breast cancer stem cells, it is worth speculating that the majority of breast cancers arise from the unipotent luminal progenitor cells. This is in keeping with several studies looking at the cell-of-origin of breast cancers. Firstly, Molyneux et al. (2010) and Melchor et al. (2014) elegantly demonstrated that the loss of BRCA1 within luminal progenitors in mice leads to the development of basal-like tumours and subsequently, depending on the initiating genetic insult, that luminal-like, basal-like and normal-like tumours can all arise from luminal progenitors. Secondly, the cell-of-origin for the luminal-like tumours that arise in MMTV-PyMT and MMTV-Neu mice and the basal-like tumours that arise in Etv6-NTRK3 mice were all found to be luminal progenitor cells (Tao et al., 2015). Lastly, transforming luminal cells isolated from normal human tissue by virally introducing a variety of oncogenes leads to the formation of both ER+ve and ER-ve breast cancers when the cells are transplanted into immunocompromised humanised mice (Proia et al., 2011; Keller et al., 2012). Given these observations, it would be interesting to know whether specifically activating Notch signalling within different cells of the luminal lineage is enough to convert the cells into a progenitor-like fate and initiate tumorigenesis.
It is also interesting to note that mature luminal cells and luminal progenitor cells are arranged in mosaic patterns within the mature mammary ductal epithelium similar to those generated by mathematical modelling of Notch signalling (Hadjivasiliou et al., 2016; Bocci et al., 2020; Dawson and Visvader, 2021). These patterns are particularly associated with lateral induction by Notch/JAG signalling which fits well with known expression of JAG1 in the mammary ductal epithelium (Raafat et al., 2011; Xu et al., 2012; Bocci et al., 2020). The role of Notch/JAG signalling within the pattern of mature luminal cells and luminal progenitor cells could be addressed by disrupting signalling specifically within the mature mammary gland.
The involvement of Notch signalling in resistance to all types of breast cancer treatment, including chemotherapy, radiotherapy, endocrine and HER2-targeting therapies, means that Notch inhibition could be valuable in a broad range of patient groups. However, to date it has proven difficult to target Notch signalling in patients. Pan Notch inhibitors have led to unacceptable side effects, whilst we have most likely failed to stratify patients appropriately for homologue-specific Notch inhibitors to be successful. However stratification to signalling through individual Notch proteins may make identifying appropriate patients for therapy too complex. An alternative approach could be to target one of the signalling events downstream of all Notch homologues. Notch activates numerous pro-tumorigenic signalling pathways, some of which have pre-existing inhibitors prime for re-appropriation into breast cancer therapy. Ideally, a pathway would be targeted that has roles in multiple hallmarks of breast cancer, but in particular apoptosis given the role Notch signalling has in cell survival and the enrichment of BCSCs observed following therapy.
Author Contributions
AE and KB contributed to the systematic review of the literature required for this article, and the writing and editing of the final text. Both authors contributed to the article and approved the submitted version.
Funding
AE was supported by Cancer Research UK via funding to the Cancer Research UK Manchester Centre (C147/A25254) and their Non-Clinical Training Programme.
Conflict of Interest
The authors declare that the research was conducted in the absence of any commercial or financial relationships that could be construed as a potential conflict of interest.
Acknowledgments
AE and KB would like to thank the past and current members of the Brennan, Gilmore, Streuli, Clarke, Swift and Ucar laboratories for the discussions that helped shape the ideas within this review article.
References
Abravanel, D. L., Belka, G. K., Pan, T. C., Pant, D. K., Collins, M. A., Sterner, C. J., et al. (2015). Notch promotes recurrence of dormant tumor cells following HER2/neu-targeted therapy. J. Clin. Invest. 125, 2484–2496. doi: 10.1172/JCI74883
Acar, A., Simões, B. M., Clarke, R. B., and Brennan, K. (2016). A role for notch signalling in breast cancer and endocrine resistance. Stem Cells Int. 2016:2498764. doi: 10.1155/2016/2498764
Agrawal, N., Frederick, M. J., Pickering, C. R., Bettegowda, C., Chang, K., Li, R. J., et al. (2011). Exome sequencing of head and neck squamous cell carcinoma reveals inactivating mutations in NOTCH1. Science 333, 1154–1157. doi: 10.1126/science.1206923
Albain, K., Czerlanis, C., Rajan, P., Zlobin, A., Godellas, C., Bova, D., et al. (2010). Abstract PD05-12: Combination of Notch Inhibitor MK-0752 and Endocrine Therapy for Early Stage ERα + Breast Cancer in a Presurgical Window Pilot Study. Thirty-Third Annual CTRC-AACR San Antonio Breast Cancer Symposium. San Antonio, TX: American Association for Cancer Research.
Amsen, D., Antov, A., Jankovic, D., Sher, A., Radtke, F., Souabni, A., et al. (2007). Direct regulation of Gata3 expression determines the T helper differentiation potential of Notch. Immunity 27, 89–99. doi: 10.1016/j.immuni.2007.05.021
Andersson, E. R., and Lendahl, U. (2014). Therapeutic modulation of Notch signalling–are we there yet? Nat. Rev. Drug Discov. 13, 357–378. doi: 10.1038/nrd4252
Andersson, E. R., Sandberg, R., and Lendahl, U. (2011). Notch signaling: simplicity in design, versatility in function. Development 138, 3593–3612. doi: 10.1242/dev.063610
Aster, J. C., Pear, W. S., and Blacklow, S. C. (2017). The varied roles of notch in cancer. Annu. Rev. Pathol. 12, 245–275. doi: 10.1146/annurev-pathol-052016-100127
Ayyanan, A., Civenni, G., Ciarloni, L., Morel, C., Mueller, N., Lefort, K., et al. (2006). Increased Wnt signaling triggers oncogenic conversion of human breast epithelial cells by a Notch-dependent mechanism. Proc. Natl. Acad. Sci. U S A 103, 3799–3804. doi: 10.1073/pnas.0600065103
Azaro, A., Baldini, C., Rodon, J., Soria, J. C., Yuen, E., Lithio, A., et al. (2021a). Phase 1 study of 2 high dose intensity schedules of the pan-Notch inhibitor crenigacestat (LY3039478) in combination with prednisone in patients with advanced or metastatic cancer. Invest. New Drugs 39, 193–201. doi: 10.1007/s10637-020-00944-z
Azaro, A., Massard, C., Tap, W. D., Cassier, P. A., Merchan, J., Italiano, A., et al. (2021b). A phase 1b study of the Notch inhibitor crenigacestat (LY3039478) in combination with other anticancer target agents (taladegib, LY3023414, or abemaciclib) in patients with advanced or metastatic solid tumors. Invest. New Drugs 2021:6. doi: 10.1007/s10637-021-01094-6
Azzam, D. J., Zhao, D., Sun, J., Minn, A. J., Ranganathan, P., Drews-Elger, K., et al. (2013). Triple negative breast cancer initiating cell subsets differ in functional and molecular characteristics and in γ-secretase inhibitor drug responses. EMBO Mol. Med. 5, 1502–1522. doi: 10.1002/emmm.201302558
Bhola, N. E., Jansen, V. M., Koch, J. P., Li, H., Formisano, L., Williams, J. A., et al. (2016). Treatment of triple-negative breast cancer with TORC1/2 inhibitors sustains a drug-resistant and notch-dependent cancer stem cell population. Cancer Res. 76, 440–452. doi: 10.1158/0008-5472.CAN-15-1640-T
Bivik, C., MacDonald, R. B., Gunnar, E., Mazouni, K., Schweisguth, F., and Thor, S. (2016). Control of neural daughter cell proliferation by multi-level notch/Su(H)/E(spl)-HLH signaling. PLoS Genet. 12:e1005984. doi: 10.1371/journal.pgen.1005984
Bocci, F., Gearhart-Serna, L., Boareto, M., Ribeiro, M., Ben-Jacob, E., Devi, G. R., et al. (2019). Toward understanding cancer stem cell heterogeneity in the tumor microenvironment. Proc. Natl. Acad. Sci. U S A 116, 148–157. doi: 10.1073/pnas.1815345116
Bocci, F., Onuchic, J. N., and Jolly, M. K. (2020). Understanding the principles of pattern formation driven by notch signaling by integrating experiments and theoretical models. Front. Physiol. 11:929. doi: 10.3389/fphys.2020.00929
Boelens, M. C., Wu, T. J., Nabet, B. Y., Xu, B., Qiu, Y., Yoon, T., et al. (2014). Exosome transfer from stromal to breast cancer cells regulates therapy resistance pathways. Cell 159, 499–513. doi: 10.1016/j.cell.2014.09.051
Bolós, V., Mira, E., Martínez-Poveda, B., Luxán, G., Cañamero, M., Martínez-A, C., et al. (2013). Notch activation stimulates migration of breast cancer cells and promotes tumor growth. Breast Cancer Res. 15:R54. doi: 10.1186/bcr3447
Borggrefe, T., and Oswald, F. (2009). The Notch signaling pathway: transcriptional regulation at Notch target genes. Cell Mol. Life Sci. 66, 1631–1646. doi: 10.1007/s00018-009-8668-7
Bouras, T., Pal, B., Vaillant, F., Harburg, G., Asselin-Labat, M. L., Oakes, S. R., et al. (2008). Notch signaling regulates mammary stem cell function and luminal cell-fate commitment. Cell Stem Cell 3, 429–441. doi: 10.1016/j.stem.2008.08.001
Brabletz, S., Bajdak, K., Meidhof, S., Burk, U., Niedermann, G., Firat, E., et al. (2011). The ZEB1/miR-200 feedback loop controls Notch signalling in cancer cells. EMBO J. 30, 770–782. doi: 10.1038/emboj.2010.349
Bray, S. J. (2006). Notch signalling: a simple pathway becomes complex. Nat. Rev. Mol. Cell Biol. 7, 678–689. doi: 10.1038/nrm2009
Bray, S. J. (2016). Notch signalling in context. Nat. Rev. Mol. Cell Biol. 17, 722–735. doi: 10.1038/nrm.2016.94
Brennan, K., and Clarke, R. B. (2013). Combining Notch inhibition with current therapies for breast cancer treatment. Ther. Adv. Med. Oncol. 5, 17–24. doi: 10.1177/1758834012457437
Brou, C., Logeat, F., Gupta, N., Bessia, C., LeBail, O., Doedens, J. R., et al. (2000). A novel proteolytic cleavage involved in notch signaling: the role of the disintegrin-metalloprotease TACE. Mol. Cell 5, 207–216. doi: 10.1016/s1097-2765(00)80417-7
Buono, K. D., Robinson, G. W., Martin, C., Shi, S., Stanley, P., Tanigaki, K., et al. (2006). The canonical Notch/RBP-J signaling pathway controls the balance of cell lineages in mammary epithelium during pregnancy. Dev. Biol. 293, 565–580. doi: 10.1016/j.ydbio.2006.02.043
Calaf, G. M., and Roy, D. (2008). Cell adhesion proteins altered by 17beta estradiol and parathion in breast epithelial cells. Oncol. Rep. 19, 165–169.
Castel, D., Mourikis, P., Bartels, S. J., Brinkman, A. B., Tajbakhsh, S., and Stunnenberg, H. G. (2013). Dynamic binding of RBPJ is determined by Notch signaling status. Genes Dev. 27, 1059–1071. doi: 10.1101/gad.211912.112
Castro, N. P., Fedorova-Abrams, N. D., Merchant, A. S., Rangel, M. C., Nagaoka, T., Karasawa, H., et al. (2015). Cripto-1 as a novel therapeutic target for triple negative breast cancer. Oncotarget 6, 11910–11929. doi: 10.18632/oncotarget.4182
Castro, N. P., Rangel, M. C., Merchant, A. S., MacKinnon, G., Cuttitta, F., Salomon, D. S., et al. (2019). Sulforaphane suppresses the growth of triple-negative breast cancer stem-like cells. Cancer Prev. Res. (Phila) 12, 147–158. doi: 10.1158/1940-6207.CAPR-18-0241
Centonze, A., Lin, S., Tika, E., Sifrim, A., Fioramonti, M., Malfait, M., et al. (2020). Heterotypic cell-cell communication regulates glandular stem cell multipotency. Nature 584, 608–613. doi: 10.1038/s41586-020-2632-y
Chakrabarti, R., Celià-Terrassa, T., Kumar, S., Hang, X., Wei, Y., Choudhury, A., et al. (2018). Notch ligand Dll1 mediates cross-talk between mammary stem cells and the macrophageal niche. Science 360:aan4153. doi: 10.1126/science.aan4153
Chao, C. H., Chang, C. C., Wu, M. J., Ko, H. W., Wang, D., Hung, M. C., et al. (2014). MicroRNA-205 signaling regulates mammary stem cell fate and tumorigenesis. J. Clin. Invest. 124, 3093–3106. doi: 10.1172/JCI73351
Chen, J., Imanaka, N., and Griffin, J. D. (2010). Hypoxia potentiates Notch signaling in breast cancer leading to decreased E-cadherin expression and increased cell migration and invasion. Br. J. Cancer 102, 351–360. doi: 10.1038/sj.bjc.6605486
Chen, Y., Fischer, W. H., and Gill, G. N. (1997). Regulation of the ERBB-2 promoter by RBPJkappa and NOTCH. J. Biol. Chem. 272, 14110–14114. doi: 10.1074/jbc.272.22.14110
Choi, S., Yu, J., Park, A., Dubon, M. J., Do, J., Kim, Y., et al. (2019). BMP-4 enhances epithelial mesenchymal transition and cancer stem cell properties of breast cancer cells via Notch signaling. Sci. Rep. 9:11724. doi: 10.1038/s41598-019-48190-5
Choy, L., Hagenbeek, T. J., Solon, M., French, D., Finkle, D., Shelton, A., et al. (2017). Constitutive NOTCH3 signaling promotes the growth of basal breast cancers. Cancer Res. 77, 1439–1452. doi: 10.1158/0008-5472.CAN-16-1022
Clarke, R. B., Howell, A., Potten, C. S., and Anderson, E. (1997). Dissociation between steroid receptor expression and cell proliferation in the human breast. Cancer Res. 57, 4987–4991.
ClinicalTrials.gov. (2016). A Phase 1b/2 Study of OMP-59R5 in Combination With Nab-Paclitaxel and Gemcitabine in Subjects With Previously Untreated Stage IV Pancreatic Cancer (ALPINE). Available Online at: https://clinicaltrials.gov/ct2/show/study/NCT01647828
ClinicalTrials.gov. (2019a). A Phase 1b/2 Study of OMP-59R5 (Tarextumab) in Combination With Etoposide and Platinum Therapy (PINNACLE). Available Online at: https://clinicaltrials.gov/ct2/show/study/NCT01859741
ClinicalTrials.gov. (2019b). RO4929097 and Whole-Brain Radiation Therapy or Stereotactic Radiosurgery in Treating Patients With Brain Metastases From Breast Cancer. Available Online at: https://clinicaltrials.gov/ct2/show/NCT01217411
Cohen, B., Shimizu, M., Izrailit, J., Ng, N. F., Buchman, Y., Pan, J. G., et al. (2010). Cyclin D1 is a direct target of JAG1-mediated Notch signaling in breast cancer. Breast Cancer Res. Treat 123, 113–124. doi: 10.1007/s10549-009-0621-9
Colaluca, I. N., Tosoni, D., Nuciforo, P., Senic-Matuglia, F., Galimberti, V., Viale, G., et al. (2008). NUMB controls p53 tumour suppressor activity. Nature 451, 76–80. doi: 10.1038/nature06412
Cubillo Gracian, A., Dean, A., Munoz, A., Hidalgo, M., Pazo-Cid, R., Martin, M., et al. (2017). YOSEMITE: A 3 Arm Double-Blind Randomized Phase 2 Study of Gemcitabine, Paclitaxel Protein-Bound Particles for Injectable Suspension (Abraxane§) and Placebo (GAP) versus Gemcitabine, Abraxane§and either 1 or 2 Truncated Courses of Demcizumab (GAD). Amsterdam: Elsevier Inc.
D’Angelo, R. C., Ouzounova, M., Davis, A., Choi, D., Tchuenkam, S. M., Kim, G., et al. (2015). Notch reporter activity in breast cancer cell lines identifies a subset of cells with stem cell activity. Mol. Cancer Ther. 14, 779–787. doi: 10.1158/1535-7163.MCT-14-0228
Dawson, C. A., and Visvader, J. E. (2021). The cellular organization of the mammary gland: insights from microscopy. J. Mammary Gland Biol. Neoplasia 2021:e9483–6. doi: 10.1007/s10911-021-09483-6
De Strooper, B., Annaert, W., Cupers, P., Saftig, P., Craessaerts, K., Mumm, J. S., et al. (1999). A presenilin-1-dependent gamma-secretase-like protease mediates release of notch intracellular domain. Nature 398, 518–522. doi: 10.1038/19083
Deftos, M. L., Huang, E., Ojala, E. W., Forbush, K. A., and Bevan, M. J. (2000). Notch1 signaling promotes the maturation of CD4 and CD8 SP thymocytes. Immunity 13, 73–84. doi: 10.1016/s1074-7613(00)00009-1
Diluvio, G., Del Gaudio, F., Giuli, M. V., Franciosa, G., Giuliani, E., Palermo, R., et al. (2018). NOTCH3 inactivation increases triple negative breast cancer sensitivity to gefitinib by promoting EGFR tyrosine dephosphorylation and its intracellular arrest. Oncogenesis 7:42. doi: 10.1038/s41389-018-0051-9
Dontu, G., Jackson, K. W., McNicholas, E., Kawamura, M. J., Abdallah, W. M., and Wicha, M. S. (2004). Role of Notch signaling in cell-fate determination of human mammary stem/progenitor cells. Breast Cancer Res. 6, 605–615. doi: 10.1186/bcr920
Dou, X. W., Liang, Y. K., Lin, H. Y., Wei, X. L., Zhang, Y. Q., Bai, J. W., et al. (2017). Notch3 maintains luminal phenotype and suppresses tumorigenesis and metastasis of breast cancer via trans-activating estrogen receptor-α. Theranostics 7, 4041–4056. doi: 10.7150/thno.19989
Du, R., Sun, W., Xia, L., Zhao, A., Yu, Y., Zhao, L., et al. (2012). Hypoxia-induced down-regulation of microRNA-34a promotes EMT by targeting the Notch signaling pathway in tubular epithelial cells. PLoS One 7:e30771. doi: 10.1371/journal.pone.0030771
Efferson, C. L., Winkelmann, C. T., Ware, C., Sullivan, T., Giampaoli, S., Tammam, J., et al. (2010). Downregulation of Notch pathway by a gamma-secretase inhibitor attenuates AKT/mammalian target of rapamycin signaling and glucose uptake in an ERBB2 transgenic breast cancer model. Cancer Res. 70, 2476–2484. doi: 10.1158/0008-5472.CAN-09-3114
El-Khoueiry, A. B., Desai, J., Padmanabhan Iyer, S., Gadgeel, S. M., Ramalingam, S. S., Horn, L., et al. (2018). A phase I study of AL101, a pan-NOTCH inhibitor, in patients (pts) with locally advanced or metastatic solid tumors. J. Clin. Oncol. 36:1. doi: 10.1200/JCO.2018.36.15_suppl.2515
Extance, A. (2010). Alzheimer’s failure raises questions about disease-modifying strategies. Nat. Rev. Drug Discov. 9, 749–751. doi: 10.1038/nrd3288
Fang, T. C., Yashiro-Ohtani, Y., Del Bianco, C., Knoblock, D. M., Blacklow, S. C., and Pear, W. S. (2007). Notch directly regulates Gata3 expression during T helper 2 cell differentiation. Immunity 27, 100–110. doi: 10.1016/j.immuni.2007.04.018
Farnie, G., Clarke, R. B., Spence, K., Pinnock, N., Brennan, K., Anderson, N. G., et al. (2007). Novel cell culture technique for primary ductal carcinoma in situ: role of Notch and epidermal growth factor receptor signaling pathways. J. Natl. Cancer Inst. 99, 616–627. doi: 10.1093/jnci/djk133
Fazio, C., and Ricciardiello, L. (2016). Inflammation and Notch signaling: a crosstalk with opposite effects on tumorigenesis. Cell Death Dis. 7:e2515. doi: 10.1038/cddis.2016.408
Ferrarotto, R., Eckhardt, G., Patnaik, A., LoRusso, P., Faoro, L., Heymach, J. V., et al. (2018). A phase I dose-escalation and dose-expansion study of brontictuzumab in subjects with selected solid tumors. Ann. Oncol. 29, 1561–1568. doi: 10.1093/annonc/mdy171
Fortini, M. E. (2009). Notch signaling: the core pathway and its posttranslational regulation. Dev. Cell 16, 633–647. doi: 10.1016/j.devcel.2009.03.010
García-Heredia, J. M., Verdugo Sivianes, E. M., Lucena-Cacace, A., Molina-Pinelo, S., and Carnero, A. (2016). Numb-like (NumbL) downregulation increases tumorigenicity, cancer stem cell-like properties and resistance to chemotherapy. Oncotarget 7, 63611–63628. doi: 10.18632/oncotarget.11553
Garg, H., Suri, P., Gupta, J. C., Talwar, G. P., and Dubey, S. (2016). Survivin: a unique target for tumor therapy. Cancer Cell Int. 16:49. doi: 10.1186/s12935-016-0326-1
Gelsomino, L., Panza, S., Giordano, C., Barone, I., Gu, G., Spina, E., et al. (2018). Mutations in the estrogen receptor alpha hormone binding domain promote stem cell phenotype through notch activation in breast cancer cell lines. Cancer Lett. 428, 12–20. doi: 10.1016/j.canlet.2018.04.023
Giuli, M. V., Giuliani, E., Screpanti, I., Bellavia, D., and Checquolo, S. (2019). Notch signaling activation as a hallmark for triple-negative breast cancer subtype. J. Oncol. 2019:8707053. doi: 10.1155/2019/8707053
Guo, S., Liu, M., and Gonzalez-Perez, R. R. (2011). Role of Notch and its oncogenic signaling crosstalk in breast cancer. Biochim. Biophys. Acta 1815, 197–213. doi: 10.1016/j.bbcan.2010.12.002
Haapasalo, A., and Kovacs, D. M. (2011). The many substrates of presenilin/γ-secretase. J. Alzheimers Dis. 25, 3–28. doi: 10.3233/JAD-2011-101065
Hadjivasiliou, Z., Hunter, G. L., and Baum, B. (2016). A new mechanism for spatial pattern formation via lateral and protrusion-mediated lateral signalling. J. R. Soc. Interface 13:484. doi: 10.1098/rsif.2016.0484
Han, J., Ma, I., Hendzel, M. J., and Allalunis-Turner, J. (2009). The cytotoxicity of gamma-secretase inhibitor I to breast cancer cells is mediated by proteasome inhibition, not by gamma-secretase inhibition. Breast Cancer Res. 11:R57. doi: 10.1186/bcr2347
Hao, L., Rizzo, P., Osipo, C., Pannuti, A., Wyatt, D., Cheung, L. W., et al. (2010). Notch-1 activates estrogen receptor-alpha-dependent transcription via IKKalpha in breast cancer cells. Oncogene 29, 201–213. doi: 10.1038/onc.2009.323
Harrison, H., Farnie, G., Howell, S. J., Rock, R. E., Stylianou, S., Brennan, K. R., et al. (2010). Regulation of breast cancer stem cell activity by signaling through the Notch4 receptor. Cancer Res. 70, 709–718. doi: 10.1158/0008-5472.CAN-09-1681
Harrison, H., Simões, B. M., Rogerson, L., Howell, S. J., Landberg, G., and Clarke, R. B. (2013). Oestrogen increases the activity of oestrogen receptor negative breast cancer stem cells through paracrine EGFR and Notch signalling. Breast Cancer Res. 15:R21. doi: 10.1186/bcr3396
Hartman, J., Lam, E. W., Gustafsson, J. A., and Ström, A. (2009). Hes-6, an inhibitor of Hes-1, is regulated by 17beta-estradiol and promotes breast cancer cell proliferation. Breast Cancer Res. 11:R79. doi: 10.1186/bcr2446
Harvey, B. M., and Haltiwanger, R. S. (2018). Regulation of notch function by O-Glycosylation. Adv. Exp. Med. Biol. 1066, 59–78. doi: 10.1007/978-3-319-89512-3_4
Hayashi, I., Takatori, S., Urano, Y., Miyake, Y., Takagi, J., Sakata-Yanagimoto, M., et al. (2012). Neutralization of the γ-secretase activity by monoclonal antibody against extracellular domain of nicastrin. Oncogene 31, 787–798. doi: 10.1038/onc.2011.265
Hidalgo, M., Cooray, P., Carrato, A., Jameson, M. B., Parnis, F., Jeffery, M., et al. (2016). A phase 1b study of the anti-cancer stem cell agent demcizumab (DEM) and gemcitabine (GEM) +/- nab-paclitaxel in patients with pancreatic cancer. J. Clin. Oncol. 34:341. doi: 10.1200/jco.2016.34.4_suppl.341
Hoey, T., Yen, W. C., Axelrod, F., Basi, J., Donigian, L., Dylla, S., et al. (2009). DLL4 blockade inhibits tumor growth and reduces tumor-initiating cell frequency. Cell Stem Cell 5, 168–177. doi: 10.1016/j.stem.2009.05.019
Hori, K., Sen, A., and Artavanis-Tsakonas, S. (2013). Notch signaling at a glance. J. Cell Sci. 126, 2135–2140. doi: 10.1242/jcs.127308
Hossain, F., Sorrentino, C., Ucar, D. A., Peng, Y., Matossian, M., Wyczechowska, D., et al. (2018). Notch signaling regulates mitochondrial metabolism and NF-κB activity in triple-negative breast cancer cells via IKKα-dependent Non-canonical pathways. Front. Oncol. 8:575. doi: 10.3389/fonc.2018.00575
Hu, C., Diévart, A., Lupien, M., Calvo, E., Tremblay, G., and Jolicoeur, P. (2006). Overexpression of activated murine Notch1 and Notch3 in transgenic mice blocks mammary gland development and induces mammary tumors. Am. J. Pathol. 168, 973–990. doi: 10.2353/ajpath.2006.050416
Hu, S., Fu, W., Li, T., Yuan, Q., Wang, F., Lv, G., et al. (2017). Antagonism of EGFR and Notch limits resistance to EGFR inhibitors and radiation by decreasing tumor-initiating cell frequency. Sci. Transl. Med. 9:eaag0339. doi: 10.1126/scitranslmed.aag0339
Huenniger, K., Krämer, A., Soom, M., Chang, I., Köhler, M., Depping, R., et al. (2010). Notch1 signaling is mediated by importins alpha 3, 4, and 7. Cell Mol. Life Sci. 67, 3187–3196. doi: 10.1007/s00018-010-0378-7
Hughes, B., Dean, A., Markman, B., Dreisbach, L., Provencio, M., Ciuffreda, L., et al. (2018). Abstract A084: DENALI: a 3-arm double-blind randomized phase 2 study of carboplatin, pemetrexed, and placebo (CPP) versus carboplatin, pemetrexed, and either 1 or 2 truncated courses of demcizumab (CPD) in patients with non-squamous non-small cell lung cancer (NSCLC). AACR-NCI-EORTC International Conference: Molecular Targets and Cancer Therapeutics; 26th-30th October 2017. Philadelphia, PA: American Association for Cancer Research.
Ibrahim, S. A., Gadalla, R., El-Ghonaimy, E. A., Samir, O., Mohamed, H. T., Hassan, H., et al. (2017). Syndecan-1 is a novel molecular marker for triple negative inflammatory breast cancer and modulates the cancer stem cell phenotype via the IL-6/STAT3, Notch and EGFR signaling pathways. Mol. Cancer 16:57. doi: 10.1186/s12943-017-0621-z
Imatani, A., and Callahan, R. (2000). Identification of a novel NOTCH-4/INT-3 RNA species encoding an activated gene product in certain human tumor cell lines. Oncogene 19, 223–231. doi: 10.1038/sj.onc.1203295
Inman, J. L., Robertson, C., Mott, J. D., and Bissell, M. J. (2015). Mammary gland development: cell fate specification, stem cells and the microenvironment. Development 142, 1028–1042. doi: 10.1242/dev.087643
Jarriault, S., Brou, C., Logeat, F., Schroeter, E. H., Kopan, R., and Israel, A. (1995). Signalling downstream of activated mammalian notch. Nature 377, 355–358. doi: 10.1038/377355a0
Jiang, H., Zhou, C., Zhang, Z., Wang, Q., Wei, H., Shi, W., et al. (2020). Jagged1-Notch1-deployed tumor perivascular niche promotes breast cancer stem cell phenotype through Zeb1. Nat. Commun. 11:5129. doi: 10.1038/s41467-020-18860-4
Jimeno, A., Moore, K. N., Gordon, M., Chugh, R., Diamond, J. R., Aljumaily, R., et al. (2019). A first-in-human phase 1a study of the bispecific anti-DLL4/anti-VEGF antibody navicixizumab (OMP-305B83) in patients with previously treated solid tumors. Invest. New Drugs 37, 461–472. doi: 10.1007/s10637-018-0665-y
Jin, S., Hansson, E. M., Tikka, S., Lanner, F., Sahlgren, C., Farnebo, F., et al. (2008). Notch signaling regulates platelet-derived growth factor receptor-beta expression in vascular smooth muscle cells. Circ. Res. 102, 1483–1491. doi: 10.1161/CIRCRESAHA.107.167965
Joshi, I., Minter, L. M., Telfer, J., Demarest, R. M., Capobianco, A. J., Aster, J. C., et al. (2009). Notch signaling mediates G1/S cell-cycle progression in T cells via cyclin D3 and its dependent kinases. Blood 113, 1689–1698. doi: 10.1182/blood-2008-03-147967
Ju, J. H., Yang, W., Oh, S., Nam, K., Lee, K. M., Noh, D. Y., et al. (2013). HER2 stabilizes survivin while concomitantly down-regulating survivin gene transcription by suppressing Notch cleavage. Biochem. J. 451, 123–134. doi: 10.1042/BJ20121716
Kallifatidis, G., Labsch, S., Rausch, V., Mattern, J., Gladkich, J., Moldenhauer, G., et al. (2011). Sulforaphane increases drug-mediated cytotoxicity toward cancer stem-like cells of pancreas and prostate. Mol. Ther. 19, 188–195. doi: 10.1038/mt.2010.216
Kangsamaksin, T., Murtomaki, A., Kofler, N. M., Cuervo, H., Chaudhri, R. A., Tattersall, I. W., et al. (2015). NOTCH decoys that selectively block DLL/NOTCH or JAG/NOTCH disrupt angiogenesis by unique mechanisms to inhibit tumor growth. Cancer Discov. 5, 182–197. doi: 10.1158/2159-8290.CD-14-0650
Kao, H. Y., Ordentlich, P., Koyano-Nakagawa, N., Tang, Z., Downes, M., Kintner, C. R., et al. (1998). A histone deacetylase corepressor complex regulates the Notch signal transduction pathway. Genes Dev. 12, 2269–2277. doi: 10.1101/gad.12.15.2269
Kawahara, T., Kawaguchi-Ihara, N., Okuhashi, Y., Itoh, M., Nara, N., and Tohda, S. (2009). Cyclopamine and quercetin suppress the growth of leukemia and lymphoma cells. Anticancer Res. 29, 4629–4632.
Keller, P. J., Arendt, L. M., Skibinski, A., Logvinenko, T., Klebba, I., Dong, S., et al. (2012). Defining the cellular precursors to human breast cancer. Proc. Natl. Acad. Sci. U S A 109, 2772–2777. doi: 10.1073/pnas.1017626108
Kiaris, H., Politi, K., Grimm, L. M., Szabolcs, M., Fisher, P., Efstratiadis, A., et al. (2004). Modulation of notch signaling elicits signature tumors and inhibits hras1-induced oncogenesis in the mouse mammary epithelium. Am. J. Pathol. 165, 695–705. doi: 10.1016/S0002-9440(10)63333-0
Kim, R. K., Kaushik, N., Suh, Y., Yoo, K. C., Cui, Y. H., Kim, M. J., et al. (2016). Radiation driven epithelial-mesenchymal transition is mediated by Notch signaling in breast cancer. Oncotarget 7, 53430–53442. doi: 10.18632/oncotarget.10802
Klinakis, A., Szabolcs, M., Politi, K., Kiaris, H., Artavanis-Tsakonas, S., and Efstratiadis, A. (2006). Myc is a Notch1 transcriptional target and a requisite for Notch1-induced mammary tumorigenesis in mice. Proc. Natl. Acad. Sci. U S A 103, 9262–9267. doi: 10.1073/pnas.0603371103
Kondo, T., Morita, R., Okuzono, Y., Nakatsukasa, H., Sekiya, T., Chikuma, S., et al. (2017). Notch-mediated conversion of activated T cells into stem cell memory-like T cells for adoptive immunotherapy. Nat. Commun. 8:15338. doi: 10.1038/ncomms15338
Kong, P., Chen, L., Yu, M., Tao, J., Liu, J., Wang, Y., et al. (2018). miR-3178 inhibits cell proliferation and metastasis by targeting Notch1 in triple-negative breast cancer. Cell Death Dis. 9:1059. doi: 10.1038/s41419-018-1091-y
Kontomanolis, E., Panteliadou, M., Giatromanolaki, A., Pouliliou, S., Efremidou, E., Limberis, V., et al. (2014). Delta-like ligand 4 (DLL4) in the plasma and neoplastic tissues from breast cancer patients: correlation with metastasis. Med. Oncol. 31:945. doi: 10.1007/s12032-014-0945-0
Kordon, E. C., and Smith, G. H. (1998). An entire functional mammary gland may comprise the progeny from a single cell. Development 125, 1921–1930. doi: 10.1242/dev.125.10.1921
Krebs, L. T., Xue, Y., Norton, C. R., Shutter, J. R., Maguire, M., Sundberg, J. P., et al. (2000). Notch signaling is essential for vascular morphogenesis in mice. Genes Dev. 14, 1343–1352.
Krishna, B. M., Jana, S., Singhal, J., Horne, D., Awasthi, S., Salgia, R., et al. (2019). Notch signaling in breast cancer: from pathway analysis to therapy. Cancer Lett. 461, 123–131. doi: 10.1016/j.canlet.2019.07.012
Krop, I., Demuth, T., Guthrie, T., Wen, P. Y., Mason, W. P., Chinnaiyan, P., et al. (2012). Phase I pharmacologic and pharmacodynamic study of the gamma secretase (Notch) inhibitor MK-0752 in adult patients with advanced solid tumors. J. Clin. Oncol. 30, 2307–2313. doi: 10.1200/JCO.2011.39.1540
Kumar, S., Srivastav, R. K., Wilkes, D. W., Ross, T., Kim, S., Kowalski, J., et al. (2019). Estrogen-dependent DLL1-mediated Notch signaling promotes luminal breast cancer. Oncogene 38, 2092–2107. doi: 10.1038/s41388-018-0562-z
Lafkas, D., Rodilla, V., Huyghe, M., Mourao, L., Kiaris, H., and Fre, S. (2013). Notch3 marks clonogenic mammary luminal progenitor cells in vivo. J. Cell. Biol. 203, 47–56. doi: 10.1083/jcb.201307046
Lagadec, C., Vlashi, E., Alhiyari, Y., Phillips, T. M., Bochkur Dratver, M., and Pajonk, F. (2013). Radiation-induced Notch signaling in breast cancer stem cells. Int. J. Radiat. Oncol. Biol. Phys. 87, 609–618. doi: 10.1016/j.ijrobp.2013.06.2064
Lai, X. X., Li, G., Lin, B., and Yang, H. (2018). Interference of Notch 1 inhibits the proliferation and invasion of breast cancer cells: involvement of the β-catenin signaling pathway. Mol. Med. Rep. 17, 2472–2478. doi: 10.3892/mmr.2017.8161
Lamy, M., Ferreira, A., Dias, J. S., Braga, S., Silva, G., and Barbas, A. (2017). Notch-out for breast cancer therapies. N. Biotechnol. 39, 215–221. doi: 10.1016/j.nbt.2017.08.004
Lawson, D. A., Bhakta, N. R., Kessenbrock, K., Prummel, K. D., Yu, Y., Takai, K., et al. (2015). Single-cell analysis reveals a stem-cell program in human metastatic breast cancer cells. Nature 526, 131–135. doi: 10.1038/nature15260
Lee, C. W., Raskett, C. M., Prudovsky, I., and Altieri, D. C. (2008a). Molecular dependence of estrogen receptor-negative breast cancer on a notch-survivin signaling axis. Cancer Res. 68, 5273–5281. doi: 10.1158/0008-5472.CAN-07-6673
Lee, C. W., Simin, K., Liu, Q., Plescia, J., Guha, M., Khan, A., et al. (2008b). A functional Notch-survivin gene signature in basal breast cancer. Breast Cancer Res. 10:R97. doi: 10.1186/bcr2200
Lee, D., Kim, D., Choi, Y. B., Kang, K., Sung, E. S., Ahn, J. H., et al. (2016). Simultaneous blockade of VEGF and Dll4 by HD105, a bispecific antibody, inhibits tumor progression and angiogenesis. MAbs 8, 892–904. doi: 10.1080/19420862.2016.1171432
Lee, Y. C., Wang, W. L., Chang, W. C., Huang, Y. H., Hong, G. C., Wang, H. L., et al. (2019). Tribbles Homolog 3 involved in radiation response of triple negative breast cancer cells by regulating Notch1 activation. Cancers (Basel) 11:127. doi: 10.3390/cancers11020127
Leong, K. G., Niessen, K., Kulic, I., Raouf, A., Eaves, C., Pollet, I., et al. (2007). Jagged1-mediated Notch activation induces epithelial-to-mesenchymal transition through Slug-induced repression of E-cadherin. J. Exp. Med. 204, 2935–2948. doi: 10.1084/jem.20071082
Leontovich, A. A., Jalalirad, M., Salisbury, J. L., Mills, L., Haddox, C., Schroeder, M., et al. (2018). NOTCH3 expression is linked to breast cancer seeding and distant metastasis. Breast Cancer Res. 20:105. doi: 10.1186/s13058-018-1020-0
Li, D., Masiero, M., Banham, A. H., and Harris, A. L. (2014). The notch ligand JAGGED1 as a target for anti-tumor therapy. Front. Oncol. 4:254. doi: 10.3389/fonc.2014.00254
Li, K., Li, Y., Wu, W., Gordon, W. R., Chang, D. W., Lu, M., et al. (2008). Modulation of Notch signaling by antibodies specific for the extracellular negative regulatory region of NOTCH3. J. Biol. Chem. 283, 8046–8054. doi: 10.1074/jbc.M800170200
Li, L., Zhao, F., Lu, J., Li, T., Yang, H., Wu, C., et al. (2014b). Notch-1 signaling promotes the malignant features of human breast cancer through NF-κB activation. PLoS One 9:e95912. doi: 10.1371/journal.pone.0095912
Li, X. J., Ji, M. H., Zhong, S. L., Zha, Q. B., Xu, J. J., Zhao, J. H., et al. (2012a). MicroRNA-34a modulates chemosensitivity of breast cancer cells to adriamycin by targeting Notch1. Arch. Med. Res. 43, 514–521. doi: 10.1016/j.arcmed.2012.09.007
Li, Y., Wang, Z., Jin, J., Zhu, S. X., He, G. Q., Li, S. H., et al. (2020). Quercetin pretreatment enhances the radiosensitivity of colon cancer cells by targeting Notch-1 pathway. Biochem. Biophys. Res. Commun. 523, 947–953. doi: 10.1016/j.bbrc.2020.01.048
Li, Y., Zhang, J., Ma, D., Zhang, L., Si, M., Yin, H., et al. (2012b). Curcumin inhibits proliferation and invasion of osteosarcoma cells through inactivation of Notch-1 signaling. FEBS J. 279, 2247–2259. doi: 10.1111/j.1742-4658.2012.08607.x
Li, Z. L., Chen, C., Yang, Y., Wang, C., Yang, T., Yang, X., et al. (2015). Gamma secretase inhibitor enhances sensitivity to doxorubicin in MDA-MB-231 cells. Int. J. Clin. Exp. Pathol. 8, 4378–4387.
Lilja, A. M., Rodilla, V., Huyghe, M., Hannezo, E., Landragin, C., Renaud, O., et al. (2018). Clonal analysis of Notch1-expressing cells reveals the existence of unipotent stem cells that retain long-term plasticity in the embryonic mammary gland. Nat. Cell. Biol. 20, 677–687. doi: 10.1038/s41556-018-0108-1
Lin, H. Y., Liang, Y. K., Dou, X. W., Chen, C. F., Wei, X. L., Zeng, D., et al. (2018). Notch3 inhibits epithelial-mesenchymal transition in breast cancer via a novel mechanism, upregulation of GATA-3 expression. Oncogenesis 7:59. doi: 10.1038/s41389-018-0069-z
Ling, H., and Jolicoeur, P. (2013). Notch-1 signaling promotes the cyclinD1-dependent generation of mammary tumor-initiating cells that can revert to bi-potential progenitors from which they arise. Oncogene 32, 3410–3419. doi: 10.1038/onc.2012.341
Ling, H., Sylvestre, J. R., and Jolicoeur, P. (2010). Notch1-induced mammary tumor development is cyclin D1-dependent and correlates with expansion of pre-malignant multipotent duct-limited progenitors. Oncogene 29, 4543–4554. doi: 10.1038/onc.2010.186
Logeat, F., Bessia, C., Brou, C., LeBail, O., Jarriault, S., Seidah, N. G., et al. (1998). The Notch1 receptor is cleaved constitutively by a furin-like convertase. Proc. Natl. Acad. Sci. U S A 95, 8108–8112. doi: 10.1073/pnas.95.14.8108
Magnani, L., Stoeck, A., Zhang, X., Lánczky, A., Mirabella, A. C., Wang, T. L., et al. (2013). Genome-wide reprogramming of the chromatin landscape underlies endocrine therapy resistance in breast cancer. Proc. Natl. Acad. Sci. U S A 110, E1490–E1499. doi: 10.1073/pnas.1219992110
Maillard, I., Tu, L., Sambandam, A., Yashiro-Ohtani, Y., Millholland, J., Keeshan, K., et al. (2006). The requirement for Notch signaling at the beta-selection checkpoint in vivo is absolute and independent of the pre-T cell receptor. J. Exp. Med. 203, 2239–2245. doi: 10.1084/jem.20061020
Mamaeva, V., Niemi, R., Beck, M., Özliseli, E., Desai, D., Landor, S., et al. (2016). Inhibiting notch activity in breast cancer stem cells by glucose functionalized nanoparticles carrying γ-secretase inhibitors. Mol. Ther. 24, 926–936. doi: 10.1038/mt.2016.42
Martin, T. A., Goyal, A., Watkins, G., and Jiang, W. G. (2005). Expression of the transcription factors snail, slug, and twist and their clinical significance in human breast cancer. Ann. Surg. Oncol. 12, 488–496. doi: 10.1245/ASO.2005.04.010
Massard, C., Azaro, A., Soria, J. C., Lassen, U., Le Tourneau, C., Sarker, D., et al. (2018). First-in-human study of LY3039478, an oral Notch signaling inhibitor in advanced or metastatic cancer. Ann. Oncol. 29, 1911–1917. doi: 10.1093/annonc/mdy244
Mavaddat, N., Barrowdale, D., Andrulis, I. L., Domchek, S. M., Eccles, D., Nevanlinna, H., et al. (2012). Pathology of breast and ovarian cancers among BRCA1 and BRCA2 mutation carriers: results from the Consortium of investigators of modifiers of BRCA1/2 (CIMBA). Cancer Epidemiol. Biomarkers Prev. 21, 134–147. doi: 10.1158/1055-9965.EPI-11-0775
Melchor, L., Molyneux, G., Mackay, A., Magnay, F. A., Atienza, M., Kendrick, H., et al. (2014). Identification of cellular and genetic drivers of breast cancer heterogeneity in genetically engineered mouse tumour models. J. Pathol. 233, 124–137. doi: 10.1002/path.4345
Meurette, O., Stylianou, S., Rock, R., Collu, G. M., Gilmore, A. P., and Brennan, K. (2009). Notch activation induces Akt signaling via an autocrine loop to prevent apoptosis in breast epithelial cells. Cancer Res. 69, 5015–5022. doi: 10.1158/0008-5472.CAN-08-3478
Miao, K., Lei, J. H., Valecha, M. V., Zhang, A., Xu, J., Wang, L., et al. (2020). NOTCH1 activation compensates BRCA1 deficiency and promotes triple-negative breast cancer formation. Nat. Commun. 11:3256. doi: 10.1038/s41467-020-16936-9
Milano, J., McKay, J., Dagenais, C., Foster-Brown, L., Pognan, F., Gadient, R., et al. (2004). Modulation of notch processing by gamma-secretase inhibitors causes intestinal goblet cell metaplasia and induction of genes known to specify gut secretory lineage differentiation. Toxicol. Sci. 82, 341–358. doi: 10.1093/toxsci/kfh254
Mittal, S., Sharma, A., Balaji, S. A., Gowda, M. C., Dighe, R. R., Kumar, R. V., et al. (2014). Coordinate hyperactivation of Notch1 and Ras/MAPK pathways correlates with poor patient survival: novel therapeutic strategy for aggressive breast cancers. Mol. Cancer Ther. 13, 3198–3209. doi: 10.1158/1535-7163.MCT-14-0280
Mittal, S., Subramanyam, D., Dey, D., Kumar, R. V., and Rangarajan, A. (2009). Cooperation of Notch and Ras/MAPK signaling pathways in human breast carcinogenesis. Mol. Cancer 8:128. doi: 10.1186/1476-4598-8-128
Moellering, R. E., Cornejo, M., Davis, T. N., Del Bianco, C., Aster, J. C., Blacklow, S. C., et al. (2009). Direct inhibition of the NOTCH transcription factor complex. Nature 462, 182–188. doi: 10.1038/nature08543
Mollen, E. W. J., Ient, J., Tjan-Heijnen, V. C. G., Boersma, L. J., Miele, L., Smidt, M. L., et al. (2018). Moving breast cancer therapy up a Notch. Front. Oncol. 8:518. doi: 10.3389/fonc.2018.00518
Molyneux, G., Geyer, F. C., Magnay, F. A., McCarthy, A., Kendrick, H., Natrajan, R., et al. (2010). BRCA1 basal-like breast cancers originate from luminal epithelial progenitors and not from basal stem cells. Cell Stem Cell 7, 403–417. doi: 10.1016/j.stem.2010.07.010
Mori, M., Tottone, L., Quaglio, D., Zhdanovskaya, N., Ingallina, C., Fusto, M., et al. (2017). Identification of a novel chalcone derivative that inhibits Notch signaling in T-cell acute lymphoblastic leukemia. Sci. Rep. 7:2213. doi: 10.1038/s41598-017-02316-9
Mungamuri, S. K., Yang, X., Thor, A. D., and Somasundaram, K. (2006). Survival signaling by Notch1: mammalian target of rapamycin (mTOR)-dependent inhibition of p53. Cancer Res. 66, 4715–4724. doi: 10.1158/0008-5472.CAN-05-3830
Nagamatsu, I., Onishi, H., Matsushita, S., Kubo, M., Kai, M., Imaizumi, A., et al. (2014). NOTCH4 is a potential therapeutic target for triple-negative breast cancer. Anticancer Res. 34, 69–80.
Nam, Y., Sliz, P., Song, L., Aster, J. C., and Blacklow, S. C. (2006). Structural basis for cooperativity in recruitment of MAML coactivators to Notch transcription complexes. Cell 124, 973–983. doi: 10.1016/j.cell.2005.12.037
Nichols, J. T., Miyamoto, A., Olsen, S. L., D’Souza, B., Yao, C., and Weinmaster, G. (2007). DSL ligand endocytosis physically dissociates Notch1 heterodimers before activating proteolysis can occur. J. Cell Biol. 176, 445–458. doi: 10.1083/jcb.200609014
Nicolas, M., Wolfer, A., Raj, K., Kummer, J. A., Mill, P., van Noort, M., et al. (2003). Notch1 functions as a tumor suppressor in mouse skin. Nat. Genet. 33, 416–421. doi: 10.1038/ng1099
Noguera-Troise, I., Daly, C., Papadopoulos, N. J., Coetzee, S., Boland, P., Gale, N. W., et al. (2006). Blockade of Dll4 inhibits tumour growth by promoting non-productive angiogenesis. Nature 444, 1032–1037. doi: 10.1038/nature05355
Nowell, C. S., and Radtke, F. (2017). Notch as a tumour suppressor. Nat. Rev. Cancer 17, 145–159. doi: 10.1038/nrc.2016.145
Nwaeburu, C. C., Bauer, N., Zhao, Z., Abukiwan, A., Gladkich, J., Benner, A., et al. (2016). Up-regulation of microRNA let-7c by quercetin inhibits pancreatic cancer progression by activation of Numbl. Oncotarget 7, 58367–58380. doi: 10.18632/oncotarget.11122
O’Neill, C. F., Urs, S., Cinelli, C., Lincoln, A., Nadeau, R. J., León, R., et al. (2007). Notch2 signaling induces apoptosis and inhibits human MDA-MB-231 xenograft growth. Am. J. Pathol. 171, 1023–1036. doi: 10.2353/ajpath.2007.061029
Onoyama, I., Nakayama, S., Shimizu, H., and Nakayama, K. I. (2020). Loss of Fbxw7 impairs development of and induces heterogeneous tumor formation in the mouse mammary gland. Cancer Res. 80, 5515–5530. doi: 10.1158/0008-5472.CAN-20-0271
Osipo, C., Golde, T. E., Osborne, B. A., and Miele, L. A. (2008a). Off the beaten pathway: the complex cross talk between Notch and NF-kappaB. Lab. Invest 88, 11–17. doi: 10.1038/labinvest.3700700
Osipo, C., Patel, P., Rizzo, P., Clementz, A. G., Hao, L., Golde, T. E., et al. (2008b). ErbB-2 inhibition activates Notch-1 and sensitizes breast cancer cells to a gamma-secretase inhibitor. Oncogene 27, 5019–5032. doi: 10.1038/onc.2008.149
Oswald, F., Liptay, S., Adler, G., and Schmid, R. M. (1998). NF-kappaB2 is a putative target gene of activated Notch-1 via RBP-Jkappa. Mol. Cell Biol. 18, 2077–2088. doi: 10.1128/mcb.18.4.2077
Oswald, F., Winkler, M., Cao, Y., Astrahantseff, K., Bourteele, S., Knöchel, W., et al. (2005). RBP-Jkappa/SHARP recruits CtIP/CtBP corepressors to silence Notch target genes. Mol. Cell Biol. 25, 10379–10390. doi: 10.1128/MCB.25.23.10379-10390.2005
Palomero, T., Lim, W. K., Odom, D. T., Sulis, M. L., Real, P. J., Margolin, A., et al. (2006). NOTCH1 directly regulates c-MYC and activates a feed-forward-loop transcriptional network promoting leukemic cell growth. Proc. Natl. Acad. Sci. U S A 103, 18261–18266. doi: 10.1073/pnas.0606108103
Palomero, T., Sulis, M. L., Cortina, M., Real, P. J., Barnes, K., Ciofani, M., et al. (2007). Mutational loss of PTEN induces resistance to NOTCH1 inhibition in T-cell leukemia. Nat. Med. 13, 1203–1210. doi: 10.1038/nm1636
Pan, H., Zhou, W., He, W., Liu, X., Ding, Q., Ling, L., et al. (2012). Genistein inhibits MDA-MB-231 triple-negative breast cancer cell growth by inhibiting NF-κB activity via the Notch-1 pathway. Int. J. Mol. Med. 30, 337–343. doi: 10.3892/ijmm.2012.990
Pandya, K., Meeke, K., Clementz, A. G., Rogowski, A., Roberts, J., Miele, L., et al. (2011). Targeting both Notch and ErbB-2 signalling pathways is required for prevention of ErbB-2-positive breast tumour recurrence. Br. J. Cancer 105, 796–806. doi: 10.1038/bjc.2011.321
Pandya, K., Wyatt, D., Gallagher, B., Shah, D., Baker, A., Bloodworth, J., et al. (2016). PKCα attenuates jagged-1-mediated notch signaling in ErbB-2-positive breast cancer to reverse trastuzumab resistance. Clin. Cancer Res. 22, 175–186. doi: 10.1158/1078-0432.CCR-15-0179
Parks, A. L., Klueg, K. M., Stout, J. R., and Muskavitch, M. A. (2000). Ligand endocytosis drives receptor dissociation and activation in the Notch pathway. Development 127, 1373–1385. doi: 10.1242/dev.127.7.1373
Parr, C., Watkins, G., and Jiang, W. G. (2004). The possible correlation of Notch-1 and Notch-2 with clinical outcome and tumour clinicopathological parameters in human breast cancer. Int. J. Mol. Med. 14, 779–786. doi: 10.3892/ijmm.14.5.779
Pece, S., Serresi, M., Santolini, E., Capra, M., Hulleman, E., Galimberti, V., et al. (2004). Loss of negative regulation by Numb over Notch is relevant to human breast carcinogenesis. J. Cell Biol. 167, 215–221. doi: 10.1083/jcb.200406140
Pei, J., and Wang, B. (2015). Notch-1 promotes breast cancer cells proliferation by regulating LncRNA GAS5. Int. J. Clin. Exp. Med. 8, 14464–14471.
Portanova, P., Notaro, A., Pellerito, O., Sabella, S., Giuliano, M., and Calvaruso, G. (2013). Notch inhibition restores TRAIL-mediated apoptosis via AP1-dependent upregulation of DR4 and DR5 TRAIL receptors in MDA-MB-231 breast cancer cells. Int. J. Oncol. 43, 121–130. doi: 10.3892/ijo.2013.1945
Proia, T., Jiang, F., Bell, A., Nicoletti, R., Kong, L., Kreuter, K., et al. (2015). 23814, an inhibitory antibody of ligand-mediated Notch1 activation, modulates angiogenesis and inhibits tumor growth without gastrointestinal toxicity. Mol. Cancer Ther. 14, 1858–1867. doi: 10.1158/1535-7163.MCT-14-1104
Proia, T. A., Keller, P. J., Gupta, P. B., Klebba, I., Jones, A. D., Sedic, M., et al. (2011). Genetic predisposition directs breast cancer phenotype by dictating progenitor cell fate. Cell Stem Cell 8, 149–163. doi: 10.1016/j.stem.2010.12.007
Qiu, M., Peng, Q., Jiang, I., Carroll, C., Han, G., Rymer, I., et al. (2013). Specific inhibition of Notch1 signaling enhances the antitumor efficacy of chemotherapy in triple negative breast cancer through reduction of cancer stem cells. Cancer Lett. 328, 261–270. doi: 10.1016/j.canlet.2012.09.023
Raafat, A., Goldhar, A. S., Klauzinska, M., Xu, K., Amirjazil, I., McCurdy, D., et al. (2011). Expression of Notch receptors, ligands, and target genes during development of the mouse mammary gland. J. Cell. Physiol. 226, 1940–1952. doi: 10.1002/jcp.22526
Rand, M. D., Grimm, L. M., Artavanis-Tsakonas, S., Patriub, V., Blacklow, S. C., Sklar, J., et al. (2000). Calcium depletion dissociates and activates heterodimeric notch receptors. Mol. Cell Biol. 20, 1825–1835. doi: 10.1128/mcb.20.5.1825-1835.2000
Rangarajan, A., Talora, C., Okuyama, R., Nicolas, M., Mammucari, C., Oh, H., et al. (2001). Notch signaling is a direct determinant of keratinocyte growth arrest and entry into differentiation. EMBO J. 20, 3427–3436. doi: 10.1093/emboj/20.13.3427
Raouf, A., Zhao, Y., To, K., Stingl, J., Delaney, A., Barbara, M., et al. (2008). Transcriptome analysis of the normal human mammary cell commitment and differentiation process. Cell Stem Cell 3, 109–118. doi: 10.1016/j.stem.2008.05.018
Reedijk, M. (2012). Notch signaling and breast cancer. Adv. Exp. Med. Biol. 727, 241–257. doi: 10.1007/978-1-4614-0899-4_18
Reedijk, M., Odorcic, S., Chang, L., Zhang, H., Miller, N., McCready, D. R., et al. (2005). High-level coexpression of JAG1 and NOTCH1 is observed in human breast cancer and is associated with poor overall survival. Cancer Res. 65, 8530–8537. doi: 10.1158/0008-5472.CAN-05-1069
Reizis, B., and Leder, P. (2002). Direct induction of T lymphocyte-specific gene expression by the mammalian Notch signaling pathway. Genes Dev. 16, 295–300. doi: 10.1101/gad.960702
Rizzo, P., Miao, H., D’Souza, G., Osipo, C., Song, L. L., Yun, J., et al. (2008). Cross-talk between notch and the estrogen receptor in breast cancer suggests novel therapeutic approaches. Cancer Res. 68, 5226–5235. doi: 10.1158/0008-5472.CAN-07-5744
Robert-Moreno, A., Espinosa, L., de la Pompa, J. L., and Bigas, A. (2005). RBPjkappa-dependent Notch function regulates Gata2 and is essential for the formation of intra-embryonic hematopoietic cells. Development 132, 1117–1126. doi: 10.1242/dev.01660
Rodilla, V., Dasti, A., Huyghe, M., Lafkas, D., Laurent, C., Reyal, F., et al. (2015). Luminal progenitors restrict their lineage potential during mammary gland development. PLoS Biol. 13:e1002069. doi: 10.1371/journal.pbio.1002069
Ronchini, C., and Capobianco, A. J. (2001). Induction of cyclin D1 transcription and CDK2 activity by Notch(ic): implication for cell cycle disruption in transformation by Notch(ic). Mol. Cell Biol. 21, 5925–5934. doi: 10.1128/mcb.21.17.5925-5934.2001
Rosen, L. S., Wesolowski, R., Baffa, R., Liao, K. H., Hua, S. Y., Gibson, B. L., et al. (2020). A phase I, dose-escalation study of PF-06650808, an anti-Notch3 antibody-drug conjugate, in patients with breast cancer and other advanced solid tumors. Invest. New Drugs 38, 120–130. doi: 10.1007/s10637-019-00754-y
Rui, X., Zhao, H., Xiao, X., Wang, L., Mo, L., and Yao, Y. (2018). MicroRNA-34a suppresses breast cancer cell proliferation and invasion by targeting Notch1. Exp. Ther. Med. 16, 4387–4392. doi: 10.3892/etm.2018.6744
Russo, J., Ao, X., Grill, C., and Russo, I. H. (1999). Pattern of distribution of cells positive for estrogen receptor alpha and progesterone receptor in relation to proliferating cells in the mammary gland. Breast Cancer Res. Treat 53, 217–227. doi: 10.1023/a:1006186719322
Rustighi, A., Zannini, A., Tiberi, L., Sommaggio, R., Piazza, S., Sorrentino, G., et al. (2014). Prolyl-isomerase Pin1 controls normal and cancer stem cells of the breast. EMBO Mol. Med. 6, 99–119. doi: 10.1002/emmm.201302909
Sahlgren, C., Gustafsson, M. V., Jin, S., Poellinger, L., and Lendahl, U. (2008). Notch signaling mediates hypoxia-induced tumor cell migration and invasion. Proc. Natl. Acad. Sci. U S A 105, 6392–6397. doi: 10.1073/pnas.0802047105
Šale, S., Lafkas, D., and Artavanis-Tsakonas, S. (2013). Notch2 genetic fate mapping reveals two previously unrecognized mammary epithelial lineages. Nat. Cell Biol. 15, 451–460. doi: 10.1038/ncb2725
Sales-Dias, J., Silva, G., Lamy, M., Ferreira, A., and Barbas, A. (2019). The Notch ligand DLL1 exerts carcinogenic features in human breast cancer cells. PLoS One 14:e0217002. doi: 10.1371/journal.pone.0217002
Sansone, P., Storci, G., Giovannini, C., Pandolfi, S., Pianetti, S., Taffurelli, M., et al. (2007a). p66Shc/Notch-3 interplay controls self-renewal and hypoxia survival in human stem/progenitor cells of the mammary gland expanded in vitro as mammospheres. Stem Cells 25, 807–815. doi: 10.1634/stemcells.2006-0442
Sansone, P., Storci, G., Tavolari, S., Guarnieri, T., Giovannini, C., Taffurelli, M., et al. (2007b). IL-6 triggers malignant features in mammospheres from human ductal breast carcinoma and normal mammary gland. J. Clin. Invest. 117, 3988–4002. doi: 10.1172/JCI32533
Santoro, A., Vlachou, T., Carminati, M., Pelicci, P. G., and Mapelli, M. (2016). Molecular mechanisms of asymmetric divisions in mammary stem cells. EMBO Rep. 17, 1700–1720. doi: 10.15252/embr.201643021
Schott, A. F., Landis, M. D., Dontu, G., Griffith, K. A., Layman, R. M., Krop, I., et al. (2013). Preclinical and clinical studies of gamma secretase inhibitors with docetaxel on human breast tumors. Clin. Cancer Res. 19, 1512–1524. doi: 10.1158/1078-0432.CCR-11-3326
Schroeter, E. H., Kisslinger, J. A., and Kopan, R. (1998). Notch-1 signalling requires ligand-induced proteolytic release of intracellular domain. Nature 393, 382–386. doi: 10.1038/30756
Sethi, N., Dai, X., Winter, C. G., and Kang, Y. (2011). Tumor-derived JAGGED1 promotes osteolytic bone metastasis of breast cancer by engaging notch signaling in bone cells. Cancer Cell 19, 192–205. doi: 10.1016/j.ccr.2010.12.022
Sha, J., Li, J., Wang, W., Pan, L., Cheng, J., Li, L., et al. (2016). Curcumin induces G0/G1 arrest and apoptosis in hormone independent prostate cancer DU-145 cells by down regulating Notch signaling. Biomed. Pharmacother. 84, 177–184. doi: 10.1016/j.biopha.2016.09.037
Shao, S., Zhao, X., Zhang, X., Luo, M., Zuo, X., Huang, S., et al. (2015). Notch1 signaling regulates the epithelial-mesenchymal transition and invasion of breast cancer in a Slug-dependent manner. Mol. Cancer 14:28. doi: 10.1186/s12943-015-0295-3
Sharma, A., Paranjape, A. N., Rangarajan, A., and Dighe, R. R. (2012). A monoclonal antibody against human Notch1 ligand-binding domain depletes subpopulation of putative breast cancer stem-like cells. Mol. Cancer Ther. 11, 77–86. doi: 10.1158/1535-7163.MCT-11-0508
Shimizu, H., Woodcock, S. A., Wilkin, M. B., Trubenová, B., Monk, N. A., and Baron, M. (2014). Compensatory flux changes within an endocytic trafficking network maintain thermal robustness of notch signaling. Cell 157, 1160–1174. doi: 10.1016/j.cell.2014.03.050
Shimizu, M., Cohen, B., Goldvasser, P., Berman, H., Virtanen, C., and Reedijk, M. (2011). Plasminogen activator uPA is a direct transcriptional target of the JAG1-Notch receptor signaling pathway in breast cancer. Cancer Res. 71, 277–286. doi: 10.1158/0008-5472.CAN-10-2523
Siebel, C., and Lendahl, U. (2017). Notch signaling in development, tissue homeostasis, and disease. Physiol. Rev. 97, 1235–1294. doi: 10.1152/physrev.00005.2017
Simões, B. M., O’Brien, C. S., Eyre, R., Silva, A., Yu, L., Sarmiento-Castro, A., et al. (2015). Anti-estrogen resistance in human breast tumors is driven by JAG1-NOTCH4-dependent cancer stem cell activity. Cell Rep. 12, 1968–1977. doi: 10.1016/j.celrep.2015.08.050
Slepicka, P. F., Somasundara, A. V. H., and Dos Santos, C. O. (2020). The molecular basis of mammary gland development and epithelial differentiation. Semin. Cell Dev. Biol. 114, 93–112. doi: 10.1016/j.semcdb.2020.09.014
Smith, D. C., Chugh, R., Patnaik, A., Papadopoulos, K. P., Wang, M., Kapoun, A. M., et al. (2019). A phase 1 dose escalation and expansion study of Tarextumab (OMP-59R5) in patients with solid tumors. Invest New Drugs 37, 722–730. doi: 10.1007/s10637-018-0714-6
Smith, G. H., Gallahan, D., Diella, F., Jhappan, C., Merlino, G., and Callahan, R. (1995). Constitutive expression of a truncated INT3 gene in mouse mammary epithelium impairs differentiation and functional development. Cell Growth Differ. 6, 563–577.
Soares, R., Balogh, G., Guo, S., Gärtner, F., Russo, J., and Schmitt, F. (2004). Evidence for the notch signaling pathway on the role of estrogen in angiogenesis. Mol. Endocrinol. 18, 2333–2343. doi: 10.1210/me.2003-0362
South, A. P., Purdie, K. J., Watt, S. A., Haldenby, S., den Breems, N., Dimon, M., et al. (2014). NOTCH1 mutations occur early during cutaneous squamous cell carcinogenesis. J. Invest. Dermatol. 134, 2630–2638. doi: 10.1038/jid.2014.154
Steinbuck, M. P., and Winandy, S. (2018). A review of notch processing with new insights into ligand-independent notch signaling in T-cells. Front. Immunol. 9:1230. doi: 10.3389/fimmu.2018.01230
Strom, A., Hartman, J., Lam, E., Lin, C., and Gustafsson, J. (2009). Function of HES6 an Inhibitor of HES1, in the Breast Cancer Cell Lines T47D and MCF-7 Is to Up-Regulate E2F-1 and Increased Proliferation. Thirty-Second Annual CTRC-AACR San Antonio Breast Cancer Symposium. San Antonio, TX: American Association for Cancer Research, 2153.
Stylianou, S., Clarke, R. B., and Brennan, K. (2006). Aberrant activation of notch signaling in human breast cancer. Cancer Res. 66, 1517–1525. doi: 10.1158/0008-5472.CAN-05-3054
Suman, S., Das, T. P., and Damodaran, C. (2013). Silencing NOTCH signaling causes growth arrest in both breast cancer stem cells and breast cancer cells. Br. J. Cancer 109, 2587–2596. doi: 10.1038/bjc.2013.642
Sun, Y., Lowther, W., Kato, K., Bianco, C., Kenney, N., Strizzi, L., et al. (2005). Notch4 intracellular domain binding to Smad3 and inhibition of the TGF-beta signaling. Oncogene 24, 5365–5374. doi: 10.1038/sj.onc.1208528
Takagi-Niidome, S., Osawa, S., Tomita, T., and Iwatsubo, T. (2013). Inhibition of γ-secretase activity by a monoclonal antibody against the extracellular hydrophilic loop of presenilin 1. Biochemistry 52, 61–69. doi: 10.1021/bi301252r
Tao, L., van Bragt, M. P., and Li, Z. (2015). A long-lived luminal subpopulation enriched with alveolar progenitors serves as cellular origin of heterogeneous mammary tumors. Stem Cell Rep. 5, 60–74. doi: 10.1016/j.stemcr.2015.05.014
Totaro, A., Castellan, M., Di Biagio, D., and Piccolo, S. (2018). Crosstalk between YAP/TAZ and notch signaling. Trends Cell Biol. 28, 560–573. doi: 10.1016/j.tcb.2018.03.001
Traina, T. A., Cobain, E. F., Evron, E., Duskin, C., and Gordon, G. (2021). Abstract OT-29-01: Tenacity: A phase 2, multicenter, open-label, single-arm study of AL101 monotherapy in patients with notch-activated triple negative breast cancer. 2020 San Antonio Breast Cancer Virtual Symposium. San Antonio: AACR.
Tu, J., Guo, Y., Hong, W., Fang, Y., Han, D., Zhang, P., et al. (2019). The regulatory effects of paeoniflorin and its derivative Paeoniflorin-6’-O-benzene sulfonate CP-25 on inflammation and immune diseases. Front. Pharmacol. 10:57. doi: 10.3389/fphar.2019.00057
Vaccari, T., Lu, H., Kanwar, R., Fortini, M. E., and Bilder, D. (2008). Endosomal entry regulates notch receptor activation in drosophila melanogaster. J. Cell Biol. 180, 755–762. doi: 10.1083/jcb.200708127
Wacker, S. A., Alvarado, C., von Wichert, G., Knippschild, U., Wiedenmann, J., Clauss, K., et al. (2011). RITA, a novel modulator of notch signalling, acts via nuclear export of RBP-J. EMBO J. 30, 43–56. doi: 10.1038/emboj.2010.289
Wang, J. W., Wei, X. L., Dou, X. W., Huang, W. H., Du, C. W., and Zhang, G. J. (2018a). The association between Notch4 expression, and clinicopathological characteristics and clinical outcomes in patients with breast cancer. Oncol. Lett. 15, 8749–8755. doi: 10.3892/ol.2018.8442
Wang, K., Zhang, Q., Li, D., Ching, K., Zhang, C., Zheng, X., et al. (2015). PEST domain mutations in Notch receptors comprise an oncogenic driver segment in triple-negative breast cancer sensitive to a γ-secretase inhibitor. Clin. Cancer Res. 21, 1487–1496. doi: 10.1158/1078-0432.CCR-14-1348
Wang, N. J., Sanborn, Z., Arnett, K. L., Bayston, L. J., Liao, W., Proby, C. M., et al. (2011). Loss-of-function mutations in Notch receptors in cutaneous and lung squamous cell carcinoma. Proc. Natl. Acad. Sci. U S A 108, 17761–17766. doi: 10.1073/pnas.1114669108
Wang, S., Liang, Y., Chang, W., Hu, B., and Zhang, Y. (2018b). Triple negative breast cancer depends on sphingosine kinase 1 (SphK1)/Sphingosine-1-Phosphate (S1P)/Sphingosine 1-phosphate receptor 3 (S1PR3)/Notch signaling for metastasis. Med. Sci. Monit. 24, 1912–1923. doi: 10.12659/msm.905833
Watson, C. J., and Khaled, W. T. (2020). Mammary development in the embryo and adult: new insights into the journey of morphogenesis and commitment. Development 147:dev169862. doi: 10.1242/dev.169862
Weerkamp, F., Luis, T. C., Naber, B. A., Koster, E. E., Jeannotte, L., van Dongen, J. J., et al. (2006). Identification of Notch target genes in uncommitted T-cell progenitors: No direct induction of a T-cell specific gene program. Leukemia 20, 1967–1977. doi: 10.1038/sj.leu.2404396
Weijzen, S., Rizzo, P., Braid, M., Vaishnav, R., Jonkheer, S. M., Zlobin, A., et al. (2002). Activation of Notch-1 signaling maintains the neoplastic phenotype in human Ras-transformed cells. Nat. Med. 8, 979–986. doi: 10.1038/nm754
Wen, X. F., Chen, M., Wu, Y., Chen, M. N., Glogowska, A., Klonisch, T., et al. (2018). Inhibitor of DNA binding 2 inhibits epithelial-mesenchymal transition via Up-regulation of Notch3 in breast cancer. Transl. Oncol. 11, 1259–1270. doi: 10.1016/j.tranon.2018.07.015
Weng, A. P., Millholland, J. M., Yashiro-Ohtani, Y., Arcangeli, M. L., Lau, A., Wai, C., et al. (2006). c-Myc is an important direct target of Notch1 in T-cell acute lymphoblastic leukemia/lymphoma. Genes Dev. 20, 2096–2109. doi: 10.1101/gad.1450406
Wilson, J. J., and Kovall, R. A. (2006). Crystal structure of the CSL-Notch-Mastermind ternary complex bound to DNA. Cell 124, 985–996. doi: 10.1016/j.cell.2006.01.035
Woodward, W. A., Chen, M. S., Behbod, F., and Rosen, J. M. (2005). On mammary stem cells. J. Cell Sci. 118, 3585–3594. doi: 10.1242/jcs.02532
Wu, L., Aster, J. C., Blacklow, S. C., Lake, R., Artavanis-Tsakonas, S., and Griffin, J. D. (2000). MAML1, a human homologue of Drosophila mastermind, is a transcriptional co-activator for NOTCH receptors. Nat. Genet. 26, 484–489. doi: 10.1038/82644
Wu, Y., Cain-Hom, C., Choy, L., Hagenbeek, T. J., de Leon, G. P., Chen, Y., et al. (2010). Therapeutic antibody targeting of individual Notch receptors. Nature 464, 1052–1057. doi: 10.1038/nature08878
Xia, J., Duan, Q., Ahmad, A., Bao, B., Banerjee, S., Shi, Y., et al. (2012). Genistein inhibits cell growth and induces apoptosis through up-regulation of miR-34a in pancreatic cancer cells. Curr. Drug Targets 13, 1750–1756. doi: 10.2174/138945012804545597
Xiao, Y. S., Zeng, D., Liang, Y. K., Wu, Y., Li, M. F., Qi, Y. Z., et al. (2019). Major vault protein is a direct target of Notch1 signaling and contributes to chemoresistance in triple-negative breast cancer cells. Cancer Lett. 441, 156–167. doi: 10.1016/j.canlet.2018.09.031
Xie, X., Kaoud, T. S., Edupuganti, R., Zhang, T., Kogawa, T., Zhao, Y., et al. (2017). c-Jun N-terminal kinase promotes stem cell phenotype in triple-negative breast cancer through upregulation of Notch1 via activation of c-Jun. Oncogene 36, 2599–2608. doi: 10.1038/onc.2016.417
Xiong, W., Tan, J., Guo, Y., Chen, S., Fan, L., and Li, Y. (2020). Notch3 knockout suppresses mouse mammary gland development and inhibits the proliferation of 4T1 murine mammary carcinoma cells via CCL2/CCR4 Axis. Front. Cell Dev. Biol. 8:594372. doi: 10.3389/fcell.2020.594372
Xu, K., Usary, J., Kousis, P. C., Prat, A., Wang, D. Y., Adams, J. R., et al. (2012). Lunatic fringe deficiency cooperates with the Met/Caveolin gene amplicon to induce basal-like breast cancer. Cancer Cell 21, 626–641. doi: 10.1016/j.ccr.2012.03.041
Yalcin-Ozuysal, O., Fiche, M., Guitierrez, M., Wagner, K. U., Raffoul, W., and Brisken, C. (2010). Antagonistic roles of Notch and p63 in controlling mammary epithelial cell fates. Cell Death Differ. 17, 1600–1612. doi: 10.1038/cdd.2010.37
Yamaguchi, N., Oyama, T., Ito, E., Satoh, H., Azuma, S., Hayashi, M., et al. (2008). NOTCH3 signaling pathway plays crucial roles in the proliferation of ErbB2-negative human breast cancer cells. Cancer Res. 68, 1881–1888. doi: 10.1158/0008-5472.CAN-07-1597
Yan, M., Callahan, C. A., Beyer, J. C., Allamneni, K. P., Zhang, G., Ridgway, J. B., et al. (2010). Chronic DLL4 blockade induces vascular neoplasms. Nature 463, 6–7. doi: 10.1038/nature08751
Yan, Y., Liu, F., Han, L., Zhao, L., Chen, J., Olopade, O. I., et al. (2018). HIF-2α promotes conversion to a stem cell phenotype and induces chemoresistance in breast cancer cells by activating Wnt and Notch pathways. J. Exp. Clin. Cancer Res. 37:256. doi: 10.1186/s13046-018-0925-x
Yun, J., Pannuti, A., Espinoza, I., Zhu, H., Hicks, C., Zhu, X., et al. (2013). Crosstalk between PKCα and Notch-4 in endocrine-resistant breast cancer cells. Oncogenesis 2:e60. doi: 10.1038/oncsis.2013.26
Zang, S., Chen, F., Dai, J., Guo, D., Tse, W., Qu, X., et al. (2010). RNAi-mediated knockdown of Notch-1 leads to cell growth inhibition and enhanced chemosensitivity in human breast cancer. Oncol. Rep. 23, 893–899. doi: 10.3892/or_00000712
Zardawi, S. J., Zardawi, I., McNeil, C. M., Millar, E. K., McLeod, D., Morey, A. L., et al. (2010). High Notch1 protein expression is an early event in breast cancer development and is associated with the HER-2 molecular subtype. Histopathology 56, 286–296. doi: 10.1111/j.1365-2559.2009.03475.x
Zavadil, J., Cermak, L., Soto-Nieves, N., and Böttinger, E. P. (2004). Integration of TGF-beta/Smad and jagged1/Notch signalling in epithelial-to-mesenchymal transition. EMBO J. 23, 1155–1165. doi: 10.1038/sj.emboj.7600069
Zhang, J., Shao, X., Sun, H., Liu, K., Ding, Z., Chen, J., et al. (2016). NUMB negatively regulates the epithelial-mesenchymal transition of triple-negative breast cancer by antagonizing Notch signaling. Oncotarget 7, 61036–61053. doi: 10.18632/oncotarget.11062
Zhang, Q., Yuan, Y., Cui, J., Xiao, T., and Jiang, D. (2016). Paeoniflorin inhibits proliferation and invasion of breast cancer cells through suppressing Notch-1 signaling pathway. Biomed. Pharmacother. 78, 197–203. doi: 10.1016/j.biopha.2016.01.019
Zhang, X., Hoey, R., Koide, A., Dolios, G., Paduch, M., Nguyen, P., et al. (2014). A synthetic antibody fragment targeting nicastrin affects assembly and trafficking of γ-secretase. J. Biol. Chem. 289, 34851–34861. doi: 10.1074/jbc.M114.609636
Zhang, X., Liu, X., Luo, J., Xiao, W., Ye, X., Chen, M., et al. (2016). Notch3 inhibits epithelial-mesenchymal transition by activating Kibra-mediated Hippo/YAP signaling in breast cancer epithelial cells. Oncogenesis 5:e269. doi: 10.1038/oncsis.2016.67
Zhang, Y., Li, F., Song, Y., Sheng, X., Ren, F., Xiong, K., et al. (2016). Numb and Numbl act to determine mammary myoepithelial cell fate, maintain epithelial identity, and support lactogenesis. FASEB J. 30, 3474–3488. doi: 10.1096/fj.201600387R
Zhang, Y., Xie, Z. Y., Guo, X. T., Xiao, X. H., and Xiong, L. X. (2019). Notch and breast cancer metastasis: current knowledge, new sights and targeted therapy. Oncol. Lett. 18, 2743–2755. doi: 10.3892/ol.2019.10653
Zhang, Z., Wang, H., Ikeda, S., Fahey, F., Bielenberg, D., Smits, P., et al. (2010). Notch3 in human breast cancer cell lines regulates osteoblast-cancer cell interactions and osteolytic bone metastasis. Am. J. Pathol. 177, 1459–1469. doi: 10.2353/ajpath.2010.090476
Zheng, H., Bae, Y., Kasimir-Bauer, S., Tang, R., Chen, J., Ren, G., et al. (2017). Therapeutic antibody targeting tumor- and osteoblastic Niche-derived jagged1 sensitizes bone metastasis to chemotherapy. Cancer Cell 32, 731–747. doi: 10.1016/j.ccell.2017.11.002
Zhong, Y., Shen, S., Zhou, Y., Mao, F., Lin, Y., Guan, J., et al. (2016). NOTCH1 is a poor prognostic factor for breast cancer and is associated with breast cancer stem cells. Onco. Targets Ther. 9, 6865–6871. doi: 10.2147/OTT.S109606
Zhou, Y. F., Sun, Q., Zhang, Y. J., Wang, G. M., He, B., Qi, T., et al. (2017). Targeted inhibition of Notch1 gene enhances the killing effects of paclitaxel on triple negative breast cancer cells. Asian Pac. J. Trop. Med. 10, 179–183. doi: 10.1016/j.apjtm.2017.01.005
Keywords: Notch signalling, mammary gland development, breast cancer, cancer hallmarks, breast cancer therapy
Citation: Edwards A and Brennan K (2021) Notch Signalling in Breast Development and Cancer. Front. Cell Dev. Biol. 9:692173. doi: 10.3389/fcell.2021.692173
Received: 07 April 2021; Accepted: 07 June 2021;
Published: 06 July 2021.
Edited by:
Lisa M. Minter, University of Massachusetts Amherst, United StatesReviewed by:
Mohit Kumar Jolly, Indian Institute of Science (IISc), IndiaChun Ju Chang, University at Buffalo, United States
Clodia Osipo, Loyola University Chicago, United States
Copyright © 2021 Edwards and Brennan. This is an open-access article distributed under the terms of the Creative Commons Attribution License (CC BY). The use, distribution or reproduction in other forums is permitted, provided the original author(s) and the copyright owner(s) are credited and that the original publication in this journal is cited, in accordance with accepted academic practice. No use, distribution or reproduction is permitted which does not comply with these terms.
*Correspondence: Keith Brennan, a2VpdGguYnJlbm5hbkBtYW5jaGVzdGVyLmFjLnVr