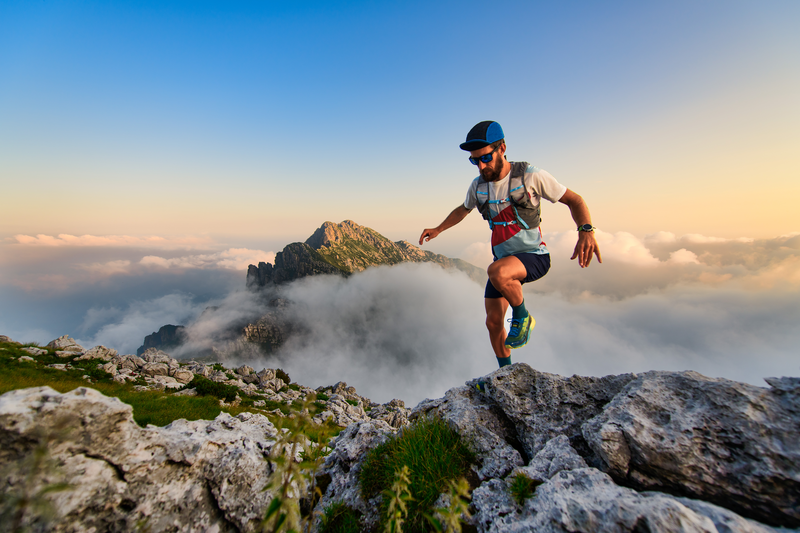
94% of researchers rate our articles as excellent or good
Learn more about the work of our research integrity team to safeguard the quality of each article we publish.
Find out more
ORIGINAL RESEARCH article
Front. Cell Dev. Biol. , 22 October 2021
Sec. Molecular and Cellular Oncology
Volume 9 - 2021 | https://doi.org/10.3389/fcell.2021.688949
This article is part of the Research Topic Advances in the Molecular Mechanisms in Gastrointestinal Tumorigenesis and Treatment View all 39 articles
A retraction of this article was approved in:
Retraction: NR2F1-AS1/miR-190a/PHLDB2 induces the epithelial–mesenchymal transformation process in gastric cancer by promoting phosphorylation of AKT3
Citation: Lv J, Zhang S, Liu Y, Li C, Guo T, Zhang S, Li Z, Jiao Z, Sun H, Zhang Y and Xu L (2021) NR2F1-AS1/miR-190a/PHLDB2 Induces the Epithelial–Mesenchymal Transformation Process in Gastric Cancer by Promoting Phosphorylation of AKT3. Front. Cell Dev. Biol. 9:688949. doi: 10.3389/fcell.2021.688949
Received: 31 March 2021; Accepted: 07 September 2021;
Published: 22 October 2021; Retracted: 11 September 2024.
Edited by:
Yu Zhang, Jinzhou Medical University, ChinaReviewed by:
Wanessa Altei, Barretos Cancer Hospital, BrazilDisclaimer: All claims expressed in this article are solely those of the authors and do not necessarily represent those of their affiliated organizations, or those of the publisher, the editors and the reviewers. Any product that may be evaluated in this article or claim that may be made by its manufacturer is not guaranteed or endorsed by the publisher.
Research integrity at Frontiers
Learn more about the work of our research integrity team to safeguard the quality of each article we publish.