- 1State Key Laboratory of Proteomics, Beijing Proteome Research Center, National Center for Protein Sciences (Beijing), Beijing Institute of Lifeomics, Beijing, China
- 2Department of Oral Pathology, Peking University School and Hospital of Stomatology, Beijing, China
- 3Department of Prosthodontics, Peking University School and Hospital of Stomatology, National Clinical Research Center for Oral Diseases, National Engineering Laboratory for Digital and Material Technology of Stomatology, Beijing Key Laboratory of Digital Stomatology, Beijing, China
Proper differentiation of odontoblasts is crucial for the development of tooth roots. Previous studies have reported the osteogenic/odontogenic potential of pre-odontoblasts during root odontoblast differentiation. However, the underlying molecular pathway that orchestrates these processes remains largely unclear. In this study, ablation of transforming growth factor-β receptor type 2 (Tgfbr2) in root pre-odontoblasts resulted in abnormal formation of root osteodentin, which was associated with ectopic osteogenic differentiation of root odontoblasts. Disrupting TGF-β signaling caused upregulation of Wnt signaling characterized by increased Wnt6, Wnt10a, Tcf-1, and Axin2 expression. Interestingly, inhibiting Wnt signaling by deleting Wntless (wls) in Osteocalcin (Ocn)-Cre; Tgfbr2fl/fl; Wlsfl/fl mice or overexpressing the Wnt antagonist Dkk1 in Ocn-Cre; Tgfbr2fl/fl; ROSA26Dkk1 mice decreased ectopic osteogenic differentiation and arrested odontoblast differentiation. Our results suggest that TGF-β signaling acts with Wnt signaling to regulate root odontogenic differentiation.
Introduction
The mechanism of crown dentinogenesis has been well studied; however, knowledge about root dentinogenesis is only emerging (Park et al., 2007; Zhang et al., 2015; Li et al., 2017). During root odontoblast differentiation, the cranial neural crest derived mesenchyme condenses around and continuously interacts with Hertwig’s epithelial root sheath (Huang et al., 2009). Subsequently, the apical papilla mesenchyme undergoes differentiation into pre-odontoblasts, which terminally differentiate to become odontoblasts (Ruch et al., 1995). This process is marked by the expression of several genes that encode collagenous and non-collagenous proteins that are also found in osteoblasts and the bone matrix (Gaikwad et al., 2001). The overlapping pattern of gene expression suggests the divergence of a common pool of progenitor cells for odontoblasts and osteoblasts. The molecular mechanism that controls the osteo/odontogenic differentiation of root dental mesenchyme remains largely unknown.
The results of our previous studies in mice revealed the critical function of Wnt/β-catenin signaling in root odontogenesis (Zhang et al., 2013); however, the precise regulatory role of Wnt signaling in root dentinogenesis remains to be clarified. Wnt proteins are a major family of developmentally important signaling molecules regulating multiple processes, including embryonic development, cell differentiation, and the specification of cell fate (Garcin and Habib, 2017; Ji et al., 2019; Xiong et al., 2019). Some Wnt ligands such as Wnt10a are spatiotemporally expressed with the differentiation stage of root odontoblasts during tooth development (Yamashiro et al., 2007). Dkk1, an inhibitor of Wnt signaling, is strongly expressed in pre-odontoblasts but its expression is lower in secretory odontoblasts (Fjeld et al., 2005). As a common Wnt target and a regulator of odontoblast differentiation, Runx2 induces transdifferentiation of odontoblasts into osteoblasts at the cell differentiation stage (Miyazaki et al., 2008). Therefore, Wnt signaling must be tightly controlled during root odontoblast differentiation; however, the molecular mechanisms that regulate this process remain unknown.
Transforming growth factor-β (TGF-β) signaling plays an important role in a broad range of cellular processes (Wu et al., 2016; Mullen and Wrana, 2017; Yu and Feng, 2019). Genetically modified mouse models have confirmed that a loss of responsiveness to TGF-β by odontoblasts results in aberrant pulp calcification; however, the underlying mechanisms have not been fully clarified (Wang et al., 2013; Ahn et al., 2015). Here, we specifically deleted TGF-β receptor type 2 (Tgfbr2) from root pre-odontoblasts and observed reduced odontogenic differentiation but ectopic expression of integrin bone sialoprotein (Ibsp) in the root odontoblast layer with upregulated Wnt signaling. Inhibiting Wnt signaling by either deleting Wntless (wls) in Ocn-Cre; Tgfbr2fl/fl; Wlsfl/fl mice or overexpressing Dkk1 by breeding with floxed ROSA26-loxP-stop-loxP-Dkk1 (ROSA26Dkk1) mice to generate Ocn-Cre; Tgfbr2fl/fl; ROSA26Dkk1 mice not only decreased ectopic osteogenic differentiation, but also arrested odontoblast differentiation. Our data suggest that during early root odontoblast differentiation, TGF-β signaling might act with Wnt signaling to initiate odontogenesis; while during root odontoblast maturation, TGF-β signaling specified the odontogenic lineage by suppressing Wnt signaling.
Materials and Methods
Generation of Mouse Strain
Generation of Osteocalcin (Ocn, also known as Bglap)-Cre; Tgfbr2fl/fl, Ocn-Cre; Wlsfl/fl, Ocn-Cre; Tgfbr2fl/fl; Wlsfl/fl, and Ocn-Cre; ROSA26EYFP mice had been described previously (Yang et al., 2014). The conditionally ROSA26Dkk1 mice (Wu et al., 2008) were bred with Ocn-Cre; Tgfbr2fl/fl. Tgfbr2fl/fl, Tgfbr2fl/fl; Wlsfl/fl, Tgfbr2fl/fl; ROSA26Dkk1 mice were used as controls. Mice were maintained on a C57BL/6 background and fed a normal rodent diet. For routine genotyping, Cre transgene were detected by PCR using primers described previously (Tan et al., 2007), and primers for ROSA26Dkk1 locus were designed as follows: forward, 5′-TACGAAGTTATTAGGTCCCTCG-3′, and reverse, 5′-TTGTTCCCGCCCTCATAGA-3′. Experimental protocols were designed based on the recommendation of the Beijing Experimental Animal Regulation Board (SYXK/JING/2005/0031).
Micro–Computed Tomography Analysis
The parameters of the Inveon MM system (Gantry-STD CT; Siemens) were set as follows: voltage of 60 kV, current of 220 mA, exposure time of 1500 ms, and effective pixel size of 8.89 μm. Sagittal images of the mandibles from postnatal day (P)30 Tgfbr2fl/fl; Wlsfl/fl mice, Ocn-Cre; Tgfbr2fl/fl mice, Ocn-Cre; Wlsfl/fl mice and Ocn-Cre; Tgfbr2fl/fl; Wlsfl/fl mice (n = 4 mice/genotype) were captured with Inveon software. The slices through the apical foramen of the first mandibular molar were chosen for quantitative analysis.
Histology and Immunohistochemistry
Mouse mandibles (n = 4 mice/genotype at P20, P30, and P120) were sectioned and were fixed in 4% paraformaldehyde/phosphate-buffered saline (PBS) overnight, then were decalcified and embedded in paraffin, and sectioned at 6 μm. Histological structures of mouse mandibular first molars were visualized using hematoxylin-eosin staining protocol. For immunohistochemical staining, antigen retrieval was performed in citrate buffer (pH 6.0) using a pressure cooker (Biocare Medical, Concord/California, biocare.net, DC2008). The primary antibodies used in the immunohistochemistry analyses were Tgfbr2 (Santa Cruz, sc-17792), β-catenin (BD Transduction Laboratories, 610153). Bound antibodies were visualized with diaminobenzidine, and sections were counterstained with hematoxylin, and observed under microscopy (Nikon, Eclipse E600).
In situ Hybridization and Multicolor Chromogenic in situ Hybridization and Immunohistochemistry
Sectioning of mouse mandibles (n = 4 mice/genotype at P12 and P20) was performed using standard protocols. Briefly, mouse mandibles were fixed in 4% paraformaldehyde, embedded in paraffin, and sectioned at 6 μm. Sections were heated at 63°C, de-waxed in xylene, rehydrated through a graded series of alcohol washes, and post-fixed with 4% paraformaldehyde in 0.01 mol L–1 PBS. 35S-dUTP (Perkin-Elmer, NEG009T001MC) and digoxigenin-11-UTP (Roche Applied Science, 11277073910) were employed to label RNA probes for collagen type I, alpha 1 (Col1a1), Ocn, dentin sialophosphoprotein (Dspp) and Ibsp using the MAXIscript in vitro transcription kit (Ambion, T3/AM1316, T7/AM1312). In situ hybridization of paraffin sections using 35S-UTP-labeled probes was performed with standard procedures (Yang et al., 2014). Sections were immersed in K5 emulsion (Ilford, 02746-50) for 5–30 days before development. For in situ hybridization with digoxigenin-11-UTP labeled RNA probes, sections were incubated in 1:2000 AP-conjugated, polyclonal sheep anti-digoxigenin antibody (Roche Applied Science, 11093274910) at 4°C for 10 hours. Immunoreactive cells were visualized with HighDef red (AP) (Enzo Life Sciences, ADI-950-140-0030). The primary antibodies used for immunohistochemistry following in situ hybridization were monoclonal rabbit anti-green fluorescent protein (GFP) (Cell Signaling Technology, 2956). HRP-conjugated secondary antibodies were then applied (ZsBio, PV-6001). Immunoreactive cells were visualized with HighDef blue (HRP) (Enzo Life Sciences, ADI-950-151-0006). The nucleus were counterstained with methyl green, and observed under microscopy (Nikon, Eclipse E600).
Statistical Analysis
Results (mean ± SD) were analyzed with Student’s t-test for independent samples or 1-way analysis of variance followed by Tukey’s post hoc test for pairwise comparisons (GraphPad Prism 7; GraphPad Software). Values of P < 0.05 were considered statistically significant.
Results
Disruption of Tgfbr2 in Odontoblasts Leads to Abnormal Osteodentin Formation in the Root
To test the role of TGF-β signaling in root dentinogenesis during the development of tooth roots, we employed the Ocn-Cre strain to disrupt TGF-β signaling in Ocn-Cre; Tgfbr2fl/fl mice. According to our previous study, Ocn-Cre recombinase activity is first detected in the primordial root at P10, in contrast to the intense expression in the developing crown odontoblasts and is active in the odontoblast lineages during root formation (Gao et al., 2009; Bae et al., 2013; Kim et al., 2013; Zhang et al., 2013). We then introduced Ocn-Cre and floxed ROSA26-loxP-stop-loxP-EYFP (ROSA26EYFP) mice to characterize which cell compartments in the tooth root harbored Ocn-Cre-mediated gene ablation. Cre-mediated recombination within the reporter locus activates enhanced yellow fluorescent protein (EYFP) expression and thus marks the recombined cells. In situ hybridization and immunohistochemical double labeling showed that odontoblasts lining the dentin layer are all EYFP+, but none of the EYFP+ cells in contact with Hertwig’s epithelial root sheath co-expressed Col1a1, Ocn, or Dspp,, which are markers of odontoblast differentiation (Supplementary Figure 1). These data demonstrate that the Ocn-Cre expression starts in pre-odontoblasts and leads to gene excision throughout the odontoblastic lineage.
Tgfbr2 protein was detected in the membrane and cytoplasm of pre-odontoblasts at P10 and P20 and was successfully ablated in pre-odontoblasts and odontoblasts in Ocn-Cre; Tgfbr2fl/fl mice as shown by immunohistochemistry (Figure 1A). The differentiating odontoblasts in Tgfbr2fl/fl mice were columnar at P30 when development of the first molar roots was near completion with the nucleus aligned away from the newly formed dentin (Figure 1B, blue arrowheads). The root dentin was deposited in a well-organized structure with a lightly stained and demarcated layer of pre-dentin (Figure 1B). However, the cells lining the Ocn-Cre; Tgfbr2fl/fl mouse tooth root were cuboidal and were entrapped in the newly formed matrix; this is similar to the way that osteoblasts differentiate (Figure 1B, red arrowheads). The pre-dentin layer of the mutant mice was lost and was replaced by osteodentin, a type of atubular dentin that resembles bone (Figure 1B).
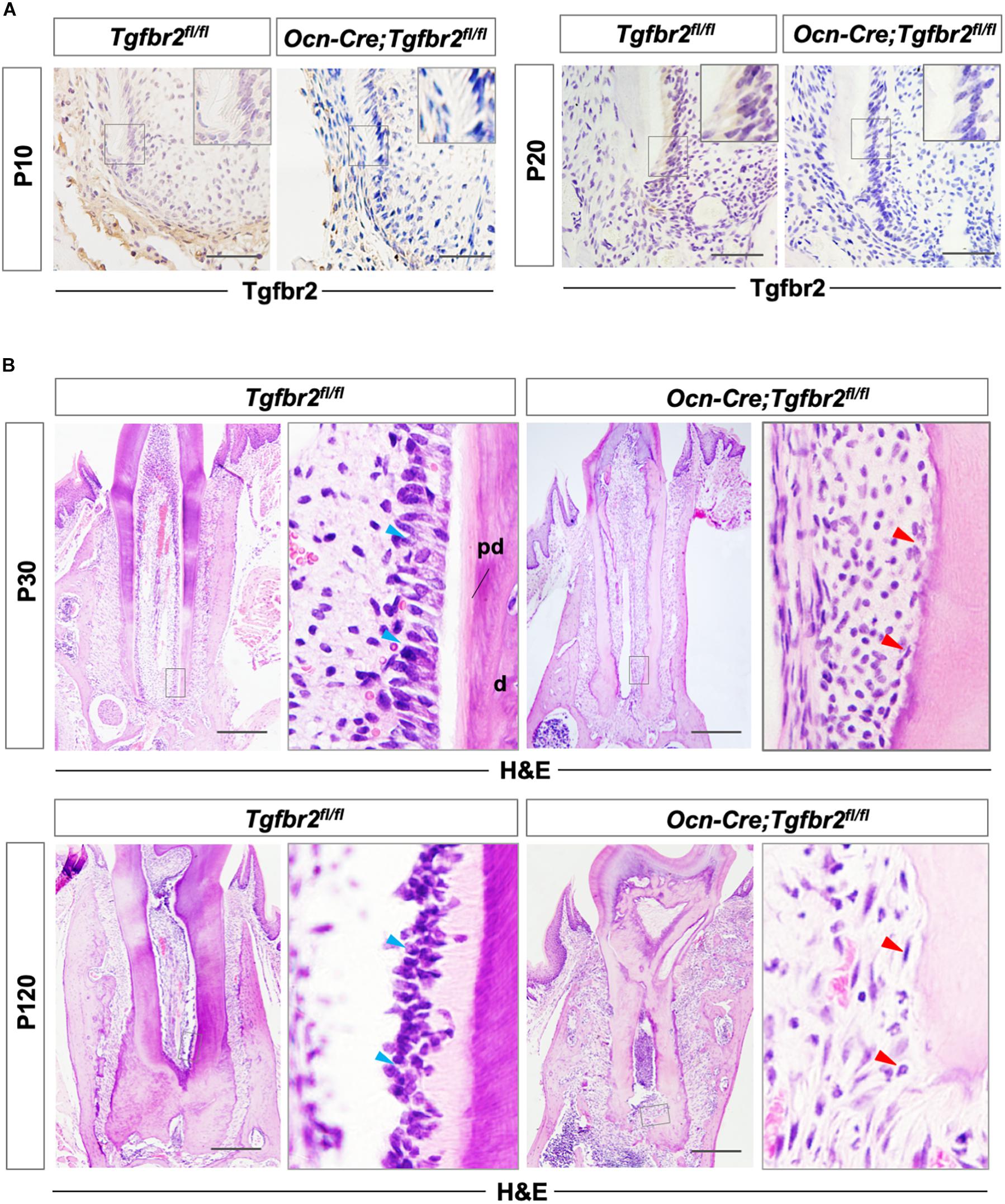
Figure 1. Disrupted tooth root development and formation of osteodentin in Ocn-Cre; Tgfbr2fl/fl mice. (A) Tgfbr2 is ablated in root odontoblasts in Ocn-Cre; Tgfbr2fl/fl mice at P10 and P20 (n = 4 mice/per genotype). The top right panels show high-magnification image of the area boxed in gray on the left. (B) Abnormal root odontoblasts and root dentin formation in Ocn-Cre; Tgfbr2fl/fl mice at P30 and P120. The right panels show the high-magnification image of the area boxed in gray on the left. Blue arrowheads indicate columnar-shaped odontoblasts in Tgfbr2fl/fl mice, red arrowheads indicate irregular-shaped odontoblasts and formation of osteodentin in Ocn-Cre; Tgfbr2fl/fl mice. pd, pre-dentin; d, dentin. Scale bars: 20 μm (A), 100 μm (B).
The Formation of Osteodentin Is Associated With Reduced Odontogenic Differentiation and Ectopic Osteogenic Differentiation
The ectopic formation of osteodentin in Ocn-Cre; Tgfbr2fl/fl mice suggests the possibility of a change in cell fate in mutant odontoblasts. To test this hypothesis, we performed in situ hybridization using osteo/odontogenic differentiation markers. The expression levels of Col1a1 and Ocn decreased in the Ocn-Cre; Tgfbr2fl/fl mice compared to Tgfbr2fl/fl mice (Figure 2A, blue and red arrowheads). Expression of Dentin matrix protein 1 (Dmp1), a marker for early odontoblast differentiation, was evident in the root odontoblast layer of Tgfbr2fl/fl mice, whereas it was sharply reduced in Ocn-Cre; Tgfbr2fl/fl mice (Figure 2B, blue and red arrowheads). As a specific marker for mature odontoblasts, expression of Dspp was barely seen in the Ocn-Cre; Tgfbr2fl/fl mice (Figure 2B, blue and red arrowheads). Interestingly, expression of the transcription factor Runx2, which is critical for osteogenic differentiation (Komori et al., 1997), increased in the odontoblast layer (Figure 2C, blue and red arrowheads). Strong ectopic expression of Ibsp, a marker for osteoblasts, was detected in the odontoblast layer of Ocn-Cre; Tgfbr2fl/fl mice, whereas it was undetectable in Tgfbr2fl/fl mice (Figure 2C, blue and red arrowheads). The ectopic expression of osteogenic differentiation markers suggested a change in cell fate in odontoblasts from Ocn-Cre; Tgfbr2fl/fl mice.
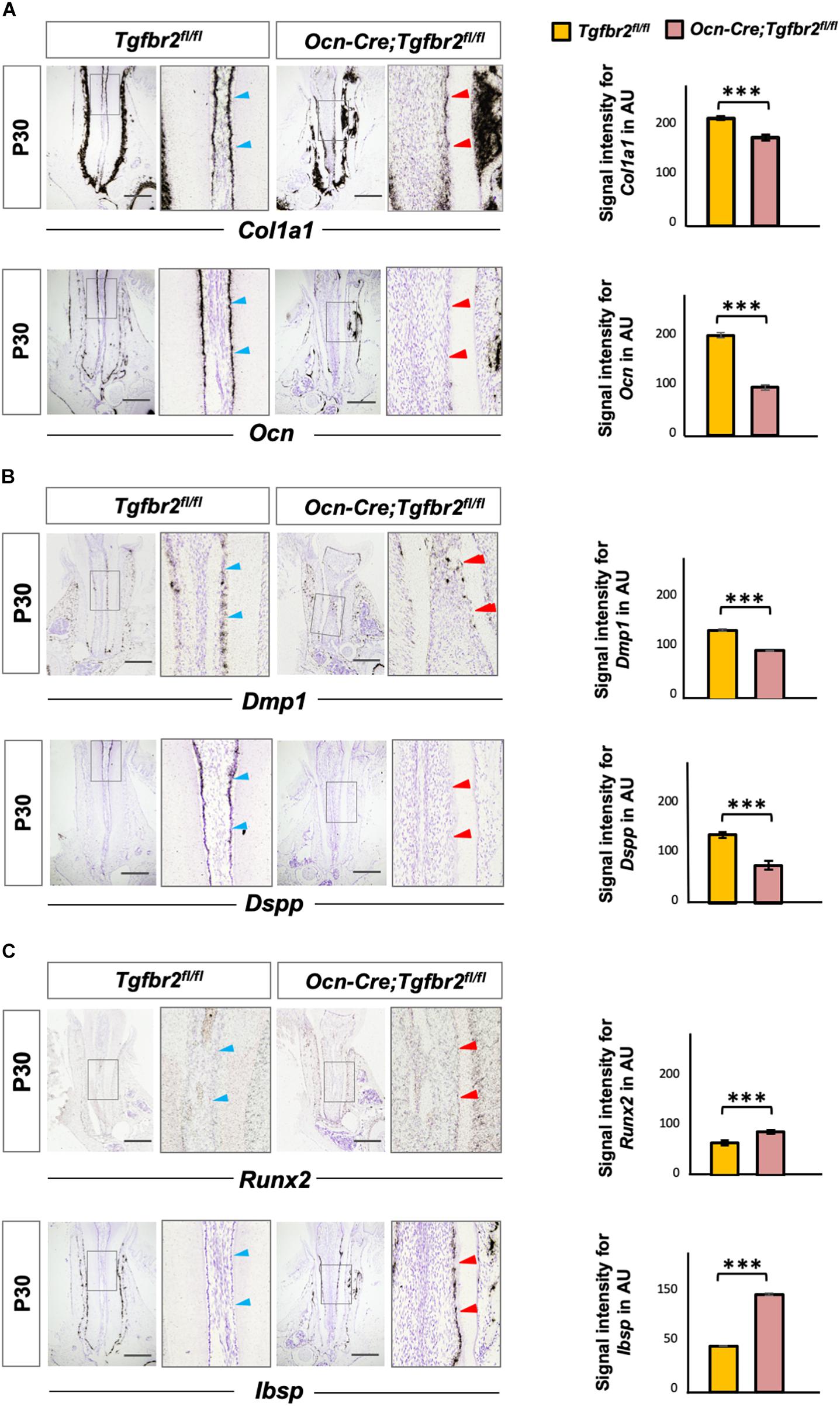
Figure 2. Decreased expression of odontogenic markers and ectopic expression of osteogenic markers in Ocn-Cre; Tgfbr2fl/fl mice. (A) Decreased expression of Col1a1 and Ocn in the root odontoblasts of Ocn-Cre; Tgfbr2fl/fl mice were indicated by red arrowheads, as compared to that in the Tgfbr2fl/fl littermates at P30 (blue arrowheads). (B) Reduced expression of Dmp1 and diminished expression of Dspp in the root odontoblasts of Ocn-Cre; Tgfbr2fl/fl mice at P30, red arrowheads indicate. (C) Upregulated expression of Runx2, and ectopic expression of Ibsp in the root odontoblasts of Ocn-Cre; Tgfbr2fl/fl mice at P30, red arrowheads indicate. The right panels show the high-magnification image of the area boxed in gray on the left in (A–C). The right images of bar graphs are quantification of signal intensity for Col1a1, Ocn, Dmp1, Dspp, Runx2 and Ibsp expression as shown in (A–C), respectively, using ImageJ. Data are presented as the means ± standard deviation (SD). ***P < 0.001, n = 4 areas from three mice. Blue arrowheads indicate expression of differentiation markers in Tgfbr2fl/fl mice, red arrowheads indicate abnormal expression of differentiation markers in Ocn-Cre; Tgfbr2fl/fl mice. AU, arbitrary unit. Scale bars: 100 μm.
Increased Wnt Signaling Activity in Teeth From Ocn-Cre; Tgfbr2fl/fl Mice
Wnt signaling is a key determinant of cell fate and a promotor of osteogenic differentiation in multiple cell types (Brack et al., 2007; Cawthorn et al., 2012; Zhu et al., 2019). We hypothesized that ectopic expression of Ibsp in mutant odontoblasts was due to over-activation of Wnt signaling. As a member of the canonical Wnt pathway, overexpressing Wnt6 could enhance the osteogenic differentiation of human dental papilla cells (hDPCs) with up-regulated expression of mineralization-related genes such as alkaline phosphatase (ALP), Col1a1, Ocn, Osteopontin, and Ibsp (Wang et al., 2010). Wnt10a has been reported to regulate osteogenesis in mesenchymal stem cells, and T cell factor 1 (Tcf-1), which is a nuclear transcription factor, potentially bound to the promoter region of Runx2 and determined the osteogenic lineage in stem cells from human apical papilla cells (Zhou et al., 2019). Therefore, we detected expression of Wnt6, Wnt10a, and Tcf-1 by in situ hybridization. Transcription of Wnt6, Wnt10a, and Tcf-1 was upregulated in Ocn-Cre; Tgfbr2fl/fl mice (Figures 3A–C, blue and red arrowheads). We also analyzed the expression of Axin2, a canonical Wnt target gene, and found it was increased significantly in Ocn-Cre; Tgfbr2fl/fl mice (Figure 3D, blue and red arrowheads).
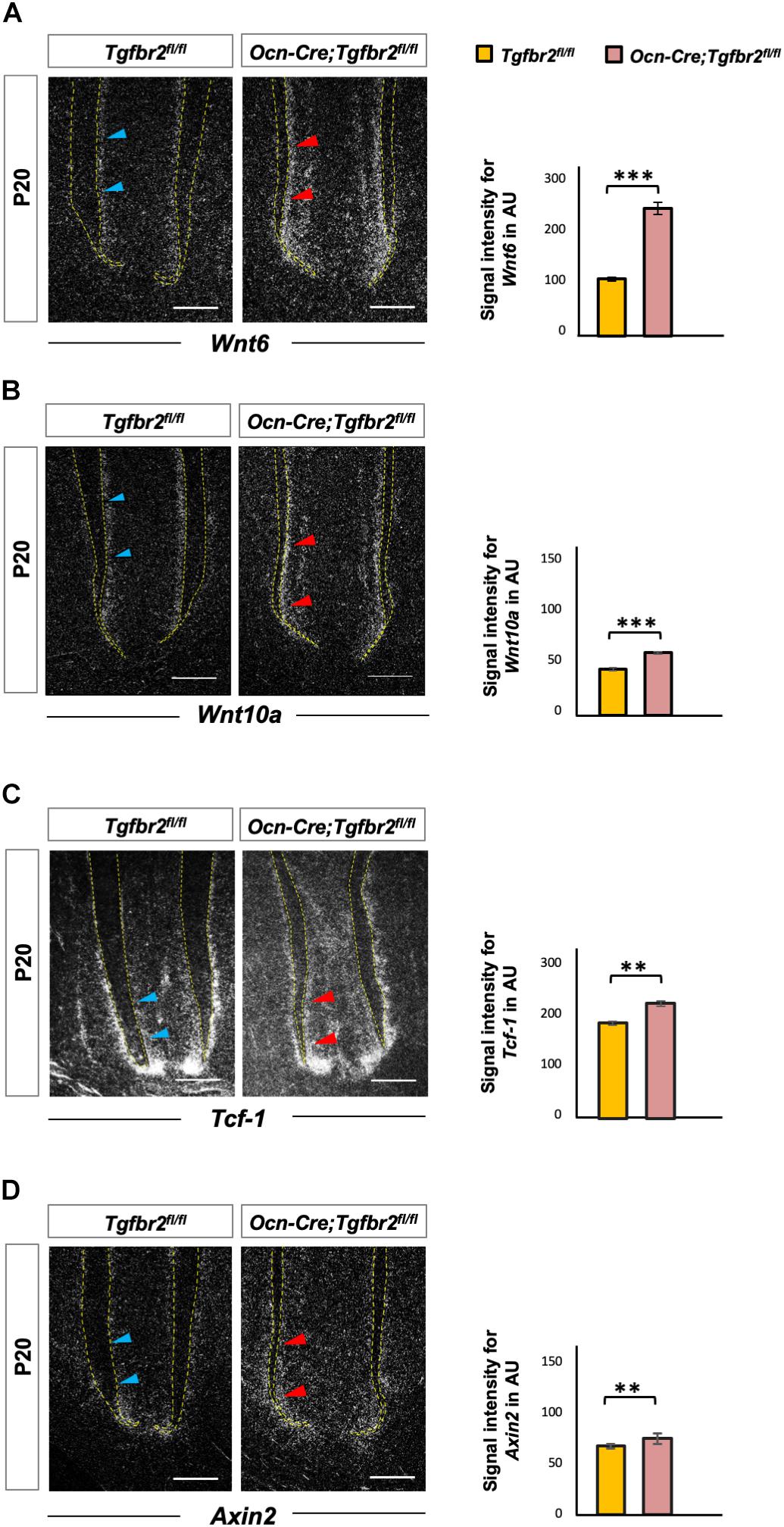
Figure 3. Increased Wnt signaling in the root pre-odontoblasts of Ocn-Cre; Tgfbr2fl/fl mice. (A,B) Elevated expression of Wnt ligands, Wnt6 and Wnt10a, in root odontoblasts of the Ocn-Cre; Tgfbr2fl/fl mice at P20 as indicated by red arrowheads. (C,D) Increased expression of Wnt signaling read-out genes in the root odontoblasts of Ocn-Cre; Tgfbr2fl/fl mice compared to the Tgfbr2fl/fl littermates as indicated by red arrowheads at P20. N = 4 mice/per genotype in (A–D). The right images of bar graphs are quantification of signal intensity for Wnt6, Wnt10a, Tcf-1, and Axin2 expression as shown in (A–D) using ImageJ. Data are presented as the means ± standard deviation (SD). ***P < 0.001, **P < 0.01, n = 4 areas from three mice. Blue arrowheads in (A–D) indicate expression of Wnt ligands and related genes in Tgfbr2fl/fl mice. Scale bars: 50 μm.
Inhibition of Wnt Signaling in Tgfbr2-Deficient Mice Decreases Ectopic Osteogenic Differentiation and Arrests Odontoblast Differentiation
To further verify the involvement of upregulated Wnt signaling in the determination of osteo/odontogenic cell fate in Ocn-Cre; Tgfbr2fl/fl mice, we specifically inhibited Wnt signaling by depleting Wls, an essential Wnt ligand transporter, to generate Ocn-Cre; Tgfbr2fl/fl; Wlsfl/fl mice. Tooth length was strikingly short in the Ocn-Cre; Tgfbr2fl/fl; Wlsfl/fl mice (Figures 4A,B). Quantification of micro-computed tomography data and Sirius Red staining revealed a striking reduction in dentin volume and dentin thickness in Ocn-Cre; Tgfbr2fl/fl; Wlsfl/fl mice compared to Tgfbr2fl/fl; Wlsfl/fl mice, Ocn-Cre; Tgfbr2fl/fl mice, and Ocn-Cre; Wlsfl/fl mice, respectively (Figures 4C,D). Dkk1, a secreted protein that functions as a negative regulator of Wnt signaling by forming a complex with LRP 5/6 to inhibit binding of Wnt ligands to receptors (Glinka et al., 1998; Wu et al., 2008), was introduced and overexpressed in pre-odontoblasts by Ocn-Cre and ROSA26Dkk1 mice. Similarly, the formation of root dentin in Ocn-Cre; ROSA26Dkk1 mice was almost the same as that in Tgfbr2fl/fl; ROSA26Dkk1 mice (Supplementary Figure 2B). In contrast, the roots were much shorter in the Ocn-Cre; Tgfbr2fl/fl; ROSA26Dkk1 mice, and the dentin were thinner than that in the control littermates (Supplementary Figure 2B). Expression of the early differentiation markers Col1a1 and Ocn decreased sharply in Ocn-Cre; Tgfbr2fl/fl; Wlsfl/fl mice (Figures 4E,F, blue and red arrowheads), indicating a decreased differentiation ability at the early stage of odontoblast differentiation. As a major marker of odontoblastic differentiation, Dspp was undetectable in the root odontoblast layer of Ocn-Cre; Tgfbr2fl/fl; Wlsfl/fl mice (Figure 4G, blue and red arrowheads). We did not observe expression of Ibsp in the dentin region of Ocn-Cre; Tgfbr2fl/fl; Wlsfl/fl mice, which was evident in the Ocn-Cre; Tgfbr2fl/fl mice (Figure 4H, blue and red arrowheads). These data suggest that disrupting Wnt signaling in Ocn-Cre; Tgfbr2fl/fl mice led to arrested differentiation of root odontoblasts.
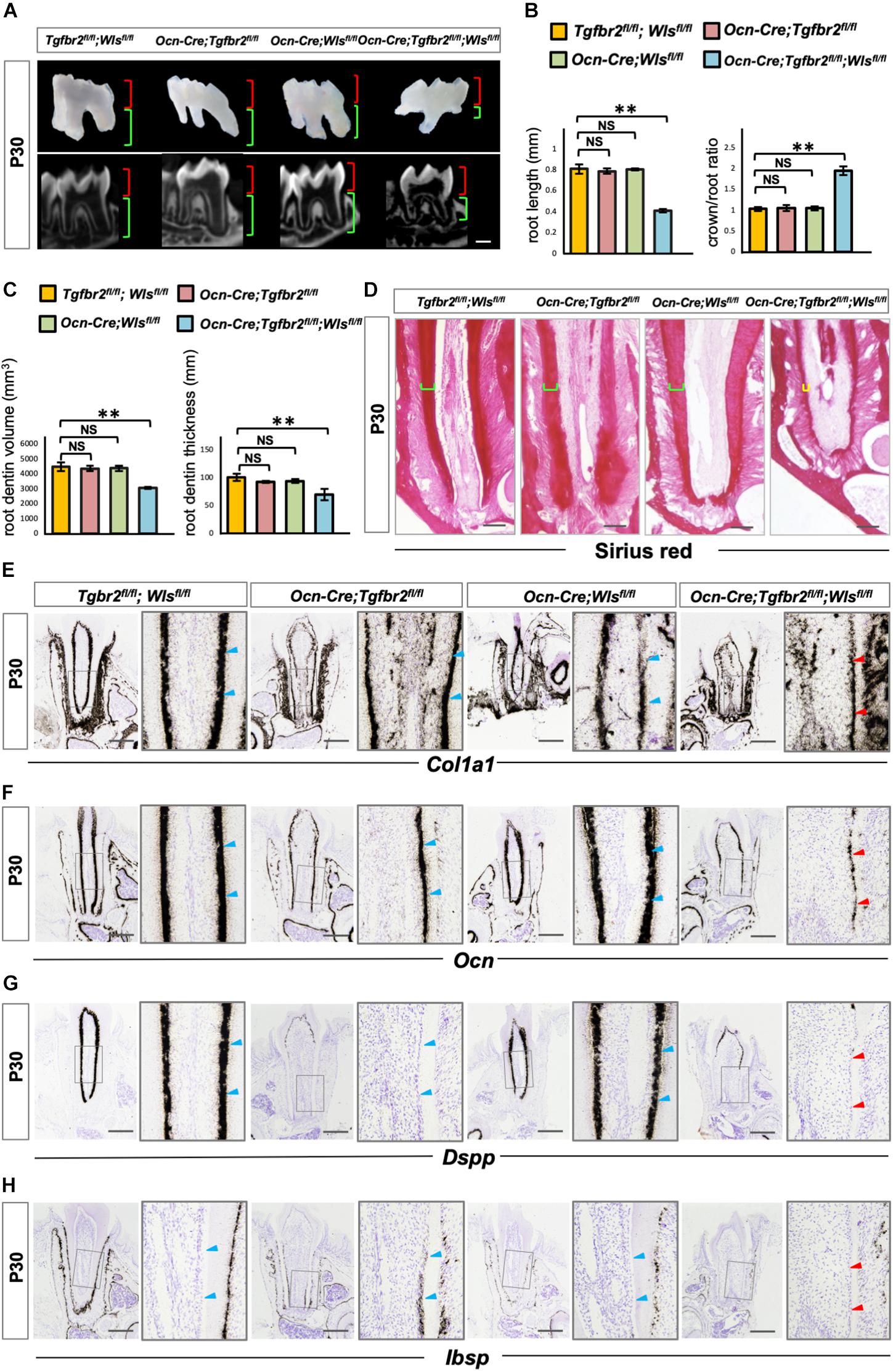
Figure 4. Arrested root odontoblast differentiation in Ocn-Cre; Tgfbr2fl/fl; Wlsfl/fl mice. (A,B) Significant short molar roots in Ocn-Cre; Tgfbr2fl/fl; Wlsfl/fl mice compared to Tgfbr2fl/fl; Wlsfl/fl mice, Ocn-Cre; Tgfbr2fl/fl mice and Ocn-Cre; Wlsfl/fl mice at P30. Data are presented as the means ± standard deviation (SD). **P < 0.01, n = 4 mice/per genotype. (C,D) Reductions in root dentin volume and dentin thickness in Ocn-Cre; Tgfbr2fl/fl; Wlsfl/fl mice at P30. Data are presented as the means ± standard deviation (SD). **P < 0.01, n = 4 mice/per genotype. (E,F) Expression of osteo/odontogenic differentiation markers is sharply reduced in Ocn-Cre; Tgfbr2fl/fl; Wlsfl/fl mice at P30 (n = 4 mice/per genotype). (G,H) Diminished expression of Dspp and Ibsp in root odontoblasts of Ocn-Cre; Tgfbr2fl/fl; Wlsfl/fl mice at P30 (n = 4 mice/per genotype). Blue arrowheads indicate expression of differentiation markers in Tgfbr2fl/fl; Wlsfl/fl mice, Ocn-Cre; Tgfbr2fl/fl mice and Ocn-Cre; Wlsfl/fl mice, red arrowheads indicate sharp reduction in expression of differentiation markers in Ocn-Cre; Tgfbr2fl/fl; Wlsfl/fl mice. The right panels show the high-magnification image of the area boxed in gray on the left in (E–H). NS, non-significant. Scale bars: 300 μm (A), 50 μm (D), 100 μm (E–H).
β-Catenin Expression is Reduced in Differentiated Root Odontoblasts From Ocn-Cre; Tgfbr2fl/fl; Wlsfl/fl Mice
Our previous study reported the critical role of β-catenin in the differentiation of root odontoblasts by showing the lack of expression of the odontogenic differentiation markers Col1a1, Ocn, and Dspp in Ocn-Cre; Ctnnb1fl/fl mice (Zhang et al., 2013). Similar to the previous study, the expression of odontoblastic differentiation markers in Ocn-Cre; Tgfbr2fl/fl; Wlsfl/fl mice decreased significantly. We hypothesized that arrested root odontoblast differentiation was correlated to the reduced expression of β-catenin. We observed attenuated expression of Wnt6, Wnt10a, Tcf-1, and Axin2 in root odontoblasts of Ocn-Cre; Tgfbr2fl/fl; Wlsfl/fl mice by in situ hybridization (Figure 5A, red arrowheads). As the direct downstream target, Runx2 expression was also downregulated in Ocn-Cre; Tgfbr2fl/fl; Wlsfl/fl mice (Figure 5A, red arrowheads). Notably, β-catenin expression was reduced in the differentiated odontoblast layer of Ocn-Cre; Tgfbr2fl/fl; Wlsfl/fl mice (Figure 5B, blue and red arrowheads), indicating that the integrity of β-catenin-mediated pathway might be required for TGF-β/Wnt signaling to initiate the root odontogenic differentiation.
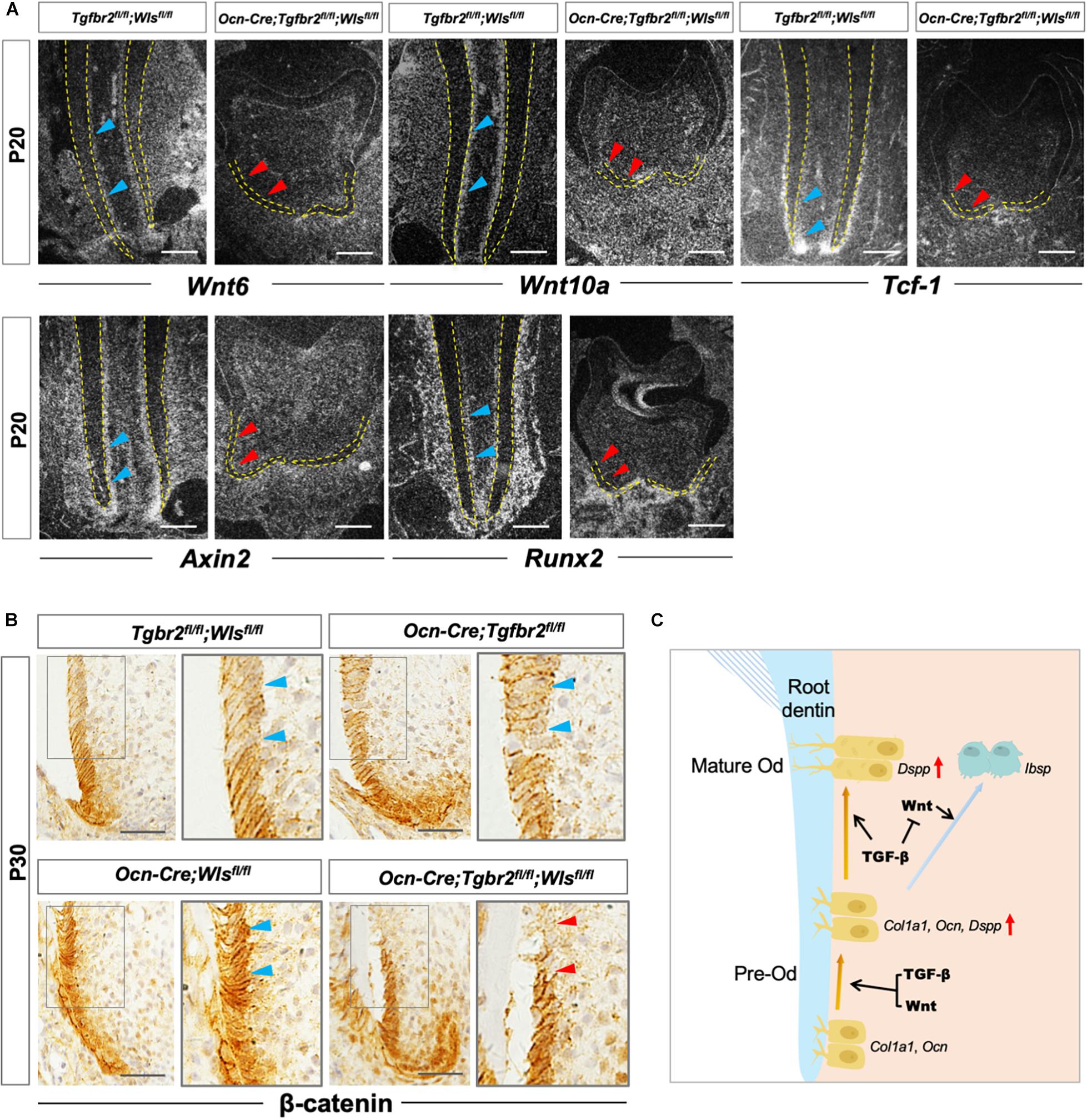
Figure 5. Compromised Wnt/β-catenin signaling in Ocn-Cre; Tgfbr2fl/fl; Wlsfl/fl mice. (A) Downregulated expression of Wnt related genes and Runx2 in Ocn-Cre; Tgfbr2fl/fl; Wlsfl/fl mice indicated by red arrowheads at P20. (B) Reduced expression of β-catenin in odontoblasts of Ocn-Cre; Tgfbr2fl/fl; Wlsfl/fl mice indicated by red arrowheads at P30. (C) The scheme representation of possible mechanisms of TGF-β and Wnt signaling pathways in pre-odontoblast differentiation during tooth root development. Blue arrowheads indicate expression of Wnt6, Wnt10a, Tcf-1, Axin2, and Runx2 in Tgfbr2fl/fl; Wlsfl/fl mice (A), and expression of β-catenin in Tgfbr2fl/fl; Wlsfl/fl mice, Ocn-Cre; Tgfbr2fl/fl mice, and Ocn-Cre; Wlsfl/fl mice (B). Red arrowheads indicate downregulated expression of Wnt6, Wnt10a, Tcf-1, Axin2, and Runx2 (A), and reduced β-catenin expression in Ocn-Cre; Tgfbr2fl/fl; Wlsfl/fl mice (B). N = 4 mice/per genotype in (A,B). The right panels show the high-magnification image of the area boxed in gray on the left. Od, odontoblast. Scale bars: 50 μm (A) and 25 μm (B).
Discussion
The role of TGF-β signaling pathway that regulates odontoblast differentiation has been reported in several animal models (Oka et al., 2007; Kim et al., 2015). For example, Smad4-mediated TGF-β/bone morphogenetic protein signaling maintain Wnt activity in the dental mesenchyme to ensure a proper cranial neural crest cell fate decision during the early stage of tooth morphogenesis (Li et al., 2011). However, root odontoblast differentiation occurs at the late stage of tooth development and was thought to have a different regulatory mechanism from crown odontoblast differentiation. Indeed, during tooth root development, p-Smad2/3 is strongly expressed in dental papilla, moderately expressed in odontoblasts, indicating a stage-specific role of TGF-β signaling in root odontoblast differentiation (Li and Pan, 2018). Interestingly, many Wnt family members are also differentially expressed in the developing tooth root pre-odontoblasts and mature odontoblasts, for example, Wnt6, Wnt10a (Yamashiro et al., 2007), and their downstream targets Tcf-1 and Axin2, suggesting that Wnt signaling might function in a synergic way with TGF-β signaling (Lohi et al., 2010). To clarify this, we generated a serial of Ocn-Cre; Tgfbr2fl/fl, Ocn-Cre; Tgfbr2fl/fl; Wlsfl/fl, and Ocn-Cre; Tgfbr2fl/fl; ROSA26Dkk1 mice, and provided in vivo evidences that TGF-β signaling is required for the root odontogenesis by cooperation with Wnt signaling, whereas inhibits osteogenic potential to insure the specified odontogenic lineage, which might be achieved through suppressing Wnt expression (Figure 5C). In accordance with our results, previous studies have also observed a calcified mass in the pulp chamber after Tgfbr2 ablation in the dental mesenchyme, however, the cell source as well as the downstream effectors that produced the ectopic bone-like structures have not been demonstrated (Wang et al., 2013; Ahn et al., 2015). Study showed that overexpressing Runx2, a master regulator of osteoblast differentiation, in odontoblasts inhibits the terminal odontogenic differentiation and reversed them to a osteogenic phenotype (Miyazaki et al., 2008). Since Wnt signaling is an evolutionarily conserved intracellular signaling that plays an important role in the osteogenic differentiation, it is likely that TGF-β might inhibit Wnt signaling to reduce Runx2 expression, which might explain how TGF-β signaling functions in directing the osteo/odontogenic phenotype of mature root odontoblasts. However, the molecular mechanisms underlying these regulations need to be further clarified.
Our study also suggested that β-catenin might mediate the cooperative function of TGF-β signaling and Wnt signaling during the early stage of odontogenic differentiation. The interaction of TGF-β and Wnt signaling has been reported and explained in several developmental events, such as formation of the organizer and differentiation of chondrocytes (Nishita et al., 2000; Zhou et al., 2004). Several studies reported the importance of target gene co-regulation by TGF-β and Wnt signaling throughout development. In human marrow stromal cells, TGF-β synergize with Wnt signaling in the inhibition of adipocyte differentiation (Zhou et al., 2004). In the Ocn-Cre; Ctnnb1fl/fl mouse model that we generated previously, we and others discovered a critical role of β-catenin in root dentinogenesis (Kim et al., 2013; Zhang et al., 2013). However, how β-catenin is regulated during root odontoblast differentiation is largely unknown. The reduced β-catenin expression in Ocn-Cre; Tgfbr2fl/fl; Wlsfl/fl differentiated root odontoblasts suggests the possible mechanism that the integrity of β-catenin is synergistically regulated by TGF-β and Wnt signaling, leading to undifferentiated odontoblasts and extremely thin root dentin. These data are consistent with the observations of other studies that TGF-β signaling interacts with Wnt signaling to activate the transcription of several genes via β-catenin-mediated signaling (Nishita et al., 2000; Zhou et al., 2004; Liu et al., 2006). TGF-β and Wnt signaling interacts physically by forming a complex composed of β-catenin, Lef1/Tcf-1 and Smad4 to bind to the twin (Xtwn) promoter region. This interaction directly and synergistically affects expression of the twin gene during formation of the organizer in Xenopus (Nishita et al., 2000). To test this hypothesis, future studies should constitutively stabilize β-catenin in mice to rescue the arrested root odontoblast differentiation in Ocn-Cre; Tgfbr2fl/fl; Wlsfl/fl mice.
Data Availability Statement
The original contributions presented in the study are included in the article/Supplementary Material, further inquiries can be directed to the corresponding author/s.
Ethics Statement
The animal study was reviewed and approved by the Beijing Experimental Animal Regulation Board (SYXK/JING/2005/0031).
Author Contributions
RZ contributed to conception and design, methodology, data acquisition, analysis, interpretation, and drafted the manuscript. JL, SY, and YL contributed to data acquisition and analysis and drafted the manuscript. QH contributed to data interpretation and analysis and drafted the manuscript. LZ contributed to data acquisition and drafted the manuscript. XY contributed to conception and design and critically revised the manuscript. GY contributed to conception and design, methodology, data acquisition, analysis, interpretation, and critically revised the manuscript. All authors gave final approval and agreed to be accountable for all aspects of the work.
Funding
This study was supported by grants from the State Key Program of National Natural Science of China (31630093), National Natural Science Foundation of China (31871476, 31571512, and 81702689), and Beijing Nova Program (Z161100004916146).
Conflict of Interest
The authors declare that the research was conducted in the absence of any commercial or financial relationships that could be construed as a potential conflict of interest.
Supplementary Material
The Supplementary Material for this article can be found online at: https://www.frontiersin.org/articles/10.3389/fcell.2021.687099/full#supplementary-material
References
Ahn, Y. H., Kim, T. H., Choi, H., Bae, C. H., Yang, Y. M., Baek, J. A., et al. (2015). Disruption of Tgfbr2 in odontoblasts leads to aberrant pulp calcification. J. Dent. Res. 94, 828–835. doi: 10.1177/0022034515577427
Bae, C. H., Lee, J. Y., Kim, T. H., Baek, J. A., Lee, J. C., Yang, X., et al. (2013). Excessive Wnt/beta-catenin signaling disturbs tooth-root formation. J. Periodontal Res. 48, 405–410. doi: 10.1111/jre.12018
Brack, A. S., Conboy, M. J., Roy, S., Lee, M., Kuo, C. J., Keller, C., et al. (2007). Increased Wnt signaling during aging alters muscle stem cell fate and increases fibrosis. Science 317, 807–810. doi: 10.1126/science.1144090
Cawthorn, W. P., Bree, A. J., Yao, Y., Du, B., Hemati, N., Martinez-Santibanez, G., et al. (2012). Wnt6, Wnt10a and Wnt10b inhibit adipogenesis and stimulate osteoblastogenesis through a beta-catenin-dependent mechanism. Bone 50, 477–489. doi: 10.1016/j.bone.2011.08.010
Fjeld, K., Kettunen, P., Furmanek, T., Kvinnsland, I. H., and Luukko, K. (2005). Dynamic expression of Wnt signaling-related Dickkopf1, -2, and -3 mRNAs in the developing mouse tooth. Dev. Dyn. 233, 161–166. doi: 10.1002/dvdy.20285
Gaikwad, J. S., Hoffmann, M., Cavender, A., Bronckers, A. L., and D’souza, R. N. (2001). Molecular insights into the lineage-specific determination of odontoblasts: the role of Cbfa1. Adv. Dent. Res. 15, 19–24. doi: 10.1177/08959374010150010501
Gao, Y., Yang, G., Weng, T., Du, J., Wang, X., Zhou, J., et al. (2009). Disruption of Smad4 in odontoblasts causes multiple keratocystic odontogenic tumors and tooth malformation in mice. Mol. Cell Biol. 29, 5941–5951. doi: 10.1128/mcb.00706-09
Garcin, C. L., and Habib, S. J. (2017). A comparative perspective on Wnt/beta-catenin signalling in cell fate determination. Results Probl. Cell Differ. 61, 323–350. doi: 10.1007/978-3-319-53150-2_15
Glinka, A., Wu, W., Delius, H., Monaghan, A. P., Blumenstock, C., and Niehrs, C. (1998). Dickkopf-1 is a member of a new family of secreted proteins and functions in head induction. Nature 391, 357–362. doi: 10.1038/34848
Huang, X., Bringas, P. Jr., Slavkin, H.C., and Chai, Y. (2009). Fate of HERS during tooth root development. Dev. Biol. 334, 22–30. doi: 10.1016/j.ydbio.2009.06.034
Ji, Y., Hao, H., Reynolds, K., Mcmahon, M., and Zhou, C. J. (2019). Wnt signaling in neural crest ontogenesis and oncogenesis. Cells 8:1173. doi: 10.3390/cells8101173
Kim, T. H., Bae, C. H., Lee, J. C., Ko, S. O., Yang, X., Jiang, R., et al. (2013). beta-catenin is required in odontoblasts for tooth root formation. J. Dent. Res. 92, 215–221. doi: 10.1177/0022034512470137
Kim, T. H., Bae, C. H., Lee, J. Y., Lee, J. C., Ko, S. O., Chai, Y., et al. (2015). Temporo-spatial requirement of Smad4 in dentin formation. Biochem. Biophys. Res. Commun. 459, 706–712. doi: 10.1016/j.bbrc.2015.03.014
Komori, T., Yagi, H., Nomura, S., Yamaguchi, A., Sasaki, K., Deguchi, K., et al. (1997). Targeted disruption of Cbfa1 results in a complete lack of bone formation owing to maturational arrest of osteoblasts. Cell 89, 755–764. doi: 10.1016/s0092-8674(00)80258-5
Li, J., Huang, X., Xu, X., Mayo, J., Bringas, P. Jr., Jiang, R., et al. (2011). SMAD4-mediated WNT signaling controls the fate of cranial neural crest cells during tooth morphogenesis. Development 138, 1977–1989. doi: 10.1242/dev.061341
Li, J., Parada, C., and Chai, Y. (2017). Cellular and molecular mechanisms of tooth root development. Development 144, 374–384. doi: 10.1242/dev.137216
Li, S., and Pan, Y. (2018). Immunolocalization of connective tissue growth factor, transforming growth factor-beta1 and phosphorylated-SMAD2/3 during the postnatal tooth development and formation of junctional epithelium. Ann. Anat. 216, 52–59. doi: 10.1016/j.aanat.2017.10.005
Liu, W., Rui, H., Wang, J., Lin, S., He, Y., Chen, M., et al. (2006). Axin is a scaffold protein in TGF-beta signaling that promotes degradation of Smad7 by Arkadia. EMBO J. 25, 1646–1658. doi: 10.1038/sj.emboj.7601057
Lohi, M., Tucker, A. S., and Sharpe, P. T. (2010). Expression of Axin2 indicates a role for canonical Wnt signaling in development of the crown and root during pre- and postnatal tooth development. Dev. Dyn. 239, 160–167.
Miyazaki, T., Kanatani, N., Rokutanda, S., Yoshida, C., Toyosawa, S., Nakamura, R., et al. (2008). Inhibition of the terminal differentiation of odontoblasts and their transdifferentiation into osteoblasts in Runx2 transgenic mice. Arch. Histol. Cytol. 71, 131–146. doi: 10.1679/aohc.71.131
Mullen, A. C., and Wrana, J. L. (2017). TGF-beta family signaling in embryonic and somatic stem-cell renewal and differentiation. Cold Spring Harb. Perspect. Biol. 9:a022186. doi: 10.1101/cshperspect.a022186
Nishita, M., Hashimoto, M. K., Ogata, S., Laurent, M. N., Ueno, N., Shibuya, H., et al. (2000). Interaction between Wnt and TGF-beta signalling pathways during formation of Spemann’s organizer. Nature 403, 781–785. doi: 10.1038/35001602
Oka, S., Oka, K., Xu, X., Sasaki, T., Bringas, P. Jr., and Chai, Y. (2007). Cell autonomous requirement for TGF-beta signaling during odontoblast differentiation and dentin matrix formation. Mech. Dev. 124, 409–415. doi: 10.1016/j.mod.2007.02.003
Park, J. C., Herr, Y., Kim, H. J., Gronostajski, R. M., and Cho, M. I. (2007). Nfic gene disruption inhibits differentiation of odontoblasts responsible for root formation and results in formation of short and abnormal roots in mice. J. Periodontol. 78, 1795–1802. doi: 10.1902/jop.2007.060363
Ruch, J. V., Lesot, H., and Begue-Kirn, C. (1995). Odontoblast differentiation. Int. J. Dev. Biol. 39, 51–68.
Tan, X., Weng, T., Zhang, J., Wang, J., Li, W., Wan, H., et al. (2007). Smad4 is required for maintaining normal murine postnatal bone homeostasis. J. Cell Sci. 120, 2162–2170. doi: 10.1242/jcs.03466
Wang, C., Ren, L., Peng, L., Xu, P., Dong, G., and Ye, L. (2010). Effect of Wnt6 on human dental papilla cells in vitro. J. Endod. 36, 238–243. doi: 10.1016/j.joen.2009.09.007
Wang, Y., Cox, M. K., Coricor, G., Macdougall, M., and Serra, R. (2013). Inactivation of Tgfbr2 in Osterix-Cre expressing dental mesenchyme disrupts molar root formation. Dev. Biol. 382, 27–37. doi: 10.1016/j.ydbio.2013.08.003
Wu, M., Chen, G., and Li, Y. P. (2016). TGF-beta and BMP signaling in osteoblast, skeletal development, and bone formation, homeostasis and disease. Bone Res. 4:16009.
Wu, X., Tu, X., Joeng, K. S., Hilton, M. J., Williams, D. A., and Long, F. (2008). Rac1 activation controls nuclear localization of beta-catenin during canonical Wnt signaling. Cell 133, 340–353. doi: 10.1016/j.cell.2008.01.052
Xiong, Y., Fang, Y., Qian, Y., Liu, Y., Yang, X., Huang, H., et al. (2019). Wnt production in dental epithelium is crucial for tooth differentiation. J. Dent. Res. 98, 580–588. doi: 10.1177/0022034519835194
Yamashiro, T., Zheng, L., Shitaku, Y., Saito, M., Tsubakimoto, T., Takada, K., et al. (2007). Wnt10a regulates dentin sialophosphoprotein mRNA expression and possibly links odontoblast differentiation and tooth morphogenesis. Differentiation 75, 452–462. doi: 10.1111/j.1432-0436.2006.00150.x
Yang, G., Zhou, J., Teng, Y., Xie, J., Lin, J., Guo, X., et al. (2014). Mesenchymal TGF-beta signaling orchestrates dental epithelial stem cell homeostasis through Wnt signaling. Stem Cells 32, 2939–2948. doi: 10.1002/stem.1772
Yu, Y., and Feng, X. H. (2019). TGF-beta signaling in cell fate control and cancer. Curr. Opin. Cell Biol. 61, 56–63. doi: 10.1016/j.ceb.2019.07.007
Zhang, H., Jiang, Y., Qin, C., Liu, Y., Ho, S. P., and Feng, J. Q. (2015). Essential role of osterix for tooth root but not crown dentin formation. J. Bone Miner. Res. 30, 742–746. doi: 10.1002/jbmr.2391
Zhang, R., Yang, G., Wu, X., Xie, J., Yang, X., and Li, T. (2013). Disruption of Wnt/beta-catenin signaling in odontoblasts and cementoblasts arrests tooth root development in postnatal mouse teeth. Int. J. Biol. Sci. 9, 228–236. doi: 10.7150/ijbs.5476
Zhou, C., Zhang, D., Zou, J., Li, X., Zou, S., and Xie, J. (2019). Substrate compliance directs the osteogenic lineages of stem cells from the human apical papilla via the processes of mechanosensing and mechanotransduction. ACS Appl. Mater. Interfaces 11, 26448–26459. doi: 10.1021/acsami.9b07147
Zhou, S., Eid, K., and Glowacki, J. (2004). Cooperation between TGF-beta and Wnt pathways during chondrocyte and adipocyte differentiation of human marrowhead stromal cells. J. Bone Miner. Res. 19, 463–470. doi: 10.1359/jbmr.0301239
Keywords: TGF-β type II receptor, Wntless, tooth root, odontoblast, dentin
Citation: Zhang R, Lin J, Liu Y, Yang S, He Q, Zhu L, Yang X and Yang G (2021) Transforming Growth Factor-β Signaling Regulates Tooth Root Dentinogenesis by Cooperation With Wnt Signaling. Front. Cell Dev. Biol. 9:687099. doi: 10.3389/fcell.2021.687099
Received: 29 March 2021; Accepted: 25 May 2021;
Published: 29 June 2021.
Edited by:
Songlin Wang, Capital Medical University, ChinaReviewed by:
Ujjal Bhawal, Nihon University School of Dentistry at Matsudo, JapanMotohiko Nagayama, Asahi University, Japan
Copyright © 2021 Zhang, Lin, Liu, Yang, He, Zhu, Yang and Yang. This is an open-access article distributed under the terms of the Creative Commons Attribution License (CC BY). The use, distribution or reproduction in other forums is permitted, provided the original author(s) and the copyright owner(s) are credited and that the original publication in this journal is cited, in accordance with accepted academic practice. No use, distribution or reproduction is permitted which does not comply with these terms.
*Correspondence: Guan Yang, yangguan@bmi.ac.cn; Xiao Yang, yangx@bmi.ac.cn