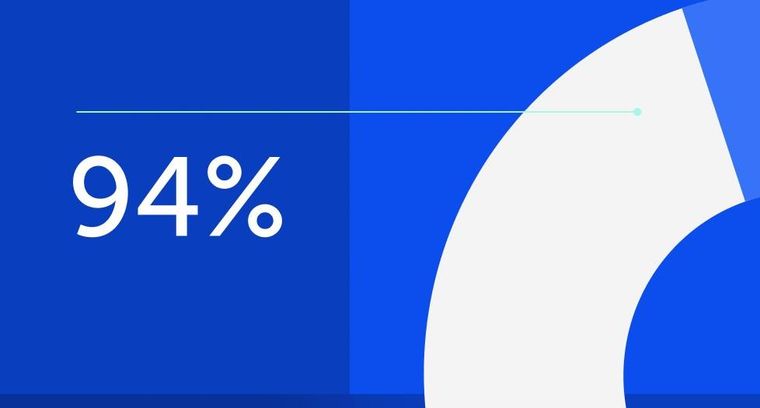
94% of researchers rate our articles as excellent or good
Learn more about the work of our research integrity team to safeguard the quality of each article we publish.
Find out more
MINI REVIEW article
Front. Cell Dev. Biol., 28 January 2021
Sec. Epigenomics and Epigenetics
Volume 9 - 2021 | https://doi.org/10.3389/fcell.2021.638542
This article is part of the Research TopicRole of Genetics and Epigenetics in Major Structural MalformationsView all 28 articles
Tbx20 is a member of the Tbx1 subfamily of T-box-containing genes and is known to play a variety of fundamental roles in cardiovascular development and homeostasis as well as cardiac remodeling in response to pathophysiological stresses. Mutations in TBX20 are widely associated with the complex spectrum of congenital heart defects (CHDs) in humans, which includes defects in chamber septation, chamber growth, and valvulogenesis. In addition, genetic variants of TBX20 have been found to be associated with dilated cardiomyopathy and heart arrhythmia. This broad spectrum of cardiac morphogenetic and functional defects is likely due to its broad expression pattern in multiple cardiogenic cell lineages and its critical regulation of transcriptional networks during cardiac development. In this review, we summarize recent findings in our general understanding of the role of Tbx20 in regulating several important aspects of cardiac development and homeostasis and heart function.
T-box (Tbx) family genes encode various transcription factors that are essential for embryonic development and organogenesis in the evolution of all metazoan, ranging from hydra to humans (Naiche et al., 2005). Cardiogenic lineage cells arise originally from the mesoderm, which is established during gastrulation (Moorman and Christoffels, 2003). Brachyury (also known as T) is considered the most ancient gene of the family and is critical to the formation and development of the posterior mesoderm (Herrmann et al., 1990); the loss of Brachyury impacts mesodermal specification and differentiation, resulting in a truncated tail in embryonic development (Papaioannou, 2001). The cardiogenic mesoderm between endodermal and mesodermal cells lumenizes and differentiates into endocardial cells (De Jong et al., 1990). Subsequently, the bilateral cardiogenic fields fuse in the midline to form the linear cardiac tube, which is followed by looping, septation, valvulogenesis, and chamber formation and maturation to become a functional heart (van den Berg and Moorman, 2009). The embryonic mesoderm of the mammalian embryo is built by a genetic network that involves master transcription factors and intracellular and intercellular signaling pathways. Previous studies have suggested that T is a direct downstream target of the Wnt3a signaling pathway, which provides a key balance between mesodermal and neuronal cell fates (Yamaguchi et al., 1999). In addition, another T-box-containing transcription factor, Eomesodermin (Eomes), plays an important role in early embryonic development, including mesodermal differentiation and migration as well as endoderm specification during gastrulation (Arnold et al., 2008). Similar to Eomes, T is also required for the formation, migration, and specification of nascent mesoderm cells (Morley et al., 2009). Along with the T-box family expanding throughout metazoan evolution (Papaioannou, 2014), family members became one of the most important master regulators in cardiovascular development, homeostasis, and function (Figure 1A). The importance of T-box family genes is further recognized by their profound contribution to inherited human disorders, including syndromic birth defects, congenital heart defects, dilated cardiomyopathy, and cardiac arrhythmogenesis (Packham and Brook, 2003; Papaioannou, 2014). The members of the TBX1 subfamily (e.g., TBX1, TBX18, and TBX20) and TBX2 subfamily (e.g., TBX2, TBX3, and TBX5) exhibit a comprehensive spatiotemporal expression pattern involved in all cardiogenic lineage cells, which are critical to cardiac development (Plageman and Yutzey, 2005; Stennard and Harvey, 2005; Just et al., 2016).
Figure 1. (A) Schematic diagram of the T-box gene family, which is important to cardiovascular development and function. (B) Schematic diagram of genomic structures of Tbx20 isoforms resulting from alternative splicing. Note, in the graph, the scales of introns and exons are not proportional to the exact sizes of each intron and exon.
Tbx1 is expressed in the pharyngeal mesoderm and endoderm, outflow tract (OFT), and second heart field (SHF) (Xu et al., 2004). A set of common CHDs, including DiGeorge syndrome (also known as 22q11. 2 deletion syndrome), tetralogy of Fallot, double outlet right ventricle, and transposition of the great arteries, are attributed to TBX1 mutations (Xu et al., 2004). Tbx18 is expressed mainly in epicardial cells and myocardial sinus horns that descend from the embryonic venous pole. The expression pattern likely underlies the major developmental program, such as myocardialization of the caval veins and differentiation of the sinus node myocardium (Christoffels et al., 2006; Wiese et al., 2009; Greulich et al., 2016). The optimal cardiac vascular network is essential for efficient perfusion in the heart. Tbx18-deficient mice develop defective coronary conduit vessels largely due to altered proepicardial cell signaling and differentiation (Cai et al., 2008; Wu et al., 2013). In this review, we will focus on the role of Tbx20 in cardiovascular development and function.
Tbx20 is a member of the Tbx1 subfamily (Figure 1A). Originally identified in the E10.5 mouse heart cDNA library and named Tbx12, it comprises a 3.9 kb nucleotide sequence coding for a protein containing 446 amino acids (Carson et al., 2000; Kraus et al., 2001). There are at least four Tbx20 isoforms derived from alternative splicing, designated Tbx20a-d (Figure 1B) (Stennard et al., 2003). Tbx20a encodes a full-length protein containing a conserved T-box DNA-binding domain encoded by exons 2–5 and exon 7 flanked by N- and C-terminal domains, whereas 158 amino acids in C-terminal regions are equipped with strong transactivation and transrepression domains (Stennard et al., 2003; Hammer et al., 2008). Tbx20b and Tbx20c are alternatively spliced isoforms with alternative exons 9 and 8 at the 3′end, respectively. Both Tbx20b and Tbx20c contain T-box-binding domains but lack exon 10 (Stennard et al., 2003; DeBenedittis and Jiao, 2011). Tbx20d is the shortest isoform with a truncated T-box domain (Muller and Herrmann, 1997; Stennard et al., 2003). Unlike Tbx20d, all three other Tbx20 isoforms can bind to DNA, interact with Nkx2-5 and GATA4, and function in the cooperative or synergistic regulation of transcription (Stennard et al., 2003; DeBenedittis and Jiao, 2011). Tbx20a is only expressed in the heart, while Tbx20b is expressed more broadly (Takeuchi et al., 2005; DeBenedittis and Jiao, 2011). The overexpression of Tbx20a, but not Tbx20b, induces the mesodermal marker Xbra, endodermal marker edd, and cardiogenic marker Nkx2-5, suggesting that the Tbx20 isoforms provide important dynamic regulation of cardiovascular development (Stennard et al., 2003; DeBenedittis and Jiao, 2011).
Tbx20 expression is found in almost all cardiogenic cell lineages throughout evolution from arthropod to vertebrate embryos (Ahn et al., 2000; Pocock et al., 2008). The Drosophila Tbx20 gene pair, neuromancer1 (nmr1, FlyBase:H15) and neuromancer2 (nmr2, FlyBase:mid), is expressed in early cardioblasts of the dorsal vessel (Griffin et al., 2000; Qian et al., 2005; Svendsen et al., 2009), a primitive heart-like organ in the fly. H15/midline is essential to the generation and maintenance of myofibrillar architecture and rhythmic contractile physiology (Qian et al., 2008). A series of studies demonstrated that Tbx20 in Xenopus, zebrafish, chicks, and mice functions in a very conserved fashion during heart development (Griffin et al., 2000; Iio et al., 2001). Tbx20 is one of the earliest markers in the Xenopus cardiogenic lineage. Tbx20 morpholino injection results in pericardial edema and reduced cardiac mass (Brown et al., 2003, 2005). Similarly, Tbx20 is important for driving cardioprogenitor formation and cardiomyocyte proliferation in zebrafish (Lu et al., 2017). The knockdown of Tbx20-homolog hrT in zebrafish leads to several cardiac developmental defects, including compromised cardiac looping and chamber formation (Szeto et al., 2002). Using explants from chick embryos, Tbx20 was shown to be involved in Bmp2 signaling in heart development (Plageman and Yutzey, 2004). These original findings indicate that Tbx20 has an indispensable role in cardiogenesis throughout evolution.
Tbx20 expression is found throughout mouse heart development in both cardiogenic heart fields (Kraus et al., 2001). Tbx20 is expressed in the early cardiac progenitor region, endocardium and myocardium, endothelial cells of outflow track endocardial cushion and atrial ventricular cushion, the precursor structure of cardiac valves, and atrioventricular septum (Stennard et al., 2003). Temporally, Tbx20 is found within the cardiogenic mesoderm as early as E7.5. As shown in Tbx20 knockouts, Tbx20 is required for heart looping and is essential for adequate proliferative activity in developing cardiomyocytes (Kraus et al., 2001; Cai et al., 2005; Stennard et al., 2005).
Tbx20 knockouts die at ~E10.5 with severe hypoplastic ventricular walls, in conjunction with a defect in cardiac looping (Cai et al., 2005; Singh et al., 2005). Interestingly, Tbx20 knockdown mice demonstrate a dose-dependent regulation of heart development; the knockdown mice exhibit OFT defects with a fused aorta and pulmonary arteries and hypoplastic right ventricles (Takeuchi et al., 2005). Consistent findings have been obtained in studies where gain-of-function Tbx20 mutations lead to a diverse array of cardiac defects, including abnormal ventricular walls, double outlet right ventricle (DORV), congenital atrial septal defects, patent foramen ovale (PFO), bicuspid aortic valve (BAV), and typical symptoms presented in familial tetralogy of Fallot (Posch et al., 2010; Zhang et al., 2011; Pan et al., 2015; Huang et al., 2017; Luyckx et al., 2019). In the adult heart, Tbx20 haploinsufficiency gives rise to left ventricular dilation, and systolic and diastolic dysfunction, which resembles dilated cardiomyopathy (DCM) in humans (Packham and Brook, 2003). Thus, Tbx20 is not only important to heart development but also contributes to adult heart function, homeostasis, and physiological and pathophysiological adaptation (Stennard et al., 2005), which is further confirmed by severe dilated cardiomyopathy, arrhythmias, and heart failure in Tbx20 conditional knockout adult cardiomyocytes (Shen et al., 2011). Collectively, these studies demonstrate the critical function of Tbx20 in cardiac development and function.
The broad expression pattern of Tbx20 in the developing heart suggests that Tbx20 is involved in multiple cardiogenic processes by interacting with broad transcriptional networks in different regions of developing hearts (Figure 2). It has been shown that Tbx20 directly interacts with Nkx2-5, GATA4, and GATA5 to synergistically regulate cardiac gene expression in various cell types and coordinate cellular proliferation, differentiation, and chamber formation (Stennard et al., 2003; Brown et al., 2005). Tbx20 knockout mouse embryos die at ~E10.5 with hypoplastic hearts, further confirming the importance of Tbx20 in cardiac development (Cai et al., 2005; Singh et al., 2005; Stennard et al., 2005). Its interaction with Nkx2.5 likely promotes cardiogenic progenitor cell proliferation and differentiation (Prall et al., 2007). Plageman and Yutzey showed that Tbx5 promotes natriuretic factor (ANF) expression, which is negatively regulated by Tbx20 (Plageman and Yutzey, 2004). Tbx20 has also been shown to function synergistically with Isl1 and Gata4 to activate Mef2c and Nkx2-5, which are required for normal formation of the right ventricle and outflow tract, providing a potentially unifying molecular mechanism for Tbx20 as a transcriptional modulator in heart development (Takeuchi et al., 2005). Interestingly, the upregulation of Isl1 is found in Tbx20 mutant hearts (Cai et al., 2005). Isl1 is a major determinant of cardiogenic progenitor cells. Chromatin immunoprecipitation (ChIP) analyses demonstrate that Tbx20 directly binds the conserved T-half sites within the Isl1 promoter sequence, suggesting that Tbx20 is a negative regulator of Isl1 expression (Cai et al., 2005).
Figure 2. Schematic diagram of the Tbx20-mediated transcriptional network in cardiovascular development and function. Tbx20 has a broad temporal and spatial expression profile and participates in a series of cardiogenic processes via its interaction with various transcriptional networks in developing and adult hearts. OFT, outflow track; AVC, atrioventricular cushion; AVS, atrioventricular septum; IVS, interventricular septum.
In addition to negatively modulating Tbx5-mediated transcriptional function, Tbx20 also inhibits Tbx2-mediated transcriptional function and directly or indirectly represses Tbx2 expression in the myocardium, providing a great stimulus to define the specification of the chamber and non-chamber myocardium, a lineage digression in the early stage of the heart underlying all subsequent morphogenesis (Stennard et al., 2005). In the developing outflow tract and atrioventricular canal, Tbx2 directly represses Nmyc1, a member of the Myc family of nuclear proto-oncogenes, resulting in relatively lower proliferative activity (Cai et al., 2005). Tbx20 can enhance proliferation efficiency by promoting Nmyc1 expression (Cai et al., 2005). Additional analysis further demonstrated that Tbx20 can directly attenuate BMP/Smad signaling to suppress Tbx2 expression in the chambers. Subsequently, Tbx2 expression is confined to the developing atrioventricular canal region (Singh et al., 2009). It is important to highlight that Tbx20 acts as an indirect repressor to restrict precocious Tbx2 transcription by sequestering BMP-activated Smad1/5/8. The mutant isoforms of Tbx20 lacking DNA binding can bind to phosphorylated Smad1/5/8 and prevent the interaction between Smad1/5/8 andco-Smad4 (Stennard et al., 2003; Singh et al., 2009; Singh and Kispert, 2010). In addition, Mandel et al. also demonstrated that high-affinity Smad-binding sites are located in Tbx20 cardiac regulatory elements. Blocking Smad-mediated signaling can specifically lead to the loss of Tbx20-mediated function (Mandel et al., 2010), further supporting the reciprocal regulation between the BMP/Smad pathway and Tbx20. Furthermore, Tbx20 is a direct regulator of COUP-TFII, which is necessary for the establishment of atrial identity; Tbx20 conditional knockouts reduce the expression of COUP-TFII (Cai et al., 2003; Watanabe et al., 2012; Boogerd et al., 2018). Additionally, Tbx20 can directly regulate Hey2, Irx4, and genes that are essential to cardiomyocyte proliferation, such as Mycn, Erbb2, and Cdc6 (Bersell et al., 2009; Boogerd et al., 2018; Ihara et al., 2020). In addition, Tbx20 can activate PI3K/AKT/GSK3β/β-catenin-dependent pathways and promote adult cardiomyocyte proliferation (Chakraborty et al., 2013). Another interesting finding is that Tbx20 overexpression in adult mouse hearts activates cardiomyocyte proliferation via Akt-, YAP-, and BMP-mediated signaling and represses inhibitory pathways via p21, Meis1, and Btg2 (Xiang et al., 2016). Apparently, Tbx20 overexpression in cardiomyocytes promotes myocardial repair or regeneration in adult hearts in response to myocardial injury, presenting a potential therapeutic strategy (Xiang et al., 2016; Fang et al., 2020). Furthermore, a study identified that myocardial Tbx20 induction enables the activation of the endocardium by promoting endocardial cell extension and proliferation at the injury site in adult zebrafish hearts, which likely occurs via the activation of endocardial bone morphogenetic protein 6 (Bmp6) signaling (Fang et al., 2020).
Another set of experiments aiming to determine the upstream events of Tbx20 also revealed the key function of Tbx20 in ventricular wall development. Neuregulin 1 (Nrg1), a member of the epidermal growth factor family, is known for its critical role in ventricular wall trabeculation (Lai et al., 2010; Del Monte-Nieto et al., 2018). Nrg1 is expressed in the endocardium but binds to its receptors ErbB2 and ErbB4 in the myocardium to initiate trabeculation (Gassmann et al., 1995; Chang et al., 1997; Hertig et al., 1999; Bersell et al., 2009). Nrg1 is found to suppress Tbx20 expression in a dose-dependent manner, which highlights the potential mechanisms by which Nrg1 can actively downregulate Tbx20 during ventricular chamber maturation (Stennard et al., 2005). Similarly, Transcription factor AP-2 gamma (Tfap2c) was also found to be a transcriptional repressor of Tbx20 expression. Decreased expression levels of Tfap2c can upregulate Tbx20 expression (Hammer et al., 2008). More interestingly, several studies suggest that BMP-mediated signaling positively regulates Tbx20 expression (Mandel et al., 2010; Zhang et al., 2011), suggesting that important positive and negative regulatory cascades regulate Tbx20 expression during heart development.
Tbx20 is known to be expressed in the endocardium. The endocardium is not only critical to ventricular wall development and maturation but also critical to valvulogenesis. The valves are derived from precursor structures known as endocardial cushions. A series of analyses revealed that Tbx20 is required for early atrial ventricular cushion formation and endocardial endothelial-mesenchymal transformation (EMT). Tbx20 is an important player in the modulation of extracellular matrix expression along with the promotion of cell proliferation in mesenchymal valve precursor structures derived from endocardial cushions (Shelton and Yutzey, 2007). An interesting finding is that Tbx20 initiates EMT action via the Bmp2-mediated regulation of Sox9, Twist1, and Msx1 expression in the developing atrial ventricular cushion (Ma et al., 2005; Cai et al., 2011). More importantly, Tbx20 can regulate Lef1, a key transcriptional mediator of the Wnt/β-catenin pathways, in the promotion of endocardial cushion maturation and valve elongation (Cai et al., 2013). In addition, Boogerd et al. demonstrated a direct role for Tbx20 in Vcan expression in endocardial lineages during septation (Boogerd et al., 2016), further suggesting the broad impact of Tbx20 in endocardial-mediated cardiogenic events.
The propagation of electrical impulses that coordinate rhythmic and synchronized cardiac contractions to facilitate systemic circulation is regulated by the cardiac conduction system (CCS) in a spatiotemporally precise manner. The CCS is a complex set of specialized structures and cell types in the heart, which include the sinoatrial node (SAN), atrioventricular node (AVN), fast-conducting atrioventricular bundle (AVB), left and right bundle branches, and Purkinje fiber (PF) network. In addition, working cardiomyocytes also play a pivotal role in propagating electrical impulses throughout the myocardium. Congenital defects of the CCS and dysregulation of CCS homeostasis can lead to CCS dysfunction, causing life-threatening arrhythmias and increasing the risk of death in both children and adults (Wolf and Berul, 2006; Mangoni and Nargeot, 2008; Christoffels et al., 2010; Munshi, 2012; van Weerd and Christoffels, 2016). Genome-wide association studies (GWAS) in human patients with various arrhythmias have revealed a close association of abnormal electrocardiography (ECG) to many ion channels, gap junction proteins, muscle structural proteins and several critical transcription factors that function in the specification, differentiation and homeostatic maintenance of the CCS (van Weerd and Christoffels, 2016). These transcription factors include the T-box genes TBX3, TBX5, and TBX20. Tbx3 and Tbx5 are two known key transcriptional regulators of CCS development (van Weerd and Christoffels, 2016). Interestingly, although Tbx20 was not initially associated with roles in CCS development, GWAS analysis revealed that single nucleotide polymorphisms (SNPs) within TBX20 are linked to prolonged QRS duration with strong linkage disequilibrium (LD) (Sotoodehnia et al., 2010; Evans et al., 2016). These SNPs are intergenic, suggesting that these regions are involved in the transcriptional regulation of TBX20. These data suggest that Tbx20 participates in the regulation of either CCS development/maintenance or myocardial conduction. It is likely that Tbx20 coordinates and maintains the spatial and temporal control of the development and function of the cardiac conduction system via either parallel or single-gene regulatory pathways. Studies by Shen et al. demonstrated that mutant mice with conditional Tbx20 ablation in adult cardiomyocytes have dilated hearts with a rapid loss of systolic function and slower conduction and severe arrhythmia (Shen et al., 2011; Sakabe et al., 2012). ChIP and enhancer analyses revealed a broad range of direct target genes of Tbx20 that contribute to regulating cardiac rhymical function (Sakabe et al., 2012). These downstream targets are largely linked to human inherited channelopathies (Priori and Napolitano, 2006; Lehnart et al., 2007; Roberts and Gollob, 2010; Shen et al., 2011). A recent study further demonstrated that Tbx20 selectively regulates the expression of Kcnh2, which encodes the channel Kv11.1 (hERG), a critical channel responsible for ventricular repolarizing currents. The human TBX20 p.R311C mutation can lead to the loss of TBX20 transcriptional activity, which subsequently causes a lower expression level of hERG and inward rectifier current, leading to prolonged action potentials (Trudeau et al., 1995; Caballero et al., 2017). Moreover, Tbx20 is also found to regulate Kcnd2, Kcnd3, Cacna1a, Cacna1c, Atp2a2, Pln, Ryr2, and Camk2d (Shen et al., 2011), strongly supporting the critical role of Tbx20 in maintaining cardiac function.
In summary, this review summarized the recent findings related to our general understanding of the role of Tbx20 in regulating several important aspects of cardiac development, homeostasis, and heart function. These advances provide a basis for the early genetic diagnosis of associated CHDs, cardiomyopathies, and heart functional defects. However, the broad associations of Tbx20 with multiple biological events in multiple cardiogenic cell lineages during cardiac development make it difficult to map out all Tbx20-mediated upstream and downstream genetic networks intercellularly and/or intracellularly, especially the correlation of its genetic mutations found in complex CHD and cardiomyopathy patients with unique disease outcomes. Future analyses using hESC- and hiPSC-based analyses may help to confirm the findings from various animal models, thus addressing the key issues in disease progression in humans.
YC drafted the manuscript. DX and LZ helped with early revision. C-LC, B-YL, and YL finalized the manuscript. All authors contributed to the article and approved the submitted version.
This work was supported in part by the National Institute of Health P01HL134599 and R01HL145060 and Riley Children Foundation.
The authors declare that the research was conducted in the absence of any commercial or financial relationships that could be construed as a potential conflict of interest.
We thank members of the Cai Lab for critical discussion and proof reading.
Ahn, D. G., Ruvinsky, I., Oates, A. C., Silver, L. M., and Ho, R. K. (2000). tbx20, a new vertebrate T-box gene expressed in the cranial motor neurons and developing cardiovascular structures in zebrafish. Mech. Dev. 95, 253–258. doi: 10.1016/S0925-4773(00)00346-4
Arnold, S. J., Hofmann, U. K., Bikoff, E. K., and Robertson, E. J. (2008). Pivotal roles for eomesodermin during axis formation, epithelium-to-mesenchyme transition, and endoderm specification in the mouse. Development 135, 501–511. doi: 10.1242/dev.014357
Bersell, K., Arab, S., Haring, B., and Kuhn, B. (2009). Neuregulin1/ErbB4 signaling induces cardiomyocyte proliferation and repair of heart injury. Cell 138, 257–270. doi: 10.1016/j.cell.2009.04.060
Boogerd, C. J., Aneas, I., Sakabe, N., Dirschinger, R. J., Cheng, Q. J., Zhou, B., et al. (2016). Probing chromatin landscape reveals roles of endocardial TBX20 in septation. J. Clin. Invest. 126, 3023–3035. doi: 10.1172/JCI85350
Boogerd, C. J., Zhu, X., Aneas, I., Sakabe, N., Zhang, L., Sobreira, D. R., et al. (2018). Tbx20 is required in mid-gestation cardiomyocytes and plays a central role in atrial development. Circ. Res. 123, 428–442. doi: 10.1161/CIRCRESAHA.118.311339
Brown, D. D., Binder, O., Pagratis, M., Parr, B. A., and Conlon, F. L. (2003). Developmental expression of the Xenopus laevis Tbx20 orthologue. Dev. Genes Evol. 212, 604–607. doi: 10.1007/s00427-002-0276-6
Brown, D. D., Martz, S. N., Binder, O., Goetz, S. C., Price, B. M., Smith, J. C., et al. (2005). Tbx5 and Tbx20 act synergistically to control vertebrate heart morphogenesis. Development 132, 553–563. doi: 10.1242/dev.01596
Caballero, R., Utrilla, R. G., Amoros, I., Matamoros, M., Perez-Hernandez, M., Tinaquero, D., et al. (2017). Tbx20 controls the expression of the KCNH2 gene and of hERG channels. Proc. Natl. Acad. Sci. U.S.A. 114, E416–E425. doi: 10.1073/pnas.1612383114
Cai, C. L., Liang, X., Shi, Y., Chu, P. H., Pfaff, S. L., Chen, J., et al. (2003). Isl1 identifies a cardiac progenitor population that proliferates prior to differentiation and contributes a majority of cells to the heart. Dev. Cell 5, 877–889. doi: 10.1016/S1534-5807(03)00363-0
Cai, C. L., Martin, J. C., Sun, Y., Cui, L., Wang, L., Ouyang, K., et al. (2008). A myocardial lineage derives from Tbx18 epicardial cells. Nature 454, 104–108. doi: 10.1038/nature06969
Cai, C. L., Zhou, W., Yang, L., Bu, L., Qyang, Y., Zhang, X., et al. (2005). T-box genes coordinate regional rates of proliferation and regional specification during cardiogenesis. Development 132, 2475–2487. doi: 10.1242/dev.01832
Cai, X., Nomura-Kitabayashi, A., Cai, W., Yan, J., Christoffels, V. M., and Cai, C. L. (2011). Myocardial Tbx20 regulates early atrioventricular canal formation and endocardial epithelial-mesenchymal transition via Bmp2. Dev. Biol. 360, 381–390. doi: 10.1016/j.ydbio.2011.09.023
Cai, X., Zhang, W., Hu, J., Zhang, L., Sultana, N., Wu, B., et al. (2013). Tbx20 acts upstream of Wnt signaling to regulate endocardial cushion formation and valve remodeling during mouse cardiogenesis. Development 140, 3176–3187. doi: 10.1242/dev.092502
Carson, C. T., Kinzler, E. R., and Parr, B. A. (2000). Tbx12, a novel T-box gene, is expressed during early stages of heart and retinal development. Mech. Dev. 96, 137–140. doi: 10.1016/S0925-4773(00)00376-2
Chakraborty, S., Sengupta, A., and Yutzey, K. E. (2013). Tbx20 promotes cardiomyocyte proliferation and persistence of fetal characteristics in adult mouse hearts. J. Mol. Cell. Cardiol. 62, 203–213. doi: 10.1016/j.yjmcc.2013.05.018
Chang, H., Riese, D. J. II, Gilbert, W., Stern, D. F., and McMahan, U. J. (1997). Ligands for ErbB-family receptors encoded by a neuregulin-like gene. Nature 387, 509–512. doi: 10.1038/387509a0
Christoffels, V. M., Mommersteeg, M. T., Trowe, M. O., Prall, O. W., de Gier-de Vries, C., Soufan, A. T., et al. (2006). Formation of the venous pole of the heart from an Nkx2-5-negative precursor population requires Tbx18. Circ. Res. 98, 1555–1563. doi: 10.1161/01.RES.0000227571.84189.65
Christoffels, V. M., Smits, G. J., Kispert, A., and Moorman, A. F. (2010). Development of the pacemaker tissues of the heart. Circ. Res. 106, 240–254. doi: 10.1161/CIRCRESAHA.109.205419
De Jong, F., Geerts, W. J., Lamers, W. H., Los, J. A., and Moorman, A. F. (1990). Isomyosin expression pattern during formation of the tubular chicken heart: a three-dimensional immunohistochemical analysis. Anat. Rec. 226, 213–227. doi: 10.1002/ar.1092260211
DeBenedittis, P., and Jiao, K. (2011). Alternative splicing of T-box transcription factor genes. Biochem. Biophys. Res. Commun. 412, 513–517. doi: 10.1016/j.bbrc.2011.08.010
Del Monte-Nieto, G., Ramialison, M., Adam, A. A. S., Wu, B., Aharonov, A., D'Uva, G., et al. (2018). Control of cardiac jelly dynamics by NOTCH1 and NRG1 defines the building plan for trabeculation. Nature 557, 439–445. doi: 10.1038/s41586-018-0110-6
Evans, D. S., Avery, C. L., Nalls, M. A., Li, G., Barnard, J., Smith, E. N., et al. (2016). Fine-mapping, novel loci identification, and SNP association transferability in a genome-wide association study of QRS duration in African Americans. Hum. Mol. Genet. 25, 4350–4368. doi: 10.1093/hmg/ddw284
Fang, Y., Lai, K. S., She, P., Sun, J., Tao, W., and Zhong, T. P. (2020). Tbx20 induction promotes zebrafish heart regeneration by inducing cardiomyocyte dedifferentiation and endocardial expansion. Front. Cell Dev. Biol. 8:738. doi: 10.3389/fcell.2020.00738
Gassmann, M., Casagranda, F., Orioli, D., Simon, H., Lai, C., Klein, R., et al. (1995). Aberrant neural and cardiac development in mice lacking the ErbB4 neuregulin receptor. Nature 378, 390–394. doi: 10.1038/378390a0
Greulich, F., Rudat, C., Farin, H. F., Christoffels, V. M., and Kispert, A. (2016). Lack of genetic interaction between Tbx18 and Tbx2/Tbx20 in mouse epicardial development. PLoS ONE 11:e0156787. doi: 10.1371/journal.pone.0156787
Griffin, K. J., Stoller, J., Gibson, M., Chen, S., Yelon, D., Stainier, D. Y., et al. (2000). A conserved role for H15-related T-box transcription factors in zebrafish and Drosophila heart formation. Dev. Biol. 218, 235–247. doi: 10.1006/dbio.1999.9571
Hammer, S., Toenjes, M., Lange, M., Fischer, J. J., Dunkel, I., Mebus, S., et al. (2008). Characterization of TBX20 in human hearts and its regulation by TFAP2. J. Cell. Biochem. 104, 1022–1033. doi: 10.1002/jcb.21686
Herrmann, B. G., Labeit, S., Poustka, A., King, T. R., and Lehrach, H. (1990). Cloning of the T gene required in mesoderm formation in the mouse. Nature 343, 617–622. doi: 10.1038/343617a0
Hertig, C. M., Kubalak, S. W., Wang, Y., and Chien, K. R. (1999). Synergistic roles of neuregulin-1 and insulin-like growth factor-I in activation of the phosphatidylinositol 3-kinase pathway and cardiac chamber morphogenesis. J. Biol. Chem. 274, 37362–37369. doi: 10.1074/jbc.274.52.37362
Huang, R. T., Wang, J., Xue, S., Qiu, X. B., Shi, H. Y., Li, R. G., et al. (2017). TBX20 loss-of-function mutation responsible for familial tetralogy of Fallot or sporadic persistent truncus arteriosus. Int. J. Med. Sci. 14, 323–332. doi: 10.7150/ijms.17834
Ihara, D., Watanabe, Y., Seya, D., Arai, Y., Isomoto, Y., Nakano, A., et al. (2020). Expression of Hey2 transcription factor in the early embryonic ventricles is controlled through a distal enhancer by Tbx20 and Gata transcription factors. Dev. Biol. 461, 124–131. doi: 10.1016/j.ydbio.2020.02.001
Iio, A., Koide, M., Hidaka, K., and Morisaki, T. (2001). Expression pattern of novel chick T-box gene, Tbx20. Dev. Genes Evol. 211, 559–562. doi: 10.1007/s00427-001-0187-y
Just, S., Raphel, L., Berger, I. M., Buhler, A., Kessler, M., and Rottbauer, W. (2016). Tbx20 is an essential regulator of embryonic heart growth in zebrafish. PLoS ONE 11:e0167306. doi: 10.1371/journal.pone.0167306
Kraus, F., Haenig, B., and Kispert, A. (2001). Cloning and expression analysis of the mouse T-box gene tbx20. Mech. Dev. 100, 87–91. doi: 10.1016/S0925-4773(00)00499-8
Lai, D., Liu, X., Forrai, A., Wolstein, O., Michalicek, J., Ahmed, I., et al. (2010). Neuregulin 1 sustains the gene regulatory network in both trabecular and nontrabecular myocardium. Circ. Res. 107, 715–727. doi: 10.1161/CIRCRESAHA.110.218693
Lehnart, S. E., Ackerman, M. J., Benson, D. W. Jr., Brugada, R., Clancy, C. E., Donahue, J. K., et al. (2007). Inherited arrhythmias: a National Heart, Lung, and Blood Institute and Office of Rare Diseases workshop consensus report about the diagnosis, phenotyping, molecular mechanisms, and therapeutic approaches for primary cardiomyopathies of gene mutations affecting ion channel function. Circulation 116, 2325–2345. doi: 10.1161/CIRCULATIONAHA.107.711689
Lu, F., Langenbacher, A., and Chen, J. N. (2017). Tbx20 drives cardiac progenitor formation and cardiomyocyte proliferation in zebrafish. Dev. Biol. 421, 139–148. doi: 10.1016/j.ydbio.2016.12.009
Luyckx, I., Kumar, A. A., Reyniers, E., Dekeyser, E., Vanderstraeten, K., Vandeweyer, G., et al. (2019). Copy number variation analysis in bicuspid aortic valve-related aortopathy identifies TBX20 as a contributing gene. Eur. J. Hum. Genet. 27, 1033–1043. doi: 10.1038/s41431-019-0364-y
Ma, L., Lu, M. F., Schwartz, R. J., and Martin, J. F. (2005). Bmp2 is essential for cardiac cushion epithelial-mesenchymal transition and myocardial patterning. Development 132, 5601–5611. doi: 10.1242/dev.02156
Mandel, E. M., Kaltenbrun, E., Callis, T. E., Zeng, X. X., Marques, S. R., Yelon, D., et al. (2010). The BMP pathway acts to directly regulate Tbx20 in the developing heart. Development 137, 1919–1929. doi: 10.1242/dev.043588
Mangoni, M. E., and Nargeot, J. (2008). Genesis and regulation of the heart automaticity. Physiol. Rev. 88, 919–982. doi: 10.1152/physrev.00018.2007
Moorman, A. F., and Christoffels, V. M. (2003). Cardiac chamber formation: development, genes, and evolution. Physiol. Rev. 83, 1223–1267. doi: 10.1152/physrev.00006.2003
Morley, R. H., Lachani, K., Keefe, D., Gilchrist, M. J., Flicek, P., Smith, J. C., et al. (2009). A gene regulatory network directed by zebrafish No tail accounts for its roles in mesoderm formation. Proc. Natl. Acad. Sci. U.S.A. 106, 3829–3834. doi: 10.1073/pnas.0808382106
Muller, C. W., and Herrmann, B. G. (1997). Crystallographic structure of the T domain-DNA complex of the Brachyury transcription factor. Nature 389, 884–888. doi: 10.1038/39929
Munshi, N. V. (2012). Gene regulatory networks in cardiac conduction system development. Circ. Res. 110, 1525–1537. doi: 10.1161/CIRCRESAHA.111.260026
Naiche, L. A., Harrelson, Z., Kelly, R. G., and Papaioannou, V. E. (2005). T-box genes in vertebrate development. Annu. Rev. Genet. 39, 219–239. doi: 10.1146/annurev.genet.39.073003.105925
Packham, E. A., and Brook, J. D. (2003). T-box genes in human disorders. Hum. Mol. Genet. 12 Spec No 1, R37–R44. doi: 10.1093/hmg/ddg077
Pan, Y., Geng, R., Zhou, N., Zheng, G. F., Zhao, H., Wang, J., et al. (2015). TBX20 loss-of-function mutation contributes to double outlet right ventricle. Int. J. Mol. Med. 35, 1058–1066. doi: 10.3892/ijmm.2015.2077
Papaioannou, V. E. (2001). T-box genes in development: from hydra to humans. Int. Rev. Cytol. 207, 1–70. doi: 10.1016/S0074-7696(01)07002-4
Papaioannou, V. E. (2014). The T-box gene family: emerging roles in development, stem cells, and cancer. Development 141, 3819–3833. doi: 10.1242/dev.104471
Plageman, T. F. Jr., and Yutzey, K. E. (2004). Differential expression and function of Tbx5 and Tbx20 in cardiac development. J. Biol. Chem. 279, 19026–19034. doi: 10.1074/jbc.M314041200
Plageman, T. F. Jr., and Yutzey, K. E. (2005). T-box genes and heart development: putting the “T” in heart. Dev. Dyn. 232, 11–20. doi: 10.1002/dvdy.20201
Pocock, R., Mione, M., Hussain, S., Maxwell, S., Pontecorvi, M., Aslam, S., et al. (2008). Neuronal function of Tbx20 conserved from nematodes to vertebrates. Dev. Biol. 317, 671–685. doi: 10.1016/j.ydbio.2008.02.015
Posch, M. G., Gramlich, M., Sunde, M., Schmitt, K. R., Lee, S. H., Richter, S., et al. (2010). A gain-of-function TBX20 mutation causes congenital atrial septal defects, patent foramen ovale, and cardiac valve defects. J. Med. Genet. 47, 230–235. doi: 10.1136/jmg.2009.069997
Prall, O. W., Menon, M. K., Solloway, M. J., Watanabe, Y., Zaffran, S., Bajolle, F., et al. (2007). An Nkx2-5/Bmp2/Smad1 negative feedback loop controls heart progenitor specification and proliferation. Cell 128, 947–959. doi: 10.1016/j.cell.2007.01.042
Priori, S. G., and Napolitano, C. (2006). Role of genetic analyses in cardiology: part I: mendelian diseases: cardiac channelopathies. Circulation 113, 1130–1135. doi: 10.1161/CIRCULATIONAHA.105.563205
Qian, L., Liu, J., and Bodmer, R. (2005). Neuromancer Tbx20-related genes (H15/midline) promote cell fate specification and morphogenesis of the Drosophila heart. Dev. Biol. 279, 509–524. doi: 10.1016/j.ydbio.2005.01.013
Qian, L., Mohapatra, B., Akasaka, T., Liu, J., Ocorr, K., Towbin, J. A., et al. (2008). Transcription factor neuromancer/TBX20 is required for cardiac function in Drosophila with implications for human heart disease. Proc. Natl. Acad. Sci. U.S.A. 105, 19833–19838. doi: 10.1073/pnas.0808705105
Roberts, J. D., and Gollob, M. H. (2010). The genetic and clinical features of cardiac channelopathies. Future Cardiol. 6, 491–506. doi: 10.2217/fca.10.27
Sakabe, N. J., Aneas, I., Shen, T., Shokri, L., Park, S. Y., Bulyk, M. L., et al. (2012). Dual transcriptional activator and repressor roles of TBX20 regulate adult cardiac structure and function. Hum. Mol. Genet. 21, 2194–2204. doi: 10.1093/hmg/dds034
Shelton, E. L., and Yutzey, K. E. (2007). Tbx20 regulation of endocardial cushion cell proliferation and extracellular matrix gene expression. Dev. Biol. 302, 376–388. doi: 10.1016/j.ydbio.2006.09.047
Shen, T., Aneas, I., Sakabe, N., Dirschinger, R. J., Wang, G., Smemo, S., et al. (2011). Tbx20 regulates a genetic program essential to adult mouse cardiomyocyte function. J. Clin. Invest. 121, 4640–4654. doi: 10.1172/JCI59472
Singh, M. K., Christoffels, V. M., Dias, J. M., Trowe, M. O., Petry, M., Schuster-Gossler, K., et al. (2005). Tbx20 is essential for cardiac chamber differentiation and repression of Tbx2. Development 132, 2697–2707. doi: 10.1242/dev.01854
Singh, R., Horsthuis, T., Farin, H. F., Grieskamp, T., Norden, J., Petry, M., et al. (2009). Tbx20 interacts with smads to confine tbx2 expression to the atrioventricular canal. Circ. Res. 105, 442–452. doi: 10.1161/CIRCRESAHA.109.196063
Singh, R., and Kispert, A. (2010). Tbx20, Smads, and the atrioventricular canal. Trends Cardiovasc. Med. 20, 109–114. doi: 10.1016/j.tcm.2010.09.004
Sotoodehnia, N., Isaacs, A., de Bakker, P. I., Dorr, M., Newton-Cheh, C., Nolte, I. M., et al. (2010). Common variants in 22 loci are associated with QRS duration and cardiac ventricular conduction. Nat. Genet. 42, 1068–1076. doi: 10.1038/ng.716
Stennard, F. A., Costa, M. W., Elliott, D. A., Rankin, S., Haast, S. J., Lai, D., et al. (2003). Cardiac T-box factor Tbx20 directly interacts with Nkx2-5, GATA4, and GATA5 in regulation of gene expression in the developing heart. Dev. Biol. 262, 206–224. doi: 10.1016/S0012-1606(03)00385-3
Stennard, F. A., Costa, M. W., Lai, D., Biben, C., Furtado, M. B., Solloway, M. J., et al. (2005). Murine T-box transcription factor Tbx20 acts as a repressor during heart development, and is essential for adult heart integrity, function, and adaptation. Development 132, 2451–2462. doi: 10.1242/dev.01799
Stennard, F. A., and Harvey, R. P. (2005). T-box transcription factors and their roles in regulatory hierarchies in the developing heart. Development 132, 4897–4910. doi: 10.1242/dev.02099
Svendsen, P. C., Formaz-Preston, A., Leal, S. M., and Brook, W. J. (2009). The Tbx20 homologs midline and H15 specify ventral fate in the Drosophila melanogaster leg. Development 136, 2689–2693. doi: 10.1242/dev.037911
Szeto, D. P., Griffin, K. J., and Kimelman, D. (2002). HrT is required for cardiovascular development in zebrafish. Development 129, 5093–5101.
Takeuchi, J. K., Mileikovskaia, M., Koshiba-Takeuchi, K., Heidt, A. B., Mori, A. D., Arruda, E. P., et al. (2005). Tbx20 dose-dependently regulates transcription factor networks required for mouse heart and motoneuron development. Development 132, 2463–2474. doi: 10.1242/dev.01827
Trudeau, M. C., Warmke, J. W., Ganetzky, B., and Robertson, G. A. (1995). HERG, a human inward rectifier in the voltage-gated potassium channel family. Science 269, 92–95. doi: 10.1126/science.7604285
van den Berg, G., and Moorman, A. F. (2009). Concepts of cardiac development in retrospect. Pediatr. Cardiol. 30, 580–587. doi: 10.1007/s00246-008-9369-y
van Weerd, J. H., and Christoffels, V. M. (2016). The formation and function of the cardiac conduction system. Development 143, 197–210. doi: 10.1242/dev.124883
Watanabe, Y., Zaffran, S., Kuroiwa, A., Higuchi, H., Ogura, T., Harvey, R. P., et al. (2012). Fibroblast growth factor 10 gene regulation in the second heart field by Tbx1, Nkx2-5, and Islet1 reveals a genetic switch for down-regulation in the myocardium. Proc. Natl. Acad. Sci. U.S.A. 109, 18273–18280. doi: 10.1073/pnas.1215360109
Wiese, C., Grieskamp, T., Airik, R., Mommersteeg, M. T., Gardiwal, A., de Gier-de Vries, C., et al. (2009). Formation of the sinus node head and differentiation of sinus node myocardium are independently regulated by Tbx18 and Tbx3. Circ. Res. 104, 388–397. doi: 10.1161/CIRCRESAHA.108.187062
Wolf, C. M., and Berul, C. I. (2006). Inherited conduction system abnormalities—one group of diseases, many genes. J. Cardiovasc. Electrophysiol. 17, 446–455. doi: 10.1111/j.1540-8167.2006.00427.x
Wu, S. P., Dong, X. R., Regan, J. N., Su, C., and Majesky, M. W. (2013). Tbx18 regulates development of the epicardium and coronary vessels. Dev. Biol. 383, 307–320. doi: 10.1016/j.ydbio.2013.08.019
Xiang, F. L., Guo, M., and Yutzey, K. E. (2016). Overexpression of Tbx20 in adult cardiomyocytes promotes proliferation and improves cardiac function after myocardial infarction. Circulation 133, 1081–1092. doi: 10.1161/CIRCULATIONAHA.115.019357
Xu, H., Morishima, M., Wylie, J. N., Schwartz, R. J., Bruneau, B. G., Lindsay, E. A., et al. (2004). Tbx1 has a dual role in the morphogenesis of the cardiac outflow tract. Development 131, 3217–3227. doi: 10.1242/dev.01174
Yamaguchi, T. P., Takada, S., Yoshikawa, Y., Wu, N., and McMahon, A. P. (1999). T (Brachyury) is a direct target of Wnt3a during paraxial mesoderm specification. Genes Dev. 13, 3185–3190. doi: 10.1101/gad.13.24.3185
Keywords: Tbx20, heart, development, function, congenital heart defects
Citation: Chen Y, Xiao D, Zhang L, Cai C-L, Li B-Y and Liu Y (2021) The Role of Tbx20 in Cardiovascular Development and Function. Front. Cell Dev. Biol. 9:638542. doi: 10.3389/fcell.2021.638542
Received: 07 December 2020; Accepted: 08 January 2021;
Published: 28 January 2021.
Edited by:
Weinian Shou, Indiana University Bloomington, United StatesReviewed by:
Wuqiang Zhu, Mayo Clinic Arizona, United StatesCopyright © 2021 Chen, Xiao, Zhang, Cai, Li and Liu. This is an open-access article distributed under the terms of the Creative Commons Attribution License (CC BY). The use, distribution or reproduction in other forums is permitted, provided the original author(s) and the copyright owner(s) are credited and that the original publication in this journal is cited, in accordance with accepted academic practice. No use, distribution or reproduction is permitted which does not comply with these terms.
*Correspondence: Bai-Yan Li, bGlieUBlbXMuaHJibXUuZWR1LmNu; Ying Liu, bGl1eWluZ0BpdS5lZHU=
Disclaimer: All claims expressed in this article are solely those of the authors and do not necessarily represent those of their affiliated organizations, or those of the publisher, the editors and the reviewers. Any product that may be evaluated in this article or claim that may be made by its manufacturer is not guaranteed or endorsed by the publisher.
Research integrity at Frontiers
Learn more about the work of our research integrity team to safeguard the quality of each article we publish.