- 1Department of Human Genetics, Yokohama City University Graduate School of Medicine, Yokohama, Japan
- 2Department of Pediatrics, Hamamatsu University School of Medicine, Hamamatsu, Japan
- 3Department of Molecular and Human Genetics, Baylor College of Medicine, Houston, TX, United States
- 4Texas Children’s Hospital, Houston, TX, United States
- 5Department of Molecular Physiology and Biophysics, Baylor College of Medicine, Houston, TX, United States
- 6Department of Biochemistry, Yokohama City University Graduate School of Medicine, Yokohama, Japan
- 7Baylor Genetics, Houston, TX, United States
- 8Department of Pediatrics, Hamamatsu City Welfare and Medical Center for Development, Hamamatsu, Japan
Background: X-linked intellectual disability (XLID), which occurs predominantly in males, is a relatively common and genetically heterogeneous disorder in which over 100 mutated genes have been reported. The OTUD5 gene at Xp11.23 encodes ovarian tumor deubiquitinase 5 protein, which is a deubiquitinating enzyme member of the ovarian tumor family. LINKage-specific-deubiquitylation-deficiency-induced embryonic defects (LINKED) syndrome, arising from pathogenic OTUD5 variants, was recently reported as a new XLID with additional congenital anomalies.
Methods: We investigated three affected males (49- and 47-year-old brothers [Individuals 1 and 2] and a 2-year-old boy [Individual 3]) from two families who showed developmental delay. Their common clinical features included developmental delay, hypotonia, short stature, and distinctive facial features, such as telecanthus and a depressed nasal bridge. Individuals 1 and 2 showed epilepsy and brain magnetic resonance imaging showed a thin corpus callosum and mild ventriculomegaly. Individual 3 showed congenital malformations, including tetralogy of Fallot, hypospadias, and bilateral cryptorchidism. To identify the genetic cause of these features, we performed whole-exome sequencing.
Results: A hemizygous OTUD5 missense variant, c.878A>T, p.Asn293Ile [NM_017602.4], was identified in one family with Individuals 1 and 2, and another missense variant, c.1210 C>T, p.Arg404Trp, in the other family with Individual 3, respectively. The former variant has not been registered in public databases and was predicted to be pathogenic by multiple in silico prediction tools. The latter variant p.Arg404Trp was previously reported as a pathogenic OTUD5 variant, and Individual 3 showed a typical LINKED syndrome phenotype. However, Individuals 1 and 2, with the novel variant (p.Asn293Ile), showed no cardiac or genitourinary malformations.
Conclusions: Unlike previous reports of LINKED syndrome, which described early lethality with congenital cardiac anomalies, our three cases are still alive. Notably, the adult brothers with the novel missense OTUD5 variant have lived into their forties. This may be indicative of a milder phenotype as a possible genotype-phenotype correlation. These findings imply a possible long-term prognosis for individuals with this new XLID syndrome, and a wider phenotypic variation than initially thought.
Introduction
X-linked intellectual disability (XLID) is a relatively common disorder that is predominantly observed in males. XLID is genetically heterogeneous, and is caused by more than 100 protein-coding genes in the X chromosome (Muthusamy et al., 2017; Neri et al., 2018). Aberrations in these X-linked genes are estimated to account for 5–10% of male patient with intellectual disability (Lubs et al., 2012; Tzschach et al., 2015; Muthusamy et al., 2017). Next-generation sequencing has led to a marked increase in identification of disease-causing variants in many new and previously unresolved cases of familial and sporadic genetic disorders (Muthusamy et al., 2017; Neri et al., 2018).
The OTUD5 gene (MIM#300713) at Xp11.23 encodes ovarian tumor (OTU) deubiquitinase 5 protein, which is a deubiquitinating enzymes (DUBs) member of the OTU family (Mevissen et al., 2013). DUBs are proteases that specifically cleave ubiquitin linkages. There are approximately 100 DUBs, including OTUD5, in humans (Komander et al., 2009; Mevissen et al., 2013). OTU DUBs are important regulators of the ubiquitin code, and therefore, they control crucial physiological processes in humans (Kulathu and Komander, 2012; Mevissen et al., 2013). Abnormalities in genes encoding OTU DUBs cause various developmental or autoinflammatorydiseases (Basar et al., 2020). Recently, a male-specific multiple congenital anomaly disorder caused by pathogenic variants in OTUD5 was reported by two groups (Beck et al., 2021; Tripolszki et al., 2021). Tripolszki et al. described a large family with 13 affected individuals (Tripolszki et al., 2021). Beck et al. independently reported ten pediatric cases from seven different families with an OTUD5 pathogenic variant and named this disease LINKage-specific-deubiquitylation-deficiency-induced embryonic defects (LINKED) syndrome (Beck et al., 2021). Affected individuals were characterized by global developmental delay, congenital cardiac anomalies, genitourinary abnormalities, and dysmorphic faces.
In the present study, we used whole-exome sequencing (WES) to identify pathogenic hemizygous variants in three individuals from two families with suspected XLID.
Materials and Methods
Subjects
We used the GeneMatcher (Sobreira et al., 2015) system to connect two centers at Yokohama City University Graduate School of Medicine (Yokohama, Japan) and Baylor College of Medicine (Houston, TX, United States). Written informed consent was obtained from the patients’ or their parents based on experimental protocols, approved by the Institutional Review Board of each center. Affected Individuals 1 and 2 were 49- and 47-year-old Japanese brothers (Figure 1A; II-2 and II-3) born to healthy consanguineous parents (the parents were second cousins). These patients had an unaffected sister (Figure 1A; II-1). Genomic DNA samples were extracted from peripheral blood leukocytes of the affected brothers and an unrelated control using a QIAamp DNA Mini Kit (Qiagen, Hilden, Germany). Individual 3 (Figure 2A; II-1) was a 2-year-old boy of European descent. He was the first child born to non-consanguineous parents (Figure 2A; I-1,2). The family history was non-contributory.
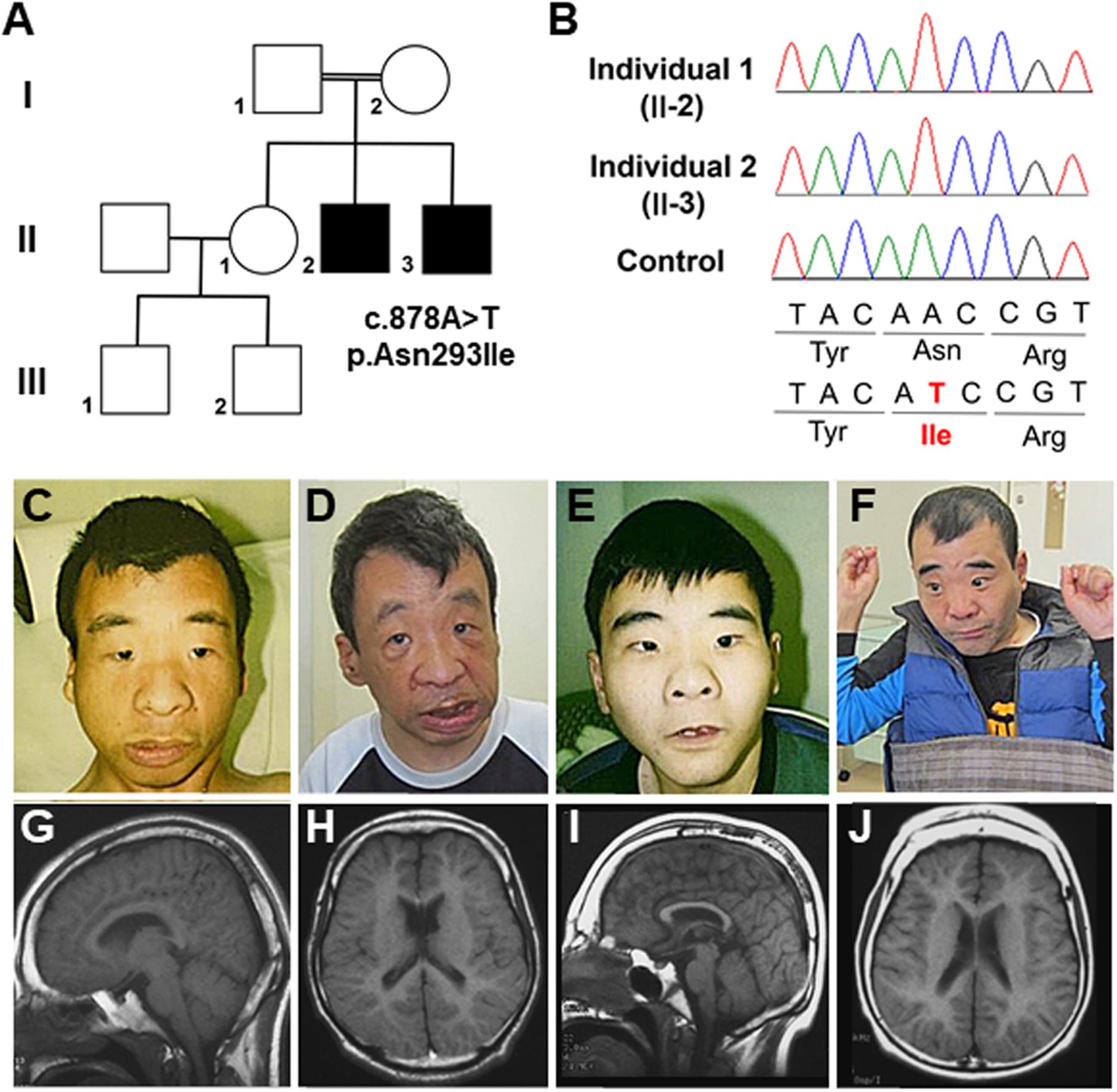
Figure 1. (A) Familial pedigree of the affected male siblings. (B) Electropherograms of the OTUD5 variant (c.878A>T, p.Asn293Ile [NM_017602.4]) in the affected brothers and the control. (C,D) Photographs of the older brother (II-2) at 22 and 48 years of age. (E,F) Photographs of the younger brother (II-3) at 20 and 46 years of age. (G,H) Brain magnetic resonance images (MRI). Sagittal and coronal views of T1 susceptibility-weighted images of the older brother at 24 years of age are shown. A thin corpus callosum, a small frontal lobe, and volume loss of the subcortical white matter and cavity of the septum pellucidum can be seen. (I,J) Brain MRI. Sagittal and coronal views of T1 susceptibility-weighted (SW) images of the younger brother at 22 years of age are shown. A thin corpus callosum, frontal lobe hypoplasia, volume loss of the subcortical white matter, colpocephaly, and hypoplasia of the cerebellar hemispheres can be seen.
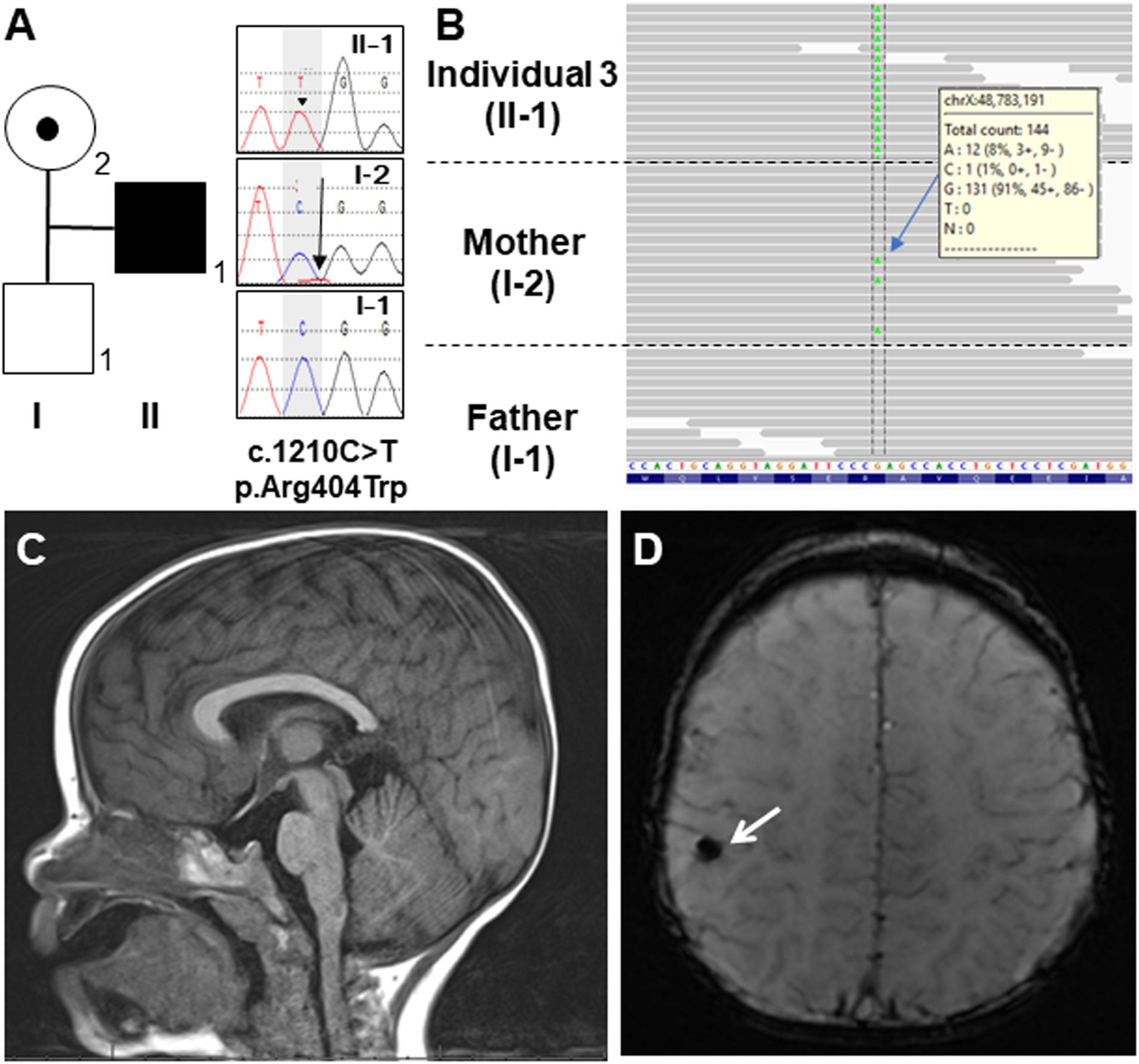
Figure 2. (A) Familial pedigree of Individual 3 and DNA sequence electropherograms illustrating the OTUD5 variant (c.1210C>T, p.Asn404Trp [NM_017602.4]). Arrow head of the proband (II-1) in the electropherogram shows the mutated allele (C>T) in a hemizygous state. The arrow in the mother’s electropherogram (I-2) indicates the mutated allele in a mosaic state. (B) Integrated genomic view demonstrating that the variant can be seen in the hemizygous state in Individual 3 and in some of the reads from his mosaic mother. (C) Sagittal view of the brain MRI of Individual 3 at 19 months of age. His corpus callosum was normal. (D) Coronal view. The white arrow showed a hypointense focus in the right perirolandic region, which most likely represents hemosiderin deposition secondary to a previous microhemorrhage.
WES and Protein Structure Analysis
For Individuals 1 and 2, WES was performed using an illumina platform as previously described (Miyake et al., 2020). The mean read depth of the RefSeq coding region was 98.3 and 113.6 reads (corresponding to 96.1%- and 96.4%-, respectively, covered by >20 reads), respectively. Analyses based on autosomal dominant (de novo), autosomal recessive (homozygous and compound heterozygous), and X-linked models were conducted. For X-linked recessive conditions, we picked up candidate genetic variants in exons and canonical splice sites (±2 bp) with a minor allele frequency of <0.005 using the Exome Aggregation Consortium browser (ExAC), NHLBI Exome Variant Server (ESP6500), Human Gene Mutation Database (HGMD) and in-house WES Japanese control data (n = 575). Candidate variants were prioritized based on the biological and clinical relevance of each gene to the phenotype of the patients. Potential candidate variants were validated using the Sanger method, using an ABI3500xL sequencer (Applied Biosystems, Foster City, CA, United States) and Sequencher 5.0 (Gene Codes Corporation, Ann Arbor, MI, United States). Structural analysis and figure preparation of the OTUD5 protein were performed using PyMOL (Schrödinger, Inc., New York, NY, United States).
For Individual 3, array-based copy number variant (CNV) analysis (CMA-HR + SNP version 11.2) and trio WES were performed on a clinical basis at Baylor Genetics1 (Yang et al., 2013, 2014; Wiszniewska et al., 2014). The following quality control metrics for exome sequencing are generally achieved: >70% of reads are aligned to the target with >95% of targeted bases covered at >20 reads, >85% of targeted bases are covered at >40 reads, and mean coverage of targeted bases is >100 reads.
Results
Clinical Findings
Individual 1, the older brother (II-2), was born at 42 weeks of gestational age by spontaneous vaginal delivery. His birth weight, length, and head circumference were 2,700 g [–0.6 standard deviation (SD)], 46.5 cm (–1.2 SD), and 31.5 cm (–1.3 SD), respectively. At 1 month of age, he appeared cyanotic directly after feeding; however, echocardiography revealed no cardiac abnormalities. To prevent cyanosis, gastric tube feeding was administered for several months. His developmental milestones were delayed. He had head control at 6 months, crawled at 30 months, and walked independently at 4 years. He spoke no meaningful words and gained no language comprehension. Dysmorphic features, including thick eyebrows, telecanthus, widely spaced eyes, down-slanted palpebral fissure, a wide and depressed nasal bridge, a wide nasal base, thin upper lip, and thick lower lip, have been noted since his infancy (Figures 1C,D). At 4 years of age, he experienced generalized tonic–clonic seizures, and his electroencephalogram showed paroxysmal discharges. Therefore, he started medication with phenobarbital and carbamazepine; however, his seizures continued once a month until adolescence. At 22 years of age, his height was 142 cm (–5.0 SD) and his weight was 41.0 kg (–2.1 SD). He was able to walk independently, although his gait was short-stepped with arm flexion and knee extension. During his most recent evaluation, at 48 years of age, he was still able to walk independently and his epileptic seizures were controlled with carbamazepine and zonisamide; however, he had intellectual disability.
Individual 2 (II-3) is the younger brother of Individual 1. He was born at 39 weeks of gestational age by spontaneous vaginal delivery, with no complications. His birth weight, length, and head circumference were 2,600 g (–1.4 SD), 46.0 cm (–1.0 SD), and 31.0 cm (–1.6 SD), respectively. He showed significant phenotypic overlap with Individual 1, including the dysmorphic features (Figures 1E,F) and delayed developmental milestones; he had head control at 7 months, sat with support at 22 months, and crawled at 24 months. He was able to stand with support at 4 years, but was never able to walk. He spoke no meaningful words and gained no language comprehension. At 1 year of age, he also began to experience generalized tonic–clonic seizures. Although he was treated with valproate, clonazepam, and carbamazepine, drop attacks occurred once every few months. At 20 years of age, his height was 134.3 cm (–6.3 SD) and his weight was 37.1kg (–2.5 SD). He was able to stand with support, with flexed knee joints and ankle valgus. During his most recent evaluation, at 46 years of age, he was unable to stand alone and had intellectual disability. He usually sat with his shoulder joints flexed forward (at approximately 80°), flexed elbow joints, forearm pronation, and with his palms grasped softly. A physical examination showed no joint contracture. Left cryptorchidism was noted. Monthly epileptic drop seizures remained, even under medication with carbamazepine, valproate, and clonazepam.
Brain magnetic resonance imaging (MRI) of Individual 1 at the age of 24 years and of Individual 2 at the age of 22 years revealed a thin corpus callosum or colpocephaly, a small frontal lobe, and volume loss of the subcortical white matter (Figures 1G–J). Ultrasonographic examination of the heart and kidney was normal. G-banded chromosome analysis revealed normal karyotypes (46, XY) in both affected brothers. Array comparative genomic hybridization analysis indicated a duplication of the 5q31.1 region (a region of chr5:130551687–130740870 in Individual 1 and chr5:130484163–130731837 in Individual 2, based on hg19) (Asahina et al., 2016). However, there have been few reports of individuals with 5q31.1 duplications, and a characteristic phenotype is not obvious (Rosenfeld et al., 2011). Moreover, our patients did not share many aspects of the phenotypes of other individuals with 5q31.1 duplications. Therefore, the pathogenic significance of the 5q31.1 duplication in our patients remains unknown.
Individual 3 is a 2-year-5-month boy with no history of prenatal teratogenic exposure. A 20-week ultrasound revealed hyperechoic areas in the brain and heart. These areas were not identified on subsequent ultrasound examinations, but unilateral, renal hyperechogenicity with pelviectasis was observed. Individual 3 was delivered at 40 weeks’ gestation via cesarean section because of failure to progress. After birth, he was found to have a cardiac murmur, penoscrotal hypospadias, bilateral cryptorchidism, bilateral inguinal hernias, ankyloglossia, and a high arched palate. An echocardiogram revealed tetralogy of Fallot (TOF) with valvular and subvalvular pulmonic stenosis. A post-natal renal ultrasound was normal. He was discharged at one week of age. His TOF was repaired at 7 months of age, and he had bilateral ochiopexy and inguinal hernia repair at 11 months of age.
At 2 months of age, Individual 3 was noted to have feeding difficulties and failure to thrive. He was diagnosed with gastroesophageal reflux disease (GERD) at 3 months of age. This diagnosis was confirmed during a videofluoroscopic swallow study performed at age 7 months that also revealed esophageal dysmotility and minimal laryngeal vestibular penetration with thin liquids. This was treated by using a different artificial nipple for feeds. His GERD is currently being treated with lansoprazole. Over time, global developmental delay and diffuse hypotonia were also observed. He rolled over at 5–7 months of age and sat at 6 months of age but is still unable to walk independently and he does not use any words or signs. Brain MRI at 19 months of age was normal except for a punctate T2 hypointense focus in the right perirolandic region with corresponding blooming on susceptibility-weighted imaging (SWI) that most likely represents hemosiderin deposition secondary to a previous microhemorrhage (Figures 2C,D).
A skeletal survey obtained at 18 months of age due to short stature and abnormal, circumferential fat distribution over the extremities was normal. However, at 19 months of age he was diagnosed with growth hormone deficiency based on the finding of undetectable insulin-like growth factor-1 and insulin-like growth factor binding protein-3 levels and bilateral pseudopapilledema (Collett-Solberg et al., 1998). He was treated with growth hormone starting at 20 months of age. After starting growth hormone treatment, his abnormal fat distribution resolved and his parents noted an increased appetite, activity and engagement in therapies. However, there was no appreciable change in his height velocity or weight. At 27 months of age his length was 74.4 cm (<0.01 centile, Z = −4.46) and his weight was 8.15 kg (<0.01 centile, Z = −4.76).
Individual 3’s last comprehensive physical examination was at 19 months of age. He had brachycephaly, a prominent forehead, deep set eyes, intermittent bilateral esotropia, and a short nose with a flat nasal root and bulbous tip. He had a left-sided supernumerary nipple and penoscrotal hypospadias. His height was 69.3 cm (<0.01 centile, Z = −4.48), his weight was 8 kg (<0.01 centile, Z = −3.82), and his head circumference was 46.5 cm (13th centile). Array-based copy number variant analysis was normal.
Identification of the Pathogenic Variant
By searching for candidate variants shared in both of the affected Individuals 1 and 2, and comparing their phenotypes with the variant’s relevance, we identified missense variants in OTUD5 (c.878A>T, p.Asn293Ile [NM_017602.4]) and ATP2B3 (c.2768G>A, p.Arg923His [NM_001001344.2]), both located on the X chromosome. Sanger sequencing confirmed that the OTUD5 and ATP2B3 variants were hemizygous in both affected siblings. Unfortunately, parental samples could not be obtained. Because the ATP2B3 variant (c.2768G>A, p.Arg923His, rs201753621) was found in the Genome Aggregation Database (gnomAD) in the hemizygous state (3 of 183,343 alleles), we felt that it was unlikely to be causative. The OTUD5 variant was absent from all searched public databases (gnomAD, ExAC, ESP6500, and HGMD) and our in-house control database. Moreover, according to the gnomAD database, OTUD5 is intolerant to amino acid substitutions and loss-of-function alleles (0 observed vs. 17.2 expected, loss-of-function intolerance score: 1.0); the missense intolerance (Z) score is 3.99 (44 observed single nucleotide variants vs. 204.9 expected single nucleotide variants). The altered amino residue in our patients was located on the OTU domain and is highly conserved in multiple species (Figure 3A). Web-based in silico software predicted this variant to be pathogenic, with a predicted value of 0.002 (deleterious) in SIFT, 0.999 (probably damaging) in PolyPhen-2, and 1 (disease-causing) in MutationTaster, and a combined annotation dependent depletion score (CADD) of 27.7 (>20). In the crystal structure of OTUD5 bound with ubiquitin (Huang et al., 2012), Asp256, Arg274, and Asn293 are located on the surface of the protein, and form no direct interactions with ubiquitin (Figure 3B). We used IntarVar to evaluate the variant (Li and Wang, 2017), and the results showed that this variant (c.878A>T, p.Asn293Ile) is currently considered a “variant of unknown significance” (VUS, PM1, PM2, and PP3) based on ACMG-AMP guidelines (Richards et al., 2015).
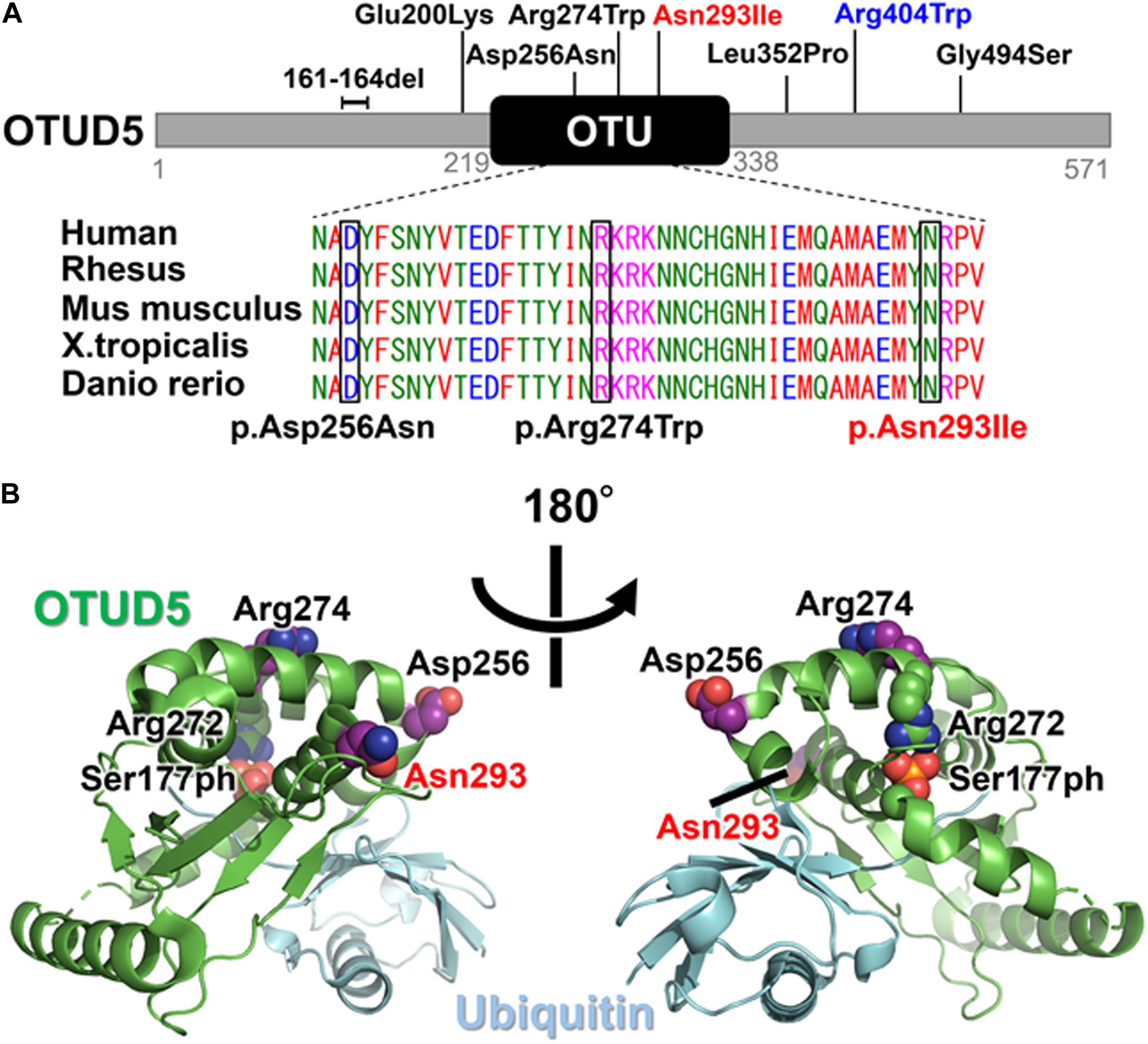
Figure 3. (A) Schematic presentation of the OTUD5 protein and the pathogenic variants. The affected amino acid in the OTU domain is conserved among several species, from humans to fish. The protein sequences of the different species were aligned using Clustal Omega. The previously reported variants are in black and the novel variant (Asn293Ile) is shown in red above the protein. The recurrent variant (Arg404Trp) identified in this study and reported by Beck et al. is shown in blue. (B) The crystal structure of the Ser177-phosphorylated form of OTUD5 bound with ubiquitin (PDB ID: 3TMP). OTUD5 and ubiquitin are shown in green and cyan, respectively. The pathogenic variant positions (Asp256, Arg274, and Asn293) are indicated.
In Individual 3, trio exome sequencing performed on a clinical basis revealed a hemizygous c.1210 C>T, p.Arg404Trp variant in OTUD5. This variant was inherited from his mother who was mosaic for the variant (Figures 2A,B). The arginine at position 404 in OTUD5 is highly conserved. This change was predicted to be deleterious by SIFT, probably damaging by PolyPhen2, disease causing by MutationTaster and has a CADD score of 33 as shown in Table 1. This variant was classified as “likely pathogenic” (PS1, PM2, PP3, and PP4) based on ACMG-AMP guidelines (Richards et al., 2015).
Discussion
In the present study, three affected individuals with OTUD5 missense variants were identified who had short stature and developmental delay with distinctive facial features. Individuals 1 and 2 were male siblings and had a novel OTUD5 variant (c.878A>T, Asn293Ile) and sporadic Individual 3 had a known OTUD5 variant (c.1210C>T, Arg404Trp). Including our patients, almost all of the identified OTUD5 variants in affected individuals have been missense variants (Beck et al., 2021; Tripolszki et al., 2021).
The high loss-of-function intolerance score and the especially high Z score (3.99, within the top 3% of genes on the X chromosome) for OTUD5 that were obtained from intolerance tests in the gnomAD database strongly suggest that this gene, with its variants, could be a strong candidate for causing X-linked disorders in humans. The OTUD5 gene is ubiquitously expressed in human tissues. OTUD5 is an essential component for chromatin remodeling of gene regulatory elements and plays an important role during embryogenesis (Beck et al., 2021).
The novel variant that was identified in the current study (c.878A>T, p.Asn293Ile) is located in the OTU domain, which confers deubiquitinase activity. Within the OTU domain, two other pathogenic variants (c.766G>A, p.Asp256Asn and c.820C>T, p.Arg274Trp) have previously been reported (Figure 3A; Beck et al., 2021). The catalytic activity of OTUD5 is activated when Ser177 is phosphorylated (Huang et al., 2012). In the crystal structure of the Ser177-phosphorylated form of OTUD5 bound with ubiquitin (Huang et al., 2012), the phosphate group has intramolecular interactions with other OTUD5 polar residues (including Arg272), and also has intermolecular interactions with the C-terminal tail of the bound ubiquitin, precisely orienting its C-terminus for catalysis. The pathogenic residues (Asp256, Arg274, and Asn293) are all mapped onto the surface of the protein, suggesting that the variant proteins may not have compromised folding activity. Furthermore, these residues form no direct interactions with either the bound ubiquitin or the phosphate group of Ser177. However, these three residues are all located on the same side of OTUD5. Therefore, these residues might cooperatively mediate interactions with some protein factors that regulate the cellular function of OTUD5, such as the Ser177 kinase. Alternatively, because Arg274 is located close to Arg272, which is one of the critical residues for phosphorylation-dependent activation, it may be involved in structural transition upon Ser177 phosphorylation.
In Individual 3, the known pathogenic variant (c.1210C>T, Arg404Trp) was inherited from his mother who was mosaic. The OTUD5 variant identified in Individuals 1 and 2 was assumed to be inherited from their mother, but unfortunately a maternal sample was unavailable for this study. Tripolszki et al. reported that females carriers were asymptomatic and X-inactivation study showed 100% inactivation of the maternally inherited X chromosome in four female carriers (Tripolszki et al., 2021).
Phenotypically, early lethality was described in both previous reports associated with OTUD5 pathogenic variants (Beck et al., 2021; Tripolszki et al., 2021). Causes of death included congenital cardiac complications or sepsis. A clinical summary of the previous studies and the three cases from the present study is shown in Table 2. Our three affected individuals are still alive. Individual 3, who had the known variant p. Arg404Trp, displayed a similar phenotype as that in a previous report, with congenital anomalies such as hypospadias and heart defects, whereas no obvious structural abnormalities in the brain, nor seizures were observed. Individuals 1 and 2, who had the novel variant p.Asn293Ile, showed a relatively milder phenotype. Both affected brothers had severe short stature and refractory epilepsy, even while taking anti-epileptic drugs. Unlike the patients in the previous reports, Individuals 1 and 2 did not show any congenital cardiac anomalies or genitourinary anomalies and have lived to almost 50 years old, suggesting that cardiac involvement may be associated with poor prognosis.
Symptoms frequently observed were global developmental delay (100%), abnormal head imaging (95%), hypotonia (87%), short stature (90%), distinctive facial features (87%), and feeding diffilulty in infancy (83%). Congenital heart malformations (64%) and hypospadias (63%) are also relatively common. Of note, the distinctive facial features of our three patients are very similar to those of cases in the previous reports mentioned above (Beck et al., 2021; Tripolszki et al., 2021). In the clinical setting, the facial gestalt approach may be useful for diagnosing this syndrome. In addition, the younger brother was unable to walk, whereas the older brother was able to walk with support. This finding suggest that differences in severity can occur even with the same variant, which was also mentioned in a previous report (Tripolszki et al., 2021).
The phenotypes of our patients may help to clarify the genotype–phenotype correlation of this new X-linked disorder and allow for better understanding of the long-term prognosis of these patients. In conclusion, we described three living patients with OTUD5 missense variants associated with LINKED syndrome. LINKED syndrome may have a longer life expectancy than previously thought.
Web Resources Used for This Study
CADD: https://cadd.gs.washington.edu/snv
Clustal Omega: https://www.ebi.ac.uk/Tools/msa/clustalo/
ExAC: http://exac.broadinstitute.org/
gnomAD: http://gnomad.broadinstitute.org/
HGMD: http://www.hgmd.cf.ac.uk/ac/index
MutationTaster: http://www.mutationtaster.org/
NHLBI Exome Variant Server (ESP6500): http://evs.gs.washington.edu/EVS/
OMIM: https://www.omim.org/
PolyPhen-2: http://genetics.bwh.harvard.edu/pph2/
Protein Data Bank: http://www.wwpdb.org/
SIFT: http://sift.jcvi.org/
Data Availability Statement
The original contributions presented in the study are included in the article/supplementary material, further inquiries can be directed to the corresponding author/s.
Ethics Statement
The studies involving human participants were reviewed and approved by The Institutional Review Board of Yokohama City University School of Medicine and Baylor College of Medicine. Written informed consent was obtained from the individual(s) for the publication of any potentially identifiable images or data included in this article.
Author Contributions
KS performed the genetic analysis, interpreted the data, and wrote the manuscript. TF recruited the patients, performed the clinical evaluation, and wrote the manuscript. DS and AN evaluated the patients and contributed to the manuscript revising. TS and KO conducted protein structure analysis. AH-G helped to organize patient data. SL designed the research protocol. MSA consented patient. HS ordered genetic testing. PL and HD performed genetic testing. TM, SM, and MA contributed to genetic data analysis. TO, NMi, and NMa conducted and supervised the study, evaluated the data, and wrote the manuscript. All authors contributed to the article and approved the submitted version.
Funding
This work was supported by the Japan Agency for Medical Research and Development (AMED) under grant numbers JP20ek0109486, JP20dm0107090, JP20ek0109301, JP20ek0109348, and JP20kk0205012 (NMa), JSPS KAKENHI under grant numbers JP17H01539 (NMa), and JP19H03621 (NMi), Intramural research grants for Neurological and Psychiatric Disorders of NCNP from the Ministry of Health, Labour and Welfare under grant numbers 30-6 (NMa) and 30-7 (NMa), and the Takeda Science Foundation (NMi and NMa). This work was also supported by the National Institutes of Health/Eunice Kennedy Shriver National Institute of Child Health and Human Development grant R01 HD098458 to DS.
Conflict of Interest
The authors declare that the research was conducted in the absence of any commercial or financial relationships that could be construed as a potential conflict of interest.
Acknowledgments
We thank the families for their participation in this study.
Abbreviations
DUBs, deubiquitinating enzymes; LINKED, LINKage-specific-deubiquitylation-deficiency-induced embryonic defects; MRI, magnetic resonance imaging; OTU, ovarian tumor; SD, standard deviation; XLID, X-linked intellectual disability; WES, whole-exome sequencing.
Footnotes
References
Asahina, M., Endoh, Y., Matsubayashi, T., Hirano, K., Fukuda, T., and Ogata, T. (2016). Genomewide array comparative genomic hybridization in 55 Japanese normokaryotypic patients with non-syndromic intellectual disability. J. Pediatr. Neurol. Disord. 2:108. doi: 10.4172/2572-5203.1000108
Basar, M. A., Beck, D. B., and Werner, A. (2020). Deubiquitylases in developmental ubiquitin signaling and congenital diseases. Cell Death Differ. doi: 10.1038/s41418-020-00697-5 [Epub ahead of print].
Beck, D. B., Basar, M. A., Asmar, A. J., Thompson, J., Oda, H., Uehara, D. T., et al. (2021). Linkage-specific deubiquitylation by OTUD5 defines an embryonic pathway intolerant to genomic variation. Sci. Adv. 7:eabe2116. doi: 10.1126/sciadv.abe2116
Collett-Solberg, P. F., Liu, G. T., Satin-Smith, M., Katz, L. L., and Moshang, T. Jr. (1998). Pseudopapilledema and congenital disc anomalies in growth hormone deficiency. J. Pediatr. Endocrinol. Metab. 11, 261–265. doi: 10.1515/jpem.1998.11.2.261
Huang, O. W., Ma, X., Yin, J., Flinders, J., Maurer, T., Kayagaki, N., et al. (2012). Phosphorylation-dependent activity of the deubiquitinase DUBA. Nat. Struct. Mol. Biol. 19, 171–175. doi: 10.1038/nsmb.2206
Komander, D., Clague, M. J., and Urbe, S. (2009). Breaking the chains: structure and function of the deubiquitinases. Nat. Rev. Mol. Cell Biol. 10, 550–563. doi: 10.1038/nrm2731
Kulathu, Y., and Komander, D. (2012). Atypical ubiquitylation – the unexplored world of polyubiquitin beyond Lys48 and Lys63 linkages. Nat. Rev. Mol. Cell Biol. 13, 508–523. doi: 10.1038/nrm3394
Li, Q., and Wang, K. (2017). InterVar: clinical interpretation of genetic variants by the 2015 ACMG-AMP guidelines. Am. J. Hum. Genet. 100, 267–280. doi: 10.1016/j.ajhg.2017.01.004
Lubs, H. A., Stevenson, R. E., and Schwartz, C. E. (2012). Fragile X and X-linked intellectual disability: four decades of discovery. Am. J. Hum. Genet. 90, 579–590. doi: 10.1016/j.ajhg.2012.02.018
Mevissen, T. E., Hospenthal, M. K., Geurink, P. P., Elliott, P. R., Akutsu, M., Arnaudo, N., et al. (2013). OTU deubiquitinases reveal mechanisms of linkage specificity and enable ubiquitin chain restriction analysis. Cell 154, 169–184. doi: 10.1016/j.cell.2013.05.046
Miyake, N., Takahashi, H., Nakamura, K., Isidor, B., Hiraki, Y., Koshimizu, E., et al. (2020). Gain-of-function MN1 truncation variants cause a recognizable syndrome with craniofacial and brain abnormalities. Am. J. Hum. Genet. 106, 13–25. doi: 10.1016/j.ajhg.2019.11.011
Muthusamy, B., Selvan, L. D. N., Nguyen, T. T., Manoj, J., Stawiski, E. W., Jaiswal, B. S., et al. (2017). Next-generation sequencing reveals novel mutations in X-linked intellectual disability. OMICS 21, 295–303. doi: 10.1089/omi.2017.0009
Neri, G., Schwartz, C. E., Lubs, H. A., and Stevenson, R. E. (2018). X-linked intellectual disability update 2017. Am. J. Med. Genet. A 176, 1375–1388. doi: 10.1002/ajmg.a.38710
Richards, S., Aziz, N., Bale, S., Bick, D., Das, S., Gastier-Foster, J., et al. (2015). Standards and guidelines for the interpretation of sequence variants: a joint consensus recommendation of the American College of Medical Genetics and Genomics and the Association for Molecular Pathology. Genet. Med. 17, 405–424. doi: 10.1038/gim.2015.30
Rosenfeld, J. A., Drautz, J. M., Clericuzio, C. L., Cushing, T., Raskin, S., Martin, J., et al. (2011). Deletions and duplications of developmental pathway genes in 5q31 contribute to abnormal phenotypes. Am. J. Med. Genet. A 155A, 1906–1916. doi: 10.1002/ajmg.a.34100
Sobreira, N., Schiettecatte, F., Valle, D., and Hamosh, A. (2015). GeneMatcher: a matching tool for connecting investigators with an interest in the same gene. Hum. Mutat. 36, 928–930. doi: 10.1002/humu.22844
Tripolszki, K., Sasaki, E., Hotakainen, R., Kassim, A. H., Pereira, C., Rolfs, A., et al. (2021). An X-linked syndrome with severe neurodevelopmental delay, hydrocephalus, and early lethality caused by a missense variation in the OTUD5 gene. Clin. Genet. 99, 303–308. doi: 10.1111/cge.13873
Tzschach, A., Grasshoff, U., Beck-Woedl, S., Dufke, C., Bauer, C., Kehrer, M., et al. (2015). Next-generation sequencing in X-linked intellectual disability. Eur. J. Hum. Genet. 23, 1513–1518. doi: 10.1038/ejhg.2015.5
Wiszniewska, J., Bi, W., Shaw, C., Stankiewicz, P., Kang, S. H., Pursley, A. N., et al. (2014). Combined array CGH plus SNP genome analyses in a single assay for optimized clinical testing. Eur. J. Hum. Genet. 22, 79–87. doi: 10.1038/ejhg.2013.77
Yang, Y., Muzny, D. M., Reid, J. G., Bainbridge, M. N., Willis, A., Ward, P. A., et al. (2013). Clinical whole-exome sequencing for the diagnosis of mendelian disorders. N. Engl. J. Med. 369, 1502–1511. doi: 10.1056/NEJMoa1306555
Keywords: OTUD5, X-linked intellectual disability, LINKED syndrome, deubiquitinase, congenital malformation
Citation: Saida K, Fukuda T, Scott DA, Sengoku T, Ogata K, Nicosia A, Hernandez-Garcia A, Lalani SR, Azamian MS, Streff H, Liu P, Dai H, Mizuguchi T, Miyatake S, Asahina M, Ogata T, Miyake N and Matsumoto N (2021) OTUD5 Variants Associated With X-Linked Intellectual Disability and Congenital Malformation. Front. Cell Dev. Biol. 9:631428. doi: 10.3389/fcell.2021.631428
Received: 23 November 2020; Accepted: 25 January 2021;
Published: 03 March 2021.
Edited by:
Antonius Plagge, University of Liverpool, United KingdomReviewed by:
Aida Bertoli-Avella, Centogene GmbH, GermanyRoger E. Stevenson, Greenwood Genetic Center, United States
Copyright © 2021 Saida, Fukuda, Scott, Sengoku, Ogata, Nicosia, Hernandez-Garcia, Lalani, Azamian, Streff, Liu, Dai, Mizuguchi, Miyatake, Asahina, Ogata, Miyake and Matsumoto. This is an open-access article distributed under the terms of the Creative Commons Attribution License (CC BY). The use, distribution or reproduction in other forums is permitted, provided the original author(s) and the copyright owner(s) are credited and that the original publication in this journal is cited, in accordance with accepted academic practice. No use, distribution or reproduction is permitted which does not comply with these terms.
*Correspondence: Naomichi Matsumoto, naomat@yokohama-cu.ac.jp; Ken Saida, t176030b@yokohama-cu.ac.jp
†These authors have contributed equally to this work