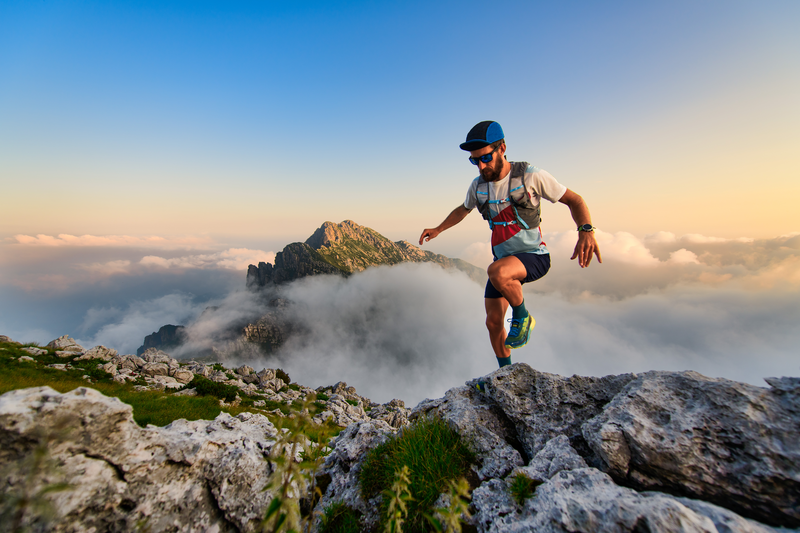
94% of researchers rate our articles as excellent or good
Learn more about the work of our research integrity team to safeguard the quality of each article we publish.
Find out more
REVIEW article
Front. Cell Dev. Biol. , 25 June 2021
Sec. Stem Cell Research
Volume 9 - 2021 | https://doi.org/10.3389/fcell.2021.681171
This article is part of the Research Topic Mesenchymal Stromal Cell Therapy for Regenerative Medicine View all 38 articles
Macrophages are involved in almost every aspect of biological systems and include development, homeostasis and repair. Mesenchymal stem cells (MSCs) have good clinical application prospects due to their ability to regulate adaptive and innate immune cells, particularly macrophages, and they have been used successfully for many immune disorders, including inflammatory bowel disease (IBD), acute lung injury, and wound healing, which have been reported as macrophage-mediated disorders. In the present review, we focus on the interaction between MSCs and macrophages and summarize their methods of interaction and communication, such as cell-to-cell contact, soluble factor secretion, and organelle transfer. In addition, we discuss the roles of MSC-macrophage crosstalk in the development of disease and maintenance of homeostasis of inflammatory microenvironments. Finally, we provide optimal strategies for applications in immune-related disease treatments.
Mesenchymal stem cells (MSCs) are observed in a variety of tissues, such as bone marrow, umbilical cord tissue, adipose tissue, and placental tissue (Pittenger et al., 1999), and they have a fibroblast-like morphology and multidirectional differentiation potential and can differentiate into cartilage, osteoblasts, and adipocytes (da Silva Meirelles et al., 2006). More importantly, MSCs can regulate the immune response and anti-inflammatory effects in a specific microenvironment by a number of pathways (da Silva Meirelles et al., 2009). Previous reports showed that MSCs regulate the immune response by interacting with various immune cells (Aggarwal et al., 2014; Caires et al., 2018) and the maturation, differentiation, proliferation and functional activation of peripheral blood mononuclear cells (PBMCs) by secreting regulatory molecules and cytokines (Di Nicola et al., 2002; da Silva Meirelles et al., 2006; Gazdic et al., 2015; Vizoso et al., 2017). Additionally, previous studies have shown that MSCs can regulate adaptive immune cells, such as modulating the proliferation and differentiation of B cells, thereby inhibiting T cell proliferation and cytokine secretion and inducing regulatory T cells (Wang R.X. et al., 2014). Moreover, MSCs also possess the potential to control the immune response from innate immune cells, including monocytes, macrophages, dendritic cells (DCs), natural killer cells (NK cells), etc. (Li et al., 2010; Chiesa et al., 2011; Cahill et al., 2015).
Macrophages are specialized phagocytic cells of the innate immune system that have several diverse functions in homeostatic and immune responses. As scavengers, macrophages constantly move around to remove dead cells, pathogenic microbes and other foreign bodies. As key modulator and effector cells in the immune response, the activation of macrophages influences and responds to the immune system. The concepts of classic and alternative activation, also known as M1 and M2, respectively, have become increasingly widespread (Gordon, 2003; Aggarwal et al., 2014), and growing evidence indicates that macrophages are pivotal for the maintenance of the tissue microenvironment and repair. For example, intestinal macrophages have roles in maintaining tissue homeostasis, particularly restoring tissue homeostasis after inflammation. Liposomes, lipoprotein A4 (LXA4) and specialized pre-degradation mediators (SPM) have been shown to direct macrophage differentiation to alternatively activated macrophages with pro-resolving capacity (Na et al., 2019). These macrophages not only inhibit Th1 and Th17 responses (Maizels, 2005) but also play an important role in re-establishing the epithelial barrier upon disruption of the intestinal epithelium (Fox et al., 2010). Similarly, alveolar macrophages are critical for tissue homeostasis, their polarization is driven by injury, and they regulate lung inflammation and resolution (Jackson et al., 2016; Hu and Christman, 2019). Moreover, macrophages constantly monitor the skin microenvironment and maintain skin homeostasis via their plasticity, such as differentiating into pro-inflammatory macrophages, pro-wound healing macrophages, or pro-resolving macrophages to promote or suppress inflammation and modulate wound healing (Barrientos et al., 2008; Mosser and Edwards, 2008; Yanez et al., 2017; Krzyszczyk et al., 2018). In recent years, increasing attention has been given to the study of MSC-macrophage interactions in tissue homeostasis and damage repair. This review will focus on the regulation of MSCs on macrophages, the counteraction of macrophages on MSCs, and the role of MSC-macrophage crosstalk in the inflammatory microenvironment. The importance of the interaction between the two is the maintenance of organization stability.
Generally, tissue-resident macrophages are either derived from circulating monocytes or established before birth and maintained long-term (Franken et al., 2016). As early as 1939, Ebert and Florey discovered that monocytes in vivo can migrate from the blood to different tissues and organs and then develop and differentiate into macrophages (Mφs) (Willis et al., 2018). The life span of Mφs in different tissues is prolonged, with some able to survive for months to years (Benoit et al., 2008a). Although Mφs have the potential for proliferation in tissues, they rarely divide and are mainly replenished by the continuous migration of monocytes in the blood. According to different activation methods, Mφ can be divided into two categories: classically activated Mφs (M1) and alternatively activated Mφs (M2). These two types have different surface receptor expression, cytokine and chemokine production, effector functions, etc. (Figure 1; Selleri et al., 2016; Heo et al., 2019).
Cytokines such as interferon gamma (IFN-γ), lipopolysaccharide (LPS), granulocyte-macrophage colony stimulating factor (GM-CSF) or tumor necrosis factor (TNF) can activate M1-type cells, which leads to increased self-antigen presentation ability, increased complement-mediated phagocytic activity, proinflammatory factor release (IL-1β, TNF-α, IL-12, IL-6, IL-23, NO, etc.), and chemokine production (CXCL9, CXCL10, etc.) (Benoit et al., 2008b; Biswas et al., 2019). By releasing these inflammatory mediators, M1 cells can promote the elimination of non-self components in vivo and play an important role in preventing tumors (Belgiovine et al., 2016). In addition, M1 cells participate in the Th1-mediated immune response as effector cells, thereby promoting inflammation and killing intracellular pathogens (Bystrom et al., 2008; Caires et al., 2016, 2018).
M2-type cells are further divided into M2a, M2b, and M2c cells (Mantovani et al., 2004). M2a can be induced by IL-13 or IL-4, and M2b can be induced by some Toll-like receptor (TLR) ligands and IL-10. M2a and M2b cells mainly perform immune regulation functions by promoting the Th2-mediated immune response (Ben-Mordechai et al., 2013; Ben-Lulu et al., 2014; Behura et al., 2019). The main function of M2c cells is to suppress the occurrence of the immune response, which plays an important role in the process of tissue remodeling (Mantovani et al., 2004).
Complementarily, the recognition and elimination of invading pathogens by macrophages is an important event in the innate immune response. Mφs express a variety of pattern recognition receptors (PRRs), including mannose receptors (MRs), scavenger receptors (SRs), TLRs and phosphatidylserine receptors (PSRs) (Kurokawa et al., 2009). Ligands are highly conserved molecular structures shared by a class or group of specific pathogenic microorganisms and their products, including LPS and lipoteichoic acid (LTA), mannose, peptidoglycan (PGN), bacterial DNA, double-stranded RNA and dextran, which are called pathogen-associated molecular patterns (PAMPs) (Li et al., 2016). When the corresponding PRR and PAMP are combined, a rapid immunobiological response can be mediated. In addition, after Mφ recognizes pathogenic microorganisms via PRR, it is activated at the same time and further produces and secretes a variety of chemokines, cytokines and chemical mediators, including MIP-1α/β, MCP-1, and IL-8, which mediate and promote inflammation. Moreover, Mφs can also secrete a large number of inflammatory mediators, such as leukotrienes, prostaglandins, elastase, lysozyme, and urokinase, to induce and strengthen local inflammation (Peng et al., 2016; Nenasheva et al., 2017). In short, macrophages play an important role in processing and presenting antigens, killing and eliminating intracellular pathogens, participating in antitumor immunity, and even maintaining tissues in environmental homeostasis (Xu et al., 2017; Willis et al., 2018).
Previous studies have shown that MSCs have the ability to regulate T cells, B cells, and DC cells (English et al., 2008; Ghannam et al., 2010; Luz-Crawford et al., 2013; Lee et al., 2014). At the same time, the interaction between MSCs and innate immune cells is becoming clearer. MSCs can regulate the polarization, phagocytosis and metabolism of macrophages. Biao Huang’s team found that in vitro, MSCs promoted the apoptosis of RAW264.7 cells, a macrophage cell line. They also reported that MSCs can downregulate the number of macrophages in the spleen (Huang et al., 2016). MSCs promote the phagocytosis of macrophages through mitochondrial transfer in distinct diseases, such as acute respiratory distress syndrome (ARDS) and sepsis (Jackson et al., 2016; Jackson and Krasnodembskaya, 2017), although fat-derived MSCs can also reduce the phagocytic capacity of macrophages (Adutler-Lieber et al., 2013). In addition, MSCs promote the differentiation of naive macrophages and enhance their bactericidal effect. These stem cells manipulate the plasticity of macrophages by changing the metabolic state of macrophages through prostaglandin E2 (PGE2) (Vasandan et al., 2016). Studies have shown that MSCs are likely to induce macrophages to transform into an anti-inflammatory/immunosuppressive phenotype (Luz-Crawford et al., 2017). Nakajima and HongLong Zhou reported that MSCs can effectively improve spinal cord injury by polarizing macrophages from the M1 to M2 type (Nakajima et al., 2012; Zhou et al., 2016). Other studies have also reported that MSCs can alleviate liver diseases, such as acute liver injury and liver fibrosis, by inhibiting proinflammatory M1 cells and inducing M2 anti-inflammatory cells (Li C. et al., 2019; Wang et al., 2021). Reports also show that MSCs can improve diabetes by inducing macrophage polarization (Yin et al., 2018; Gao et al., 2019). Liao et al. (2020) demonstrated that MSCs can induce macrophages toward an anti-inflammatory M2 phenotype in ischemic myocardium, and other investigations have illustrated that MSCs can polarize macrophages via direct cell–cell contact or indirectly, such as through soluble factors.
Mesenchymal stem cells have been reported to affect immune cells through direct cell-to-cell contact (Augello et al., 2005; Gu et al., 2013). Although most reports indicate that the immunomodulatory properties of MSCs depend largely on the secretion of soluble factors, cell-to-cell contact is also an important functional mechanism. Li Y. et al. (2019) found that the direct interaction between M1 macrophages and MSCs is essential for the treatment of LPS-induced abortion by MSCs. Using an abortion model, they demonstrated that MSCs induce immune tolerance based on a TSG-6-dependent paracrine effect and by cell-to-cell contact between MSCs and proinflammatory macrophages (Li Y. et al., 2019). Espagnolle reported that the inhibitory effect of MSCs on T cell proliferation was increased due to the upregulation of CD54 in hMSCs in contact with M1 macrophages (Espagnolle et al., 2017).
Mesenchymal stem cells can polarize monocytes or M1 macrophages into M2-type macrophages by secreting soluble factors. Previous studies have demonstrated that paracrine activity plays an important role in the anti-inflammatory effect of MSCs (da Silva Meirelles et al., 2009). We have summarized the main soluble factors by which MSCs regulate macrophage polarization.
Prostaglandin E2 (PGE2) is the main product of arachidonic acid metabolism in mammalian cells and plays an important role in immunosuppression and alleviating inflammation (Peebles, 2019). The Zongjin Li group found that human placenta-derived MSCs can improve 2,4,6-trinitrobenzene sulfonic acid (TNBS)-induced mouse colitis. This therapeutic potency was possibly mediated by MSC production of PGE2 to polarize M2 macrophages (Cao et al., 2020). Wang et al. (2021) reported that MSCs can protect against D-galactosamine (D-Gal)-induced liver failure, and they found that MSCs regulate macrophage polarization by secreting PGE2. In addition, studies have reported that MSCs improve cardiac injury by increasing M2 macrophages through the COX-2-PGE2 pathway (Jin et al., 2019, 2020). In summary, PGE2 is an important factor that mediates MSC polarization of macrophages.
Transforming growth factor β (TGF-β) plays a very important role in the immunosuppressive function of MSCs (Eggenhofer et al., 2014). TGF-β, a well-known immunosuppressive factor, can inhibit excessive inflammation (Robertson and Rifkin, 2013; Noh et al., 2016; de Araujo Farias et al., 2018). In addition, TGF-β induces M2 polarization of macrophages and reduces the inflammatory response mediated by macrophages (Li et al., 2006; Byrne et al., 2008; Robertson and Rifkin, 2013). Moreover, Pender et al. (1996) showed that TGF-β improves the survival rate of rats exposed to endotoxic shock by regulating the production of inflammatory mediators in peritoneal macrophages. Liu F. et al. (2019) showed that in a coculture system, TGF-β secreted by MSCs reduced the level of M1 markers, upregulated M2 macrophage marker levels, and inhibited excessive activation of the inflammatory response. Another group reported that TGF-β-licensed MSCs can improve the secretome of inflammatory bone marrow-derived macrophages (Lynch et al., 2020). Based on these findings, TGF-β secreted by MSCs can promote the M2-like polarization of macrophages and improve inflammatory conditions.
Indoleamine 2,3-dioxygenase (IDO), an intracellular enzyme, can be induced by interferon-γ (IFN-γ), and it catalyzes tryptophan into kynurenine (Ball et al., 2009). Metabolites of kynurenine have been shown to inhibit T cell proliferation and induce T cell apoptosis (Munn et al., 2005; Fallarino et al., 2006), and it has also been shown to regulate macrophage phenotype. Francois et al. (2012) and other investigators found that MSCs induce the polarization of monocytes toward M2 macropage and reduce monocytes infiltration dependent on IDO activity (Kang J.Y. et al., 2020; Galipeau, 2021; Lim et al., 2021). Lee et al. (2017) also found that melatonin can promote MSCs and improve blood flow perfusion and vessel regeneration by reducing the infiltration of macrophages in an IDO-dependent manner.
Tumor necrosis factor-α-induced gene/protein 6 (TSG6) has been reported to reduce inflammatory responses in corneal injury, lung injury, peritonitis and skin wounds (Danchuk et al., 2011; Qi et al., 2014; He et al., 2016). Studies on the effect of MSC-induced TSG-6 expression on macrophages have also been performed. Ko et al. (2016) reported that TSG-6 is required for MSCs to induce a suppressive monocyte/macrophage population. In a mouse colitis model, BM-MSCs had a therapeutic function induced by dextran sodium sulfate (DSS) depending on the production of TSG-6, which polarized macrophages from the M1 to M2 type (Sala et al., 2015). MSCs have also been shown to regulate microglia by secreting TSG-6. In this case, murine BM-MSCs inhibited the production of TNF-a, IL-1β, iNOS, and IL-6 by BV2 microglia stimulated by LPS in a TSG-6-dependent manner (Liu et al., 2014). In short, TSG-6 may play an important role in MSC function related to the programming of macrophages.
CCL2 is a chemokine classically associated with the recruitment of macrophages and monocytes during angiogenesis (Dipietro et al., 2001; Khan et al., 2013). MSCs secrete CCL2 at very low concentrations in the resting state (Kinnaird et al., 2004; Zhang et al., 2010). When stimulated with inflammatory cytokines, such as TNFα, MSCs secrete up to 10-fold more CCL2 (Ren et al., 2012). Using a lymphoma transplantation model, Ren et al. (2012) demonstrated that tumor-resident MSCs promote tumor growth by recruiting monocytes/macrophages through CCL2 production. C-X-C motif chemokine ligand 12 (CXCL12) plays a major role in macrophages located in regulatory niches (Sugiyama et al., 2006). Perivascular mesenchymal stem/stromal cells, endothelial cells, mature osteoblasts, and osteoprogenitors can also express CXCL12 (Asada et al., 2017). Papa et al. (2018) demonstrated that MSCs alleviate spinal cord injury through the recruitment of macrophages and conversion of M1 cells to an M2 neuroprotective phenotype by secreting CCL2. Recently, Giri et al. (2020) identified that MSCs can upregulate IL-10 expression in CCR2+ macrophages by secreting CCL2 and CXCL12, which cooperate as a heterodimer in vitro.
An increasing number of studies have shown that MSCs perform many paracrine functions by releasing extracellular vesicles (EVs). Several recently published studies indicated that MSC-based alleviation of colitis mainly relies on MSC-EV-induced suppression of colon macrophages (Wu et al., 2014; Phinney et al., 2015; Cao et al., 2019). Exosomes upregulate the nuclear factor kappaB (NF-κB) signal of macrophages, and the uptake of mitochondria increases their phagocytic capacity (Wu et al., 2014). Cao et al. (2019) showed that MSC-EVs can significantly reduce colitis caused by DSS in mice by inducing colonic macrophage polarization in the immunosuppressive M2 phenotype, and they observed more IL-10-producing M2 macrophages in MSC-EV-treated mice, which was associated with weight loss, intestinal epithelial cell damage and increased colon length. Yang et al. (2015) suggested that MSC-EV effects on the phenotype and function of macrophages occur by the regulating the damaged intestinal antioxidant/oxidant balance. That is, MSC-EV-mediated inhibition of intestinal NO-driven injury is accompanied by a decrease in the activities of myeloperoxidase and malondialdehyde and an increase in the activities of superoxide dismutase and glutathione.
Studies have also shown that other mechanisms may have a significant impact on the interaction between MSCs and macrophages. Phinney et al. (2015) described a peculiar process in which macrophages engulf the mitochondria that pass through mitochondrial MSCs and shuttle to the cell membrane. Jackson et al. (2016) also reported that in ARDS, the transfer of MSC mitochondria to macrophages in the lung occurs by tunneling through a nanotube-like structure and direct coculture of MSCs and monocyte-derived macrophages can enhance their swallowing ability (Jackson and Krasnodembskaya, 2017). Morrison et al. (2017) reported that the transfer of mitochondria via MSC-derived EVs promotes phagocytosis and suppresses proinflammatory cytokine secretion by human macrophages. It is worth noting that the above studies have shown that with the uptake of MSC mitochondria, the bioenergy and phagocytic capacity of macrophages are enhanced, which may help increase the clearance of microorganisms in diseases such as pneumonia and sepsis.
As mentioned above, MSCs can induce the transformation of macrophages. In addition, macrophages, as the target to be regulated, also have a feedback effect on MSCs that includes differentiation, migration, apoptosis and immunomodulatory functions (Figure 2).
Guihard et al. (2012) found that conditioned media from human monocytes stimulated with LPS or TLR ligands enhanced bone formation by human bone marrow MSCs. Enhanced osteogenesis was also observed by several groups, they demonstrated that M1 macrophages promote osteogenesis in MSCs via the COX-2-PGE2 pathway (Guihard et al., 2012; Lu et al., 2017; Zhang et al., 2017; Nathan et al., 2019; Tang et al., 2019). Recently, the Shi group reported that macrophage-derived supernatants can inhibit the adipogenic differentiation of human adipose-derived mesenchymal stem cells (hADSCs) in vitro, irrespective of the polarization status (M0, M1, or M2 macrophages) (Ma et al., 2020). In summary, macrophages also have an important influence on the differentiation ability of MSCs.
Studies have also clarified that macrophages affect the proliferation, migration, and survival of MSCs, with M2-type macrophages promoting MSC proliferation and migration and M1-type macrophages inducing MSC apoptosis (Yu et al., 2016; Xia Y. et al., 2020). Other reports also demonstrated that M2 macrophages can promote the growth of hMSCs, while M1 type inhibits the growth of hMSCs in vitro. In addition, macrophages can mediate the protective and repair effects of MSCs on infarction (Ben-Mordechai et al., 2013; Freytes et al., 2013; Maldonado-Lasuncion et al., 2021). These studies have shown that macrophages can affect MSCs, especially M2 type, and can promote MSCs proliferation and engraftment. This provides new support for targeted macrophage therapy in tissue repair.
In terms of immune regulation, the Nicolas team reported in detail that MSCs express more immune regulatory genes after coculture with macrophages (Lynch et al., 2020). The contact of MSCs with macrophages induces CD54 expression on MSCs and mediates calcium influx in MSCs, thereby promoting the immune regulation function of MSCs (Mercier et al., 1993). de Witte et al. (2018) demonstrated that the phagocytosis of MSCs by monocytes plays a crucial role in the immune regulation of MSCs. Moreover, Li Y. et al. (2019) found that the contact of MSCs with proinflammatory macrophages increases the production of TSG-6 by MSCs, thereby enhancing the inhibitory regulation of MSCs on T cells and macrophages. In addition, proinflammatory macrophages in contact with MSCs upregulated the expression of CD200 on MSCs and promoted the anti-inflammatory transformation of macrophages through the interaction of CD200 and CD200R. The TSG-6-mediated paracrine effect was enhanced by the cell-to-cell contact between MSCs and proinflammatory macrophages (Li Y. et al., 2019).
It is worth noting that macrophage depletion prevents the beneficial effects of MSC-Exos. Similarly, inducing macrophages to produce IL-10 is also partly involved in the beneficial effects of MSC-Exos (Liu H. et al., 2019). Krisztián Németh’s team stated that when mouse-derived bone marrow MSCs are cocultured with macrophages, MSCs will stimulate the release of IL-10 by macrophages through a PGE2-dependent pathway after LPS stimulation. However, in coculture systems, TNF-α and iNOS secreted by macrophages are necessary for MSCs to secrete PGE2 (Nemeth et al., 2009). Reading et al. (2015) suggested that proinflammatory cytokines produced by macrophages stimulate MSCs to produce prostaglandin E2 (PGE2) and interleukin (IL)-1RA, among other immune modulators.
The studies summarized above suggest that after being activated by proinflammatory factors, macrophages secrete cytokines to activate MSCs. After activation, MSCs regulate the immune response and react on macrophages. In the microenvironment of disease, a closed loop interaction occurs between macrophages and MSCs. In other words, MSCs and macrophages present a coordinated relationship to maintain the homeostasis of the inflammatory microenvironment.
Macrophages are pivotal for the maintenance of the tissue microenvironment and tissue repair (Ehninger and Trumpp, 2011; Liu et al., 2016). At present, the immunosuppressive function of MSCs derived from bone marrow and cord blood makes them useful in clinical trials and as a treatment method for diseases, including GVHD (Le Blanc and Davies, 2015; Granot et al., 2020), Crohn’s disease (Bamias and Cominelli, 2006), stroke, systemic lupus erythematosus and arthritis (Choi et al., 2012; Steiman et al., 2015) and other diseases. MSCs can improve different diseases by educating macrophages to maintain microenvironment homeostasis (Table 1).
Human gingiva-derived MSCs can enhance cutaneous wound healing by polarizing M2 macrophages (Vasandan et al., 2016; Zhao et al., 2016; Xu et al., 2017). In addition, melatonin (MT)-pretreated MSC-derived exosomes (MSC-Exos) can exert better effects on diabetic wound healing (Liu et al., 2020). MSC-Exos significantly inhibit macrophage expression of the proinflammatory factors IL-1β, TNF-α, and iNOS and simultaneously upregulate the anti-inflammatory factor IL-10, thereby increasing the polarization ratio of M1 to M2 to mediate the therapeutic effect (Philipp et al., 2018; Saldana et al., 2019). In colitis, MSCs are reported to regulate the polarization of M1 to M2 cells in a CCL2-dependent manner by upregulating the expression of IL-10 to alleviate intestinal inflammation (Giri et al., 2020). Liu H. et al. (2019) indicated that the systemic administration of exosomes from human bone marrow-derived mesenchymal stromal cells (BM-MSC-Exos) can effectively alleviate inflammatory bowel disease by interacting with colonic macrophages. Previous studies indicated that IL-1β derived from macrophages may stimulate MSCs to secrete IL-1RA and PGE2, which leads to the polarization of M2 macrophages, thereby forming a feedback pathway that enables MSC therapy improvement in hepatic injury (Bartosh et al., 2013; Lee et al., 2015; Reading et al., 2015; Luz-Crawford et al., 2016). Ko et al. (2020) reported that macrophage-derived amphiregulin is essential for MSC-mediated CD4+ T cell suppression and preserves epithelial stem cells to alleviate autoimmune uveoretinitis. More importantly, MSCs alleviate inflammatory injury depending on the presence of macrophages in the body, and pharmacological depletion of macrophages weakens the therapeutic effect of MSCs (Ko et al., 2016; Giri et al., 2020).
Mesenchymal stem cells and macrophages are pivotal for the repair and maintenance of the bone marrow microenvironment. Macrophages can be reprogrammed by MSCs to an arginine 1-positive phenotype and have tissue repair functions, and these functions are extremely important in the leukemia microenvironment. The reprogramming of macrophages by MSCs changes the bone marrow microenvironment and inhibits the development of leukemia (Xia C. et al., 2020). In the leukemia microenvironment, leukemia cell exosomes change the immune characteristics of MSCs and macrophages (Jafarzadeh et al., 2019; Nwabo Kamdje et al., 2020). In addition, MSCs can reprogram macrophages into M2 macrophages with phagocytosis functions and can reduce the secretion of proinflammatory factors to control the tumor microenvironmental immunity (Wolfe et al., 2016; Francois et al., 2019; Wang et al., 2019).
It is worth noting that MSCs and macrophages are also extremely important in bone repair, where macrophages regulate the osteogenic differentiation of adipose tissue MSCs and their transition from M1 to anti-inflammatory M2 is beneficial to the proliferation and osteogenic differentiation of MSCs (Zhang et al., 2017). Moreover, macrophages can promote the osteogenic differentiation of MSCs by secreting extracellular vesicles to promote bone regeneration (Kang M. et al., 2020). Gong et al. (2016) also found that macrophage polarization plays an extremely important role in regulating MSC osteogenic differentiation to maintain bone homeostasis and bone regeneration. In addition, the MSC-derived extracellular matrix alleviates macrophage inflammation to promote bone regeneration (Deng et al., 2020). Other studies have shown that for certain materials, the interaction between MSCs and macrophages includes the MSC-induced transformation macrophage phenotypes and macrophage-induced promotion of MSC differentiation; therefore, the physiological functions of MSCs and macrophages are regulated to improve osseointegration and promote bone repair (He et al., 2019; Mahon et al., 2020).
Dichloromethane (DCM) rats have abnormal lipid metabolism and cardiac inflammation. MSCs improve metabolic abnormalities and downregulate blood glucose levels by increasing M2-phenotype macrophages to alleviate cardiac inflammation in DCM rats (Sun et al., 2018; Jin et al., 2019). Yan Liao’s laboratory also reported that MSCs have a promising therapeutic effect on myocardial infarction by promoting the polarization of M2 macrophages by secreting periostin (Liao et al., 2020).
In summary, in various diseases, MSCs interact with macrophages to adjust the inflammatory microenvironment and thus affect the occurrence and development of the disease.
Mesenchymal stem cells are an emerging treatment for inflammatory and degenerative diseases, and they are entering early phase clinical trials. MSCs can regulate the biological function of innate and adapted immune cells. Macrophages are important innate immune cells that are found in nearly all inflammatory tissues, where they play a key role in maintaining normal tissue homeostasis. Evidence was provided showing that MSCs regulate the chemotaxis and function of macrophages and MSC-derived signals can contribute to disease remission by modulating macrophage function in certain cases (Figure 2). However, the exact role of MSCs in regulating macrophages remains to be determined.
The effects of MSCs on macrophages include inducing the transition from the proinflammatory M1 phenotype to the anti-inflammatory M2 phenotype, which reduces the secretion of proinflammatory factors and increases the secretion of anti-inflammatory factors. Moreover, MSCs could reduce the recruitment of proinflammatory macrophages to the site of inflammation. Furthermore, MSCs reciprocally regulate macrophage function. Studies investigating how macrophages influence the function of MSCs have gradually increased. Macrophages have a feedback effect on MSCs that includes differentiation, migration, apoptosis, and immunomodulatory functions. The synergistic effect of macrophages and MSCs maintains microenvironmental homeostasis.
At present, certain problems remain in the research on MSC and macrophage interactions. Soluble factors secreted by MSCs have been proven to have different effects on macrophages. The biological functions mediated by MSCs on macrophages vary with different inflammatory conditions. Further studies are needed to discover reliable markers for defining different subpopulations of macrophages, clarify the heterogeneity among different subpopulations of macrophages used for specific treatments and clarify the potential mechanisms by which MSCs regulate macrophages. Based on the current literature, the following two strategies to enhance MSC efficacy by targeting macrophage-MSC crosstalk can be outlined: (1) modulating the number of macrophages at the inflammatory site and (2) modulating the injection methods and dosages of MSCs in inflammatory diseases.
Research on the immunosuppressive effects of MSCs is underway, but additional research is required to clarify the interaction between MSCs and macrophages and identify the mechanism by which MSCs regulate macrophages to provide better solutions for the treatment of disease. In clinical applications that combine MSCs with M2 macrophages for the treatment of immune diseases, the stability and flexibility of the treatments should be closely considered and optimized to achieve the appropriate modulation of inflammatory responses at different stages of disease progression.
DL and YX searched the literature and wrote the manuscript. QL and QZ critically revised the manuscript and final approval of the work. All authors contributed to the article and approved the submitted version.
This work was supported by the National Key Research and Development Program of China (2017YFA0106100), National Science and Technology Major Project (2018ZX10723203), National Natural Science Foundation of China (81971526 and 81970537), and Guangdong Basic and Applied Basic Research Foundation (2020A1515010272 and 2020A151501138).
The authors declare that the research was conducted in the absence of any commercial or financial relationships that could be construed as a potential conflict of interest.
Adutler-Lieber, S., Ben-Mordechai, T., Naftali-Shani, N., Asher, E., Loberman, D., Raanani, E., et al. (2013). Human macrophage regulation via interaction with cardiac adipose tissue-derived mesenchymal stromal cells. J. Cardiovasc. Pharmacol. Ther. 18, 78–86. doi: 10.1177/1074248412453875
Aggarwal, N. R., King, L. S., and D’alessio, F. R. (2014). Diverse macrophage populations mediate acute lung inflammation and resolution. Am. J. Physiol. Lung. Cell. Mol. Physiol. 306, L709–L725. doi: 10.1152/ajplung.00341.2013
Asada, N., Kunisaki, Y., Pierce, H., Wang, Z., Fernandez, N. F., Birbrair, A., et al. (2017). Differential cytokine contributions of perivascular haematopoietic stem cell niches. Nat. Cell Biol. 19, 214–223. doi: 10.1038/ncb3475
Augello, A., Tasso, R., Negrini, S. M., Amateis, A., Indiveri, F., Cancedda, R., et al. (2005). Bone marrow mesenchymal progenitor cells inhibit lymphocyte proliferation by activation of the programmed death 1 pathway. Eur. J. Immunol. 35, 1482–1490. doi: 10.1002/eji.200425405
Ball, H. J., Yuasa, H. J., Austin, C. J., Weiser, S., and Hunt, N. H. (2009). Indoleamine 2,3-dioxygenase-2; a new enzyme in the kynurenine pathway. Int. J. Biochem. Cell Biol. 41, 467–471. doi: 10.1016/j.biocel.2008.01.005
Bamias, G., and Cominelli, F. (2006). Novel strategies to attenuate immune activation in Crohn’s disease. Curr. Opin. Pharmacol. 6, 401–407. doi: 10.1016/j.coph.2006.03.008
Barrientos, S., Stojadinovic, O., Golinko, M. S., Brem, H., and Tomic-Canic, M. (2008). Growth factors and cytokines in wound healing. Wound Repair Regen. 16, 585–601. doi: 10.1111/j.1524-475X.2008.00410.x
Bartosh, T. J., Ylostalo, J. H., Bazhanov, N., Kuhlman, J., and Prockop, D. J. (2013). Dynamic compaction of human mesenchymal stem/precursor cells into spheres self-activates caspase-dependent IL1 signaling to enhance secretion of modulators of inflammation and immunity (PGE2, TSG6, and STC1). Stem Cells 31, 2443–2456. doi: 10.1002/stem.1499
Behura, S. K., Kelleher, A. M., and Spencer, T. E. (2019). Evidence for functional interactions between the placenta and brain in pregnant mice. FASEB J. 33, 4261–4272. doi: 10.1096/fj.201802037R
Belgiovine, C., D’incalci, M., Allavena, P., and Frapolli, R. (2016). Tumor-associated macrophages and anti-tumor therapies: complex links. Cell Mol. Life Sci. 73, 2411–2424. doi: 10.1007/s00018-016-2166-5
Ben-Lulu, S., Ziv, T., Admon, A., Weisman-Shomer, P., and Benhar, M. (2014). A substrate trapping approach identifies proteins regulated by reversible S-nitrosylation. Mol. Cell Proteomics 13, 2573–2583. doi: 10.1074/mcp.M114.038166
Ben-Mordechai, T., Holbova, R., Landa-Rouben, N., Harel-Adar, T., Feinberg, M. S., Abd Elrahman, I., et al. (2013). Macrophage subpopulations are essential for infarct repair with and without stem cell therapy. J. Am. Coll. Cardiol. 62, 1890–1901. doi: 10.1016/j.jacc.2013.07.057
Benoit, M., Desnues, B., and Mege, J. L. (2008a). Macrophage polarization in bacterial infections. J. Immunol. 181, 3733–3739. doi: 10.4049/jimmunol.181.6.3733
Benoit, M., Ghigo, E., Capo, C., Raoult, D., and Mege, J. L. (2008b). The uptake of apoptotic cells drives Coxiella burnetii replication and macrophage polarization: a model for Q fever endocarditis. PLoS Pathog. 4:e1000066. doi: 10.1371/journal.ppat.1000066
Biswas, S., Mandal, G., Roy Chowdhury, S., Purohit, S., Payne, K. K., Anadon, C., et al. (2019). Exosomes produced by mesenchymal stem cells drive differentiation of myeloid cells into immunosuppressive M2-polarized macrophages in breast cancer. J. Immunol. 203, 3447–3460. doi: 10.4049/jimmunol.1900692
Byrne, S. N., Knox, M. C., and Halliday, G. M. (2008). TGFbeta is responsible for skin tumour infiltration by macrophages enabling the tumours to escape immune destruction. Immunol. Cell Biol. 86, 92–97. doi: 10.1038/sj.icb.7100116
Bystrom, J., Evans, I., Newson, J., Stables, M., Toor, I., Van Rooijen, N., et al. (2008). Resolution-phase macrophages possess a unique inflammatory phenotype that is controlled by cAMP. Blood 112, 4117–4127. doi: 10.1182/blood-2007-12-129767
Cahill, E. F., Tobin, L. M., Carty, F., Mahon, B. P., and English, K. (2015). Jagged-1 is required for the expansion of CD4+ CD25+ FoxP3+ regulatory T cells and tolerogenic dendritic cells by murine mesenchymal stromal cells. Stem Cell Res. Ther. 6:19. doi: 10.1186/s13287-015-0021-5
Caires, H. R., Barros Da Silva, P., Barbosa, M. A., and Almeida, C. R. (2018). A co-culture system with three different primary human cell populations reveals that biomaterials and MSC modulate macrophage-driven fibroblast recruitment. J. Tissue Eng. Regen. Med. 12, e1433–e1440. doi: 10.1002/term.2560
Caires, H. R., Esteves, T., Quelhas, P., Barbosa, M. A., Navarro, M., and Almeida, C. R. (2016). Macrophage interactions with polylactic acid and chitosan scaffolds lead to improved recruitment of human mesenchymal stem/stromal cells: a comprehensive study with different immune cells. J. R. Soc. Interface 13:20160570. doi: 10.1098/rsif.2016.0570
Cao, L., Xu, H., Wang, G., Liu, M., Tian, D., and Yuan, Z. (2019). Extracellular vesicles derived from bone marrow mesenchymal stem cells attenuate dextran sodium sulfate-induced ulcerative colitis by promoting M2 macrophage polarization. Int. Immunopharmacol. 72, 264–274. doi: 10.1016/j.intimp.2019.04.020
Cao, X., Duan, L., Hou, H., Liu, Y., Chen, S., Zhang, S., et al. (2020). IGF-1C hydrogel improves the therapeutic effects of MSCs on colitis in mice through PGE2-mediated M2 macrophage polarization. Theranostics 10, 7697–7709. doi: 10.7150/thno.45434
Chen, X., Yang, B., Tian, J., Hong, H., Du, Y., Li, K., et al. (2018). Dental follicle stem cells ameliorate lipopolysaccharide-induced inflammation by secreting TGF-beta3 and TSP-1 to elicit macrophage M2 polarization. Cell Physiol. Biochem. 51, 2290–2308. doi: 10.1159/000495873
Chiesa, S., Morbelli, S., Morando, S., Massollo, M., Marini, C., Bertoni, A., et al. (2011). Mesenchymal stem cells impair in vivo T-cell priming by dendritic cells. Proc. Natl. Acad. Sci. U. S. A. 108, 17384–17389. doi: 10.1073/pnas.1103650108
Choi, E. W., Shin, I. S., Park, S. Y., Park, J. H., Kim, J. S., Yoon, E. J., et al. (2012). Reversal of serologic, immunologic, and histologic dysfunction in mice with systemic lupus erythematosus by long-term serial adipose tissue-derived mesenchymal stem cell transplantation. Arthritis Rheum. 64, 243–253. doi: 10.1002/art.33313
da Silva Meirelles, L., Chagastelles, P. C., and Nardi, N. B. (2006). Mesenchymal stem cells reside in virtually all post-natal organs and tissues. J. Cell Sci. 119, 2204–2213. doi: 10.1242/jcs.02932
da Silva Meirelles, L., Fontes, A. M., Covas, D. T., and Caplan, A. I. (2009). Mechanisms involved in the therapeutic properties of mesenchymal stem cells. Cytokine Growth Factor Rev. 20, 419–427. doi: 10.1016/j.cytogfr.2009.10.002
Danchuk, S., Ylostalo, J. H., Hossain, F., Sorge, R., Ramsey, A., Bonvillain, R. W., et al. (2011). Human multipotent stromal cells attenuate lipopolysaccharide-induced acute lung injury in mice via secretion of tumor necrosis factor-alpha-induced protein 6. Stem Cell Res. Ther. 2:27. doi: 10.1186/scrt68
de Araujo Farias, V., Carrillo-Galvez, A. B., Martin, F., and Anderson, P. (2018). TGF-beta and mesenchymal stromal cells in regenerative medicine, autoimmunity and cancer. Cytokine Growth Factor Rev. 43, 25–37. doi: 10.1016/j.cytogfr.2018.06.002
de Mendonca, L., Felix, N. S., Blanco, N. G., Da Silva, J. S., Ferreira, T. P., Abreu, S. C., et al. (2017). Mesenchymal stromal cell therapy reduces lung inflammation and vascular remodeling and improves hemodynamics in experimental pulmonary arterial hypertension. Stem Cell Res. Ther. 8:220. doi: 10.1186/s13287-017-0669-0
de Witte, S. F. H., Luk, F., Sierra Parraga, J. M., Gargesha, M., Merino, A., Korevaar, S. S., et al. (2018). Immunomodulation by therapeutic mesenchymal stromal cells (MSC) is triggered through phagocytosis of MSC by monocytic cells. Stem Cells 36, 602–615. doi: 10.1002/stem.2779
Deng, M., Tan, J., Hu, C., Hou, T., Peng, W., Liu, J., et al. (2020). Modification of PLGA Scaffold by MSC-derived extracellular matrix combats macrophage inflammation to initiate bone regeneration via TGF-beta-induced protein. Adv. Healthc. Mater. 9:e2000353. doi: 10.1002/adhm.202000353
Di Nicola, M., Carlo-Stella, C., Magni, M., Milanesi, M., Longoni, P. D., Matteucci, P., et al. (2002). Human bone marrow stromal cells suppress T-lymphocyte proliferation induced by cellular or nonspecific mitogenic stimuli. Blood 99, 3838–3843. doi: 10.1182/blood.v99.10.3838
Dipietro, L. A., Reintjes, M. G., Low, Q. E., Levi, B., and Gamelli, R. L. (2001). Modulation of macrophage recruitment into wounds by monocyte chemoattractant protein-1. Wound Repair Regen. 9, 28–33. doi: 10.1046/j.1524-475x.2001.00028.x
Eggenhofer, E., Luk, F., Dahlke, M. H., and Hoogduijn, M. J. (2014). The life and fate of mesenchymal stem cells. Front. Immunol. 5:148. doi: 10.3389/fimmu.2014.00148
Ehninger, A., and Trumpp, A. (2011). The bone marrow stem cell niche grows up: mesenchymal stem cells and macrophages move in. J. Exp. Med. 208, 421–428. doi: 10.1084/jem.20110132
Elchaninov, A., Fatkhudinov, T., Usman, N., Arutyunyan, I., Makarov, A., Lokhonina, A., et al. (2018). Multipotent stromal cells stimulate liver regeneration by influencing the macrophage polarization in rat. World J. Hepatol. 10, 287–296. doi: 10.4254/wjh.v10.i2.287
English, K., Barry, F. P., and Mahon, B. P. (2008). Murine mesenchymal stem cells suppress dendritic cell migration, maturation and antigen presentation. Immunol. Lett. 115, 50–58. doi: 10.1016/j.imlet.2007.10.002
Espagnolle, N., Balguerie, A., Arnaud, E., Sensebe, L., and Varin, A. (2017). CD54-Mediated interaction with pro-inflammatory macrophages increases the immunosuppressive function of human mesenchymal stromal cells. Stem Cell Rep. 8, 961–976. doi: 10.1016/j.stemcr.2017.02.008
Fallarino, F., Grohmann, U., You, S., Mcgrath, B. C., Cavener, D. R., Vacca, C., et al. (2006). The combined effects of tryptophan starvation and tryptophan catabolites down-regulate T cell receptor zeta-chain and induce a regulatory phenotype in naive T cells. J. Immunol. 176, 6752–6761. doi: 10.4049/jimmunol.176.11.6752
Fox, S., Leitch, A. E., Duffin, R., Haslett, C., and Rossi, A. G. (2010). Neutrophil apoptosis: relevance to the innate immune response and inflammatory disease. J. Innate Immun. 2, 216–227. doi: 10.1159/000284367
Francois, M., Romieu-Mourez, R., Li, M., and Galipeau, J. (2012). Human MSC suppression correlates with cytokine induction of indoleamine 2,3-dioxygenase and bystander M2 macrophage differentiation. Mol. Ther. 20, 187–195. doi: 10.1038/mt.2011.189
Francois, S., Usunier, B., Forgue-Lafitte, M. E., L’homme, B., Benderitter, M., Douay, L., et al. (2019). Mesenchymal stem cell administration attenuates colon cancer progression by modulating the immune component within the colorectal tumor microenvironment. Stem Cells Transl. Med. 8, 285–300. doi: 10.1002/sctm.18-0117
Franken, L., Schiwon, M., and Kurts, C. (2016). Macrophages: sentinels and regulators of the immune system. Cell Microbiol. 18, 475–487. doi: 10.1111/cmi.12580
Freytes, D. O., Kang, J. W., Marcos-Campos, I., and Vunjak-Novakovic, G. (2013). Macrophages modulate the viability and growth of human mesenchymal stem cells. J. Cell Biochem. 114, 220–229. doi: 10.1002/jcb.24357
Galipeau, J. (2021). Macrophages at the nexus of mesenchymal stromal cell potency: the emerging role of chemokine cooperativity. Stem Cells. doi: 10.1002/stem.3380 [Epub ahead of print].
Gao, C., Wang, X., Lu, J., Li, Z., Jia, H., Chen, M., et al. (2020). Mesenchymal stem cells transfected with sFgl2 inhibit the acute rejection of heart transplantation in mice by regulating macrophage activation. Stem Cell Res. Ther. 11:241. doi: 10.1186/s13287-020-01752-1
Gao, J., Cheng, Y., Hao, H., Yin, Y., Xue, J., Zhang, Q., et al. (2019). Decitabine assists umbilical cord-derived mesenchymal stem cells in improving glucose homeostasis by modulating macrophage polarization in type 2 diabetic mice. Stem Cell Res. Ther. 10:259. doi: 10.1186/s13287-019-1338-2
Gazdic, M., Simovic Markovic, B., Jovicic, N., Misirkic-Marjanovic, M., Djonov, V., Jakovljevic, V., et al. (2017). Mesenchymal stem cells promote metastasis of lung cancer cells by downregulating systemic antitumor immune response. Stem Cells Int. 2017:6294717. doi: 10.1155/2017/6294717
Gazdic, M., Volarevic, V., Arsenijevic, N., and Stojkovic, M. (2015). Mesenchymal stem cells: a friend or foe in immune-mediated diseases. Stem Cell Rev. Rep. 11, 280–287. doi: 10.1007/s12015-014-9583-3
Ghannam, S., Pene, J., Moquet-Torcy, G., Jorgensen, C., and Yssel, H. (2010). Mesenchymal stem cells inhibit human Th17 cell differentiation and function and induce a T regulatory cell phenotype. J. Immunol. 185, 302–312. doi: 10.4049/jimmunol.0902007
Giri, J., Das, R., Nylen, E., Chinnadurai, R., and Galipeau, J. (2020). CCL2 and CXCL12 derived from mesenchymal stromal cells cooperatively polarize IL-10+ tissue macrophages to mitigate gut injury. Cell Rep. 30, 1923–1934.e4. doi: 10.1016/j.celrep.2020.01.047
Gong, L., Zhao, Y., Zhang, Y., and Ruan, Z. (2016). The macrophage polarization regulates MSC osteoblast differentiation in vitro. Ann. Clin. Lab. Sci. 46, 65–71.
Gordon, S. (2003). Alternative activation of macrophages. Nat. Rev. Immunol. 3, 23–35. doi: 10.1038/nri978
Granot, N., Rezvani, A. R., Pender, B. S., Storer, B. E., Sandmaier, B. M., Storb, R., et al. (2020). Impact of rituximab and host/donor Fc receptor polymorphisms after allogeneic hematopoietic cell transplantation for CD20(+) B cell malignancies. Biol. Blood Marrow Transplant. 26, 1811–1818. doi: 10.1016/j.bbmt.2020.07.014
Gu, Y. Z., Xue, Q., Chen, Y. J., Yu, G. H., Qing, M. D., Shen, Y., et al. (2013). Different roles of PD-L1 and FasL in immunomodulation mediated by human placenta-derived mesenchymal stem cells. Hum. Immunol. 74, 267–276. doi: 10.1016/j.humimm.2012.12.011
Guihard, P., Danger, Y., Brounais, B., David, E., Brion, R., Delecrin, J., et al. (2012). Induction of osteogenesis in mesenchymal stem cells by activated monocytes/macrophages depends on oncostatin M signaling. Stem Cells 30, 762–772. doi: 10.1002/stem.1040
He, Y., Yang, X., Yuan, Z., Shen, X., Xu, K., Lin, C., et al. (2019). Regulation of MSC and macrophage functions in bone healing by peptide LL-37-loaded silk fibroin nanoparticles on a titanium surface. Biomater. Sci. 7, 5492–5505. doi: 10.1039/c9bm01158g
He, Z., Hua, J., Qian, D., Gong, J., Lin, S., Xu, C., et al. (2016). Intravenous hMSCs ameliorate acute pancreatitis in mice via secretion of tumor necrosis factor-alpha stimulated gene/protein 6. Sci. Rep. 6:38438. doi: 10.1038/srep38438
Heo, J. S., Choi, Y., and Kim, H. O. (2019). Adipose-derived mesenchymal stem cells promote M2 macrophage phenotype through exosomes. Stem Cells Int. 2019:7921760. doi: 10.1155/2019/7921760
Hu, G., and Christman, J. W. (2019). Editorial: alveolar macrophages in lung inflammation and resolution. Front. Immunol. 10:2275. doi: 10.3389/fimmu.2019.02275
Huang, B., Cheng, X., Wang, H., Huang, W., La Ga Hu, Z., Wang, D., et al. (2016). Mesenchymal stem cells and their secreted molecules predominantly ameliorate fulminant hepatic failure and chronic liver fibrosis in mice respectively. J. Transl. Med. 14:45. doi: 10.1186/s12967-016-0792-1
Huang, R., Qin, C., Wang, J., Hu, Y., Zheng, G., Qiu, G., et al. (2019). Differential effects of extracellular vesicles from aging and young mesenchymal stem cells in acute lung injury. Aging (Albany NY) 11, 7996–8014. doi: 10.18632/aging.102314
Hwang, Y., Kim, J. C., and Tae, G. (2019). Significantly enhanced recovery of acute liver failure by liver targeted delivery of stem cells via heparin functionalization. Biomaterials 209, 67–78. doi: 10.1016/j.biomaterials.2019.04.019
Jackson, M. V., and Krasnodembskaya, A. D. (2017). Analysis of mitochondrial transfer in direct co-cultures of human monocyte-derived macrophages (MDM) and mesenchymal stem cells (MSC). Bio. Protoc. 7:e2255. doi: 10.21769/BioProtoc.2255
Jackson, M. V., Morrison, T. J., Doherty, D. F., Mcauley, D. F., Matthay, M. A., Kissenpfennig, A., et al. (2016). Mitochondrial transfer via tunneling nanotubes is an important mechanism by which mesenchymal stem cells enhance macrophage phagocytosis in the in vitro and in vivo models of ARDS. Stem Cells 34, 2210–2223. doi: 10.1002/stem.2372
Jafarzadeh, N., Safari, Z., Pornour, M., Amirizadeh, N., Forouzandeh Moghadam, M., and Sadeghizadeh, M. (2019). Alteration of cellular and immune-related properties of bone marrow mesenchymal stem cells and macrophages by K562 chronic myeloid leukemia cell derived exosomes. J. Cell Physiol. 234, 3697–3710. doi: 10.1002/jcp.27142
Jerkic, M., Masterson, C., Ormesher, L., Gagnon, S., Goyal, S., Rabani, R., et al. (2019). Overexpression of IL-10 enhances the efficacy of human umbilical-cord-derived mesenchymal stromal cells in E. coli pneumosepsis. J. Clin. Med. 8:847. doi: 10.3390/jcm8060847
Jin, L., Deng, Z., Zhang, J., Yang, C., Liu, J., Han, W., et al. (2019). Mesenchymal stem cells promote type 2 macrophage polarization to ameliorate the myocardial injury caused by diabetic cardiomyopathy. J. Transl. Med. 17:251. doi: 10.1186/s12967-019-1999-8
Jin, L., Zhang, J., Deng, Z., Liu, J., Han, W., Chen, G., et al. (2020). Mesenchymal stem cells ameliorate myocardial fibrosis in diabetic cardiomyopathy via the secretion of prostaglandin E2. Stem Cell Res. Ther. 11:122. doi: 10.1186/s13287-020-01633-7
Kang, J. Y., Oh, M. K., Joo, H., Park, H. S., Chae, D. H., Kim, J., et al. (2020). Xeno-free condition enhances therapeutic functions of human wharton’s jelly-derived mesenchymal stem cells against experimental colitis by upregulated indoleamine 2,3-dioxygenase activity. J. Clin. Med. 9:2913. doi: 10.3390/jcm9092913
Kang, M., Huang, C. C., Lu, Y., Shirazi, S., Gajendrareddy, P., Ravindran, S., et al. (2020). Bone regeneration is mediated by macrophage extracellular vesicles. Bone 141:115627. doi: 10.1016/j.bone.2020.115627
Kawata, Y., Tsuchiya, A., Seino, S., Watanabe, Y., Kojima, Y., Ikarashi, S., et al. (2019). Early injection of human adipose tissue-derived mesenchymal stem cell after inflammation ameliorates dextran sulfate sodium-induced colitis in mice through the induction of M2 macrophages and regulatory T cells. Cell Tissue Res. 376, 257–271. doi: 10.1007/s00441-018-02981-w
Khan, B., Rangasamy, S., Mcguire, P. G., and Howdieshell, T. R. (2013). The role of monocyte subsets in myocutaneous revascularization. J. Surg. Res. 183, 963–975. doi: 10.1016/j.jss.2013.02.019
Kinnaird, T., Stabile, E., Burnett, M. S., Shou, M., Lee, C. W., Barr, S., et al. (2004). Local delivery of marrow-derived stromal cells augments collateral perfusion through paracrine mechanisms. Circulation 109, 1543–1549. doi: 10.1161/01.CIR.0000124062.31102.57
Ko, J. H., Kim, H. J., Jeong, H. J., Lee, H. J., and Oh, J. Y. (2020). Mesenchymal stem and stromal cells harness macrophage-derived amphiregulin to maintain tissue homeostasis. Cell Rep. 30, 3806–3820.e6. doi: 10.1016/j.celrep.2020.02.062
Ko, J. H., Lee, H. J., Jeong, H. J., Kim, M. K., Wee, W. R., Yoon, S. O., et al. (2016). Mesenchymal stem/stromal cells precondition lung monocytes/macrophages to produce tolerance against allo- and autoimmunity in the eye. Proc. Natl. Acad. Sci. U. S. A. 113, 158–163. doi: 10.1073/pnas.1522905113
Kojima, Y., Tsuchiya, A., Ogawa, M., Nojiri, S., Takeuchi, S., Watanabe, T., et al. (2019). Mesenchymal stem cells cultured under hypoxic conditions had a greater therapeutic effect on mice with liver cirrhosis compared to those cultured under normal oxygen conditions. Regen. Ther. 11, 269–281. doi: 10.1016/j.reth.2019.08.005
Krzyszczyk, P., Schloss, R., Palmer, A., and Berthiaume, F. (2018). The role of macrophages in acute and chronic wound healing and interventions to promote pro-wound healing phenotypes. Front. Physiol. 9:419. doi: 10.3389/fphys.2018.00419
Kurokawa, I., Danby, F. W., Ju, Q., Wang, X., Xiang, L. F., Xia, L., et al. (2009). New developments in our understanding of acne pathogenesis and treatment. Exp. Dermatol. 18, 821–832. doi: 10.1111/j.1600-0625.2009.00890.x
Le Blanc, K., and Davies, L. C. (2015). Mesenchymal stromal cells and the innate immune response. Immunol. Lett. 168, 140–146. doi: 10.1016/j.imlet.2015.05.004
Lee, D. S., Yi, T. G., Lee, H. J., Kim, S. N., Park, S., Jeon, M. S., et al. (2014). Mesenchymal stem cells infected with Mycoplasma arginini secrete complement C3 to regulate immunoglobulin production in B lymphocytes. Cell Death Dis. 5:e1192. doi: 10.1038/cddis.2014.147
Lee, J. H., Han, Y. S., and Lee, S. H. (2017). Potentiation of biological effects of mesenchymal stem cells in ischemic conditions by melatonin via upregulation of cellular prion protein expression. J. Pineal. Res. 62:e12385. doi: 10.1111/jpi.12385
Lee, K. C., Lin, H. C., Huang, Y. H., and Hung, S. C. (2015). Allo-transplantation of mesenchymal stem cells attenuates hepatic injury through IL1Ra dependent macrophage switch in a mouse model of liver disease. J. Hepatol. 63, 1405–1412. doi: 10.1016/j.jhep.2015.07.035
Li, C., Jin, Y., Wei, S., Sun, Y., Jiang, L., Zhu, Q., et al. (2019). Hippo signaling controls NLR family pyrin domain containing 3 activation and governs immunoregulation of mesenchymal stem cells in mouse liver injury. Hepatology 70, 1714–1731. doi: 10.1002/hep.30700
Li, F. R., Wang, X. G., Deng, C. Y., Qi, H., Ren, L. L., and Zhou, H. X. (2010). Immune modulation of co-transplantation mesenchymal stem cells with islet on T and dendritic cells. Clin. Exp. Immunol. 161, 357–363. doi: 10.1111/j.1365-2249.2010.04178.x
Li, M. O., Wan, Y. Y., Sanjabi, S., Robertson, A. K., and Flavell, R. A. (2006). Transforming growth factor-beta regulation of immune responses. Annu. Rev. Immunol. 24, 99–146. doi: 10.1146/annurev.immunol.24.021605.090737
Li, Q., Sun, W., Wang, X., Zhang, K., Xi, W., and Gao, P. (2015). Skin-derived mesenchymal stem cells alleviate atherosclerosis via modulating macrophage function. Stem Cells Transl. Med. 4, 1294–1301. doi: 10.5966/sctm.2015-0020
Li, X., Liu, L., Yang, J., Yu, Y., Chai, J., Wang, L., et al. (2016). Exosome derived from human umbilical cord mesenchymal stem cell mediates MiR-181c attenuating burn-induced excessive inflammation. EBioMedicine 8, 72–82. doi: 10.1016/j.ebiom.2016.04.030
Li, Y., Sheng, Q., Zhang, C., Han, C., Bai, H., Lai, P., et al. (2021). STAT6 up-regulation amplifies M2 macrophage anti-inflammatory capacity through mesenchymal stem cells. Int. Immunopharmacol. 91:107266. doi: 10.1016/j.intimp.2020.107266
Li, Y., Zhang, D., Xu, L., Dong, L., Zheng, J., Lin, Y., et al. (2019). Cell-cell contact with proinflammatory macrophages enhances the immunotherapeutic effect of mesenchymal stem cells in two abortion models. Cell Mol. Immunol. 16, 908–920. doi: 10.1038/s41423-019-0204-6
Li, Y. W., Zhang, C., Sheng, Q. J., Bai, H., Ding, Y., and Dou, X. G. (2017). Mesenchymal stem cells rescue acute hepatic failure by polarizing M2 macrophages. World J. Gastroenterol. 23, 7978–7988. doi: 10.3748/wjg.v23.i45.7978
Liang, X., Li, T., Zhou, Q., Pi, S., Li, Y., Chen, X., et al. (2019). Mesenchymal stem cells attenuate sepsis-induced liver injury via inhibiting M1 polarization of Kupffer cells. Mol. Cell Biochem. 452, 187–197. doi: 10.1007/s11010-018-3424-7
Liao, Y., Li, G., Zhang, X., Huang, W., Xie, D., Dai, G., et al. (2020). Cardiac Nestin(+) mesenchymal stromal cells enhance healing of ischemic heart through periostin-mediated M2 macrophage polarization. Mol. Ther. 28, 855–873. doi: 10.1016/j.ymthe.2020.01.011
Lim, J. Y., Kim, B. S., Ryu, D. B., Kim, T. W., Park, G., and Min, C. K. (2021). The therapeutic efficacy of mesenchymal stromal cells on experimental colitis was improved by the IFN-gamma and poly(I:C) priming through promoting the expression of indoleamine 2,3-dioxygenase. Stem Cell Res. Ther. 12:37. doi: 10.1186/s13287-020-02087-7
Liu, C., Wu, C., Yang, Q., Gao, J., Li, L., Yang, D., et al. (2016). Macrophages mediate the repair of brain vascular rupture through direct physical adhesion and mechanical traction. Immunity 44, 1162–1176. doi: 10.1016/j.immuni.2016.03.008
Liu, F., Qiu, H., Xue, M., Zhang, S., Zhang, X., Xu, J., et al. (2019). MSC-secreted TGF-beta regulates lipopolysaccharide-stimulated macrophage M2-like polarization via the Akt/FoxO1 pathway. Stem Cell Res. Ther. 10:345. doi: 10.1186/s13287-019-1447-y
Liu, H., Liang, Z., Wang, F., Zhou, C., Zheng, X., Hu, T., et al. (2019). Exosomes from mesenchymal stromal cells reduce murine colonic inflammation via a macrophage-dependent mechanism. JCI Insight 4:e131273. doi: 10.1172/jci.insight.131273
Liu, W., Yu, M., Xie, D., Wang, L., Ye, C., Zhu, Q., et al. (2020). Melatonin-stimulated MSC-derived exosomes improve diabetic wound healing through regulating macrophage M1 and M2 polarization by targeting the PTEN/AKT pathway. Stem Cell Res. Ther. 11:259. doi: 10.1186/s13287-020-01756-x
Liu, Y., Lou, G., Li, A., Zhang, T., Qi, J., Ye, D., et al. (2018). AMSC-derived exosomes alleviate lipopolysaccharide/d-galactosamine-induced acute liver failure by miR-17-mediated reduction of TXNIP/NLRP3 inflammasome activation in macrophages. EBioMedicine 36, 140–150. doi: 10.1016/j.ebiom.2018.08.054
Liu, Y., Zhang, R., Yan, K., Chen, F. F., Huang, W. Y., Lv, B. K., et al. (2014). Mesenchymal stem cells inhibit lipopolysaccharide-induced inflammatory responses of BV2 microglial cells through TSG-6. J. Neuroinflamm. 11:Artn135. doi: 10.1186/1742-2094-11-135
Lu, L. Y., Loi, F., Nathan, K., Lin, T. H., Pajarinen, J., Gibon, E., et al. (2017). Pro-inflammatory M1 macrophages promote Osteogenesis by mesenchymal stem cells via the COX-2-prostaglandin E2 pathway. J. Orthop. Res. 35, 2378–2385. doi: 10.1002/jor.23553
Luo, X. Y., Meng, X. J., Cao, D. C., Wang, W., Zhou, K., Li, L., et al. (2019). Transplantation of bone marrow mesenchymal stromal cells attenuates liver fibrosis in mice by regulating macrophage subtypes. Stem Cell Res. Ther. 10:16. doi: 10.1186/s13287-018-1122-8
Luz-Crawford, P., Djouad, F., Toupet, K., Bony, C., Franquesa, M., Hoogduijn, M. J., et al. (2016). Mesenchymal stem cell-derived interleukin 1 receptor antagonist promotes macrophage polarization and inhibits B cell differentiation. Stem Cells 34, 483–492. doi: 10.1002/stem.2254
Luz-Crawford, P., Jorgensen, C., and Djouad, F. (2017). Mesenchymal stem cells direct the immunological fate of macrophages. Results Probl. Cell Differ. 62, 61–72. doi: 10.1007/978-3-319-54090-0_4
Luz-Crawford, P., Kurte, M., Bravo-Alegria, J., Contreras, R., Nova-Lamperti, E., Tejedor, G., et al. (2013). Mesenchymal stem cells generate a CD4+CD25+Foxp3+ regulatory T cell population during the differentiation process of Th1 and Th17 cells. Stem Cell Res. Ther. 4:65. doi: 10.1186/scrt216
Lv, H., Liu, Q., Sun, Y., Yi, X., Wei, X., Liu, W., et al. (2020). Mesenchymal stromal cells ameliorate acute lung injury induced by LPS mainly through stanniocalcin-2 mediating macrophage polarization. Ann. Transl. Med. 8:334. doi: 10.21037/atm.2020.02.105
Lynch, K., Treacy, O., Chen, X., Murphy, N., Lohan, P., Islam, M. N., et al. (2020). TGF-beta1-licensed murine MSCs show superior therapeutic efficacy in modulating corneal allograft immune rejection in vivo. Mol. Ther. 28, 2023–2043. doi: 10.1016/j.ymthe.2020.05.023
Ma, H., Li, Y. N., Song, L., Liu, R., Li, X., Shang, Q., et al. (2020). Macrophages inhibit adipogenic differentiation of adipose tissue derived mesenchymal stem/stromal cells by producing pro-inflammatory cytokines. Cell Biosci. 10:88. doi: 10.1186/s13578-020-00450-y
Mahon, O. R., Browe, D. C., Gonzalez-Fernandez, T., Pitacco, P., Whelan, I. T., Von Euw, S., et al. (2020). Nano-particle mediated M2 macrophage polarization enhances bone formation and MSC osteogenesis in an IL-10 dependent manner. Biomaterials 239:119833. doi: 10.1016/j.biomaterials.2020.119833
Maizels, R. M. (2005). Infections and allergy – helminths, hygiene and host immune regulation. Curr. Opin. Immunol. 17, 656–661. doi: 10.1016/j.coi.2005.09.001
Maldonado-Lasuncion, I., O’neill, N., Umland, O., Verhaagen, J., and Oudega, M. (2021). Macrophage-derived inflammation induces a transcriptome makeover in mesenchymal stromal cells enhancing their potential for tissue repair. Int. J. Mol. Sci. 22:781. doi: 10.3390/ijms22020781
Mantovani, A., Sica, A., Sozzani, S., Allavena, P., Vecchi, A., and Locati, M. (2004). The chemokine system in diverse forms of macrophage activation and polarization. Trends Immunol. 25, 677–686. doi: 10.1016/j.it.2004.09.015
Mao, F., Wu, Y., Tang, X., Wang, J., Pan, Z., Zhang, P., et al. (2017). Human umbilical cord mesenchymal stem cells alleviate inflammatory bowel disease through the regulation of 15-LOX-1 in macrophages. Biotechnol. Lett. 39, 929–938. doi: 10.1007/s10529-017-2315-4
Mercier, B., Granier, P., Mercier, J., Trouquet, J., and Prefaut, C. (1993). Anaerobic and aerobic components during arm-crank exercise in sprint and middle-distance swimmers. Eur. J. Appl. Physiol. Occup. Physiol. 66, 461–466. doi: 10.1007/BF00599622
Morrison, T. J., Jackson, M. V., Cunningham, E. K., Kissenpfennig, A., Mcauley, D. F., O’kane, C. M., et al. (2017). Mesenchymal stromal cells modulate macrophages in clinically relevant lung injury models by extracellular vesicle mitochondrial transfer. Am. J. Respir. Crit. Care Med. 196, 1275–1286. doi: 10.1164/rccm.201701-0170OC
Mosser, D. M., and Edwards, J. P. (2008). Exploring the full spectrum of macrophage activation. Nat. Rev. Immunol. 8, 958–969. doi: 10.1038/nri2448
Munn, D. H., Sharma, M. D., Baban, B., Harding, H. P., Zhang, Y., Ron, D., et al. (2005). GCN2 kinase in T cells mediates proliferative arrest and anergy induction in response to indoleamine 2,3-dioxygenase. Immunity 22, 633–642. doi: 10.1016/j.immuni.2005.03.013
Na, Y. R., Stakenborg, M., Seok, S. H., and Matteoli, G. (2019). Macrophages in intestinal inflammation and resolution: a potential therapeutic target in IBD. Nat. Rev. Gastroenterol. Hepatol. 16, 531–543. doi: 10.1038/s41575-019-0172-4
Nakajima, H., Uchida, K., Guerrero, A. R., Watanabe, S., Sugita, D., Takeura, N., et al. (2012). Transplantation of mesenchymal stem cells promotes an alternative pathway of macrophage activation and functional recovery after spinal cord injury. J. Neurotrauma 29, 1614–1625. doi: 10.1089/neu.2011.2109
Nathan, K., Lu, L. Y., Lin, T., Pajarinen, J., Jamsen, E., Huang, J. F., et al. (2019). Precise immunomodulation of the M1 to M2 macrophage transition enhances mesenchymal stem cell osteogenesis and differs by sex. Bone Joint Res. 8, 481–488. doi: 10.1302/2046-3758.810.BJR-2018-0231.R2
Nemeth, K., Leelahavanichkul, A., Yuen, P. S., Mayer, B., Parmelee, A., Doi, K., et al. (2009). Bone marrow stromal cells attenuate sepsis via prostaglandin E(2)-dependent reprogramming of host macrophages to increase their interleukin-10 production. Nat. Med. 15, 42–49. doi: 10.1038/nm.1905
Nenasheva, T., Nikolaev, A., Diykanov, D., Sukhanova, A., Tcyganov, E., Panteleev, A., et al. (2017). The introduction of mesenchymal stromal cells induces different immunological responses in the lungs of healthy and M. tuberculosis infected mice. PLoS One 12:e0178983. doi: 10.1371/journal.pone.0178983
Noh, M. Y., Lim, S. M., Oh, K. W., Cho, K. A., Park, J., Kim, K. S., et al. (2016). Mesenchymal stem cells modulate the functional properties of microglia via TGF-beta secretion. Stem Cells Transl. Med. 5, 1538–1549. doi: 10.5966/sctm.2015-0217
Nwabo Kamdje, A. H., Seke Etet, P. F., Simo Tagne, R., Vecchio, L., Lukong, K. E., and Krampera, M. (2020). Tumor microenvironment uses a reversible reprogramming of mesenchymal stromal cells to mediate pro-tumorigenic effects. Front. Cell Dev. Biol. 8:545126. doi: 10.3389/fcell.2020.545126
Ohara, M., Ohnishi, S., Hosono, H., Yamamoto, K., Yuyama, K., Nakamura, H., et al. (2018). Extracellular vesicles from amnion-derived mesenchymal stem cells ameliorate hepatic inflammation and fibrosis in rats. Stem Cells Int. 2018:3212643. doi: 10.1155/2018/3212643
Papa, S., Vismara, I., Mariani, A., Barilani, M., Rimondo, S., De Paola, M., et al. (2018). Mesenchymal stem cells encapsulated into biomimetic hydrogel scaffold gradually release CCL2 chemokine in situ preserving cytoarchitecture and promoting functional recovery in spinal cord injury. J. Control Release 278, 49–56. doi: 10.1016/j.jconrel.2018.03.034
Park, J., Kim, S., Lim, H., Liu, A., Hu, S., Lee, J., et al. (2019). Therapeutic effects of human mesenchymal stem cell microvesicles in an ex vivo perfused human lung injured with severe E. coli pneumonia. Thorax 74, 43–50. doi: 10.1136/thoraxjnl-2018-211576
Peebles, R. S. Jr. (2019). Prostaglandins in asthma and allergic diseases. Pharmacol. Ther. 193, 1–19. doi: 10.1016/j.pharmthera.2018.08.001
Pender, B. S., Chen, H., Ashton, S., Wise, W. C., Zingarelli, B., Cusumano, V., et al. (1996). Transforming growth factor beta 1 alters rat peritoneal macrophage mediator production and improves survival during endotoxic shock. Eur. Cytokine Netw. 7, 137–142.
Peng, Y., Pan, W., Ou, Y., Xu, W., Kaelber, S., Borlongan, C. V., et al. (2016). Extracardiac-lodged mesenchymal stromal cells propel an inflammatory response against myocardial infarction via paracrine effects. Cell Transplant. 25, 929–935. doi: 10.3727/096368915X689758
Philipp, D., Suhr, L., Wahlers, T., Choi, Y. H., and Paunel-Gorgulu, A. (2018). Preconditioning of bone marrow-derived mesenchymal stem cells highly strengthens their potential to promote IL-6-dependent M2b polarization. Stem Cell Res. Ther. 9:286. doi: 10.1186/s13287-018-1039-2
Phinney, D. G., Di Giuseppe, M., Njah, J., Sala, E., Shiva, S., St Croix, C. M., et al. (2015). Mesenchymal stem cells use extracellular vesicles to outsource mitophagy and shuttle microRNAs. Nat. Commun. 6:8472. doi: 10.1038/ncomms9472
Pittenger, M. F., Mackay, A. M., Beck, S. C., Jaiswal, R. K., Douglas, R., Mosca, J. D., et al. (1999). Multilineage potential of adult human mesenchymal stem cells. Science 284, 143–147. doi: 10.1126/science.284.5411.143
Porzionato, A., Zaramella, P., Dedja, A., Guidolin, D., Bonadies, L., Macchi, V., et al. (2021). Intratracheal administration of mesenchymal stem cell-derived extracellular vesicles reduces lung injuries in a chronic rat model of bronchopulmonary dysplasia. Am. J. Physiol. Lung Cell. Mol. Physiol. 320, L688–L704. doi: 10.1152/ajplung.00148.2020
Qi, Y., Jiang, D., Sindrilaru, A., Stegemann, A., Schatz, S., Treiber, N., et al. (2014). TSG-6 released from intradermally injected mesenchymal stem cells accelerates wound healing and reduces tissue fibrosis in murine full-thickness skin wounds. J. Invest. Dermatol. 134, 526–537. doi: 10.1038/jid.2013.328
Reading, J. L., Vaes, B., Hull, C., Sabbah, S., Hayday, T., Wang, N. S., et al. (2015). Suppression of IL-7-dependent effector T-cell expansion by multipotent adult progenitor cells and PGE2. Mol. Ther. 23, 1783–1793. doi: 10.1038/mt.2015.131
Ren, G., Zhao, X., Wang, Y., Zhang, X., Chen, X., Xu, C., et al. (2012). CCR2-dependent recruitment of macrophages by tumor-educated mesenchymal stromal cells promotes tumor development and is mimicked by TNFalpha. Cell Stem Cell 11, 812–824. doi: 10.1016/j.stem.2012.08.013
Ren, W., Hou, J., Yang, C., Wang, H., Wu, S., Wu, Y., et al. (2019). Extracellular vesicles secreted by hypoxia pre-challenged mesenchymal stem cells promote non-small cell lung cancer cell growth and mobility as well as macrophage M2 polarization via miR-21-5p delivery. J. Exp. Clin. Cancer Res. 38:62. doi: 10.1186/s13046-019-1027-0
Robertson, I. B., and Rifkin, D. B. (2013). Unchaining the beast; insights from structural and evolutionary studies on TGFbeta secretion, sequestration, and activation. Cytokine Growth Factor Rev. 24, 355–372. doi: 10.1016/j.cytogfr.2013.06.003
Sala, E., Genua, M., Petti, L., Anselmo, A., Arena, V., Cibella, J., et al. (2015). Mesenchymal stem cells reduce colitis in mice via release of TSG6, independently of their localization to the intestine. Gastroenterology 149, 163–176.e20. doi: 10.1053/j.gastro.2015.03.013
Saldana, L., Bensiamar, F., Valles, G., Mancebo, F. J., Garcia-Rey, E., and Vilaboa, N. (2019). Immunoregulatory potential of mesenchymal stem cells following activation by macrophage-derived soluble factors. Stem Cell Res. Ther. 10:58. doi: 10.1186/s13287-019-1156-6
Selleri, S., Bifsha, P., Civini, S., Pacelli, C., Dieng, M. M., Lemieux, W., et al. (2016). Human mesenchymal stromal cell-secreted lactate induces M2-macrophage differentiation by metabolic reprogramming. Oncotarget 7, 30193–30210. doi: 10.18632/oncotarget.8623
Shao, M., Xu, Q., Wu, Z., Chen, Y., Shu, Y., Cao, X., et al. (2020). Exosomes derived from human umbilical cord mesenchymal stem cells ameliorate IL-6-induced acute liver injury through miR-455-3p. Stem Cell Res. Ther. 11:37. doi: 10.1186/s13287-020-1550-0
Sheng, M., Lin, Y., Xu, D., Tian, Y., Zhan, Y., Li, C., et al. (2021). CD47-mediated Hedgehog/SMO/GLI1 signaling promotes mesenchymal stem cell immunomodulation in mouse liver inflammation. Hepatology doi: 10.1002/hep.31831 [Epub ahead of print].
Song, J. Y., Kang, H. J., Hong, J. S., Kim, C. J., Shim, J. Y., Lee, C. W., et al. (2017). Umbilical cord-derived mesenchymal stem cell extracts reduce colitis in mice by re-polarizing intestinal macrophages. Sci. Rep. 7:9412. doi: 10.1038/s41598-017-09827-5
Song, N., Scholtemeijer, M., and Shah, K. (2020). Mesenchymal stem cell immunomodulation: mechanisms and therapeutic potential. Trends Pharmacol. Sci. 41, 653–664. doi: 10.1016/j.tips.2020.06.009
Song, W. J., Li, Q., Ryu, M. O., Ahn, J. O., Bhang, D. H., Jung, Y. C., et al. (2018). TSG-6 released from intraperitoneally injected canine adipose tissue-derived mesenchymal stem cells ameliorate inflammatory bowel disease by inducing M2 macrophage switch in mice. Stem Cell Res. Ther. 9:91. doi: 10.1186/s13287-018-0841-1
Song, W. J., Li, Q., Ryu, M. O., Ahn, J. O., Ha Bhang, D., Chan Jung, Y., et al. (2017). TSG-6 secreted by human adipose tissue-derived mesenchymal stem cells ameliorates DSS-induced colitis by inducing M2 macrophage polarization in mice. Sci. Rep. 7:5187. doi: 10.1038/s41598-017-04766-7
Steiman, A. J., Gladman, D. D., Ibanez, D., Noamani, B., Landolt-Marticorena, C., Urowitz, M. B., et al. (2015). Lack of interferon and proinflammatory cyto/chemokines in serologically active clinically quiescent systemic lupus erythematosus. J. Rheumatol. 42, 2318–2326. doi: 10.3899/jrheum.150040
Sugiyama, T., Kohara, H., Noda, M., and Nagasawa, T. (2006). Maintenance of the hematopoietic stem cell pool by CXCL12-CXCR4 chemokine signaling in bone marrow stromal cell niches. Immunity 25, 977–988. doi: 10.1016/j.immuni.2006.10.016
Sun, X., Shan, A., Wei, Z., and Xu, B. (2018). Intravenous mesenchymal stem cell-derived exosomes ameliorate myocardial inflammation in the dilated cardiomyopathy. Biochem. Biophys. Res. Commun. 503, 2611–2618. doi: 10.1016/j.bbrc.2018.08.012
Tang, H., Husch, J. F. A., Zhang, Y., Jansen, J. A., Yang, F., and Van Den Beucken, J. (2019). Coculture with monocytes/macrophages modulates osteogenic differentiation of adipose-derived mesenchymal stromal cells on poly(lactic-co-glycolic) acid/polycaprolactone scaffolds. J. Tissue Eng. Regen. Med. 13, 785–798. doi: 10.1002/term.2826
Ti, D., Hao, H., Tong, C., Liu, J., Dong, L., Zheng, J., et al. (2015). LPS-preconditioned mesenchymal stromal cells modify macrophage polarization for resolution of chronic inflammation via exosome-shuttled let-7b. J. Transl. Med. 13:308. doi: 10.1186/s12967-015-0642-6
Vasandan, A. B., Jahnavi, S., Shashank, C., Prasad, P., Kumar, A., and Prasanna, S. J. (2016). Human mesenchymal stem cells program macrophage plasticity by altering their metabolic status via a PGE2-dependent mechanism. Sci. Rep. 6:38308. doi: 10.1038/srep38308
Vizoso, F. J., Eiro, N., Cid, S., Schneider, J., and Perez-Fernandez, R. (2017). Mesenchymal stem cell secretome: toward cell-free therapeutic strategies in regenerative medicine. Int. J. Mol. Sci. 18:1852. doi: 10.3390/ijms18091852
Wang, C., Chen, J., Sun, L., and Liu, Y. (2014). TGF-beta signaling-dependent alleviation of dextran sulfate sodium-induced colitis by mesenchymal stem cell transplantation. Mol. Biol. Rep. 41, 4977–4983. doi: 10.1007/s11033-014-3364-6
Wang, J., Liu, Y., Ding, H., Shi, X., and Ren, H. (2021). Mesenchymal stem cell-secreted prostaglandin E2 ameliorates acute liver failure via attenuation of cell death and regulation of macrophage polarization. Stem Cell Res. Ther. 12:15. doi: 10.1186/s13287-020-02070-2
Wang, R. X., Yu, C. R., Dambuza, I. M., Mahdi, R. M., Dolinska, M. B., Sergeev, Y. V., et al. (2014). Interleukin-35 induces regulatory B cells that suppress autoimmune disease. Nat. Med. 20, 633–641. doi: 10.1038/nm.3554
Wang, S., Su, X., Xu, M., Xiao, X., Li, X., Li, H., et al. (2019). Exosomes secreted by mesenchymal stromal/stem cell-derived adipocytes promote breast cancer cell growth via activation of Hippo signaling pathway. Stem Cell Res. Ther. 10:117. doi: 10.1186/s13287-019-1220-2
Willis, G. R., Fernandez-Gonzalez, A., Reis, M., Mitsialis, S. A., and Kourembanas, S. (2018). Macrophage immunomodulation: the gatekeeper for mesenchymal stem cell derived-exosomes in pulmonary arterial hypertension? Int. J. Mol. Sci. 19:2534. doi: 10.3390/ijms19092534
Wolfe, A. R., Trenton, N. J., Debeb, B. G., Larson, R., Ruffell, B., Chu, K., et al. (2016). Mesenchymal stem cells and macrophages interact through IL-6 to promote inflammatory breast cancer in pre-clinical models. Oncotarget 7, 82482–82492. doi: 10.18632/oncotarget.12694
Wu, X. F., Ouyang, Z. J., Feng, L. L., Chen, G., Guo, W. J., Shen, Y., et al. (2014). Suppression of NF-kappaB signaling and NLRP3 inflammasome activation in macrophages is responsible for the amelioration of experimental murine colitis by the natural compound fraxinellone. Toxicol. Appl. Pharmacol. 281, 146–156. doi: 10.1016/j.taap.2014.10.002
Xia, C., Wang, T., Cheng, H., Dong, Y., Weng, Q., Sun, G., et al. (2020). Mesenchymal stem cells suppress leukemia via macrophage-mediated functional restoration of bone marrow microenvironment. Leukemia 34, 2375–2383. doi: 10.1038/s41375-020-0775-3
Xia, Y., He, X. T., Xu, X. Y., Tian, B. M., An, Y., and Chen, F. M. (2020). Exosomes derived from M0, M1 and M2 macrophages exert distinct influences on the proliferation and differentiation of mesenchymal stem cells. PeerJ 8:e8970. doi: 10.7717/peerj.8970
Xu, C., Fu, F., Li, X., and Zhang, S. (2017). Mesenchymal stem cells maintain the microenvironment of central nervous system by regulating the polarization of macrophages/microglia after traumatic brain injury. Int. J. Neurosci. 127, 1124–1135. doi: 10.1080/00207454.2017.1325884
Xu, R., Zhang, F., Chai, R., Zhou, W., Hu, M., Liu, B., et al. (2019). Exosomes derived from pro-inflammatory bone marrow-derived mesenchymal stem cells reduce inflammation and myocardial injury via mediating macrophage polarization. J. Cell. Mol. Med. 23, 7617–7631. doi: 10.1111/jcmm.14635
Yanez, D. A., Lacher, R. K., Vidyarthi, A., and Colegio, O. R. (2017). The role of macrophages in skin homeostasis. Pflugers Arch. 469, 455–463. doi: 10.1007/s00424-017-1953-7
Yang, J., Liu, X. X., Fan, H., Tang, Q., Shou, Z. X., Zuo, D. M., et al. (2015). Extracellular vesicles derived from bone marrow mesenchymal stem cells protect against experimental colitis via attenuating colon inflammation, oxidative stress and apoptosis. PLoS One 10:e0140551. doi: 10.1371/journal.pone.0140551
Yin, Y., Hao, H., Cheng, Y., Gao, J., Liu, J., Xie, Z., et al. (2018). The homing of human umbilical cord-derived mesenchymal stem cells and the subsequent modulation of macrophage polarization in type 2 diabetic mice. Int. Immunopharmacol. 60, 235–245. doi: 10.1016/j.intimp.2018.04.051
Yu, B., Sondag, G. R., Malcuit, C., Kim, M. H., and Safadi, F. F. (2016). Macrophage-associated osteoactivin/GPNMB mediates mesenchymal stem cell survival, proliferation, and migration via a CD44-dependent mechanism. J. Cell Biochem. 117, 1511–1521. doi: 10.1002/jcb.25394
Zhang, Q. Z., Su, W. R., Shi, S. H., Wilder-Smith, P., Xiang, A. P., Wong, A., et al. (2010). Human gingiva-derived mesenchymal stem cells elicit polarization of m2 macrophages and enhance cutaneous wound healing. Stem Cells 28, 1856–1868. doi: 10.1002/stem.503
Zhang, Y., Bose, T., Unger, R. E., Jansen, J. A., Kirkpatrick, C. J., and Van Den Beucken, J. (2017). Macrophage type modulates osteogenic differentiation of adipose tissue MSCs. Cell Tissue Res. 369, 273–286. doi: 10.1007/s00441-017-2598-8
Zhao, G., Miao, H., Li, X., Chen, S., Hu, Y., Wang, Z., et al. (2016). TGF-beta3-induced miR-494 inhibits macrophage polarization via suppressing PGE2 secretion in mesenchymal stem cells. FEBS Lett. 590, 1602–1613. doi: 10.1002/1873-3468.12200
Keywords: mesenchymal stem cells, macrophage, clinic therapy, microenvironment, homeostasis
Citation: Lu D, Xu Y, Liu Q and Zhang Q (2021) Mesenchymal Stem Cell-Macrophage Crosstalk and Maintenance of Inflammatory Microenvironment Homeostasis. Front. Cell Dev. Biol. 9:681171. doi: 10.3389/fcell.2021.681171
Received: 16 March 2021; Accepted: 28 May 2021;
Published: 25 June 2021.
Edited by:
Guido Moll, Charité – Universitätsmedizin Berlin, GermanyReviewed by:
Bofeng Li, University of Science and Technology of China, ChinaCopyright © 2021 Lu, Xu, Liu and Zhang. This is an open-access article distributed under the terms of the Creative Commons Attribution License (CC BY). The use, distribution or reproduction in other forums is permitted, provided the original author(s) and the copyright owner(s) are credited and that the original publication in this journal is cited, in accordance with accepted academic practice. No use, distribution or reproduction is permitted which does not comply with these terms.
*Correspondence: Qiuli Liu, bGl1cWxpM0BtYWlsLnN5c3UuZWR1LmNu; Qi Zhang, emhhbmdxMjdAbWFpbC5zeXN1LmVkdS5jbg==
Disclaimer: All claims expressed in this article are solely those of the authors and do not necessarily represent those of their affiliated organizations, or those of the publisher, the editors and the reviewers. Any product that may be evaluated in this article or claim that may be made by its manufacturer is not guaranteed or endorsed by the publisher.
Research integrity at Frontiers
Learn more about the work of our research integrity team to safeguard the quality of each article we publish.