- Department of Biology and Chemistry, College of Liberal Arts and Sciences, National University of Defense Technology, Changsha, China
Long non-coding RNAs (lncRNAs) regulate gene expression in a variety of ways at epigenetic, chromatin remodeling, transcriptional, and translational levels. Accumulating evidence suggests that lncRNA X-inactive specific transcript (lncRNA Xist) serves as an important regulator of cell growth and development. Despites its original roles in X-chromosome dosage compensation, lncRNA Xist also participates in the development of tumor and other human diseases by functioning as a competing endogenous RNA (ceRNA). In this review, we comprehensively summarized recent progress in understanding the cellular functions of lncRNA Xist in mammalian cells and discussed current knowledge regarding the ceRNA network of lncRNA Xist in various diseases. Long non-coding RNAs (lncRNAs) are transcripts that are more than 200 nt in length and without an apparent protein-coding capacity (Furlan and Rougeulle, 2016; Maduro et al., 2016). These RNAs are believed to be transcribed by the approximately 98–99% non-coding regions of the human genome (Derrien et al., 2012; Fu, 2014; Montalbano et al., 2017; Slack and Chinnaiyan, 2019), as well as a large variety of genomic regions, such as exonic, tronic, and intergenic regions. Hence, lncRNAs are also divided into eight categories: Intergenic lncRNAs, Intronic lncRNAs, Enhancer lncRNAs, Promoter lncRNAs, Natural antisense/sense lncRNAs, Small nucleolar RNA-ended lncRNAs (sno-lncRNAs), Bidirectional lncRNAs, and non-poly(A) lncRNAs (Ma et al., 2013; Devaux et al., 2015; St Laurent et al., 2015; Chen, 2016; Quinn and Chang, 2016; Richard and Eichhorn, 2018; Connerty et al., 2020). A range of evidence has suggested that lncRNAs function as key regulators in crucial cellular functions, including proliferation, differentiation, apoptosis, migration, and invasion, by regulating the expression level of target genes via epigenomic, transcriptional, or post-transcriptional approaches (Cao et al., 2018). Moreover, lncRNAs detected in body fluids were also believed to serve as potential biomarkers for the diagnosis, prognosis, and monitoring of disease progression, and act as novel and potential drug targets for therapeutic exploitation in human disease (Jiang W. et al., 2018; Zhou et al., 2019a). Long non-coding RNA X-inactive specific transcript (lncRNA Xist) are a set of 15,000–20,000 nt sequences localized in the X chromosome inactivation center (XIC) of chromosome Xq13.2 (Brown et al., 1992; Debrand et al., 1998; Kay, 1998; Lee et al., 2013; da Rocha and Heard, 2017; Yang Z. et al., 2018; Brockdorff, 2019). Previous studies have indicated that lncRNA Xist regulate X chromosome inactivation (XCI), resulting in the inheritable silencing of one of the X-chromosomes during female cell development. Also, it serves a vital regulatory function in the whole spectrum of human disease (notably cancer) and can be used as a novel diagnostic and prognostic biomarker and as a potential therapeutic target for human disease in the clinic (Liu et al., 2018b; Deng et al., 2019; Dinescu et al., 2019; Mutzel and Schulz, 2020; Patrat et al., 2020; Wang et al., 2020a). In particular, lncRNA Xist have been demonstrated to be involved in the development of multiple types of tumors including brain tumor, Leukemia, lung cancer, breast cancer, and liver cancer, with the prominent examples outlined in Table 1. It was also believed that lncRNA Xist (Chaligne and Heard, 2014; Yang Z. et al., 2018) contributed to other diseases, such as pulmonary fibrosis, inflammation, neuropathic pain, cardiomyocyte hypertrophy, and osteoarthritis chondrocytes, and more specific details can be found in Table 2. This review summarizes the current knowledge on the regulatory mechanisms of lncRNA Xist on both chromosome dosage compensation and pathogenesis (especially cancer) processes, with a focus on the regulatory network of lncRNA Xist in human disease.
The Role of LncRNA Xist in X Chromosome Dosage Compensation
In most mammals, sex is determined by a system based on X and Y chromosomes (Deng et al., 2014), with males holding the XY chromosome and females XX. Dosage compensation is thus needed to ensure equivalent expression levels of sex-linked and autosomal genes (Polito et al., 1990; Bone and Kuroda, 1996; Larsson and Meller, 2006; Disteche, 2012, 2016; Ferrari et al., 2014) despite the presence of an extra X-chromosome in female cells (Deng et al., 2014). X-chromosome inactivation (XCI), which refers to the random selection and transcriptional silence of one of two X-chromosomes in females at the early stages of embryonic development, is a unique dosage compensation mechanism in mammals (Waldron, 2016; Bar et al., 2019; Strehle and Guttman, 2020; Yu B. et al., 2020). In most placental mammals, there are two waves of XCI: the imprinted XCI exists in the fertilized embryo and extraembryonic tissues, and the random XCI persists in the inner cell mass (after implantation around embryonic day 5.5), yet humans lack the imprinted XCI and instead have X chromosome dampening (XCD) (Ropers et al., 1978; Harper et al., 1982; Kung et al., 2013; Lee et al., 2013; van Bemmel et al., 2016; Finestra and Gribnau, 2017; Sahakyan et al., 2018).
XCI is subdivided into distinct phases: initiation, establishment, and maintenance of the inactive X-chromosome (Gontan et al., 2011; Maduro et al., 2016). Initiation phase is a stochastic process (Spatz et al., 2004; Maduro et al., 2016; Jegu et al., 2017) that involves X-X pairing, counting, and XCI activation (xist activation, etc.) processes, and ensures that any number of X chromosomes randomly generate only one active X chromosome (Xa) expressed in each female cell and inactive X chromosome (Xi) is hetero-chromatinized and silenced in female cells. Establishment phase (Spatz et al., 2004; Maduro et al., 2016; Colognori et al., 2020) involves building a chromosomal memory that persists through the ensuring maintenance phase and ensures stable retention of repressive heterochromatin. Once the establishment phase is completed, the XCI is remarkably stable and becomes more difficult to reactivate. Maintenance phase is keeping the silenced state of XCI after the establishment phase via continuing lncRNA Xist expression. Once Xi is established, the Xi fully maintains its silent configuration and is clonally propagated throughout cell divisions (Maduro et al., 2016; Finestra and Gribnau, 2017). Numorous studies suggest that all three phases of XCI are governed by the lncRNA Xist (Lu et al., 2017; Sahakyan et al., 2018; Sidorenko et al., 2019).
XIC is the X-linked minimal genetic region which contains various factors and genes, such as Xist and Tsix, that are necessary and sufficient to initiate the XCI process in female cells (Willard, 1996; Sherstyuk et al., 2013; Hwang et al., 2015; Loda and Heard, 2019). XIC (Figure 1) is located in 100–500 kb region of mouse X chromosome and 2.3 Mb syntenic region of human X chromosome, and includes a cluster of lncRNA loci, such as Ftx, Jpx, Xist, Tsix, Xite, RepA, and so on (Spatz et al., 2004; Augui et al., 2011; Maduro et al., 2016; Jegu et al., 2017; Loda and Heard, 2019; Sidorenko et al., 2019). lncRNA Xist exists inside XIC, specifically at a location 15 kb downstream from Tsix antisense (Sado and Brockdorff, 2013; Gendrel and Heard, 2014; Mira-Bontenbal and Gribnau, 2016; da Rocha and Heard, 2017; Pintacuda et al., 2017b; Monfort and Wutz, 2020), and contains several functional domains that are a series of conservation repetitive motifs of A-to-F repeats (Figure 1). lncRNA Xist is transcriptionally activated with the initiation of the XIC process and is also believed to contribute to the complete process of XCI as a master regulator.
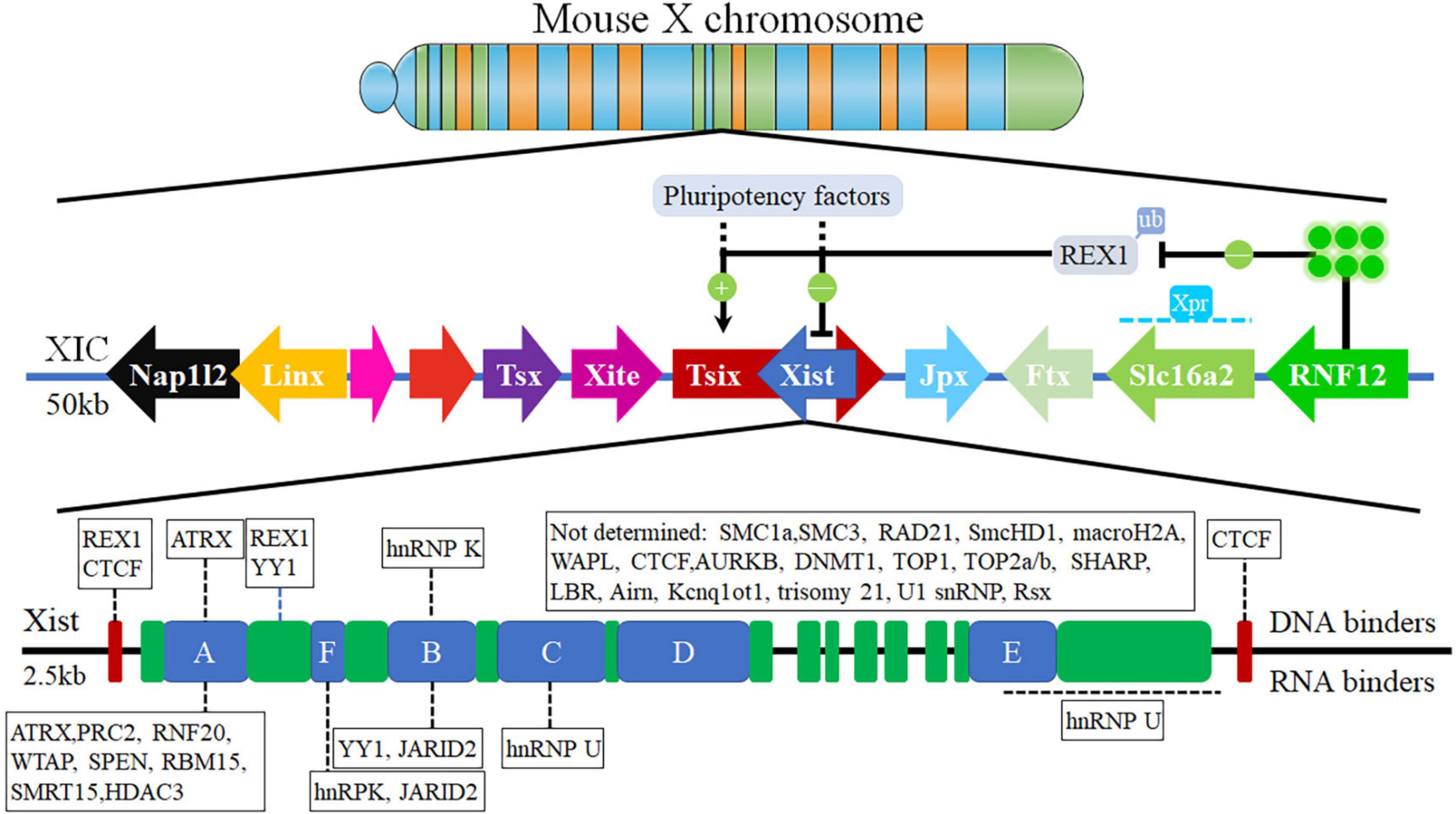
Figure 1. The X-chromosome Inactivation Center (Maduro et al., 2016). The X inactivation center consists of the different genes located and multiple genes encoding lncRNA, containing Xist, Tsix, Tsx, Xite, Jpx, Ftx, DNA binders, and RNA binders.
lncRNA Xist and its associated chromatin modifying complex plays a vital role in the regulation of the XCI process (Figure 2). A detailed description of the XCI process is beyond the scope of this review, and more specific detail is given in references (Spatz et al., 2004; Augui et al., 2011; Jegu et al., 2017), We briefly described regulatory process involved in LncRNA Xist in the review (Figure 2). During the initiation phase, the complex factors (OCT4, CTCF, Tsix, Xite, etc.), which separately bind the Xa and Xi, facilitates the X chromosome pairing and counting in the embryo after fertilization (Xu et al., 2006; Donohoe et al., 2009; Kung et al., 2015). After counting and pairing, XCI initiation is also accompanied by Tsix, Xist, etc. upregulation which is controlled by the network of genetic interactions (Figure 2B), such as Tsix, Sox2, PRDM14, OCT4, Jpx, Rnf12, and RepA (Augui et al., 2011; Khamlichi and Feil, 2018). When complete onset of XCI occurs, they employ divergent transcription fates with one becoming the Xa chromosome and the other becoming the Xi chromosome (Jegu et al., 2017). In Xi, lncRNA Xist activation and expression is modulated by numerous factors, such as pluripotency factor (NANOG, OCT4, SOX2, PRDM14, and REX1), RNF12, Tsix, and RepA (PcG protein recruitment), and more information is given in reference (Augui et al., 2011; Khamlichi and Feil, 2018). The regulation of Tsix expression is beyond the scope of this review, and more specific details can be found in references (Willard, 1996; Gontan et al., 2012; Gayen et al., 2015). Once Xist expression has been activated, Xist binds Polycomb repressive complex 2 (PRC2) via Repeat A formed Xist-PRC2 complex, and YY1 tethers the PRC2-Xist complex through Repeat C to the Xi nucleation center which obtains lncRNA Xist-PRC2 complex by the RNA polymerase II (RNA Pol II) (Jeon and Lee, 2011; Thorvaldsen et al., 2011; Makhlouf et al., 2014; Chigi et al., 2017).
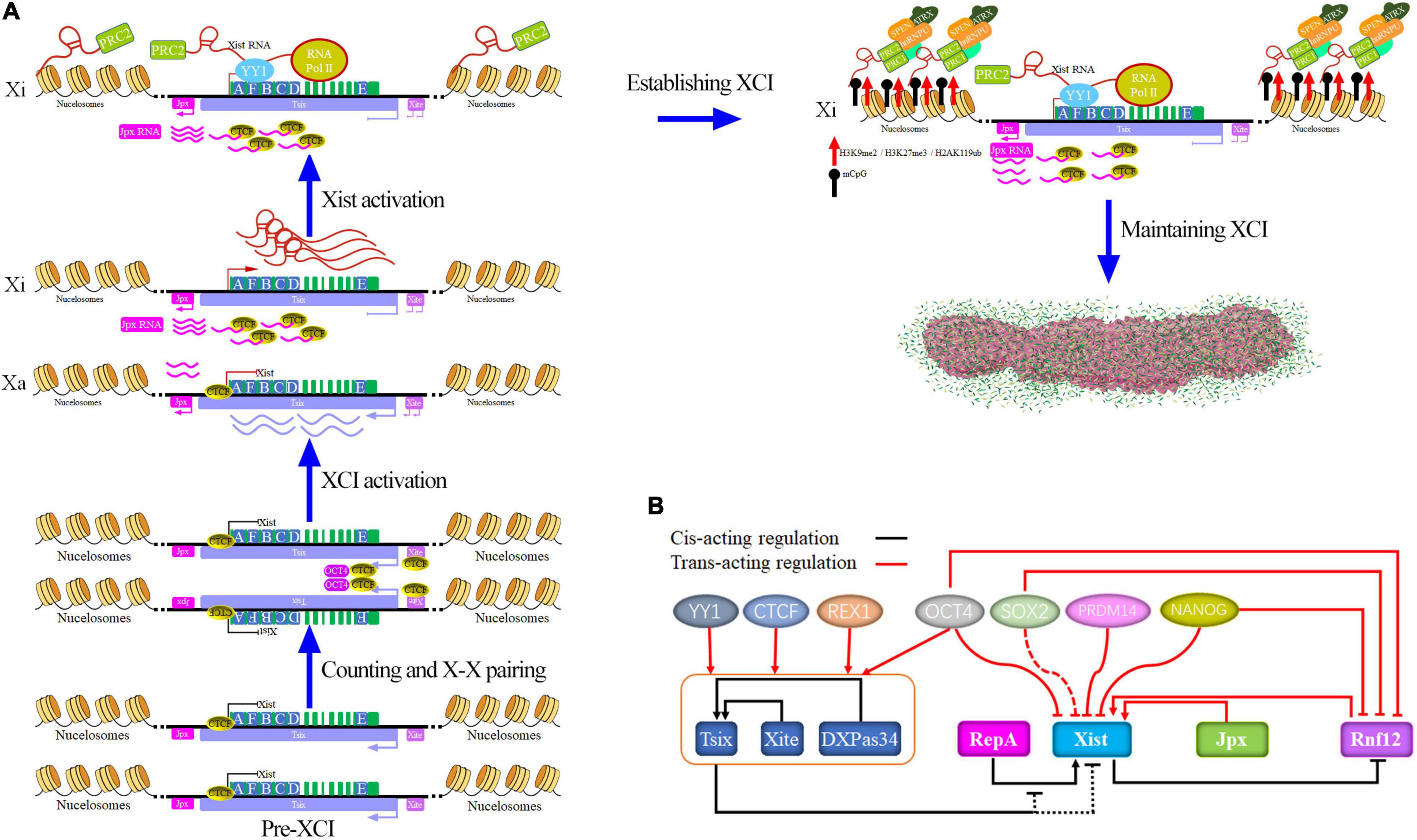
Figure 2. Model for Xist and Xist regulation at the process of XCI. (A) The process of dynamic and multifaceted modulation of XCI by lncRNA Xist. lncRNA Xist is a multitasking RNA that recruits protein complexes (such as OCT4, CTCF, Tsix, Xite, PRC1, PRC2, SPEN, ATRX, hnRNPU, hnRNPK, SHARP, HDAC3, LBR, Airn, Kcnq1ot1, RBM15, WTAP, trisomy 21, U1 snRNP, Rsx, Sox2, PRDM14, Jpx, Rnf12, and RepA) to initiate, establish, and maintain the XCI state by histone modifications, DNA methylation, and H4 hypoacetylation. (B) LncRNA Xist regulation network of genetic interactions (Augui et al., 2011). Note that here arrows do not necessarily imply direct regulation.
After the initiation phase, LncRNA Xist recruits protein complex factors excluding RNA Pol II, and induces a global suppression of lncRNA Xist topologically associated domains (TAD), which is involved in epigenetic modification and chromatin compaction to the Xi chromosome to spreads along the Xi at the established phase (Giorgetti et al., 2016; Mira-Bontenbal and Gribnau, 2016; Finestra and Gribnau, 2017; van Bemmel et al., 2019; Galupa et al., 2020). These protein complexes (Figure 2A) include the heterogeneous nuclear protein U (hnRNPU; also known as SAF-A), which is required for lncRNA Xist localization (Hasegawa et al., 2010; Kolpa et al., 2016; Sakaguchi et al., 2016; Loda and Heard, 2019), heterogeneous nuclear ribonucleoprotein K (hnRNPK), which is required for Xist-mediated chromatin modifications and Polycomb recruitment but not lncRNA Xist localization (Chu et al., 2015; Pintacuda et al., 2017a; Loda and Heard, 2019; Wang et al., 2019g), and the gene-silencing factor Spen, which is not required for Xist RNA localization (Chu et al., 2015; Monfort et al., 2015; Loda and Heard, 2019; Dossin et al., 2020) and binds to C, B, F, and A repeats at the 5′ end of the lncRNA Xist. ATRX directs binding to two major Polycomb repressive complexes (PRCs). -PRC1 and -PRC2 are involved in epigenetic silencing (acetylation of histone H3 and H4 and CpG island methylation, etc.) (Sarma et al., 2014; Minajigi et al., 2015; Pinheiro and Heard, 2017; Colognori et al., 2019; Lee et al., 2019; Wang et al., 2019a; Chen and Zhang, 2020). Other protein complexes (Mira-Bontenbal and Gribnau, 2016; Loda and Heard, 2019) also take part in the lncRNA Xist spreading procession, such as SHARP (McHugh et al., 2015), HDAC3 (Zylicz et al., 2019), LBR (Chen C.K. et al., 2016; Nesterova et al., 2019), Airn and Kcnq1ot1 (Schertzer et al., 2019), RBM15 and WTAP (Mira-Bontenbal and Gribnau, 2016), trisomy 21 (Jiang et al., 2013), U1 snRNP (Yin et al., 2020), Rsx (Grant et al., 2012), and CdK8 (Postlmayr et al., 2020). LncRNA Xist recruits repressive complexes, which leads to immediate histone modifications and DNA methylation (such as H2AK119Ub, H3K27me3, and CpG island) and coats on the Xi to build Xi (Mira-Bontenbal and Gribnau, 2016; Pinheiro and Heard, 2017; Wang et al., 2020a). Taken together, the Xi has been established and maintained in an inactive state by continuous synthesis of lncRNA Xist RNA.
The Role of LncRNA Xist in Cancer
Cancer, of which there are over 200 different types, is a complex disease in which cells in a specific tissue are no longer fully responsive to the signals within the tissue that regulate cellular differentiation, survival, proliferation, and death. As a result, these cells accumulate within the tissue, causing local damage and inflammation Cancer cells proliferate (growth) out of control, spread to other tissues (metastasize), and lose the ability to die via the normal process of cell apoptosis (death). The discovery of lncRNA Xist has contributed to cancer development and progression by regulation of the downstream signaling processes (Table 1). This also provides a window into the understanding of aberrant expression of lncRNA Xist associated with tumorigenesis, metastasis, and tumor stage. lncRNA Xist is a novel potential biomarker and potentially could be used in diagnosis and therapy for different types of cancer.
LncRNA Xist in Bladder Cancer
Bladder cancer is more common in men than in women, with respective incidence and mortality rates of 9.6 and 3.2 per 100,000 in men, which is about 4 times that of women globally (Chen W. et al., 2016; Bray et al., 2018). lncRNA Xist has recently been reported to regulate bladder cancer development through regulating several miRNAs or other target genes. lncRNA Xist exerts an oncogenic role through binding to miR-124, miR-139-5p, miR-200c, miR-133a, and miR-335 targets AR, Wnt1, TET1, and p53, which affect cell growth, invasion and migration, and metastasis (Hu et al., 2017; Xiong et al., 2017; Xu R. et al., 2018; Hu B. et al., 2019; Zhou et al., 2019c; Chen D. et al., 2020). This research uncovered that lncRNA Xist may be invoked as a potential therapeutic and prognostic biomarker for bladder cancer.
LncRNA Xist in Breast Cancer
Breast cancer accounts for almost one in four cancer cases among women, with respective incidence and mortality rates of 24.2 and 15.0%, and is the most commonly diagnosed cancer and leading cause of cancer death in women globally (Chen W. et al., 2016; Bray et al., 2018). Some previous studies have suggested that deregulation of lncRNA Xist plays a vital role in the pathogenesis of both inherited and sporadic breast cancer (Kawakami et al., 2004; Soudyab et al., 2016). The Breast Cancer 1 protein (BRCA1) is a tumor suppressor. Reduced expression of BRCA1 leads to increased risk of breast cancer development (Romagnolo et al., 2015). LncRNA Xist, which is dependent on the production of BRCA1 and may participate in regulating breast cancer development, is highly expressed in BRCA1-like breast cancer as a predictive biomarker (Sirchia et al., 2005, 2009; Vincent-Salomon et al., 2007; Schouten et al., 2016). It is thought that histone modifications (histone deacetylase inhibitor) and DNA methylation plays a critical role in breast cancer growth and metastasis (Librizzi et al., 2015; Shukla et al., 2019). Some research indicated that breast tumors frequently display major epigenetic instability of XI which is mediated by lncRNA Xist, and this phenomenon regulates breast cancer cells’ proliferation and differentiation (Salvador et al., 2013; Chaligne et al., 2015). In addition to the indirect regulation of competing endogenous RNA (ceRNAs), studies published to date have demonstrated that knockdown or overexpressed LncRNA Xist in breast cancer results in sponging five miRNAs, containing miR-155, miR-20a, miR-200c-3p, miR-125b-5p, and miR-362-5p, and positively regulates the downstream targets including CDX1, TP53, ANLN, NLRC5, and UBAP1, which affects breast cancer cells’ growth, proliferation, metastasis, migration, invasion, apoptosis, epithelial mesenchymal transition (EMT), and doxorubicin resistance (Zhao L. et al., 2018; Zheng R. et al., 2018; Li et al., 2020e; Liu et al., 2020a; Zhang et al., 2020a).
Triple-negative breast cancer (TNBC) is a subtype of breast cancer that accounts for approximately 10–20% of total breast cancer cases (Prat et al., 2015; Bianchini et al., 2016; Medina et al., 2020). The deficiency of estrogen, progesterone, and ERBB2 receptor expression leads to its highly invasive nature and relatively low response to current therapeutics approaches. Collectively, lncRNA Xist interacts with miR-454 to inhibit cell growth in TNBC (Li et al., 2020d). And lncRNA Xist sponges with miR-92b/Slug/ESA signaling pathway to suppresses TNBC growth (Li et al., 2020c). lncRNA Xist also positively regulates PHLPP1 expression via sequestering HDAC3 from the PHLPP1 promoter to influence cells’ viability (Huang et al., 2016). In cancer immunity and brain metastasis, lncRNA Xist involves cancer immunity in high expression programmed cell death protein 1 ligand TNBC cells via activating both OCT4 and NANOG though activating PI3K/AKT/mTOR signaling pathway (Salama et al., 2019). lncRNA Xist also promotes brain metastasis in breast cancer by activating the MSN-c-Met pathway and augmenting secretion of exosomal miR-503 (Xing et al., 2018), which may serve as an effective target for the treatment of brain metastasis. These findings demonstrate LncRNA Xist may contribute to a significant approach to the treatment of breast cancer.
LncRNA Xist in Colorectal Cancer
Colorectal cancer, with respective incidence and mortality rates of 10.2 and 9.2% in the world and which presents a rising trend in recent decades in China, ranks third in term of incidence but second in terms of mortality (Chen W. et al., 2016; Bray et al., 2018). As previously mentioned, lncRNA Xist exerts its function in colorectal cancer cells’ development by serving as a miRNA sponge. Zhang et al. (2019e) reported that lncRNA Xist, which modulates tumor size, plays a critical role in clinical prognosis and progression of colorectal cancer. Growing evidence from recent studies has shown that lncRNA Xist facilitates proliferation, metastasis, invasion, and EMT of colorectal cancer cells by functioning as an endogenous sponge of miR-200b-3p, miR-137, miR-132-3p, miR-486-5p, and miR-93-5p, thus affecting the expression of miRNAs target gene containing ZEB1, EZH2, MAPK1, NRP-2, and HIF-1A (Chen D.L. et al., 2017; Song et al., 2017; Liu et al., 2018a, 2019a; Yang L.G. et al., 2020). But beyond that, lncRNA Xist has been identified as the downstream target of methyltransferase-like14 (METTL14) by RNA-seq and Me-RIP, and its expression negatively correlating with METTL14 and YTHDF proteins 2 (YTHDF2) has been observed in colorectal cancer tissues (Yang X. et al., 2020). Yang X. et al. (2020) identified that METTL14-YTHDF2-lncRNA Xist axis mediated cells’ proliferation and metastasis in colorectal cancer.
In drug resistance of colorectal cancer cells, lncRNA Xist has been implicated in the resistance of colorectal cancer cells to chemoresistance via serving as a miRNA sponge. lncRNA Xist participates in the processes of drug resistance by modulating the axis of miR-124/serum and SGK1, miR-338-3p/PAX5, and miR-30a-5p/ROR1 (Zhu J. et al., 2018; Zhang et al., 2019d; Ma et al., 2020). Interestingly, Xiao et al. (2017) reported that overexpression of lncRNA Xist in colorectal cancer confers a potent poor therapeutic efficacy, and lncRNA Xist enjoys 5FU resistance via enhancing the expression of thymidylate synthase. In summary, this information indicates that lncRNA Xist may serve as an independent risk factor for colorectal cancer prognosis, and could be a potential therapeutic target and prognostic biomarker for colorectal cancer patients (Yu J. et al., 2020).
LncRNA Xist in Glioblastoma
Glioblastoma (GBM), with incidence rates of 3.2 per 100,000 and relative 5-year mortality rate of just 94.9%, is the most common and lethal primary intracranial tumor with few advances in treatment over the last several decades (Batash et al., 2017; McFaline-Figueroa and Wen, 2017; Kim et al., 2018). Accumulating evidence suggests that lncRNA Xist has a pivotal role in regulating glioma cells’ properties by interacting with miRNA. lncRNA Xist affects glioblastoma development by directly binding miR-152 and miR-429 (Yao et al., 2015; Cheng Z.H. et al., 2017). However, the downstream target gene of miR-152 and miR-429 remains unclear. In addition, lncRNA Xist mediates glioma progression, tumorigenesis, metastasis, proliferation, apoptosis, and glucose metabolism by positively regulating Bcl-2, FOXC1, ZO-2, Rac1, ASCT2, SLC1A5, SOX4, Smurf1, and IRS1 by functioning as a ceRNA of miR-204-5p, miR-137, miR-133a, miR-27a, and miR-126 (Wang Z. et al., 2017; Yu H. et al., 2017; Cheng Z.H. et al., 2020; Luo C.X. et al., 2020; Shen J. et al., 2020; Sun Y. et al., 2020; Yao et al., 2020; Zhao Q. et al., 2020). In drug resistance of glioblastoma cells, lncRNA Xist has been demonstrated in the resistance of human glioblastoma cells to Temozolomide (TMZ) via the miR-29c/DNA mismatch repair (MMR) pathway (Du P. et al., 2017). And Velázquez-Flores et al. (2020) have reported that XIST and XIST-210 may act as potential biomarkers for Diffuse intrinsic pontine gliomas diagnosis and prognostic biomarkers. In summary, these findings revealed that lncRNA Xist has an oncogenic role in the tumorigenesis of glioma and may serve as a novel and potential therapeutic target for patients with glioblastoma.
LncRNA Xist in Hepatocellular Carcinoma
Liver cancer, with respective incidence and mortality rates of 4.7 and 8.2%, was predicted to be the sixth most commonly diagnosed cancer and the fourth leading cause of cancer death worldwide in 2018 (Chen W. et al., 2016; Bray et al., 2018). The major risk factors of hepatocellular carcinoma are chronic infection with hepatitis B virus (HBV) or hepatitis C virus (HCV), aflatoxin-contaminated foodstuffs, heavy alcohol intake, obesity, smoking, and type 2 diabetes, and accounts for about 75–85% of primary live cancer (Chen W. et al., 2016; Bray et al., 2018). Recent studies have proposed that lncRNA Xist exerts tumorigenesis in hepatocellular carcinoma (Ma W.J. et al., 2017; Ma X. et al., 2017). LncRNA Xist, which functions as a ceRNA to regulate target HMGB1, SOX6, Smad7, PDK1/AKT, MAPK1, PDCD4, and PTEN expression by sponging miR-29b, miR-155-5p, miR-92b, miR-139-5p, miR-194-5p, miR-497-5p, and miR-181a, facilitates cells’ growth, autophagy, metastasis, and invasion via activating the miRNA/target signaling pathway (Zhuang et al., 2016; Chang et al., 2017; Mo et al., 2017; Kong et al., 2018; Lin et al., 2018; Xie et al., 2019; Zhang et al., 2019h). Analogously, Liu and Xu (2019) also demonstrated that silencing lncRNA Xist, whose expression level is significantly higher in hepatocellular carcinoma tissue compared with adjacent tissues, inhibits cell growth and tumor formation in hepatocellular carcinoma by directly interacting with miR-200b-3p, but the downstream target gene of miR-200b-3p remains unclear. All in all, these studies will contribute to providing a promising treatment for hepatocellular carcinoma.
LncRNA Xist in Nasopharyngeal Carcinoma
Nasopharyngeal carcinoma, with incidence rates of 0.7% and unknown mortality rates, is relatively uncommon compared with other cancers and is one of the most common malignant tumors in the head and neck (Chua et al., 2016; Wei K.R. et al., 2017). Accumulating studies suggests that the molecular function of lncRNA Xist has a pivotal function in nasopharyngeal carcinoma properties, such as cell proliferation, migration, and invasion. Knockdown of lncRNA Xist, which negatively regulates expression of miR-29c and miR-491-5p whose target gene remains unclear, suppressed cell proliferation, invasion, and growth and induces apoptosis in nasopharyngeal carcinoma (Han et al., 2017; Cheng Q. et al., 2018). Analogously, lncRNA Xist, which is highly expressed in nasopharyngeal carcinoma tissues and cell lines, facilitates nasopharyngeal carcinoma development via activating miR-34a-5p/E2F3, miR-148a-3p/ADAM17, and miR-381-3p/NEK5 axis (Song et al., 2016; Shi et al., 2020; Zhao C.H. et al., 2020). In drug resistance of nasopharyngeal carcinoma cells, lncRNA Xist, which may present a novel and potential therapeutic target in nasopharyngeal carcinoma, has been implicated in the resistance of human nasopharyngeal carcinoma cells to cisplatin (DDP) by facilitating programmed cell death 4 (PDCD4) and Fas ligand (Fas-L) expression (Wang et al., 2019b). On the whole, these reports will be play a novel role in the treatment of nasopharyngeal carcinoma.
LncRNA Xist in Lung Cancer
Lung cancer, with respective incidence and mortality rates of 11.6 and 18.4%, is the second most common cancer and remains the leading cause of cancer incidence and mortality worldwide (Chen W. et al., 2016; Bray et al., 2018). Emerging research demonstrates that lncRNA Xist are usually dysregulated in lung cancer and play a pivotal function in lung carcinoma initiation, progression, and therapy. lncRNA Xist, which has an oncogenic role in lung carcinoma, is closely correlated with tumor progression via regulating miR-140/iASPP axis and TCF-4 expression (Tang et al., 2017; Sun and Xu, 2019). Lung adenocarcinoma, which account for approximately 40% of total lung carcinoma, is also the most common histological subtype of NSCLC (Rong et al., 2020). lncRNA Xist expedites cancer progression and the resistance of cisplatin in lung adenocarcinoma via mediating the miR-363-3p/MDM2 and let-7i/BAG-1 signaling pathway (Sun J. et al., 2017; Rong et al., 2020). These results indicated that lncRNA Xist is likely to be a new marker and potential therapeutic target for patients with lung adenocarcinoma.
Non-small cell lung carcinoma (NSCLC), which accounts for 85% of lung cancer cases, is the most common subtype of lung cancer (Zhou et al., 2018). Accumulating evidence has revealed that lncRNA Xist is a pivotal regulator of cell proliferation, EMT, migration, invasion, and drug resistance in NSCLC. lncRNA Xist acts as an oncogene in NSCLC by modulating HIF1A-AS1 and KLF2 expression (Tantai et al., 2015; Fang et al., 2016). lncRNA Xist also positively mediates Bcl-2, LARP1, PXN and Notch-1, CBLL1, and RING1 expression by functioning as a ceRNA of miR-449a, miR-374a, miR-137, miR-212-3p, and miR-744, which are involved in cell proliferation, migration, invasion, EMT, and death in NSCLC (Xu Z.Z. et al., 2017; Zhang Y.L. et al., 2017; Jiang H.J. et al., 2018; Wang et al., 2018c, 2019c; Qiu et al., 2019). In addition, lncRNA Xist (Wang H.Y. et al., 2017), which has a higher expression in NSCLC cell lines and tissues, increases cell proliferation and invasion by negatively regulating miR-186-5p expression; however, the downstream target gene of miR-186-5p remains unclear.
It has been reported (Li C. et al., 2018) that TGF-β (Transforming growth factor β)-induced EMT serves a vital role in NSCLC metastasis and invasion. lncRNA Xist promotes TGF-β-induced EMT by positively regulating ZEB2 via interacting with miR-367 and miR-141 (Li C. et al., 2018). Analogously, lncRNA Xist inhibits NSCLC progression by sponging miR-16, miR-335, and miR-142-5p, and regulating target CDK8, SOD2/ROS, and PAX6 expression (Liu et al., 2019b; Zhou et al., 2019e; Jiang et al., 2020). Drug resistance is one of the most common reasons for therapeutic failure in patients with NSCLC and a persistent issue that requires continued investigation. Emerging evidence indicated that lncRNA Xist is associated with cisplatin resistance in NSCLC by TGF-β effector SMAD2 signaling pathway, miRNA-144-3p/MDR1 and MRP1, and miR-17/ATG7 axis (Sun W. et al., 2017; Tian et al., 2019; Xu et al., 2020). All in all, this evidence suggests that lncRNA Xist may offer a hopeful diagnostic and therapeutic choice for the treatment of NSCLC.
LncRNA Xist in Osteosarcoma
Bone cancer, with respective incidence and mortality rates of 0.20 and 0.28%, occurs frequently in children, adolescents, and young adults aged 15 to 29 years (Siegel et al., 2019, 2020). Osteosarcoma, which accounts for 20 to 40% of all bone tumors, are the most frequent morphological subtypes of bone cancer, representing a worldwide and common primary malignant bone tumor in children and adolescents (Balmant et al., 2019; Muller and Silvan, 2019). Growing evidence from recent studies has shown that lncRNA Xist is aberrantly regulated in osteosarcoma. LncRNA Xist, which participated in osteosarcoma development processes, including cell proliferation, migration, invasion, EMT, and apoptosis, is involved in gene regulation through a variety of mechanisms, primarily by functioning as a miRNA sponge and via interacting with its targets (Li et al., 2017; Wang et al., 2019f; Han and Shen, 2020), such as miR-153/SNAI1 pathway (Wen et al., 2020), EZH2, PUMA, and NF-kB (Xu T. et al., 2017; Gao et al., 2019).
In addition to indirect modulation of ceRNAs, studies published to date have indicated that high lncRNA Xist expression in osteosarcoma results in sponging six miRNAs, namely miR-21-5p, miR-193a-3p, miR-195-5p, miR-320b, miR-137, and miR-375-3p, which affects osteosarcoma progression (Wu D.P. et al., 2017; Zhang and Xia, 2017; Lv et al., 2018; Yang C. et al., 2018; Li H. et al., 2019; Sun X. et al., 2019). lncRNA, which regulates miR-21-5p/PDCD4 axis, miR-193a-3p/RSF1 axis, miR-195-5p/YAP axis, miR-137, miR-320b/RAP2B axis, and miR-375-3p/KT/mTOR axis, contributes to osteosarcoma cell growth, metastasis, and invasion by activating MAPK signaling pathway, NF-kB signaling pathway, and PI3K-AKT-mTOR signaling pathway. Taking all into account, these studies indicated that lncRNA Xist may act as a candidate prognostic biomarker and a promising therapeutic target for osteosarcoma (Wu D.P. et al., 2017; Zhang and Xia, 2017; Lv et al., 2018; Yang C. et al., 2018; Li H. et al., 2019; Sun X. et al., 2019).
LncRNA Xist in Pancreatic Cancer
Pancreatic cancer, with respective incidence and mortality rates of 2.5% (China, 2.1%, 2015) and 4.5% (China, 2.8%, 2015), was the seventh leading cause of cancer death worldwide in both males and females in 2018 (Chen W. et al., 2016; Bray et al., 2018). Accumulating evidence indicated that lncRNA Xist interacts with additional miRNAs, such as miR-133a, miR-140 and miR-124, miR-34a-5p, miR-34a, miR-141-3p, and miR-429 in pancreatic cancer, and is involved in the development and progression of pancreatic cancer (Liang et al., 2017; Wei W. et al., 2017; Sun Z. et al., 2018; Shen et al., 2019; Sun and Zhang, 2019; Zou et al., 2020). As aforementioned, lncRNA Xist promotes pancreatic cancer cells’ proliferation by binding miR-133a, thus affecting the miR-133a downstream target gene EGFR (epidermal growth factor receptor) which is positively correlated with lncRNA Xist (Wei W. et al., 2017). lncRNA Xist also facilitates miR-140/miR-124/iASPP/CDK1 axis, miR-34a/YAP axis, miR-141-3p/TGF-β2 axis, miR-429/ZEB1 axis and miR-34a-5p, which contributes to carcinoma cell growth, EMT, migration, and invasion (Liang et al., 2017; Sun Z. et al., 2018; Shen et al., 2019; Sun and Zhang, 2019; Zou et al., 2020). However, the downstream target gene of miR-34a-5p remains unknown. Taken together, the above research results suggested that lncRNA Xist could be regarded as a candidate prognostic biomarker and a potential therapeutic target in human pancreatic carcinoma.
LncRNA Xist in Retinoblastoma
Retinoblastoma, which has a significant effect on mortality in emerging countries but is more curable in industrialized countries, is an aggressive eye cancer that affects infants and children (Cassoux et al., 2017). Recently, abundant studies demonstrated that dysregulation lncRNA was involved in tumorigenesis and cancer progression of retinoblastoma (Yang and Wei, 2019). Compared to healthy controls, lncRNA Xist was significantly upregulated in plasma of retinoblastoma patients which was inversely associated with lncRNA NKILA (Lyu et al., 2019). LncRNA Xist overexpression promotes retinoblastoma cells proliferation, migration, and invasion rates via negatively regulating lncRNA NKILA, but the causality has not been fully validated. In addition, lncRNA Xist, which indirectly interacts with miR-21-5p, miR-124, miR-101, miR-140-5p, and miR-200a-3p, and positively regulates VEGF, STAT3, ZEB1 and ZEB2, SOX4, and NRP1 expression, facilitates apoptosis, migration, EMT, proliferation, and invasion by activating signaling pathways, such as PI3K-Akt signaling pathway and MAPK-ERK signaling pathway (Hu et al., 2018; Cheng Y. et al., 2019; Dong et al., 2020; Wang et al., 2020c; Zhao H. et al., 2020). All in all, these studies suggested that lncRNA Xist serves a potential and promising clinical application for diagnosis, prognosis, and treatment.
LncRNA Xist in Cervical Cancer
Cervical cancer, with respective incidence and mortality rates of 3.2 and 3.3%, ranked as the fourth most frequently diagnosed cancer and the fourth leading cause of cancer death in women in 2018 worldwide (Chen W. et al., 2016; Bray et al., 2018). Recently, numerous reports found that dysregulation of lncRNA Xist was involved in regulating cervical cancer progression via binding to miRNAs. Zhu H. et al. (2018) demonstrated lncRNA Xist, which is extremely highly expressed in cervical cancer tissues and cell lines, accelerates cervical cancer progression via upregulating Fus through functioning as a ceRNA of miR-200a. In additional, lncRNA Xist upregulation, which positively facilitates ORC1 expression and acts as a ceRNA of miR-140-5p, contributes to the cervical cancer progression by activating miR-140-5p/ORC1 axis (Chen X. et al., 2019c). Similarly, Liu et al. (2020d) found that lncRNA Xist, which was highly expressed in cervical cancer cells and tissue, promoted cervical cancer cells’ proliferation, migration, and invasion and hindered apoptosis by inhibiting miR-889-3p and positively mediating SIXI expression. Taken together these studies demonstrated that lncRNA Xist may play a role in epigenetic diagnostics and therapeutics in cervical cancer.
LncRNA Xist in Gastric Cancer
Stomach cancer (cardia and non-cardiac gastric cancer combined), with respective incidence and mortality rates of 5.7 and 8.2%, was the fifth most frequently diagnosed cancer and the third leading cause of cancer death in 2018 worldwide, and remains an important cancer (Chen W. et al., 2016; Bray et al., 2018). Recently, some reports founded that lncRNA Xist exerts its function in gastric cancer progression by acting as a miRNA sponge, It acts on miRNA, such as miR-101, miR-497, miR-185, and miR-337. lncRNA Xist, which acts as a molecular sponge of miR-101, miR-497, miR-185, and miR-337 to mediate EZH2, MACC1, TGF-β1, and JAK2 expression, is involved in gastric cancer progression through mediating miR-101/EZH2 axis, miR-497/MACC1 axis, miR-185/TGF-β1 axis, and miR-337/JAK2 axis (Chen D.L. et al., 2016; Ma L. et al., 2017; Zhang Q. et al., 2018; Zheng W. et al., 2020). In drug resistance of gastric carcinoma cells, Li Y.D. et al. (2019) demonstrated that lncRNA Xist contributes to drug resistance of gastric cancer cells though positively facilitating the related gene MDR1 (multidrug resistance gene 1) and MRP1 (multi-drug resistance protein 1) of multidrug resistance, and is helpful for the molecule-targeted treatment of gastric cancer. Taken together, these findings suggest that lncRNA Xist may be a candidate prognostic biomarker and a new therapy target in gastric cancer patients.
LncRNA Xist in Melanoma
Melanoma, with respective incidence and mortality rates of 1.6 and 0.6%, was the most fatal form of skin cancer in 2018 worldwide and the rates are increasing faster than any other currently preventable cancers (Chen W. et al., 2016; Bray et al., 2018; Schadendorf et al., 2018). Recently, some findings suggested that a major role of lncRNA Xist is facilitating melanoma progression via acting as a miRNAs sponge to regulate its downstream target genes, such as lncRNA Xist (Zhang et al., 2019f), which promoted malignant melanoma growth and metastasis by functioning as a ceRNA though miR-217. However, the downstream target gene of miR-217 remains unclear. Analogously, lncRNA Xist (Pan et al., 2019; Tian K. et al., 2020), which functions as a ceRNA to positively regulate ROCK1 and PI3KRI and AKT expression by sponging miR-139-5p and miR-21, respectively, facilitates proliferation, invasion, and oxaliplatin resistance of melanoma cells. In summary, this evidence shows that lncRNA Xist could provide a novel insight into the pathogenesis and underlying therapeutic targets for melanoma.
LncRNA Xist in Esophageal Cancer
Esophageal cancer, with respective incidence and mortality rates of 3.2 and 5.3%, ranks seventh in terms of incidence and sixth in mortality overall, the latter signifying that esophageal cancer was responsible for an estimated 1 in every 20 cancer deaths in 2018 worldwide (Chen W. et al., 2016; Bray et al., 2018; Schadendorf et al., 2018). Recently, numerous reports demonstrated that dysregulation of lncRNA Xist was involved in regulating esophageal cancer development via binding to miRNAs. lncRNA Xist involves esophageal squamous cell carcinoma development via regulation of miR-101/EZH2 axis (Wu X. et al., 2017), and facilitates esophageal squamous cell carcinoma proliferation, apoptosis, migration, and invasion via regulation miR-494/CDK6 axis and activation of JAK2/STAT3 signal pathway (Chen et al., 2019f). In addition, lncRNA Xist predicts the presence of lymph node metastases in human esophageal squamous cells (Li and He, 2019; Wang et al., 2020b). Taken together, these results demonstrated that lncRNA Xist may provide a novel candidate prognostic biomarker and a new insight for esophageal carcinoma therapy.
LncRNA Xist in Laryngeal Squamous Cell Carcinoma
Laryngeal cancer, with respective incidence and mortality rates of 1.0 and 1.0%, was one of the most common tumors of the respiratory tract in 2018 worldwide (Chen W. et al., 2016; Steuer et al., 2017; Bray et al., 2018). Squamous cell carcinoma, accounting for approximately 90% of malignant neoplasms of the larynx, is the most common malignancy of the larynx (Thompson, 2017; Bradford et al., 2020). Recently, some evidence suggested that a major role of lncRNA Xist, which is notably up-regulated in laryngeal squamous cell carcinoma tissues and cells, promotes laryngeal squamous cell carcinoma progression via interacting with miRNAs to regulate its downstream target gene. lncRNA Xist increases the aggressiveness of laryngeal squamous cell carcinoma by functioning as a ceRNA sponge of miR-124 to regulate EZH2 expression (Xiao D. et al., 2019), and promotes progression of laryngeal squamous cell carcinoma via activating the miR-144/IRS1 axis (Cui et al., 2020), and promotes the malignance of laryngeal squamous cell carcinoma cells through functioning as a ceRNA of miR-125b-5p to positively modulate TRIB2 expression (Liu et al., 2020b). All together, these studies demonstrated that lncRNA Xist may serve as a new potential prognostic biomarker and putative target in the therapy of laryngeal squamous cell carcinoma.
LncRNA Xist in Ovarian Cancer
Ovarian cancer, which is the leading cause of death for women of reproductive age around the world and has a 5-year survival rate below 45%, is in eighth place among the most common cancers in women and the fifth leading cause of death among women worldwide, including 4% of all cancers (Webb and Jordan, 2017; Moga et al., 2018; Stewart et al., 2019). In order to prove the lncRNA Xist participated in ovarian cancer development, Wang et al. (2018a) revelated that lncRNA Xist is involved in ovarian cancer development by negatively regulating miR-214-3p expression. This confirmed that lncRNA Xist is closely associated with the tumor grade and distant metastasis in the ovarian cancer patients (Zuo et al., 2019). This result suggested that lncRNA Xist plays a role in tumor development. In addition, lncRNA Xist (Wang and Li, 2020), which functions as a ceRNA to positively mediate the expression of PDCD4 (programmed cell death protein 4) through binding to miR-150-5p and is significantly decreased in ovarian cancer tissues and cell lines compared with the normal tissue and cells, inhibits ovarian cancer cell growth and metastasis via regulating miR-150-5p/PDCD4 signaling pathway. All in all, these studies evaluated that lncRNA Xist provides insight into the potential target for the treatment of ovarian cancer, and a new evaluation of the diagnosis and prognosis of ovarian cancer.
LncRNA Xist in Others Cancer
Growing evidence from recent studies has shown that lncRNA Xist facilitates tumor development, including pituitary neuroendocrine tumor, neuroblastoma, thyroid cancer, colon cancer, renal cell carcinoma, and prostate cancer (Chaligne and Heard, 2014; Yang Z. et al., 2018; Liu et al., 2019c). In the pituitary neuroendocrine tumor cells (Zhou et al., 2019b), lncRNA Xist, which functions as a ceRNA to sequester miR-424-5p to elevate the expression of the its target bFGF, and exhibits high expression in invasive pituitary neuroendocrine tumor tissues as compared to non-invasive tumor tissues, promotes cancer progression in invasive pituitary neuroendocrine tumor via activating the miR-424-5p/bFGF signaling pathway. In neuroblastoma (Zhang et al., 2019a), lncRNA Xist, which interacts with EZH2 to downregulate DKK1 by inducing H3 histone methylation, promotes neuroblastoma cell growth, proliferation, migration, and invasion via modulating H3 histone methylation of DKK1 in neuroblastoma. In addition, lncRNA Xist (Yang H. et al., 2020) repressed tumor growth and boosted radiosensitivity of neuroblastoma via modulating the miR-375/L1CAM axis. In thyroid cancer, lncRNA Xist (Liu et al., 2018c), which positively regulates MET by sponging miR-34a, modulates the cell proliferation and tumor growth through activating the PI3K/AKT signaling pathway. Analogously, Xu Y. et al. (2018) demonstrated that lncRNA Xist, whose high expression is positively associated with TNM stage and lymph node metastasis, promotes cell proliferation and invasion by interacting with miR-141 in papillary thyroid carcinoma. However, the downstream target gene of miR-141 remains unknown. In colon cancer cells, lncRNA Xist (Sun N.N. et al., 2018), which functions as a ceRNA by binding to miR-34a and positively modulates WNT1 expression, has a crucial function in colon cancer progression via the miR-34a/WNT1 axis to activate the Wnt/β-catenin signaling pathway.
In addition to indirect regulation of ceRNAs, studies published to date have manifested that low lncRNA Xist expression in renal cell carcinoma results in sponging two miRNAs, miR-106b-5p and miR-302c, which regulates tumor development (Zhang J. et al., 2017; Sun K. et al., 2019). lncRNA Xist, which positively facilitates P21 and SDC1 expression through sponging miR-106b-5p and miR-302c, facilitates cell proliferation and apoptosis via miR-106b-5p/P21 signaling pathway and miR-302c/SDC1 axis. In prostate cancer cells, lncRNA Xist, which weakly expresses in normal prostate tissues but not in leukocytes, contributes prostate cancer development (cell proliferation and metastasis) by activating miR-23a/RKIP signaling pathway (Laner et al., 2005; Du Y. et al., 2017). In addition, SQ. Hai (2020) have indicated that LncRNA XIST/miR-124-3p/iASPP Pathway Promotes Growth of Human Chordoma Cells. Analogously, Lobo et al. (2019) manifested that demethylated and methylated XIST promoter may be involved in testicular germ cell tumor development. Altogether, this evidence pronounced that lncRNA Xist may shed new light on epigenetic diagnostics and therapeutics for cancer patients.
The Role of LncRNA Xist in Non-Cancer Diseases
Diseases are abnormal conditions that have a specific set of signs and symptoms. Diseases can have an external cause, such as an infection, or an internal cause, such as autoimmune disease (such as Alzheimer’s disease). Accumulating evidence has suggested that lncRNA Xist participates in non-cancer related diseases’ development and progression (Table 2) as a ceRNA regulatory network of miRNA-mRNA. It also provides a window into the understanding of aberrant expression of lncRNA Xist associated with non-cancer related diseases. lncRNA Xist is a novel potential biomarker and could potentially be involved in the diagnosis and therapy of different types of diseases.
LncRNA Xist in Cardiac Disease
Cardiac diseases, including coronary artery disease (CAD), myocardial infarction (MI), cardiac hypertrophy, and heart failure (HF), are among the leading causes of morbidity and mortality worldwide (Greco et al., 2018; Colpaert and Calore, 2019). Emerging evidence has revealed that lncRNA Xist acted as powerful and dynamic modifier of cardiac physiological and pathological processes. Cardiac hypertrophy, recognized as a risk predictor of sudden cardiac death, is an adaptive reaction in response to altered stress or injury to maintain cardiac function (Li Y. et al., 2018; Wehbe et al., 2019; Luo X. et al., 2020). lncRNA Xist (Sohrabifar, 2020) participates in the pathogenesis of complex diseases and also serves as a diagnostic marker. lncRNA Xist also positively regulates S100B expression through functioning as a ceRNA to bind miR-330-3p (Chen Y. et al., 2018) and functions as a ceRNA of miR-101 to enhance TLR2 expression (Xiao L. et al., 2019) and modulates the progression of cardiomyocyte hypertrophy by miR-330-3p/S100B pathway and miR-101/TLR2 axis.
Myocardial infarction (MI), colloquially known as “heart attack,” is caused by decreased or complete cessation of blood flow to a portion of the myocardium and by the rupture of atherosclerotic plaques, which results in damage to cardiomyocytes due to lack of oxygen (Colpaert and Calore, 2019; Ojha and Dhamoon, 2020). lncRNA Xist, which positively mediates PDE4D expression via interacting miR-130a-3p (Zhou et al., 2019d) and targets miR-101a-3p through regulating FOS (Lin B. et al., 2020), promotes myocardial infarction development and cell apoptosis, and inhibits cell proliferation though the miR-130a-3p/PDE4D aixs and miR-101a-3p/FOS aixs.
Acute MI (AMI) is characterized by ischemic injury and cardiomyocyte apoptosis, while myocardial injury, which is also an entity in itself, is a prerequisite for the diagnosis of MI in the setting of acute myocardial ischemia (Sandoval and Thygesen, 2017; Sandoval et al., 2017; Colpaert and Calore, 2019). lncRNA Xist, which interacts directly with miRNA (miR-150-5p, miR-122-5p, miR-125b, miR-133a, and miR-449) to positively regulate expression levels of mRNA (Bax, FOXP2, hexokianse 2, SOCS2, and Notch1), protects hypoxia-induced cardiomyocyte injury and represses the myocardial cell apoptosis though miR-150-5p/Bax pathway, miR-122-5p/FOXP2 axis, miR-125b/hexokianse 2 axis, miR-133a/SOCS2 pathway, and miR-449/Notch1 signaling pathway (Li Z.Q. et al., 2019; Zhang et al., 2019c; Fan et al., 2020; Peng et al., 2020; Zhou et al., 2020). These results indicated that lncRNA Xist represents a very promising potential pharmacotherapeutic target and biomarker for cardiac disease.
LncRNA Xist in Neuropathic Pain
Neuropathic pain, including central pain, peripheral pain, and cancer pain, is pain that arises as lesions or diseases of the somatosensory system, either at the peripheral or at the central level, and are treated by first-line (include antidepressants and anticonvulsants acting at calcium channels), second-, and third-line (include topical lidocaine and opioids) pharmacologicals (Xu et al., 2016; Fornasari, 2017; Eberlin, 2019). Growing studies have revealed that lncRNA Xist, which has been characterized as a key modulator of neuronal functions, plays a pivotal role in the development of neuropathic pain. In Down’s syndrome, lncRNA Xist (Czerminski and Lawrence, 2020), which fully corrects trisomy 21 dosage in neural cells, promotes differentiation of trisomic NSCs (neural stem cells) to neurons by silencing Trisomy 21 and activating Notch signaling pathway. In Parkinson’s disease (PD) animals, it has been shown that lncRNA Xist/miR-133b-3p/Pitx3 axis protect dopaminergic neurons through activation of CB2R with AM1241, which alleviates PD (He et al., 2020). In addition, lncRNA Xist participated in neuropathic pain though interacting with miRNAs in CCI (chronic constriction injury) rat models, including miR-154-5p, miR-137, miR-544, and miR-150. lncRNA Xist, which functions as a ceRNA to positively modulate mRNA expression (TLR5, TNFAIP1, STAT3, and ZEB1) by sponging miRNA (miR-154-5p, miR-137, miR-544, and miR-150), contributes to neuropathic pain development by facilitating miR-154-5p/TLR5 axis, miR-137/TNFAIP1 axis, miR-544/STAT3 axis, and miR-150/ZEB1 axis in CCI rat models (Jin et al., 2018; Yan et al., 2018; Zhao Y. et al., 2018; Wei et al., 2019).
Alzheimer’s disease (AD), which is a growing global health concern with huge implications for individuals and society, is a chronic progressive and irreversible neurodegenerative disorder (Scheltens et al., 2016; Lane et al., 2018; Chanda and Mukhopadhyay, 2020). Silencing lncRNA Xist (Wang et al., 2018b) attenuated Aβ(amyloid-beta peptide)25-35-induced toxicity, oxidative stress, and apoptosis in primary cultured rat hippocampal neurons by negatively mediating miR-132 expression. But the downstream target gene of miR-132 remains unclear. Similarly, Du Y. et al. (2020) showed that lncRNA Xist, which was significantly upregulated in hydrogen peroxide (H2O2)-induced AD mice models and in H2O2-treated N2a cells, is involved in Alzheimer’s disease development though positively regulating BACE1 expression by interacting with miR-124. These studies suggested that lncRNA Xist might provide novel therapeutic avenues for neuropathic diseases.
LncRNA Xist in Osteoarthritis
Osteoarthritis (OA), which is the most common joint disorder that affects one or several diarthrodial joints including small joints (such as those in the hand) and large joints (such as the knee and hip joints), is the most frequently diagnosed musculoskeletal disease and leads to functional decline and loss in quality of life (Kraus, 2014; Pereira et al., 2015). Accumulated evidence manifested that lncRNA Xist is associated with development and progression of OA. lncRNA Xist, which acts as a ceRNA of miR-211 to positively mediate miR-211-interacted CXCR4 expression, promotes the proliferation and apoptosis of OA through the miR-211/CXCR4 axis activating MAPK signaling pathway (Li L. et al., 2018). And lncRNA Xist (Liao et al., 2019), which positively regulates AHNAK expression to activate BMP2 Signaling Pathway by target with miR-17-5p, may influence Cervical Ossification of the PLL through facilitating of miR-17-5P/AHNAK/BMP2 axis. In periodontal ligament stem cells (PDLSCs), lncRNA Xist, which was elevated in osteogenic inducted PDLSCs, promoted Osteogenic Differentiation by negatively regulating the expression of miR-214-3p, but the downstream target gene of miR-214-3p remains unknown (Feng et al., 2020).
In osteoporosis (OP), lncRNA Xist (Chen et al., 2019a,d), which was highly expressed in the serum and monocytes of patients with OP, regulates osteoporosis through recruiting DNA methyltransferase and inhibiting bone marrow mesenchymal stem cell differentiation. In addition, a major role of lncRNA Xist is facilitating gene expression and affecting osteoarthritis development and progression via sponging to miRNAs. The lncRNA Xist/miR-9-5p/ALPL (Zheng C. et al., 2020) and lncRNA Xist/miR-1277-5p/MMP-13 and ADAMTS5 (Wang et al., 2019e) signaling pathway has been identified as a ceRNA regulatory network involved in osteoarthritis development. And other ceRNA regulatory networks have also been shown to contribute to the progression of Osteoarthritis, such as lncRNA Xist/miR-376c-5p/OPN signaling pathway (Li et al., 2020b), lncRNA Xist/miR-142-5p/SGTB signaling pathway (Ghaderian et al., 2020), lncRNA Xist/miR-149-5p/DNMT3A signaling pathway (Liu et al., 2020e), and lncRNA Xist/miR-675-3p/GNG5 signaling pathway (Shen X.F. et al., 2020). Increasing studies have shown that lncRNA Xist might act as a novel therapeutic target for OA patients.
LncRNA Xist in Inflammation
Inflammation, which is activated by inflammasomes that are innate immune system receptors and sensors that regulate the activation of caspase-1, is a protective immune response mounted by the evolutionarily conserved innate immune system in response to harmful stimuli, such as pathogens, dead cells, or irritants, and is tightly regulated by the host (Guo et al., 2015). Recent findings demonstrated the pivotal role of lncRNA Xist in the progression of the inflammatory response. NF-κB (nuclear factor-κB) signaling pathway, which plays a vital role in inflammation and innate immunity, were involved in cell proliferation and apoptosis and regulated the production of inflammatory cytokines including tumor necrosis factor (TNF)-α, interleukin (IL)-1β, IL−6, and IL−8 (Ma et al., 2019; Shenoda et al., 2020). lncRNA Xist facilitates acute inflammatory responses and bovine mammary epithelial cell inflammatory responses via NF-κB/NLRP3 inflammasome signaling pathway (Levey and James, 2017; Yang et al., 2019).
In addition to the indirect regulation of ceRNAs, studies published to date have demonstrated that high lncRNA Xist expression in inflammatory cells results in sponging four miRNAs, namely miR-27a-3p, miR-30c, miR-34c, and 146a, which responded to the inflammatory development process (Shenoda et al., 2018; Sun W.B. et al., 2018; Hu W.N. et al., 2019; Zhao Q. et al., 2020). The regulation of lncRNA Xist in most inflammation processes, including acute inflammation response in female cells, apoptosis, and inflammatory injury of microglia cells after spinal cord injury, cell apoptosis of HUVEC (Human umbilical vein endothelial cells) and ox-LDL (oxidized low-density lipoprotein)-induced the inflammatory response and inflammatory pain in rat. The pathways involved included lncRNA Xist/miR-34a/YY1 signaling pathway (Shenoda et al., 2018), lncRNA Xist/miR-27a/Smurf1 signaling pathway (Zhao Q. et al., 2020), lncRNA Xist/miR-30c-5p/PTEN signaling pathway (Hu W.N. et al., 2019), and miR-146a/Nav1.7 signaling pathway (Sun W.B. et al., 2018). These results suggest that lncRNA Xist could be involved in a promising strategy against inflammation and be a potential target for inflammatory patients.
LncRNA Xist in Kidney and Cardiovascular Disease
As the kidney and heart are intricately linked, abnormal function in one can lead to pathological function in the other (Lorenzen and Thum, 2016). Acute kidney injury (formerly known as acute renal failure), which is typically diagnosed by the accumulation of end products of nitrogen metabolism (urea and creatinine) or decreased urine output, or both, is a syndrome characterized by the rapid loss of the function of glomerular filtration rate (Levey and James, 2017; Ronco et al., 2019). Recent studies indicated lncRNA Xist exerts its function by serving as a miRNA sponge in the development of kidney injury. In diabetic nephropathy, lncRNA Xist, which is highly expressed in the kidney tissue of diabetic nephropathy mice and high glucose-exposed HK-2 cells, is involved in diabetic nephropathy development by positively facilitating CDKN1A (cyclin-dependent kinase inhibitor 1A) expression via functioning as a ceRNA of miR-93-5p (Yang et al., 2019). In LPS-induced SCI (Spinal cord injury) microglia cells and lncRNA Xist, which interacts with miR-27a to mediate the downstream target gene of Smurf1 expression, alleviated the apoptosis and inflammatory injury of microglia cells after SCI through activating miR-27a/Smurf1 axis (Zhao Q. et al., 2020). These signaling pathways, which include lncRNA Xist/miR-494/PTEN/PI3K/AKT signaling pathway (Gu et al., 2017), lncRNA Xist/miR-142-5p/PDCD4 signaling pathway (Tang et al., 2020), lncRNA Xist/miR-217/TLR4 signaling pathway (Jin et al., 2019), lncRNA Xist/miR-32-5p/Notch-1 signaling pathway (Cheng X. et al., 2020), and lncRNA Xist/miR-15a-5p/CUL3signaling pathway (Xu et al., 2019), participated in the SCI, acute kidney injury, and nephropathy procession. Cheng and Wang (2020) identified that lncRNA Xist could act as a ceRNA to sponge miR-212-3p and miR-122-5p to facilitate kidney transplant acute kidney injury progression via regulating the expression of ASF1A, BRWD1, and PFKFB2 using GEO database assay.
In contrast to kidney diseases, the study of lncRNA Xist in cardiovascular diseases is still in its infancy. Chen G. et al. (2018) suggested that lncRNA Xist, which is disrupted by aberrant expression of PFOS (Perfluorooctane sulfonate) in prenatal cells, facilitates placental angiogenesis by regulation of miR-429/VEGF-A axis. Similarly, lncRNA Xist (Hu C. et al., 2019), which positively modulates SOX7 (SRY-box 7) expression by sponging miR-485, participated in hypoxia-induced angiogenesis to activate VEGF signaling pathway, ERK1/2, and Akt signaling pathway through regulation miR-485/SCX7 axis. In additional, Stanford Type A Aortic Dissection (TAAD) is one of the most lethal cardiovascular diseases with an extremely high morbidity and mortality rate. Zhang et al. (2020b) have suggested that lncRNA Xist, which positively regulates PTEN expression via its competitive target miR-17, modulates the proliferation and apoptosis of vascular smooth muscle cells to affect Stanford Type A Aortic Dissection. All in all, these findings provide a new orientation for lncRNA Xist in kidney and cardiovascular diseases.
LncRNA Xist in Other Disease and Cells
A growing number of studies exhibited (Agrelo and Wutz, 2010; Shi et al., 2013; Cantone and Fisher, 2017) that lncRNA Xist participated in disease-associated processes, such as pulmonary disease, diabetic nephropathy, dermal diseases, and hereditary diseases, and mediated cellular functions of cells, such as somatic cell, B cells, and embryonic stem (ES) cells. In acute pneumonia, lncRNA Xist was robustly increased in serum of patients with acute-stage pneumonia and LPS (lipopolysaccharide)-induced WI-38 (normal human fibroblast WI-38 cell line) human lung fibroblasts cells, which shows it is involved in the progression of cell inflammatory response (Zhang et al., 2019g). Consequently, knockdown lncRNA Xist, which functions as a ceRNA to positively modulate TLR4 expression by sponging miR-370-3p, remarkably alleviates LPS-induced cell injury through regulating miR-370-3p/TLR4 axis to activate JAK/STAT and NF−κB signaling pathways (Zhang et al., 2019g).
In pulmonary fibrosis, Wang Y.C. et al. (2017) have revealed that lncRNA Xist regulates bleomycin (BLM)-induced extracellular matrix (ECM) and pulmonary fibrosis via modulation of miR-139/β-catenin axis. Primary graft dysfunction (PGD), which is a major cause of fatality post-lung transplantation, is a known acute lung injury (ALI). Li et al. (2020a) found that lncRNA Xist, which positively elevates the expression of IL-12A by acting as a ceRNA of miR-21, induces NET (neutrophil extracellular trap) formation and accelerates PGD after lung transplantation by activating the network of miR-21/IL-12A.
Diarrhea-predominant irritable bowel syndrome (IBS-D) is prevalent and has a high incidence rate in children. Zhang et al. (2020d) have demonstrated that lncRNA Xist, which is highly expressed in visceral hypersensitivity mice with IBS-D, modulates HT (5-hydroxytrytophan)-induced visceral hypersensitivity by epigenetic silencing of the SERT gene in mice with diarrhea-predominant IBS. In addition, Shen X.F. et al. (2020) have suggested that the silencing of lncRNA Xist, which is highly expressed in serum of patients, protects against sepsis-induced acute liver injury via inhibition of BRD4 expression. In diabetic nephropathy, Wang (2020) reported that lncRNA Xist silencing, which positively modulates PSMB8 expression via acting as a sponge for miR-485 in HMCs (human mesangial cells) treated with high glucose, alleviates inflammation and mesangial cell proliferation via interacting with miR-485/PSMB8.
Autoimmune disorders, such as Hashimoto’s thyroiditis, Sjögren’s Syndrome, systemic lupus erythematosus (SLE), and Grave’s disease, where 85–95% of patients are women, exhibited a strong female bias (Syrett et al., 2019, 2020). In recent years, lncRNA Xist (Syrett et al., 2017, 2019, 2020; Zhang et al., 2020c), which serves a vital function in SLE by RNA-seq data, promotes SLE development in NZB/WF1 mice with lupus-like disease. Taken together, these studies provide an important insight into how lncRNA Xist provides a therapeutic opportunity in female-biased autoimmune disorders.
Rett syndrome (RS), which is a debilitating neurological disorder affecting mostly girls, was caused by heterozygous mutations in the gene encoding the methyl-CpG–binding protein MeCP2 on the X chromosome (Sripathy et al., 2017). lncRNA Xist facilitates RS development through regulation of the bone morphogenetic protein (BMP)/TGF-β signaling pathway (Sripathy et al., 2017), and contributes to mouse brain development through reactivating MeCP2 expression (Adrianse et al., 2018).
Acute respiratory distress syndrome (ARDS), which is associated with diffuse alveolar injury and capillary endothelial damage, is a common clinical syndrome with high a mortality rate (Wang et al., 2019d). lncRNA Xist (Wang et al., 2019d), which acts as a ceRNA to negatively upregulate IRF2 (interferon regulatory factor 2) expression to sponge miR-204, significantly decreases the PaO2/FiO2 ratio and aggravates lipopolysaccharide-induced ARDS in mice by regulating the miR-204/IRF2 axis.
In the parthenogenetic development of pigs, silencing lncRNA Xist remarkedly increased the total blastocyst cell number but did not influence the rate of embryo cleavage and blastocyst formation compared with the control group (Chen et al., 2019e). This study suggested that lncRNA Xist may play a role in a new approach for improving the quality of porcine parthenogenetic embryos. lncRNA Xist (Zhang et al., 2019b) facilitates cells development in somatic cells by TALE-based designer transcriptional factor, and regulates embryonic stem cells’ fates (Chelmicki et al., 2014; An et al., 2020). lncRNA Xist promotes hair follicle regeneration in Dermal papilla cells via regulating miR-424/Shh axis to activate hedgehog signaling (Lin B.J. et al., 2020), and regulates HT cell proliferation and invasion in human trophoblast (HT) cells via miR-144/Titin axis by activating the downstream MAPK and MMPs pathway (Yu N.H. et al., 2017). In polycystic ovary syndrome (PCOS), lncRNA Xist is correlated with adverse pregnancy outcomes (Liu et al., 2020c). In addition, these signaling pathways, which include lncRNA Xist/miR-203-3p/ZFPM2 (Niu et al., 2020), lncRNA Xist/let-7c-5p/STAT3 (Wang et al., 2020d), and lncRNA Xist/miR-320/NOD2 (Xu X.H. et al., 2018), have been identified as a ceRNA regulatory network and participated in osteoblast development and ox-LDL (oxidative low-density lipoprotein)-induced endothelial cells injury.
A previous study reported that lncRNA Xist contributed to human skin fibroblasts by serving as a miRNA sponge. However, lncRNA Xist (Guo et al., 2018; Cao and Feng, 2019) regulates these processes containing skin fibroblasts proliferation, migration, and ECM (extracellular matrix) synthesis after thermal injury by sponging miRNAs (miR-29a and 29b-3p) to promote the expression of target genes (LIN28A and COL1A1). Additionally, lncRNA Xist/miR-181a/COL4A1 axis (Tian R. et al., 2020) is involved in the development and progression of keratoconus using transcriptome RNA-seq data assay. All in all, these results demonstrated that lncRNA Xist plays a pivotal function in non-cancer diseases.
Discussion and Perspectives
LncRNA Xist, which is conserved among eutherians (human, Rat, mouse, cow, dog, and elephant) but not non-eutherian vertebrates, is an important initiator of the process of XCI in eutherian mammals (Brockdorff et al., 1991; Duret et al., 2006; Galupa et al., 2020). lncRNA Xist is produced by Xist gene and is up-regulated from the Xi chromosome during the XCI process, and recruits protein complexes to reprogram chromosomes [such as H3K27me3 and H2AK119ub trimethylation (Postlmayr et al., 2020)]. In addition to its original XCI functions, numerous studies (Chaligne and Heard, 2014; Dey et al., 2014; Schmitz et al., 2016; Yang Z. et al., 2018; Cheng J.T. et al., 2019; Yan et al., 2019) have also indicated that lncRNA Xist is related to the pathogenic process of multiple diseases by regulating of cell migration, invasion, apoptosis, differentiation, proliferation, and drug resistance. Further investigation of lncRNA, which is considered to function as a miRNA or gene regulator, may aid in addressing disease etiology, such as lung cancer, breast cancer, glioblastoma, osteoarthritis, neuropathic pain, heart disease, and inflammation (Tables 1, 2). By summarizing current knowledge, we noticed that the regulatory network of lncRNA Xist in the majority of biological processions varies considerably. However, lncRNA Xist appears to regulate these processes primarily by interacting with miRNAs to positively facilitate downstream target gene expression (Figure 3). Further studies showed that the regulatory network of lncRNA Xist participated in various signaling pathways, such as TGF-beta signaling pathway, PIK3/AKT signaling pathway, Wnt/β-catenin signaling pathway, FOXO signaling pathway, NF-kB signaling pathway, mTOR signaling pathway, MAPK signaling pathway, Toll-like receptor signaling pathway, JAK-STAT signaling pathway, T cell receptor signaling pathway, and B cell receptor signaling pathway (Tables 1, 2). Although lncRNA Xist taking part in these signaling pathway functions has rarely been demonstrated, there is no reason to believe that the unexplored functions of lncRNA Xist will not be understanded in these ways. These mechanisms of lncRNA Xist action in diseases can indirectly and directly provide recommendations for future research, and more functions of lncRNA Xist can be confirmed.
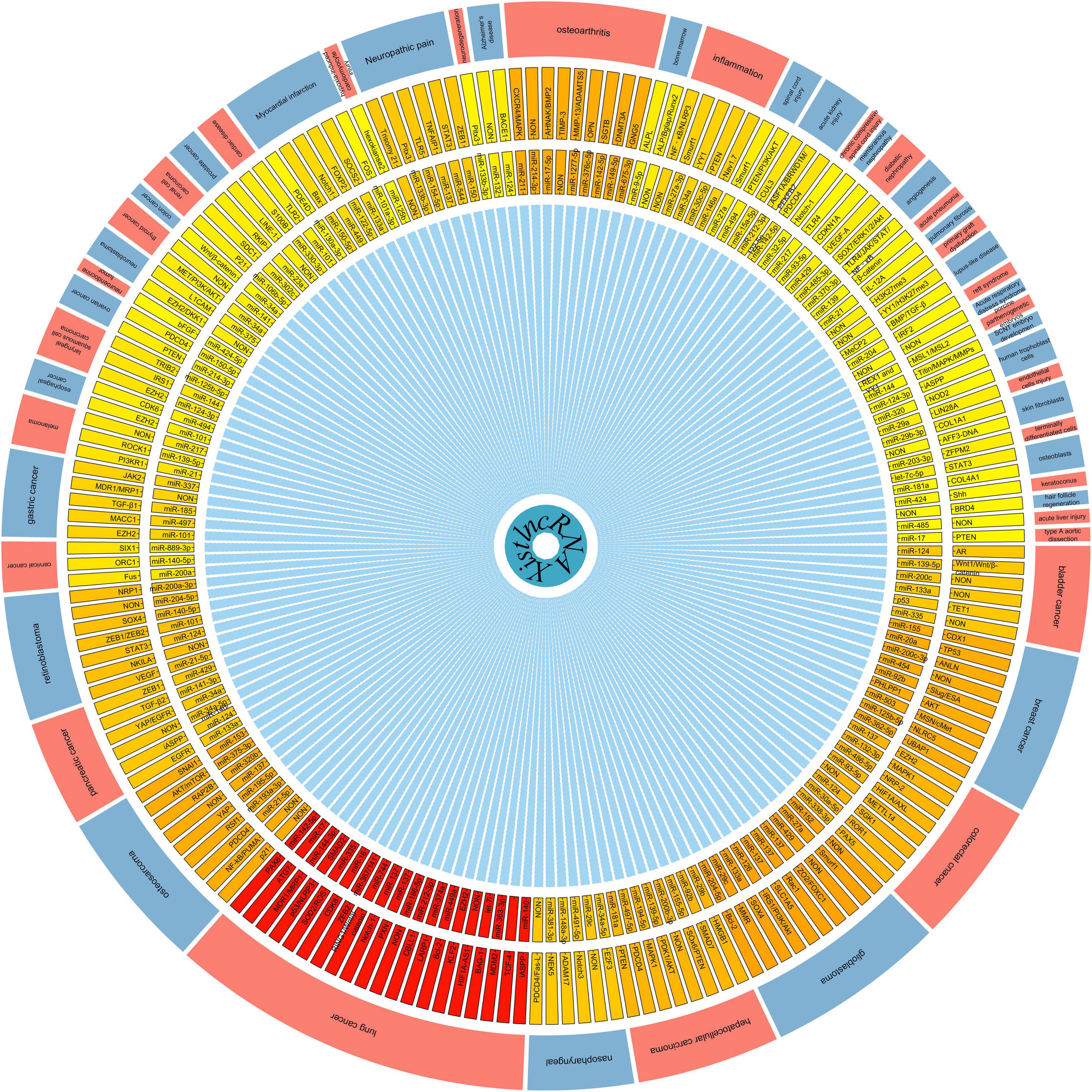
Figure 3. Overview of the regulatory network of lncRNA Xist involved in mammalian diseases and cells. NON, The downstream target Unclear.
In theory, the genes lncRNA Xist over-expresses and silences are numerous. But over-expression of lncRNA Xist, which is a 15–17 kb RNA polymerase II transcript that is both spliced and polyadenylated (Brockdorff, 2019), is different when using plasmids. By contrast, lncRNA Xist may be inhibited using small molecule inhibitors that block specific binding sites (Matoba et al., 2011). Based on the above reasons, understanding of the function of lncRNA Xist is in its infancy for various diseases and cells. With the developing genome editing technology (Du and Qi, 2016; Chen et al., 2019b; Yi and Li, 2020), CRISPR/Cas9 system is emerging as a powerful tool for sequence-specific control of lncRNA Xist expression in mammalian cells. Recently, numerous studies (Yue and Ogawa, 2018; Colognori et al., 2019; Waśko et al., 2019; Deng et al., 2020) have indicated that CRISPR/Cas9 system is useful for studying lncRNA Xist function and related ceRNA regulatory networks. By combining other future technologies, the function and mechanism of lncRNA Xist will certainly be found. Investigation of lncRNA Xist in virous cells may uncover numerous novel therapeutic approaches for disease treatment in the future. At the same time, it might result in a better understanding of how lncRNA Xist contributes to the XCI and diseases in mammals, potentially opening new avenues for research and therapeutic manipulation of these diseases.
Author Contributions
DZ, WW, and LZ: manuscript design. WW, JM, and XW: literature collection and summary. WW and LZ: drafting of the manuscript. WW, LM, and CL: figure drawing. XQ, WW, DZ, and LZ: revising of the manuscript. All authors have read and approved the final submitted manuscript.
Funding
This work was supported by grants from the Hunan Provincial Innovation Foundation for Postgraduate (No. 434517123Q), the Natural Science Foundation of Hunan Province (No. 2020JJ5656), and the National Natural Science Foundation of China (31870855).
Conflict of Interest
The authors declare that the research was conducted in the absence of any commercial or financial relationships that could be construed as a potential conflict of interest.
Acknowledgments
We would like to thank all the members of the research groups of LZ. We apologize to colleagues whose work we were unable to cite or discuses due to space limitations.
References
Adrianse, R. L., Smith, K., Gatbonton-Schwager, T., Sripathy, S. P., Lao, U., Foss, E. J., et al. (2018). Perturbed maintenance of transcriptional repression on the inactive X-chromosome in the mouse brain after Xist deletion. Epigenet. Chromatin. 11, 1–13.
An, C., Feng, G., Zhang, J., Cao, S., Wang, Y., Wang, N., et al. (2020). Overcoming autocrine FGF signaling-induced heterogeneity in naive human ESCs enables modeling of random X chromosome inactivation. Cell Stem Cell 27, 482.e4–497.e4.
Augui, S., Nora, E. P., and Heard, E. (2011). Regulation of X-chromosome inactivation by the X-inactivation centre. Nat. Rev. Genet. 12, 429–442. doi: 10.1038/nrg2987
Balmant, N. V., Reis, R. D., Santos, M. D., Maschietto, M., and de Camargo, B. (2019). Incidence and mortality of bone cancer among children, adolescents and young adults of Brazil. Clinics 74:e858.
Bar, S., Seaton, L. R., Weissbein, U., Eldar-Geva, T., and Benvenisty, N. (2019). Global characterization of X chromosome inactivation in human pluripotent stem cells. Cell Rep. 27, 20.e3–29.e3.
Batash, R., Asna, N., Schaffer, P., Francis, N., and Schaffer, M. (2017). Glioblastoma multiforme, diagnosis and treatment; recent literature review. Curr. Med. Chem. 24, 3002–3009.
Bianchini, G., Balko, J. M., Mayer, I. A., Sanders, M. E., and Gianni, L. (2016). Triple-negative breast cancer: challenges and opportunities of a heterogeneous disease. Nat. Rev. Clin. Oncol. 13, 674–690. doi: 10.1038/nrclinonc.2016.66
Bone, J. R., and Kuroda, M. I. (1996). Dosage compensation regulatory proteins and the evolution of sex chromosomes in Drosophila. Genetics 144, 705–713. doi: 10.1093/genetics/144.2.705
Bradford, C. R., Ferlito, A., Devaney, K. O., Makitie, A. A., and Rinaldo, A. (2020). Prognostic factors in laryngeal squamous cell carcinoma. Laryngosc. Investig. Otolaryngol. 5, 74–81.
Bray, F., Ferlay, J., Soerjomataram, I., Siegel, R. L., Torre, L. A., and Jemal, A. (2018). Global cancer statistics 2018: GLOBOCAN estimates of incidence and mortality worldwide for 36 cancers in 185 countries. Cancer J. Clin. 68, 394–424. doi: 10.3322/caac.21492
Brockdorff, N. (2019). Localized accumulation of Xist RNA in X chromosome inactivation. Open Biol. 9, 19213–19224.
Brockdorff, N., Ashworth, A., Kay, G. F., Cooper, P., Smith, S., McCabe, V. M., et al. (1991). Conservation of position and exclusive expression of mouse Xist from the inactive X chromosome. Nature 351, 329–331. doi: 10.1038/351329a0
Brown, C. J., Hendrich, B. D., Rupert, J. L., Lafreniere, R. G., Xing, Y., Lawrence, J., et al. (1992). The human XIST gene: analysis of a 17 kb inactive X-specific RNA that contains conserved repeats and is highly localized within the nucleus. Cell 71, 527–542. doi: 10.1016/0092-8674(92)90520-m
Cantone, I., and Fisher, A. G. (2017). Human X chromosome inactivation and reactivation: implications for cell reprogramming and disease. Philos. Trans. R. Soc. B 372:20160358. doi: 10.1098/rstb.2016.0358
Cao, H. F., Wahlestedt, C., and Kapranov, P. (2018). Strategies to annotate and characterize long noncoding RNAs: advantages and pitfalls. Trends Genet. 34, 704–721. doi: 10.1016/j.tig.2018.06.002
Cao, W., and Feng, Y. P. (2019). LncRNA XIST promotes extracellular matrix synthesis, proliferation and migration by targeting miR-29b-3p/COL1A1 in human skin fibroblasts after thermal injury. Biol. Res. 52, 52–63.
Cassoux, N., Lumbroso, L., Levy-Gabriel, C., Aerts, I., Doz, F., and Desjardins, L. (2017). Retinoblastoma: update on current management. Asia Pac. J. Ophthalmol. 6, 290–295.
Chaligne, R., and Heard, E. (2014). X-chromosome inactivation in development and cancer. FEBS Lett. 588, 2514–2522. doi: 10.1016/j.febslet.2014.06.023
Chaligne, R., Popova, T., Mendoza-Parra, M. A., Saleem, M. A. M., Gentien, D., Ban, K., et al. (2015). The inactive X chromosome is epigenetically unstable and transcriptionally labile in breast cancer. Genome Res. 25, 488–503. doi: 10.1101/gr.185926.114
Chanda, K., and Mukhopadhyay, D. (2020). LncRNA Xist, X- chromosome Instability and Alzheimer’s disease. Curr. Alzheimer Res. 17, 499–507. doi: 10.2174/1567205017666200807185624
Chang, S. Z., Chen, B. H., Wang, X. Y., Wu, K. Q., and Sun, Y. Q. (2017). Long non-coding RNA XIST regulates PTEN expression by sponging miR-181a and promotes hepatocellular carcinoma progression. BMC Cancer 17:248. doi: 10.1186/s12885-017-3216-6
Chelmicki, T., Dundar, F., Turley, M. J., Khanam, T., Aktas, T., Ramirez, F., et al. (2014). MOF-associated complexes ensure stem cell identity and Xist repression. eLife 3:e02024.
Chen, C. K., Blanco, M., Jackson, C., Aznauryan, E., Ollikainen, N., Surka, C., et al. (2016). Xist recruits the X chromosome to the nuclear lamina to enable chromosome-wide silencing. Science 354, 468–472. doi: 10.1126/science.aae0047
Chen, D., Chen, T., Guo, Y., Wang, C., Dong, L., and Lu, C. (2020). Platycodin D (PD) regulates LncRNA-XIST/miR-335 axis to slow down bladder cancer progression in vitro and in vivo. Exp. Cell Res. 20:112281. doi: 10.1016/j.yexcr.2020.112281
Chen, D. L., Chen, L. Z., Lu, Y. X., Zhang, D. S., Zeng, Z. L., Pan, Z. Z., et al. (2017). Long noncoding RNA XIST expedites metastasis and modulates epithelial-mesenchymal transition in colorectal cancer. Cell Death Dis. 8:e3011. doi: 10.1038/cddis.2017.421
Chen, D. L., Ju, H. Q., Lu, Y. X., Chen, L. Z., Zeng, Z. L., Zhang, D. S., et al. (2016). Long non-coding RNA XIST regulates gastric cancer progression by acting as a molecular sponge of miR-101 to modulate EZH2 expression. J. Exp. Clin. Cancer Res. 35, 142–155.
Chen, G., Xu, L. L., Huang, Y. F., Wang, Q., Wang, B. H., Yu, Z. H., et al. (2018). Prenatal exposure to perfluorooctane sulfonate impairs placental angiogenesis and induces aberrant expression of LncRNA xist. Biomed. Environ. Sci. 31, 843–847.
Chen, H. W., Yang, S. D., and Shao, R. Y. (2019a). Long non-coding XIST raises methylation of TIMP-3 promoter to regulate collagen degradation in osteoarthritic chondrocytes after tibial plateau fracture. Arthrit. Res. Ther. 21, 271–284.
Chen, L. L. (2016). Linking long noncoding RNA localization and function. Trends Biochem. Sci. 41, 761–772. doi: 10.1016/j.tibs.2016.07.003
Chen, W., Zhang, G. Q., Li, J., Zhang, X., Huang, S. L., Xiang, S. L., et al. (2019b). CRISPRlnc: a manually curated database of validated sgRNAs for lncRNAs. Nucleic Acids Res. 47, D63–D68.
Chen, W., Zheng, R., Baade, P. D., Zhang, S., Zeng, H., Bray, F., et al. (2016). Cancer statistics in China, 2015. Cancer J. Clin. 66, 115–132. doi: 10.3322/caac.21338
Chen, X., Xiong, D., Ye, L., Wang, K., Huang, L., Mei, S., et al. (2019c). Up-regulated lncRNA XIST contributes to progression of cervical cancer via regulating miR-140-5p and ORC1. Cancer Cell Int. 19, 45–64.
Chen, X., Yang, L., Ge, D. W., Wang, W. W., Yin, Z. W., Yan, J. W., et al. (2019d). Long non-coding RNA XIST promotes osteoporosis through inhibiting bone marrow mesenchymal stem cell differentiation. Exp. Ther. Med. 17, 803–811.
Chen, X. Y., Zhu, Z. W., Yu, F. X., Huang, J., Jia, R. X., and Pan, J. Z. (2019e). Effect of shRNA-mediated Xist knockdown on the quality of porcine parthenogenetic embryos. Dev. Dyn. 248, 140–148. doi: 10.1002/dvdy.24660
Chen, Y., Liu, X., Chen, L., Chen, W., Zhang, Y., Chen, J., et al. (2018). The long noncoding RNA XIST protects cardiomyocyte hypertrophy by targeting miR-330-3p. Biochem. Biophys. Res. Commun. 505, 807–815. doi: 10.1016/j.bbrc.2018.09.135
Chen, Z., and Zhang, Y. (2020). Maternal H3K27me3-dependent autosomal and X chromosome imprinting. Nat. Rev. Genet. 21, 555–571. doi: 10.1038/s41576-020-0245-9
Chen, Z., Hu, X., Wu, Y., Cong, L., He, X., Lu, J., et al. (2019f). Long non-coding RNA XIST promotes the development of esophageal cancer by sponging miR-494 to regulate CDK6 expression. Biomed. Pharmacother. 109, 2228–2236. doi: 10.1016/j.biopha.2018.11.049
Cheng, J. T., Wang, L. Z., Wang, H., Tang, F. R., Cai, W. Q., Sethi, G., et al. (2019). Insights into biological role of LncRNAs in epithelial-mesenchymal transition. Cells Basel 8, 1178–1203. doi: 10.3390/cells8101178
Cheng, Q., and Wang, L. (2020). LncRNA XIST serves as a ceRNA to regulate the expression of ASF1A, BRWD1M, and PFKFB2 in kidney transplant acute kidney injury via sponging hsa-miR-212-3p and hsa-miR-122-5p. Cell Cycle 19, 290–299. doi: 10.1080/15384101.2019.1707454
Cheng, Q., Xu, X. Y., Jiang, H., Xu, L. E., and Li, Q. (2018). Knockdown of long non-coding RNA XIST suppresses nasopharyngeal carcinoma progression by activating miR-491-5p. J. Cell. Biochem. 119, 3936–3944. doi: 10.1002/jcb.26535
Cheng, X., Xu, J., Yu, Z., Xu, J., and Long, H. (2020). LncRNA xist contributes to endogenous neurological repair after chronic compressive spinal cord injury by promoting angiogenesis through the miR-32-5p/Notch-1 axis. J. Cell Sci. 8, 744–758.
Cheng, Y., Chang, Q., Zheng, B., Xu, J., Li, H., and Wang, R. (2019). LncRNA XIST promotes the epithelial to mesenchymal transition of retinoblastoma via sponging miR-101. Eur. J. Pharmacol. 843, 210–216. doi: 10.1016/j.ejphar.2018.11.028
Cheng, Z. H., Li, Z. S. N., Ma, K., Li, X. Y., Tian, N., Duan, J. Y., et al. (2017). Long Non-coding RNA XIST Promotes Glioma Tumorigenicity and Angiogenesis by Acting as a Molecular Sponge of miR-429. J. Cancer 8, 4106–4116. doi: 10.7150/jca.21024
Cheng, Z. H., Luo, C., and Guo, Z. L. (2020). LncRNA-XIST/microRNA-126 sponge mediates cell proliferation and glucose metabolism through the IRS1/PI3K/Akt pathway in glioma. J. Cell. Biochem. 121, 2170–2183. doi: 10.1002/jcb.29440
Chigi, Y., Sasaki, H., and Sado, T. (2017). The 5 ‘ region of Xist RNA has the potential to associate with chromatin through the A-repeat. RNA 23, 1894–1901. doi: 10.1261/rna.062158.117
Chu, C., Zhang, Q. F. C., da Rocha, S. T., Flynn, R. A., Bharadwaj, M., Calabrese, J. M., et al. (2015). Systematic discovery of Xist RNA binding proteins. Cell 161, 404–416. doi: 10.1016/j.cell.2015.03.025
Chua, M. L. K., Wee, J. T. S., Hui, E. P., and Chan, A. T. C. (2016). Nasopharyngeal carcinoma. Lancet 387, 1012–1024.
Colognori, D., Sunwoo, H., Kriz, A. J., Wang, C. Y., and Lee, J. T. (2019). Xist deletional analysis reveals an interdependency between xist RNA and polycomb complexes for spreading along the inactive X. Mol. Cell 74, 101–117. doi: 10.1016/j.molcel.2019.01.015
Colognori, D., Sunwoo, H., Wang, D., Wang, C. Y., and Lee, J. T. (2020). Xist Repeats A and B account for two distinct phases of X inactivation establishment. Dev. Cell 54, 21.e5–32.e5.
Colpaert, R. M. W., and Calore, M. (2019). MicroRNAs in cardiac diseases. Cells Basel 8, 737–759. doi: 10.3390/cells8070737
Connerty, P., Lock, R. B., and de Bock, C. E. (2020). Long non-coding RNAs: major regulators of cell stress in cancer. Front. Oncol. 10:285. doi: 10.3389/fonc.2020.00285
Cui, C. L., Li, Y. N., Cui, X. Y., and Wu, X. (2020). lncRNA XIST promotes the progression of laryngeal squamous cell carcinoma by sponging miR144 to regulate IRS1 expression. Oncol. Rep. 43, 525–535.
Czerminski, J. T., and Lawrence, J. B. (2020). Silencing trisomy 21 with XIST in neural stem cells promotes neuronal differentiation. Dev. Cell 52, 294–308. doi: 10.1016/j.devcel.2019.12.015
da Rocha, S. T., and Heard, E. (2017). Novel players in X inactivation: insights into Xist-mediated gene silencing and chromosome conformation. Nat. Struct. Mol. Biol. 24, 197–204. doi: 10.1038/nsmb.3370
Debrand, E., Heard, E., and Avner, P. (1998). Cloning and localization of the murine Xpct gene: evidence for complex rearrangements during the evolution of the region around the Xist gene. Genomics 48, 296–303. doi: 10.1006/geno.1997.5173
Deng, C., Hu, X., Wu, K., Tan, J., and Yang, C. (2019). Long non-coding RNA XIST predicting advanced clinical parameters in cancer: a Meta-Analysis and case series study in a single institution. Oncol. Lett. 18, 2192–2202.
Deng, M., Liu, Z., Chen, B., Cai, Y., Wan, Y., and Wang, F. (2020). Locus-specific regulation of xist expression using the CRISPR-Cas9-based system. DNA Cell Biol. 39, 572–578. doi: 10.1089/dna.2019.4945
Deng, X., Berletch, J. B., Nguyen, D. K., and Disteche, C. M. (2014). X chromosome regulation: diverse patterns in development, tissues and disease. Nat. Rev. Genet. 15, 367–378. doi: 10.1038/nrg3687
Derrien, T., Johnson, R., Bussotti, G., Tanzer, A., Djebali, S., Tilgner, H., et al. (2012). The GENCODE v7 catalog of human long noncoding RNAs: analysis of their gene structure, evolution, and expression. Genome Res. 22, 1775–1789. doi: 10.1101/gr.132159.111
Devaux, Y., Zangrando, J., Schroen, B., Creemers, E. E., Pedrazzini, T., Chang, C. P., et al. (2015). Long noncoding RNAs in cardiac development and ageing. Nat. Rev. Cardiol. 12, 415–425. doi: 10.1038/nrcardio.2015.55
Dey, B. K., Mueller, A. C., and Dutta, A. (2014). Long non-coding RNAs as emerging regulators of differentiation, development, and disease. Transcription 5:e944014. doi: 10.4161/21541272.2014.944014
Dinescu, S., Ignat, S., Lazar, A. D., Constantin, C., Neagu, M., and Costache, M. (2019). Epitranscriptomic signatures in lncRNAs and their possible roles in cancer. Genes 10, 52–79. doi: 10.3390/genes10010052
Disteche, C. M. (2012). Dosage compensation of the sex chromosomes. Annu. Rev. Genet. 46, 537–560. doi: 10.1146/annurev-genet-110711-155454
Disteche, C. M. (2016). Dosage compensation of the sex chromosomes and autosomes. Semin. Cell Dev. Biol. 56, 9–18. doi: 10.1016/j.semcdb.2016.04.013
Dong, Y., Wan, G., Peng, G., Yan, P., Qian, C., and Li, F. (2020). Long non-coding RNA XIST regulates hyperglycemia-associated apoptosis and migration in human retinal pigment epithelial cells. Biomed. Pharmacother. 125:109959. doi: 10.1016/j.biopha.2020.109959
Donohoe, M. E., Silva, S. S., Pinter, S. F., Xu, N., and Lee, J. T. (2009). The pluripotency factor Oct4 interacts with Ctcf and also controls X-chromosome pairing and counting. Nature 460, 128–U147.
Dossin, F., Pinheiro, I., Zylicz, J. J., Roensch, J., Collombet, S., and Saux, A. Le, et al. (2020). SPEN integrates transcriptional and epigenetic control of X-inactivation. Nature 578, 455–460. doi: 10.1038/s41586-020-1974-9
Du, D., and Qi, L. S. (2016). CRISPR technology for genome activation and repression in mammalian cells. Cold Spring Harb. Protoc. 2016:rot090175.
Du, P., Zhao, H. T., Peng, R. J., Liu, Q., Yuan, J., Peng, G., et al. (2017). LncRNA-XIST interacts with miR-29c to modulate the chemoresistance of glioma cell to TMZ through DNA mismatch repair pathway. Biosci. Rep. 37:BSR20170696.
Du, Y., Gao, G. Q., Li, J. X., Suo, S., Liu, S., Sun, Y., et al. (2020). Silencing of long noncoding RNA XIST attenuated Alzheimer’s disease-related BACE1 alteration through miR-124. Cell Biol. Int. 44, 630–636. doi: 10.1002/cbin.11263
Du, Y., Weng, X. D., Wang, L., Liu, X. H., Zhu, H. C., Guo, J., et al. (2017). LncRNA XIST acts as a tumor suppressor in prostate cancer through sponging miR-23a to modulate RKIP expression. Oncotarget 8, 94358–94370. doi: 10.18632/oncotarget.21719
Duret, L., Chureau, C., Samain, S., Weissenbach, J., and Avner, P. (2006). The Xist RNA gene evolved in eutherians by pseudogenization of a protein-coding gene. Science 312, 1653–1655. doi: 10.1126/science.1126316
Eberlin, K. R. (2019). Invited commentary targeted muscle reinnervation: a significant advance in the prevention and treatment of post-amputation neuropathic pain. J. Am. Coll. Surgeons 228, 226–227. doi: 10.1016/j.jamcollsurg.2018.12.008
Fan, J. L., Zhu, T. T., Xue, Z. Y., Ren, W. Q., Guo, J. Q., Zhao, H. Y., et al. (2020). lncRNA-XIST protects the hypoxia-induced cardiomyocyte injury through regulating the miR-125b-hexokianse 2 axis. In Vitro Cell Dev. An. 56, 349–357. doi: 10.1007/s11626-020-00459-0
Fang, J., Sun, C. C., and Gong, C. (2016). Long noncoding RNA XIST acts as an oncogene in non-small cell lung cancer by epigenetically repressing KLF2 expression. Biochem. Biophys. Res. Commun. 478, 811–817. doi: 10.1016/j.bbrc.2016.08.030
Feng, Y. M., Wan, P. B., and Yin, L. L. (2020). Long noncoding RNA X-inactive specific transcript (XIST) promotes osteogenic differentiation of periodontal ligament stem cells by sponging MicroRNA-214-3p. Med. Sci. Monitor 26, e918931–e918932.
Ferrari, F., Alekseyenko, A. A., Park, P. J., and Kuroda, M. I. (2014). Transcriptional control of a whole chromosome: emerging models for dosage compensation. Nat. Struct. Mol. Biol. 21, 118–125. doi: 10.1038/nsmb.2763
Finestra, T. R., and Gribnau, J. (2017). X chromosome inactivation: silencing, topology and reactivation. Curr. Opin. Cell Biol. 46, 54–61. doi: 10.1016/j.ceb.2017.01.007
Fornasari, D. (2017). Pharmacotherapy for neuropathic pain: a review. Pain Ther. 6, 25–33. doi: 10.1007/s40122-017-0091-4
Fu, X. D. (2014). Non-coding RNA: a new frontier in regulatory biology. Natl. Sci. Rev. 1, 190–204. doi: 10.1093/nsr/nwu008
Furlan, G., and Rougeulle, C. (2016). Function and evolution of the long noncoding RNA circuitry orchestrating X-chromosome inactivation in mammals. Wires RNA 7, 702–722. doi: 10.1002/wrna.1359
Galupa, R., Nora, E. P., Worsley-Hunt, R., Picard, C., Gard, C., van Bemmel, J. G., et al. (2020). A conserved noncoding locus regulates random monoallelic xist expression across a topological boundary. Mol. Cell 77, 352–367. doi: 10.1016/j.molcel.2019.10.030
Gao, W., Gao, J., Chen, L., Ren, Y., and Ma, J. (2019). Targeting XIST induced apoptosis of human osteosarcoma cells by activation of NF-kB/PUMA signal. Bioengineered 10, 261–270. doi: 10.1080/21655979.2019.1631104
Gayen, S., Maclary, E., Buttigieg, E., Hinten, M., and Kalantry, S. (2015). A primary role for the tsix lncRNA in maintaining random X-chromosome inactivation. Cell Rep. 11, 1251–1265. doi: 10.1016/j.celrep.2015.04.039
Gendrel, A. V., and Heard, E. (2014). Noncoding RNAs and epigenetic mechanisms during X-chromosome inactivation. Annu. Rev. Cell Dev. Biol. 30, 561–580. doi: 10.1146/annurev-cellbio-101512-122415
Ghaderian, S. M. H., Alipour Parsa, S., Ghaedi, H., Jafari, H., Sun, P., and Wu, Y. (2020). miR-142-5p protects against osteoarthritis through competing with lncRNA XIST. Arch. Physiol. Biochem. 22:e3158.
Giorgetti, L., Lajoie, B. R., Carter, A. C., Attia, M., Zhan, Y., Xu, J., et al. (2016). Structural organization of the inactive X chromosome in the mouse. Nature 535, 575–579. doi: 10.1038/nature18589
Gontan, C., Jonkers, I., and Gribnau, J. (2011). “Long noncoding RNAs and X chromosome inactivation,” in Long Non-Coding RNAs, ed. D. Ugarkovic (Berlin: Springer), 43–64. doi: 10.1007/978-3-642-16502-3_3
Gontan, C., Achame, E. M., Demmers, J., Barakat, T. S., Rentmeester, E., van, I. W., et al. (2012). RNF12 initiates X-chromosome inactivation by targeting REX1 for degradation. Nature 485, 386–390. doi: 10.1038/nature11070
Grant, J., Mahadevaiah, S. K., Khil, P., Sangrithi, M. N., Royo, H., Duckworth, J., et al. (2012). Rsx is a metatherian RNA with Xist-like properties in X-chromosome inactivation. Nature 487, 254–258. doi: 10.1038/nature11171
Greco, S., Salgado Somoza, A., Devaux, Y., and Martelli, F. (2018). Long noncoding RNAs and cardiac disease. Antioxid. Redox Signal. 29, 880–901.
Gu, S. X., Xie, R., Liu, X. D., Shou, J. J., Gu, W. T., and Che, X. M. (2017). Long Coding RNA XIST Contributes to Neuronal Apoptosis through the Downregulation of AKT phosphorylation and is negatively regulated by miR-494 in rat spinal cord injury. Int. J. Mol. Sci. 18, 732–749. doi: 10.3390/ijms18040732
Guo, H., Callaway, J. B., and Ting, J. P. (2015). Inflammasomes: mechanism of action, role in disease, and therapeutics. Nat. Med. 21, 677–687. doi: 10.1038/nm.3893
Guo, L., Huang, X., Liang, P. F., Zhang, P. H., Zhang, M. H., Ren, L. C., et al. (2018). Role of XIST/miR-29a/LIN28A pathway in denatured dermis and human skin fibroblasts (HSFs) after thermal injury. J. Cell. Biochem. 119, 1463–1474. doi: 10.1002/jcb.26307
Hai, B. (2020). LncRNA XIST promotes growth of human chordoma cells by regulating miR-124-3p/iASPP pathway. J. Cell. Physiol. 13, 4755–4765. doi: 10.2147/ott.s252195
Han, J., and Shen, X. (2020). Long noncoding RNAs in osteosarcoma via various signaling pathways. J. Clin. Lab. Anal. 34:e23317.
Han, Q., Li, L., Liang, H., Li, Y., Xie, J., and Wang, Z. (2017). Downregulation of lncRNA X inactive specific transcript (XIST) suppresses cell proliferation and enhances radiosensitivity by upregulating mir-29c in nasopharyngeal carcinoma cells. Med. Sci. Monit. 23, 4798–4807. doi: 10.12659/msm.905370
Harper, M. I., Fosten, M., and Monk, M. (1982). Preferential paternal X inactivation in extraembryonic tissues of early mouse embryos. J. Embryol. Exp. Morphol. 67, 127–135. doi: 10.1242/dev.67.1.127
Hasegawa, Y., Brockdorff, N., Kawano, S., Tsutui, K., Tsutui, K., and Nakagawa, S. (2010). The matrix protein hnRNP U is required for chromosomal localization of Xist RNA. Dev. Cell 19, 469–476. doi: 10.1016/j.devcel.2010.08.006
He, X. L., Yang, L., Huang, R. Q., Lin, L. J., Shen, Y. J., Cheng, L. M., et al. (2020). Activation of CB2R with AM1241 ameliorates neurodegeneration via the Xist/miR-133b-3p/Pitx3 axis. J. Cell. Physiol. 235, 6032–6042. doi: 10.1002/jcp.29530
Hu, B., Shi, G., Li, Q., Li, W., and Zhou, H. (2019). Long noncoding RNA XIST participates in bladder cancer by downregulating p53 via binding to TET1. J. Cell. Biochem. 120, 6330–6338. doi: 10.1002/jcb.27920
Hu, C., Bai, X., Liu, C., and Hu, Z. (2019). Long noncoding RNA XIST participates hypoxia-induced angiogenesis in human brain microvascular endothelial cells through regulating miR-485/SOX7 axis. Am. J. Transl. Res. 11, 6487–6497.
Hu, C., Liu, S., Han, M., Wang, Y., and Xu, C. (2018). Knockdown of lncRNA XIST inhibits retinoblastoma progression by modulating the miR-124/STAT3 axis. Biomed. Pharmacother. 107, 547–554. doi: 10.1016/j.biopha.2018.08.020
Hu, W. N., Duan, Z. Y., Wang, Q., and Zhou, D. H. (2019). The suppression of ox-LDL-induced inflammatory response and apoptosis of HUVEC by lncRNA XIAT knockdown via regulating miR-30c-5p/PTEN axis. Eur. Rev. Med. Pharmacol. 23, 7628–7638.
Hu, Y., Deng, C., Zhang, H., Zhang, J., Peng, B., and Hu, C. (2017). Long non-coding RNA XIST promotes cell growth and metastasis through regulating miR-139-5p mediated Wnt/beta-catenin signaling pathway in bladder cancer. Oncotarget 8, 94554–94568. doi: 10.18632/oncotarget.21791
Huang, Y. S., Chang, C. C., Lee, S. S., Jou, Y. S., and Shih, H. M. (2016). Xist reduction in breast cancer upregulates AKT phosphorylation via HDAC3-mediated repression of PHLPP1 expression. Oncotarget 7, 43256–43266. doi: 10.18632/oncotarget.9673
Hwang, J. Y., Oh, J. N., Park, C. H., Lee, D. K., and Lee, C. K. (2015). Dosage compensation of X-chromosome inactivation center-linked genes in porcine preimplantation embryos: non-chromosome-wide initiation of X-chromosome inactivation in blastocysts. Mech. Dev. 138, 246–255. doi: 10.1016/j.mod.2015.10.005
Jeon, Y., and Lee, J. T. (2011). YY1 tethers Xist RNA to the inactive X nucleation center. Cell 146, 119–133. doi: 10.1016/j.cell.2011.06.026
Jiang, H. J., Zhang, H. Z., Hu, X. G., and Li, W. B. (2018). Knockdown of long non-coding RNA XIST inhibits cell viability and invasion by regulating miR-137/PXN axis in non-small cell lung cancer. Int. J. Biol. Macromol. 111, 623–631. doi: 10.1016/j.ijbiomac.2018.01.022
Jiang, J., Jing, Y., Cost, G. J., Chiang, J. C., Kolpa, H. J., Cotton, A. M., et al. (2013). Translating dosage compensation to trisomy 21. Nature 500, 296–300.
Jiang, Q., Xing, W., Cheng, J., and Yu, Y. (2020). Knockdown of lncRNA XIST suppresses cell tumorigenicity in human non-small cell lung cancer by regulating miR-142-5p/PAX6 axis. OncoTargets Ther. 13, 4919–4929. doi: 10.2147/ott.s238808
Jiang, W., Lv, Y. L., and Wang, S. Y. (2018). Prediction of non-coding RNAs as drug targets. Adv. Exp. Med. Biol. 1094, 109–115. doi: 10.1007/978-981-13-0719-5_11
Jin, H., Du, X. J., Zhao, Y., and Xia, D. L. (2018). XIST/miR-544 axis induces neuropathic pain by activating STAT3 in a rat model. J. Cell. Physiol. 233, 5847–5855. doi: 10.1002/jcp.26376
Jin, L. W., Pan, M., Ye, H. Y., Zheng, Y., Chen, Y., Huang, W. W., et al. (2019). Down-regulation of the long non-coding RNA XIST ameliorates podocyte apoptosis in membranous nephropathy via the miR-217-TLR4 pathway. Exp. Physiol. 104, 220–230.
Kawakami, T., Zhang, C., Taniguchi, T., Kim, C. J., Okada, Y., Sugihara, H., et al. (2004). Characterization of loss-of-inactive X in Klinefelter syndrome and female-derived cancer cells. Oncogene 23, 6163–6169. doi: 10.1038/sj.onc.1207808
Khamlichi, A. A., and Feil, R. (2018). Parallels between mammalian mechanisms of monoallelic gene expression. Trends Genet. 34, 954–971. doi: 10.1016/j.tig.2018.08.005
Kim, M. M., Umemura, Y., and Leung, D. (2018). Bevacizumab and glioblastoma: past, present, and future directions. Cancer J. 24, 180–186. doi: 10.1097/ppo.0000000000000326
Kolpa, H. J., Fackelmayer, F. O., and Lawrence, J. B. (2016). SAF-A requirement in anchoring XIST RNA to chromatin varies in transformed and primary cells. Dev. Cell 39, 9–10. doi: 10.1016/j.devcel.2016.09.021
Kong, Q. L., Zhang, S. Q., Liang, C. Q., Zhang, Y., Kong, Q. C., Chen, S. X., et al. (2018). LncRNA XIST functions as a molecular sponge of miR-194-5p to regulate MAPK1 expression in hepatocellular carcinoma cell. J. Cell. Biochem. 119, 4458–4468. doi: 10.1002/jcb.26540
Kung, J. T., Kesner, B., An, J. Y., Ahn, J. Y., Cifuentes-Rojas, C., Colognori, D., et al. (2015). Locus-specific targeting to the X chromosome revealed by the RNA interactome of CTCF. Mol. Cell 57, 361–375. doi: 10.1016/j.molcel.2014.12.006
Kung, J. T. Y., Colognori, D., and Lee, J. T. (2013). Long noncoding RNAs: past, present, and future. Genetics 193, 651–669. doi: 10.1534/genetics.112.146704
Laner, T., Schulz, W. A., Engers, R., Muller, M., and Florl, A. R. (2005). Hypomethylation of the XIST gene promoter in prostate cancer. Oncol. Res. 15, 257–264. doi: 10.3727/096504005776404607
Larsson, J., and Meller, V. H. (2006). Dosage compensation, the origin and the afterlife of sex chromosomes. Chromosome Res. 14, 417–431. doi: 10.1007/s10577-006-1064-3
Lee, H. J., Gopalappa, R., Sunwoo, H., Choi, S. W., Ramakrishna, S., Lee, J. T., et al. (2019). En bloc and segmental deletions of human XIST reveal X chromosome inactivation-involving RNA elements. Nucleic Acids Res. 47, 3875–3887.
Lee, J. T., Bartolomei, M. S., and Inactivation, X. - (2013). Imprinting, and long noncoding RNAs in health and disease. Cell 152, 1308–1323. doi: 10.1016/j.cell.2013.02.016
Li, C., Wan, L., Liu, Z., Xu, G., Wang, S., Su, Z., et al. (2018). Long non-coding RNA XIST promotes TGF-beta-induced epithelial-mesenchymal transition by regulating miR-367/141-ZEB2 axis in non-small-cell lung cancer. Cancer Lett. 418, 185–195. doi: 10.1016/j.canlet.2018.01.036
Li, G. L., Wu, Y. X., Li, Y. M., and Li, J. (2017). High expression of long non-coding RNA XIST in osteosarcoma is associated with cell proliferation and poor prognosis. Eur. Rev. Med. Pharmacol. 21, 2829–2834.
Li, H., Cui, J., Xu, B., He, S., Yang, H., and Liu, L. (2019). Long non-coding RNA XIST serves an oncogenic role in osteosarcoma by sponging miR-137. Exp Ther. Med. 17, 730–738.
Li, J., and He, D. (2019). Long noncoding RNA Xist predicts the presence of lymph node metastases in human oesophageal squamous cell carcinoma. Br. J. Biomed. Sci. 76, 147–149. doi: 10.1080/09674845.2019.1594489
Li, J. W., Wei, L., Han, Z. J., Chen, Z., and Zhang, Q. (2020a). Long non-coding RNA X-inactive specific transcript silencing ameliorates primary graft dysfunction following lung transplantation through microRNA-21-dependent mechanism. EBioMedicine 52, 102600–102613. doi: 10.1016/j.ebiom.2019.102600
Li, L., Lv, G. H., Wang, B., and Kuang, L. (2018). The role of lncRNA XIST/miR-211 axis in modulating the proliferation and apoptosis of osteoarthritis chondrocytes through CXCR4 and MAPK signaling. Biochem. Biophys. Res. Commun. 503, 2555–2562. doi: 10.1016/j.bbrc.2018.07.015
Li, L., Lv, G., Wang, B., and Kuang, L. (2020b). XIST/miR-376c-5p/OPN axis modulates the influence of proinflammatory M1 macrophages on osteoarthritis chondrocyte apoptosis. J. Cell. Physiol. 235, 281–293. doi: 10.1002/jcp.28968
Li, M., Pan, M., You, C. Z., Zhao, F. S., Wu, D., Guo, M., et al. (2020c). MiR-7 reduces the BCSC subset by inhibiting XIST to modulate the miR-92b/Slug/ESA axis and inhibit tumor growth. Breast Cancer Res. 22, 1–14.
Li, X., Hou, L., Yin, L., and Zhao, S. (2020d). LncRNA XIST interacts with miR-454 to inhibit cells proliferation, epithelial mesenchymal transition and induces apoptosis in triple-negative breast cancer. J. Biosciences 45, 1–11.
Li, X., Jia, Y., Zong, Y., Zhang, Y., Hou, D., Xu, J., et al. (2020e). The lncRNA XIST promotes the progression of breast cancer by sponging miR-125b-5p to modulate NLRC5. J. Gene Med. 12, 3501–3511.
Li, Y., Liang, Y., Zhu, Y., Zhang, Y., and Bei, Y. (2018). Noncoding RNAs in cardiac hypertrophy. J. Cardiovasc. Transl. Res. 11, 439–449. doi: 10.1007/s12265-018-9797-x
Li, Y. D., Zhang, Q., and Tang, X. Q. (2019). Long non-coding RNA XIST contributes into drug resistance of gastric cancer cell. Minerva Med. 110, 270–273.
Li, Z. Q., Zhang, Y. P., Ding, N., Zhao, Y. D., Ye, Z. K., Shen, L., et al. (2019). Inhibition of lncRNA XIST improves myocardial I/R injury by targeting miR-133a through inhibition of autophagy and regulation of SOCS2. Mol. Ther. Nucl. Acids 18, 764–773. doi: 10.1016/j.omtn.2019.10.004
Liang, S., Gong, X., Zhang, G., Huang, G., Lu, Y., and Li, Y. (2017). The lncRNA XIST interacts with miR-140/miR-124/iASPP axis to promote pancreatic carcinoma growth. Oncotarget 8, 113701–113718. doi: 10.18632/oncotarget.22555
Liao, X. Y., Tang, D. Z., Yang, H. S., Chen, Y., Chen, D. Y., Jia, L. S., et al. (2019). Long non-coding RNA XIST may influence cervical ossification of the posterior longitudinal ligament through regulation of miR-17-5P/AHNAK/BMP2 signaling pathway. Calcif. Tissue Int. 105, 670–680. doi: 10.1007/s00223-019-00608-y
Librizzi, M., Chiarelli, R., Bosco, L., Sansook, S., Gascon, J. M., Spencer, J., et al. (2015). The histone deacetylase inhibitor JAHA down-regulates pERK and global DNA methylation in MDA-MB231 breast cancer cells. Materials 8, 7041–7047. doi: 10.3390/ma8105358
Lin, B. J., Zhu, J. Y., Ye, J., Lu, S. D., Liao, M. D., Meng, X. C., et al. (2020). LncRNA-XIST promotes dermal papilla induced hair follicle regeneration by targeting miR-424 to activate hedgehog signaling. Cell. Signal. 72, 109623–109633. doi: 10.1016/j.cellsig.2020.109623
Lin, B., Xu, J., Wang, F., Wang, J., Zhao, H., and Feng, D. (2020). LncRNA XIST promotes myocardial infarction by regulating FOS through targeting miR-101a-3p. J. Cell. Mol. Med. 12, 7232–7247. doi: 10.18632/aging.103072
Lin, X. Q., Huang, Z. M., Chen, X., Wu, F., and Wu, W. (2018). XIST induced by JPX suppresses hepatocellular carcinoma by sponging miR-155-5p. Yonsei Med. J. 59, 816–826. doi: 10.3349/ymj.2018.59.7.816
Liu, A., Liu, L., and Lu, H. (2019a). LncRNA XIST facilitates proliferation and epithelial-mesenchymal transition of colorectal cancer cells through targeting miR-486-5p and promoting neuropilin-2. J. Cell. Physiol. 234, 13747–13761. doi: 10.1002/jcp.28054
Liu, B., Luo, C., Lin, H., Ji, X., Zhang, E., and Li, X. (2020a). Long noncoding RNA XIST acts as a ceRNA of miR-362-5p to suppress breast cancer progression. Cancer Biother. Radiopharm. [Epub ahead of print]. doi: 10.7150/jca.45676
Liu, C., Lu, Z., Liu, H., Zhuang, S., and Guo, P. (2020b). LncRNA XIST promotes the progression of laryngeal squamous cell carcinoma via sponging miR-125b-5p to modulate TRIB2. Bioscience Rep. 40:BSR20193172.
Liu, H., Deng, H. Y., Zhao, Y. J., Li, C., and Liang, Y. (2018c). LncRNA XIST/miR-34a axis modulates the cell proliferation and tumor growth of thyroid cancer through MET-PI3K-AKT signaling. J. Exp. Clin. Cancer Res. 37, 1–12.
Liu, J. L., Yao, L., Zhang, M. Y., Jiang, J., Yang, M. P., and Wang, Y. (2019b). Downregulation of LncRNA-XIST inhibited development of non-small cell lung cancer by activating miR-335/SOD2/ROS signal pathway mediated pyroptotic cell death. Aging Us 11, 7830–7846. doi: 10.18632/aging.102291
Liu, J. L., Zhang, W. Q., Zhao, M., and Huang, M. Y. (2019c). Upregulation of long noncoding RNA XIST is associated with poor prognosis in human cancers. J. Cell. Physiol. 234, 6594–6600. doi: 10.1002/jcp.27400
Liu, M., Zhu, H., Li, Y., Zhuang, J., Cao, T., and Wang, Y. (2020c). Expression of serum lncRNA-Xist in patients with polycystic ovary syndrome and its relationship with pregnancy outcome. Taiwan J. Obstet. Gynecol. 59, 372–376. doi: 10.1016/j.tjog.2020.03.006
Liu, W. G., and Xu, Q. (2019). Long non-coding RNA XIST promotes hepatocellular carcinoma progression by sponging miR-200b-3p. Eur. Rev. Med. Pharmacol. 23, 9857–9862.
Liu, X., Cui, L., and Hua, D. (2018a). Long noncoding RNA XIST regulates miR-137-EZH2 axis to promote tumor metastasis in colorectal cancer. Oncol. Res. 27, 99–106. doi: 10.3727/096504018x15195193936573
Liu, X., Ming, X., Jing, W., Luo, P., Li, N., Zhu, M., et al. (2018b). Long non-coding RNA XIST predicts worse prognosis in digestive system tumors: a systemic review and meta-analysis. Bioscience Rep. 38:BSR20180169.
Liu, X., Xie, S., Zhang, J., and Kang, Y. (2020d). Long noncoding RNA XIST contributes to cervical cancer development through targeting miR-889-3p/SIX1 axis. Cancer Biother. Radiopharm. [Epub ahead f print].
Liu, Y., Liu, K., Tang, C., Shi, Z., Jing, K., and Zheng, J. (2020e). Long non-coding RNA XIST contributes to osteoarthritis progression via miR-149-5p/DNMT3A axis. Biomed. Pharmacother. 128, 110349–110359. doi: 10.1016/j.biopha.2020.110349
Lobo, J., Nunes, S. P., Gillis, A. J. M., Barros-Silva, D., Miranda-Goncalves, V., van den Berg, A., et al. (2019). XIST-promoter demethylation as tissue biomarker for testicular germ cell tumors and spermatogenesis quality. Cancers 11, 1385–1400. doi: 10.3390/cancers11091385
Loda, A., and Heard, E. (2019). Xist RNA in action: past, present, and future. PLoS Genet. 15:e1008333. doi: 10.1371/journal.pgen.1008333
Lorenzen, J. M., and Thum, T. (2016). Long noncoding RNAs in kidney and cardiovascular diseases. Nat. Rev. Nephrol. 12, 360–373. doi: 10.1038/nrneph.2016.51
Lu, Z., Carter, A. C., and Chang, H. Y. (2017). Mechanistic insights in X-chromosome inactivation. Philos. Trans. R. Soc. Lond. Ser. B Biol. Sci. 372:20160356. doi: 10.1098/rstb.2016.0356
Luo, C. X., Quan, Z. P., Zhong, B., Zhang, M., Zhou, B., Wang, S. B., et al. (2020). lncRNA XIST promotes glioma proliferation and metastasis through miR-133a/SOX4. Exp. Ther. Med. 19, 1641–1648.
Luo, X., Luo, P., and Zhang, Y. (2020). Identification of differentially expressed long non-coding RNAs associated with dilated cardiomyopathy using integrated bioinformatics approaches. J. Clin. Lab. Anal. 14, 181–186. doi: 10.5582/ddt.2020.01010
Lv, G. Y., Miao, J., and Zhang, X. L. (2018). Long noncoding RNA XIST promotes osteosarcoma progression by targeting ras-related protein RAP2B via miR-320b. Oncol. Res. 26, 837–846. doi: 10.3727/096504017x14920318811721
Lyu, X., Ma, Y., Wu, F., Wang, L., and Wang, L. (2019). LncRNA NKILA Inhibits Retinoblastoma by Downregulating lncRNA XIST. Curr. Eye Res. 44, 975–979. doi: 10.1080/02713683.2019.1606253
Ma, L., Bajic, V. B., and Zhang, Z. (2013). On the classification of long non-coding RNAs. RNA Biol. 10, 925–933.
Ma, L., Zhou, Y. J., Luo, X. J., Gao, H., Deng, X. B., and Jiang, Y. J. (2017). Long non-coding RNA XIST promotes cell growth and invasion through regulating miR-497/MACC1 axis in gastric cancer. Oncotarget 8, 4125–4135. doi: 10.18632/oncotarget.13670
Ma, M., Pei, Y., Wang, X., Feng, J., Zhang, Y., and Gao, M. Q. (2019). LncRNA XIST mediates bovine mammary epithelial cell inflammatory response via NF-kappaB/NLRP3 inflammasome pathway. Cell Prolif. 52:e12525. doi: 10.1111/cpr.12525
Ma, W. J., Wang, H. T., Jing, W., Zhou, F. L., Chang, L., Hong, Z. F., et al. (2017). Downregulation of long non-coding RNAs JPX and XIST is associated with the prognosis of hepatocellular carcinoma. Clin. Res. Hepatol. Gas 41, 163–170. doi: 10.1016/j.clinre.2016.09.002
Ma, X., Yuan, T. D., Yang, C., Wang, Z. S., Zang, Y. J., Wu, L. Q., et al. (2017). X-inactive-specific transcript of peripheral blood cells is regulated by exosomal Jpx and acts as a biomarker for female patients with hepatocellular carcinoma. Ther. Adv. Med. Oncol. 9, 665–677. doi: 10.1177/1758834017731052
Ma, Y., Liu, X., Zhu, B., Li, W., He, Y., and Cheng, Z. (2020). Long noncoding RNA XIST knockdown suppresses the growth of colorectal cancer cells via regulating microRNA-338-3p/PAX5 axis. OncoTargets Ther. 596, 132–142. doi: 10.1097/cej.0000000000000596
Maduro, C., de Hoon, B., and Gribnau, J. (2016). Fitting the puzzle pieces: the bigger picture of XCI. Trends Biochem. Sci. 41, 138–147. doi: 10.1016/j.tibs.2015.12.003
Makhlouf, M., Ouimette, J. F., Oldfield, A., Navarro, P., Neuillet, D., and Rougeulle, C. (2014). A prominent and conserved role for YY1 in Xist transcriptional activation. Nat. Commun. 5, 1–12.
Matoba, S., Inoue, K., Kohda, T., Sugimoto, M., Mizutani, E., Ogonuki, N., et al. (2011). RNAi-mediated knockdown of Xist can rescue the impaired postimplantation development of cloned mouse embryos. Proc. Natl. Acad. Sci. U.S.A. 108, 20621–20626. doi: 10.1073/pnas.1112664108
McFaline-Figueroa, J. R., and Wen, P. Y. (2017). The viral connection to glioblastoma. Curr. Infect. Dis. Rep. 19, 1–5.
McHugh, C. A., Chen, C. K., Chow, A., Surka, C. F., Tran, C., McDonel, P., et al. (2015). The Xist lncRNA interacts directly with SHARP to silence transcription through HDAC3. Nature 521, 232–236. doi: 10.1038/nature14443
Medina, M. A., Oza, G., Sharma, A., Arriaga, L. G., Hernandez Hernandez, J. M., Rotello, V. M., et al. (2020). Triple-negative breast cancer: a review of conventional and advanced therapeutic strategies. Int. J. Environ. Res. Public Health 17, 2078–2110.
Minajigi, A., Froberg, J. E., Wei, C. Y., Sunwoo, H., Kesner, B., Colognori, D., et al. (2015). A comprehensive Xist interactome reveals cohesin repulsion and an RNA-directed chromosome conformation. Science 349, aab22761–aab227612.
Mira-Bontenbal, H., and Gribnau, J. (2016). New Xist-interacting proteins in X-chromosome inactivation. Curr. Biol. 26, 338–342.
Mo, Y., Lu, Y., Wang, P., Huang, S., He, L., Li, D., et al. (2017). Long non-coding RNA XIST promotes cell growth by regulating miR-139-5p/PDK1/AKT axis in hepatocellular carcinoma. Tumour Biol. 39:1010428317690999.
Moga, M. A., Balan, A., Anastasiu, C. V., Dimienescu, O. G., Neculoiu, C. D., and Gavris, C. (2018). An overview on the anticancer activity of azadirachta indica (Neem) in gynecological cancers. Int. J. Mol. Sci. 19, 3989–4024.
Monfort, A., Di Minin, G., Postlmayr, A., Freimann, R., Arieti, F., Thore, S., et al. (2015). Identification of spen as a crucial factor for xist function through forward genetic screening in haploid embryonic stem cells. Cell Rep. 12, 554–561. doi: 10.1016/j.celrep.2015.06.067
Monfort, A., and Wutz, A. (2020). The B-side of Xist. F1000Research 9, 1–12. doi: 10.1109/tmag.2013.2278570
Montalbano, A., Canver, M. C., and Sanjana, N. E. (2017). High-throughput approaches to pinpoint function within the noncoding genome. Mol. Cell 68, 44–59. doi: 10.1016/j.molcel.2017.09.017
Muller, D. A., and Silvan, U. (2019). On the biomechanical properties of osteosarcoma cells and their environment. Int. J. Dev. Biol. 63, 1–8. doi: 10.1387/ijdb.190019us
Mutzel, V., and Schulz, E. G. (2020). Dosage sensing, threshold responses, and epigenetic memory: a systems biology perspective on random X-chromosome inactivation, bioessays : news and reviews in molecular. Cell. Dev. Biol. 42:e1900163.
Nesterova, T. B., Wei, G. F., Coker, H., Pintacuda, G., Bowness, J. S., Zhang, T. Y., et al. (2019). Systematic allelic analysis defines the interplay of key pathways in X chromosome inactivation. Nat. Commun. 10, 1–15.
Niu, S., Xiang, F., and Jia, H. (2020). Downregulation of lncRNA XIST promotes proliferation and differentiation, limits apoptosis of osteoblasts through regulating miR-203-3p/ZFPM2 axis. Nat. Methods [Epub ahead of print]. doi: 10.1080/03008207.2020.1752200
Pan, B., Lin, X., Zhang, L., Hong, W., and Zhang, Y. (2019). Long noncoding RNA X-inactive specific transcript promotes malignant melanoma progression and oxaliplatin resistance. Melanoma Res. 29, 254–262. doi: 10.1097/cmr.0000000000000560
Patrat, C., Ouimette, J. F., and Rougeulle, C. (2020). X chromosome inactivation in human development. Development 147:dev183095.
Peng, H., Luo, Y. X., and Ying, Y. J. (2020). lncRNA XIST attenuates hypoxia-induced H9c2 cardiomyocyte injury by targeting the miR-122-5p/FOXP2 axis. Mol. Cell. Probes 50, 101500–101509. doi: 10.1016/j.mcp.2019.101500
Pinheiro, I., and Heard, E. (2017). X chromosome inactivation: new players in the initiation of gene silencing. F1000Research 6, 1–10.
Pintacuda, G., Wei, G. F., Roustan, C., Kirmizitas, B. A., Solcan, N., Cerase, A., et al. (2017a). hnRNPK recruits PCGF3/5-PRC1 to the Xist RNA B-repeat to establish polycomb-mediated chromosomal silencing. Mol. Cell 68, 955–969. doi: 10.1016/j.molcel.2017.11.013
Pintacuda, G., Young, A. N., and Cerase, A. (2017b). Function by structure: spotlights on Xist long non-coding RNA. Front. Mol. Biosci. 4:90. doi: 10.3389/fmolb.2017.00090
Polito, C., Pannuti, A., and Lucchesi, J. C. (1990). Dosage compensation in Drosophila melanogaster male and female embryos generated by segregation distortion of the sex chromosomes. Dev. Genet. 11, 249–253. doi: 10.1002/dvg.1020110402
Postlmayr, A., Dumeau, C. E., and Wutz, A. (2020). Cdk8 is required for establishment of H3K27me3 and gene repression by Xist and mouse development. Development 147, 175141–175151.
Prat, A., Pineda, E., Adamo, B., Galvan, P., Fernandez, A., Gaba, L., et al. (2015). Clinical implications of the intrinsic molecular subtypes of breast cancer. Breast 24, 26–35.
Qiu, H. B., Yang, K., Yu, H. Y., and Liu, M. (2019). Downregulation of long non-coding RNA XIST inhibits cell proliferation, migration, invasion and EMT by regulating miR-212-3p/CBLL1 axis in non-small cell lung cancer cells. Eur. Rev. Med. Pharmacol. 23, 8391–8402.
Quinn, J. J., and Chang, H. Y. (2016). Unique features of long non-coding RNA biogenesis and function. Nat. Rev. Genet. 17, 47–62. doi: 10.1038/nrg.2015.10
Richard, J. L. C., and Eichhorn, P. J. A. (2018). Deciphering the roles of lncRNAs in breast development and disease. Oncotarget 9, 20179–20212. doi: 10.18632/oncotarget.24591
Romagnolo, A. P. G., Romagnolo, D. F., and Selmin, O. I. (2015). BRCA1 as target for breast cancer prevention and therapy. Anti Cancer Agent. Meas. 15, 4–14. doi: 10.2174/1871520614666141020153543
Rong, H., Chen, B., Wei, X., Peng, J., Ma, K., Duan, S., et al. (2020). Long non-coding RNA XIST expedites lung adenocarcinoma progression through upregulating MDM2 expression via binding to miR-363-3p. Thorac. Cancer 11, 659–671. doi: 10.1111/1759-7714.13310
Ropers, H. H., Wolff, G., and Hitzeroth, H. W. (1978). Preferential X inactivation in human placenta membranes: is the paternal X inactive in early embryonic development of female mammals? Hum. Genet. 43, 265–273. doi: 10.1007/bf00278833
Sado, T., and Brockdorff, N. (2013). Advances in understanding chromosome silencing by the long non-coding RNA Xist. Philos. Trans. R. Soc. Lond. Ser. B Biol. Sci. 368:20110325. doi: 10.1098/rstb.2011.0325
Sahakyan, A., Yang, Y., and Plath, K. (2018). The role of xist in X-chromosome dosage compensation. Trends Cell Biol. 28, 999–1013. doi: 10.1016/j.tcb.2018.05.005
Sakaguchi, T., Hasegawa, Y., Brockdorff, N., Tsutsui, K., Tsutsui, K. M., Sado, T., et al. (2016). Control of chromosomal localization of Xist by hnRNP U family molecules. Dev. Cell. 39, 11–12. doi: 10.1016/j.devcel.2016.09.022
Salama, E. A., Adbeltawab, R. E., and El Tayebi, H. M. (2019). XIST and TSIX: novel cancer immune biomarkers in PD-L1-overexpressing breast cancer patients. Front. Oncol. 9:1459. doi: 10.3389/fonc.2019.01459
Salvador, M. A., Wicinski, J., Cabaud, O., Toiron, Y., Finetti, P., Josselin, E., et al. (2013). The histone deacetylase inhibitor abexinostat induces cancer stem cells differentiation in breast cancer with low Xist expression. Clin. Cancer Res. 19, 6520–6531. doi: 10.1158/1078-0432.ccr-13-0877
Sandoval, Y., Smith, S. W., Sexter, A., Thordsen, S. E., Bruen, C. A., Carlson, M. D., et al. (2017). Type 1 and 2 myocardial infarction and myocardial injury: clinical transition to high-sensitivity cardiac troponin I. Am. J. Med. 130, 1431–1439.
Sandoval, Y., and Thygesen, K. (2017). Myocardial infarction type 2 and myocardial injury. Clin. Chem. 63, 101–107. doi: 10.1373/clinchem.2016.255521
Sarma, K., Cifuentes-Rojas, C., Ergun, A., del Rosario, A., Jeon, Y., White, F., et al. (2014). ATRX directs binding of PRC2 to Xist RNA and polycomb targets. Cell 159, 869–883. doi: 10.1016/j.cell.2014.10.019
Schadendorf, D., van Akkooi, A. C. J., Berking, C., Griewank, K. G., Gutzmer, R., Hauschild, A., et al. (2018). Melanoma. Lancet 392, 971–984.
Scheltens, P., Blennow, K., Breteler, M. M. B., de Strooper, B., Frisoni, G. B., Salloway, S., et al. (2016). Alzheimer’s disease. Lancet 388, 505–517.
Schertzer, M. D., Braceros, K. C. A., Starmer, J., Cherney, R. E., Lee, D. M., Salazar, G., et al. (2019). lncRNA-induced spread of polycomb controlled by genome architecture, RNA abundance, and CpG Island DNA. Mol. Cell 75, 523–537. doi: 10.1016/j.molcel.2019.05.028
Schmitz, S. U., Grote, P., and Herrmann, B. G. (2016). Mechanisms of long noncoding RNA function in development and disease. Cell Mol. Life Sci. 73, 2491–2509. doi: 10.1007/s00018-016-2174-5
Schouten, P. C., Vollebergh, M. A., Opdam, M., Jonkers, M., Loden, M., Wesseling, J., et al. (2016). High XIST and low 53BP1 expression predict poor outcome after high-dose alkylating chemotherapy in patients with a BRCA1-like breast cancer. Mol. Cancer Ther. 15, 190–198. doi: 10.1158/1535-7163.mct-15-0470
Shen, C., and Li, J. (2020). LncRNA XIST silencing protects against sepsis-induced acute liver injury via inhibition of BRD4 expression. Inflammation 1321, 1–12.
Shen, J., Hong, L., Yu, D., Cao, T., Zhou, Z., and He, S. (2019). LncRNA XIST promotes pancreatic cancer migration, invasion and EMT by sponging miR-429 to modulate ZEB1 expression. Int. J. Biochem. Cell Biol. 113, 17–26. doi: 10.1016/j.biocel.2019.05.021
Shen, J., Xiong, J., Shao, X., Cheng, H., Fang, X., Sun, Y., et al. (2020). Knockdown of the long noncoding RNA XIST suppresses glioma progression by upregulating miR-204-5p. J. Cancer 11, 4550–4559.
Shen, X. F., Cheng, Y., Dong, Q. R., and Zheng, M. Q. (2020). MicroRNA-675-3p regulates IL-1β-stimulated human chondrocyte apoptosis and cartilage degradation by targeting GNG5. Biochem. Biophys. Res. Commun. 527, 458–465. doi: 10.1016/j.bbrc.2020.04.044
Shenoda, B. B., Ramanathan, S., Gupta, R., Tian, Y., Jean-Toussaint, R., Alexander, G. M., et al. (2020). Xist attenuates acute inflammatory response by female cells. Cell Mol. Life Sci. 2020, 1–18.
Shenoda, B. B., Tian, Y. Z., Alexander, G. M., Aradillas-Lopez, E., Schwartzman, R. J., and Ajit, S. K. (2018). miR-34a-mediated regulation of XIST in female cells under inflammation. J. Pain Res. 11, 935–945. doi: 10.2147/jpr.s159458
Sherstyuk, V. V., Shevchenko, A. I., Mazurok, N. A., and Zakian, S. M. (2013). Replication origin activity in vole X chromosome inactivation center in different cell types, Doklady. Biochem. Biophys. 450, 164–166. doi: 10.1134/s1607672913030125
Shi, J. F., Tan, S. L., Song, L. M., Song, L. S., and Wang, Y. S. (2020). LncRNA XIST knockdown suppresses the malignancy of human nasopharyngeal carcinoma through XIST/miRNA-148a-3p/ADAM17 pathway in vitro and in vivo. Biomed. Pharmacother. 121:109620. doi: 10.1016/j.biopha.2019.109620
Shi, X., Sun, M., Liu, H., Yao, Y., and Song, Y. (2013). Long non-coding RNAs: a new frontier in the study of human diseases. Cancer Lett. 339, 159–166. doi: 10.1016/j.canlet.2013.06.013
Shukla, S., Penta, D., Mondal, P., and Meeran, S. M. (2019). Epigenetics of breast cancer: clinical status of epi-drugs and phytochemicals. Adv. Exp. Med. Biol. 1152, 293–310. doi: 10.1007/978-3-030-20301-6_16
Sidorenko, J., Kassam, I., Kemper, K. E., Zeng, J., Lloyd-Jones, L. R., Montgomery, G. W., et al. (2019). The effect of X-linked dosage compensation on complex trait variation. Nat. Commun. 10, 3009–3020.
Siegel, R. L., Miller, K. D., and Jemal, A. (2019). Cancer statistics, 2019. CA Cancer J. Clin. 69, 7–34.
Siegel, R. L., Miller, K. D., and Jemal, A. (2020). Cancer statistics, 2020. CA Cancer J. Clin. 70, 7–30.
Sirchia, S. M., Ramoscelli, L., Grati, F. R., Barbera, F., Coradini, D., Rossella, F., et al. (2005). Loss of the inactive X chromosome and replication of the active x in BRCA1-defective and wild-type breast cancer cells. Cancer Res. 65, 2139–2146. doi: 10.1158/0008-5472.can-04-3465
Sirchia, S. M., Tabano, S., Monti, L., Recalcati, M. P., Gariboldi, M., Grati, F. R., et al. (2009). Misbehaviour of XIST RNA in breast cancer cells. PLoS One 4:e5559. doi: 10.1371/journal.pone.0005559
Slack, F. J., and Chinnaiyan, A. M. (2019). The role of non-coding RNAs in oncology. Cell 179, 1033–1055. doi: 10.1016/j.cell.2019.10.017
Sohrabifar, N. (2020). Variation in the expression level of MALAT1, MIAT and XIST lncRNAs in coronary artery disease patients with and without type 2 diabetes mellitus. Cell Biol. Int. [Epub ahead of print].
Song, H., He, P., Shao, T. S., Li, Y., Li, J., and Zhang, Y. J. (2017). Long non-coding RNA XIST functions as an oncogene in human colorectal cancer by targeting miR-132-3p. J. Buon 22, 696–703.
Song, P., Ye, L. F., Zhang, C., Peng, T., and Zhou, X. H. (2016). Long non-coding RNA XIST exerts oncogenic functions in human nasopharyngeal carcinoma by targeting miR-34a-5p. Gene 592, 8–14. doi: 10.1016/j.gene.2016.07.055
Soudyab, M., Iranpour, M., and Ghafouri-Fard, S. (2016). The role of long non-coding RNAs in breast cancer. Arch. Iranian Med. 19, 508–517.
Spatz, A., Borg, C., and Feunteun, J. (2004). X-chromosome genetics and human cancer. Nat. Rev. Cancer 4, 617–629.
Sripathy, S., Leko, V., Adrianse, R. L., Loe, T., Foss, E. J., Dalrymple, E., et al. (2017). Screen for reactivation of MeCP2 on the inactive X chromosome identifies the BMP/TGF-beta superfamily as a regulator of XIST expression. Proc. Natl. Acad. Sci. U.S.A. 114, 1619–1624. doi: 10.1073/pnas.1621356114
St Laurent, G., Wahlestedt, C., and Kapranov, P. (2015). The Landscape of long noncoding RNA classification. Trends Genet. 31, 239–251. doi: 10.1016/j.tig.2015.03.007
Steuer, C. E., El-Deiry, M., Parks, J. R., Higgins, K. A., and Saba, N. F. (2017). An update on larynx cancer. CA Cancer J. Clin. 67, 31–50. doi: 10.3322/caac.21386
Stewart, C., Ralyea, C., and Lockwood, S. (2019). Ovarian cancer: an integrated review. Semin. Oncol. Nurs. 35, 151–156.
Strehle, M., and Guttman, M. (2020). Xist drives spatial compartmentalization of DNA and protein to orchestrate initiation and maintenance of X inactivation. Mol. Genet. Genom. Med. 64, 139–147. doi: 10.1016/j.ceb.2020.04.009
Sun, J., Pan, L. M., Chen, L. B., and Wang, Y. (2017). LncRNA XIST promotes human lung adenocarcinoma cells to cisplatin resistance via let-7i/BAG-1 axis. Cell Cycle 16, 2100–2107. doi: 10.1080/15384101.2017.1361071
Sun, J. M., and Zhang, Y. B. (2019). LncRNA XIST enhanced TGF-beta 2 expression by targeting miR-141-3p to promote pancreatic cancer cells invasion. Bioscience Rep. 39:BSR20190332.
Sun, K., Jia, Z., Duan, R., Yan, Z., Jin, Z., Yan, L., et al. (2019). Long non-coding RNA XIST regulates miR-106b-5p/P21 axis to suppress tumor progression in renal cell carcinoma. Biochem. Biophys. Res. Commun. 510, 416–420. doi: 10.1016/j.bbrc.2019.01.116
Sun, N. N., Zhang, G. Z., and Liu, Y. Y. (2018). Long non-coding RNA XIST sponges miR-34a to promotes colon cancer progression via Wnt/beta-catenin signaling pathway. Gene 665, 141–148. doi: 10.1016/j.gene.2018.04.014
Sun, W., Zu, Y. K., Fu, X. N., and Deng, Y. (2017). Knockdown of lncRNA-XIST enhances the chemosensitivity of NSCLC cells via suppression of autophagy. Oncol. Rep. 38, 3347–3354.
Sun, W. B., Ma, M. N., Yu, H. M., and Yu, H. (2018). Inhibition of lncRNA X inactivate-specific transcript ameliorates inflammatory pain by suppressing satellite glial cell activation and inflammation by acting as a sponge of miR-146a to inhibit Na(v)1.7. J. Cell. Biochem. 119, 9888–9898. doi: 10.1002/jcb.27310
Sun, X., Wei, B., Peng, Z. H., Fu, Q. L., Wang, C. J., Zheng, J. C., et al. (2019). Knockdown of lncRNA XIST suppresses osteosarcoma progression by inactivating AKT/mTOR signaling pathway by sponging miR-375-3p. Int. J. Clin. Exp. Pathol. 12, 1507–1517.
Sun, Y., Lv, B., and Zhang, X. (2020). Knock-down of LncRNA-XIST induced glioma cell death and inhibited tumorigenesis by regulating miR-137/SLC1A5 axis-mediated ROS production. Naunyn Schmiedebergs Arch. Pharmacol. 394:557. doi: 10.1007/s00210-020-01831-3
Sun, Y. B., and Xu, J. J. (2019). TCF-4 regulated lncRNA-XIST promotes M2 polarization of macrophages and is associated with lung cancer. OncoTargets Ther. 12, 8055–8062. doi: 10.2147/ott.s210952
Sun, Z., Zhang, B., and Cui, T. (2018). Long non-coding RNA XIST exerts oncogenic functions in pancreatic cancer via miR-34a-5p. Oncol. Rep. 39, 1591–1600.
Syrett, C. M., Paneru, B., Sandoval-Heglund, D., Wang, J. L., Banerjee, S., Sindhava, V., et al. (2019). Altered X-chromosome inactivation in T cells may promote sex-biased autoimmune diseases. JCI Insight 4:e126751.
Syrett, C. M., Sierra, I., Beethem, Z. T., Dubin, A. H., and Anguera, M. C. (2020). Loss of epigenetic modifications on the inactive X chromosome and sex-biased gene expression profiles in B cells from NZB/W F1 mice with lupus-like disease. J. Autoimmun. 107:102357. doi: 10.1016/j.jaut.2019.102357
Syrett, C. M., Sindhava, V., Hodawadekar, S., Myles, A., Liang, G. X., Zhang, Y., et al. (2017). Loss of Xist RNA from the inactive X during B cell development is restored in a dynamic YY1-dependent two-step process in activated B cells. PLoS Genet. 13:e1007050. doi: 10.1371/journal.pgen.1007050
Tang, B., Li, W., Ji, T., Li, X., Qu, X., Feng, L., et al. (2020). Downregulation of XIST ameliorates acute kidney injury by sponging miR-142-5p and targeting PDCD4. J. Cell. Physiol. 2020, 1–12. doi: 10.1155/2021/6624702
Tang, Y., He, R., An, J., Deng, P., Huang, L., and Yang, W. (2017). lncRNA XIST interacts with miR-140 to modulate lung cancer growth by targeting iASPP. Oncol. Rep. 38, 941–948. doi: 10.3892/or.2017.5751
Tantai, J. C., Hu, D. Z., Yang, Y., and Geng, J. F. (2015). Combined identification of long non-coding RNA XIST and HIF1A-AS1 in serum as an effective screening for non-small cell lung cancer. Int. J. Clin. Exp. Pathol. 8, 7887–7895.
Thompson, L. D. (2017). Laryngeal dysplasia, squamous cell carcinoma, and variants. Surg. Pathol. Clin. 10, 15–33. doi: 10.1016/j.path.2016.10.003
Thorvaldsen, J. L., Weaver, J. R., and Bartolomei, M. S. (2011). A YY1 bridge for X inactivation. Cell 146, 11–13. doi: 10.1016/j.cell.2011.06.029
Tian, K., Sun, D., Chen, M., Yang, Y., Wang, F., Guo, T., et al. (2020). Long Noncoding RNA X-inactive specific transcript facilitates cellular functions in melanoma via miR-139-5p/ROCK1 pathway. OncoTargets Ther. 13, 1277–1287. doi: 10.2147/ott.s225661
Tian, L. J., Wu, Y. P., Wang, D., Zhou, Z. H., Shou, B., Zhang, D. Y., et al. (2019). Upregulation of long noncoding RNA (lncRNA) X-inactive specific transcript (XIST) is associated with cisplatin resistance in non-small cell lung cancer (NSCLC) by downregulating MicroRNA-144-3p. Med. Sci. Monit. 25, 8095–8104. doi: 10.12659/msm.916075
Tian, R., Wang, L. F., Zou, H., Song, M. J., Liu, L., and Zhang, H. (2020). Role of the XIST-miR-181a-COL4A1 axis in the development and progression of keratoconus. Mol. Vis. 26, 1–13.
van Bemmel, J. G., Galupa, R., Gard, C., Servant, N., Picard, C., Davies, J., et al. (2019). The bipartite TAD organization of the X-inactivation center ensures opposing developmental regulation of Tsix and Xist. Nat. Genet. 51, 1024–1034. doi: 10.1038/s41588-019-0412-0
van Bemmel, J. G., Mira-Bontenbal, H., and Gribnau, J. (2016). Cis- and trans-regulation in X inactivation. Chromosoma 125, 41–50. doi: 10.1007/s00412-015-0525-x
Velázquez-Flores, M., Rodríguez-Corona, J. M., ópez-Aguilar, J. E. L., Siordia-Reyes, G., Ramírez-Reyes, G., ánchez-Rodríguez, G. S., et al. (2020). Noncoding RNAs as potential biomarkers for DIPG diagnosis and prognosis: XIST and XIST-210 involvement. Clin. Transl. Oncol. 7, 1–13. doi: 10.1155/2020/8822859
Vincent-Salomon, A., Ganem-Elbaz, C., Manie, E., Raynal, V., Sastre-Garau, X., Stoppa-Lyonnet, D., et al. (2007). X inactive-specific transcript RNA coating and genetic instability of the X chromosome in BRCA1 breast tumors. Cancer Res. 67, 5134–5140. doi: 10.1158/0008-5472.can-07-0465
Wang, C. H., Qi, S., Xie, C., Li, C. F., Wang, P., and Liu, D. M. (2018a). Upregulation of long non-coding RNA XIST has anticancer effects on epithelial ovarian cancer cells through inverse downregulation of hsa-miR-214-3p. J. Gynecol. Oncol. 29:e99.
Wang, C. Y., Colognori, D., Sunwoo, H., Wang, D. N., and Lee, J. T. (2019a). PRC1 collaborates with SMCHD1 to fold the X-chromosome and spread Xist RNA between chromosome compartments. Nat. Commun. 10, 1–18.
Wang, D., Tang, L., Wu, Y., Fan, C., Zhang, S., Xiang, B., et al. (2020a). Abnormal X chromosome inactivation and tumor development. Cell Mol. Life Sci. 77, 2949–2958. doi: 10.1007/s00018-020-03469-z
Wang, H., Li, W., and Tan, G. (2019b). Long non-coding RNA XIST modulates cisplatin resistance by altering PDCD4 and Fas-Lexpressions in human nasopharyngeal carcinoma HNE1 cells in vitro. Nan Fang Yi Ke Da Xue Xue Bao 39, 357–363.
Wang, H. Y., Shen, Q. M., Zhang, X., Yang, C. L., Cui, S., Sun, Y. B., et al. (2017). The long non-coding RNA XIST controls non-small cell lung cancer proliferation and invasion by modulating miR-186-5p. Cell. Physiol. Biochem. 41, 2221–2229. doi: 10.1159/000475637
Wang, J. L., Cai, H. B., Dai, Z. X., and Wang, G. (2019c). Down-regulation of lncRNA XIST inhibits cell proliferation via regulating miR-744/RING1 axis in non-small cell lung cancer. Clin. Sci. 133, 1567–1579. doi: 10.1042/cs20190519
Wang, Q. (2020). XIST silencing alleviated inflammation and mesangial cells proliferation in diabetic nephropathy by sponging miR-485. Arch. Physiol. Biochem. 2020, 1–7. doi: 10.1080/13813455.2020.1789880
Wang, Q. Y., Peng, L., Chen, Y., Liao, L. D., Chen, J. X., Li, M., et al. (2020b). Characterization of super-enhancer-associated functional lncRNAs acting as ceRNAs in ESCC. Development 14, 2203–2230. doi: 10.1002/1878-0261.12726
Wang, S. G., Cao, F., Gu, X. S., Chen, J. N., Xu, R. X., Huang, Y. N., et al. (2019d). LncRNA XIST, as a ceRNA of miR-204, aggravates lipopolysaccharide-induced acute respiratory distress syndrome in mice by upregulating IRF2. Int. J. Clin. Exp. Pathol. 12, 2425–2434.
Wang, S. L., and Li, G. Z. (2020). LncRNA XIST inhibits ovarian cancer cell growth and metastasis via regulating miR-150-5p/PDCD4 signaling pathway. N. S. Arch. Pharmacol. 13, 1–13.
Wang, T., Liu, Y., Wang, Y., Huang, X., Zhao, W., and Zhao, Z. (2019e). Long non-coding RNA XIST promotes extracellular matrix degradation by functioning as a competing endogenous RNA of miR-1277-5p in osteoarthritis. Int. J. Mol. Med. 44, 630–642.
Wang, W., Shen, H., Cao, G., and Huang, J. (2019f). Long non-coding RNA XIST predicts poor prognosis and promotes malignant phenotypes in osteosarcoma. Oncol. Lett. 17, 256–262.
Wang, X., Wang, C., Geng, C., and Zhao, K. (2018b). LncRNA XIST knockdown attenuates Abeta25-35-induced toxicity, oxidative stress, and apoptosis in primary cultured rat hippocampal neurons by targeting miR-132. Int. J. Clin. Exp. Pathol. 11, 3915–3924.
Wang, X., Zhang, G. J., Cheng, Z., Dai, L. L., Jia, L. Q., Jing, X. G., et al. (2018c). Knockdown of LncRNA-XIST suppresses proliferation and TGF-beta 1-induced EMT in NSCLC through the Notch-1 pathway by regulation of miR-137. Genet. Test. Mol. Biomark. 22, 333–342. doi: 10.1089/gtmb.2018.0026
Wang, Y., Sun, D., Sheng, Y., Guo, H., Meng, F., and Song, T. (2020c). XIST promotes cell proliferation and invasion by regulating miR-140-5p and SOX4 in retinoblastoma. World J. Surg. Oncol. 18, 49–57.
Wang, Y. C., Liang, Y., Luo, J. M., Nie, J., Yin, H. M., Chen, Q., et al. (2017). XIST/miR-139 axis regulates bleomycin (BLM)-induced extracellular matrix (ECM) and pulmonary fibrosis through beta-catenin. Oncotarget 8, 65359–65369. doi: 10.18632/oncotarget.18310
Wang, Z., Yuan, J., Li, L., Yang, Y., Xu, X., and Wang, Y. (2017). Long non-coding RNA XIST exerts oncogenic functions in human glioma by targeting miR-137. Am. J. Transl. Res. 9, 1845–1855.
Wang, Z. Q., Xiu, D. H., Jiang, J. L., and Liu, G. F. (2020d). Long non-coding RNA XIST binding to let-7c-5p contributes to rheumatoid arthritis through its effects on proliferation and differentiation of osteoblasts via regulation of STAT3. Nature 2020:e23496.
Wang, Z. Y., Qiu, H., He, J. B., Liu, L. X., Xue, W., Fox, A., et al. (2019g). The emerging roles of hnRNPK. J. Cell. Physiol. 235, 1–14.
Waśko, U., Zheng, Z., and Bhatnagar, S. (2019). Visualization of Xist long noncoding RNA with a fluorescent CRISPR/Cas9 system. Epitranscriptomics 2019, 41–50. doi: 10.1007/978-1-4939-8808-2_3
Webb, P. M., and Jordan, S. J. (2017). Epidemiology of epithelial ovarian cancer, Best practice & research. Clin. Obstetr. Gynaecol. 41, 3–14. doi: 10.1016/j.bpobgyn.2016.08.006
Wehbe, N., Nasser, S. A., Pintus, G., Badran, A., Eid, A. H., and Baydoun, E. (2019). MicroRNAs in cardiac hypertrophy. Int. J. Mol. Sci. 20, 4714–4731. doi: 10.3390/ijms20194714
Wei, K. R., Zheng, R. S., Zhang, S. W., Liang, Z. H., Li, Z. M., and Chen, W. Q. (2017). Nasopharyngeal carcinoma incidence and mortality in China, 2013. Chinese J. Cancer 36, 90–98.
Wei, M., Li, L., Zhang, Y., Zhang, Z. J., Liu, H. L., and Bao, H. G. (2019). LncRNA X inactive specific transcript contributes to neuropathic pain development by sponging miR-154-5p via inducing toll-like receptor 5 in CCI rat models. J. Cell. Biochem. 120, 1271–1281. doi: 10.1002/jcb.27088
Wei, W., Liu, Y., Lu, Y. B., Yang, B., and Tang, L. (2017). LncRNA XIST promotes pancreatic cancer proliferation through miR-133a/EGFR. J. Cell. Biochem. 118, 3349–3358. doi: 10.1002/jcb.25988
Wen, J. F., Jiang, Y. Q., Li, C., Dai, X. K., Wu, T., and Yin, W. Z. (2020). LncRNA-XIST promotes the oxidative stress-induced migration, invasion, and epithelial-to-mesenchymal transition of osteosarcoma cancer cells through miR-153-SNAI1 axis. Cell Biol. Int. 10, 1991–2001. doi: 10.3389/fonc.2020.577280
Willard, H. F. (1996). X chromosome inactivation, XIST, and pursuit of the X-inactivation center. Cell 86, 5–7. doi: 10.1016/s0092-8674(00)80071-9
Wu, D. P., Nie, X. G., Ma, C., Liu, X. H., Liang, X., An, Y. B., et al. (2017). RSF1 functions as an oncogene in osteosarcoma and is regulated by XIST/miR-193a-3p axis. Biomed. Pharmacother. 95, 207–214. doi: 10.1016/j.biopha.2017.08.068
Wu, X., Dinglin, X., Wang, X., Luo, W., Shen, Q., Li, Y., et al. (2017). Long noncoding RNA XIST promotes malignancies of esophageal squamous cell carcinoma via regulation of miR-101/EZH2. Oncotarget 8, 76015–76028.
Xiao, D., Cui, X., and Wang, X. (2019). Long noncoding RNA XIST increases the aggressiveness of laryngeal squamous cell carcinoma by regulating miR-124-3p/EZH2. Exp. Cell Res. 381, 172–178. doi: 10.1016/j.yexcr.2019.04.034
Xiao, L., Gu, Y., Sun, Y., Chen, J., Wang, X., Zhang, Y., et al. (2019). The long noncoding RNA XIST regulates cardiac hypertrophy by targeting miR-101. J. Cell. Physiol. 234, 13680–13692. doi: 10.1002/jcp.28047
Xiao, Y., Yurievich, U. A., and Yosypovych, S. V. (2017). Long noncoding RNA XIST is a prognostic factor in colorectal cancer and inhibits 5-fluorouracil-induced cell cytotoxicity through promoting thymidylate synthase expression. Oncotarget 8, 83171–83182. doi: 10.18632/oncotarget.20487
Xie, Z. Y., Wang, F. F., Xiao, Z. H., Liu, S. F., Lai, Y. L., and Tang, S. L. (2019). Long noncoding RNA XIST enhances ethanol-induced hepatic stellate cells autophagy and activation via miR-29b/HMGB1 axis. IUBMB Life 71, 1962–1972. doi: 10.1002/iub.2140
Xing, F., Liu, Y., Wu, S. Y., Wu, K., Sharma, S., Mo, Y. Y., et al. (2018). Loss of XIST in breast cancer activates MSN-c-met and reprograms microglia via exosomal miRNA to promote brain metastasis. Cancer Res. 78, 4316–4330. doi: 10.1158/0008-5472.can-18-1102
Xiong, Y., Wang, L., Li, Y., Chen, M., He, W., and Qi, L. (2017). The long non-coding RNA XIST interacted with MiR-124 to modulate bladder cancer growth, invasion and migration by targeting androgen receptor (AR). Cell. Physiol. Biochem. 43, 405–418. doi: 10.1159/000480419
Xu, G. H., Mo, L. J., Wu, C. N., Shen, X. Y., Dong, H. L., Yu, L. F., et al. (2019). The miR-15a-5p-XIST-CUL3 regulatory axis is important for sepsis-induced acute kidney injury. Renal Fail. 41, 955–966. doi: 10.1080/0886022x.2019.1669460
Xu, L., Zhang, Y. G., and Huang, Y. G. (2016). Advances in the treatment of neuropathic pain. Transl. Res. Pain Itch 904, 117–129.
Xu, N., Tsai, C. L., and Lee, J. T. (2006). Transient homologous chromosome pairing marks the onset of X inactivation. Science 311, 1149–1152. doi: 10.1126/science.1122984
Xu, R., Zhu, X., Chen, F., Huang, C., Ai, K., Wu, H., et al. (2018). LncRNA XIST/miR-200c regulates the stemness properties and tumourigenicity of human bladder cancer stem cell-like cells. Cancer Cell Int. 18, 1–10.
Xu, T., Jiang, W., Fan, L., Gao, Q., and Li, G. (2017). Upregulation of long noncoding RNA Xist promotes proliferation of osteosarcoma by epigenetic silencing of P21. Oncotarget 8, 101406–101417. doi: 10.18632/oncotarget.20738
Xu, X. H., Ma, C. M., Liu, C., Duan, Z. H., and Zhang, L. (2018). Knockdown of long noncoding RNA XIST alleviates oxidative low-density lipoprotein-mediated endothelial cells injury through modulation of miR-320/NOD2 axis. Biochem. Biophys. Res. Commun. 503, 586–592. doi: 10.1016/j.bbrc.2018.06.042
Xu, X. H., Zhou, X. Y., Chen, Z. J., Gao, C., Zhao, L., and Cui, Y. S. (2020). Silencing of lncRNA XIST inhibits non-small cell lung cancer growth and promotes chemosensitivity to cisplatin. Aging Us 12, 4711–4726. doi: 10.18632/aging.102673
Xu, Y., Wang, J., and Wang, J. (2018). Long noncoding RNA XIST promotes proliferation and invasion by targeting miR-141 in papillary thyroid carcinoma. OncoTargets Ther. 11, 5035–5043. doi: 10.2147/ott.s170439
Xu, Z. Z., Xu, J., Lu, H. Q., Lin, B., Cai, S. P., Guo, J., et al. (2017). LARP1 is regulated by the XIST/miR-374a axis and functions as an oncogene in non-small cell lung carcinoma. Oncol. Rep. 38, 3659–3667.
Yan, F. R., Wang, X. D., and Zeng, Y. M. (2019). 3D genomic regulation of lncRNA and Xist in X chromosome. Semin. Cell Dev. Biol. 90, 174–180. doi: 10.1016/j.semcdb.2018.07.013
Yan, X. T., Lu, J. M., Wang, Y., Cheng, X. L., He, X. H., Zheng, W. Z., et al. (2018). XIST accelerates neuropathic pain progression through regulation of miR-150 and ZEB1 in CCI rat models. J. Cell. Physiol. 233, 6098–6106. doi: 10.1002/jcp.26453
Yang, C., Wu, K., Wang, S., and Wei, G. (2018). Long non-coding RNA XIST promotes osteosarcoma progression by targeting YAP via miR-195-5p. J. Cell. Biochem. 119, 5646–5656. doi: 10.1002/jcb.26743
Yang, H., Zhang, X., Zhao, Y., Sun, G., Zhang, J., Gao, Y., et al. (2020). Downregulation of lncRNA XIST represses tumor growth and boosts radiosensitivity of neuroblastoma via modulation of the miR-375/L1CAM axis. Neurochem. Res. 9, 1–12.
Yang, J., Shen, Y., Yang, X., Long, Y., Chen, S., Lin, X., et al. (2019). Silencing of long noncoding RNA XIST protects against renal interstitial fibrosis in diabetic nephropathy via microRNA-93-5p-mediated inhibition of CDKN1A. Am. J. Physiol. Renal Physiol. 317, F1350–F1358.
Yang, L. G., Cao, M. Z., Zhang, J., Li, X. Y., and Sun, Q. L. (2020). LncRNA XIST modulates HIF-1A/AXL signaling pathway by inhibiting miR-93-5p in colorectal cancer. Mol. Genet. Genom. Med. 8:e1112.
Yang, M., and Wei, W. B. (2019). Long non-coding RNAs in retinoblastoma. Pathol. Res. Pract. 215, 1–6.
Yang, X., Zhang, S., He, C., Xue, P., Zhang, L., He, Z., et al. (2020). METTL14 suppresses proliferation and metastasis of colorectal cancer by down-regulating oncogenic long non-coding RNA XIST. Mol. Cancer 19, 46–63.
Yang, Z., Jiang, X. D., Jiang, X. F., and Zhao, H. Y. (2018). X-inactive-specific transcript: a long noncoding RNA with complex roles in human cancers. Gene 679, 28–35. doi: 10.1016/j.gene.2018.08.071
Yao, L., Yang, L., Song, H., Liu, T. G., and Yan, H. (2020). Silencing of lncRNA XIST suppresses proliferation and autophagy and enhances vincristine sensitivity in retinoblastoma cells by sponging miR-204-5p. Eur. Rev. Med. Pharmacol. Sci. 24, 3526–3537.
Yao, Y., Ma, J., Xue, Y., Wang, P., Li, Z., Liu, J., et al. (2015). Knockdown of long non-coding RNA XIST exerts tumor-suppressive functions in human glioblastoma stem cells by up-regulating miR-152. Cancer Lett. 359, 75–86. doi: 10.1016/j.canlet.2014.12.051
Yi, W., and Li, J. (2020). CRISPR-assisted detection of RNA-protein interactions in living cells. eLife 17, 685–688. doi: 10.1038/s41592-020-0866-0
Yin, Y., Lu, J. Y., Zhang, X., Shao, W., Xu, Y., Li, P., et al. (2020). U1 snRNP regulates chromatin retention of noncoding RNAs. Nature 580, 147–150. doi: 10.1038/s41586-020-2105-3
Yu, B., van Tol, H. T. A., and Stout, T. A. E. (2020). Initiation of X chromosome inactivation during bovine embryo development. Cells Basel 9, 1016–1034. doi: 10.3390/cells9041016
Yu, H., Xue, Y., Wang, P., Liu, X., Ma, J., Zheng, J., et al. (2017). Knockdown of long non-coding RNA XIST increases blood-tumor barrier permeability and inhibits glioma angiogenesis by targeting miR-137. Oncogenesis 6:e303. doi: 10.1038/oncsis.2017.7
Yu, J., Dong, W., and Liang, J. (2020). Extracellular vesicle-transported long non-coding RNA (LncRNA) X inactive-specific transcript (XIST) in serum is a potential novel biomarker for colorectal cancer diagnosis. Med. Sci. Monit. 26:e924448.
Yu, N. H., Liang, Y., Zhu, H., Mo, H. Y., and Pei, H. P. (2017). CsA promotes XIST expression to regulate human trophoblast cells proliferation and invasion through miR-144/Titin axis. J. Cell. Biochem. 118, 2208–2218. doi: 10.1002/jcb.25867
Yue, M. H., and Ogawa, Y. (2018). CRISPR/Cas9-mediated modulation of splicing efficiency reveals short splicing isoform of Xist RNA is sufficient to induce X-chromosome inactivation. Nucleic Acids Res. 46:e26. doi: 10.1093/nar/gkx1227
Zhang, J., Cao, Z., Ding, X., Wei, X., Zhang, X., Hou, J., et al. (2017). The lncRNA XIST regulates the tumorigenicity of renal cell carcinoma cells via the miR-302c/SDC1 axis. Int. J. Clin. Exp. Pathol. 10, 7481–7491.
Zhang, J., Li, W. Y., Yang, Y., Yan, L. Z., Zhang, S. Y., He, J., et al. (2019a). LncRNA XIST facilitates cell growth, migration and invasion via modulating H3 histone methylation of DKK1 in neuroblastoma. Cell Cycle 18, 1882–1892. doi: 10.1080/15384101.2019.1632134
Zhang, J. D., Gao, X. F., Yang, J., Fan, X. Y., Wang, W., Liang, Y. F., et al. (2019b). Xist intron 1 repression by transcriptional-activator-like effectors designer transcriptional factor improves somatic cell reprogramming in mice. Stem Cells 37, 599–608. doi: 10.1002/stem.2928
Zhang, M., Liu, H. Y., Han, Y. L., Wang, L., Zhai, D. D., Ma, T., et al. (2019c). Silence of lncRNA XIST represses myocardial cell apoptosis in rats with acute myocardial infarction through regulating miR-449. Eur. Rev. Med. Pharmacol. Sci. 23, 8566–8572.
Zhang, M., Wang, F., Xiang, Z., Huang, T., and Zhou, W. B. (2020a). LncRNA XIST promotes chemoresistance of breast cancer cells to doxorubicin by sponging miR-200c-3p to upregulate ANLN. Clin. Exp. Pharmacol. Physiol. 47, 1464–1472. doi: 10.1111/1440-1681.13307
Zhang, Q., Chen, B. Y., Liu, P., and Yang, J. (2018). XIST promotes gastric cancer (GC) progression through TGF-beta 1 via targeting miR-185. J. Cell. Biochem. 119, 2787–2796. doi: 10.1002/jcb.26447
Zhang, R., Wang, Z., Yu, Q., Shen, J., He, W., Zhou, D., et al. (2019d). Atractylenolide II reverses the influence of lncRNA XIST/miR-30a-5p/ROR1 axis on chemo-resistance of colorectal cancer cells. J. Cell. Mol. Med. 23, 3151–3165. doi: 10.1111/jcmm.14148
Zhang, R., and Xia, T. (2017). Long non-coding RNA XIST regulates PDCD4 expression by interacting with miR-21-5p and inhibits osteosarcoma cell growth and metastasis. Int. J. Oncol. 51, 1460–1470. doi: 10.3892/ijo.2017.4127
Zhang, X., Wu, H., Mai, C., and Qi, Y. (2020b). Long noncoding RNA XIST/miR-17/PTEN axis modulates the proliferation and apoptosis of vascular smooth muscle cells to affect stanford type A aortic dissection. J. Cardiovasc. Pharmacol. 76, 53–62. doi: 10.1097/fjc.0000000000000835
Zhang, X. T., Pan, S. X., Wang, A. H., Kong, Q. Y., Jiang, K. T., and Yu, Z. B. (2019e). Long non-coding RNA (lncRNA) X-inactive specific transcript (XIST) plays a critical role in predicting clinical prognosis and progression of colorectal cancer. Med. Sci. Monit. 25, 6429–6435. doi: 10.12659/msm.915329
Zhang, Y., Li, X., Gibson, A., Edberg, J., Kimberly, R. P., and Absher, D. M. (2020c). Skewed allelic expression on X chromosome associated with aberrant expression of XIST on systemic lupus erythematosus lymphocytes. Hum. Mol. Genet. 29, 2523–2534. doi: 10.1093/hmg/ddaa131
Zhang, Y., Liu, J., Gao, Y., Zhang, Z., and Zhang, H. (2019f). Long noncoding RNA XIST acts as a competing endogenous RNA to promote malignant melanoma growth and metastasis by sponging miR-217. Panminerva Med. 7, 63901–63912. doi: 10.18632/oncotarget.11564
Zhang, Y., Zhang, H., Zhang, W., Zhang, Y., Wang, W., and Nie, L. (2020d). LncRNA XIST modulates 5-hydroxytrytophan-induced visceral hypersensitivity by epigenetic silencing of the SERT gene in mice with diarrhea-predominant IBS. Cell. Signal. 73, 109674–109683. doi: 10.1016/j.cellsig.2020.109674
Zhang, Y., Zhu, Y., Gao, G., and Zhou, Z. (2019g). Knockdown XIST alleviates LPS-induced WI-38 cell apoptosis and inflammation injury via targeting miR-370-3p/TLR4 in acute pneumonia. Cell Biochem. Funct. 37, 348–358. doi: 10.1002/cbf.3392
Zhang, Y. L., Li, X. B., Hou, Y. X., Fang, N. Z., You, J. C., and Zhou, Q. H. (2017). The lncRNA XIST exhibits oncogenic properties via regulation of miR-449a and Bcl-2 in human non-small cell lung cancer. Acta Pharmacol. Sin. 38, 371–381. doi: 10.1038/aps.2016.133
Zhang, Y. X., Zhu, Z. B., Huang, S. Z., Zhao, Q., Huang, C. J., Tang, Y. H., et al. (2019h). lncRNA XIST regulates proliferation and migration of hepatocellular carcinoma cells by acting as miR-497-5p molecular sponge and targeting PDCD4. Cancer Cell Int. 19, 198–211.
Zhao, C. H., Bai, X. F., and Hu, X. H. (2020). Knockdown of lncRNA XIST inhibits hypoxia-induced glycolysis, migration and invasion through regulating miR-381-3p/NEK5 axis in nasopharyngeal carcinoma. Eur. Rev. Med. Pharmacol. 24, 2505–2517.
Zhao, H., Wan, J., and Zhu, Y. (2020). Carboplatin inhibits the progression of retinoblastoma through IncRNA XIST/miR-200a-3p/NRP1 axis. Drug Design Dev. Ther. 14, 3417–3427. doi: 10.2147/dddt.s256813
Zhao, L., Zhao, Y., He, Y., Li, Q., and Mao, Y. (2018). The functional pathway analysis and clinical significance of miR-20a and its related lncRNAs in breast cancer. Cell. Signal. 51, 152–165. doi: 10.1016/j.cellsig.2018.08.004
Zhao, Q., Lu, F., Su, Q. L., Liu, Z., Xia, X. M., Yan, Z. Z., et al. (2020). Knockdown of long noncoding RNA XIST mitigates the apoptosis and inflammatory injury of microglia cells after spinal cord injury through miR-27a/Smurf1 axis. Neurosci. Lett. 715, 134649–134658. doi: 10.1016/j.neulet.2019.134649
Zhao, Y., Li, S., Xia, N., Shi, Y., and Zhao, C. M. (2018). Effects of XIST/miR-137 axis on neuropathic pain by targeting TNFAIP1 in a rat model. J. Cell. Physiol. 233, 4307–4316. doi: 10.1002/jcp.26254
Zheng, C., Bai, C., Sun, Q., Zhang, F., Yu, Q., Zhao, X., et al. (2020). Long noncoding RNA XIST regulates osteogenic differentiation of human bone marrow mesenchymal stem cells by targeting miR-9-5p. Mech. Dev. 162, 103612–103621. doi: 10.1016/j.mod.2020.103612
Zheng, R., Lin, S., Guan, L., Yuan, H., Liu, K., Liu, C., et al. (2018). Long non-coding RNA XIST inhibited breast cancer cell growth, migration, and invasion via miR-155/CDX1 axis. Biochem. Biophys. Res. Commun. 498, 1002–1008. doi: 10.1016/j.bbrc.2018.03.104
Zheng, W., Li, J., Zhou, X., Cui, L., and Wang, Y. (2020). The lncRNA XIST promotes proliferation, migration and invasion of gastric cancer cells by targeting miR-337. Arab J. Gastroenterol. 21, 199–206. doi: 10.1016/j.ajg.2020.07.010
Zhou, H., Sun, L. N., and Wan, F. S. (2019a). Molecular mechanisms of TUG1 in the proliferation, apoptosis, migration and invasion of cancer cells. Oncol. Lett. 18, 4393–4402.
Zhou, H. Q., Xian, W., Zhang, Y. X., Chen, G., Zhao, S., Chen, X., et al. (2018). Trends in incidence and associated risk factors of suicide mortality in patients with non-small cell lung cancer. Cancer Med. 7, 4146–4155. doi: 10.1002/cam4.1656
Zhou, J., Li, D., Yang, B. P., and Cui, W. J. (2020). LncRNA XIST inhibits hypoxia-induced cardiomyocyte apoptosis via mediating miR-150-5p/Bax in acute myocardial infarction. Eur. Rev. Med. Pharmacol. 24, 1357–1366.
Zhou, K., Li, S., Du, G., Fan, Y., Wu, P., Sun, H., et al. (2019b). LncRNA XIST depletion prevents cancer progression in invasive pituitary neuroendocrine tumor by inhibiting bFGF via upregulation of microRNA-424-5p. OncoTargets Ther. 12, 7095–7109. doi: 10.2147/ott.s208329
Zhou, K., Yang, J., Li, X., and Chen, W. (2019c). Long non-coding RNA XIST promotes cell proliferation and migration through targeting miR-133a in bladder cancer. Exp. Ther. Med. 18, 3475–3483.
Zhou, T., Qin, G., Yang, L., Xiang, D., and Li, S. (2019d). LncRNA XIST regulates myocardial infarction by targeting miR-130a-3p. J. Cell. Physiol. 234, 8659–8667. doi: 10.1002/jcp.26327
Zhou, X. Y., Xu, X. H., Gao, C., and Cui, Y. S. (2019e). XIST promote the proliferation and migration of non-small cell lung cancer cells via sponging miR-16 and regulating CDK8 expression. Am. J. Transl. Res. 11, 6196–6206.
Zhu, H., Zheng, T., Yu, J., Zhou, L. X., and Wang, L. (2018). LncRNA XIST accelerates cervical cancer progression via upregulating Fus through competitively binding with miR-200a. Biomed. Pharmacother. 105, 789–797. doi: 10.1016/j.biopha.2018.05.053
Zhu, J., Zhang, R., Yang, D., Li, J., Yan, X., Jin, K., et al. (2018). Knockdown of long non-coding RNA XIST inhibited doxorubicin resistance in colorectal cancer by upregulation of miR-124 and downregulation of SGK1. Cell. Physiol. Biochem. 51, 113–128. doi: 10.1159/000495168
Zhuang, L. K., Yang, Y. T., Ma, X., Han, B., Wang, Z. S., Zhao, Q. Y., et al. (2016). MicroRNA-92b promotes hepatocellular carcinoma progression by targeting Smad7 and is mediated by long non-coding RNA XIST. Cell Death Dis. 7:e2203. doi: 10.1038/cddis.2016.100
Zou, L., Chen, F. R., Xia, R. P., Wang, H. W., Xie, Z. R., Xu, Y., et al. (2020). Long noncoding RNA XIST regulates the EGF receptor to promote TGF-beta1-induced epithelial-mesenchymal transition in pancreatic cancer. Biochem. Cell Biol. 98, 267–276. doi: 10.1139/bcb-2018-0274
Zuo, K., Zhao, Y., Zheng, Y., Chen, X. L., Du, S., and Liu, Q. (2019). Long non-coding RNA XIST promotes malignant behavior of epithelial ovarian cancer. OncoTargets Ther. 12, 7261–7267. doi: 10.2147/ott.s204369
Glossary
lncRNAs, Long non-coding RNAs; lncRNA Xist, Long non-coding RNA X-inactive specific transcript; XIC, X chromosome inactivation center; ceRNA, competing endogenous RNA; XCI, X-chromosome inactivation; Xa, active X chromosome; Xi, inactive X chromosome; Ftx, Ftx transcript, Xist regulator; Jpx, Jpx transcript, Xist activator; Tsix, Tsix transcript, Xist antisense RNA; OCT4, also known as Pou5f1; CTCF, CCCTC-binding factor; PRC1 and PRC2, Polycomb repressive complexes (PRCs) -PRC1 and -PRC2; RNA Pol II, RNA polymerase II; hnRNPU (also known as SAF-A), heterogeneous nuclear protein U; ATRX, ATRX chromatin remodeler; hnRNPK, Heterogeneous nuclear ribonucleoprotein K; SHARP, SPEN family transcriptional repressor; HDAC3, histone deacetylase 3; LBR, lamin B receptor; Airn, antisense of IGF2R non-protein coding RNA; Kcnq1ot1, KCNQ1 opposite strand/antisense transcript 1; RBM15, RNA binding motif protein 15; WTAP, WT1 associated protein; U1 snRNP, U1 small nuclear ribonucleoprotein; Rsx, RNA-on-the-silent X; NANOG, Nanog homeobox; SOX2, SRY-box transcription factor 2; PRDM14, PR/SET domain 14; REX1, ZFP42 zinc finger protein; RNF12, RING-H2 finger protein; CdK8, cyclin dependent kinase 8; AR, Androgen Receptor; BCSC, bladder cancer stem cell; PD, Platycodin D; BRCA1, Breast Cancer 1 protein; EMT, epithelial mesenchymal transition; CDX1, caudal-type homeobox 1; TNBC, Triple-negative breast cancer; ESA, epithelium-specific antigen; RELA, NF-κB subunit; BCSCs, Breast cancer stem cells; PHLPP1, PH domain and leucine-rich repeat phosphatase 1; ZEB1, zinc finger E-box binding homeobox 1; EZH2, enhancer of zeste homolog 2; MAPK1, mitogen-activated protein kinase 1; NRP-2, neuropilin-2; HIF-1A, hypoxia inducible factor 1, alpha subunit; METTL14, methyltransferase-like14; YTHDF2, YTHDF proteins 2; SGK1, serum and glucocorticoid-inducible kinase 1; ROR1, receptor-tyrosine-kinase-like orphan receptor 1; Smurf1, smad ubiquitination regulatory factor 1; FOXC1, forkhead box C1; ZO-2, zonula occludens 2; Rac1, Rac family small GTPase 1; ASCT2 and SLC1A5, alanine-, serine-, and cysteine-preferring transporter 2; PI3K, phosphatidylinositol-4,5-bisphosphate 3-kinase; IRS1, insulin receptor substrate 1; SOX4, Y-related high-mobility group box 4; TMZ, Temozolomide; MMR, DNA mismatch repair; HBV, hepatitis B virus; HCV, hepatitis C virus; HMGB1, high-mobility group box-1; PDK1, pyruvate dehydrogenase kinase 1; TNM, tumor-node-metastasis; PDCD4, programmed cell death 4; PTEN, phosphatase and tensin homolog; E2F3, E2F transcription factor 3; ADAM17, a disintegrin and metalloproteinase 17; NEK5, NIMA related kinase 5; TAM, tumor-associated macrophage; TCF-4T, T-cell-specific transcription factor 4; MDM2, mouse double minute clone 2; BAG-1, bcl-2 associated athanogene-1; NSCLC, Non-small cell lung carcinoma; LARP1, La-related protein 1; CBLL1, Casitas B-lineage proto-oncogene like 1; TGF-β1, Transforming growth factor β; ZEB2, zinc finger E-box binding homeobox 2; ATG7, autophagy associated gene 7; PUMA, p53 upregulated modulator of apoptosis; NF-kB, nuclear factor-kappa B; YAP, Hippo/yes-associated protein; EGFR, epidermal growth factor receptor; iASPP, inhibitor of apoptosis-stimulating protein of p53; CDK1, Cyclin-dependent kinase 1; VEGF, vascular endothelial growth factor; STAT3, signal transducer and activator of transcription 3; MACC1, MET transcriptional regulator; MDR1, multidrug resistance gene 1; MRP1, multi-drug resistance protein 1; bFGF, basic fibroblast growth factor; SDC1:Syndecan-1; TGCT, testicular germ cell tumor; CAD, coronary artery disease; MI, myocardial infarction; HF, heart failure; TLR2, toll-like receptor 2; PDE4D, Phosphodiesterase 4D; CB2R, cannabinoid receptor type II; LR5, toll-like receptor 5; NFAIP1, tumor necrosis factor alpha-induced protein 1; CCI, chronic constriction injury; AD, Alzheimer’s disease; OA, Osteoarthritis; PDLSCs, periodontal ligament stem cells; OP, osteoporosis; ALP, alkaline phosphatase; MMP-13, matrix metalloproteinase 13; ADAMTS5, ADAM metallopeptidase with thrombospondin type 1 motif 5; OPN, overexpression of osteopontin; TLRS, Toll−like receptors; CRPS, Complex regional pain syndrome; YY1, Yin-Yang 1; SCI, Spinal cord injury; CCSCI, Compressive Spinal Cord Injury; CDKN1A, cyclin-dependent kinase inhibitor 1A; PFOS, Perfluorooctane sulfonate; SOX7, SRY-box 7; PGD, Primary graft dysfunction; NET, neutrophil extracellular trap; NOD2, Nucleotide-Binding Oligomerization Domain 2; COL1A1, collagen 1 alpha 1.
Keywords: long non-coding RNA, lncRNA Xist, cancer, disease, X-chromosome inactivation, X-chromosome inactivation center
Citation: Wang W, Min L, Qiu X, Wu X, Liu C, Ma J, Zhang D and Zhu L (2021) Biological Function of Long Non-coding RNA (LncRNA) Xist. Front. Cell Dev. Biol. 9:645647. doi: 10.3389/fcell.2021.645647
Received: 23 December 2020; Accepted: 12 May 2021;
Published: 10 June 2021.
Edited by:
Aamir Ahmad, University of Alabama at Birmingham, United StatesReviewed by:
Joanna Sztuba-Solinska, Auburn University, United StatesFrancesco Esposito, Istituto per l’Endocrinologia e l’oncologia “Gaetano Salvatore”, Consiglio Nazionale delle Ricerche (CNR), Italy
Copyright © 2021 Wang, Min, Qiu, Wu, Liu, Ma, Zhang and Zhu. This is an open-access article distributed under the terms of the Creative Commons Attribution License (CC BY). The use, distribution or reproduction in other forums is permitted, provided the original author(s) and the copyright owner(s) are credited and that the original publication in this journal is cited, in accordance with accepted academic practice. No use, distribution or reproduction is permitted which does not comply with these terms.
*Correspondence: Dongyi Zhang, ZG9uZ3lpemhhbmdAbnVkdC5lZHUuY24=; Lingyun Zhu, bGluZ3l1bnpodUBudWR0LmVkdS5jbg==