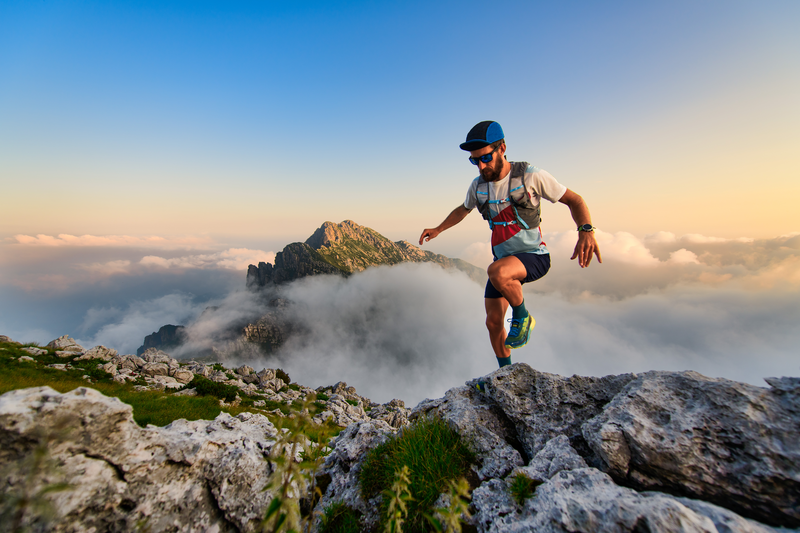
95% of researchers rate our articles as excellent or good
Learn more about the work of our research integrity team to safeguard the quality of each article we publish.
Find out more
ORIGINAL RESEARCH article
Front. Cell Dev. Biol. , 22 April 2021
Sec. Molecular and Cellular Oncology
Volume 9 - 2021 | https://doi.org/10.3389/fcell.2021.641978
This article is part of the Research Topic Liquid Biopsy View all 27 articles
Purpose: Monocarboxylate transporter 4 (MCT4) can influence the amount of lactate in the tumor microenvironment and further control cancer cell proliferation, migration, and angiogenesis. We investigated for the first time the expression of MCT4 in circulating tumor cells (CTCs) derived from early stage Non-Small Cell Lung Cancer patients (NSCLC) and whether this is associated with clinical outcome.
Experimental Design: A highly sensitive RT-qPCR assay for quantification of MCT4 transcripts was developed and validated and applied to study MCT4 expression in CTC isolated through the Parsortix size-dependent microfluidic device from 53 and 9 peripheral blood (PB) samples of NSCLC patients at baseline (pre-surgery) and at relapse, respectively, as well as the “background noise” was evaluated using peripheral blood samples from 10 healthy donors (HD) in exactly the same way as patients.
Results: MCT4 was differentially expressed between HD and NSCLC patients. Overexpression of MCT4 was detected in 14/53 (26.4%) and 3/9 (33.3%) patients at baseline and at progression disease (PD), respectively. The expression levels of MCT4 was found to increase in CTCs at the time of relapse. Kaplan-Meier analysis showed that the overexpression of MCT4 was significantly (P = 0.045) associated with progression-free survival (median: 12.5 months, range 5–31 months).
Conclusion: MCT4 overexpression was observed at a high frequency in CTCs from early NSCLC patients supporting its role in metastatic process. MCT4 investigated as clinically relevant tumor biomarker characterizing tumor aggressiveness and its potential value as target for cancer therapy. We are totally convinced that MCT4 overexpression in CTCs merits further evaluation as a non-invasive circulating tumor biomarker in a large and well-defined cohort of patients with NSCLC.
Lung cancer remains the most commonly diagnosed cancer and the leading cause of cancer death globally. Non-Small Cell Lung Cancer (NSCLC) is the common histological subtype of the disease, accounting for 85% of all lung cancer diagnoses (Molina et al., 2008). Almost 45% of patients with operable early stage NSCLC relapse within the first 18 months and the probability of patients’ survival depends on the possibility of early detection of relapse (Siegel et al., 2015). The use of targeted therapy such as tyrosine kinase inhibitors (TKIs) and/or immunotherapy has led to unprecedented survival benefits in selected patients (Wu et al., 2020). Moreover, the use of therapeutic modalities against the Minimal Residual Disease (MRD) and before the development of clinically detectable metastatic lesions seems to be emerged as an important advancement in the management of early stage NSCLC. Indeed, it has been recently reported that the administration of Osimertinib in the context of adjuvant treatment in resected EGFR mutant NSCLC significantly reduced the relapse rate and prolonged the overall survival (Wu et al., 2020). The possibility of probing the early detection of NSCLC via a blood draw –termed as “liquid biopsy”- has attracted remarkable interest among the oncology community (Pawlikowska et al., 2019; Tamminga et al., 2019; Frick et al., 2020). Different tumor-derived components can be isolated from blood, including Circulating Tumor Cells (CTCs), circulating tumor DNA (ctDNA), cellfree RNA (cfRNA), exosomes, and tumor-educated platelets (TEP), providing information about the dynamic tumor profile over time (Heidrich et al., 2020). The FDA has approved the use of ctDNA for the response prediction and monitoring development resistance to EGFR TKI therapy in NSCLC patients (Wang et al., 2017; Zhang et al., 2017).
The application of liquid biopsy in NSCLC is being used for the diagnosis, prognosis and monitoring of disease based on signature molecular markers (Luo et al., 2018). However, the clinical significance of CTC enumeration in NSCLC is yet to be established since due to the EMT process, EpCAM-independent methods are required in order to isolate and characterize CTCs from NSCLC patients. Indeed, molecular characterization of CTCs got potential for the improvement of our knowledge in the field of metastatic process, the identification of new treatment predictive markers and stratification of patients into prognostic groups.
The CellSearch® system, which is the only FDA approved assay for CTC detection in metastatic breast, colorectal and prostate cancers but is able to identify CTCs only in about 23–39% of stage IV NSCLC patients (Krebs et al., 2012). Detection of CTCs in NSCLC has been challenging due to the rarity of these cells in circulation and the presence of non-epithelial characteristics due to epithelial mesenchymal transition (EMT) (Lianidou et al., 2015). EMT is characterized by down-regulation of epithelial markers, such as cytokeratin’s (CKs), and up-regulation of mesenchymal markers like Vimentin (VIM).
Three metabolic properties of cancer cells (a) glucose uptake, (b) lactate secretion and (c) oxygen availability constitute the Warburg effect which attracts the interest of the scientific community for a many years (Warburg, 1956; DeBerardinis and Chandel, 2020). Moreover, Reprogramming Energy Metabolism is an Emerging Hallmark for cancer (Hanahan and Weinberg, 2011). Monocarboxylic acids including lactate play a crucial role in cellular metabolism, and their regulation has become a new target for understanding the pathogenesis of abnormal cellular processes such as oncogenesis (Poole and Halestrap, 1993; Halestrap and Price, 1999). These acids must be rapidly transported across the plasma membrane of cells and this transportation is mediated by proton-linked monocarboxylate transporters (MCTs). MCT4 is highly expressed in glycolytic tissues such as white skeletal muscle fibers, astrocytes, white blood cells, and chondrocytes, and it plays an important role in lactate efflux from cells (Meredith and Christian, 2008). MCT4 can control the amount of lactate in the tumor microenvironment regulating cancer cell proliferation, migration, and angiogenesis. MCT4 expression in the tumor microenvironment has been associated with decreased overall survival (OS) (Nakayama et al., 2012; Baek et al., 2014; Doyen et al., 2014; Zhu et al., 2014), and decreased disease-free survival (DFS) in cancer patients (Curry et al., 2013; Doyen et al., 2014; Zhu et al., 2014). Moreover, MCT4 has been proposed as a new therapeutic target in several tumor types including NSCLC (Kim et al., 2018; Kuo et al., 2020; Puri and Juvale, 2020).
In the current study, we first developed and validated a highly sensitive RT-qPCR assay for the quantification of MCT4 transcripts, and report for the first time that MCT4 is overexpressed in CTC isolated from patients with early NSCLC. We further evaluated whether MCT4 overexpression in CTC is associated with DFS. Our findings indicate that MCT4 overexpression in CTCs should be prospectively evaluated as a potential biomarker for early relapse in patients with resected NSCLC.
Fifty three patients with early stage NSCLC were enrolled in the study and 62 peripheral blood samples (25 mL in EDTA tubes) from these patients were prospectively collected; 53 samples were obtained at baseline (pre-surgery), and 9 samples at the time of relapse whilst 10 peripheral blood samples from healthy donors (HD) were used as controls. For 12 patients that have been randomly chosen, 10 mL peripheral blood from the same blood draws were used to perform CTCs’ IF analysis. The first 5 mL of blood were discarded in order, to avoid contamination from skin epithelial cells. All patients gave a written informed consent to participate in the study, which was approved by the Ethics and Scientific Committee of Thoracic Diseases General Hospital Sotiria. All HD had no known illness or fever at the time of draw, no history of malignant disease, and were ≥35 years old. Clinical samples were collected from 32 men and 21 women (median age: 65.2 years, range: 39–81) and all patients were diagnosed with operable (stage IA–IIIA) NSCLC. 23 patients were diagnosed with adenocarcinoma (ADC), 26 with Squamous Cell Carcinoma (SCC) and 4 with undifferentiated (NOS) NSCLC. Thirty-eight (71.6%) patients had no evidence of disease infiltration in resected lymph nodes (N0 disease). The main patients’ characteristics are summarized in Supplementary Table 1.
Micro-fluidic device named as Parsortix (ANGLE plc, United Kingdom) (Hvichia et al., 2016) was used to for the isolation of CTCs from 25 mL whole blood. A microscope slide sized disposable cassette was used for the division of blood components (Chudziak et al., 2016; Porras et al., 2018). After that, CTCs were collected in a total volume of 200 μL of PBS into tubes. The isolation of total RNA from enriched CTCs was performed by TRIZOL-LS (Thermo Fisher Scientific, United States), and finally cDNA synthesis of the extracted total RNA was carried out as previously described (Strati et al., 2017; Zavridou et al., 2018).
For the isolation of CTCs with the ISET (Isolation by SizE of Tumor cells) platform (Rarecells Diagnostics, France) 10 mL of peripheral blood was used. At first, each sample was diluted in 1:10 ISET buffer (Rarecells Diagnostics) and was incubated for 10 min at room temperature (RT). 100 mL of the diluted sample was filtered using a depression tab adjusted at −10 kPa. Finally, the membrane was dried for 2 h at RT and stored at −20°C. Each membrane spot was used for identification of CTCs after immunostaining and fluorescence microscopy analysis (Kallergi et al., 2016).
In silico study for the design of the primers and TaqMan probes for MCT4 and B2M (used as a reference gene) was carefully performed using Primer Premier 5.0 software. In order to ensure the specificity of all primers and probe sequences BLAST analysis was carried out (NCBI, nucleotide BLAST). Moreover, we carefully designed our primers and probes to completely avoid primer–dimer formation, false priming sites, formation of hairpin structures and hybridization to genomic DNA. The sequences of primers and probes are available in Supplementary Table 3.
RT-qPCR was performed in the LightCycler® 480 instrument (Roche, Germany). Detailed optimization experiments were carried out (results not shown). The amplification reaction mixture for MCT4 contained 2 μL of the PCR synthesis buffer (5×), 1 μL MgCl2 (25 mM), 0.2 μL dNTPs (10 mM), 0.15 μL BSA (10 μg/μL), 0.1 μL Hot-Start DNA polymerase (Promega), 0.3 μL of forward and reverse primer (10 μM), 1 μL hydrolysis probe (3 μM) and H2O to a final volume of 10 μL while the amplification reaction mixture for B2M contained 1 μL of PCR synthesis buffer (5×), 1.2 μL MgCl2 (25 mM), 0.15 μL dNTPs (10 mM), 0.3 μL BSA (10 μg/μL), 0.1 μL Hot-Start DNA polymerase (Promega), 0.25 μL of forward and reverse primer (10 μM), 0.83 μL hydrolysis probe (3 μM) and H2O to a final volume of 10 μL. Each experimental procedure included one positive and one negative control. cDNA from MCF-7 cell line was used as a positive control. In order to ensure that amplification of gDNA was completely avoided, four genomic DNAs at high concentrations were used as templates. None of these DNA samples were amplified. B2M was used as a reference gene for RT-qPCR. I n addition, single RT-qPCR was performed for epithelial markers (CK-19, CK-8, CK-18) and for EMT markers (TWIST-1 and VIM) as previously described (Markou et al., 2018; Strati et al., 2019).
RT-qPCR data for MCT4 expression were normalized in respect to B2M expression in the same cDNAs, using the 2–ΔΔCt approach (Livak and Schmittgen, 2001). CTCs isolated through micro-fluidics device are not 100% pure; since the presence of co-isolated PBMC in CTC fractions could affect the specificity of the MCT4 assay, we evaluated this “background noise” by analyzing peripheral blood samples from 10 HD in exactly the same way as patients. We estimated a cut-off based on MCT4 normalized expression in respect to B2M expression in this control group. Using this approach we defined a sample as positive for MCT4 overexpression (MCT4 positive) based on the fold change of MCT4 expression in the CTC fraction in respect to the corresponding fraction in the group of the 10 HD.
ISET filters were washed with PBS and permeabilized with 0.5% Triton-X-100 in PBS for 10 min at 20°C. Non-specific antibody binding was blocked by incubation with 10% FBS in PBS for 1 h at 20°C. To identify CK, filters were incubated with a cocktail of mouse anti-CK7 antibody (Invitrogen, United States; 1:100 dilution in PBS with 1% FBS) and mouse A45 B/B3 anti-human cytokeratin (Amgen, United States); 1:70 dilution in PBS with 1% FBS) for 1 h at 20°C. Filters were washed (3 × 5 min) with PBS and then incubated with goat anti-mouse Alexa Fluor® 488 secondary antibody (Life Technologies, United States; 1:500 dilution in PBS with 1% FBS) for 45 min at 20°C. Filters were washed (3 × 5 min) with PBS and then incubated with rabbit anti-VIM antibody (Abcam, 1:500 dilution in PBS with 1% FBS) for 1 h at 20°C. After washing (3 × 5 min) with PBS, filters were incubated with goat anti-rabbit Alexa Fluor® 555 secondary antibody (Life Technologies; 1:600 dilution in PBS with 1% FBS) for 45 min at 20°C. Filters were washed (3 × 5 min) with PBS and mounted in Prolong anti-fade mounting medium containing DAPI (Cell signaling, United States). Slides were stored at −20°C before confocal imaging.
Cytospins with H 1299 cells were used as controls (Supplementary Figure 1). One positive control (stained with all antibodies) and two negative controls (omitting one of the first antibodies) were used. Slides were washed with PBS and cells were fixed/permeabilized with ice-cold acetone/methanol (9:1) for 20 min and, thereafter, non-specific antibody binding was blocked by incubation with 5% FBS overnight at 4°C.
All imaging used a Leica (Germany) TCS SP8 confocal microscope with a ×40 oil-immersion objective. In all dual-labeling analyses we confirmed, with the use of the controls, that there was no bleed-through between the two wavelengths. Cyto-morphological criteria proposed by Meng et al. (2004) (such as high nuclear/cytoplasmic ratio, etc.) were used in order to characterize a CK-positive cell as a CTC.
SPSS program was used for the statistical analysis of our data (SPSS Statistics 25.0, company, Armonk, NY, United States). In order to estimate the differences between groups chi-square test of independence or Fisher exact test (SPSS, version 25.0) was used. Kaplan–Meier analysis used to evaluate “time-to-event” data. Parametric and non-parametric tests were used to compare continuous variables between groups. Non-parametric tests were used to analyze the relationship between MCT4 expression and various clinicopathological characteristics for each patient (the Mann–Whitney and χ2-test between 2 groups and the Kruskall Wallis test for 3 or more groups). All P-values are two-sided. A level of P < 0.05 is considered statistically significant unless specified otherwise.
The outline of the study is shown in Figure 1.
Figure 1. Outline of the experimental procedure. (A) Gene expression profiling of CTCs. (B) Phenotypic evaluation of CTCs.
CTCs were detected in 14 of 16 (87.5%) patients with early stage NSCLC. The mean and median numbers of CTCs per patient were 4.6 and 1 (range, 0–20), respectively. The absolute number of CTCs per patient for each distinct phenotype is shown in Table 1. Double-staining experiments (VIM/CK) and confocal laser scanning analysis revealed an heterogeneous expression of CK and VIM. Indeed, CTCs had low expression of CKs (lower than the CK expression in control H 1299 cells) (Supplementary Figure 2); VIM+CKlow CTCs could be detected in 12 out of 16 patients (75%) and VIM–CKlowCTCs in 37.5% (6 of 16) of patients.
cDNAs isolated from clinical samples were further analyzed for epithelial markers (CK8, CK18, CK19) using our previously developed and analytically validated RT-qPCR assays. At baseline, CK8 expression was detected in 8/53 (15.1%), while CK18 and CK19 were detected in 14/53 (26.4%) and 14/53 (26.4%), respectively. In total, in 27/53 (50.9%) samples were detected at least one CK marker (Figure 2).
Figure 2. Heat map of CK-8/CK-18/CK-19 epithelial markers, VIM, TWIST-1, and MCT4 as quantified by RT-qPCR.
The expression of EMT markers TWIST-1 and VIM was also evaluated in the same cDNAs, using our previously developed and analytically validated RT-qPCR assay (Markou et al., 2018; Strati et al., 2019). TWIST-1 was overexpressed in 11/53 (20.7%) of samples, while VIM in 35/53 (66%) of samples. In total, at least one EMT marker was detected in 41/53 (77.3%) samples (Figure 2).
Overexpression of MCT4 transcripts could be detected in 14/53 (26.4%) patient samples. The evaluation of the differences in the expression of MCT4 in CTC between HD and early stage NSCLC patients revealed a significantly higher expression of MCT4. As can be seen in Figure 3, the overexpression of MCT4 differed significantly in patient’s samples compared to HD’s samples (P = 0.036; Figure 3). For a subgroup of these patients (n = 9), peripheral blood samples were available both at baseline and at the time of relapse. In this group, MCT4 overexpression was observed in 6/18 (33.3%) CTC fraction samples [in 3/9 (33.3%) at baseline and 3/9 (33.3%) at disease progression. In two of these three cases both CTC fractions at baseline and time of relapse were found to be positive for MCT4 overexpression. There was one case where MCT4 overexpression was detected only in the baseline, but not at progression disease whereas there was one case where the sample was found to be positive for MCT4 overexpression in the time of relapse. Moreover, there was only one case where the patient was identified to decrease the expression levels of MCT4 at the time of relapse in respect to the baseline sample but it seemed to remain overexpressed (Figure 4).
Figure 3. Expression levels of MCT4 in CTC fractions of HD (n = 10) and early stage NSCLC patients (n = 53).
Figure 4. Relative fold change (2–ΔΔCq) of MCT4 in CTCs of 9 pairs of early stage NSCLC patient samples at the baseline and at the time of relapse.
The correlation between the expression levels of MCT4 in CTCs and prognosis was further analyzed. During of follow-up period (median: 12.5, range: 5–31 months) 14/53 (26.4%) NSCLC patients developed metastases. Kaplan–Meier survival analysis demonstrated that patients who overexpressed MCT4 (n = 14) had a significantly shorter DFI than those without MCT4 overexpression (P = 0.045; Figure 5). Moreover, as can been seen in Supplementary Table 2, MCT4 overexpression was not correlated with gender, smoking history, tumor size, lymph node status, and stage of disease in the population studied (P > 0.05).
Figure 5. Kaplan–Meier estimates of DFI of early stage NSCLC patients in respect to MCT4 overexpression in CTCs.
Increased rates of glycolysis is one of the molecular mechanisms that has been studied for initiation of cancer cell metastasis (Wang et al., 2015). Warburg et al. (1927) have shown that cancer cells have an increased rate of glucose uptake and favored production of lactate, even in the normoxic conditions. It is well-known that cancer cells in order to avoid apoptosis and cellular acidosis export the intracellular lactate and monocarboxylate transporters (MCTs) play a critical role in this exportation (Halestrap and Price, 1999). This mainly performed through the action of MCT1 and MCT4 that control intracellular pH in cells relying on high glycolysis rates, such as red blood cells, skeletal muscle cells and tumor cells (Halestrap and Price, 1999; Halestrap, 2013). Although the expression of MCT4 in the tumor microenvironment has been associated with poor prognosis (Nakayama et al., 2012; Baek et al., 2014) and MCT4 has been studied as a new therapeutic target (Kim et al., 2018; Puri and Juvale, 2020). Immunofluoresence has been used for the detection of MCT 1 and MCT4 in cancer patient’s CTC by Kershaw et al. (2015) but so far there is no any other reference on MCT4 expression at the mRNA level on CTCs.
In the present study, we evaluated for the first time MCT4 expression in CTC fractions isolated by a size- based EpCAM independent technology (Parsortix) from early stage NSCLC patients using a highly sensitive and specific RT-qPCR assay. MCT4 plays a critical role in energy production, tumor proliferation and invasion since lactic acid is secreted by cancer cells and acidify the tumor microenvironment (Shu et al., 2016). The inhibition of MCT4 protein has been suggested as a novel therapeutic approach for many malignancies including NSCLC (Kuo et al., 2020). Bioinformatic analyses of the TCGA datasets demonstrated that MCT4 is elevated in 9% of lung adenocarcinomas cases. Our findings indicate that MCT4 is overexpressed at 14/53 (26.4%) on CTC fractions of early stage NSCLC patients. A similar positivity rate (26.4%) was also detected for epithelial markers (CK18 and CK19) while the positivity rate was increased (66%) regarding the EMT marker VIM indicating that in NSCLC a large fraction of CTCs is under EMT status which is further supported by the observation that 13 out of 14 (92.8%) of MCT4 overexpressed samples were found to express at least one EMT marker; conversely, the correspondent proportion of blood samples harboring at least one epithelial marker was 50%. These findings are consistent with previous studies demonstrating that the vast majority of CTC in NSCLC patients express EMT markers (Allard et al., 2004; Lecharpentier et al., 2011; Zhang et al., 2019).
The expression of MCT4 in the CTC fraction of patients was significantly higher than that observed in the corresponding “PBMC” fraction of HD (P = 0.036). Moreover, a higher expression level of MCT4 in CTC were observed at the time of tumor relapse. This finding, which is in agreement with the reported increased tumoral expression of MCT4 when the tumor progress to higher grade or metastasis, strongly which suggests that MCT4 expression is associated with the migration and/or invasion of cancer cells (Gerlinger et al., 2012; Choi et al., 2014; Kim et al., 2015).
According to our results, overexpression of MCT4 had a prognostic implication for early stage NSCLC patients, since it was associated with significantly reduced DFI; but conversely, there was no correlation with other patients’ clinic-pathological characteristics. On the contrary, neither the expression of epithelial markers nor EMT markers provided prognostic information for NSCLC patients. However, these observations and conclusions have to be been taken with cautious because of the relatively small number of enrolled patients in the study and the low number of observed relapses during the follow-up period and need further confirmation in larger future studies. In a previous study, Ruan et al. (2017) reported that the expression MCT-4protein in the primary tumor cells was significantly associated with the depth of tumoral invasion (P = 0.034) and with a decreased overall survival (P = 0.001).
In recent years, MCT4 inhibitors are still in the discovery phase. Very recently Kuo et al. (2020) suggested a new therapeutic approach for the control of MCT4 in the aerobic glycolysis-preference NSCLC cell subtype. Moreover, the elimination of lactace secretion could be more effective by developing drugs that co-inhibit MCT1 and MCT4. The experimental approach in vivo or in vitro establishes that not only the inhibition of MCTs can be useful, but also MCTs could serve as vehicles for new anticancer drugs (Baltazar et al., 2014).
In conclusion, we show that overexpression MCT4 in CTCs has prognostic significance in early stage NSCLC patients. It would be valuable to extend this study in a prospective large study as well as in different cancers, since most studies so far are based on the expression of MCT4 in paired fresh frozen tissues. Taking into account the importance of MCT4 in cancer, its promising potential as a new specific liquid biopsy prognostic biomarker as well as molecular targets for the development of novel cancer therapeutics, we strongly believe that our results will be of importance for both clinical researchers and those who design novel cancer therapeutics.
The data that support the findings of this study are available from the corresponding author (AM), upon reasonable request.
The studies involving human participants were reviewed and approved by Ethics and Scientific Committee of Thoracic Diseases General Hospital Sotiria. The patients/participants provided their written informed consent to participate in this study.
AM conceived the original idea, supervised the findings of this work, and supervised the project. GK and EP contributed to immunostaining and confocal imaging. VG and AK contributed to provision of clinical samples. ET carried out the experiment. AM wrote the manuscript in consultation with EL and VG. EL helped supervise the project. All authors contributed to the article and approved the submitted version.
This project has received funding from the Hellenic Foundation for Research and Innovation (HFRI) and the General Secretariat for Research and Technology (GSRT), under Grant Agreement No. 1964.
The authors declare that the research was conducted in the absence of any commercial or financial relationships that could be construed as a potential conflict of interest.
The Supplementary Material for this article can be found online at: https://www.frontiersin.org/articles/10.3389/fcell.2021.641978/full#supplementary-material
Supplementary Figure 1 | Positive and negative controls of CK/A45/VIM staining. (A) Cytospin with H1299 cells stained with CK/A45 (green) anti-mouse, Alexa 488 anti-mouse, VIM (red) anti-rabbit and Alexa 555 anti-rabbit (positive control). (B) Cytospin with H1299 cells stained with Alexa 488 anti-mouse, VIM (red) anti-rabbit and Alexa 555 anti-rabbit (negative control for CK). (C) Cytospin with H1299 cells stained with CK/A45 (green) anti-mouse, Alexa 488 anti-mouse and Alexa 555 anti-rabbit (negative control for VIM).
Supplementary Figure 2 | Phenotypic characterization of CKlow/CK– CTCs. Patients’ samples were stained with DAPI (blue; first column), CK/A45 (green; second column) and VIM (red; third column). The fourth column shows overlays. Representative images from confocal laser scanning microscopy of (A) CKlow/CK–/VIM– and (B) CKlow/CK–/VIM+ CTCs. Scale bars = 10 μm.
Supplementary Table 1 | Patients clinicopathological characteristics.
Supplementary Table 2 | MCT4 overexpression in respect to patients clinicopathological characteristics.
Supplementary Table 3 | Primers and probe sequence for the RT-qPCR of MCT4.
Allard, W. J., Matera, J., Miller, M. C., Repollet, M., Connelly, M. C., Rao, C., et al. (2004). Tumor cells circulate in the peripheral blood of all major carcinomas but not in healthy subjects or patients with nonmalignant diseases. Clin. Cancer Res. 10, 6897–6904. doi: 10.1158/1078-0432.CCR-04-0378
Baek, G., Tse, Y. F., Hu, Z., Cox, D., Buboltz, N., McCue, P., et al. (2014). MCT4 defines a glycolytic subtype of pancreatic cancer with poor prognosis and unique metabolic dependencies. Cell Rep. 9, 2233–2249. doi: 10.1016/j.celrep.2014.11.025
Baltazar, F., Pinheiro, C., Morais-Santos, F., Azevedo-Silva, J., Queirós, O., Preto, A., et al. (2014). Monocarboxylate transporters as targets and mediators in cancer therapy response. Histol. Histopathol. 29, 1511–1524. doi: 10.14670/HH-29.1511
Choi, J. W., Kim, Y., Lee, J. H., and Kim, Y. S. (2014). Prognostic significance of lactate/proton symporters MCT1, MCT4, and their chaperone CD147 expressions in urothelial carcinoma of the bladder. Urology 84, 245.e9–245.e15. doi: 10.1016/j.urology.2014.03.031
Chudziak, J., Burt, D. J., Mohan, S., Rothwell, D. G., Mesquita, B., Antonello, J., et al. (2016). Clinical evaluation of a novel microfluidic device for epitope-independent enrichment ofcirculatingtumour cells in patients with small cell lung cancer. Analyst 141, 669–678. doi: 10.1039/c5an02156a
Curry, J. M., Tuluc, M., Whitaker-Menezes, D., Ames, J. A., Anantharaman, A., Butera, A., et al. (2013). Cancer metabolism, stemness and tumor recurrence: MCT1 and MCT4 are functional biomarkers of metabolic symbiosis in head and neck cancer. Cell Cycle 12, 1371–1384. doi: 10.4161/cc.24092
DeBerardinis, R. J., and Chandel, N. S. (2020). We need to talk about the Warburg effect. Nat. Metab. 2, 127–129. doi: 10.1038/s42255-020-0172-2
Doyen, J., Trastour, C., Ettore, F., Peyrottes, I., Toussant, N., Gal, J., et al. (2014). Expression of the hypoxia-inducible monocarboxylate transporter MCT4 is increased in triple negative breast cancer and correlates independently with clinical outcome. Biochem. Biophys. Res. Commun. 451, 54–61. doi: 10.1016/j.bbrc.2014.07.050
Frick, M. A., Feigenberg, S. J., Jean-Baptiste, S. R., Aguarin, L. A., Mendes, A., Chinniah, C., et al. (2020). Circulating tumor cells are associated with recurrent disease in patients with early-stage non-small cell lung cancer treated with stereotactic body radiotherapy. Clin. Cancer Res. 26:2380.
Gerlinger, M., Santos, C. R., Spencer-Dene, B., Martinez, P., Endesfelder, D., Burrell, R. A., et al. (2012). Genome-wide RNA interference analysis of renal carcinoma survival regulators identifies MCT4 as a Warburg effect metabolic target. J. Pathol. 227, 146–156. doi: 10.1002/path.4006
Halestrap, A. P. (2013). The SLC16 gene family - structure, role and regulation in health and disease. Mol. Aspects Med. 34, 337–349. doi: 10.1016/j.mam.2012.05.003
Halestrap, A. P., and Price, N. T. (1999). The proton-linked monocarboxylate transporter (MCT) family: structure, function and regulation. Biochem. J. 343(Pt 2), 281–299. doi: 10.1042/0264-6021:3430281
Hanahan, D., and Weinberg, R. A. (2011). Hallmarks of cancer: the next generation. Cell 144, 646–674. doi: 10.1016/j.cell.2011.02.013
Heidrich, I., Ačkar, L., MossahebiMohammadi, P., and Pantel, K. (2020). Liquid biopsies: potential and challenges. Int. J. Cancer 148, 528–545. doi: 10.1002/ijc.33217
Hvichia, G. E., Parveen, Z., Wagner, C., Janning, M., Quidde, J., Stein, A., et al. (2016). A novel microfluidic platform for size and deformability based separation and the subsequent molecular characterization of viable circulating tumor cells. Int. J. Cancer 138, 2894–2904. doi: 10.1002/ijc.30007
Kallergi, G., Politaki, E., Alkahtani, S., Stournaras, C., and Georgoulias, V. (2016). Evaluation of isolation methods for circulating tumor cells (CTCs). Cell Physiol. Biochem. 40, 411–419. doi: 10.1159/000452556
Kershaw, S., Cummings, J., Morris, K., Tugwood, J., and Dive, C. (2015). Optimisation of immunofluorescence methods to determine MCT1 and MCT4 expression in circulating tumour cells. BMC Cancer 15:387. doi: 10.1186/s12885-015-1382-y
Kim, H. K., Lee, I., Bang, H., Kim, H. C., Lee, W. Y., Yun, S. H., et al. (2018). MCT4 expression is a potential therapeutic target in colorectal cancer with peritoneal carcinomatosis. Mol. Cancer Ther. 17, 838–848. doi: 10.1158/1535-7163.MCT-17-0535
Kim, Y., Choi, J. W., Lee, J. H., and Kim, Y. S. (2015). Expression of lactate/H? symporters MCT1 and MCT4 and their chaperone CD147 predicts tumor progression in clear cell renal cell carcinoma: immunohistochemical and The Cancer Genome Atlas data analyses. Hum. Pathol. 46, 104–112. doi: 10.1016/j.humpath.2014.09.013
Krebs, M. G., Hou, J. M., Sloane, R., Lancashire, L., Priest, L., Nonaka, D., et al. (2012). Analysis of circulating tumor cells in patients with non-small cell lung cancer using epithelial marker-dependent and -independent approaches. J. Thorac. Oncol. 7, 306–315. doi: 10.1097/jto.0b013e31823c5c16
Kuo, T. C., Huang, K. Y., Yang, S. C., Wu, S., Chung, W. C., Chang, Y. L., et al. (2020). Monocarboxylate transporter 4 is a therapeutic target in non-small cell lung cancer with aerobic glycolysis preference. Mol. Ther. Oncolytics 18, 189–201. doi: 10.1016/j.omto.2020.06.012
Lecharpentier, A., Vielh, P., Perez-Moreno, P., Planchard, D., Soria, J. C., and Farace, F. (2011). Detection of circulating tumour cells with a hybrid (epithelial/mesenchymal) phenotype in patients with metastatic non-small cell lung cancer. Br. J. Cancer 105, 1338–1341. doi: 10.1038/bjc.2011.405
Lianidou, E. S., Markou, A., and Strati, A. (2015). The role of CTCs as tumor biomarkers. Adv. Exp. Med. Biol. 867, 341–367. doi: 10.1007/978-94-017-7215-0_21
Livak, K. J., and Schmittgen, T. D. (2001). Analysis of relative gene expression data using real-time quantitative PCR and the 2(-Delta DeltaC(T)) method. Methods 25, 402–408. doi: 10.1006/meth.2001.1262
Luo, W., Rao, M., Qu, J., and Luo, D. (2018). Applications of liquid biopsy in lung cancer-diagnosis, prognosis prediction, and disease monitoring. Am. J. Transl. Res. 10, 3911–3923.
Markou, A., Lazaridou, M., Paraskevopoulos, P., Chen, S., Swierczewska, M., Budna, J., et al. (2018). Multiplex gene expression profiling of in vivo isolated circulating tumor cells in high-risk prostate cancer patients. Clin. Chem. 64, 297–306. doi: 10.1373/clinchem.2017.275503
Meng, S., Tripathy, D., Frenkel, E. P., Shete, S., Naftalis, E. Z., Huth, J. F., et al. (2004). Circulating tumor cells in patients with breast cancer dormancy. Clin. Cancer Res. 10, 8152–8162.
Meredith, D., and Christian, H. C. (2008). The SLC16 monocaboxylate transporter family. Xenobiotica 38, 1072–1106. doi: 10.1080/00498250802010868
Molina, J. R., Yang, P., Cassivi, S. D., Schild, S. E., and Adjei, A. A. (2008). Non-small cell lung cancer: epidemiology, risk factors, treatment, and survivorship. Mayo Clin. Proc. 83, 584–594. doi: 10.4065/83.5.584
Nakayama, Y., Torigoe, T., Inoue, Y., Minagawa, N., Izumi, H., Kohno, K., et al. (2012). Prognostic significance of monocarboxylate transporter 4 expression in patients with colorectal cancer. Exp. Ther. Med. 3, 25–30. doi: 10.3892/etm.2011.361
Pawlikowska, P., Faugeroux, V., Oulhen, M., Aberlenc, A., Tayoun, T., Pailler, E., et al. (2019). Circulating tumor cells (CTCs) for the noninvasive monitoring and personalization of non-small cell lung cancer (NSCLC) therapies. J. Thorac. Dis. 11, S45–S56.
Poole, R. C., and Halestrap, A. P. (1993). Transport of lactate and other monocarboxylates across mammalian plasma membranes. Am. J. Physiol. 264, C761–C782. doi: 10.1152/ajpcell.1993.264.4.C761
Porras, T. B., Kaur, P., Ring, A., Schechter, N., and Lang, J. E. (2018). Challenges in using liquid biopsies for gene expression profiling. Oncotarget 9, 7036–7053. doi: 10.18632/oncotarget.24140
Puri, S., and Juvale, K. (2020). Monocarboxylate transporter 1 and 4 inhibitors as potential therapeutics for treating solid tumours: a review with structure-activity relationship insights. Eur. J. Med. Chem. 199:112393. doi: 10.1016/j.ejmech.2020.112393
Ruan, Y., Zeng, F., Cheng, Z., Zhao, X., Fu, P., and Chen, H. (2017). High expression of monocarboxylate transporter 4 predicts poor prognosis in patients with lung adenocarcinoma. Oncol. Lett. 14, 5727–5734. doi: 10.3892/ol.2017.6964
Shu, Q. H., Ge, Y. S., Ma, H. X., Gao, X. Q., Pan, J., Liu, D., et al. (2016). Prognostic value of polarized macrophages in patients with hepatocellular carcinoma after curative resection. J. Mol. Med. 94, 155–171.
Siegel, R. L., Miller, K. D., and Jemal, A. (2015). Cancer statistics, 2015. CA Cancer J. Clin. 65, 5–29. doi: 10.3322/caac.21254
Strati, A., Koutsodontis, G., Papaxoinis, G., Angelidis, I., Zavridou, M., Economopoulou, P., et al. (2017). Prognostic significance of PD-L1 expression on circulating tumor cells in patients with head and neck squamous cell carcinoma. Ann. Oncol. 28, 1923–1933. doi: 10.1093/annonc/mdx206
Strati, A., Nikolaou, M., Georgoulias, V., and Lianidou, E. S. (2019). Prognostic significance of TWIST1, CD24, CD44, and ALDH1 transcript quantification in EpCAM-positive circulating tumor cells from early stage breast cancer patients. Cells 8:652. doi: 10.3390/cells8070652
Tamminga, M., de Wit, S., Schuuring, E., Timens, W., Terstappen, L. W. M. M., Hiltermann, T. J. N., et al. (2019). Circulating tumor cells in lung cancer are prognostic and predictive for worse tumor response in both targeted- and chemotherapy. Transl. Lung Cancer Res. 8, 854–861. doi: 10.21037/tlcr.2019.11.06
Wang, R., Lu, Y. Y., and Fan, D. M. (2015). Reasons for cancer metastasis: a holistic perspective. Mol. Clin. Oncol. 3, 1199–1202. doi: 10.3892/mco.2015.623
Wang, W., Song, Z., and Zhang, Y. A. (2017). Comparison of ddPCR and ARMS for detecting EGFR T790M status in ctDNA from advanced NSCLC patients with acquired EGFR-TKI resistance. Cancer Med. 6, 154–162. doi: 10.1002/cam4.978
Warburg, O. (1956). On the origin of cancer cells. Science 123, 309–314. doi: 10.1126/science.123.3191.309
Warburg, O., Wind, F., and Negelstein, E. (1927). The metabolism of tumors in the body. J. Gen. Physiol. 8, 519–530. doi: 10.1085/jgp.8.6.519
Wu, Y. L., Tsuboi, M., He, J., John, T., Grohe, C., Majem, M., et al. (2020). Osimertinib in resected EGFR-mutated non-small-cell lung cancer. N. Engl. J. Med. 383, 1711–1723. doi: 10.1056/NEJMoa2027071
Zavridou, M., Mastoraki, S., Strati, A., Tzanikou, E., Chimonidou, M., and Lianidou, E. (2018). Evaluation of preanalytical conditions and implementation of quality control steps for reliable gene expression and DNA methylation analyses in liquid biopsies. Clin. Chem. 64, 1522–1533. doi: 10.1373/clinchem.2018.292318
Zhang, X., Chang, N., Yang, G., Zhang, Y., Ye, M., Cao, J., et al. (2017). A comparison of ARMS-Plus and droplet digital PCR for detecting EGFR activating mutations in plasma. Oncotarget 8, 112014–112023. doi: 10.18632/oncotarget.22997
Zhang, X., Wei, L., Li, J., Zheng, J., Zhang, S., and Zhou, J. (2019). Epithelial-mesenchymal transition phenotype of circulating tumor cells is associated with distant metastasis in patients with NSCLC. Mol. Med. Rep. 19, 601–608.
Keywords: liquid biopsy, CTCs, NSCLC, MCT4, RT-qPCR, EMT 3
Citation: Markou A, Tzanikou E, Kallergi G, Pantazaka E, Georgoulias V, Kotsakis A and Lianidou E (2021) Evaluation of Monocarboxylate Transporter 4 (MCT4) Expression and Its Prognostic Significance in Circulating Tumor Cells From Patients With Early Stage Non-Small-Cell Lung Cancer. Front. Cell Dev. Biol. 9:641978. doi: 10.3389/fcell.2021.641978
Received: 15 December 2020; Accepted: 23 March 2021;
Published: 22 April 2021.
Edited by:
Catherine Alix-Panabieres, Centre Hospitalier Universitaire de Montpellier, FranceReviewed by:
Holger Kalthoff, University of Kiel, GermanyCopyright © 2021 Markou, Tzanikou, Kallergi, Pantazaka, Georgoulias, Kotsakis and Lianidou. This is an open-access article distributed under the terms of the Creative Commons Attribution License (CC BY). The use, distribution or reproduction in other forums is permitted, provided the original author(s) and the copyright owner(s) are credited and that the original publication in this journal is cited, in accordance with accepted academic practice. No use, distribution or reproduction is permitted which does not comply with these terms.
*Correspondence: Athina Markou, YXRtYXJrb3VAY2hlbS51b2EuZ3I=
Disclaimer: All claims expressed in this article are solely those of the authors and do not necessarily represent those of their affiliated organizations, or those of the publisher, the editors and the reviewers. Any product that may be evaluated in this article or claim that may be made by its manufacturer is not guaranteed or endorsed by the publisher.
Research integrity at Frontiers
Learn more about the work of our research integrity team to safeguard the quality of each article we publish.