- 1Research Unit of Non-Coding RNAs and Genome Editing, Division of Clinical Oncology, Department of Internal Medicine, Comprehensive Cancer Center Graz, Medical University of Graz, Graz, Austria
- 2Department of Translational Molecular Pathology, The University of Texas MD Anderson Cancer Center, Houston, TX, United States
- 3Research Centre for Functional Genomics and Translational Medicine, Iuliu Hatieganu University of Medicine and Pharmacy, Cluj-Napoca, Romania
- 4Department of Tumor Biology, Center of Experimental Medicine, University Medical Center Hamburg-Eppendorf, Hamburg, Germany
- 5Central European Institute of Technology, Masaryk University, Brno, Czechia
- 6Department of Comprehensive Cancer Care, Masaryk Memorial Cancer Institute, Brno, Czechia
- 7Department of Experimental Therapeutics, The University of Texas MD Anderson Cancer Center, Houston, TX, United States
Liquid biopsy—the determination of circulating cells, proteins, DNA or RNA from biofluids through a “less invasive” approach—has emerged as a novel approach in all cancer entities. Circulating non-(protein) coding RNAs including microRNAs (miRNAs), long non-coding RNAs (lncRNAs), and YRNAs can be passively released by tissue or cell damage or actively secreted as cell-free circulating RNAs, bound to lipoproteins or carried by exosomes. In renal cell carcinoma (RCC), a growing body of evidence suggests circulating non-coding RNAs (ncRNAs) such as miRNAs, lncRNAs, and YRNAs as promising and easily accessible blood-based biomarkers for the early diagnosis of RCC as well as for the prediction of prognosis and treatment response. In addition, circulating ncRNAs could also play a role in RCC pathogenesis and progression. This review gives an overview over the current study landscape of circulating ncRNAs and their involvement in RCC pathogenesis as well as their potential utility as future biomarkers in RCC diagnosis and treatment.
Introduction
Renal cell carcinoma (RCC) makes up for more than 90% of malignant kidney tumors, which measures up to 338,000 newly diagnosed patients each year worldwide and makes RCC the second most common urogenital malignancy in men and the third most frequent in women (Ferlay et al., 2015; Znaor et al., 2015; Siegel et al., 2019). The most frequent histological subtype is clear cell renal cell carcinoma (ccRCC) accounting for 70–80%, followed by papillary and chromophobe RCC (Rini et al., 2009). Roughly 20–30% of patients are diagnosed with an initially metastatic disease (Janzen et al., 2003), which only allows palliative treatment strategies (Powles et al., 2017). Although patient outcomes in these treatment settings have significantly improved since the introduction of immune checkpoint inhibitor therapy and combinations with antiangiogenetic drugs, most patients eventually experience a progressive disease (Motzer et al., 2015, 2018; Cella et al., 2019; Rini et al., 2019).
To date, there are no screening programs or reliable and economically reasonable biomarkers available for the early diagnosis of RCC that would drastically improve the disease outcomes (Rossi et al., 2018). Moreover, although many easily available blood-based biomarkers for the prognosis and the prediction of treatment outcome have been assessed in different stages of treatment and disease, they have not entered clinical routine yet (Dalpiaz et al., 2017; Farber et al., 2017; Seles et al., 2017). In the recent years, liquid biopsies such as circulating tumor cells (CTCs) or circulating ribonucleic acids, obtained from, for instance, blood or urine samples, are emerging (Ellinger et al., 2016; Li G. et al., 2017; Bergerot et al., 2018; Cimadamore et al., 2019). These liquid biopsies serve as novel and promising tools for the diagnosis, prognosis prediction, and selection of appropriate treatment options in RCC patients (Cimadamore et al., 2019; Smith et al., 2020).
Circulating non-coding RNAs (ncRNAs), including microRNAs (miRNAs) and long non-coding RNAs (lncRNAs), have been repeatedly suggested as well-accessible blood-based biomarkers in numerous cancer entities, including urogenital malignancies such as prostate (Su et al., 2015), bladder (Nandagopal and Sonpavde, 2016), testicular (Dieckmann et al., 2019), and kidney cancer (Ellinger et al., 2016). miRNAs are a class of non-protein coding RNAs that are about 20 nucleotides in length (Schwarzenbacher et al., 2019). They bind to the mRNA of their target proteins at the 3′UTR, which results in limited translation and increased degradation of the mRNA (Bartel, 2004). Thus, miRNAs are regulators of many cell functions including cell growth and proliferation and therefore play a principal role in tumorigenesis and progression of RCC and thus may represent useful biomarkers (Schanza et al., 2017; Barth et al., 2019). On the other hand, lncRNAs are over 200 nucleotides in length and regulate cellular processes by different mechanisms (Novikova et al., 2013). They sponge miRNAs, thereby preventing them from binding to their respective targets, which leads to the preserved expression of these targets. Furthermore, they can act as scaffold and decoy for miRNAs or proteins or can act as guides for proteins to influence gene transcription. lncRNAs were demonstrated to regulate cancer development and progression as either oncogenic or tumor-suppressive factors (Chi et al., 2019), and thus therapeutic concepts utilizing the key role of lncRNAs in cancer have emerged over the last decade (Pan et al., 2018b; Pichler et al., 2020).
In RCC, the utility of circulating ncRNAs as biomarkers has been previously evaluated (Ellinger et al., 2016); nevertheless, in the recent years, further evidence has been added to this emerging field. The present review aims to give an update and overview on the progress of circulating ncRNAs as biomarkers across various treatment settings in RCC and highlights pathogenetic implications (see Tables 1, 2).
Challenges of Circulating ncRNAs as Biomarkers in Cancer
As of yet, the development and the use of circulating RNA-based biomarkers comes with certain challenges (Zaporozhchenko et al., 2018). In contrast to cell-free DNA, which is mainly set free in the course of cell death, the largest proportion of circulating RNAs is actively secreted by normal and tumor cells and therefore could reflect current cellular processes and states to disease better, although they can be released during apoptosis and other types of cell death as well (Javidi et al., 2014; Zaporozhchenko et al., 2018). In its nature, cell-free RNA molecules are rather unstable in biofluids such as blood and urine, among others, due to the underlying environmental conditions, including pH and the enzymatic activity of ribonucleases (Tzimagiorgis et al., 2011; Bryzgunova and Laktionov, 2015; Koczera et al., 2016; Zaporozhchenko et al., 2018); nevertheless, miRNAs are astonishingly stable under these extreme conditions (Cortez et al., 2011). Interestingly, in cancer, the activity of ribonucleases in the blood may be decreased, granting a higher stability of circulating RNA products (Huang et al., 2014). Circulating RNAs are often encapsulated in microvesicles, such as exosomes or apoptotic bodies, or can be bound to lipoproteins, such as Ago2 or high-density lipoprotein, in order to protect them from degeneration (Figure 1) (Arroyo et al., 2011; Gallo et al., 2012; Wagner et al., 2013; Javidi et al., 2014; Zaporozhchenko et al., 2018).
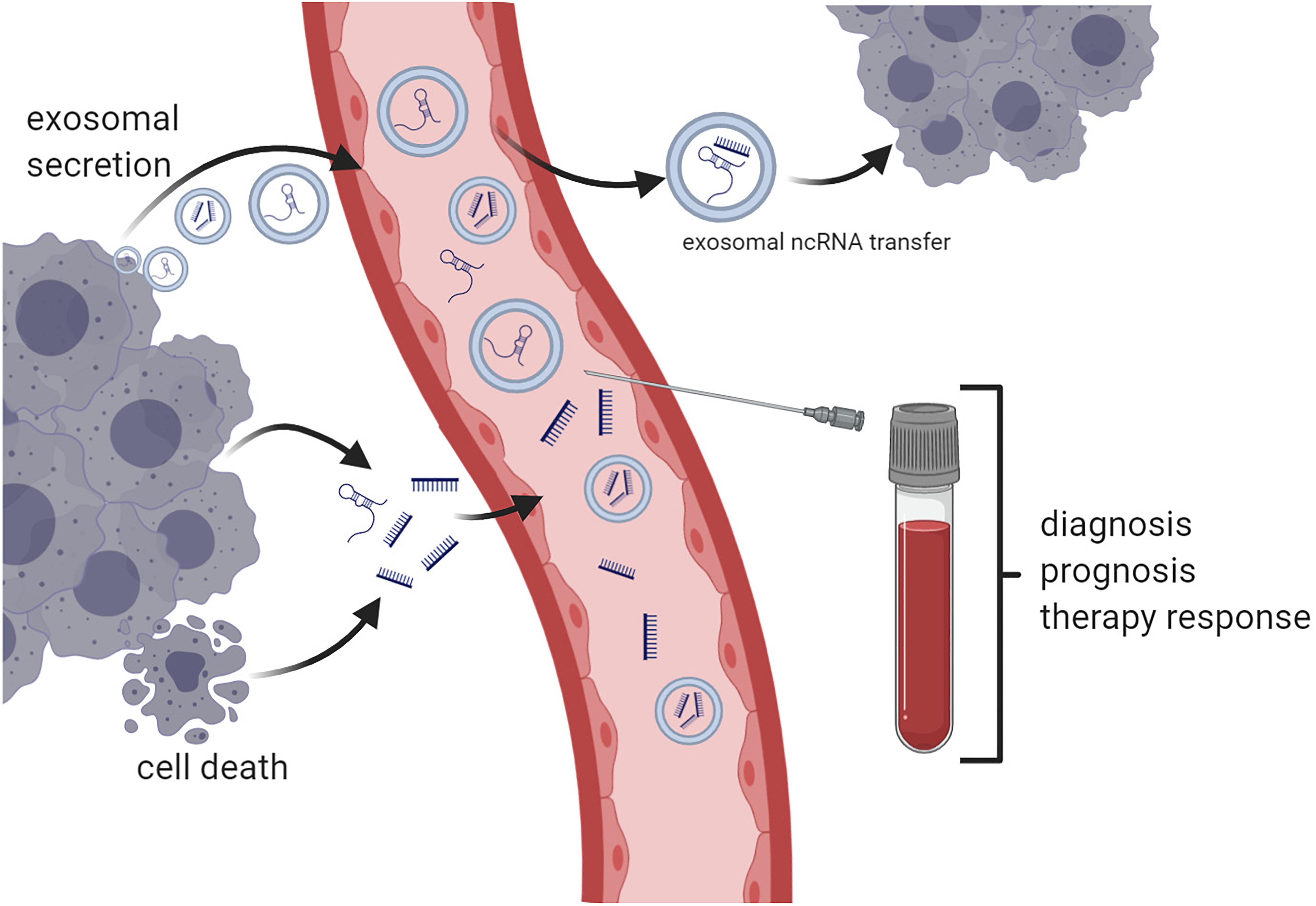
Figure 1. Principles of circulating non-coding RNA (ncRNA)-based liquid biopsy. Non-coding RNA products such as microRNAs and long non-coding RNAs are either actively secreted or released in the course of cell death. Exosomal ncRNAs can be transferred between different tumor sites and thereby disseminate metastatic potential (e.g., miR-19b-3p) or drug resistance (e.g., lncRNA ARSR). Blood samples may be used for diagnosis or prediction of prognosis and response to cancer therapies (created with Biorender.com).
Exosomes act as an intercellular communication channel, with signals encrypted in the form of the encapsulated DNA, RNA, or protein cargo. The composition of this cargo is highly cell specific and can be a status indicator of cellular homeostasis of the originating cell. Thus, the presence of a specific exosomal cargo signature, specifically miRNA composition, has been previously indicated as having indicative biomarker potential in a number of oncological diseases, including renal carcinoma (Butz et al., 2016; Du et al., 2017). Exosomes participate in intercellular communication and several cellular processes, including the regulation of angiogenesis, apoptosis, and metastasis, and may be utilized as biomarkers or therapeutic targets (Gurunathan et al., 2019; Zhang and Yu, 2019). Exosomes are actively secreted by most cell types through the endosomal sorting complexes required for transport pathway (Colombo et al., 2013), resulting in the fusion of the late endosomal multivesicular body and the cellular membrane (Trajkovic et al., 2008). Moreover, exosomes may transfer miRNAs and lncRNAs between tumor cells, thereby transferring drug resistance and metastatic potential as there is cumulative proof regarding the increased secretion and the differential miRNA signature identified in tumor-derived exosomes when compared to normal cells (Vojtech et al., 2014). While the miRNA sorting mechanisms have not been completely elucidated, recent insight points toward the interaction with a series of RNA binding proteins, such as members of the heterogeneous nuclear ribonucleoprotein family that have been proven to form vesicle-specific miRNA–protein complexes (Villarroya-Beltri et al., 2013). Other proposed mechanisms are based on the activity of the neutral sphingomyelinase 2 and the affinity of miRNAs to membrane raft-like structures containing ceramide as a possible loading mechanism (Groot and Lee, 2020). While it is not yet clear whether the currently proposed miRNA exosomal sorting mechanisms have any clinical relevance, the presence of specific miRNA signatures is indicated to have both mechanistic (Squadrito et al., 2014) and biomarker implications in cancer (Qu et al., 2016; Hosseini et al., 2017; Jiang N. et al., 2019; Wang et al., 2019).
As of yet, when considering cell-free circulating ncRNAs as blood-based biomarkers, differences in the study designs, including the choice of samples (serum versus plasma) and analyzing methods such as quantitative real-time PCR (qRT-PCR), next-generation sequencing, or microarrays, should be taken into account as they can heavily influence experimental outcomes (Lee et al., 2017). For instance, the total concentration of RNA extracted from samples is higher in serum as in plasma, which might be due to differences in processing, leading to the release of RNA when the blood is coagulating (Wang et al., 2012; Lee et al., 2017). Last but not least, differences in normalization of ncRNA measurements to molecules that are consistently expressed in healthy individuals and cancer patients can influence experimental outcomes. In addition, differences in sample collection, storage, and processing may impact the outcome of studies which, once again, impedes the comparability between studies, underlining the necessity of validation studies (Lee et al., 2017).
Circulating miRNAs in RCC
Circulating miRNAs as Potential Diagnostic Tools
Zhao et al. (2013) first discovered the potential of miR-210 in the diagnosis of RCC. Besides the upregulation of miR-210 in malignant tissues as compared to healthy adjacent parenchyma, they also discovered significantly increased miR-210 levels in serum samples of RCC patients as compared to healthy controls (Zhao et al., 2013). These results were later confirmed by several studies which found increased serum and plasma miR-210 levels in RCC (Iwamoto et al., 2014; Fedorko et al., 2015; Liu T. Y. et al., 2016; Dias et al., 2017; Chen et al., 2018a; Wang X. et al., 2018). Interestingly, the miR-210 serum levels were additionally reported to decline after curative surgery was performed (Fedorko et al., 2015; Wang X. et al., 2018). The serum miR-210 levels could successfully differentiate RCC patients form healthy individuals; however, both sensitivity and specificity are strongly varying and are 81 and 79.4% (Zhao et al., 2013), 67.5 and 70% (Wang X. et al., 2018), and 65 and 83% (Iwamoto et al., 2014) in the different studies, respectively. However, cutoffs for biomarker dichotomization were determined using ROC curve analysis, which makes them difficult to compare. Moreover, no validation cohorts for diagnostic testing were implemented in the studies (Zhao et al., 2013; Iwamoto et al., 2014; Wang X. et al., 2018). Therefore, although there is strong evidence for a potential clinical utility of miR-210 serum levels, future studies need to address these issues. Interestingly enough, serum-derived exosomal miR-210 may offer additional insight for its biomarker potential in comparison to free circulating miR-210 (Wang X. et al., 2018; Zhang et al., 2018). As expected, miR-210 was again upregulated in RCC tissues in a study by Wang X. et al. (2018), which also matched the patient’s serum samples. This indicates that changes of miR-210 expressions in RCC cells are perfectly reflected by miR-210 circulating in the patient’s blood. However, there may be significant enrichment differences between exosomal and free circulating miR-210, as the latter is increased to a comparably lesser degree. This is also reflected in the diagnostic capability of the two approaches as indicated by significantly varying sensitivity and specificity for exosomal (AUC = 0.8779, 82.5, and 80%) and exosome-free (AUC = 0.7888, 67.5, and 70%) miR-210, respectively (Wang X. et al., 2018). Furthermore, the authors demonstrated increased exosomal miR-210 secretion of RCC cell lines under hypoxic experimental conditions (Wang X. et al., 2018), which is in line with another study investigating serum miR-210 levels in RCC (Dias et al., 2017). miR-210 is strongly connected to hypoxia as it is induced by the absence of functional von Hippel–Lindau (VHL) tumor suppressor gene, which is frequently encountered in RCC tumorigenesis (Shen and Kaelin, 2013), and by increasing hypoxia-inducible factor (HIF) activity (Neal et al., 2010; McCormick et al., 2013; Liu L. L. et al., 2017). Reciprocally, miR-210 may also target hypoxia-inducible factor 1α (HIF-1α), indicating a feedback loop, and thereby suppress hypoxia-induced apoptosis (Liu L. L. et al., 2017). miR-210 upregulation under hypoxic conditions results in increased proliferation, genetic instability, angiogenesis, and metastasis and adapts mitochondrial function (Dang and Myers, 2015). An increased release of miR-210 was observed when exposing RCC cell lines to hypoxic conditions in the previously discussed study by Dias et al. (2017), corroborating these results.
Fedorko et al. (2015) also demonstrated the diagnostic potential of serum miR-210; however, in their study, significantly elevated serum levels of miR-378 were better able to differentiate RCC patients from healthy controls with AUCs of 0.74 and 0.82 for miR-210 and miR-378, respectively. Nonetheless, the discriminatory ability of miR-210 and miR-378 combined performed best and discriminated RCC cases with a sensitivity and a specificity of 80 and 78%, respectively (Fedorko et al., 2015). Even so, evidence for the utility of miR-378 in blood-based liquid biopsy is not unambiguous. Although Hauser et al. (2012) could identify serum miR-378 to be significantly higher in a set of ccRCC patients and could discriminate cancer patients form healthy controls, they could not verify their results in the validation cohort. Furthermore, another study that aimed to evaluate a diagnostic panel including five miRNAs reported the miR-378 levels to be reduced in RCC patients as compared to healthy controls (Wang et al., 2015). As opposed to this, Redova et al. (2012) again reported that miR-378 serum levels significantly increased in patients with RCC, which they could further verify in a validation cohort. Apart from miR-378, Redova et al. (2012) also analyzed the diagnostic capability of miR-451 which was found to be significantly decreased in patient serum. This was also verified in a validation cohort. However, interestingly, the combination of miR-451 with the formerly discussed miR-378 could better differentiate the serum samples of healthy individuals from RCC patients with a sensitivity of 81% and a specificity of 83% (Redova et al., 2012). The varying and partly conflicting results regarding serum miR-378 may be due to differences in cohort size as well as baseline characteristics, yet collectively the clinical utility of serum miR-378 in RCC diagnostics at that time is uncertain. In RCC, the pathogenetic role of miR-378 has not been entirely resolved; however, miR-378 may be a regulator of angiogenesis, but this hypothesis is lacking a clear experimental in vitro and in vivo evidence (Li H. C. et al., 2014). In other cancer types, a pro-angiogenetic effect of miR-378 was demonstrated (Lee et al., 2007; Krist et al., 2015), and it could serve as a biomarker for antiangiogenetic therapy in ovarian cancer (Chan et al., 2014). Its role as an oncogenic or tumor-suppressive miRNA seems to vary depending on the cancer type (Zhang et al., 2014; Chen et al., 2016; Li S. et al., 2017; Zeng et al., 2017; Ma et al., 2019), which is frequently observed in other miRNAs (Svoronos et al., 2016).
As already mentioned before, Wang et al. (2015) developed a diagnostic miRNA signature enabling the diagnosis of early ccRCC, which, besides miR-378, also includes miR-193a-3p, miR-362, miR-572, and miR-28-5p. Apart from miR-378 and miR-28-5p, which were significantly decreased, the other miRNAs contained in the diagnostic panel were significantly overexpressed in the serum of ccRCC patients. The panel was able to differentiate patients with ccRCC from non-cancerous controls, especially at the early stages of the disease, accounting for 80% sensitivity and 71% specificity in stage I ccRCC (Wang et al., 2015). However, since corresponding tissue samples were not analyzed in the study, conclusions on the altered expression levels in RCC tissue and their potential reflection in serum miRNA expression and additional involvement in ccRCC pathogenesis cannot be drawn. As for miR-193a-3p, it was shown to target the tumor suppressor phosphatase and tensin homolog (PTEN) and mediate the PI3K/Akt pathway, thereby promoting RCC cell growth and progression (Liu L. et al., 2017; Pan Y. et al., 2018). miR-572 likewise represents an oncomir in RCC pathogenesis by enhancing proliferation and invasion and inhibiting tumor cell apoptosis by suppressing NF2/Hippo signaling (Guan et al., 2018; Pan et al., 2018a). On the other hand, miR-28-5p (Wang et al., 2016) and miR-362 (Zou et al., 2016; Zhu et al., 2020) were suggested as tumor-suppressive miRNAs in RCC, although the miR-362 serum levels were increased in the study by Wang et al. (2015).
Another miRNA that was reported to qualify as liquid diagnostic biomarker is miR-144-3p, which is overexpressed in the plasma of RCC patients and could successfully discriminate RCC from both healthy individuals and patients with renal angiomyolipoma. The sensitivity and the specificity were at 87.1 and 83.02% or 75 and 71.7%, respectively (Lou et al., 2017). The miR-144-3p levels in RCC tissue were likewise increased, and the plasma levels drastically dropped after curative surgery was performed (Xiao et al., 2017). miR-144-3p may promote ccRCC progression and induce resistance to the receptor tyrosine kinase inhibitor sunitinib by targeting AT-rich interaction domain 1A (ARID1A), which is the gene of the SWI/SNF subunit in the SWI/SNF chromatin remodeling complex (Xiao et al., 2017; Mathur, 2018). ARID1A influences cell cycle, apoptosis, and p53 targets, thus acting as a tumor suppressor (Mathur, 2018). However, the role of miR-144-3p in RCC pathogenesis may not be clear as another study conversely suggested miR-144-3p both being decreased in RCC tissue and acting as a tumor suppressor by targeting mitogen-activated protein kinase 8 (MAP3K8) (Liu F. et al., 2016).
miR-508-3p and miR-509-5p were both demonstrated to play a role in RCC pathogenesis and additionally altered tissue and plasma expression levels were reported. As for miR-508-3p, the expression levels in tissue, plasma, and serum are consistently decreased as reported by two studies (Zhai et al., 2012; Liu et al., 2019a), and serum miR-508-3p was able to differentiate RCC and non-cancer samples (AUC = 0.8), which even improved when combined with increased serum levels of miR-885-5p (AUC = 0.9) (Liu et al., 2019a). As for miR-509-5p, the evidence is not as clear since its expression was found to be decreased in the plasma of RCC patients, yet the difference was not significant in every available study although the baseline characteristics of the study population were similar (Zhai et al., 2012; Zhang et al., 2013). Nonetheless, both miR-508-3p and miR-509-5p may act as tumor suppressors in RCC by inducing apoptosis and inhibiting cell proliferation and migration in vitro (Zhai et al., 2012; Zhang et al., 2013; Liu et al., 2019a). Zinc finger E-box-binding homeobox 1 and nuclear factor kappa B subunit 1 may represent targets of miR-508-3p as demonstrated in cervical and gastric cancer (Huang et al., 2016; Guo et al., 2018).
Furthermore, Chen et al. (2018a) also investigated several plasmatic miRNAs for their diagnostic potential in RCC, including miR-210, which we already discussed earlier. In their study, besides miR-210, the authors included miR-224 and miR-141 and analyzed various plasmatic combinations of the three miRNAs. Interestingly, the combination of the significantly elevated miR-224 and the decreased miR-141 plasma levels in a miR-224/miR-141 ratio showed the best diagnostic capability (AUC = 0.9898, sensitivity 97.06%, specificity 98.53%), representing a promising potential diagnostic tool in RCC patients (Chen et al., 2018a). However, this was not tested in a validation cohort, and external validation is missing. Considering the varying and partly conflicting results in the analysis of miRNAs in a larger body of evidence, at this point, it should be considered hypothesis-generating until confirmed by future studies.
miR-21, which has been repeatedly shown to be overexpressed in RCC tissue and has been suggested as a prognostic marker in RCC patients (Faragalla et al., 2012; Zaman et al., 2012; Vergho et al., 2014), was also reported to be elevated in patients’ serum samples and positively correlated to tumor stages, indicating a potential role as a blood-based diagnostic biomarker (Cheng et al., 2013; Tusong et al., 2017). Additionally, a decline in miR-21 serum levels after surgery was reported (Tusong et al., 2017). However, Sanders et al. (2012) could not confirm the potential clinical value of miR-21, as, in their analysis, the expression levels were highly similar between controls and urological cancers including RCC. This may be due to differences in the study populations as serum miR-21 could be correlated to tumor stages (Cheng et al., 2013). Interestingly, increased serum levels of miR-21 were observed in other cancer types as well, including prostate, lung, and breast cancer (Zhang H. L. et al., 2011; Liu et al., 2012; Schwarzenbach et al., 2012). Besides miR-21, Cheng et al. (2013) also discovered that miR-34a and miR-224 were overexpressed, and miR-141 expression was significantly decreased in the sera of RCC patients, corroborating previously discussed results of miR-224 and miR-141 (Chen et al., 2018a).
In RCC cells, miR-21 was proposed to act as an oncogenic miRNA and enhance tumor progression and proliferation while inhibiting apoptosis (Zhang A. et al., 2011; Li X. et al., 2014; Petrozza et al., 2015). Additionally, it may target VHL mRNA (Sun et al., 2019; Zang et al., 2019), the loss of which plays a major role in RCC tumorigenesis and promotes normoxic HIF signaling (Shen and Kaelin, 2013).
miR-106a is another candidate for a diagnostic miRNA in ccRCC as it was significantly overexpressed in the serum of 30 ccRCC patients with matched controls and was reduced after curative surgery, which indicates a potential use in post-surgery biomarker monitoring (Tusong et al., 2017). In addition, miR-106a could differentiate ccRCC patients from controls with an AUC of 0.819 and a sensitivity and a specificity of 86.7 and 70%, respectively (Tusong et al., 2017). However, the members of the miR-106 family miR-106a-5p and miR-106a∗ were previously reported to be downregulated in RCC tissue (Ma et al., 2015; Pan et al., 2017), which is in contrast to the findings of increased miR-106a levels in patient serum by Tusong et al. (2017). miR-106a-5p overexpression was shown to inhibit both cell migration and invasion in vitro and reduced metastases in vivo by targeting p21 activated kinase 5 (Pan et al., 2017). Moreover, miR-106a∗ was found to suppress the proliferation of RCC cell lines by inhibiting IRS-2 and thus inhibiting PI3K/Akt signaling (Ma et al., 2015). Therefore, the serum expression of miR-106a should be interpreted with caution unless validation in larger cohorts was performed.
Another miRNA which may qualify as a future marker in the diagnosis of RCC is miR-625-3p. It was reported to be overexpressed in ccRCC tissues yet decreased in the serum of ccRCC patients (Zhao et al., 2019a). A high tissue expression of miR-625-3p was an independent predictor for poor OS (HR = 2.949, 95%CI 1.413–6.152, p = 0.004), whereas serum expression could significantly differentiate ccRCC patients from healthy controls (AUC = 0.792, sensitivity 70.3%, specificity 80%) (Zhao et al., 2019a). However, the inconsistency in miR-625-3p expression in tumor tissue and patient serum demands further validation. In functional investigations in RCC cell lines, miR-625-3p was shown to enhance RCC cell migration and invasion and additionally inhibit apoptosis (Zhao et al., 2019a).
Wulfken et al. (2011) identified miR-1233, which showed significantly higher levels in both RCC tissue and patients’ serum, as a biomarker candidate in RCC diagnostics. The diagnostic utility was verified in a multicenter validation cohort including 84 RCC patients and 93 controls, yet although miR-1233 was able to significantly discriminate RCC patients from healthy individuals, the results in the validation cohort may not support its use in clinical routine (AUC = 0.588, sensitivity 77.4%, specificity 37.6%) (Wulfken et al., 2011). Even so, the combination of miR-1233 with miR-141 could promisingly enhance the diagnostic capability, reaching a sensitivity of 100% and a specificity of 73.3% (Yadav et al., 2017). In addition, Zhang et al. (2018) found that serum exosomal miR-1233 increased in their study including 82 RCC patients and successfully differentiated RCC from non-cancer samples, corroborating previous results. The pathogenetic role of miR-1233 in RCC to date is not well investigated; however, the observed increase of miR-1233 levels upon hypoxia in RCC cell lines indicates its involvement in hypoxic cell signaling (Dias et al., 2017).
Apart from acting as biomarkers, exosomal miRNAs were suggested to promote RCC progression. Wang et al. (2019) reported that exosomal miR-19b-3p that originated from ccRCC cancer stem cells (CSC) in RCC metastases transfers EMT potential to renal cancer cells by being incorporated by the tumor cells. Mechanistically, miR-19b-3p targets the tumor suppressor PTEN and enhances lung metastases (Wang et al., 2019). This was also verified in rodent models, and CD103 overexpression in CSC exosomes was suggested to guide exosomes to their destinations in tumor and lung tissue. Corroborating these results, in a cohort of 209 ccRCC patients, CD103+ exosomes were significantly increased in patients with metastatic disease, indicating a potential use in the diagnosis and the monitoring of metastasized ccRCC (Wang et al., 2019).
Circulating miRNAs as Prognostic Biomarkers in RCC
Dias et al. (2017) aimed to analyze the potential prognostic utility of the already afore-discussed miR-210, miR-221, and miR-1233 in the blood plasma of RCC patients. Interestingly, the increased plasma levels of all investigated miRNAs were significantly associated with poor cancer-specific survival (CSS) and independently predicted for CSS when combined as a single biomarker (HR = 3.02, 95%CI 1.19–7.64, p = 0.014). Moreover, this miRNA panel complements prognostic parameters, such as tumor stage, Fuhrman grade, age, and gender, as it significantly improves their prognostic capacity when combined to an index with already established clinico-pathological parameters (Dias et al., 2017). As for miR-221, this corroborates the findings of Teixeira et al. (2014) who reported miR-221 to be upregulated in the plasma of RCC patients. Moreover, plasma levels were found to be even higher in patients who already had metastatic disease when first diagnosed with RCC. Increased plasmatic miR-221 expression levels represented an independent prognostic marker for OS (Teixeira et al., 2014). In the same study, miR-222, the plasmatic expression of which was also observed to be increased in RCC patients versus healthy controls, did not validate as an independent predictor of overall survival (OS). Interestingly, the addition of plasmatic miR-221 levels to the already established clinico-pathological parameters TNM stage, Fuhrman grade, and age combined could improve their prognostic capability (c-index 0.8, HR = 4.5, 95%CI 1.17–17.34, p = 0.029 versus c-index 0.961, HR = 10.7, 95%CI 1.33–85.65, p = 0.26) (Teixeira et al., 2014). Both miR-221 and miR-222 were found to be significantly overexpressed in RCC tissue vs. healthy renal parenchyma and have already been considered as promoters of RCC proliferation and progression in numerous studies (Lu et al., 2015; Liu et al., 2019b; Zhao et al., 2019b; Lv et al., 2020). Furthermore, both miRNAs were proposed as predictive markers for treatment response to sunitinib therapy and were demonstrated to enhance angiogenesis via targeting the vascular endothelial growth factor receptor 2 (VEGFR2) (Khella et al., 2015; Garcia-Donas et al., 2016). This mechanism was also shown to regulate the response to sunitinib treatment in prostate cancer (Krebs et al., 2020). miR-221 was additionally suggested in the regulation of HIF-1α, which plays a major role in RCC pathogenesis (Penolazzi et al., 2019), and beyond that, it could enhance RCC cell proliferation, migration, and invasion by targeting the tissue inhibitor of metalloproteinases 2 (TIMP2) as found in cell culture models (Lu et al., 2015).
miR-224 was already shown to have a potential diagnostic utility in the previous chapter (Chen et al., 2018a). As for its impact on RCC prognosis, Fujii et al. (2017) analyzed exosomal miR-224 in RCC patients. miR-224 was upregulated in RCC tissue and the high exosomal miR-224 in the blood of 108 RCC patients independently predicted for shorter CSS, progression-free survival (PFS), and OS (CSS: HR = 1.6, 95%CI 1.1–2.5, p = 0.014; PFS: HR = 11, 95%CI 3.3–68.7, p ≤ 0.0001; OS: HR = 9.1, 95%CI 1.8–166.1, p = 0.0043), respectively (Fujii et al., 2017). Functional experiments revealed an oncogenic role of miR-224 in RCC pathogenesis. Exosomes containing miR-224 from a metastatic RCC cell line that were added to a cell line of primary RCC enhanced proliferation and invasion and, moreover, increased the intracellular miR-224 levels in primary cell lines (Fujii et al., 2017). This indicates the importance of exosomal miRNAs in cancer progression (Fujii et al., 2017; Kulkarni et al., 2019). Furthermore, miR-224 was shown to influence RCC progression by directly targeting alpha-2,3-sialyltransferase IV and impacting the PI3K/AKT pathway (Pan Y. et al., 2018).
Another study that investigated the prognostic utility of plasma exosomal miRNAs was conducted by Du et al. (2017). In their study, in a first step, plasma exosomal miRNA expression levels were evaluated in a discovery cohort including 44 patients with metastatic RCC. In a second step, the most promising miRNAs were confirmed in a validation cohort of 65 patients. Eventually, miR-26a-1-3p (HR = 0.43, 95%CI 0.1–0.84, p = 0.025), miR-615-3p (HR = 0.36, 95%CI 0.11–0.54, p = 0.0007), and miRNA let-7i-5p (HR = 0.49, 95%CI 0.21–0.84, p = 0.018) retained their prognostic value in the validation set, and decreased plasma exosomal expression was significantly associated with adverse prognosis (Du et al., 2017). Moreover, Du et al. (2017) compared the prognostic ability of the established Memorial Sloan-Kettering Cancer Center (MSKCC) score, which takes multiple clinico-pathological factors into consideration, versus the MSKCC score with the inclusion of let-7i-5p. In their analysis, the addition of let-7i-5p improved the prognostic capability (AUC = 0.64) as compared to the MSKCC score alone (AUC = 0.58) (HR = 3.43, 95%CI 2.73–24.15, p = 0.0002 for the combination) (Du et al., 2017). The miRNA let-7 family mainly exerts tumor-suppressive functions in cancer, yet several subtypes may also act as oncogenes (Chirshev et al., 2019). However, let7i-5p and its role in RCC pathogenesis have not been evaluated yet. The members of the let7-family let7b and let7c were reported to be decreased in RCC tissue and were able to suppress proliferation and restore chemoresistance to 5-fluorouracile in in vitro experiments (Peng et al., 2015). In contrast, let-7g-5p and let-7i-5p were significantly overexpressed in a study including 94 ccRCC specimens (Gowrishankar et al., 2014). The differing results across the let-7 subtype underline the different biological roles of let-7 subtypes, and further research is therefore necessary to clarify the functional role of exosomal let-7i-3p in RCC. Results in other cancer types suggest a tumor-suppressive role of let-7i as, for instance, they are downregulated in ovarian and bladder cancer and inhibited tumor proliferation and chemoresistance (Yang et al., 2008; Qin et al., 2019). As for miR-615-3p, Wang Q. et al. (2018) found it to be sponged by the lncRNA HOXA transcript at the distal tip (HOTTIP) and to target insulin-like growth factor 2 in RCC. In their study, HOTTIP was shown to be upregulated in RCC tissue, and the overexpression of HOTTIP was reported to promote RCC proliferation, migration, and invasion in vitro, implicating a tumor-suppressive role of miR-615-3p (Wang Q. et al., 2018).
Chanudet et al. (2017) screened for circulating miRNAs in RCC using microRNA arrays in plasma samples of 94 ccRCC patients of all clinical stages and 100 healthy controls. Although they could not recommend single miRNAs or miRNA signatures for the early diagnosis of ccRCC, thereby contradicting previous results, the authors found lower miR-150 expression in the plasma of ccRCC patients to be independently associated with cancer-specific survival (HR = 1.3, 95%CI 1.0–1.8, p = 0.03) (Chanudet et al., 2017). Even though miR-150 has not been functionally investigated in RCC yet, the utility of exosomal serum miR-150 levels in colorectal cancer for prognosis prediction supports the notion of its future clinical utility in RCC (Zhao Y. J. et al., 2019; Zou et al., 2019). However, miR-150 may exert different functions in different cancer types as, for instance, it promotes metastasis in lung cancer, whereas it may behave as a tumor suppressor in colorectal cancer (Li et al., 2016; Chen et al., 2018b).
Circulating miRNAs as Predictive Biomarkers for Therapy Response
Circulating miRNAs might also be involved in acquiring resistance to treatment regimens in RCC. A recent study suggests that miR-35-5p carried in extracellular vesicles participates in the development of resistance to the multi-targeted receptor tyrosine kinase inhibitor sunitinib (He et al., 2020). In the study, sorafenib-sensitive cell lines were exposed to extracellular vesicles of sorafenib-resistant RCC cells, which contained increased levels of miR-31-5p. This resulted in increasing sorafenib resistance in the former treatment-sensitive cell lines, indicating transmitted therapy resistance by miR-31-5p via extracellular vesicles. The role of miR-31-5p in the induction of sorafenib resistance was subsequently demonstrated in further in vitro and in vivo experiments, and functional analysis revealed MutL homolog 1 (MLH1) as a direct target of miR-31-5p. The authors speculate that reduced MLH1 expression and subsequent increased genomic instability may facilitate the development of drug resistance (He et al., 2020). Ultimately, He et al. (2020) found significantly increased levels of miR-31-5p within extracellular vesicles in the serum of RCC patients, who had progressed on sorafenib-based treatment, as compared to serum levels before treatment initiation. This indicates a possible role as a predictive biomarker for the development of sorafenib resistance; however, the authors did not further investigate miR-31-5p levels and the potential impact on clinical endpoints such as PFS or response rates (He et al., 2020). Nevertheless, the role of miR-31-5p in RCC pathogenesis is not without inconsistencies. A previous study suggested that miR-31-5p may act as a tumor suppressor in RCC by inhibiting proliferation, migration, and invasion via targeting cyclin-dependent kinase 1 (Li et al., 2019). Moreover, the expression of miR-31-5p has been reported to be significantly lower in RCC tissue and cell lines (Li et al., 2019).
Gamez-Pozo et al. (2012) developed several predictive models of miRNA expression in the peripheral blood for resistance to sunitinib therapy in a prospective observational multicenter study including 38 metastatic RCC patients who received first-line treatment with sunitinib. Blood samples were taken before and 2 weeks after treatment initiation, and micro-array assays and subsequent verification of promising miRNA candidates with qPCR were performed. The authors developed 12 predictive models for each response group, whereas poor response was defined as progression earlier than 6 months and prolonged response was defined as progression later than 18 months after therapy initiation. The expression levels of up to four miRNAs per individual model and 28 miRNAs in total were significantly related with their respective prognostic groups. One model of the poor response group, including miR-192, miR-193-3p, and miR-501-3p, and one model of the prolonged response group, which included miR-miR-410, miR-1181, and miR-424∗, prevailed their predictive ability in the validation with qRT-PCR (Gamez-Pozo et al., 2012). However, due to the relatively small patient sample, further research and validation is required.
Circulating Long Non-Coding RNAs in RCC
Although the utility of circulating lncRNAs as biomarkers in cancer and potential pathomechanisms have been reported repeatedly, in RCC data remain limited.
The lncRNA gradually increased during hepatocarcinogenesis (GIHCG) may represent a promising biomarker in RCC (He et al., 2018). GIHCG is overexpressed in RCC tissue as compared to healthy adjacent tissue and is also elevated in the serum of RCC patients, showing a highly significant correlation with tumor tissue expression levels. Moreover, the GIHCG serum levels were able to discriminate RCC patients and healthy individuals with a sensitivity and a specificity of 87 and 84.8%, respectively (AUC = 0.920) (He et al., 2018). In addition, GIHCG retained its diagnostic ability when only early-stage RCC and healthy controls were compared, and RCC tissue expression levels were significantly associated with OS; however, no uni- and multivariate analyses were performed (He et al., 2018). GIHCG was first described in hepatocellular carcinoma, where it was demonstrated to enhance tumor cell proliferation, migration, and metastases both in vitro and in vivo by recruiting the transcription factors EZH2 and DNMT1 to the miR-200b/a/429 promoter, thereby epigenetically silencing miR-200b/a/429 expression (Sui et al., 2016). Since then, GIHCG’s oncogenic role has been reported in various cancers such as ovarian, colorectal, gastric, and cervical cancer (Yao et al., 2018; Jiang X. et al., 2019; Liu G. et al., 2019; Zhang et al., 2019). He et al. (2018) eventually confirmed this role in RCC in knockdown experiments.
Wu et al. (2016) attempted to develop a panel including several lncRNAs as a novel diagnostic marker for RCC. In their study, potential lncRNAs for further investigation were first discovered in RCC tissue, and the serum expression of lncRNA candidates was analyzed in a discovery cohort including 25 patients with ccRCC, pRCC, or chRCC in a second step. Subsequently, promising lncRNAs were evaluated in a validation set of 37 patients and 35 healthy controls. A panel of five lncRNAs, low expressed in tumor (LET), plasmacytoma variant translocation 1 (PVT1), promoter of CDKN1A antisense DNA damage activated RNA (PANDAR), phosphatase and tensin homolog pseudogene 1 (PTENP1), and linc00963, was able to successfully discriminate non-cancer from RCC patients (AUC = 0.823) and retained their diagnostic ability throughout the different clinical TNM stages, also indicating their utility as early markers for RCC (Wu et al., 2016). In an additional small clinical patient set which also included patients with benign renal tumors, the cancer patients showed significantly higher risk indices as compared to controls (Wu et al., 2016). lncRNA LET is considered a tumor suppressor in a variety of cancer types which also accounts for RCC, where LET was shown to enhance apoptosis and impair mitochondrial membrane potential by targeting miR-373-3p, thereby regulating Dickkopf 1 and tissue TIMP2 expression (Weidle et al., 2017; Ye et al., 2019). Moreover, PTENP1, too, acts as a tumor-suppressive factor in RCC by acting as a competing endogenous RNA for miR-21, which would otherwise promote cell proliferation, migration, and invasion by the suppression of PTEN, an important tumor suppressor (Zhang A. et al., 2011; Song et al., 2012; Yu et al., 2014). In contrast, PVT1 (Pan et al., 2018b; Derderian et al., 2019) and PANDAR (Li J. et al., 2017; Han et al., 2019) are well-known oncogenic lncRNAs, and their role in RCC tumorigenesis and negative impact on RCC outcome have been reported (Bao et al., 2017; Xu et al., 2017; Yang et al., 2017; Li et al., 2018). Linc00963 has not been investigated in RCC yet but was indicated to promote cancer progression in a variety of malignancies such as head and neck cancer osteosarcoma and breast cancer (Zhou et al., 2019b; Lee et al., 2020; Wu et al., 2020). However, Wu et al. (2016) found that the expression levels of PVT1 and linc00963 were significantly downregulated in the serum of RCC patients as compared to tissue expression levels.
The lncRNA activated in RCC with sunitinib resistance (ARSR) was first described by Qu et al. (2016), who suggested the involvement of exosomal ARSR in the development of resistance to sunitinib in RCC and discovered its potential pathomechanism. ARSR expression was increased in the RCC tissue of patients who were resistant to sunitinib as well as in resistant RCC cell lines. Moreover, plasma levels were significantly elevated in RCC patients as compared to healthy controls, and detectable ARSR levels dropped after surgical treatment and increased upon tumor recurrence. Higher plasma ARSR levels independently predicted for shorter PFS for sunitinib treatment (HR = 2.9, 95%CI 1.2–7.1, p = 0.017) (Qu et al., 2016). This indicates that the circulating ARSR in RCC patients is a promising biomarker for response to sunitinib (Qu et al., 2016). Interestingly, ARSR has also been associated with drug resistance in osteosarcoma and hepatocellular carcinoma (Li Y. et al., 2017; Shen and Cheng, 2020). Furthermore, Qu et al. (2016) elucidated the underlying mechanism in RCC and demonstrated that ARSR upregulation is indispensable for the development of sunitinib resistance. ARSR was shown to sponge both miR-34 and miR-449, thereby preventing them from binding to their targets AXL and c-MET, thus inhibiting their degradation. As a result, ARSR upregulation results in a likewise increase of AXL and c-MET and further activation of the downstream STAT3, AKT, and ERK signaling pathway (Qu et al., 2016). The involvement of ARSR in STAT3 and AKT regulation was confirmed in liver cancer (Li Y. et al., 2017; Yang et al., 2019). Moreover, in RCC, AKT was found to promote ARSR expression by inhibiting forkhead box protein O1/3a (FOXO1 and FOXO3a), which results in the formation of a positive feedback loop (Qu et al., 2016). Interestingly, the exosomal secretion of ARSR was reported to transfer sunitinib resistance to former sunitinib-sensitive cells both in vitro and in vivo, indicating the exosomal dissemination of sunitinib resistance. In addition, therapeutically targeting ARSR could restore sunitinib sensitivity in vivo (Qu et al., 2016).
Circulating YRNAs in RCC
YRNAs are small ncRNA molecules that form characteristic stem-loop formations because of their complementary 5′ and 3′ UTRs (Pruijn et al., 1993). Originally, they were reported in rheumatic diseases where they were found to be part of soluble ribonucleoproteins (Hendrick et al., 1981). YRNAs comprise the four subtypes hY1, hY3, hY4, and hY5 and are abundant in both serum and plasma (Dhahbi et al., 2013; Yeri et al., 2017). Recently, they have been suggested as circulating biomarkers in breast as well as head and neck cancer (Dhahbi et al., 2014; Victoria Martinez et al., 2015). In RCC, although Nientiedt et al. (2018) reported significant alterations in the expression levels of hY3 and hY4 in malignant versus healthy tissue, they could not find any differences in serum expression levels. Thus, YRNAs do not bring any additional value as easily available blood-based biomarkers in RCC (Nientiedt et al., 2018).
Comparison to Current Standards
The early diagnosis of RCC still remains difficult due to the lack of broad screening programs and a specific panel of symptoms already noticeable at early-stage disease. Many patients are diagnosed incidentally when they present with symptoms like hematuria, abdominal mass, or flank pain (Vasudev et al., 2020). Since many of these symptoms are non-specific, the majority of patients do not get completely evaluated within the first 6 months after initial presentation (Zhou et al., 2019a), thus reducing the number of patients diagnosed at an early stage. This is a major issue since early diagnosis is crucial for successful therapy and is associated with better patient survival (Tsui et al., 2000; Palsdottir et al., 2012). Hence, finding liquid biomarkers for early-stage disease detection might prove to be beneficial for patients. Due to the minimal invasiveness of liquid biopsies, they could be performed routinely. However, up to now, there is no established biomarker available for clinical use. RCC is reported to present with lower circulating tumor DNA (ctDNA) levels compared to other tumor entities (Smith et al., 2020), excluding it as a feasible diagnostic marker in RCC. Also, CTCs have not been established for clinical use in RCC diagnosis yet due to heterogenous surface marker expression (Bu et al., 2020), which makes an enrichment with the CellSearchTM system, the only FDA-approved CTC capture platform, difficult (Bai et al., 2018). Therefore, circulating ncRNAs might represent useful and easily available biomarkers in the diagnosis and treatment of RCC. As summarized in this review, the analysis of various circulating ncRNAs can distinguish RCC patients from healthy donors. However, systematic screenings and larger-scale studies, which would be necessary for a potential clinical implementation in the diagnosis of early-stage RCC, are missing.
For prognostic purposes, the International Metastatic RCC Database Consortium (IMDC) and MSKCC risk scores are the current standard tools in RCC. Both use a panel of six or five clinical parameters to predict patient outcome, respectively (Tanaka et al., 2016). Even though they are widely validated, there are also reports showing their limitations. Indeed they performed fairly accurate in predicting OS in different cohorts of mRCC patients undergoing first- and second-line targeted therapy (Heng et al., 2009; Ko et al., 2015; Tanaka et al., 2016). Their respective prognoses for around 23% of patients in a treatment-naïve cohort undergoing the first line of targeted therapy do not match, suggesting limited prognostic capacity (Okita et al., 2019). They also do not perform well in predicting OS in patients undergoing cytoreductive nephrectomy (Westerman et al., 2020). Thus, implementation of additional or new biomarkers, to reliably stratify RCC patients in the era of targeted therapies, might be beneficial. It was already shown that the inclusion of an additional miRNA as a marker could improve the prognostic capacity of the MSKCC score (Du et al., 2017). Various ncRNAs, as described in this review, have prognostic capacities for OS (Teixeira et al., 2014) or therapy response (Gamez-Pozo et al., 2012; Li et al., 2019; He et al., 2020). In general, multiple miRNAs or lncRNAs summarized in a distinctive panel or signature appear to bring higher predictive values. Hence, comparisons of ncRNAs alone or in combination with well-established clinical scores, with scores like the MSKCC and IMDC, might be interesting to evaluate to check whether prognosis prediction in various settings can be improved.
Conclusion
In order to make circulating ncRNAs feasible biomarkers for diagnosis and prognosis in RCC, comparisons with current, well-established standards are necessary to determine whether they add any value. Therefore, sensitivity, specificity, and accuracy would have to be compared or added to scores like the IMDC or the MSKCC or other promising methods such as genomic profiling.
However, due to missing validations and an ongoing discussion about, for example, optimal sample processing and normalization, circulating ncRNAs are not yet to enter clinical practice in RCC, and further research is needed.
Author Contributions
DB and MP conceptualized this work. DB contributed to visualization and writing—preparing the original draft. DB, RD, LO, LF, OS, GC, and MP contributed to writing—reviewing and editing the manuscript. MP supervised the study. OS, GC, and MP took charge of funding acquisition. All the authors have read and agreed to the published version of the manuscript.
Funding
This project has received funding from the European Union’s Horizon 2020 Research and Innovation Program under the Marie Skłodowska-Curie grant agreement no. 824036.
Conflict of Interest
The authors declare that the research was conducted in the absence of any commercial or financial relationships that could be construed as a potential conflict of interest.
Acknowledgments
DB thanks the Society for Cancer Patients (Verein für Krebskranke) of the Medical University of Graz for the financial support during his traineeship at the MD Anderson Cancer Center.
References
Arroyo, J. D., Chevillet, J. R., Kroh, E. M., Ruf, I. K., Pritchard, C. C., Gibson, D. F., et al. (2011). Argonaute2 complexes carry a population of circulating microRNAs independent of vesicles in human plasma. Proc. Natl. Acad. Sci. U.S.A. 108, 5003–5008. doi: 10.1073/pnas.1019055108
Bai, M., Zou, B., Wang, Z., Li, P., Wang, H., Ou, Y., et al. (2018). Comparison of two detection systems for circulating tumor cells among patients with renal cell carcinoma. Int. Urol. Nephrol. 50, 1801–1809. doi: 10.1007/s11255-018-1954-2
Bao, X., Duan, J., Yan, Y., Ma, X., Zhang, Y., Wang, H., et al. (2017). Upregulation of long noncoding RNA PVT1 predicts unfavorable prognosis in patients with clear cell renal cell carcinoma. Cancer Biomark 21, 55–63. doi: 10.3233/cbm-170251
Barth, D. A., Slaby, O., Klec, C., Juracek, J., Drula, R., Calin, G. A., et al. (2019). Current concepts of non-coding RNAs in the pathogenesis of non-clear cell renal cell carcinoma. Cancers (Basel) 11:10.3390/cancers11101580.
Bergerot, P. G., Hahn, A. W., Bergerot, C. D., Jones, J., and Pal, S. K. (2018). The role of circulating tumor DNA in renal cell carcinoma. Curr. Treat. Options Oncol. 19:10.
Bryzgunova, O. E., and Laktionov, P. P. (2015). Extracellular nucleic acids in urine: sources, structure, diagnostic potential. Acta Naturae 7, 48–54. doi: 10.32607/20758251-2015-7-3-48-54
Bu, J., Nair, A., Kubiatowicz, L. J., Poellmann, M. J., Jeong, W. J., Reyes-Martinez, M., et al. (2020). Surface engineering for efficient capture of circulating tumor cells in renal cell carcinoma: From nanoscale analysis to clinical application. Biosens. Bioelectron. 162:112250. doi: 10.1016/j.bios.2020.112250
Butz, H., Nofech-Mozes, R., Ding, Q., Khella, H. W. Z., Szabo, P. M., Jewett, M., et al. (2016). Exosomal MicroRNAs are diagnostic biomarkers and can mediate cell-cell communication in renal cell carcinoma. Eur. Urol. Focus 2, 210–218. doi: 10.1016/j.euf.2015.11.006
Cella, D., Grunwald, V., Escudier, B., Hammers, H. J., George, S., Nathan, P., et al. (2019). Patient-reported outcomes of patients with advanced renal cell carcinoma treated with nivolumab plus ipilimumab versus sunitinib (CheckMate 214): a randomised, phase 3 trial. Lancet Oncol. 20, 297–310. doi: 10.1016/s1470-2045(18)30778-2
Chan, J. K., Kiet, T. K., Blansit, K., Ramasubbaiah, R., Hilton, J. F., Kapp, D. S., et al. (2014). MiR-378 as a biomarker for response to anti-angiogenic treatment in ovarian cancer. Gynecol. Oncol. 133, 568–574. doi: 10.1016/j.ygyno.2014.03.564
Chanudet, E., Wozniak, M. B., Bouaoun, L., Byrnes, G., Mukeriya, A., Zaridze, D., et al. (2017). Large-scale genome-wide screening of circulating microRNAs in clear cell renal cell carcinoma reveals specific signatures in late-stage disease. Int. J. Cancer 141, 1730–1740. doi: 10.1002/ijc.30845
Chen, Q. G., Zhou, W., Han, T., Du, S. Q., Li, Z. H., Zhang, Z., et al. (2016). MiR-378 suppresses prostate cancer cell growth through downregulation of MAPK1 in vitro and in vivo. Tumour Biol. 37, 2095–2103. doi: 10.1007/s13277-015-3996-8
Chen, X., Lou, N., Ruan, A., Qiu, B., Yan, Y., Wang, X., et al. (2018a). miR-224/miR-141 ratio as a novel diagnostic biomarker in renal cell carcinoma. Oncol. Lett. 16, 1666–1674.
Chen, X., Xu, X., Pan, B., Zeng, K., Xu, M., Liu, X., et al. (2018b). miR-150-5p suppresses tumor progression by targeting VEGFA in colorectal cancer. Aging (Albany NY) 10, 3421–3437. doi: 10.18632/aging.101656
Cheng, T., Wang, L., Li, Y., Huang, C., Zeng, L., and Yang, J. (2013). Differential microRNA expression in renal cell carcinoma. Oncol. Lett. 6, 769–776. doi: 10.3892/ol.2013.1460
Chi, Y., Wang, D., Wang, J., Yu, W., and Yang, J. (2019). Long non-coding RNA in the pathogenesis of cancers. Cells 8:1015. doi: 10.3390/cells8091015
Chirshev, E., Oberg, K. C., Ioffe, Y. J., and Unternaehrer, J. J. (2019). Let-7 as biomarker, prognostic indicator, and therapy for precision medicine in cancer. Clin. Transl. Med. 8:24.
Cimadamore, A., Gasparrini, S., Massari, F., Santoni, M., Cheng, L., Lopez-Beltran, A., et al. (2019). Emerging molecular technologies in renal cell carcinoma: liquid biopsy. Cancers (Basel) 11:196. doi: 10.3390/cancers11020196
Colombo, M., Moita, C., van Niel, G., Kowal, J., Vigneron, J., Benaroch, P., et al. (2013). Analysis of ESCRT functions in exosome biogenesis, composition and secretion highlights the heterogeneity of extracellular vesicles. J. Cell. Sci. 126(Pt 24), 5553–5565. doi: 10.1242/jcs.128868
Cortez, M. A., Bueso-Ramos, C., Ferdin, J., Lopez-Berestein, G., Sood, A. K., and Calin, G. A. (2011). MicroRNAs in body fluids–the mix of hormones and biomarkers. Nat. Rev. Clin. Oncol. 8, 467–477. doi: 10.1038/nrclinonc.2011.76
Dalpiaz, O., Luef, T., Seles, M., Stotz, M., Stojakovic, T., Pummer, K., et al. (2017). Critical evaluation of the potential prognostic value of the pretreatment-derived neutrophil-lymphocyte ratio under consideration of C-reactive protein levels in clear cell renal cell carcinoma. Br. J. Cancer 116, 85–90. doi: 10.1038/bjc.2016.393
Dang, K., and Myers, K. A. (2015). The role of hypoxia-induced miR-210 in cancer progression. Int. J. Mol. Sci. 16, 6353–6372. doi: 10.3390/ijms16036353
Derderian, C., Orunmuyi, A. T., Olapade-Olaopa, E. O., and Ogunwobi, O. O. (2019). PVT1 signaling is a mediator of cancer progression. Front. Oncol. 9:502. doi: 10.3389/fonc.2019.00502
Dhahbi, J. M., Spindler, S. R., Atamna, H., Boffelli, D., and Martin, D. I. (2014). Deep sequencing of serum small RNAs identifies patterns of 5’ tRNA half and YRNA fragment expression associated with breast cancer. Biomark Cancer 6, 37–47.
Dhahbi, J. M., Spindler, S. R., Atamna, H., Boffelli, D., Mote, P., and Martin, D. I. (2013). 5’-YRNA fragments derived by processing of transcripts from specific YRNA genes and pseudogenes are abundant in human serum and plasma. Physiol. Genomics 45, 990–998. doi: 10.1152/physiolgenomics.00129.2013
Dias, F., Teixeira, A. L., Ferreira, M., Adem, B., Bastos, N., Vieira, J., et al. (2017). Plasmatic miR-210, miR-221 and miR-1233 profile: potential liquid biopsies candidates for renal cell carcinoma. Oncotarget 8, 103315–103326. doi: 10.18632/oncotarget.21733
Dieckmann, K. P., Radtke, A., Geczi, L., Matthies, C., Anheuser, P., Eckardt, U., et al. (2019). Serum levels of MicroRNA-371a-3p (M371 Test) as a new biomarker of testicular germ cell tumors: results of a prospective multicentric study. J. Clin. Oncol. 37, 1412–1423. doi: 10.1200/jco.18.01480
Du, M., Giridhar, K. V., Tian, Y., Tschannen, M. R., Zhu, J., Huang, C. C., et al. (2017). Plasma exosomal miRNAs-based prognosis in metastatic kidney cancer. Oncotarget 8, 63703–63714. doi: 10.18632/oncotarget.19476
Ellinger, J., Gevensleben, H., Muller, S. C., and Dietrich, D. (2016). The emerging role of non-coding circulating RNA as a biomarker in renal cell carcinoma. Expert Rev. Mol. Diagn. 16, 1059–1065. doi: 10.1080/14737159.2016.1239531
Faragalla, H., Youssef, Y. M., Scorilas, A., Khalil, B., White, N. M., Mejia-Guerrero, S., et al. (2012). The clinical utility of miR-21 as a diagnostic and prognostic marker for renal cell carcinoma. J. Mol. Diagn. 14, 385–392. doi: 10.1016/j.jmoldx.2012.02.003
Farber, N. J., Kim, C. J., Modi, P. K., Hon, J. D., Sadimin, E. T., and Singer, E. A. (2017). Renal cell carcinoma: the search for a reliable biomarker. Transl. Cancer Res. 6, 620–632.
Fedorko, M., Stanik, M., Iliev, R., Redova-Lojova, M., Machackova, T., Svoboda, M., et al. (2015). Combination of MiR-378 and MiR-210 serum levels enables sensitive detection of renal cell carcinoma. Int. J. Mol. Sci. 16, 23382–23389. doi: 10.3390/ijms161023382
Ferlay, J., Soerjomataram, I., Dikshit, R., Eser, S., Mathers, C., Rebelo, M., et al. (2015). Cancer incidence and mortality worldwide: sources, methods and major patterns in GLOBOCAN 2012. Int. J. Cancer 136, E359–E386.
Fujii, N., Hirata, H., Ueno, K., Mori, J., Oka, S., Shimizu, K., et al. (2017). Extracellular miR-224 as a prognostic marker for clear cell renal cell carcinoma. Oncotarget 8, 109877–109888. doi: 10.18632/oncotarget.22436
Gallo, A., Tandon, M., Alevizos, I., and Illei, G. G. (2012). The majority of microRNAs detectable in serum and saliva is concentrated in exosomes. PLoS One 7:e30679. doi: 10.1371/journal.pone.0030679
Gamez-Pozo, A., Anton-Aparicio, L. M., Bayona, C., Borrega, P., Gallegos Sancho, M. I., Garcia-Dominguez, R., et al. (2012). MicroRNA expression profiling of peripheral blood samples predicts resistance to first-line sunitinib in advanced renal cell carcinoma patients. Neoplasia 14, 1144–1152.
Garcia-Donas, J., Beuselinck, B., Inglada-Perez, L., Grana, O., Schoffski, P., Wozniak, A., et al. (2016). Deep sequencing reveals microRNAs predictive of antiangiogenic drug response. JCI Insight 1:e86051.
Gowrishankar, B., Ibragimova, I., Zhou, Y., Slifker, M. J., Devarajan, K., Al-Saleem, T., et al. (2014). MicroRNA expression signatures of stage, grade, and progression in clear cell RCC. Cancer Biol. Ther. 15, 329–341. doi: 10.4161/cbt.27314
Groot, M., and Lee, H. (2020). Sorting mechanisms for MicroRNAs into extracellular vesicles and their associated diseases. Cells 9:1044. doi: 10.3390/cells9041044
Guan, Y., Gong, Z., Xiao, T., and Li, Z. (2018). Knockdown of miR-572 suppresses cell proliferation and promotes apoptosis in renal cell carcinoma cells by targeting the NF2/Hippo signaling pathway. Int. J. Clin. Exp. Pathol. 11, 5705–5714.
Guo, S. J., Zeng, H. X., Huang, P., Wang, S., Xie, C. H., and Li, S. J. (2018). MiR-508-3p inhibits cell invasion and epithelial-mesenchymal transition by targeting ZEB1 in triple-negative breast cancer. Eur. Rev. Med. Pharmacol. Sci. 22, 6379–6385.
Gurunathan, S., Kang, M. H., Jeyaraj, M., Qasim, M., and Kim, J. H. (2019). Review of the isolation, characterization, biological function, and multifarious therapeutic approaches of exosomes. Cells 8:307. doi: 10.3390/cells8040307
Han, L., Wang, B., Wang, R., Wang, Z., Gong, S., Chen, G., et al. (2019). Prognostic and clinicopathological significance of long non-coding RNA PANDAR expression in cancer patients: a meta-analysis. Front. Oncol. 9:1337. doi: 10.3389/fonc.2019.01337
Hauser, S., Wulfken, L. M., Holdenrieder, S., Moritz, R., Ohlmann, C. H., Jung, V., et al. (2012). Analysis of serum microRNAs (miR-26a-2∗, miR-191, miR-337-3p and miR-378) as potential biomarkers in renal cell carcinoma. Cancer Epidemiol. 36, 391–394. doi: 10.1016/j.canep.2012.04.001
He, J., He, J., Min, L., He, Y., Guan, H., Wang, J., et al. (2020). Extracellular vesicles transmitted miR-31-5p promotes sorafenib resistance by targeting MLH1 in renal cell carcinoma. Int. J. Cancer 146, 1052–1063. doi: 10.1002/ijc.32543
He, Z. H., Qin, X. H., Zhang, X. L., Yi, J. W., and Han, J. Y. (2018). Long noncoding RNA GIHCG is a potential diagnostic and prognostic biomarker and therapeutic target for renal cell carcinoma. Eur. Rev. Med. Pharmacol. Sci. 22, 46–54.
Hendrick, J. P., Wolin, S. L., Rinke, J., Lerner, M. R., and Steitz, J. A. (1981). Ro small cytoplasmic ribonucleoproteins are a subclass of La ribonucleoproteins: further characterization of the Ro and La small ribonucleoproteins from uninfected mammalian cells. Mol. Cell. Biol. 1, 1138–1149. doi: 10.1128/mcb.1.12.1138
Heng, D. Y., Xie, W., Regan, M. M., Warren, M. A., Golshayan, A. R., Sahi, C., et al. (2009). Prognostic factors for overall survival in patients with metastatic renal cell carcinoma treated with vascular endothelial growth factor-targeted agents: results from a large, multicenter study. J. Clin. Oncol. 27, 5794–5799. doi: 10.1200/jco.2008.21.4809
Hosseini, M., Khatamianfar, S., Hassanian, S. M., Nedaeinia, R., Shafiee, M., Maftouh, M., et al. (2017). Exosome-encapsulated microRNAs as potential circulating biomarkers in colon cancer. Curr. Pharm. Des. 23, 1705–1709. doi: 10.2174/1381612822666161201144634
Huang, T., Kang, W., Zhang, B., Wu, F., Dong, Y., Tong, J. H., et al. (2016). miR-508-3p concordantly silences NFKB1 and RELA to inactivate canonical NF-kappaB signaling in gastric carcinogenesis. Mol. Cancer 15:9.
Huang, W., Zhao, M., Wei, N., Wang, X., Cao, H., Du, Q., et al. (2014). Site-specific RNase A activity was dramatically reduced in serum from multiple types of cancer patients. PLoS One 9:e96490. doi: 10.1371/journal.pone.0096490
Iwamoto, H., Kanda, Y., Sejima, T., Osaki, M., Okada, F., and Takenaka, A. (2014). Serum miR-210 as a potential biomarker of early clear cell renal cell carcinoma. Int. J. Oncol. 44, 53–58. doi: 10.3892/ijo.2013.2169
Janzen, N. K., Kim, H. L., Figlin, R. A., and Belldegrun, A. S. (2003). Surveillance after radical or partial nephrectomy for localized renal cell carcinoma and management of recurrent disease. Urol. Clin. North Am. 30, 843–852. doi: 10.1016/s0094-0143(03)00056-9
Javidi, M. A., Ahmadi, A. H., Bakhshinejad, B., Nouraee, N., Babashah, S., and Sadeghizadeh, M. (2014). Cell-free microRNAs as cancer biomarkers: the odyssey of miRNAs through body fluids. Med. Oncol. 31:295.
Jiang, N., Pan, J., Fang, S., Zhou, C., Han, Y., Chen, J., et al. (2019). Liquid biopsy: Circulating exosomal long noncoding RNAs in cancer. Clin. Chim. Acta 495, 331–337. doi: 10.1016/j.cca.2019.04.082
Jiang, X., Li, Q., Zhang, S., Song, C., and Zheng, P. (2019). Long noncoding RNA GIHCG induces cancer progression and chemoresistance and indicates poor prognosis in colorectal cancer. Onco Targets Ther. 12, 1059–1070. doi: 10.2147/ott.s192290
Khella, H. W. Z., Butz, H., Ding, Q., Rotondo, F., Evans, K. R., Kupchak, P., et al. (2015). miR-221/222 are involved in response to sunitinib treatment in metastatic renal cell carcinoma. Mol. Ther. 23, 1748–1758. doi: 10.1038/mt.2015.129
Ko, J. J., Xie, W., Kroeger, N., Lee, J. L., Rini, B. I., Knox, J. J., et al. (2015). The international metastatic renal cell carcinoma database consortium model as a prognostic tool in patients with metastatic renal cell carcinoma previously treated with first-line targeted therapy: a population-based study. Lancet Oncol. 16, 293–300. doi: 10.1016/s1470-2045(14)71222-7
Koczera, P., Martin, L., Marx, G., and Schuerholz, T. (2016). The Ribonuclease A superfamily in humans: canonical RNases as the buttress of innate immunity. Int. J. Mol. Sci. 17:1278. doi: 10.3390/ijms17081278
Krebs, M., Solimando, A. G., Kalogirou, C., Marquardt, A., Frank, T., Sokolakis, I., et al. (2020). miR-221-3p regulates VEGFR2 expression in high-risk prostate cancer and represents an escape mechanism from sunitinib In Vitro. J. Clin. Med. 9:670. doi: 10.3390/jcm9030670
Krist, B., Florczyk, U., Pietraszek-Gremplewicz, K., Jozkowicz, A., and Dulak, J. (2015). The role of miR-378a in metabolism, angiogenesis, and muscle biology. Int. J. Endocrinol. 2015:281756.
Kulkarni, B., Kirave, P., Gondaliya, P., Jash, K., Jain, A., Tekade, R. K., et al. (2019). Exosomal miRNA in chemoresistance, immune evasion, metastasis and progression of cancer. Drug Discov. Today 24, 2058–2067. doi: 10.1016/j.drudis.2019.06.010
Lee, D. Y., Deng, Z., Wang, C. H., and Yang, B. B. (2007). MicroRNA-378 promotes cell survival, tumor growth, and angiogenesis by targeting SuFu and Fus-1 expression. Proc. Natl. Acad. Sci. U.S.A. 104, 20350–20355. doi: 10.1073/pnas.0706901104
Lee, I., Baxter, D., Lee, M. Y., Scherler, K., and Wang, K. (2017). The importance of standardization on analyzing circulating RNA. Mol. Diagn. Ther. 21, 259–268. doi: 10.1007/s40291-016-0251-y
Lee, S. P., Hsieh, P. L., Fang, C. Y., Chu, P. M., Liao, Y. W., Yu, C. H., et al. (2020). LINC00963 promotes cancer stemness, metastasis, and drug resistance in head and neck carcinomas via ABCB5 regulation. Cancers (Basel) 12:10.3390/cancers12051073.
Li, G., Zhao, A., Peoch, M., Cottier, M., and Mottet, N. (2017). Detection of urinary cell-free miR-210 as a potential tool of liquid biopsy for clear cell renal cell carcinoma. Urol. Oncol. 35, 294–299. doi: 10.1016/j.urolonc.2016.12.007
Li, H., Ouyang, R., Wang, Z., Zhou, W., Chen, H., Jiang, Y., et al. (2016). MiR-150 promotes cellular metastasis in non-small cell lung cancer by targeting FOXO4. Sci. Rep. 6:39001.
Li, H. C., Li, J. P., Wang, Z. M., Fu, D. L., Li, Z. L., Zhang, D., et al. (2014). Identification of angiogenesis-related miRNAs in a population of patients with renal clear cell carcinoma. Oncol. Rep. 32, 2061–2069. doi: 10.3892/or.2014.3403
Li, J., Li, Z., Zheng, W., Li, X., Wang, Z., Cui, Y., et al. (2017). PANDAR: a pivotal cancer-related long non-coding RNA in human cancers. Mol. Biosyst. 13, 2195–2201. doi: 10.1039/c7mb00414a
Li, S., Yang, F., Wang, M., Cao, W., and Yang, Z. (2017). miR-378 functions as an onco-miRNA by targeting the ST7L/Wnt/β-catenin pathway in cervical cancer. Int. J. Mol. Med. 40, 1047–1056. doi: 10.3892/ijmm.2017.3116
Li, W., Zheng, Z., Chen, H., Cai, Y., and Xie, W. (2018). Knockdown of long non-coding RNA PVT1 induces apoptosis and cell cycle arrest in clear cell renal cell carcinoma through the epidermal growth factor receptor pathway. Oncol. Lett. 15, 7855–7863.
Li, X., Xin, S., He, Z., Che, X., Wang, J., Xiao, X., et al. (2014). MicroRNA-21 (miR-21) post-transcriptionally downregulates tumor suppressor PDCD4 and promotes cell transformation, proliferation, and metastasis in renal cell carcinoma. Cell Physiol. Biochem. 33, 1631–1642. doi: 10.1159/000362946
Li, Y., Quan, J., Chen, F., Pan, X., Zhuang, C., Xiong, T., et al. (2019). MiR-31-5p acts as a tumor suppressor in renal cell carcinoma by targeting cyclin-dependent kinase 1 (CDK1). Biomed. Pharmacother. 111, 517–526. doi: 10.1016/j.biopha.2018.12.102
Li, Y., Ye, Y., Feng, B., and Qi, Y. (2017). Long noncoding RNA lncARSR promotes doxorubicin resistance in hepatocellular carcinoma via modulating PTEN-PI3K/Akt pathway. J. Cell. Biochem. 118, 4498–4507. doi: 10.1002/jcb.26107
Liu, F., Chen, N., Xiao, R., Wang, W., and Pan, Z. (2016). miR-144-3p serves as a tumor suppressor for renal cell carcinoma and inhibits its invasion and metastasis by targeting MAP3K8. Biochem. Biophys. Res. Commun. 480, 87–93. doi: 10.1016/j.bbrc.2016.10.004
Liu, G., Jiang, Z., Qiao, M., and Wang, F. (2019). Lnc-GIHCG promotes cell proliferation and migration in gastric cancer through miR- 1281 adsorption. Mol. Genet. Genomic Med. 7:e711. doi: 10.1002/mgg3.711
Liu, L., Li, Y., Liu, S., Duan, Q., Chen, L., Wu, T., et al. (2017). Downregulation of miR-193a-3p inhibits cell growth and migration in renal cell carcinoma by targeting PTEN. Tumour Biol. 39:1010428317711951.
Liu, L. L., Li, D., He, Y. L., Zhou, Y. Z., Gong, S. H., Wu, L. Y., et al. (2017). miR-210 protects renal cell against hypoxia-induced apoptosis by targeting HIF-1 alpha. Mol. Med. 23, 258–271. doi: 10.2119/molmed.2017.00013
Liu, S., Deng, X., and Zhang, J. (2019a). Identification of dysregulated serum miR-508-3p and miR-885-5p as potential diagnostic biomarkers of clear cell renal carcinoma. Mol. Med. Rep. 20, 5075–5083.
Liu, S., Wang, Y., Li, W., Yu, S., Wen, Z., Chen, Z., et al. (2019b). miR-221-5p acts as an oncogene and predicts worse survival in patients of renal cell cancer. Biomed. Pharmacother. 119:109406. doi: 10.1016/j.biopha.2019.109406
Liu, T. Y., Zhang, H., Du, S. M., Li, J., and Wen, X. H. (2016). Expression of microRNA-210 in tissue and serum of renal carcinoma patients and its effect on renal carcinoma cell proliferation, apoptosis, and invasion. Genet. Mol. Res. 15:15017746.
Liu, X. G., Zhu, W. Y., Huang, Y. Y., Ma, L. N., Zhou, S. Q., Wang, Y. K., et al. (2012). High expression of serum miR-21 and tumor miR-200c associated with poor prognosis in patients with lung cancer. Med. Oncol. 29, 618–626. doi: 10.1007/s12032-011-9923-y
Lou, N., Ruan, A. M., Qiu, B., Bao, L., Xu, Y. C., Zhao, Y., et al. (2017). miR-144-3p as a novel plasma diagnostic biomarker for clear cell renal cell carcinoma. Urol. Oncol. 35, 36.e7–36.e14. doi: 10.1016/j.urolonc.2016.07.012
Lu, G. J., Dong, Y. Q., Zhang, Q. M., Di, W. Y., Jiao, L. Y., Gao, Q. Z., et al. (2015). miRNA-221 promotes proliferation, migration and invasion by targeting TIMP2 in renal cell carcinoma. Int. J. Clin. Exp. Pathol. 8, 5224–5229.
Lv, J., Zhu, Y., and Zhang, Q. (2020). An increased level of MiR-222-3p is associated with TMP2 suppression, ERK activation and is associated with metastasis and a poor prognosis in renal clear cell carcinoma. Cancer Biomark. 28, 141–149. doi: 10.3233/cbm-190264
Ma, J., Wu, D., Yi, J., Yi, Y., Zhu, X., Qiu, H., et al. (2019). MiR-378 promoted cell proliferation and inhibited apoptosis by enhanced stem cell properties in chronic myeloid leukemia K562 cells. Biomed. Pharmacother. 112:108623. doi: 10.1016/j.biopha.2019.108623
Ma, Y., Zhang, H., He, X., Song, H., Qiang, Y., Li, Y., et al. (2015). miR-106a∗ inhibits the proliferation of renal carcinoma cells by targeting IRS-2. Tumour Biol. 36, 8389–8398. doi: 10.1007/s13277-015-3605-x
Mathur, R. (2018). ARID1A loss in cancer: Towards a mechanistic understanding. Pharmacol. Ther. 190, 15–23. doi: 10.1016/j.pharmthera.2018.05.001
McCormick, R. I., Blick, C., Ragoussis, J., Schoedel, J., Mole, D. R., Young, A. C., et al. (2013). miR-210 is a target of hypoxia-inducible factors 1 and 2 in renal cancer, regulates ISCU and correlates with good prognosis. Br. J. Cancer 108, 1133–1142. doi: 10.1038/bjc.2013.56
Motzer, R. J., Escudier, B., McDermott, D. F., George, S., Hammers, H. J., Srinivas, S., et al. (2015). Nivolumab versus everolimus in advanced renal-cell carcinoma. N. Engl. J. Med. 373, 1803–1813.
Motzer, R. J., Tannir, N. M., McDermott, D. F., Aren Frontera, O., Melichar, B., Choueiri, T. K., et al. (2018). Nivolumab plus ipilimumab versus sunitinib in advanced renal-cell carcinoma. N. Engl. J. Med. 378, 1277–1290.
Nandagopal, L., and Sonpavde, G. (2016). Circulating biomarkers in bladder cancer. Bladder Cancer 2, 369–379. doi: 10.3233/blc-160075
Neal, C. S., Michael, M. Z., Rawlings, L. H., Van der Hoek, M. B., and Gleadle, J. M. (2010). The VHL-dependent regulation of microRNAs in renal cancer. BMC Med. 8:64. doi: 10.1186/1741-7015-8-64
Nientiedt, M., Schmidt, D., Kristiansen, G., Muller, S. C., and Ellinger, J. (2018). YRNA expression profiles are altered in clear cell renal cell carcinoma. Eur. Urol. Focus 4, 260–266. doi: 10.1016/j.euf.2016.08.004
Novikova, I. V., Hennelly, S. P., and Sanbonmatsu, K. Y. (2013). Tackling structures of long noncoding RNAs. Int. J. Mol. Sci. 14, 23672–23684. doi: 10.3390/ijms141223672
Okita, K., Hatakeyama, S., Tanaka, T., Ikehata, Y., Tanaka, T., Fujita, N., et al. (2019). Impact of disagreement between two risk group models on prognosis in patients with metastatic renal-cell carcinoma. Clin. Genitourin. Cancer 17, e440–e446. doi: 10.1016/j.clgc.2019.01.006
Palsdottir, H. B., Hardarson, S., Petursdottir, V., Jonsson, A., Jonsson, E., Sigurdsson, M. I., et al. (2012). Incidental detection of renal cell carcinoma is an independent prognostic marker: results of a long-term, whole population study. J. Urol. 187, 48–53. doi: 10.1016/j.juro.2011.09.025
Pan, X., Li, Z., Zhao, L., Quan, J., Zhou, L., Xu, J., et al. (2018a). microRNA-572 functions as an oncogene and a potential biomarker for renal cell carcinoma prognosis. Oncol. Rep. 40, 3092–3101.
Pan, X., Zheng, G., and Gao, C. (2018b). LncRNA PVT1: a novel therapeutic target for cancers. Clin. Lab. 64, 655–662.
Pan, Y., Hu, J., Ma, J., Qi, X., Zhou, H., Miao, X., et al. (2018). MiR-193a-3p and miR-224 mediate renal cell carcinoma progression by targeting alpha-2,3-sialyltransferase IV and the phosphatidylinositol 3 kinase/Akt pathway. Mol. Carcinog. 57, 1067–1077. doi: 10.1002/mc.22826
Pan, Y. J., Wei, L. L., Wu, X. J., Huo, F. C., Mou, J., and Pei, D. S. (2017). MiR-106a-5p inhibits the cell migration and invasion of renal cell carcinoma through targeting PAK5. Cell Death Dis. 8:e3155. doi: 10.1038/cddis.2017.561
Peng, J., Mo, R., Ma, J., and Fan, J. (2015). Let-7b and Let-7c are determinants of intrinsic chemoresistance in renal cell carcinoma. World J. Surg. Oncol. 13:175.
Penolazzi, L., Bonaccorsi, G., Gafa, R., Ravaioli, N., Gabriele, D., Bosi, C., et al. (2019). SLUG/HIF1-alpha/miR-221 regulatory circuit in endometrial cancer. Gene 711:143938. doi: 10.1016/j.gene.2019.06.028
Petrozza, V., Carbone, A., Bellissimo, T., Porta, N., Palleschi, G., Pastore, A. L., et al. (2015). Oncogenic MicroRNAs characterization in clear cell renal cell carcinoma. Int. J. Mol. Sci. 16, 29219–29225.
Pichler, M., Rodriguez-Aguayo, C., Nam, S. Y., Dragomir, M. P., Bayraktar, R., Anfossi, S., et al. (2020). Therapeutic potential of FLANC, a novel primate-specific long non-coding RNA in colorectal cancer. Gut doi: 10.1136/gutjnl-2019-318903 [Epub ahead of print].
Powles, T., Albiges, L., Staehler, M., Bensalah, K., Dabestani, S., Giles, R. H., et al. (2017). Updated European association of urology guidelines recommendations for the treatment of first-line metastatic clear cell renal cancer. Eur. Urol. 73, 311–315. doi: 10.1016/j.eururo.2017.11.016
Pruijn, G. J., Wingens, P. A., Peters, S. L., Thijssen, J. P., and van Venrooij, W. J. (1993). Ro RNP associated Y RNAs are highly conserved among mammals. Biochim. Biophys. Acta 1216, 395–401. doi: 10.1016/0167-4781(93)90006-y
Qin, M. M., Chai, X., Huang, H. B., Feng, G., Li, X. N., Zhang, J., et al. (2019). let-7i inhibits proliferation and migration of bladder cancer cells by targeting HMGA1. BMC Urol. 19:53. doi: 10.1186/s12894-019-0485-1
Qu, L., Ding, J., Chen, C., Wu, Z. J., Liu, B., Gao, Y., et al. (2016). Exosome-transmitted lncARSR promotes sunitinib resistance in renal cancer by acting as a competing endogenous RNA. Cancer Cell 29, 653–668. doi: 10.1016/j.ccell.2016.03.004
Redova, M., Poprach, A., Nekvindova, J., Iliev, R., Radova, L., Lakomy, R., et al. (2012). Circulating miR-378 and miR-451 in serum are potential biomarkers for renal cell carcinoma. J. Transl. Med. 10:55. doi: 10.1186/1479-5876-10-55
Rini, B. I., Plimack, E. R., Stus, V., Gafanov, R., Hawkins, R., Nosov, D., et al. (2019). Pembrolizumab plus Axitinib versus Sunitinib for advanced renal-cell carcinoma. N. Engl. J. Med. 380, 1116–1127.
Rossi, S. H., Klatte, T., Usher-Smith, J., and Stewart, G. D. (2018). Epidemiology and screening for renal cancer. World J. Urol. 36, 1341–1353.
Sanders, I., Holdenrieder, S., Walgenbach-Brunagel, G., von Ruecker, A., Kristiansen, G., Muller, S. C., et al. (2012). Evaluation of reference genes for the analysis of serum miRNA in patients with prostate cancer, bladder cancer and renal cell carcinoma. Int. J. Urol. 19, 1017–1025. doi: 10.1111/j.1442-2042.2012.03082.x
Schanza, L. M., Seles, M., Stotz, M., Fosselteder, J., Hutterer, G. C., Pichler, M., et al. (2017). MicroRNAs associated with von hippel-lindau pathway in renal cell carcinoma: a comprehensive review. Int. J. Mol. Sci. 18:10.3390/ijms18112495.
Schwarzenbach, H., Milde-Langosch, K., Steinbach, B., Muller, V., and Pantel, K. (2012). Diagnostic potential of PTEN-targeting miR-214 in the blood of breast cancer patients. Breast Cancer Res. Treat. 134, 933–941. doi: 10.1007/s10549-012-1988-6
Schwarzenbacher, D., Klec, C., Pasculli, B., Cerk, S., Rinner, B., Karbiener, M., et al. (2019). MiR-1287-5p inhibits triple negative breast cancer growth by interaction with phosphoinositide 3-kinase CB, thereby sensitizing cells for PI3Kinase inhibitors. Breast Cancer Res. 21:20.
Seles, M., Posch, F., Pichler, G. P., Gary, T., Pummer, K., Zigeuner, R., et al. (2017). Blood platelet volume represents a novel prognostic factor in patients with nonmetastatic renal cell carcinoma and improves the predictive ability of established prognostic scores. J. Urol. 198, 1247–1252. doi: 10.1016/j.juro.2017.07.036
Shen, C., and Kaelin, W. G. Jr. (2013). The VHL/HIF axis in clear cell renal carcinoma. Semin. Cancer Biol. 23, 18–25. doi: 10.1016/j.semcancer.2012.06.001
Shen, P., and Cheng, Y. (2020). Long noncoding RNA lncARSR confers resistance to Adriamycin and promotes osteosarcoma progression. Cell Death Dis. 11:362.
Siegel, R. L., Miller, K. D., and Jemal, A. (2019). Cancer statistics, 2019. CA Cancer J. Clin. 69, 7–34.
Smith, C. G., Moser, T., Mouliere, F., Field-Rayner, J., Eldridge, M., Riediger, A. L., et al. (2020). Comprehensive characterization of cell-free tumor DNA in plasma and urine of patients with renal tumors. Genome Med. 12:23.
Song, M. S., Salmena, L., and Pandolfi, P. P. (2012). The functions and regulation of the PTEN tumour suppressor. Nat. Rev. Mol. Cell. Biol. 13, 283–296. doi: 10.1038/nrm3330
Squadrito, M. L., Baer, C., Burdet, F., Maderna, C., Gilfillan, G. D., Lyle, R., et al. (2014). Endogenous RNAs modulate microRNA sorting to exosomes and transfer to acceptor cells. Cell Rep. 8, 1432–1446. doi: 10.1016/j.celrep.2014.07.035
Su, Y. J., Yu, J., Huang, Y. Q., and Yang, J. (2015). Circulating long noncoding RNA as a potential target for prostate cancer. Int. J. Mol. Sci. 16, 13322–13338. doi: 10.3390/ijms160613322
Sui, C. J., Zhou, Y. M., Shen, W. F., Dai, B. H., Lu, J. J., Zhang, M. F., et al. (2016). Long noncoding RNA GIHCG promotes hepatocellular carcinoma progression through epigenetically regulating miR-200b/a/429. J. Mol. Med. (Berl) 94, 1281–1296. doi: 10.1007/s00109-016-1442-z
Sun, J., Jiang, Z., Li, Y., Wang, K., Chen, X., and Liu, G. (2019). Downregulation of miR-21 inhibits the malignant phenotype of pancreatic cancer cells by targeting VHL. Onco Targets Ther. 12, 7215–7226. doi: 10.2147/ott.s211535
Svoronos, A. A., Engelman, D. M., and Slack, F. J. (2016). OncomiR or tumor suppressor? The duplicity of MicroRNAs in cancer. Cancer Res. 76, 3666–3670. doi: 10.1158/0008-5472.can-16-0359
Tanaka, N., Mizuno, R., Ito, K., Shirotake, S., Yasumizu, Y., Masunaga, A., et al. (2016). External validation of the MSKCC and IMDC risk models in patients treated with targeted therapy as a first-line and subsequent second-line treatment: a Japanese multi-institutional study. Eur. Urol. Focus 2, 303–309. doi: 10.1016/j.euf.2015.11.001
Teixeira, A. L., Ferreira, M., Silva, J., Gomes, M., Dias, F., Santos, J. I., et al. (2014). Higher circulating expression levels of miR-221 associated with poor overall survival in renal cell carcinoma patients. Tumour Biol. 35, 4057–4066. doi: 10.1007/s13277-013-1531-3
Trajkovic, K., Hsu, C., Chiantia, S., Rajendran, L., Wenzel, D., Wieland, F., et al. (2008). Ceramide triggers budding of exosome vesicles into multivesicular endosomes. Science 319, 1244–1247. doi: 10.1126/science.1153124
Tsui, K. H., Shvarts, O., Smith, R. B., Figlin, R., de Kernion, J. B., and Belldegrun, A. (2000). Renal cell carcinoma: prognostic significance of incidentally detected tumors. J. Urol. 163, 426–430. doi: 10.1016/s0022-5347(05)67892-5
Tusong, H., Maolakuerban, N., Guan, J., Rexiati, M., Wang, W. G., Azhati, B., et al. (2017). Functional analysis of serum microRNAs miR-21 and miR-106a in renal cell carcinoma. Cancer Biomark. 18, 79–85. doi: 10.3233/cbm-160676
Tzimagiorgis, G., Michailidou, E. Z., Kritis, A., Markopoulos, A. K., and Kouidou, S. (2011). Recovering circulating extracellular or cell-free RNA from bodily fluids. Cancer Epidemiol. 35, 580–589. doi: 10.1016/j.canep.2011.02.016
Vasudev, N. S., Wilson, M., Stewart, G. D., Adeyoju, A., Cartledge, J., Kimuli, M., et al. (2020). Challenges of early renal cancer detection: symptom patterns and incidental diagnosis rate in a multicentre prospective UK cohort of patients presenting with suspected renal cancer. BMJ Open 10:e035938. doi: 10.1136/bmjopen-2019-035938
Vergho, D., Kneitz, S., Rosenwald, A., Scherer, C., Spahn, M., Burger, M., et al. (2014). Combination of expression levels of miR-21 and miR-126 is associated with cancer-specific survival in clear-cell renal cell carcinoma. BMC Cancer 14:25. doi: 10.1186/1471-2407-14-25
Victoria Martinez, B., Dhahbi, J. M., Nunez Lopez, Y. O., Lamperska, K., Golusinski, P., Luczewski, L., et al. (2015). Circulating small non-coding RNA signature in head and neck squamous cell carcinoma. Oncotarget 6, 19246–19263. doi: 10.18632/oncotarget.4266
Villarroya-Beltri, C., Gutiérrez-Vázquez, C., Sánchez-Cabo, F., Pérez-Hernández, D., Vázquez, J., Martin-Cofreces, N., et al. (2013). Sumoylated hnRNPA2B1 controls the sorting of miRNAs into exosomes through binding to specific motifs. Nat. Commun. 4:2980.
Vojtech, L., Woo, S., Hughes, S., Levy, C., Ballweber, L., Sauteraud, R. P., et al. (2014). Exosomes in human semen carry a distinctive repertoire of small non-coding RNAs with potential regulatory functions. Nucleic Acids Res. 42, 7290–7304. doi: 10.1093/nar/gku347
Wagner, J., Riwanto, M., Besler, C., Knau, A., Fichtlscherer, S., Röxe, T., et al. (2013). Characterization of levels and cellular transfer of circulating lipoprotein-bound microRNAs. Arterioscler Thromb Vasc. Biol. 33, 1392–1400. doi: 10.1161/atvbaha.112.300741
Wang, C., Hu, J., Lu, M., Gu, H., Zhou, X., Chen, X., et al. (2015). A panel of five serum miRNAs as a potential diagnostic tool for early-stage renal cell carcinoma. Sci. Rep. 5:7610.
Wang, C., Wu, C., Yang, Q., Ding, M., Zhong, J., Zhang, C. Y., et al. (2016). miR-28-5p acts as a tumor suppressor in renal cell carcinoma for multiple antitumor effects by targeting RAP1B. Oncotarget 7, 73888–73902. doi: 10.18632/oncotarget.12516
Wang, K., Yuan, Y., Cho, J. H., McClarty, S., Baxter, D., and Galas, D. J. (2012). Comparing the MicroRNA spectrum between serum and plasma. PLoS One 7:e41561. doi: 10.1371/journal.pone.0041561
Wang, L., Yang, G., Zhao, D., Wang, J., Bai, Y., Peng, Q., et al. (2019). CD103-positive CSC exosome promotes EMT of clear cell renal cell carcinoma: role of remote MiR-19b-3p. Mol. Cancer 18:86.
Wang, Q., Wu, G., Zhang, Z., Tang, Q., Zheng, W., Chen, X., et al. (2018). Long non-coding RNA HOTTIP promotes renal cell carcinoma progression through the regulation of the miR-615/IGF-2 pathway. Int. J. Oncol. 53, 2278–2288.
Wang, X., Wang, T., Chen, C., Wu, Z., Bai, P., Li, S., et al. (2018). Serum exosomal miR-210 as a potential biomarker for clear cell renal cell carcinoma. J. Cell. Biochem. 120, 1492–1502. doi: 10.1002/jcb.27347
Weidle, U. H., Birzele, F., Kollmorgen, G., and Ruger, R. (2017). Long non-coding RNAs and their role in metastasis. Cancer Genomics Proteomics 14, 143–160.
Westerman, M. E., Shapiro, D. D., Tannir, N. M., Campbell, M. T., Matin, S. F., Karam, J. A., et al. (2020). Survival following cytoreductive nephrectomy: a comparison of existing prognostic models. BJU Int. doi: 10.1111/bju.15160 [Epub ahead of print].
Wu, Y., Wang, Y. Q., Weng, W. W., Zhang, Q. Y., Yang, X. Q., Gan, H. L., et al. (2016). A serum-circulating long noncoding RNA signature can discriminate between patients with clear cell renal cell carcinoma and healthy controls. Oncogenesis 5:e192. doi: 10.1038/oncsis.2015.48
Wu, Z., Wang, W., Wang, Y., Wang, X., Sun, S., Yao, Y., et al. (2020). Long noncoding RNA LINC00963 promotes breast cancer progression by functioning as a molecular sponge for microRNA-625 and thereby upregulating HMGA1. Cell Cycle 19, 610–624. doi: 10.1080/15384101.2020.1728024
Wulfken, L. M., Moritz, R., Ohlmann, C., Holdenrieder, S., Jung, V., Becker, F., et al. (2011). MicroRNAs in renal cell carcinoma: diagnostic implications of serum miR-1233 levels. PLoS One 6:e25787. doi: 10.1371/journal.pone.0025787
Xiao, W., Lou, N., Ruan, H., Bao, L., Xiong, Z., Yuan, C., et al. (2017). Mir-144-3p promotes cell proliferation, metastasis, sunitinib resistance in clear cell Renal cell carcinoma by downregulating ARID1A. Cell Physiol. Biochem. 43, 2420–2433. doi: 10.1159/000484395
Xu, Y., Tong, Y., Zhu, J., Lei, Z., Wan, L., Zhu, X., et al. (2017). An increase in long non-coding RNA PANDAR is associated with poor prognosis in clear cell renal cell carcinoma. BMC Cancer 17:373. doi: 10.1186/s12885-017-3339-9
Yadav, S., Khandelwal, M., Seth, A., Saini, A. K., Dogra, P. N., and Sharma, A. (2017). Serum microRNA expression profiling: potential diagnostic implications of a panel of serum microRNAs for clear cell renal cell cancer. Urology 104, 64–69. doi: 10.1016/j.urology.2017.03.013
Yang, C., Cai, W. C., Dong, Z. T., Guo, J. W., Zhao, Y. J., Sui, C. J., et al. (2019). lncARSR promotes liver cancer stem cells expansion via STAT3 pathway. Gene 687, 73–81. doi: 10.1016/j.gene.2018.10.087
Yang, N., Kaur, S., Volinia, S., Greshock, J., Lassus, H., Hasegawa, K., et al. (2008). MicroRNA microarray identifies Let-7i as a novel biomarker and therapeutic target in human epithelial ovarian cancer. Cancer Res. 68, 10307–10314. doi: 10.1158/0008-5472.can-08-1954
Yang, T., Zhou, H., Liu, P., Yan, L., Yao, W., Chen, K., et al. (2017). lncRNA PVT1 and its splicing variant function as competing endogenous RNA to regulate clear cell renal cell carcinoma progression. Oncotarget 8, 85353–85367. doi: 10.18632/oncotarget.19743
Yao, N., Yu, L., Zhu, B., Gan, H. Y., and Guo, B. Q. (2018). LncRNA GIHCG promotes development of ovarian cancer by regulating microRNA-429. Eur. Rev. Med. Pharmacol. Sci. 22, 8127–8134.
Ye, Z., Duan, J., Wang, L., Ji, Y., and Qiao, B. (2019). LncRNA-LET inhibits cell growth of clear cell renal cell carcinoma by regulating miR-373-3p. Cancer Cell Int. 19:311.
Yeri, A., Courtright, A., Reiman, R., Carlson, E., Beecroft, T., Janss, A., et al. (2017). Total extracellular small RNA profiles from plasma, saliva, and urine of healthy subjects. Sci. Rep. 7:44061.
Yu, G., Yao, W., Gumireddy, K., Li, A., Wang, J., Xiao, W., et al. (2014). Pseudogene PTENP1 functions as a competing endogenous RNA to suppress clear-cell renal cell carcinoma progression. Mol. Cancer Ther. 13, 3086–3097. doi: 10.1158/1535-7163.mct-14-0245
Zaman, M. S., Shahryari, V., Deng, G., Thamminana, S., Saini, S., Majid, S., et al. (2012). Up-regulation of microRNA-21 correlates with lower kidney cancer survival. PLoS One 7:e31060. doi: 10.1371/journal.pone.0031060
Zang, C., Sun, J., Liu, W., Chu, C., Jiang, L., and Ge, R. (2019). miRNA-21 promotes cell proliferation and invasion via VHL/PI3K/AKT in papillary thyroid carcinoma. Hum. Cell 32, 428–436. doi: 10.1007/s13577-019-00254-4
Zaporozhchenko, I. A., Ponomaryova, A. A., Rykova, E. Y., and Laktionov, P. P. (2018). The potential of circulating cell-free RNA as a cancer biomarker: challenges and opportunities. Expert Rev. Mol. Diagn. 18, 133–145. doi: 10.1080/14737159.2018.1425143
Zeng, M., Zhu, L., Li, L., and Kang, C. (2017). miR-378 suppresses the proliferation, migration and invasion of colon cancer cells by inhibiting SDAD1. Cell Mol. Biol. Lett. 22:12.
Zhai, Q., Zhou, L., Zhao, C., Wan, J., Yu, Z., Guo, X., et al. (2012). Identification of miR-508-3p and miR-509-3p that are associated with cell invasion and migration and involved in the apoptosis of renal cell carcinoma. Biochem. Biophys. Res. Commun. 419, 621–626. doi: 10.1016/j.bbrc.2012.02.060
Zhang, A., Liu, Y., Shen, Y., Xu, Y., and Li, X. (2011). miR-21 modulates cell apoptosis by targeting multiple genes in renal cell carcinoma. Urology 78, 474.e13–474.e19. doi: 10.1016/j.urology.2011.03.030
Zhang, G. J., Zhou, H., Xiao, H. X., Li, Y., and Zhou, T. (2014). MiR-378 is an independent prognostic factor and inhibits cell growth and invasion in colorectal cancer. BMC Cancer 14:109. doi: 10.1186/1471-2407-14-109
Zhang, H. L., Yang, L. F., Zhu, Y., Yao, X. D., Zhang, S. L., Dai, B., et al. (2011). Serum miRNA-21: elevated levels in patients with metastatic hormone-refractory prostate cancer and potential predictive factor for the efficacy of docetaxel-based chemotherapy. Prostate 71, 326–331. doi: 10.1002/pros.21246
Zhang, L., and Yu, D. (2019). Exosomes in cancer development, metastasis, and immunity. Biochim. Biophys. Acta Rev. Cancer 1871, 455–468. doi: 10.1016/j.bbcan.2019.04.004
Zhang, W., Ni, M., Su, Y., Wang, H., Zhu, S., Zhao, A., et al. (2018). MicroRNAs in serum exosomes as potential biomarkers in clear-cell renal cell carcinoma. Eur. Urol. Focus 4, 412–419. doi: 10.1016/j.euf.2016.09.007
Zhang, W. B., Pan, Z. Q., Yang, Q. S., and Zheng, X. M. (2013). Tumor suppressive miR-509-5p contributes to cell migration, proliferation and antiapoptosis in renal cell carcinoma. Ir. J. Med. Sci. 182, 621–627. doi: 10.1007/s11845-013-0941-y
Zhang, X., Mao, L., Li, L., He, Z., Wang, N., and Song, Y. (2019). Long noncoding RNA GIHCG functions as an oncogene and serves as a serum diagnostic biomarker for cervical cancer. J. Cancer 10, 672–681. doi: 10.7150/jca.28525
Zhao, A., Li, G., Peoc’h, M., Genin, C., and Gigante, M. (2013). Serum miR-210 as a novel biomarker for molecular diagnosis of clear cell renal cell carcinoma. Exp. Mol. Pathol. 94, 115–120. doi: 10.1016/j.yexmp.2012.10.005
Zhao, L., Liu, K., Pan, X., Quan, J., Zhou, L., Li, Z., et al. (2019a). miR-625-3p promotes migration and invasion and reduces apoptosis of clear cell renal cell carcinoma. Am. J. Transl. Res. 11, 6475–6486.
Zhao, L., Quan, J., Li, Z., Pan, X., Wang, J., Xu, J., et al. (2019b). MicroRNA-222-3p promotes tumor cell migration and invasion and inhibits apoptosis, and is correlated with an unfavorable prognosis of patients with renal cell carcinoma. Int. J. Mol. Med. 43, 525–534.
Zhao, Y. J., Song, X., Niu, L., Tang, Y., Song, X., and Xie, L. (2019). Circulating Exosomal miR-150-5p and miR-99b-5p as diagnostic biomarkers for colorectal cancer. Front. Oncol. 9:1129. doi: 10.3389/fonc.2019.01129
Zhou, Y., van Melle, M., Singh, H., Hamilton, W., Lyratzopoulos, G., and Walter, F. M. (2019a). Quality of the diagnostic process in patients presenting with symptoms suggestive of bladder or kidney cancer: a systematic review. BMJ Open 9:e029143. doi: 10.1136/bmjopen-2019-029143
Zhou, Y., Yin, L., Li, H., Liu, L. H., and Xiao, T. (2019b). The LncRNA LINC00963 facilitates osteosarcoma proliferation and invasion by suppressing miR-204-3p/FN1 axis. Cancer Biol. Ther. 20, 1141–1148. doi: 10.1080/15384047.2019.1598766
Zhu, H., Wang, S., Shen, H., Zheng, X., and Xu, X. (2020). SP1/AKT/FOXO3 signaling is involved in miR-362-3p-mediated inhibition of cell-cycle pathway and EMT progression in renal cell carcinoma. Front. Cell Dev. Biol. 8:297. doi: 10.3389/fcell.2020.00297
Znaor, A., Lortet-Tieulent, J., Laversanne, M., Jemal, A., and Bray, F. (2015). International variations and trends in renal cell carcinoma incidence and mortality. Eur. Urol. 67, 519–530. doi: 10.1016/j.eururo.2014.10.002
Zou, S. L., Chen, Y. L., Ge, Z. Z., Qu, Y. Y., Cao, Y., and Kang, Z. X. (2019). Downregulation of serum exosomal miR-150-5p is associated with poor prognosis in patients with colorectal cancer. Cancer Biomark 26, 69–77. doi: 10.3233/cbm-190156
Keywords: biomarker, liquid biopsy, prognosis, long non-coding RNA, microRNA, prognosis, diagnosis, renal cell carcinoma
Citation: Barth DA, Drula R, Ott L, Fabris L, Slaby O, Calin GA and Pichler M (2020) Circulating Non-coding RNAs in Renal Cell Carcinoma—Pathogenesis and Potential Implications as Clinical Biomarkers. Front. Cell Dev. Biol. 8:828. doi: 10.3389/fcell.2020.00828
Received: 01 July 2020; Accepted: 04 August 2020;
Published: 15 September 2020.
Edited by:
Mohd Wasim Nasser, University of Nebraska Medical Center, United StatesReviewed by:
Maria Francesca Baietti, VIB KU Leuven Center for Cancer Biology, BelgiumAli M. Ardekani, Avicenna Research Institute (ARI), Iran
Vinay Sagar, Northwestern Medicine, United States
Copyright © 2020 Barth, Drula, Ott, Fabris, Slaby, Calin and Pichler. This is an open-access article distributed under the terms of the Creative Commons Attribution License (CC BY). The use, distribution or reproduction in other forums is permitted, provided the original author(s) and the copyright owner(s) are credited and that the original publication in this journal is cited, in accordance with accepted academic practice. No use, distribution or reproduction is permitted which does not comply with these terms.
*Correspondence: Martin Pichler, martin.pichler@medunigraz.at