Expression of Concern: Mitochondrial tRNA mutation and regulation of the adiponectin pathway in maternally inherited hypertension in Chinese Han
- 1Medical School of Chinese People's Liberation Army (PLA), Beijing, China
- 2Department of Cardiology, The Sixth Medical Center, Chinese People's Liberation Army (PLA) General Hospital, Beijing, China
- 3Hainan LANBO Health Management Co. Ltd., Sanya, China
- 4Department of Gerontology, Union Hospital, Tongji Medical College, Huazhong University of Science and Technology, Wuhan, China
Some essential hypertension (EH) patients show maternal inheritance, which is the mode of mitochondrial DNA inheritance. This study examines the mechanisms by which mitochondrial mutations cause EH characterized by maternal inheritance. The study enrolled 115 volunteers, who were divided into maternally inherited EH (group A, n = 17), non-maternally inherited EH (group B, n = 65), and normal control (group C, n = 33) groups. A mitochondrial tRNA (15910 C>T) gene mutation was significantly correlated with EH and may play an important role in the pathogenesis of maternally inherited EH. Examining two families carrying the mitochondrial tRNA 15910 C>T mutation, which disrupted base pairing and may affect the stability and function of mitochondrial tRNAThr, we find that the overall incidence of EH was 59.3% in the maternal family members and 90% in males, significantly higher than in the general population in China (23.2%), and that the EH began at a younger age in those carrying mitochondrial tRNA 15910 C>T. To reveal the mechanism through which mitochondrial tRNA 15910 C>T causes maternally inherited EH, we cultured human peripheral blood mononuclear cells from family A2 in vitro. We find that cells carrying mitochondrial tRNA 15910 C>T were more viable and proliferative, and the increased ATP production resulted in raised intracellular reactive oxygen species (ROS). Moreover, the mitochondrial dysfunction resulted in reduced APN levels, causing hypoadiponectinemia, which promoted cell proliferation, and produced more ROS. This vicious cycle promoted the occurrence of EH with maternally inherited mitochondrial tRNA 15910 C>T. The mitochondrial tRNA 15910 C>T mutation may induce hypertension by changing the APN, AdipoR1, PGC-1α, and ERRα signaling pathways to elevate blood pressure. We discover a new mitochondrial mutation (tRNA 15910 C>T) related to EH, reveal part of the mechanism by which mitochondrial mutations lead to the occurrence and development of maternally inherited EH, and discuss the role of APN in it.
Introduction
Essential hypertension (EH) is the most common cardiovascular disease. The global prevalence of EH is increasing annually, and the latest national survey showed that the prevalence of hypertension in Chinese adults was 23.2% (Wang et al., 2018). EH is a complex disease caused by genetic susceptibility and environmental factors. Previous studies on EH genetics mainly focused on nuclear genes, which cannot fully explain the pathogenesis of EH (Levy et al., 2009; Padmanabhan et al., 2010). In the past decade, some patients with matrilineal EH have been found; matrilineal inheritance is unique to mitochondrial DNA (mtDNA) transmission. Wang et al. explored a large Chinese family, including 106 subjects, and found a significant correlation between mitochondrial tRNAIle 4263A>G mutation and EH (Wang et al., 2011). Zhou et al. report a mitochondrial tRNAMet 4435A>G mutation that they believe affected the structure of mitochondrial tRNA and mitochondrial function, thereby inducing maternally inherited hypertension (Zhou et al., 2018). Previously, we found that the frequency and density of mtDNA variation in Chinese Han with EH were higher than in the normotensive population, and some EH patients showed matrilineal inheritance (Li et al., 2009; Liu et al., 2009).
Adiponectin (APN) secreted by adipose tissue has obvious cardioprotective effects, and hypoadiponectinemia is a risk factor of various cardiovascular diseases. In mice overexpressing the APN gene, APN reduced blood pressure by improving endothelial dysfunction (Zhu et al., 2008). Ruan et al. observed perivascular inflammation and vascular injury regulated by complement-mediated inhibition of APN in patients with hypertension (Ruan et al., 2017). Increasingly, studies are reporting a significant correlation between APN and mitochondrial function. Mitochondrial dysfunction can directly reduce the secretion of APN and may indirectly reduce APN synthesis via the endoplasmic reticulum stress response although supplementation of exogenous APN can improve mitochondrial function (Wang et al., 2013). APN can also promote mitochondrial production via the AdipoR1, AMPK/SIRT1, and PGC-1α pathways. In other words, there are negative correlations between APN and the occurrence of EH and between APN and mitochondrial dysfunction; the latter plays an important role in the occurrence and development of maternally inherited EH. Therefore, we speculate that the APN pathway is a potential mechanism of maternally inherited EH.
Matrilineal EH is related to mtDNA mutations, but the mechanism remains unknown. To solve this problem, we study the relationship between mtDNA mutations and EH and reveal the role and possible mechanism of APN in maternally inherited EH.
Methods
Subject, Inclusion, and Exclusion Criteria
The subjects were Chinese Han seen by the Department of Cardiology of the Chinese People's Liberation Army (PLA) General Hospital from July 1, 2013, to December 31, 2013. The subjects were not related by blood. The criteria for judging EH and maternally inherited EH pedigrees were based on the criteria in our previous study (Liu et al., 2014). In the non-maternally inherited EH pedigrees, the subject was an EH patient, and no matrilineal members within three generations were clearly diagnosed with EH. The admission criteria for the control group were no EH in any family member within three generations, including the subject.
Patients were excluded if they had secondary hypertension, severe heart failure, cardiomyopathy, rheumatic heart disease, congenital heart disease, another diagnosed mitochondrial disease, malignant tumors, hematological disease, hepatitis, human immunodeficiency virus, syphilis, or other infectious disease; were taking contraceptives or were pregnant or preparing for pregnancy within 6 months; or were judged as unsuitable to participate in the study by a clinician.
The study was approved by the Ethics Committee of Chinese PLA General Hospital, and all subjects provided informed consent. The 115 members who met the study conditions were divided into the maternally inherited EH (group A, n = 17), non-maternally inherited EH (group B, n = 65), and control (group C, n = 33) groups. All subjects completed a questionnaire about themselves and their family members.
Whole Mitochondrial Gene Sequencing
From each subject, 400 μL whole blood was treated according to the SimGEN Blood DNA MiniKit instructions. DNA was extracted to measure optical density (OD) and was subjected to electrophoretic detection. Because the Cambridge Human Mitochondrial Gene has 16,569 bases, we divided the mitochondrial gene into 17 fragments and sequenced the entire mitochondrial gene. Then PCR amplification, purification, and extension were carried out, and the products were sequenced.
Extraction and Culture of Human PBMCs and Plotting the Cell Growth Curve
Human peripheral blood mononuclear cells (PBMCs) were extracted and cultured in 10% FBS (GibcoTM), 1% penicillin/streptomycin (10,000 U/mL, GibcoTM), and 89% RPMI 1640 medium (GibcoTM). The state of cell growth was observed and photographed every day. The cells were counted regularly, and a cell growth curve was plotted.
Determination of Plasma APN
Human plasma APN was quantified using an enzyme-linked immunosorbent assay (ELISA). The human ELISA kit was produced by Abcam. A standard sample or plasma, horseradish peroxidase-labeled antihuman APN monoclonal antibody, chromogenic agent, and terminator were added to solid-phase 96-well plates in turn. The degree of chromogenic reaction was positively correlated with the APN content of the plasma or standard sample. Using the absorbance corresponding to the concentration of a standard APN sample, a standard curve was drawn using the ELISA Calc regression/fitting calculation program provided by the reagent manufacturer and a four-parameter logistic curve fitting equation. The APN content in the sample was calculated from the absorbance.
ATP, Caspase3/7, and ROS of Cells
The ATP and Caspase3/7 produced by cells were detected following the instructions of the CellTiter-Glo® Luminescent Cell Viability and Caspase-Glo® 3/7 Assays (Promega). The cell viability and apoptosis curves of each group were generated using the total number of cells as the abscissa and ATPase and Caspase3/7 as the ordinates. The fluorescent probe DCFH-DA was diluted in serum-free RPMI1640 and added to the cell suspension at a final concentration of 1 nM. The fluorescence was measured via flow cytometry; the total fluorescence equaled the amount of ROS produced. A serum-free RPMI1640 cell suspension that contained the same number of cells with no fluorescent probe was used as a blank control, and a positive control with the same number of cells was added with 50 μg/mL ROSup 10 min after adding the probe.
RT-qPCR Analyses
Total RNA was extracted from myocardial tissues of mice using RNAprep Pure reagent and reverse transcribed to cDNA with the TIANScript cDNA First-Strand synthesis kit. Specific PCR primers for AdipoR1, AdipoR2, PGC-1α, and ERRα were synthesized by Tiangen Biotechnology. Then, q-PCR was conducted in 20 μL reaction volumes using a 7900HT Fast Real-Time PCR System (ABI, USA). The data were analyzed with Stratagene Mx3000 software. Agarose gel electrophoresis and the 2−ΔΔCT relative quantitative calculation formula were used to obtain the difference in mRNA transcription levels of the target gene.
Statistical Methods
Spss21.0, Origin8.5, and GraphPad Prism8 were used for analyses and processing. Results are expressed as the mean ± SD. The data with normal distributions were compared between two groups using t-tests and among multiple groups using ANOVA. Otherwise, the rank sum test was adopted. P < 0.05 was defined as statistically significant, and P < 0.01 indicated that the difference was highly significant.
Results
Comparison of Mitochondrial tRNA Mutation Rate and Blood Biochemistry Between Maternally and Non-maternally Inherited EH Patients
The detailed mitochondrial tRNA mutation is shown in Table 1. As the genes encoding mitochondrial tRNA contain 1504 bases, the total base number in each group is “n × 1,504.” Among them, the total mutation rates of the mitochondrial tRNA gene in groups A, B, and C were 0.28%, 0.12, and 0.05%, respectively (Figure 1). The mutation rate of the mitochondrial tRNA gene was significantly (P < 0.05) higher in groups A and B than in group C, and there were no significant differences between the two EH groups.
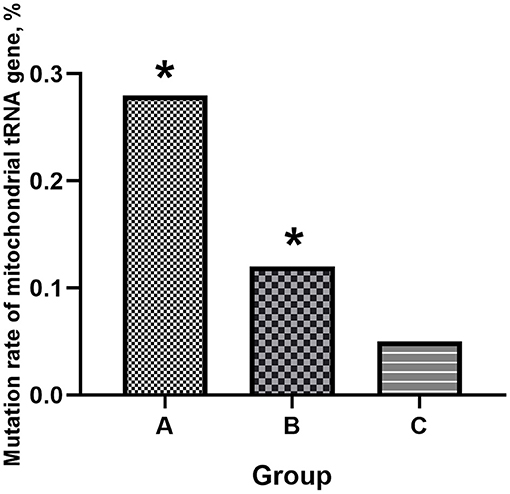
Figure 1. Mitochondrial tRNA gene mutation rates in the three groups. Compared to the controls, the two EH groups had higher mitochondrial tRNA mutation rates (*P < 0.05 vs. group C). Group A: maternally inherited EH, Group B: non-maternally inherited EH, Group C: control.
Mitochondrial tRNA 15910 C>T Mutation
The whole mitochondrial gene sequencing analyses of the 17 maternally inherited EH pedigrees (group A families) found that pedigrees A1 (Inner Mongolia, China) and A2 (Hebei, China) (Figure 2) had the same tRNA 15910 C>T mutation.
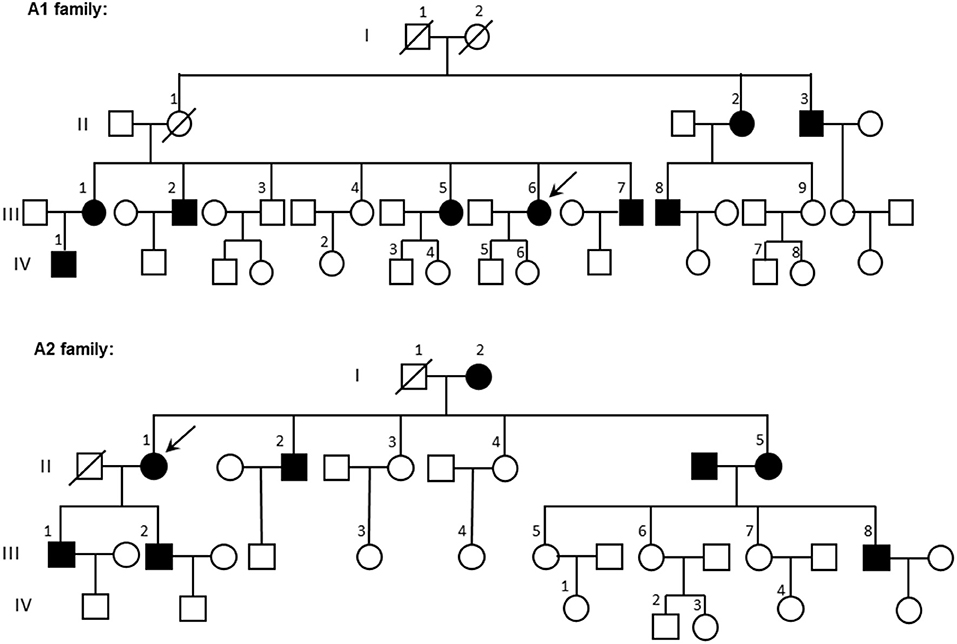
Figure 2. Family trees of pedigrees A1 and A2. Patients with hypertension are in black; the arrow indicates the proband. ◦, female; □, male.
Mitochondrial tRNA 15910 is located in the mitochondrial tRNAThr gene. The wild-type base at 15910 should be C but had mutated to T in the maternal members of pedigrees A1 and A2 (Figure 3A). Site 15910, which is a base in the D-loop arm of the mitochondrial tRNAThr gene (Figure 3B), forms the first base pair (C–G) in the D-loop with the G at site 15897. After mutation from C to T, the base pair cannot form, which may affect the function and stability of mitochondrial tRNAThr.
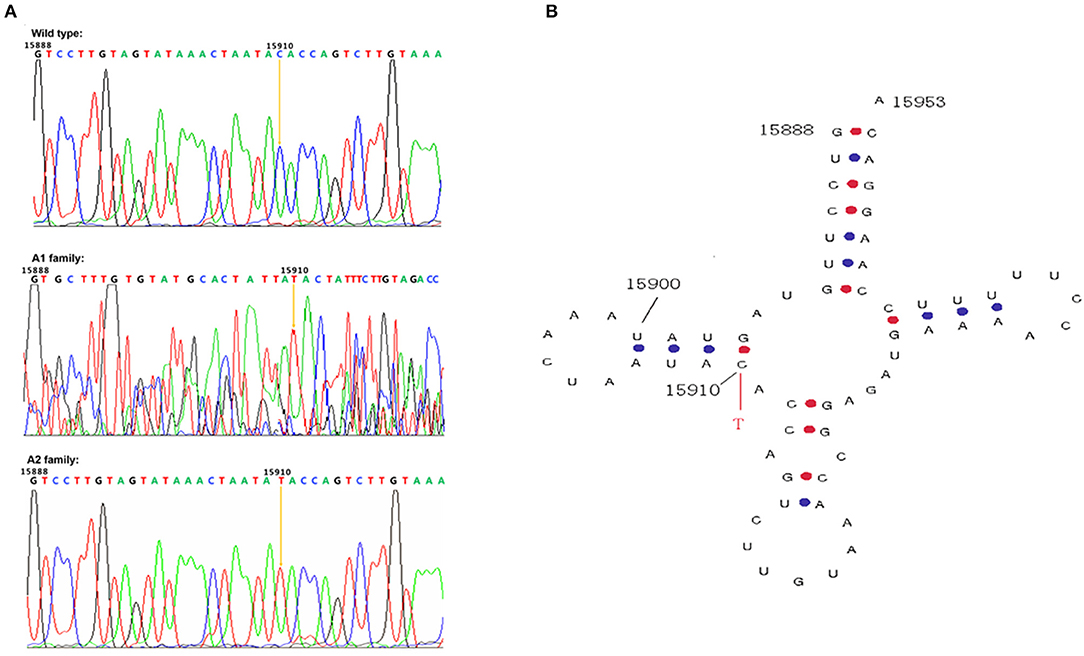
Figure 3. Mitochondrial gene sequencing and secondary structure of tRNAThr 15910. (A) The mitochondrial tRNAThr C>T mutation in families A1 and A2; (B) The first base pair in the D-loop formed by the C at 15910 and G at 15897 is disrupted by the tRNAThr C>T mutation.
Characteristics of Pedigrees Carrying tRNA 15910 C>T
The occurrence of EH in pedigrees A1 and A2 was obviously matrilineal. Table 2 shows the incidence of EH in adult matrilineal members of families A1 and A2 with a total incidence of 59.3% and an incidence in males as high as 90.0%.
There were 16 adult matrilineal members with EH in two families. Based on sex and age, 40 eligible people were selected from the non-maternally inherited EH group (group B) and compared with the two pedigrees with tRNA 15910 C>T mutations (Table 3). The onset age of EH was significantly (P < 0.01) younger in matrilineal members of the two families carrying tRNA 15910 C>T (43.8 ± 8.6 vs. 52.3 ± 7.7 years for non-maternally inherited EH patients).
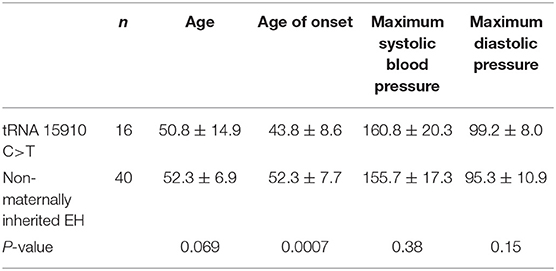
Table 3. Onset age of EH and blood pressure of tRNA 15910 C>T patients with EH and patients with non-maternally inherited EH.
APN Level and APN-Related Molecules in Families Carrying tRNA 15910 C>T
To compare plasma APN levels, 24 subjects were divided into four groups of six each according to their family background and whether they had EH: (1) EH+Mu group (matrilineal members of family A2 carrying tRNA 15910 C>T and suffering from EH); (2) Mu group (matrilineal members of family A2 with tRNA 15910 C>T, but without EH); (3) EH group (from group B); (4) Control group (from group C). The ages of the Mu and control groups matched that of the other two groups.
The average plasma APN level was measured in each group (Figure 4A) and was significantly lower in the EH+Mu group than in the other groups. The APN level in the Mu group was also significantly lower than that in the control. There were no significant differences between the Mu and EH groups.
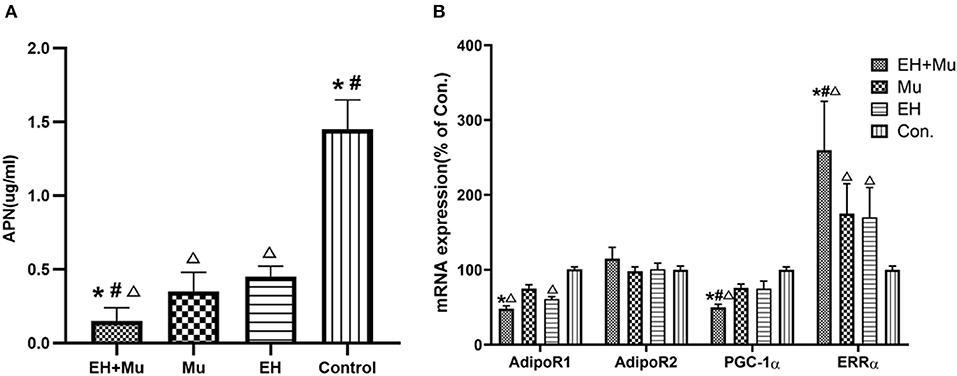
Figure 4. Plasma APN and expression of APN-related molecules. (A) The plasma APN levels of patients with or without tRNA 15910 C>T and/or EH (*P < 0.05 vs. Mu group; #P < 0.05 vs. EH group; ΔP < 0.05 vs. control group); (B) The mRNA levels of APN-related molecules in each group. (*P < 0.05 vs. Mu group; #P < 0.05 vs. EH group; ΔP < 0.05 vs. control group).
PBMCs were extracted and cultured to analyze the AdipoR1/2, PGC-1α, and ERRα mRNA and protein expression in each group (Figure 4B). The expression of AdipoR1 mRNA was 52.5% lower in the EH+Mu group than in the controls and was 36.0% lower than that in the Mu group. The PGC-1α gene expression in each group was consistent with the change in direction of AdipoR1. There were no significant differences in AdipoR2 mRNA among groups. The expression of the ERRα gene in the EH+Mu group was significantly higher than in the other three groups. The expression of the ERRα gene in the Mu and EH groups was also significantly higher than in the controls.
PBMC Growth and Apoptosis in tRNA 15910 C>T Carriers
The PBMCs of the EH+Mu and Mu groups proliferated in vitro (Figure 5A). The PBMC growth curve (Figure 5B) indicated that the maximum cell proliferation in the EH+Mu and Mu groups was about four times that in the EH and control groups. The PBMC viability curve of each group showed that PBMC activity was strongest in the Mu group with a significant difference in ATP production compared to the other three groups. Cell viability was significantly higher in the EH+Mu group than in the EH and control groups (Figure 5C). However, there were no significant differences in the Caspase3/7 apoptosis curve of PBMCs among the different groups (Figure 5D).
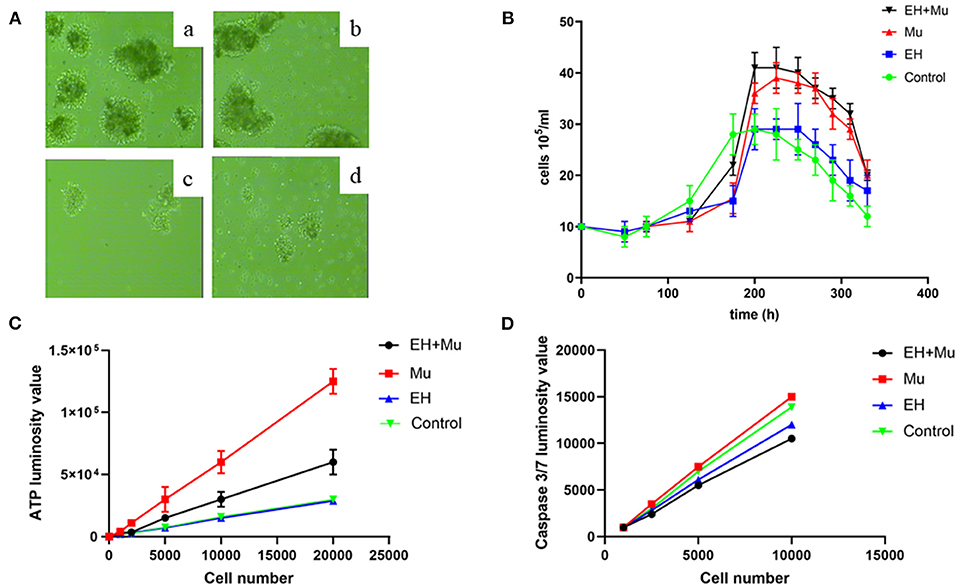
Figure 5. PBMCs growth and apoptosis in each group. (A) PBMC growth observed at 100× magnification on day 7; (a) EH+Mu; (b) Mu; (c) EH; (d) Control; (B) The PBMC growth curves in each group; (C) The generation of ATP in each group; (D) Caspase 3/7 apoptosis in each group.
Changes in the ROS of PBMCs Carrying tRNA 15910 C>T
Comparing groups, the EH+Mu group produced significantly more ROS than the other three groups (Figure 6). The Mu group produced significantly more ROS than the EH, positive control (ROSup 50 μg/mL), and control groups. ROS generation in the EH group was also significantly higher than that in the control and positive control groups.
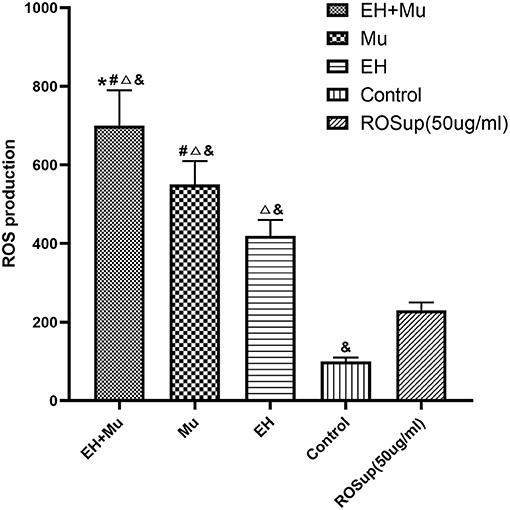
Figure 6. Comparison of ROS produced by cells in each group. The elevated ROS production in groups carrying tRNA 15910 C>T mutation (*P < 0.05 vs. Mu group; #P < 0.05 vs. EH group; ΔP < 0.05 vs. control group; &P < 0.05 vs. ROSup [50 μg/mL]).
Discussion
Two pedigrees with maternally inherited EH carried the same mitochondrial tRNA 15910 C>T mutants. Analyses of all matrilineal members of these two families show that the incidence of hypertension is as high as 59.3% although the latest survey reports that the prevalence of hypertension in Chinese adults is 23.2% and that of adult males is 24.5% (Wang et al., 2018). The incidence of hypertension in all adult matrilineal members of the two families is significantly (P < 0.05) higher than that in the population, suggesting that the mitochondrial tRNA 15910 C>T mutation is closely related to the occurrence of maternally inherited hypertension. Moreover, the onset age in the matrilineal members is younger than that of non-maternally inherited EH, and it decreased by generation, which might be related to the heterogeneity and homogeneity of mtDNA and the genetic mode of maternally inherited EH. In theory, the effects of matrilineal inheritance on EH should increase over the generations because EH can, in turn, induce mitochondrial mutations, which are transmitted to maternal offspring via maternal inheritance. Due to the genetic process of mtDNA, a heterogeneous mtDNA mutant may drift into a simple mtDNA mutant after one or two generations of matrilineal inheritance, and the mutation threshold increases, which makes mitochondrial functional damage more likely. Our results concur with the literature on EH (Zhu et al., 2016). Our analyses of mitochondrial tRNA 15910 C>T are horizontal analyses of biological evolution. Then, we analyzed the biological conservatism of this locus longitudinally. In the mitochondrial gene sequences of other mammals, the base of the corresponding human mitochondrial tRNA 15910 locus is C (Calvo et al., 2016), indicating that this locus is highly conserved, and the base at this locus plays an important role in maintaining the function and stability of mitochondrial tRNAThr. The matrilineal members of two different families carrying mtDNAC15910T had a very high prevalence of EH, which shows maternal inheritance, suggesting that mitochondrial tRNA 15910 C>T is closely related to the occurrence of EH.
Furthermore, the ROS of matrilineal members carrying mitochondrial tRNA 15910 C>T was markedly increased, which was more obvious in cells from EH patients. Their AdipoR1 expression was significantly downregulated, and the plasma APN level was also significantly decreased, suggesting that the increase in ROS was negatively correlated with the expression of AdipoR1 and plasma APN. In addition, the APN level was positively correlated with the expression of peroxisome proliferator-activated receptor γ coactivator-1α (PGC-1α) and negatively correlated with the level of estrogen-related receptor alpha (ERRα).
People with other diseases caused by mitochondrial mutations had low APN levels (Ninomiya et al., 2016), and APN protected against incident hypertension independent of the body fat distribution (Peri-Okonny et al., 2017). Moreover, artificially induced mitochondrial dysfunction and excessive ROS production reduced APN secretion in adipose tissue (Wang et al., 2013). In APN-deficient mice, endothelium-dependent vasodilation was impaired, endothelial nitric oxide (eNOS) activity decreased, NO decreased, and systolic blood pressure was higher, which could be reversed by APN intervention (Zhu et al., 2008). APN has a cardioprotective effect, which is mainly mediated by adiponectin receptors (AdipoR1 and AdipoR2), and angiotensin II (Ang II) inhibits AdipoR1 expression in cardiomyocytes in vivo and in vitro, and the combination of Ang II (AT1 and AT2) and adiponectin receptors instigates the inhibition of the cytoprotective action of adiponectin receptors (Zha et al., 2017). Reducing the production of ROS can reverse the effects of Ang II on the expression of AdipoR1, indicating that ROS play a pivotal role in regulating Ang II in AdipoR1 expression. When mitochondrial function is insufficient, ROS production increases, which impairs APN secretion by adipocytes and downregulates AdipoR1 expression.
As a transcriptional activator of the nuclear receptor family, PGC-1α is the transcriptional activator of PPAR-γ, both of which regulate the expression of genes related to energy metabolism (Di et al., 2018). PGC-1α, which mainly regulates mitochondrial production and the homeostasis of mitochondrial copy number, is the main regulator of mitochondrial production downstream in the AMPK pathway (Dong et al., 2020). It regulates the dynamic balance between mitochondrial function and the energy produced, activates the antioxidant enzyme system, and scavenges ROS (Suntar et al., 2020). When PGC-1α is deleted, the activities of mitochondrial antioxidant enzymes decrease significantly, and ROS production and oxidative stress are both enhanced (Lu et al., 2010). APN and its receptor AdipoR1 can increase the expression of PGC-1α, enhance its activity, and enhance mitochondrial biosynthesis, which is consistent with our results (Pal et al., 2020).
ERRα, an orphan nuclear receptor, is an important regulator of blood pressure, mainly by affecting the balance of sodium and potassium in the kidneys (Wang et al., 2016). Loss of ERRα expression, whether stimulated by high sodium, low potassium, or high renin, leads to hypotension instead of hypertension. Most PGC-1α effector genes have ERRα binding sites, and PGC-1α not only cooperates with ERRα to activate the target gene transcription, but also regulates the ERRα activity (Brown et al., 2018). Therefore, tissues with high ERRα expression generally also overexpress ERRα. However, our results suggest that the expression of PGC-1α differs from that of ERRα. Comparing the relationship between the expression of PGC-1α and ERRα in the four groups, this phenomenon is seen in every group, and the proportions are similar, which should exclude the deviation caused by test error. Previous studies report that ERRα could regulate cardiovascular function without relying on PGC-1α. When congestive heart failure occurred, ERRα significantly decreased, and AMPKa2 activation increased the ERRα expression to improve cardiomyocyte mitochondrial function and heart failure, but the expression of PGC-1α did not increase (Hu et al., 2011). Histone deacetylation and PGC-1α expression downregulation also occurred in cardiomyocytes during the initial stage of hypoxia (within 12 h), and ERRα overexpression increased the PGC-1α expression (Ramjiawan et al., 2013). This indicates that ERRα can also regulate the expression of PGC-1α, and there may be a feedback mechanism between them. Hypoadiponectinemia downregulated PGC-1α expression and induced an increase in ERRα expression, which might be related to their mutual regulation.
In summary, we speculate that mitochondrial tRNA 15910 C>T impairs mitochondrial function, increases ROS production, downregulates the expression of APN and its receptor AdipoR1, and then suppresses the expression of PGC-1α and elevated ERRα via the mutual regulation between ERRα and PGC-1α, consequently resulting in further mitochondrial dysfunction and high blood pressure.
Cell proliferation is the main form of cell activity. At present, there are two methods to detect cell proliferation. One is a direct method to evaluate the proliferation ability of cells by directly measuring the number of cells undergoing division, such as the BrdU test. The other is an indirect method, namely the cell viability test, which evaluates cell proliferation by detecting the number of healthy cells in the sample, such as MTT and ATP detection. ATP is the direct energy source of cells, so the proliferation state of cells can be directly reflected by detecting the content of ATP in cells (Adan et al., 2016). The mitochondrial tRNA 15910 C>T mutation resulted in a significant increase in the cell proliferation rate, cell activity, ATP production, and ROS production as a byproduct in cultured PBMCs from each group, particularly in those with the mitochondrial mutation combined with EH, consistent with our previous research (Liu et al., 2017). However, there were no significant differences in the Caspase3/7 apoptosis curve among groups, demonstrating that mitochondrial tRNA 15910 C>T mainly affects proliferation and not apoptosis.
The analyses of the mtDNA mutation and EH show that the mutation rates of the mitochondrial tRNA gene in the control, non-maternally inherited EH, and maternally inherited EH groups were 0.048, 0.12, and 0.28%, respectively. Compared to the controls, there were significantly more mitochondrial tRNA gene mutations in the EH groups (P < 0.05), which concurs with the literature (Zhu et al., 2018), suggesting that there is a close correlation between the occurrence of EH and the mitochondrial tRNA gene mutation. The mechanism might be related to the increase in Ang II in hypertensive people. Studies show that Ang II can increase intracellular ROS, and the interaction between them affects mtDNA damage (Wilcox et al., 2019; Zhang et al., 2019). However, a difference in the mitochondrial tRNA mutation rate between the maternally inherited and non-maternally inherited EH populations could not be elucidated, which might be due to the small sample size, particularly subjects with maternally inherited hypertension. The effect of matrilineal inheritance on EH was 20.73%, lower than that in the Framingham Heart study (35.2%) (Yang et al., 2007). Our study definitely had a small sample, and the Framingham study enrolled 1,593 families.
As mitochondria play an important role in the pathogenesis of hypertension, the treatments targeting mitochondria to decrease blood pressure have been research hot spots. Coenzyme Q10 can prevent hypertension through antioxidant properties, preserving NO, and boosting the production of the prostaglandin prostacyclin (Yang et al., 2015). Eisenberg et al. discovered that oral spermidine facilitated cardioprotective effects in elderly mice through strengthening cardiac autophagy and mitophagy. In salt-sensitive rats, spermidine treatment also delayed the progression of hypertensive heart disease in company with decreased arterial blood pressure (Eisenberg et al., 2017).
There are some limitations to this study. First, the sample size is small. A sufficient sample size helps to ensure the accuracy of the research results, and we analyzed only 115 subjects and families. Second, this is a retrospective study, and it is necessary to alter the key genes in animal and cell experiments to verify their effects.
The maternal inheritance of mtDNA can help us predict the risk for diseases related to mtDNA mutations in offspring and lead to prevention, early diagnosis, and timely treatment. Although there is no radical cure for EH, early initiation of treatment and blood pressure control can reduce the complications of hypertension. We find a new mitochondrial tRNA mutation 15910 C>T related to maternally inherited EH, reveal part of the mechanism by which it led to the occurrence and development of maternally inherited EH, and discuss the role of APN in it and its possible mechanism. This provides a new theoretical and experimental basis for the study of maternally inherited EH and probably a new target for the prevention and treatment of it.
Data Availability Statement
All datasets generated for this study are included in the article/supplementary material.
Ethics Statement
The studies involving human participants were reviewed and approved by the Ethics Committee of Chinese PLA General Hospital, Chinese People's Liberation Army General Hospital. The patients/participants provided their written informed consent to participate in this study.
Author Contributions
JB and QM completed the experiments. YLa, YC, SM, JL, CL, ZF, XL, YH, and YLi contributed to the conception, drafted the manuscript, critically revised the manuscript, gave final approval, and agreed to be accountable for all aspects of work ensuing integrity and accuracy. All authors contributed to the article and approved the submitted version.
Funding
This study was supported by grants from the National Natural Science Foundation of China (Nos. 81870249, 82070328, and 82070434).
Conflict of Interest
YLa was employed by the company Hainan LANBO Health Management Co. Ltd.
The remaining authors declare that the research was conducted in the absence of any commercial or financial relationships that could be construed as a potential conflict of interest.
References
Adan, A., Kiraz, Y., and Baran, Y. (2016). Cell proliferation and cytotoxicity assays. Curr. Pharm. Biotechnol. 17, 1213–1221. doi: 10.2174/1389201017666160808160513
Brown, E. L., Foletta, V. C., Wright, C. R., Sepulveda, P. V., Konstantopoulos, N., Sanigorski, A., et al. (2018). PGC-1α and PGC-1β increase protein synthesis via ERRα in C2C12 myotubes. Front. Physiol. 9:1336. doi: 10.3389/fphys.2018.01336
Calvo, S. E., Clauser, K. R., and Mootha, V. K. (2016). MitoCarta2.0: an updated inventory of mammalian mitochondrial proteins. Nucleic Acids Res. 44, D1251–D1257. doi: 10.1093/nar/gkv1003
Di, W., Lv, J., Jiang, S., Lu, C., Yang, Z., Ma, Z., et al. (2018). PGC-1: the energetic regulator in cardiac metabolism. Curr. Issues Mol. Biol. 28, 29–46. doi: 10.21775/cimb.028.029
Dong, Y. Z., Li, L., Espe, M., Lu, K. L., and Rahimnejad, S. (2020). Hydroxytyrosol Attenuates hepatic fat accumulation via activating mitochondrial biogenesis and autophagy through the AMPK pathway. J. Agric. Food Chem. 68, 9377–9386. doi: 10.1021/acs.jafc.0c03310
Eisenberg, T., Abdellatif, M., Zimmermann, A., Schroeder, S., Pendl, T., Harger, A., et al. (2017). Dietary spermidine for lowering high blood pressure. Autophagy 13, 767–769. doi: 10.1080/15548627.2017.1280225
Hu, X., Xu, X., Lu, Z., Zhang, P., Fassett, J., Zhang, Y., et al. (2011). AMP activated protein kinase-α2 regulates expression of estrogen-related receptor-α, a metabolic transcription factor related to heart failure development. Hypertension 58, 696–703. doi: 10.1161/HYPERTENSIONAHA.111.174128
Levy, D., Ehret, G. B., Rice, K., Verwoert, G. C., Launer, L. J., Dehghan, A., et al. (2009). Genome-wide association study of blood pressure and hypertension. Nat. Genet. 41, 677–687. doi: 10.1038/ng.384
Li, R., Liu, Y., Li, Z., Yang, L., Wang, S., and Guan, M. X. (2009). Failures in mitochondrial tRNAMet and tRNAGln metabolism caused by the novel 4401A>G mutation are involved in essential hypertension in a Han Chinese Family. Hypertension 54, 329–337. doi: 10.1161/HYPERTENSIONAHA.109.129270
Liu, Y., Li, R., Li, Z., Wang, X. J., Yang, L., Wang, S., et al. (2009). Mitochondrial transfer RNAMet 4435A>G mutation is associated with maternally inherited hypertension in a Chinese pedigree. Hypertension 53, 1083–1090. doi: 10.1161/HYPERTENSIONAHA.109.128702
Liu, Y., Li, Y., Zhu, C., Tian, L., Guan, M., and Chen, Y. (2017). Mitochondrial biogenesis dysfunction and metabolic dysfunction from a novel mitochondrial tRNA(Met) 4467 C>A mutation in a Han Chinese family with maternally inherited hypertension. Sci. Rep. 7:3034. doi: 10.1038/s41598-017-03303-w
Liu, Y., Zhu, Q., Zhu, C., Wang, X., Yang, J., Yin, T., et al. (2014). Systematic analysis of the clinical and biochemical characteristics of maternally inherited hypertension in Chinese Han families associated with mitochondrial. BMC Med. Genomics 7, 73. doi: 10.1186/s12920-014-0073-x
Lu, Z., Xu, X., Hu, X., Fassett, J., Zhu, G., Tao, Y., et al. (2010). PGC-1 alpha regulates expression of myocardial mitochondrial antioxidants and myocardial oxidative stress after chronic systolic overload. Antioxid. Redox Signal. 13, 1011–1022. doi: 10.1089/ars.2009.2940
Ninomiya, H., Hirata, A., Kozawa, J., Nakata, S., Kimura, T., Kitamura, T., et al. (2016). Treatment of mitochondrial diabetes with a Peroxisome Proliferator-activated Receptor (PPAR)-gamma agonist. Intern. Med. 55, 1143–1147. doi: 10.2169/internalmedicine.55.4418
Padmanabhan, S., Melander, O., Johnson, T., Di Blasio, A. M., Lee, W. K., Gentilini, D., et al. (2010). Genome-wide association study of blood pressure extremes identifies variant near UMOD associated with hypertension. PLoS Genet. 6:e1001177. doi: 10.1371/journal.pgen.1001177
Pal, S., Porwal, K., Rajak, S., Sinha, R. A., and Chattopadhyay, N. (2020). Selective dietary polyphenols induce differentiation of human osteoblasts by adiponectin receptor 1-mediated reprogramming of mitochondrial energy metabolism. Biomed. Pharmacother. 127:110207. doi: 10.1016/j.biopha.2020.110207
Peri-Okonny, P. A., Ayers, C., Maalouf, N., Das, S. R., de Lemos, J. A., Berry, J. D., et al. (2017). Adiponectin protects against incident hypertension independent of body fat distribution: observations from the Dallas Heart Study. Diabetes Metab. Res. Rev. 33:10.1002/dmrr.2840. doi: 10.1002/dmrr.2840
Ramjiawan, A., Bagchi, R. A., Blant, A., Albak, L., Cavasin, M. A., Horn, T. R., et al. (2013). Roles of histone deacetylation and AMP kinase in regulation of cardiomyocyte PGC-1α gene expression in hypoxia. Am. J. Physiol. Cell Physiol. 304, C1064–C1072. doi: 10.1152/ajpcell.00262.2012
Ruan, C. C., Ma, Y., Ge, Q., Li, Y., Zhu, L. M., Zhang, Y., et al. (2017). Complement-mediated inhibition of adiponectin regulates perivascular inflammation and vascular injury in hypertension. FASEB J. 31, 1120–1129. doi: 10.1096/fj.201600780R
Suntar, I., Sureda, A., Belwal, T., Sanches Silva, A., Vacca, R. A., Tewari, D., et al. (2020). Natural products, PGC-1 α, and Duchenne muscular dystrophy. Acta Pharm. Sin. B 10, 734–745. doi: 10.1016/j.apsb.2020.01.001
Wang, C. H., Wang, C. C., Huang, H. C., and Wei, Y. H. (2013). Mitochondrial dysfunction leads to impairment of insulin sensitivity and adiponectin secretion in adipocytes. FEBS J. 280, 1039–1050. doi: 10.1111/febs.12096
Wang, D., Wang, Y., Liu, F. Q., Yuan, Z. Y., and Mu, J. J. (2016). High salt diet affects renal sodium excretion and ERRα expression. Int. J. Mol. Sci. 17:480. doi: 10.3390/ijms17040480
Wang, S., Li, R., Fettermann, A., Li, Z., Qian, Y., Liu, Y., et al. (2011). Maternally inherited essential hypertension is associated with the novel 4263A>G mutation in the mitochondrial tRNAIle gene in a large Han Chinese family. Circ. Res. 108, 862–870. doi: 10.1161/CIRCRESAHA.110.231811
Wang, Z., Chen, Z., Zhang, L., Wang, X., Hao, G., Zhang, Z., et al. (2018). Status of hypertension in china: results from the china hypertension survey, 2012-2015. Circulation 137, 2344–2356. doi: 10.1161/CIRCULATIONAHA.117.032380
Wilcox, C. S., Wang, C., and Wang, D. (2019). Endothelin-1-induced microvascular ROS and contractility in angiotensin-II-infused mice depend on COX and TP receptors. Antioxidants 8:193. doi: 10.3390/antiox8060193
Yang, Q., Kim, S. K., Sun, F., Cui, J., Larson, M. G., Vasan, R. S., et al. (2007). Maternal influence on blood pressure suggests involvement of mitochondrial DNA in the pathogenesis of hypertension: the Framingham Heart Study. J. Hypertens. 25, 2067–2073. doi: 10.1097/HJH.0b013e328285a36e
Yang, Y. K., Wang, L. P., Chen, L., Yao, X. P., Yang, K. Q., Gao, L. G., et al. (2015). Coenzyme Q10 treatment of cardiovascular disorders of ageing including heart failure, hypertension and endothelial dysfunction. Clin. Chim. Acta 450, 83–89. doi: 10.1016/j.cca.2015.08.002
Zha, D., Cheng, H., Li, W., Wu, Y., Li, X., Zhang, L., et al. (2017). High glucose instigates tubulointerstitial injury by stimulating hetero-dimerization of adiponectin and angiotensin II receptors. Biochem. Biophys. Res. Commun. 493, 840–846. doi: 10.1016/j.bbrc.2017.08.047
Zhang, F., Dong, Z., Gao, S., Chen, G., and Liu, D. (2019). AT1R-mediated apoptosis of bone marrow mesenchymal stem cells is associated with mtROS production and mtDNA reduction. Oxid. Med. Cell. Longev. 2019:4608165. doi: 10.1155/2019/4608165
Zhou, M., Xue, L., Chen, Y., Li, H., He, Q., Wang, B., et al. (2018). A hypertension-associated mitochondrial DNA mutation introduces an mG37 modification into tRNA(Met), altering its structure and function. J. Biol. Chem. 293, 1425–1438. doi: 10.1074/jbc.RA117.000317
Zhu, W., Cheng, K. K., Vanhoutte, P. M., Lam, K. S., and Xu, A. (2008). Vascular effects of adiponectin: molecular mechanisms and potential therapeutic intervention. Clin. Sci. 114, 361–374. doi: 10.1042/CS20070347
Zhu, Y., Gu, X., and Xu, C. (2016). A mitochondrial DNA A8701G mutation associated with maternally inherited hypertension and dilated cardiomyopathy in a Chinese pedigree of a consanguineous marriage. Chin. Med. J. 129, 259–266. doi: 10.4103/0366-6999.174491
Keywords: mitochondrial tRNA, mutation, maternally inherited hypertension, adiponectin, Chinese Han
Citation: Bai J, Ma Q, Lan Y, Chen Y, Ma S, Li J, Liu C, Fu Z, Lu X, Huang Y and Li Y (2021) Mitochondrial tRNA Mutation and Regulation of the Adiponectin Pathway in Maternally Inherited Hypertension in Chinese Han. Front. Cell Dev. Biol. 8:623450. doi: 10.3389/fcell.2020.623450
Received: 30 October 2020; Accepted: 01 December 2020;
Published: 22 January 2021.
Edited and reviewed by: Hao Zhou, People's Liberation Army General Hospital, China
Copyright © 2021 Bai, Ma, Lan, Chen, Ma, Li, Liu, Fu, Lu, Huang and Li. This is an open-access article distributed under the terms of the Creative Commons Attribution License (CC BY). The use, distribution or reproduction in other forums is permitted, provided the original author(s) and the copyright owner(s) are credited and that the original publication in this journal is cited, in accordance with accepted academic practice. No use, distribution or reproduction is permitted which does not comply with these terms.
*Correspondence: Yang Li, bGl5YW5nYnNoJiN4MDAwNDA7MTYzLmNvbQ==; Yun Huang, aHVhbmd5dW4xMjA3JiN4MDAwNDA7MTYzLmNvbQ==
†These authors have contributed equally to this work and share first authorship