- 1ImmunoSurgery Unit, Champalimaud Centre for the Unknown, Lisbon, Portugal
- 2Digestive Unit, Champalimaud Centre for the Unknown, Lisbon, Portugal
- 3University of Heidelberg, Heidelberg, Germany
- 4Department of Pathology, Champalimaud Centre for the Unknown, Lisbon, Portugal
- 5Lisbon Centre for Blood and Transplantation, Instituto Português do Sangue e Transplantação, Lisbon, Portugal
- 6Division of Infection and Immunity, NIHR Biomedical Research Centre, University College London Hospitals NHS Foundation Trust, University College London, London, United Kingdom
The intricate interplay between the immune system and microbes is an essential part of the physiological homeostasis in health and disease. Immunological recognition of commensal microbes, such as bacterial species resident in the gut or lung as well as dormant viral species, i.e., cytomegalovirus (CMV) or Epstein-Barr virus (EBV), in combination with a balanced immune regulation, is central to achieve immune-protection. Emerging evidence suggests that immune responses primed to guard against commensal microbes may cause unexpected pathological outcomes, e.g., chronic inflammation and/or malignant transformation. Furthermore, translocation of immune cells from one anatomical compartment to another, i.e., the gut-lung axis via the lymphatics or blood has been identified as an important factor in perpetrating systemic inflammation, tissue destruction, as well as modulating host-protective immune responses. We present in this review immune response patterns to pathogenic as well as non-pathogenic microbes and how these immune-recognition profiles affect local immune responses or malignant transformation. We discuss personalized immunological therapies which, directly or indirectly, target host biological pathways modulated by antimicrobial immune responses.
Background
Our understanding of the immune system stems, in great part, from studying the host response to infection, which in most individuals leads to the absence of clinical disease and establishment of highly apt immunological memory. The host immune response in relation to opportunistic pathogens as well as to the endogenous microbiota is pivotal to deter not only infectious diseases, yet also central to providing general physiological health (Manfredo Vieira et al., 2018; Mathieu et al., 2018a; Schachter et al., 2018). Chronic infections, particularly those which are primarily characterized by an asymptomatic intracellular life cycle, e.g., latent Mycobacterium tuberculosis infection (LTBI), hepatitis B virus (HBV) infection, Chlamydia trachomatis infection, cytomegalovirus (CMV) or Epstein-Barr virus (EBV) infections, present a unique premise to decipher the fine balance between protective host immune responses, immunopathology and full-fledged clinical disease. Nevertheless, while a chronic host immune response driven by pathogens may be protective against clinical disease, it may also elevate the risk of inflammation-induced dysplasia. The association of certain human leukocyte antigen (HLA) alleles which predispose individuals to a greater risk of harmful inflammation and disease (Mignot et al., 2001; De la Herran-Arita et al., 2013; Tafti et al., 2016; Matzaraki et al., 2017) play a central role in pro-inflammatory processes. We will first highlight some of the major neoplasia-associated infections of clinical relevance in the context of neoplasia and immune response modulation.
Although overt inflammatory responses play a major role in malignant transformation of host cells following an infection, it is a disbalanced immune responses, which contribute to drive malignant transformation. Thus, the local immunological milieu in tissue compartments forms the nature and magnitude of the host responses, i.e., frequencies of regulatory T cells (Tregs) vs. T-helper 17 (Th17) cells, amount of pro-inflammatory cytokines vs. anti-inflammatory cytokines, extent of neutrophilia and antigen-presenting-cell (APC) activation, among others. The second part of the review discusses potential host-directed interventional strategies based on existing translational and clinical knowledge of infection-induced inflammation, as well as cancer initiation/progression models.
Pathogen-Driven Inflammation and Neoplasia: Existing Knowledge and New Insights
Viral Pathogens and Immuno-Oncogenesis
Most infection-induced cancers worldwide are attributed to viral pathogens, possibly representing up to 80% of cases reported (Chang Y. et al., 2017). Although harbored by at least 90% of the world’s population, EBV causes malignant transformation only in a handful of individuals, which has been in part linked to the genetic variations in the infecting strain (Tzellos and Farrell, 2012). EBV-induced cancers, such as nasopharyngeal carcinoma (NPC) and B-cell lymphomas in the form of severe lymphoproliferative disease (LPD) following stem cell transplantation, non-Hodgkin’s lymphoma (NHL) as well as Hodgkin’s lymphoma (HL) are well documented (comprehensively reviewed in Saha and Robertson, 2011; Farrell, 2019). LPDs can also involve some populations of T cells (thus, manifesting as a T-cell lymphoma) and natural killer (NK) cells (Kim et al., 2017). The fact that patients with some cancer histologies/molecular profiles respond to immune checkpoint inhibitors (ICI), such as anti-PD-1, anti-CTLA-4, and anti-PD-L1 allows the study their impact on non-target T-cell populations (those not directed specifically against cancer-associated mutations or neoantigens), i.e., on CMV or EBV-reactive T cells. A clinical study with anti-PD-1 blockade in patients with lung cancer showed that EBV-specific T cells were not expanded during lung cancer treatment (Kamphorst et al., 2017). There is also a clinical trial currently underway to treat patients with EBV-positive NHL or other LPDs with EBV-specific cytotoxic T cells activated using antigen-pulsed dendritic cells in combination with nivolumab (anti-PD-1 antibody) (ClinicalTrials.gov identifier: NCT02973113). EBV-specific tumour infiltrating lymphocytes (TILs)/T cells have also been shown to mediate tumor killing in vitro as well as disease remission in patients with NPC (He et al., 2012; Li et al., 2015). HLA-B35, along with HLA-B2, -A2 and -A11 have been shown to be associated with a higher risk of developing post-transplant lymphoproliferative disease (PTLD) post solid-organ transplantation (Pourfarziani et al., 2007), while another study in Denmark showed that HLA-B45 and HLA-DR13 pose an increased PTLD risk (Vase et al., 2015). Indeed, a HLAB35-restricted epitope from EBV BZLF1 protein was previously shown to elicit strong cytotoxic T-cell responses (Tynan et al., 2005), while circulating IFN-γ+ CD8+ T cells in patients with PTLD were dominantly reactive to a HLA-B35-restricted epitope from EBV Epstein-Barr nuclear antigen 1 (EBNA1) (Jones et al., 2010). Interestingly, EBNA1 is also involved in downregulation of the HLA class I molecule to avoid immune surveillance (Levitskaya et al., 1995), while, more recently, the late lytic cycle associated EBV protein BDLF3 (recombinant EBV probable membrane antigen GP85) was shown to downregulate HLA class I and class II, CD54 (ICAM-1, important for cell trafficking and adhesion) and CD71 (transferrin receptor, necessary for iron homeostasis) (Quinn et al., 2015). EBV-derived IL-10 has been shown to induce pro-inflammatory polarization in human monocytes by STAT3 (signal transducer and activator of transcription 3) downregulation (Jog et al., 2018) and is more efficient than human IL-10 at inducing B-cell proliferation along with a high density of IL-10R1 expression on the cell surface (Yoon et al., 2012). The Cancer Genome Atlas Research Network published a comprehensive molecular analysis of gastric cancer (GC) samples to identify possible mutational signatures and found that an EBV-associated gene expression profile, particularly in tumor tissue isolated from the gastric fundus (upper part of the stomach) in line with a DNA hypermethylation pattern, formed a distinct clinical subtype of GC (Cancer Genome Atlas Research, 2014).
Merkel cell polyomavirus (MCPyV) is associated with most cases of Merkel cell carcinoma (MCC), considered an aggressive, though relatively rare, neuroendocrine skin cancer (DeCaprio, 2017; Miller et al., 2018). Interestingly, both MCC “virus-positive” or “virus-negative” are very immunogenic and elicit not only a CD8+ and CD4+ T-cell response (Iyer et al., 2011), but also a B-cell response against MCPyV T-antigen proteins (Paulson et al., 2010, 2017). A striking feature concerning the MCPyV is the fact that it can cause a lifelong, but relatively innocuous infection, even in people at an early age (DeCaprio, 2017).
Merkel cell polyomavirus has two transcriptional units coding four splices mRNAs encoding four proteins, which includes a large T-antigen (LT), 57kT, small T-antigen (ST) and ALTO, and also two viral coat proteins (VP1 and VP2) (Carter et al., 2013; Theiss et al., 2015). It is described that approximately 80% of all MCC contains clonally integrated copies of these virus, leading in mutations resulting in LT truncation with deletion of its DNA binding and helicase domains and 57 kT growth suppressing domain at the carboxyl-terminus loss (Shuda et al., 2008; Li et al., 2013). Typically, virus-positive MCC tumors express ST and truncated LT, supporting their role in the initiation and maintenance of MCC. Besides the presence of viral DNA and protein expression, tumor genomes are significantly different between virus-positive and virus-negative MCC. Indeed, “virus-positive” MCC contains few somatic mutations and copy number alterations. On the other hand, “virus-negative” MCC have a high frequency of DNA mutations, since these MCC are usually associated to UV damage (Wong et al., 2015; DeCaprio, 2017; Starrett et al., 2017). Another difference is that most “virus-positive” MCC still contains a non-mutated retinoblastoma suppressor gene (RB1), contrarily to “virus-negative” MCC, in which RB1 is usually mutated (Harms et al., 2016). The protein coded by this gene can restrict cell-cycle progression by inhibiting the entrance to S phase due to E2F family repression. Therefore, MCPyV MCC will fail to stop in G1/S and increase the proliferation rate of such cells (Borchert et al., 2014; DeCaprio, 2017).
There is a close relationship between MCC and other malignancies associated to immune cells, such as chronic lymphatic leukemia, multiple myeloma or non-Hodgkin lymphoma. Indeed there is an increased incidence of MCC in patients that also have one of the diseases mentioned above (Tadmor et al., 2011), probably due to the compromised immune responses in lymphoid malignancies. Indeed, these patients will probably have a higher propensity for colonization with MCPyV, which would then increase the incidence of MCC. Besides, patients with lymphoid malignancies are also frequently subjected to T-cell-suppressive therapies, that would influence MCPyV infection and the course of MCC (Tadmor et al., 2011; Brewer et al., 2012; Triozzi and Fernandez, 2013).
Various human papilloma virus (HPV) genotypes are generally associated with oncogenesis, although HPV-16 and -18 are implicated in the pathogenesis of several cancers in addition to cervical cancer (also HPV-31, -33, -35, -39, -45, -51, -52, -56, -58, -59), such as cancers of the oral cavity, oropharynx, and tonsils (Cogliano et al., 2011; Bansal et al., 2016). HPV-38, which is associated with skin cancer (Kocjan et al., 2009), interacts with the eukaryotic elongation factor 1 A (eEF1A) via the viral E7 protein to remodel actin fibers in the cytoskeleton and thereafter promoting aberrant cell proliferation, as shown in human keratinocytes (Yue et al., 2011). The double methylation of eEF1A Lys55 by the human methyltransferase-like 13 (METTL13) protein was recently found to be a crucial facilitator of mutant KRAS-driven oncogenesis in the pancreas and lungs (Liu et al., 2019). METTL13, which is also annotated as FEAT has anti-apoptotic functions (Liang et al., 2015) and has been linked to cirrhotic lesions in the human liver adjacent to hepatocellular carcinoma (HCC) tissue, suggesting a role in inflammation (Takahashi et al., 2011). The only approved vaccine against HPV-associated cervical cancer, Gardasil®, targets the L1 protein from HPV-6, -16, -11, and -18 and offers remarkable immunological protection of up to 5 years against HPV-6/-11-driven genital warts, as well as HPV-16/-18-linked cervical intraepithelial neoplasia grade 2 (CIN-2+) lesions (Harper et al., 2010). Patients with HPV+ head and neck cancer have been shown to have a higher number of PD-1+ CD8+ tumor infiltrative lymphocytes (TIL) infiltrating their tumors, compared to HPV-naïve patients further to experiencing better clinical outcomes following standard therapy (Kansy et al., 2017). In a clinical study testing TIL efficacy against HPV+ cervical cancer, nine patients with metastatic cervical cancer were given TIL, which in four of the patients had specific T cell recognizing HPV E6 and E7 peptides that were also detected post-infusion. Two patients who exhibited complete responses lasting between 15 and 22 months after T-cell therapy showed shared CD4+ T-cell reactivity to HPV E75–19 (the first patient also has CD4+ T-cell responses to two other E7 peptides, including E79–19) (Stevanovic et al., 2015), indicating the potential of HPV-specific T cells in mediating clinically meaningful responses in HPV malignancies. In another study, thirty patients with vulvar intraepithelial neoplasia infected with HPV-16 who were treated with long peptides from the HPV-16 E6 and E7 proteins (two patients received three vaccinations, while the remaining 28 patients were given four vaccinations) showed very favorable clinical results (9/30 patients with complete response at 12 months post-vaccination, which last a further 12 months – thus a total of 24 months) (Kenter et al., 2009). A cervical cancer-derived CD8+ T cell specific for an HLA-A2-restricted HPV-16 E6 epitope has also been shown to be enriched in the tumor compared with peripheral blood (Draper et al., 2015). Furthermore, the corresponding TCR was transduced into naïve T cells and tested for anti-tumor activity against several HPV+ cancer cell lines, resulting in target-cell elimination. These clinically relevant results are indicative of the potential anti-tumor functionality of HPV-specific T cells either directly or by means of modulating the local immunological milieu by molecular mimicry to potentiate disease control.
A link between immune evasion in cervical cancer cells, perpetrated by HPV, has been shown in the context of HLA class II molecules. The effect of IFN-γ on HPC+ cervical cancer cells has been shown to mobilize class II-associated invariant chain peptide (CLIP) and HLA-DMA into the cytoplasm while HLA-DMA dynamics (HLA-DM being a “molecular editor” for loading peptides into the MHC class II binding cleft) was not affected, although HLA-DR expression at the cell surface was increased (Zehbe et al., 2005). In line with this, IFN-γ treatment of HPV+ ME180 cervical cancer cells promoted immune recognition by a HLA-DR4-restricted CD4+ T-cell clone CCA1 (which is specific for a peptide derived from the HPV E7 protein) (Zehbe et al., 2005). Nevertheless, CLIP expression was not affected by IFN-γ treatment, hinting at alternate strategies by which HLA class II immune surveillance may be increased in HPV-associated malignancies.
The involvement of hepatitis B and C viruses (HBV/HCV) in the pathogenesis of HCC is also well-established. A combination of natural selection of antagonistic HLA class I viral epitopes and upregulation of PD-1 on infected hepatocytes contributes to immune evasion of HBC/HCV in the liver (Bertoletti et al., 1994; Cox et al., 2005; Golden-Mason et al., 2007). Virus-mediated counter-regulation of cholesterol metabolism in host cells by manipulating the subtilisin Kexin Isozyme-1 (SKI-1)/Site-1 Protease (SKI-1/S1P) pathway has been implicated in the establishment of a successful infection (Olmstead et al., 2012). This was further strengthened by the finding that pharmacological inhibition of the SKI-1/S1P pathway limited viral particle generation by reducing the amount of intracellular lipid droplet formation. A similar scenario has been observed in the infection of liver cells with dengue virus, another highly clinically relevant flavivirus, and it’s blockade with PF-429242, specific inhibitor of the SKI-1/S1P pathway (Hyrina et al., 2017). The HBV-encoded HBx protein has been previously shown to interact with the afore-mentioned eEF1A, which is a downstream target of the METTL13/FEAT enzyme and participates in remodeling of actin filaments in hepatoma cells by blocking eEF1A dimerization (Lin et al., 2012). Anti-PD-1 therapy with nivolumab induced strong anti-viral activity to the effect of reducing viral load by approximately 4-log, with the viral load reduced to undetectable levels in two patients (Gardiner et al., 2013). Similarly, anti-CTLA-4 blockade (tremelimumab) in patients with HCC and chronic HCV infection extended the time to disease progression, with almost 60 patients experiencing stable disease and in addition to dramatic reduction in HCV load (Sangro et al., 2013). IFN-γ-producing T cells, in response to HCV antigen exposure, were also detected in peripheral blood of some patients up to 300 days post tremelimumab treatment initiation (after at least three cycles had been completed). Apoptosis-inducing cytotoxic T cells with engineered with anti-HCV TCRs (directed against the non-structural proteins NS3 and NS5) as well as those targeting HBV are being developed (Balasiddaiah et al., 2017; Bertoletti et al., 2017; Kah et al., 2017).
Kaposi’s sarcoma herpes virus (KSHV), presently known as human herpesvirus-8 (HPV-8), is the etiological agent of Kaposi’s sarcoma, a form of endothelial cancer which can manifest in mucosal tissue surfaces, i.e., lymph nodes and skin as well as two other lymphoproliferative disorders, namely multicentric Castleman’s disease (MCD) and primary effusion lymphoma (PEL) (Robey et al., 2011). Kaposi’s sarcoma (KS) was, in the 1980s and early 1990s, identified as a major co-morbidity of HIV/AIDS-related immunosuppression (Chang et al., 1994; Strickler et al., 1999). KSHV can dampen innate immune responses via several strategies (reviewed in Lee et al., 2010), simultaneously producing viral interleukin (IL) 6 which promotes the pathogenesis of MCD and PEL (Sakakibara and Tosato, 2011). Importantly, latent KSHV has been reported to upregulate host IL-6 production (by infected cells) triggered by the KSHV latency-associated nuclear antigen (LANA), hinting at an ongoing inflammatory response despite absence of clinical disease as well as in MCD and PEL (An et al., 2002). Central memory T-cell responses (IFN-γ) to the virus has been observed to the crucial even in the presence of sirolimus therapy (rapamycin, immunosuppressant) following renal transplantation (Barozzi et al., 2008). In a recent clinical study investigating the therapeutic efficacy of anti-PD-1 (nivolumab or pembrolizumab, anti-PD-1 antibodies) blockade in HIV-associated KS, six out of nine patients who received the treatment showed objective responses (five patients = partial responses; one patient = complete remission) (Galanina et al., 2018). Interestingly, some of the patients who responded to anti-PD-1 therapy (n = 4) displayed low PD-L1 levels in their tumors, coupled with low CD4 counts and high HIV burden.
Although not related to cancer, influenza vaccine-mediated narcolepsy development in susceptible individuals presents a very clinically relevant human modality pertinent to antigen cross-reactivity influenced by the restricting HLA elements in an individual (Mignot et al., 2001; De la Herran-Arita et al., 2013; Tafti et al., 2016; Bomfim et al., 2017). HLA-DQB1∗0602 confers protective immune responses against developing type 1 diabetes mellitus (T1DM), while the same individuals suffer a greater risk of contracting narcolepsy (Mignot et al., 1997, 2001; Siebold et al., 2004). Similarly, some HLA class I alleles, i.e., HLA-A11:01, HLA-C04:01, and HLA-B35:01, are implicated in the susceptibility of certain groups of individuals to develop narcolepsy (Tafti et al., 2016). HLA-DQB1∗0602 in association with HLA-DRB1∗1501/02/03 present a propensity to induce development of Th17 and Th1 cells, which is necessary for the control of bacterial infections at very early stages, but not later due to the effect it has on culminating in autoimmune pathology (more reflective of Th17 cells) (Mangalam et al., 2013). Asian populations have a higher frequency of the HLA-DQB1∗0602 allele, while West African have at least twice as more polymorphisms in the HLA class II allele pools compared to White Caucasians, which may have evolved to provide superior protection against the former populations against infections agents such as Mycobacterium tuberculosis, Klebsiella sp., Leishmania sp. (Mangalam et al., 2013). In contrast, HLA-DQB∗0601 is associated with resistance to developing autoimmune disease, based on studies in mice with experimental autoimmune encephalomyelitis (Mangalam et al., 2008), the murine model of multiple sclerosis (MS).
Bacterial Pathogens, Inflammation, and Oncogenesis
Helicobacter pylori is perhaps the best described and most known oncogenic bacterial pathogen in relation to gastric cancer (GC) thus classified as a group I carcinogen (Wroblewski et al., 2010). Proteins produced by H. pylori, comprising the vac and cag families, induce molecular changes in stomach epithelial cells leading to malignant transformation (Wroblewski et al., 2010). Bacterial urease, on the other hand, promotes apoptosis of epithelial cells by direct binding to the HLA class II molecules (Fan et al., 2000). Downregulation of HLA class II molecules by H. pylori strain N6 via enhanced expression of CD300E and miRNA-4270 suppression in human macrophages was recently found to be an immune evasion strategy employed by this pathogen (Pagliari et al., 2017). H. pylori VacA (from strain CCUG17874) was previously shown to interfere with the synthesis and presentation of nascent HLA class II molecules (Molinari et al., 1998), as well as the interaction between B7-H2 and CD28 on CD4+ T cell to trigger immune activation marked by early IL-17 production to control infection (Lina et al., 2014). Thus, deterring CD4+ T-cell responses appears to play an important role in H. pylori-mediated immune evasion, but the same effect may play potentiate anti-cancer responses. An interesting example being the observation of IFN-γ+ CD4+ T cells responding to HLA-DRB1∗1501-restricted H. pylori hemagglutinin (HpaA88–100) in H. pylori-infected patients with GC who exhibited less severe disease (Chen et al., 2013).
The local immune responses in the stomach to H. pylori has also been shown to be strain-dependent, augmenting the release of a panel of pro-inflammatory cytokines, such as IL-6, TNF-α, IL-12, and IL-1β (Andres et al., 2011). Also, the suppression of microRNA Let-7b expression in gastric cells by H. pylori infection promotes TLR4 expression and downstream Myd88 activation to trigger NF-κB-mediated immune responses (Teng et al., 2013). The finding that H. pylori infection leads to upregulation of vitamin D receptor (VDR) expression in gastric epithelial cells and extracellular supply of vitamin D3 is able to improve intracellular bacterial killing (Guo et al., 2014) indicates that a subpopulation of patients may be able to control bacterial infection via vitamin D-associated mechanisms. A recent review of clinical trial data suggests that vitamin D may have a protective effect in cancer although further controlled real-world evidence is required to strengthen this stance (Grant, 2018).
Escherichia coli-derived colibactin and the risk of colon/colorectal cancer (CRC) has been proposed due to the DNA alkylating nature of the bacterial toxin. The strain of E. coli which produces colibactin is prevalent in the human gut, but not everyone with colibactin + E. coli develops malignancies. An earlier preclinical study showed that the colibactin gene is encoded in the pks genomic island of certain E. coli strains, which have also been recovered from some patients with CRC (Dalmasso et al., 2014). Furthermore, using cell culture and a mouse model of human CRC, pks + E. coli negatively affects p53 SUMOylation and, thereafter, the structural integrity of the p53 protein leading to DNA breaks. Infection of cells (as well as mice) with pks + E. coli also induced the production of growth factors, i.e., HGF, FGF, and GM-CSF associated with tumor outgrowth and poor outcome. Enteropathogenic E. coli (EPEC) which produces Shiga toxin, the causative agent of haemolytic uraemic syndrome (HUS), may also be involved in the pathogenesis of CRC based on the findings of a clinical study published in 2015 (Magdy et al., 2015). Citrobacter rodentium infection of the mouse colon, which represents similar pathological features to EPEC infection and intestinal inflammation in humans, has been shown to promote Th17 induction with the involvement of the resistin-like molecule alpha (RELMα) (Osborne et al., 2013).
In their meta-analysis of 22 clinical studies, Zhu and colleagues further strengthened the existing link between C. trachomatis infection and the occurrence of cervical cancer (Paavonen, 2001), while identifying co-infection with HPV to promote a higher risk of developing malignant disease. A 2019 clinical study showed that women with serum antibodies against the Pgp3 protein of C. trachomatis suffer a twofold risk of developing ovarian cancer (Trabert et al., 2019). Pgp3 is a plasmid-encoded virulence factor which can form a complex with human cathelicidin (also known as LL-37) to induce IL-6 and IL-8 production in neutrophils (Hou et al., 2019). Mechanistic studies using cell culture systems have provided empirical proof that infection with C. trachomatis induces DNA double-strand breaks (DSBs) in cells while impairing the repair process by inhibiting recruitment of ataxia telangiectasia mutated kinases (ATM) and p53-binding protein 1 (53BP1), further to inducing production of the epidermal growth factor (EGF), which is implicated in dysplasia (Chumduri et al., 2013; Patel et al., 2014).
To date, no direct link has been established between Mycobacterium tuberculosis (Mtb) infection and oncogenesis in the lungs. However, some existing clinical and translational evidence suggest that Mtb infection-induced fibrotic lesions in the lungs may contribute to malignant transformation, partly due to genetic aberrations caused by the local inflammatory response comprising an armament of IFN-γ, TNF-α, IL-12, IL-18, IL-17, and IL-6 to clear the pathogen (Kaufmann et al., 2014; Zumla et al., 2015; Nikitina et al., 2018). Mutations in the epidermal growth factor receptor (EGFR) gene, associated with lung adenocarcinoma pathogenesis, have been linked to the presence of old pulmonary tuberculosis (TB) lesions in patients with lung cancer (Luo et al., 2012). In agreement with this, the presence of old pulmonary TB lesions has been shown to be an independent predictor of shortened survival among patients with squamous cell carcinoma of the lung (SCC) (Zhou et al., 2013). A 2006 report showed that 25% of patients in a New York City study cohort with an underlying cancer diagnosis, i.e., lung cancer, Hodgkin’s and NHL, head and neck cancer or leukemia, died within three months of being diagnosed with pulmonary TB (Kamboj and Sepkowitz, 2006). Taiwanese patients with various solid cancers (particularly lung cancer) and TB, compared to those who did not have cancer, exhibited a significantly lower anti-TB treatment success rate (43 vs. 75.8%) and almost four times the mortality rate (48.4 vs. 13.9%) (Chiang et al., 2009). Mtb infection has been shown to induce DSBs in host macrophages, reminiscent of pre-apoptotic DNA fragmentation (Castro-Garza et al., 2018), hinting at an added mechanism of promoting malignant transformation of host cells.
Some bacterial pathogens, such as Salmonella, Klebsiella, and Yersinia sp., which are etiological agents of enteric infection, have been associated with downregulation of the HLA-B27 allele in PBMCs of infected patients (Kirveskari et al., 1999). These pathogens have also been associated with the pathogenesis of ankylosing spondylitis (AS), particularly in HLA-B27+ individuals (Martínez et al., 2004). HLA-B27 expression is a risk factor for AS and patients with AS have an increased risk for developing hematological malignancies, colon, bone as well as prostate cancer (Chang C.C. et al., 2017). An association between HLA-B27 and immune responses against ovarian cancer – associated antigens has been reported (Szender et al., 2016). Taking these into consideration, it would be clinically relevant to investigate the relationship between bacterial infection at mucosal surfaces, local immune responses therein and the modulation of HLA alleles in relation to neoplasia. Although beyond the scope of this paper, a recent genome-wide association study (GWAS) in more than 200,000 individuals of European ancestry provided evidence of susceptibility loci in the HLA class I and class II alleles (Tian et al., 2017) to various infections (bacterial, viral, and fungal), thus paving the way for future high-throughput large-scale studies to better understand the fine details of infection and immunomodulation.
Helminths in the Inflammation-Induced Oncogenesis
Besides the role of viral and bacterial pathogens as well as the microbiome discussed above, several helminth infections – mainly affecting the developing world – are an important cause of infection-induced inflammation and oncogenesis. Schistosoma haematobium, commonly known as the urinary blood fluke, is a parasitic flatworm prevalent in Sub-Saharan Africa and the Middle East and recognized by the IACR as a human carcinogen (Cogliano et al., 2011). Eggs deposited in the bladder wall has been shown to induce chronic inflammation following long-term interaction with the host’s immune cells (Ishida and Hsieh, 2018). Furthermore, S. haematobium-induced inflammation can result in KRAS mutations leading to oncogenic transformation of bladder cells (Botelho et al., 2013), while parasite-derived antigen preparations can directly induce inflammation leading to dysplasia in otherwise normal and healthy mice (Botelho et al., 2011). Similarly, the Southeast Asian liver fluke Opisthorchis viverrini and Chinese blood fluke Clonorchis sinensis have also been shown to perpetrate liver and bile duct cancer by means of establishing chronic inflammation (Sripa et al., 2007, 2012; Kim et al., 2016). O. viverrini also secretes an important mitogenic factor, namely granulin-like Ov-GRN-1, which promotes aberrant growth of host cells in the bile duct further to activating Myd88-dependent NF-κB-driven inflammation (Sripa et al., 2012). During the chronic phase of C. sinensis infection, a disrupted balance between pro- and anti-inflammatory immune responses (Th1 suppression, Th2 increase) leads to DNA damage and neoplastic transformation of host cells (Kim et al., 2016). As such, the immunological axes involved in helminth-induced oncogenesis are akin to those observed in cancers with bacterial and viral pathogens.
The Microbiome in Inflammation-Induced Neoplasia
The role of the microbiome, especially in the gut, play a quintessential role in maintaining physiological balance and homeostasis in health and disease (Roy and Trinchieri, 2017). Metabolites derived from gut bacteria, such as butyrate, are essential for maintaining gut barrier function (Zheng et al., 2017), which is necessary for deterring unwanted inflammation. The gut microbiota is also directly involved in drug metabolism and control of drug-associated toxicity (Zimmermann et al., 2019). Dysbiosis of the lung microbiota is implicated in the development of autoimmune pathology (O’Dwyer et al., 2016), while immune activation of gut fungal microbiota-specific T cells can influence and alter immune responses in the lung (Bacher et al., 2019). Among the constituents of the microbiome which often emerge in clinical studies are Bacteroides and Prevotella species which, in addition to the gut, are also members of the lung microbiome (Mathieu et al., 2018b; Pragman et al., 2018). The former has been associated with desirable clinical outcomes in patients with metastatic melanoma who undergo anti-CTLA-4 therapy (Chaput et al., 2017). Translational and clinical studies have linked an abundance of Prevotella sp. in the gut to improved protection to influenza infection (Lee et al., 2019), clinical TB (Maji et al., 2018), and general immunological homeostasis in the airways (Huffnagle et al., 2017), thus providing a link between this commensal and pulmonary immune equilibrium. Prevotella abundance has been reported in patients with ulcerative colitis (UC) who are also smokers (Pascal et al., 2017), in agreement with a previous study which also found an abundance of Bacteroides (Lucke et al., 2006). In contrast, pediatric patients with new-onset Crohn disease (CD) (thus, treatment-naive) do not have a link to Prevotella in the gut microbiota but, however, display a reduced abundance of Bacteroidales (Gevers et al., 2017). It is also relevant to refer to another recent finding of a megaphage that specifically targets Prevotella species in the human gut (Devoto et al., 2019), warranting further investigation in relation to induction of intestinal inflammation and neoplasia.
Mechanistic studies performed in preclinical models have shed light on some hitherto unknown immune-related mechanisms driving – potentially also contributing to neoplastic transformation of tissue – with a link to the local microbiota. IL-17B production by APCs following exposure to bacterial outer membrane vesicles (OMVs) has recently been shown to enhance the development pathogenesis of bleomycin-induced pulmonary fibrosis (PF) (Yang et al., 2019). Most strikingly, the OMV which appeared to be most prominently promoting fibrotic transformation in the alveoli of mice derived from Bacteroides and Prevotella species. The lung microbiome in mice – but not including Bacteroides and Prevotella – were shown to augment IL-1β and IL-23-driven (also involved in IL-17 production) T-cell pathology which promoted mutant KRAS and p53 lung adenocarcinoma development (Jin et al., 2019). This pathway was eventually mapped to the activation of Vγ6Vδ1+ T cells and their local production of IL-17 perpetrating malignant transformation. In another study that investigated the role of TCR γδ T cells in celiac disease, a specific population of intraepithelial lymphocytes (IEL) comprising cytolytic Vγ4Vδ1+ T cells recognizing butyrophilin (BTLN) 8/3 were shown to be decreased following gluten-induced inflammation concomitant with BTLN8 downregulation (Mayassi et al., 2019). Instead, a new subset of Vδ1+ T cells, which did not recognize BTLN8/3, occupied the same IEL niche. Provision of a gluten-free diet allowed for recuperation of BTLN8 expression, although the host-protective Vγ4Vδ1+ IEL populations could not be rejuvenated. Celiac disease has been linked to predisposing patients to a higher risk of developing esophageal cancer (Han et al., 2015), thus making it a relevant model to study early events in inflammation-induced neoplasia.
Commensal bacteria which trigger IL-17 and IL-22 production in the gut may in fact do so via Mincle-Syk kinase and C-type lectin 4e (Clec4e) signaling in dendritic cells (DCs), as recently shown in murine Peyer’s patches (Martínez-López et al., 2019). The signaling pathway, in response to sensing of microbiome-associated molecular patterns, produce IL-6 and IL-23p19, which in turn promote the development of Th17 and the production of IL-22 from type 3 innate lymphoid cells (ILC3) in the intestines. Aberrations in the Mincle-Syk pathway led to reduced gut barrier integrity, bacterial translocation to the liver and promotion of inflammation, in addition to compromised production of IgA by resident plasma cells. Adhesion-mediated endocytic uptake of antigenic cargo derived from the cell wall components of commensal gut bacteria by intestinal epithelial cells (IECs) appears to induce Th17 development in mice, requiring the activity of cell division control protein 42 homolog (CDC42) (Ladinsky et al., 2019). Thus, this system postulates one important mechanism by which microbiota-directed T-cell responses are primed in the gut and how this may play an induction of deleterious inflammation in disease.
Elevated levels of CD4+ T cells which produce IL-17 (Th17 subset) in peripheral blood of patients with type 2 diabetes mellitus (T2DM) has been recognized as a major contributor to chronic inflammation in these individuals (Jagannathan-Bogdan et al., 2011). Importantly, perturbation in the gut microbiome is very intimately linked to DM development (Harsch and Konturek, 2018), thus making the IL-17 axis a very relevant accomplice in this regard. More recently, a meta-analysis of 121 cohorts comprising 20 million individuals from across the globe confirmed that DM predisposes patients to developing various cancers, generally putting women more at risk than men (Ohkuma et al., 2018). A clinical study reported in 2016 showed that patients with T1DM and chronic periodontitis (oral bacterial infection) carry polymorphisms in the IL17A gene perpetrating exaggerated cytokine production and pathology (Borilova Linhartova et al., 2016). Patients with T2DM and pulmonary TB also exhibit high levels of serum IL-17A, in addition to TNF-α and IFN-γ, concomitant with disease severity in the lungs and reduced functionality of CD8+ cytotoxic T cells and NK cells (Kumar et al., 2013, 2015). These findings hint at a role for IL-17A in predisposing individuals with DM, particularly those harboring infections which trigger IL-17 responses, to neoplastic transformation.
Another interesting point was raised by Yoshimoto et al. (2013), that an obesity-associated distinct microbiome appears to be associated with higher incidence of liver cancer; the increase in deoxycholic acid (DCA) – associated to microbiome alterations, as a result of obesity – may provoke a senescence-associated secretory phenotype (SASP) in hepatic stellate cells, and subsequently increase the production of inflammatory molecules and tumor-promoting factors in the liver associated with an increase in HCC development after exposure to chemical carcinogens (Yoshimoto et al., 2013). Another study showed evidence of SASP signs in hepatic stellate cells in non-alcoholic steatohepatitis (Sun and Karin, 2012), demonstrating that a broad array of different factors may affect the microbiome and its complex impact on harmful or protective immune responses. Preferential colonization of mucosal tissues by certain microbiome-associated bacterial species – influenced by infections, drug intake, dietary changes and other lifestyle practices, e.g., smoking, breastfeeding (Victora et al., 2016) – can trigger unexpected immunological programs which can either promote neoplastic disease or offer protection. The microbiome does not only impact on the clinical outcome of patients with cancer treated with checkpoint inhibitors, yet also in long term survival of patients with pancreatic cancer. A high tumor microbiome diversity appears to be associated with immune activation; even a distinct intratumoral microbiome signature, defined by Pseudoxanthomonas, Streptomyces, Saccaropolyspora, and Bacillus clausii, has been shown to be associated with increased survival in patients with pancreatic cancer (Riquelme et al., 2019). In contrast to these beneficial microbiome signatures, bacterial or fungal species may drive harmful inflammation: Saccaropolyspora rectivirgula drives in general a pro-inflammatory environment, including hypersensitivity pneumonitis (Kim et al., 2010): the mycobiome (i.e., fungal commensals or pathogens) impacts on PDAC development mediated via (intratumoral) Malassezia that drives PDAC progression via complement activated inflammation (Aykut et al., 2019).
Among the currently discussed strategies to manipulate the microbiota, particularly in the gut and possibly also in the lung, is the use of CRISPR technology as well as the use of bacteriophages to select for preferential colonization of tissue with bacteria, fungi, perhaps in conjunction with certain bacteriophages, to promote host-beneficial immuno-physiology (Lee et al., 2018). Another use of CRISPR has also been to study the horizontal gene transfer between bacterial species comprising the microbiota and potentially pathogenic genetic islands that can perpetrate strong inflammatory responses and tissue transformation (Munck et al., 2018).
Molecular Mimicry of Pathogen- and Host-Derived Targets: Implications in Anti-Cancer Immune Responses
Early work using T-cell lines specific for the melanoma-associated antigen Melan-A/MART-127–35 HLA-A2 epitope (AAGIGILTV) showed cross-reactivity with an E. coli methionine synthase-derived peptide (AAGIGIIQI) and a peptide from the HSV-1 glycoprotein C (GIGIGVLAA), eliciting lysis and IL-2 production (Loftus et al., 1996; Carrabba et al., 2003). The binding of Mtb-derived epitopes with mimicry to host peptides to common HLA class I and class II molecules has also been shown using bioinformatic analysis (Chodisetti et al., 2012). This concept has now been reawakened in line with clinical biomarkers and immunotherapy in cancer, with a seminal article dissecting the immunological characteristics of long-term survivors of pancreatic cancer in whom polyclonal, neoantigen-specific CD8+ T-cell responses along with a high load of neoantigens emerged as the quintessential determinant of protection against disease progression (Balachandran et al., 2017). The authors also incorporated data linking the high quality of neoantigen-directed TCRs – which had not been negatively selected during disease pathogenesis due to their cross reactivity to microbial peptides (Balachandran et al., 2017). These cross-reactive T cells were found in blood and tumor tissue. This appears to be biologically (and immunologically) plausible, as it is in the host’s interest to fight pathogens and, therefore, not to remove these TCRs from the memory pool.
Another study, based on mathematical modeling of neoantigen characteristics and mimicry of pathogen-derived targets, showed that increased dissimilarity of neoantigens to (non mutant) target sequences increased the possibility to produce meaningful immuno-protective responses after immune checkpoint blockade therapy – designated as “neoantigen fitness” – further to binding to TCRs which also recognize infectious disease-related targets (Luksza et al., 2017). Thus, the concept of molecular mimicry which allows for pathogen- and host-reactive T cells to elicit immune responses may be crucial in mediating clinically relevant responses in cancer as well as infectious diseases.
Infection, Inflammation, and Neoplasia: Implications for Personalized Immunotherapy
Targeting the immune system at early stages of infection may be detrimental, as the developing anti-pathogen response mounted by the host is crucial for augmenting innate and adaptive immune responses to control the pathogen’s replication as well as to form long-term immunological memory. The window of opportunity for modulating the host immune responses lies in clinically intervening when the inflammatory milieu becomes overt and perpetrates unnecessary tissue pathology – potentially leading to oncogenesis. Particularly, this time point is almost impossible to capture in humans, with some exceptions, as tissue transformation in patients with H. pylori (Dore et al., 2018) or EBV infection (post-transplantation) (Shannon-Lowe et al., 2017), which can be monitored more closely using appropriate diagnostics and immunological testing, respectively. Primarily, the timing of intervention herein is of utmost importance. For instance, in patients with drug-sensitive pulmonary TB who undergo the standard 6-month antibiotic therapy, IL-6 overproduction at diagnosis (serum levels) can be predictive of individuals who will sustain severe lung damage at the end of treatment – albeit successfully clearing the pathogen from the lungs (Nagu, 2017). In contrast, patients who produce too little IL-6 are more likely to succumb to TB and die within the first 2 months post-diagnosis and after initiating treatment. Thus, and as previously shown, IL-6 is necessary to control Mtb infection in patients, but overt circulating amounts of the cytokine may be deleterious and can, therefore, be considered for therapeutic targeting. Therefore, aberrant inflammatory processes which do not result in optimal clinical outcomes offer the ideal Achilles heel for intervention. Along these lines, platforms such as spatial transcriptomics (ST) (Berglund et al., 2018) and immunohistology-based mathematical modeling (hyperspectral cell sociology) (Enfield et al., 2019) can be useful in determining areas in diseased tissue where inflammation occurs and how this affects neoplastic transformation at the gene expression level even before pathological features and clinical symptoms can be observed.
Another important fact is the existence of microbiome variations due to geographical and ethnical differences, which should also be considered in personalized therapy. Indeed, there are several studies reporting significant variations in microbiome composition in healthy individuals from different ethnicities (Nasidze et al., 2009; Nam et al., 2011; Yap et al., 2011; Yatsunenko et al., 2012; Gupta et al., 2017). These differences may also predispose individuals for some malignancies, such as inflammatory bowel disease (IBD), or increase the risk of a viral infection with oncogenic potential. An example is association between EBV and NPC, which is endemic in southern Chinese population, with special incidence in individuals of Cantonese origin. Most likely, genetic susceptibility and environmental factors, such as the consumption of dietary nitrosamines, play a role in EBV and NPC incidence (Tsao et al., 2017).
Using Immunological Effectors as Host-Directed Therapeutics
Recent evaluation of human T-cell responses to opportunistic pathogens has also revealed some interesting insights into immune priming and the risk of tissue pathology. Bacher et al. (2019) showed that CD4+ T cells which produce in response to Candida albicans, a commensal fungus which can cause opportunistic infections, readily cross-react with at least 30 other fungal species, based on assessment of peripheral blood-derived T-cell responses. Importantly, IL-17+ CD4+ T cells which recognize C. albicans antigens and cross-react with Aspergillus fumigatus, were identified to be specifically expanded in tissue samples from patients with airway disease (chronic obstructive pulmonary disease, asthma, and cystic fibrosis). The same cell subset was found to be increased in blood from patients with Crohn’s disease, indicating their potential to travel to the lung and promote pulmonary inflammation. Effector memory CD4+ T cells which arise early in development and produce tumor necrosis factor (TNF-α) have also been shown to promote intestinal tissue growth, which is compromised in prematurely born infants (Schreurs et al., 2019).
For instance, influenza-specific tissue-resident memory (TRM) CD8+ T cells are indispensable for protection to full-fledged flu-associated pathology in the lung (Pizzolla et al., 2018). TRM cells are likely to be established in non-lymphoid tissue compartments and not even detected in blood, meaning that the highly specialized TCR repertoire in tissue differs from that seen in peripheral circulation. Indeed, TIL from human cancer tissue have an arsenal of TCRs which are specific for viral antigens, i.e., influenza CMV and EBV, but also react to cancer neoantigens (Simoni et al., 2018; Scheper et al., 2019). Thus, there is a high chance that a ‘pre-wired’ matrix of the tissue-resident TCR repertoire might participate in orchestrating pathology and disease due to molecular mimicry between immunogenic epitopes of microbes and host-associated antigens. One preclinical study showed that influenza-primed T-cell responses were able to recognize the overexpression of host proteins by the 3LL lung tumor cell line and confer protection against lung cancer development in a murine model (Iheagwara et al., 2014). TRM CD8+ T-cells have been shown to express a similar gene program as compared to TIL (Milner et al., 2017). These TRMs, characterized by a specific transcription factors (Hobbit, Blimp-1, Notch, Runx3) (Milner et al., 2017) are believed to reside in non-lymphoid tissues in order to be able to rapidly respond to infectious pathogens – or to cancer (Kumar et al., 2017) TRMs require mitochondria dependent lipid oxidation (Pan et al., 2017), a pathway that is not properly functioning in chronic inflammation, chronic infection as well as in cancer lesions, due to the immune-suppressive environment and a “frozen,” non-functional chromatin state (Philip et al., 2017), that appears to be at least in part be reversible (Li et al., 2019). The transcription factor Bhlhe40 maintains immune fitness in TRMs and in TIL, where a subpopulation of stem-cell like TCF1+ TIL have been shown to be responsive to checkpoint inhibition – and may indeed by responsible for clonal expansion in response to pathogens or cancer cells. Of note, checkpoint inhibitors are thought to revert dysfunctional in situ T-cell responses leading to clinically relevant anti-tumor responses (Pauken et al., 2016). However, clinically relevant immune responses were associated with recruitment of new T-cell clones accumulating into cancer lesions, since pre-existing (exhausted) in situ T-cell clones could not be reverted by checkpoint inhibitors, most likely due to “fixed” epigenetic imprints (Kurtulus et al., 2019; Yost et al., 2019). A “fixed” epigenetic landscape appears also to be associated with T-cell phenotypes (Ghoneim et al., 2017) that can be manipulated by affecting the transcriptional regulator Tcf7/Tcf1 (Kurtulus et al., 2019) or Bhlhe40 (Mehta et al., 2017) promoting and maintaining TRMs and tumor-infiltrating immune cells by increased mitochondrial metabolism (Li et al., 2019), a situation that can be also be achieved using the genetic modifier HDAC (histone deacetylase inhibitor tubastatin A) or fatty acid acetate by affecting (Bhlhe40-negative) T-cells leading to increased IFN-γ production (Li et al., 2019).
The pathogenesis of IBDs, such as CD and UC, have also been attributed to arise from dysregulated host responses to microbial products, i.e., increased prevalence of gut microbiota-derived sphingolipids such as ceramide and induction of pro-inflammatory responses locally (Bryan et al., 2016; Franzosa et al., 2019). Bacterial strains from the gut microbiota of a patient with UC have also been shown to induce Th17 cells in germ-free mice following oral inoculation (Atarashi et al., 2015). As IBDs pose a high risk for CRC development (Althumairi et al., 2016), therapeutic interventions manipulating the microbiome and inflammation may represent a viable option to prevent malignant transformation. Along these lines, α4β7 integrin-expressing regulatory T cells (Tregs) can be used a cellular product to neutralize deleterious local inflammation to improve disease outcomes (Goldberg et al., 2019). Since Tregs from patients with IBDs express low levels of α4β7, a combination of rapamycin (sirolimus) and all-trans retinoic acid (ATRA) can be used to generate α4β7-expressing Tregs in vitro for cellular therapy. Pertaining to resolution of intestinal inflammation and antimicrobial defense, administration of BT-11, a locally active immuno-modulatory drug which enhances oxidative phosphorylation in immune cells, was shown to potentiate Treg induction and suppression of Th1 as well as Th17 responses in the murine model of C. rodentium-driven intestinal inflammation without compromising anti-C. rodentium immunological memory and clearance (Leber et al., 2019). Importantly, BT-11 targets the lanthionine synthetase C-like 2 (LANCL2) pathway, which happens to be central to inducing IL-10 production and amelioration of overt inflammation during infection, as shown in the context of influenza virus (Leber et al., 2017) and H. pylori (Leber et al., 2016) infections. These findings highlight LANCL2 as a host-directed therapy (HDT) target, which is expressed in the cell membrane of immune cells. Whether this approach would concomitantly promote exacerbation of an underlying bacterial infection in humans has to be formally tested in a suitable preclinical setting prior to clinical evaluation in patients.
Killer receptor NKG2D-expressing immune effector cells such as Vγ9Vδ2 T cells, NK cells and some populations of TCR αβ CD8+ cytotoxic T cells which recognize the HLA class I-like molecules MICA/B, represent an essential component of the immune system’s arsenal against targeted elimination of diseased tissue, i.e., transformed cells in cancer and infectious diseases (Huergo-Zapico et al., 2014). For example, the FAK/Src pathway has been shown to trigger downregulation of the MICA in cells, which is reversible with the use of focal adhesion kinase (FAK) inhibitors (Moncayo et al., 2017). Although MICA/B were recently described to be localized intracellularly in many tumor cell types (Ghadially et al., 2017), inhibition of FAK may be able to increase its expression on the cell surface to promote cell-mediated cytotoxicity. At the transcriptional level, miR-10b has been shown to downregulate MICB expression in tumor cells, which is also reversible (Tsukerman et al., 2012). HBV, via upregulation of the transcription factors GATA2 and GATA3 – which is also involved in Th2 and ILC2 development in humans (Wan, 2014), can force the downregulation of MICA/B in hepatoma cells to escape NK-cell surveillance (Guan et al., 2016). As mentioned in the first part of this review, pharmacological inhibition of FAK, with the clinical candidates GSK2256098, defactinib and BI-853520, which are already in several clinical trials involving patients with solid and hematological malignancies [Clinical Trials.gov identifiers NCT00787033, NCT01778803, NCT03727880, NCT01951690, NCT01943292, NCT01138033 (Doi et al., 2019)], may also augment NKG2D-mediated immunomodulation. Figure 1 is a schematic representation of how these above-mentioned pathogen-directed immune responses may be used for HDT for timely intervention after host responses to infectious agent are detected.
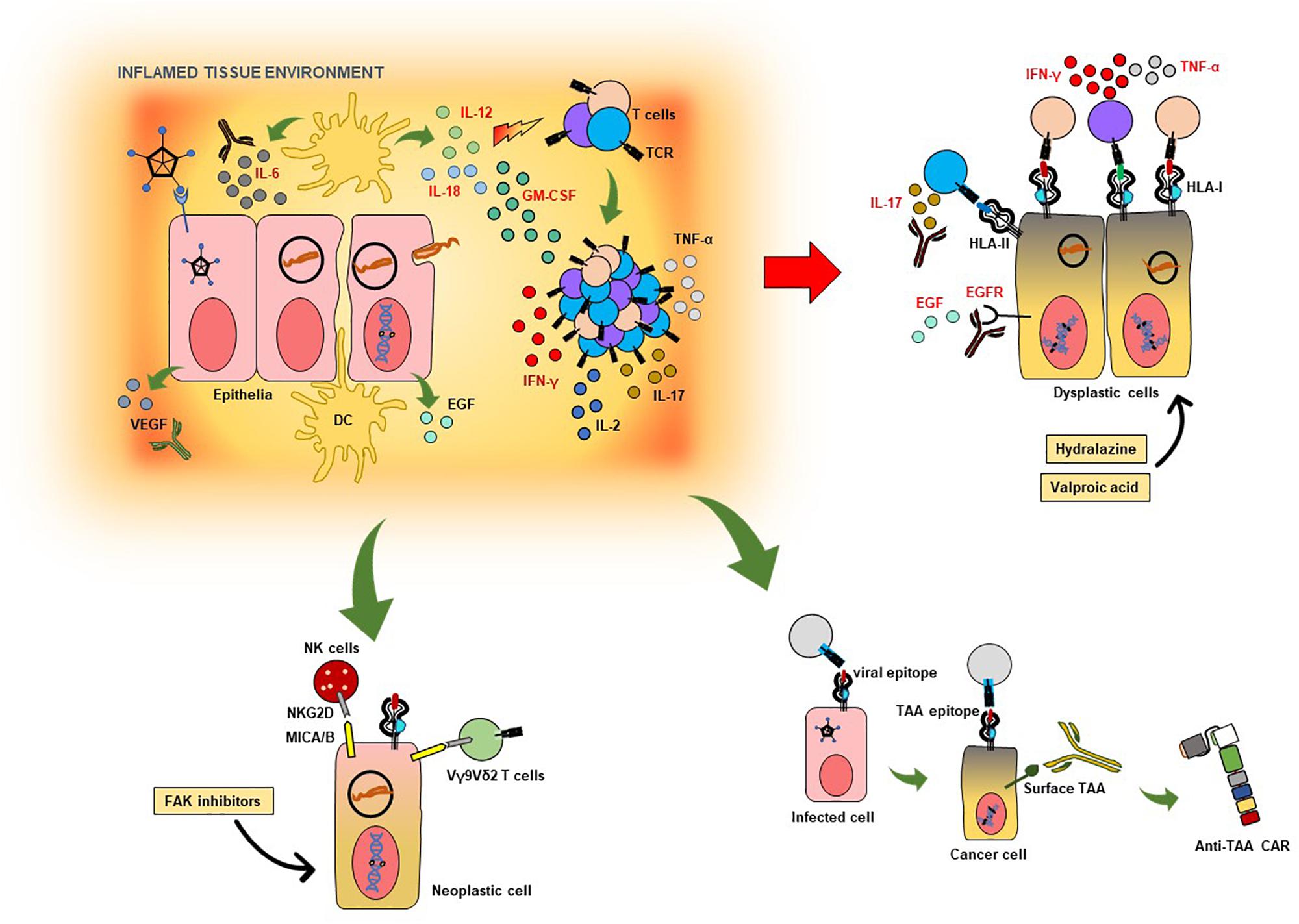
Figure 1. Targeted immunotherapeutic strategies against infection- and inflammation-induced neoplasia. The schematic shows some of the possible strategies which can be pursued in developing targeted host-directed immunotherapies based on biomarkers in infection-induced inflammation and oncogenesis. Cells infected with viruses or bacteria may induce physiological and anatomical changes in tissue, leading to reduced barrier functions and tissue integrity. This contributes to the overall local immunological milieu associated with the production of pro-inflammatory cytokines, as IL-6, IL-18, IL-12, and GM-CSF. In some cases of viral infection, VEGF production is also observed, which leads to neovascularization and poses a risk for malignant transformation. The same is true for EGF release by host cells in some bacterial infections. The ensuing T-cell activation culminates in production of IFN-γ, TNF-α, IL-2 as well as IL-17 in several infections – which can be pathogenic and contribute to chronic inflammation and neoplasia. To the right of the schematic are dysplastic cells, which can present antigenic epitopes to T cells, some of which may be protective (HLA class I-restricted pathogen- or host cell-derived structures), while others may exacerbate the IL-17 response (HLA class-II-restricted Th17 epitopes). Excess IL-17 as well as IL-6 can be neutralized using therapeutic antibodies which are clinically licensed. VEGF neutralization, as well as EGFR blockade, may be clinically useful in halting disease progression due to aberrant inflammation induced by infection. Hydralazine and valproic acid, both of which are clinically approved medications, can increase HLA class I expression in host cells, thereby augmenting and enhancing CD8+ T cell-mediated immune control. FAK inhibitors may be able to increase MICA/B expression in neoplastic cells (as well dysplastic ones) to improve immune surveillance by TCR Vγ9Vδ2 T cells and NK cells expressing the killer receptor NKG2D. Virus-induced T-cell responses against certain host-cell epitopes may educate T cells to subsequently recognize tumor cells; such T-cell populations may be used in active cell therapies and the nominal (antigen-specific) TCR may be utilized for transfer into recipient surrogate immune cells. Host cell surface molecules associated with malignant transformation induced by infection and inflammation, if recognized by circulating as well as tissue-associated antibodies, may be used as as templates for inducing ADCC as well as viable targets for biologically and clinically relevant chimeric antigen receptors (CARs) transgenically expressed by immune effector cells.
Pharmacological Agents for Therapeutic Immunomodulation in Infection and Neoplasia
Small molecules, such as valproic acid (VPA), a histone deacetylase inhibitor (HDI) and hydralazine may be used as adjuvants to improve immune responses in HPV+ patients and therefore may be a viable option due to the drugs’ capacity to upregulate HLA class expression in infected cells and CD8+ T-cell responses against them (Mora-Garcia Mde et al., 2006). Vorinostat, another HDI currently in clinical trials for cancer treatment (Bubna, 2015), along with VPA can actively inhibit Mtb growth in human cells and may shorten the treatment period with conventional first-line anti-TB drugs (Rao et al., 2018). Studies in mice suggest that carboplatin-derived cancer drugs exert their effects also via autophagy (Michaud et al., 2011), which facilitates anti-bacterial immune responses and clearance (Jo et al., 2013; Kaufmann et al., 2014). It may, therefore, be possible to investigate the immunological profile of cells isolated from platinum-treated patients with cancer for their responsiveness to pathogen-derived antigens and whether these cells can be used for therapeutic purposes. Rifampicin, which is a first-line anti-TB drug, has shown anti-angiogenic properties in preclinical studies, with inhibitory effects on human liver cancer cells and sarcoma cells (Shichiri and Tanaka, 2010), further to decreasing HCC to only a single occurrence in six patients who were HCV+ in over 97 months (8 years) (Shichiri et al., 2009). Since rifampicin is used not only to treat mycobacterial infections, but also those caused by meningococci, staphylococci and enterococci (Forrest and Tamura, 2010), the immunomodulatory properties of this drug may apply across the gastrointestinal and pulmonary organ systems. Based on experience in cancer treatment, low-dose cyclophosphamide-induced immunological fine-tuning by modulating CD8+ T and regulatory T-cell activity may also be useful in changing the immune milieu in immunocompetent individuals (Sistigu et al., 2011).
With regard to helminth infections, praziquantel has shown very good efficacy particularly in women and children based on the WHO-recommended parasite egg reduction rate (ERR) to measure control of parasite burden (Sayasone et al., 2017; Kabuyaya et al., 2018). While S. haematobium and O. viverrini infections exhibit better treatment outcomes, the treatment efficacy is somewhat reduced in the context of C. sinensis, with repeated doses required (Hong et al., 2001). This also increases the chances of drug resistance while not addressing the existing chronic inflammation in patients. Furthermore, praziquantel on its own can induce neutrophil activity in the bladder after treatment of patients with S. haematobium infection (Stecher et al., 2017). Allicin, a sulfur-containing compound extracted from garlic and available over-the-counter, was able to reduce inflammation as well as parasite burden albeit to a lesser extent than praziquantel in a mouse model of schistosomiasis (Metwally et al., 2018). Interestingly, allicin was also shown to reduce the viability of human CRC, breast and lung cancer cell lines in a dose-dependent manner (Gruhlke et al., 2016) resonating with a preclinical study in mice reported in 1960 (DiPaolo and Carruthers, 1960), thus making it a good candidate for testing in patients with helminth-induced liver and bile duct cancers.
Conclusion
The chain of molecular and immunological events occurring between infection and neoplasia in humans is challenging to study. Nevertheless, biomarkers obtained via clinical and translational studies (the role of METTL13/FEAT in infection-driven neoplasia is a good example), as well as ongoing drug trials provide an avenue to examine fluctuations which reflect different stages of the immunopathological process. A combination of measurement of soluble biological mediators in blood, immunohistological methods, next-generation sequencing and existing clinical knowledge will be able to identify and design personalized therapies. Furthermore, constituents of the study cohorts in question, as inclusion of uninfected household contacts, unrelated healthy donors and geographical distribution play a remarkably important role to more accurately decipher the tenets of protective vs. pathological immune and genetic biomarkers which may have theranostic value.
Author Contributions
MR, JL, AZ, and MM initiated, conceptualized, and wrote the first draft of the manuscript. All authors contributed with suggestions, revisions, and interpretation of different aspects in immune-interventions.
Funding
This work was supported by the Champalimaud Foundation.
Conflict of Interest
The authors declare that the research was conducted in the absence of any commercial or financial relationships that could be construed as a potential conflict of interest.
References
Althumairi, A. A., Lazarev, M. G., and Gearhart, S. L. (2016). Inflammatory bowel disease associated neoplasia: a surgeon’s perspective. World J. Gastroenterol. 22, 961–973. doi: 10.3748/wjg.v22.i3.961
An, J., Lichtenstein, A. K., Brent, G., and Rettig, M. B. (2002). The Kaposi sarcoma–associated herpesvirus (KSHV) induces cellular interleukin 6 expression: role of the KSHV latency-associated nuclear antigen and the AP1 response element. Blood 99, 649–654. doi: 10.1182/blood.v99.2.649
Andres, S., Schmidt, H. M., Mitchell, H., Rhen, M., Maeurer, M., and Engstrand, L. (2011). Helicobacter pylori defines local immune response through interaction with dendritic cells. FEMS Immunol. Med. Microbiol. 61, 168–178. doi: 10.1111/j.1574-695X.2010.00761.x
Atarashi, K., Tanoue, T., Ando, M., Kamada, N., Nagano, Y., and Narushima, S. (2015). Th17 cell induction by adhesion of microbes to intestinal epithelial cells. Cell 163, 367–380. doi: 10.1016/j.cell.2015.08.058
Aykut, B., Pushalkar, S., Chen, R., Li, Q., Abengozar, R., and Kim, J. I. (2019). The fungal mycobiome promotes pancreatic oncogenesis via activation of MBL. Nature 574, 264–267. doi: 10.1038/s41586-019-1608-2
Bacher, P., Hohnstein, T., Beerbaum, E., Blango, M. G., Kaufmann, S., and Eschenhagen, P. (2019). Human anti-fungal Th17 immunity and pathology rely on cross-reactivity against Candida albicans. Cell 6, 1340–1355. doi: 10.1016/j.cell.2019.01.041
Balachandran, V. P., Luksza, M., Zhao, J. N., Makarov, V., Moral, J. A., and Remark, R. (2017). Identification of unique neoantigen qualities in long-term survivors of pancreatic cancer. Nature 551, 512–516. doi: 10.1038/nature24462
Balasiddaiah, A., Davanian, H., Aleman, S., Pasetto, A., Frelin, L., and Lohmann, V. (2017). Hepatitis C virus-specific T cell receptor mRNA-engineered human T cells: impact of antigen specificity on functional properties. J. Virol. 91:e00010-e17. doi: 10.1128/JVI.00010-17
Bansal, A., Singh, M. P., and Rai, B. (2016). Human papillomavirus-associated cancers: a growing global problem. Int. J. Appl. Basic Med. Res. 6, 84–89. doi: 10.4103/2229-516X.179027
Barozzi, P., Bonini, C., Potenza, L., Masetti, M., Cappelli, G., and Gruarin, P. (2008). Changes in the immune responses against human herpesvirus-8 in the disease course of posttransplant Kaposi sarcoma. Transplantation 86, 738–744. doi: 10.1097/TP.0b013e318184112c
Berglund, E., Maaskola, J., Schultz, N., Friedrich, S., Marklund, M., and Tarish, F. (2018). Spatial maps of prostate cancer transcriptomes reveal an unexplored landscape of heterogeneity. Nat. Commun. 9:2419. doi: 10.1038/s41467-018-04724-4725
Bertoletti, A., Sette, A., Chisari, F. V., Penna, A., Levrero, M., and Fiaccadori, F. (1994). Natural variants of cytotoxic epitopes are T-cell receptor antagonists for antiviral cytotoxic T cells. Nature 369, 407–410. doi: 10.1038/369407a0
Bertoletti, A., Tan, A. T., and Koh, S. (2017). T-cell therapy for chronic viral hepatitis. Cytotherapy 19, 1317–1324. doi: 10.1016/j.jcyt.2017.07.011
Bomfim, I. L., Lamb, F., Fink, K., Szakacs, A., Silveira, A., and Franzen, L. (2017). The immunogenetics of narcolepsy associated with A(H1N1)pdm09 vaccination (Pandemrix) supports a potent gene-environment interaction. Genes Immun. 18, 75–81. doi: 10.1038/gene.2017.1
Borchert, S., Neumann, F., Schmidt, C., Wimmer, P., Dobner, T., and Grundhoff, A. (2014). High-affinity Rb binding, p53 inhibition, subcellular localization, and transformation by wild-type or tumor-derived shortened Merkel cell polyomavirus large T antigens. J. Virol. 88, 3144–3160. doi: 10.1128/JVI.02916-13
Borilova Linhartova, P., Kastovsky, J., Lucanova, S., Bartova, J., Poskerova, H., and Vokurka, J. (2016). Interleukin-17A gene variability in patients with type 1 diabetes mellitus and chronic Periodontitis: its correlation with IL-17 levels and the occurrence of periodontopathic bacteria. Media. Inflamm. 2016, 2979846–2979846. doi: 10.1155/2016/2979846
Botelho, M. C., Oliveira, P. A., Lopes, C., Correia da Costa, J. M., and Machado, J. C. (2011). Urothelial dysplasia and inflammation induced by Schistosoma haematobium total antigen instillation in mice normal urothelium. Urol. Oncol. 29, 809–814. doi: 10.1016/j.urolonc.2009.09.017
Botelho, M. C., Veiga, I., Oliveira, P. A., Lopes, C., Teixeira, M., and Machado, J. C. (2013). Carcinogenic ability of Schistosoma haematobium possibly through oncogenic mutation of KRAS gene. Adv. Cancer Res. Treat. 2013:876585.
Brewer, J. D., Shanafelt, T. D., Otley, C. C., Roenigk, R. K., Cerhan, J. R., and Kay, N. E. (2012). Chronic lymphocytic leukemia is associated with decreased survival of patients with malignant melanoma and merkel cell carcinoma in a SEER population-based study. J. Clin. Oncol. 30, 843–849. doi: 10.1200/JCO.2011.34.9605
Bryan, P. F., Karla, C., Edgar Alejandro, M. T., Sara Elva, E. P., Gemma, F., and Luz, C. (2016). Sphingolipids as mediators in the crosstalk between microbiota and intestinal cells: implications for inflammatory bowel disease. Media. Inflamm. 2016, 9890141–9890141.
Bubna, A. K. (2015). Vorinostat-an overview. Indian J Dermatol. 60:419. doi: 10.4103/0019-5154.160511
Cancer Genome Atlas Research (2014). Comprehensive molecular characterization of gastric adenocarcinoma. Nature 513, 202–209. doi: 10.1038/nature13480
Carrabba, M. G., Castelli, C., Maeurer, M. J., Squarcina, P., Cova, A., and Pilla, L. (2003). Suboptimal activation of CD8+ T cells by melanoma-derived altered peptide ligands. Role of Melan-A/MART-1 optimized analogues. Cancer Res. 63, 1560–1567.
Carter, J. J., Daugherty, M. D., Qi, X. J., Wipf, G. C., Robinson, K., and Roman, A. (2013). Identification of an overprinting gene in Merkel cell polyomavirus provides evolutionary insight into the birth of viral genes. Proc. Natl. Acad. Sci. U.S.A. 110, 12744–12749. doi: 10.1073/pnas.1303526110
Castro-Garza, J., Luevano-Martinez, M. L., Villarreal-Trevino, L., Gosalvez, J., Fernandez, J. L., and Davila-Rodriguez, M. I. (2018). Mycobacterium tuberculosis promotes genomic instability in macrophages. Mem. Inst. Oswaldo Cruz 113, 161–166. doi: 10.1590/0074-02760170281
Chang, C. C., Chang, C. W., Nguyen, P. A. A., Chang, T. H., Shih, Y. L., Chang, W. Y., et al. (2017). Ankylosing spondylitis and the risk of cancer. Oncol. Lett. 14, 1315–1322.
Chang, Y., Moore, P. S., and Weiss, R. A. (2017). Human oncogenic viruses: nature and discovery. Philos. Trans. R. Soc. Lond. B Biol. Sci. 372:20160264. doi: 10.1098/rstb.2016.0264
Chang, Y., Cesarman, E., Pessin, M. S., Lee, F., Culpepper, J., and Knowles, D. M. (1994). Identification of herpesvirus-like DNA sequences in AIDS-associated Kaposi’s sarcoma. Science 266, 1865–1869. doi: 10.1126/science.7997879
Chaput, N., Lepage, P., Coutzac, C., Soularue, E., Monot, C., and Boselli, L. (2017). Baseline gut microbiota predicts clinical response and colitis in metastatic melanoma patients treated with ipilimumab. Ann. Oncol. 28, 1368–1379. doi: 10.1093/annonc/mdx108
Chen, L., Li, B., Yang, W. C., He, J. L., Li, N. Y., and Hu, J. (2013). A dominant CD4(+) T-cell response to Helicobacter pylori reduces risk for gastric disease in humans. Gastroenterology 144, 591–600. doi: 10.1053/j.gastro.2012.12.002
Chiang, C. Y., Lee, J. J., Yu, M. C., Enarson, D. A., Lin, T. P., and Luh, K. T. (2009). Tuberculosis outcomes in Taipei: factors associated with treatment interruption for 2 months and death. Int. J. Tuberc. Lung Dis. 13, 105–111.
Chodisetti, S. B., Rai, P. K., Gowthaman, U., Pahari, S., and Agrewala, J. N. (2012). Potential T cell epitopes of Mycobacterium tuberculosis that can instigate molecular mimicry against host: implications in autoimmune pathogenesis. BMC Immunol. 13:13. doi: 10.1186/1471-2172-13-13
Chumduri, C., Gurumurthy, R. K., Zadora, P. K., Mi, Y., and Meyer, T. F. (2013). Chlamydia infection promotes host DNA damage and proliferation but impairs the DNA damage response. Cell Host Microbe 13, 746–758. doi: 10.1016/j.chom.2013.05.010
Cogliano, V. J., Baan, R., Straif, K., Grosse, Y., Bouvard, V., and Guha, N. (2011). Preventable exposures associated with human cancers. J. Natl. Cancer Inst. 103, 1827–1839. doi: 10.1093/jnci/djr483
Cox, A. L., Mosbruger, T., Mao, Q., Liu, Z., Wang, X. H., and Yang, H. C. (2005). Cellular immune selection with hepatitis C virus persistence in humans. J. Exp. Med. 201, 1741–1752. doi: 10.1084/jem.20050121
Dalmasso, G., Cougnoux, A., Delmas, J., Darfeuille-Michaud, A., and Bonnet, R. (2014). The bacterial genotoxin colibactin promotes colon tumor growth by modifying the tumor microenvironment. Gut Microb. 5, 675–680. doi: 10.4161/19490976.2014.969989
De la Herran-Arita, A. K., Kornum, B. R., Mahlios, J., Jiang, W., Lin, L., and Hou, T. (2013). CD4+ T cell autoimmunity to hypocretin/orexin and cross-reactivity to a 2009 H1N1 influenza A epitope in narcolepsy. Sci. Transl. Med. 5:2013.
DeCaprio, J. A. (2017). Merkel cell polyomavirus and Merkel cell carcinoma. Philos. Trans. R. Soc. Lond. B Biol. Sci. 372:20160276. doi: 10.1098/rstb.2016.0276
Devoto, A. E., Santini, J. M., Olm, M. R., Anantharaman, K., Munk, P., and Tung, J. (2019). Megaphages infect Prevotella and variants are widespread in gut microbiomes. Nat. Microbiol. 4, 693–700. doi: 10.1038/s41564-018-0338-9
DiPaolo, J. A., and Carruthers, C. (1960). The effect of allicin from garlic on tumor growth. Cancer Res. 20, 431–434.
Doi, T., Yang, J. C., Shitara, K., Naito, Y., Cheng, A. L., and Sarashina, A. (2019). Phase I study of the focal adhesion kinase inhibitor BI 853520 in Japanese and Taiwanese patients with advanced or metastatic solid tumors. Target Oncol. 14, 57–65. doi: 10.1007/s11523-019-00620-0
Dore, M. P., Cipolli, A., Ruggiu, M. W., Manca, A., Bassotti, G., and Pes, G. M. (2018). Helicobacter pylori eradication may influence timing of endoscopic surveillance for gastric cancer in patients with gastric precancerous lesions: a retrospective study. Medicine 97:e9734. doi: 10.1097/MD.0000000000009734
Draper, L. M., Kwong, M. L. M., Gros, A., Tran, E., Kerkar, S., and Raffeld, M. (2015). Targeting of HPV-16+ epithelial cancer cells by TCR gene engineered T cells directed against E6. Clin. Cancer Res. 21, 4431–4439. doi: 10.1158/1078-0432.CCR-14-3341
Enfield, K. S. S., Martin, S. D., Marshall, E. A., Kung, S. H. Y., Gallagher, P., and Milne, K. (2019). Hyperspectral cell sociology reveals spatial tumor-immune cell interactions associated with lung cancer recurrence. J. ImmunoTher. Cancer 7:13. doi: 10.1186/s40425-018-0488-486
Fan, X., Gunasena, H., Cheng, Z., Espejo, R., Crowe, S. E., Ernst, P. B., et al. (2000). Helicobacter pylori urease binds to class II MHC on gastric epithelial cells and induces their apoptosis. J. Immunol. 165, 1918–1924. doi: 10.4049/jimmunol.165.4.1918
Forrest, G. N., and Tamura, K. (2010). Rifampin combination therapy for nonmycobacterial infections. Clin. Microbiol. Rev. 23, 14–34. doi: 10.1128/CMR.00034-09
Franzosa, E. A., Fornelos, N., Haiser, H. J., Reinker, S., Vatanen, T., and Hall, A. B. (2019). Gut microbiome structure and metabolic activity in inflammatory bowel disease. Nat. Microbiol. 4, 293–305. doi: 10.1038/s41564-018-0306-4
Galanina, N., Goodman, A. M., Cohen, P. R., Frampton, G. M., and Kurzrock, R. (2018). Successful treatment of HIV-associated kaposi sarcoma with immune checkpoint blockade. Cancer Immunol. Res. 6, 1129–1135. doi: 10.1158/2326-6066.CIR-18-0121
Gardiner, D., Lalezari, J., Lawitz, E., DiMicco, M., Ghalib, R., and Reddy, K. R. (2013). A randomized, double-blind, placebo-controlled assessment of BMS-936558, a fully human monoclonal antibody to programmed death-1 (PD-1), in patients with chronic hepatitis C virus infection. PLoS One 8:e63818. doi: 10.1371/journal.pone.0063818
Gevers, D., Kugathasan, S., Knights, D., Kostic, A. D., Knight, R., and Xavier, R. J. (2017). A microbiome foundation for the Study of Crohn’s disease. Cell Host Microbe 21, 301–304. doi: 10.1016/j.chom.2017.02.012
Ghadially, H., Brown, L., Lloyd, C., Lewis, L., Lewis, A., and Dillon, J. (2017). MHC class I chain-related protein A and B (MICA and MICB) are predominantly expressed intracellularly in tumour and normal tissue. Br. J. Cancer 116, 1208–1217. doi: 10.1038/bjc.2017.79
Ghoneim, H. E., Fan, Y., Moustaki, A., Abdelsamed, H. A., Dash, P., and Dogra, P. (2017). De Novo epigenetic programs inhibit PD-1 blockade-mediated T cell rejuvenation. Cell 170:e19. doi: 10.1016/j.cell.2017.06.007
Goldberg, R., Scotta, C., Cooper, D., Nir, E., Tasker, S., and Irving, P. M. (2019). Correction of defective T-regulatory cells from patients with Crohn’s disease by ex vivo ligation of retinoic acid receptor alpha. Gastroenterology 156, 1775–1787. doi: 10.1053/j.gastro.2019.01.025
Golden-Mason, L., Palmer, B., Klarquist, J., Mengshol, J. A., Castelblanco, N., and Rosen, H. R. (2007). Upregulation of PD-1 expression on circulating and intrahepatic hepatitis C virus-specific CD8+ T cells associated with reversible immune dysfunction. J. Virol. 81, 9249–9258. doi: 10.1128/jvi.00409-07
Grant, W. B. (2018). A review of the evidence supporting the vitamin D-cancer prevention hypothesis in 2017. Anticancer. Res. 38, 1121–1136.
Gruhlke, M. C. H., Nicco, C., Batteux, F., and Slusarenko, A. J. (2016). The effects of allicin, a reactive Sulfur species from garlic, on a selection of mammalian cell lines. Antioxidants 6:1. doi: 10.3390/antiox6010001
Guan, Y., Li, W., Hou, Z., Han, Q., Lan, P., and Zhang, J. (2016). HBV suppresses expression of MICA/B on hepatoma cells through up-regulation of transcription factors GATA2 and GATA3 to escape from NK cell surveillance. Oncotarget 7, 56107–56119. doi: 10.18632/oncotarget.11271
Guo, L., Chen, W., Zhu, H., Chen, Y., Wan, X., and Yang, N. (2014). Helicobacter pylori induces increased expression of the vitamin d receptor in immune responses. Helicobacter 19, 37–47. doi: 10.1111/hel.12102
Gupta, V. K., Paul, S., and Dutta, C. (2017). Geography, ethnicity or subsistence-specific variations in human microbiome composition and diversity. Front. Microbiol. 8:1162. doi: 10.3389/fmicb.2017.01162
Han, Y., Chen, W., Li, P., and Ye, J. (2015). Association between Coeliac disease and risk of any malignancy and gastrointestinal malignancy: a meta-analysis. Medicine 94:e1612. doi: 10.1097/MD.0000000000001612
Harms, P. W., Collie, A. M., Hovelson, D. H., Cani, A. K., Verhaegen, M. E., and Patel, R. M. (2016). Next generation sequencing of Cytokeratin 20-negative Merkel cell carcinoma reveals ultraviolet-signature mutations and recurrent TP53 and RB1 inactivation. Mod. Pathol. 29, 240–248. doi: 10.1038/modpathol.2015.154
Harper, D. M., Vierthaler, S. L., and Santee, J. A. (2010). Review of gardasil. J. Vaccines Vaccin. 1:e1000107.
Harsch, I. A., and Konturek, P. C. (2018). The role of gut microbiota in obesity and type 2 and type 1 diabetes Mellitus: new insights into “Old”. diseases. Med. Sci. 6:32. doi: 10.3390/medsci6020032
He, J., Tang, X. F., Chen, Q. Y., Mai, H. Q., Huang, Z. F., Li, J., et al. (2012). Ex vivo expansion of tumor-infiltrating lymphocytes from nasopharyngeal carcinoma patients for adoptive immunotherapy. Chin. J. Cancer 31, 287–294. doi: 10.5732/cjc.011.10376
Hong, S. T., Rim, H. J., Min, D. Y., Li, X., Xu, J., Feng, Z., et al. (2001). Control of clonorchiasis by repeated treatments with praziquantel. Korean J. Parasitol. 39, 285–292.
Hou, S., Sun, X., Dong, X., Lin, H., Tang, L., and Xue, M. (2019). Chlamydial plasmid-encoded virulence factor Pgp3 interacts with human cathelicidin peptide LL-37 to modulate immune response. Microbes Infect. 21, 50–55. doi: 10.1016/j.micinf.2018.06.003
Huergo-Zapico, L., Acebes-Huerta, A., López-Soto, A., Villa-Álvarez, M., Gonzalez-Rodriguez, A. P., and Gonzalez, S. (2014). Molecular bases for the regulation of NKG2D Ligands in cancer. Front. Immunol. 5:106. doi: 10.3389/fimmu.2014.00106
Huffnagle, G. B., Dickson, R. P., and Lukacs, N. W. (2017). The respiratory tract microbiome and lung inflammation: a two-way street. Mucosal Immunol. 10, 299–306. doi: 10.1038/mi.2016.108
Hyrina, A., Meng, F., McArthur, S. J., Eivemark, S., Nabi, I. R., and Jean, F. (2017). Human Subtilisin Kexin Isozyme-1 (SKI-1)/Site-1 Protease (S1P) regulates cytoplasmic lipid droplet abundance: a potential target for indirect-acting anti-dengue virus agents. PLoS One 12:e0174483. doi: 10.1371/journal.pone.0174483
Iheagwara, U. K., Beatty, P. L., Van, P. T., Ross, T. M., Minden, J. S., and Finn, O. J. (2014). Influenza virus infection elicits protective antibodies and T cells specific for host cell antigens also expressed as tumor-associated antigens: a new view of cancer immunosurveillance. Cancer Immunol. Res. 2, 263–273. doi: 10.1158/2326-6066.CIR-13-0125
Ishida, K., and Hsieh, M. H. (2018). Understanding urogenital schistosomiasis-related bladder cancer: an update. Front. Med. 5:223. doi: 10.3389/fmed.2018.00223
Iyer, J. G., Afanasiev, O. K., McClurkan, C., Paulson, K., Nagase, K., and Jing, L. (2011). Merkel cell polyomavirus-specific CD8(+) and CD4(+) T-cell responses identified in Merkel cell carcinomas and blood. Clin. Cancer Res. 17, 6671–6680. doi: 10.1158/1078-0432.CCR-11-1513
Jagannathan-Bogdan, M., McDonnell, M. E., Shin, H., Rehman, Q., Hasturk, H., Apovian, C. M., et al. (2011). Elevated proinflammatory cytokine production by a skewed T cell compartment requires monocytes and promotes inflammation in type 2 diabetes. J. Immunol. 186, 1162–1172. doi: 10.4049/jimmunol.1002615
Jin, C., Lagoudas, G. K., Zhao, C., Bullman, S., Bhutkar, A., and Hu, B. (2019). Commensal microbiota promote lung cancer development via gammadelta T cells. Cell 176:998-1013.e16. doi: 10.1016/j.cell.2018.12.040
Jo, E. K., Yuk, J. M., Shin, D. M., and Sasakawa, C. (2013). Roles of autophagy in elimination of intracellular bacterial pathogens. Front. Immunol. 4:97. doi: 10.3389/fimmu.2013.00097
Jog, N. R., Chakravarty, E. F., Guthridge, J. M., and James, J. A. (2018). Epstein barr virus interleukin 10 suppresses anti-inflammatory phenotype in human monocytes. Front. Immunol. 9:2198. doi: 10.3389/fimmu.2018.02198
Jones, K., Nourse, J. P., Morrison, L., Nguyen-Van, D., Moss, D. J., and Burrows, S. R. (2010). Expansion of EBNA1-specific effector T cells in posttransplantation lymphoproliferative disorders. Blood 116, 2245–2252. doi: 10.1182/blood-2010-03-274076
Kabuyaya, M., Chimbari, M. J., and Mukaratirwa, S. (2018). Efficacy of praziquantel treatment regimens in pre-school and school aged children infected with schistosomiasis in sub-Saharan Africa: a systematic review. Infect. Dis. Povert. 7:73. doi: 10.1186/s40249-018-0448
Kah, J., Koh, S., Volz, T., Ceccarello, E., Allweiss, L., and Bertoletti, A. (2017). Lymphocytes transiently expressing virus-specific T cell receptors reduce hepatitis B virus infection. J. Clin. Invest. 127, 3177–3188. doi: 10.1172/JCI93024
Kamboj, M., and Sepkowitz, K. A. (2006). The risk of tuberculosis in patients with cancer. Clin. Infect. Dis 42, 1592–1595.
Kamphorst, A. O., Pillai, R. N., Yang, S., Nasti, T. H., Akondy, R. S., and Wieland, A. (2017). Proliferation of PD-1+ CD8 T cells in peripheral blood after PD-1-targeted therapy in lung cancer patients. Proc. Natl. Acad. Sci. U.S.A. 114, 4993–4998. doi: 10.1073/pnas.1705327114
Kansy, B. A., Srivastava, R. M., Shayan, G., Lei, Y., Moskovitz, J., and Moy, J. (2017). PD-1 status in CD8+ T cells associates with survival and anti-PD-1 therapeutic outcomes in head and neck cancer. Cancer Res. 77, 6353–6364. doi: 10.1158/0008-5472.CAN-16-3167
Kaufmann, S. H., Lange, C., Rao, M., Balaji, K. N., Lotze, M., and Schito, M. (2014). Progress in tuberculosis vaccine development and host-directed therapies–a state of the art review. Lancet Respir. Med. 2, 301–320. doi: 10.1016/S2213-2600(14)70033-5
Kenter, G. G., Welters, M. J. P., Lowik, M. J. G., Vloon, A. P. G., Essahsah, F., and Fathers, L. M. (2009). Vaccination against HPV-16 oncoproteins for vulvar intraepithelial neoplasia. New Eng. J. Med. 361, 1838–1847. doi: 10.1056/nejmoa0810097
Kim, H. J., Ko, Y. H., Kim, J. E., Lee, S. S., Lee, H., and Park, G. (2017). Hematopathology study group of the Korean society of, epstein-barr virus-associated Lymphoproliferative disorders: review and update on 2016 WHO classification. J. Pathol. Transl. Med. 51, 352–358. doi: 10.4132/jptm.2017.03.15
Kim, T. S., Pak, J. H., Kim, J. B., and Bahk, Y. Y. (2016). Clonorchis sinensis, an oriental liver fluke, as a human biological agent of cholangiocarcinoma: a brief review. BMB Rep. 49, 590–597. doi: 10.5483/bmbrep.2016.49.11.109
Kim, Y. I., Park, J. E., Brand, D. D., Fitzpatrick, E. A., and Yi, A. K. (2010). Protein kinase D1 is essential for the proinflammatory response induced by hypersensitivity pneumonitis-causing thermophilic actinomycetes Saccharopolyspora rectivirgula. J. Immunol. 184, 3145–3156. doi: 10.4049/jimmunol.0903718
Kirveskari, J., He, Q., Leirisalo-Repo, M., Mäki-Ikola, O., Wuorela, M., Putto-Laurila, A., et al. (1999). Enterobacterial infection modulates major histocompatibility complex class I expression on mononuclear cells. Immunology 97, 420–428. doi: 10.1046/j.1365-2567.1999.00803.x
Kocjan, B. J., Seme, K., Cimerman, M., Kovanda, A., Potocnik, M., and Poljak, M. (2009). Genomic diversity of human papillomavirus (HPV) genotype 38. J. Med. Virol. 81, 288–295. doi: 10.1002/jmv.21392
Kumar, B. V., Ma, W., Miron, M., Granot, T., Guyer, R. S., and Carpenter, D. J. (2017). Human tissue-resident memory T cells are defined by core transcriptional and functional signatures in lymphoid and mucosal sites. Cell Rep. 20, 2921–2934. doi: 10.1016/j.celrep.2017.08.078
Kumar, N. P., Sridhar, R., Banurekha, V. V., Jawahar, M. S., Fay, M. P., Nutman, T. B., et al. (2013). Type 2 diabetes mellitus coincident with pulmonary tuberculosis is associated with heightened systemic type 1, type 17, and other proinflammatory cytokines. Ann. Am. Thoracic Soc. 10, 441–449. doi: 10.1513/AnnalsATS.201305-112OC
Kumar, N. P., Sridhar, R., Nair, D., Banurekha, V. V., Nutman, T. B., and Babu, S. (2015). Type 2 diabetes mellitus is associated with altered CD8(+) T and natural killer cell function in pulmonary tuberculosis. Immunology 144, 677–686. doi: 10.1111/imm.12421
Kurtulus, S., Madi, A., Escobar, G., Klapholz, M., Nyman, J., and Christian, E. (2019). Checkpoint blockade immunotherapy induces dynamic changes in PD-1(-)CD8(+) tumor-infiltrating T cells. Immunity 50:e6. doi: 10.1016/j.immuni.2018.11.014
Ladinsky, M. S., Araujo, L. P., Zhang, X., Veltri, J., Soualhi, S., and Lee, C. (2019). Endocytosis of commensal antigens by intestinal epithelial cells regulates mucosal T cell homeostasis. Science 363:eaat4042. doi: 10.1126/science.aat4042
Leber, A., Bassaganya-Riera, J., Tubau-Juni, N., Zoccoli-Rodriguez, V., Lu, P., and Godfrey, V. (2017). Lanthionine synthetase C-Like 2 modulates immune responses to influenza virus infection. Front. Immunol. 8:178. doi: 10.3389/fimmu.2017.00178
Leber, A., Bassaganya-Riera, J., Tubau-Juni, N., Zoccoli-Rodriguez, V., Viladomiu, M., and Abedi, V. (2016). Modeling the role of lanthionine synthetase C-Like 2 (LANCL2) in the modulation of immune responses to Helicobacter pylori infection. PLoS One 11:e0167440. doi: 10.1371/journal.pone.0167440
Leber, A., Hontecillas, R., Zoccoli-Rodriguez, V., Chauhan, J., and Bassaganya-Riera, J. (2019). Oral treatment with BT-11 ameliorates inflammatory Bowel disease by enhancing regulatory T cell responses in the Gut. J. Immunol. 202, 2095–2104. doi: 10.4049/jimmunol.1801446
Lee, H. L., Shen, H., Hwang, I. Y., Ling, H., Yew, W. S., Lee, Y. S., et al. (2018). Targeted approaches for in situ gut microbiome manipulation. Genes 9:E351. doi: 10.3390/genes9070351
Lee, H. R., Lee, S., Chaudhary, P. M., Gill, P., and Jung, J. U. (2010). Immune evasion by Kaposi’s sarcoma-associated herpesvirus. Future Microbiol. 5, 1349–1365. doi: 10.2217/fmb.10.105
Lee, K. H., Gordon, A., Shedden, K., Kuan, G., Ng, S., and Balmaseda, A. (2019). The respiratory microbiome and susceptibility to influenza virus infection. PLoS One 14:e0207898. doi: 10.1371/journal.pone.0207898
Levitskaya, J., Coram, M., Levitsky, V., Imreh, S., Klein, G., and Kurilla, M. G. (1995). Inhibition of antigen processing by the internal repeat region of the Epstein-Barr virus nuclear antigen-1. Nature 375, 685–688. doi: 10.1038/375685a0
Li, C., Zhu, B., Son, Y. M., Wang, Z., Jiang, L., and Xiang, M. (2019). The transcription factor Bhlhe40 programs mitochondrial regulation of resident CD8(+) T cell fitness and functionality. Immunity 51, 491–507e7. doi: 10.1016/j.immuni.2019.08.013
Li, J., Chen, Q. Y., He, J., Li, Z. L., Tang, X. F., and Chen, S. P. (2015). Phase I trial of adoptively transferred tumor-infiltrating lymphocyte immunotherapy following concurrent chemoradiotherapy in patients with locoregionally advanced nasopharyngeal carcinoma. OncoImmunology 4:e976507. doi: 10.4161/23723556.2014.976507
Li, J., Wang, X., Diaz, J., Tsang, S. H., Buck, C. B., and You, J. (2013). Merkel cell polyomavirus large T antigen disrupts host genomic integrity and inhibits cellular proliferation. J. Virol. 87, 9173–9188. doi: 10.1128/JVI.01216-13
Liang, H., Fu, Z., Jiang, X., Wang, N., Wang, F., and Wang, X. (2015). miR-16 promotes the apoptosis of human cancer cells by targeting FEAT. BMC Cancer 15:448. doi: 10.1186/s12885-015-1458-1458
Lin, W. S., Jiao, B. Y., Wu, Y. L., Chen, W. N., and Lin, X. (2012). Hepatitis B virus X protein blocks filamentous actin bundles by interaction with eukaryotic translation elongat ion factor 1 alpha 1. J. Med. Virol. 84, 871–877. doi: 10.1002/jmv.23283
Lina, T. T., Alzahrani, S., Gonzalez, J., Pinchuk, I. V., Beswick, E. J., and Reyes, V. E. (2014). Immune evasion strategies used by Helicobacter pylori. World J. Gastroenterol. 20, 12753–12766. doi: 10.3748/wjg.v20.i36.12753
Liu, S., Hausmann, S., Carlson, S. M., Fuentes, M. E., Francis, J. W., and Pillai, R. (2019). METTL13 methylation of eEF1A increases translational output to promote Tumorigenesis. Cell 176, 491.e–504.e. doi: 10.1016/j.cell.2018.11.038
Loftus, D. J., Castelli, C., Clay, T. M., Squarcina, P., Marincola, F. M., and Nishimura, M. I. (1996). Identification of epitope mimics recognized by CTL reactive to the melanoma/melanocyte-derived peptide MART-1(27-35). J. Exp. Med. 184, 647–657. doi: 10.1084/jem.184.2.647
Lucke, K., Miehlke, S., Jacobs, E., and Schuppler, M. (2006). Prevalence of Bacteroides and Prevotella spp. in ulcerative colitis. J. Med. Microbiol. 55, 617–624. doi: 10.1099/jmm.0.46198-0
Luksza, M., Riaz, N., Makarov, V., Balachandran, V. P., Hellmann, M. D., and Solovyov, A. (2017). A neoantigen fitness model predicts tumour response to checkpoint blockade immunotherapy. Nature 551, 517–520. doi: 10.1038/nature24473
Luo, Y. H., Wu, C. H., Wu, W. S., Huang, C. Y., Su, W. J., and Tsai, C. M. (2012). Association between tumor epidermal growth factor receptor mutation and pulmonary tuberculosis in patients with adenocarcinoma of the lungs. J. Thorac. Oncol. 7, 299–305. doi: 10.1097/JTO.0b013e31823c588d
Magdy, A., Elhadidy, M., Abdallah, E., Thabet, W., Youssef, M., and Khafagy, W. (2015). Enteropathogenic Escherichia coli (EPEC): does it have a role in colorectal tumourigenesis? A prospective Cohort study. Int. J. Surg. 18, 169–173. doi: 10.1016/j.ijsu.2015.04.077
Maji, A., Misra, R., Dhakan, D. B., Gupta, V., Mahato, N. K., and Saxena, R. (2018). Gut microbiome contributes to impairment of immunity in pulmonary tuberculosis patients by alteration of butyrate and propionate producers. Environ. Microbiol. 20, 402–419. doi: 10.1111/1462-2920.14015
Manfredo Vieira, S., Hiltensperger, M., Kumar, V., Dehner, C., Khan, N., and Costa, F. R. C. (2018). Translocation of a gut pathobiont drives autoimmunity in mice and humans. Science 359, 1156–1161. doi: 10.1126/science.aar7201
Mangalam, A., Luckey, D., Basal, E., Behrens, M., Rodriguez, M., and David, C. (2008). HLA-DQ6 (DQB1∗0601)-restricted T cells protect against experimental autoimmune encephalomyelitis in HLA-DR3.DQ6 double-transgenic mice by generating anti-inflammatory IFN-gamma. J. Immunol. 180, 7747–7756. doi: 10.4049/jimmunol.180.11.7747
Mangalam, A. K., Taneja, V., and David, C. S. (2013). HLA class II molecules influence susceptibility versus protection in inflammatory diseases by determining the cytokine profile. J. Immunol. 190, 513–518. doi: 10.4049/jimmunol.1201891
Martínez, A., Pacheco-Tena, C., Vázquez-Mellado, J., and Burgos-Vargas, R. (2004). Relationship between disease activity and infection in patients with spondyloarthropathies. Ann. Rheum. Dis. 63, 1338–1340. doi: 10.1136/ard.2003.011882
Martínez-López, M., Iborra, S., Mastrangelo, A., Danne, C., Mann, E. R., and Reid, D. M. (2019). Microbiota sensing by Mincle-Syk Axis in Dendritic cells regulates interleukin-17 and -22 production and promotes intestinal barrier integrity. Immunity 50, 446.e–461.e. doi: 10.1016/j.immuni.2018.12.020
Mathieu, E., Descamps, D., Cherbuy, C., Langella, P., Riffault, S., Remot, A., et al. (2018a). Paradigms of lung microbiota functions in health and disease, particularly, in Asthma. Front. Physiol. 9:1168. doi: 10.3389/fphys.2018.01168
Mathieu, E., Escribano-Vazquez, U., Descamps, D., Cherbuy, C., Langella, P., and Riffault, S. (2018b). Paradigms of lung microbiota functions in health and disease, particularly, in Asthma. Front. Physiol. 9:1168. doi: 10.3389/fphys.2018.01168
Matzaraki, V., Kumar, V., Wijmenga, C., and Zhernakova, A. (2017). The MHC locus and genetic susceptibility to autoimmune and infectious diseases. Genome Biol. 18, 76–76. doi: 10.1186/s13059-017-1207-1
Mayassi, T., Ladell, K., Gudjonson, H., McLaren, J. E., Shaw, D. G., and Tran, M. T. (2019). Chronic inflammation permanently reshapes tissue-resident immunity in Celiac disease. Cell 176, 967.e–981.e. doi: 10.1016/j.cell.2018.12.039
Mehta, M. M., Weinberg, S. E., and Chandel, N. S. (2017). Mitochondrial control of immunity: beyond ATP. Nat. Rev. Immunol. 17, 608–620. doi: 10.1038/nri.2017.66
Metwally, D. M., Al-Olayan, E. M., Alanazi, M., Alzahrany, S. B., and Semlali, A. (2018). Antischistosomal and anti-inflammatory activity of garlic and allicin compared with that of praziquantel in vivo. BMC Complement Altern. Med. 18:135. doi: 10.1186/s12906-018-2191-z
Michaud, M., Martins, I., Sukkurwala, A. Q., Adjemian, S., Ma, Y., and Pellegatti, P. (2011). Autophagy-dependent anticancer immune responses induced by chemotherapeutic agents in mice. Science 334, 1573–1577. doi: 10.1126/science.1208347
Mignot, E., Hayduk, R., Black, J., Grumet, F. C., and Guilleminault, C. (1997). HLA DQB1∗0602 is associated with cataplexy in 509 narcoleptic patients. Sleep 20, 1012–1020.
Mignot, E., Lin, L., Rogers, W., Honda, Y., Qiu, X. H., and Lin, X. Y. (2001). Complex HLA-DR and -DQ interactions confer risk of narcolepsy-cataplexy in three ethnic groups. Am. J. Hum. Genet. 68, 686–699. doi: 10.1086/318799
Miller, N. J., Church, C. D., Fling, S. P., Kulikauskas, R., Ramchurren, N., and Shinohara, M. M. (2018). Merkel cell polyomavirus-specific immune responses in patients with Merkel cell carcinoma receiving anti-PD-1 therapy. J. Immunother. Cancer 6:131. doi: 10.1186/s40425-018-0450-457
Milner, J. J., Toma, C., Yu, B., Zhang, K., Omilusik, K., and Phan, A. T. (2017). Runx3 programs CD8(+) T cell residency in non-lymphoid tissues and tumours. Nature 552, 253–257. doi: 10.1038/nature24993
Molinari, M., Salio, M., Galli, C., Norais, N., Rappuoli, R., Lanzavecchia, A., et al. (1998). Selective inhibition of Ii-dependent antigen presentation by Helicobacter pylori toxin VacA. J. Exp. Med. 187, 135–140. doi: 10.1084/jem.187.1.135
Moncayo, G., Lin, D., McCarthy, M. T., Watson, A. A., and O’Callaghan, C. A. (2017). MICA expression is regulated by cell adhesion and contact in a FAK/Src-dependent manner. Front. Immunol. 7:687. doi: 10.3389/fimmu.2016.00687
Mora-Garcia Mde, L., Duenas-Gonzalez, A., Hernandez-Montes, J., De la Cruz-Hernandez, E., Perez-Cardenas, E., Weiss-Steider, B., et al. (2006). Up-regulation of HLA class-I antigen expression and antigen-specific CTL response in cervical cancer cells by the demethylating agent hydralazine and the histone deacetylase inhibitor valproic acid. J. Transl. Med. 4:55. doi: 10.1186/1479-5876-4-55
Munck, C., Sheth, R. U., Freedberg, D. E., and Wang, H. H. (2018). Real-time capture of horizontal gene transfers from gut microbiota by engineered CRISPR-Cas acquisition. bioRxiv doi: 10.1101/492751 [Preprint].
Nagu, T. (2017). ). Improving Treatment Outcomes for Patients With Pulmonary Tuberculosis in Tanzania: Host and Pathogen Factors. 64. Thesis, Karolinska Institutet, Stockholm.
Nam, Y. D., Jung, M. J., Roh, S. W., Kim, M. S., and Bae, J. W. (2011). Comparative analysis of Korean human gut microbiota by barcoded pyrosequencing. PLoS One 6:e22109. doi: 10.1371/journal.pone.0022109
Nasidze, I., Li, J., Quinque, D., Tang, K., and Stoneking, M. (2009). Global diversity in the human salivary microbiome. Genome Res. 19, 636–643. doi: 10.1101/gr.084616.108
Nikitina, I. Y., Panteleev, A. V., Kosmiadi, G. A., Serdyuk, Y. V., Nenasheva, T. A., and Nikolaev, A. A. (2018). Th1, Th17, and Th1Th17 lymphocytes during Tuberculosis: Th1 lymphocytes predominate and appear as low-differentiated CXCR3(+)CCR6(+) cells in the blood and highly differentiated CXCR3(±)CCR6(-) cells in the lungs. J. Immunol. 200, 2090–2103. doi: 10.4049/jimmunol.1701424
O’Dwyer, D. N., Dickson, R. P., and Moore, B. B. (2016). The lung microbiome, immunity and the pathogenesis of chronic lung disease. J. Immunol. 196, 4839–4847. doi: 10.4049/jimmunol.1600279
Ohkuma, T., Peters, S. A. E., and Woodward, M. (2018). Sex differences in the association between diabetes and cancer: a systematic review and meta-analysis of 121 cohorts including 20 million individuals and one million events. Diabetologia 61, 2140–2154. doi: 10.1007/s00125-018-4664-5
Olmstead, A. D., Knecht, W., Lazarov, I., Dixit, S. B., and Jean, F. (2012). Human subtilase SKI-1/S1P is a master regulator of the HCV Lifecycle and a potential host cell target for developing indirect-acting antiviral agents. PLoS Pathog. 8:e1002468. doi: 10.1371/journal.ppat.1002468
Osborne, L. C., Joyce, K. L., Alenghat, T., Sonnenberg, G. F., Giacomin, P. R., and Du, Y. (2013). Resistin-like molecule alpha promotes pathogenic Th17 cell responses and bacterial-induced intestinal inflammation. J. Immunol. 190, 2292–2300. doi: 10.4049/jimmunol.1200706
Paavonen, J. (2001). Chlamydia trachomatis and cancer. Sex Transm. Infect. 77, 154–156. doi: 10.1136/sti.77.3.154
Pagliari, M., Munari, F., Toffoletto, M., Lonardi, S., Chemello, F., and Codolo, G. (2017). Helicobacter pylori affects the antigen presentation activity of macrophages modulating the expression of the immune receptor CD300E through miR-4270. Front. Immunol. 8:1288. doi: 10.3389/fimmu.2017.01288
Pan, Y., Tian, T., Park, C. O., Lofftus, S. Y., Mei, S., and Liu, X. (2017). Survival of tissue-resident memory T cells requires exogenous lipid uptake and metabolism. Nature 543, 252–256. doi: 10.1038/nature21379
Pascal, V., Pozuelo, M., Borruel, N., Casellas, F., Campos, D., and Santiago, A. (2017). A microbial signature for Crohn’s disease. Gut 66, 813–822. doi: 10.1136/gutjnl-2016-313235
Patel, A. L., Chen, X., Wood, S. T., Stuart, E. S., Arcaro, K. F., and Molina, D. P. (2014). Activation of epidermal growth factor receptor is required for Chlamydia trachomatis development. BMC Microbiol. 14:277. doi: 10.1186/s12866-014-0277-274
Pauken, K. E., Sammons, M. A., Odorizzi, P. M., Manne, S., Godec, J., and Khan, O. (2016). Epigenetic stability of exhausted T cells limits durability of reinvigoration by PD-1 blockade. Science 354, 1160–1165. doi: 10.1126/science.aaf2807
Paulson, K. G., Carter, J. J., Johnson, L. G., Cahill, K. W., Iyer, J. G., and Schrama, D. (2010). Antibodies to merkel cell polyomavirus T antigen oncoproteins reflect tumor burden in merkel cell carcinoma patients. Cancer Res. 70, 8388–8397. doi: 10.1158/0008-5472.CAN-10-2128
Paulson, K. G., Lewis, C. W., Redman, M. W., Simonson, W. T., Lisberg, A., and Ritter, D. (2017). Viral oncoprotein antibodies as a marker for recurrence of Merkel cell carcinoma: a prospective validation study. Cancer 123, 1464–1474. doi: 10.1002/cncr.30475
Philip, M., Fairchild, L., Sun, L., Horste, E. L., Camara, S., and Shakiba, M. (2017). Chromatin states define tumour-specific T cell dysfunction and reprogramming. Nature 545, 452–456. doi: 10.1038/nature22367
Pizzolla, A., Nguyen, T. H., Sant, S., Jaffar, J., Loudovaris, T., and Mannering, S. I. (2018). Influenza-specific lung-resident memory T cells are proliferative and polyfunctional and maintain diverse TCR profiles. J. Clin. Invest. 128, 721–733. doi: 10.1172/JCI96957
Pourfarziani, V., Einollahi, B., Taheri, S., Nemati, E., Nafar, M., and Kalantar, E. (2007). Associations of Human Leukocyte Antigen (HLA) haplotypes with risk of developing lymphoproliferative disorders after renal transplantation. Ann. Transplant. 12, 16–22.
Pragman, A. A., Lyu, T., Baller, J. A., Gould, T. J., Kelly, R. F., and Reilly, C. S. (2018). The lung tissue microbiota of mild and moderate chronic obstructive pulmonary disease. Microbiome 6:7. doi: 10.1186/s40168-017-0381-384
Quinn, L. L., Williams, L. R., White, C., Forrest, C., Zuo, J., and Rowe, M. (2015). The missing link in epstein-barr virus immune evasion: the BDLF3 gene induces ubiquitination and downregulation of major histocompatibility complex class I (MHC-I) and MHC-II. J. Virol. 90, 356–367. doi: 10.1128/JVI.02183-15
Rao, M., Valentini, D., Zumla, A., and Maeurer, M. (2018). Evaluation of the efficacy of valproic acid and suberoylanilide hydroxamic acid (vorinostat) in enhancing the effects of first-line tuberculosis drugs against intracellular Mycobacterium tuberculosis. Int. J. Infect. Dis. 69, 78–84. doi: 10.1016/j.ijid.2018.02.021
Riquelme, E., Zhang, Y., Zhang, L., Montiel, M., Zoltan, M., and Dong, W. (2019). Tumor microbiome diversity and composition influence pancreatic cancer outcomes. Cell 178:e12. doi: 10.1016/j.cell.2019.07.008
Robey, R. C., Mletzko, S., and Gotch, F. M. (2011). The T-cell immune response against Kaposi’s sarcoma-associated herpesvirus. Adv. Virol. 2010:e340356.
Roy, S., and Trinchieri, G. (2017). Microbiota: a key orchestrator of cancer therapy. Nat. Rev. Cancer 17, 271–285. doi: 10.1038/nrc.2017.13
Saha, A., and Robertson, E. S. (2011). Epstein-Barr virus-associated B-cell lymphomas: pathogenesis and clinical outcomes. Clin. Cancer Res 17, 3056–3063. doi: 10.1158/1078-0432.ccr-10-2578
Sakakibara, S., and Tosato, G. (2011). Viral interleukin-6: role in Kaposi’s sarcoma-associated herpesvirus: associated malignancies. J. Interf. Cytokine Res. 31, 791–801. doi: 10.1089/jir.2011.0043
Sangro, B., Inarrairaegui, M., Garralda, E., Barrera, P., Larrea, E., and Alfaro, C. (2013). A clinical trial of CTLA-4 blockade with tremelimumab in patients with hepatocellular carcinoma and chronic hepatitis C. J. Hepatol. 59, 81–88. doi: 10.1016/j.jhep.2013.02.022
Sayasone, S., Meister, I., Andrews, J. R., Odermatt, P., Vonghachack, Y., and Xayavong, S. (2017). Efficacy and safety of praziquantel against light infections of Opisthorchis viverrini: a randomized parallel single-blind dose-ranging trial. Clin. Infect. Dis. 64, 451–458. doi: 10.1093/cid/ciw785
Schachter, J., Martel, J., Lin, C. S., Chang, C. J., Wu, T. R., Lu, C. C., et al. (2018). Effects of obesity on depression: a role for inflammation and the gut microbiota. Brain Behav. Immun. 69, 1–8. doi: 10.1016/j.bbi.2017.08.026
Scheper, W., Kelderman, S., Fanchi, L. F., Linnemann, C., Bendle, G., and Hirt, C. (2019). Low and variable tumor reactivity of the intratumoral TCR repertoire in human cancers. Nat. Med. 25, 89–94. doi: 10.1038/s41591-018-0266-5
Schreurs, R., Baumdick, M. E., Sagebiel, A. F., Kaufmann, M., Mokry, M., and Klarenbeek, P. L. (2019). Human fetal TNF-alpha-Cytokine-producing CD4(+) effector memory T cells promote intestinal development and mediate inflammation early in life. Immunity 50:e8. doi: 10.1016/j.immuni.2018.12.010
Shannon-Lowe, C., Rickinson, A. B., and Bell, A. I. (2017). Epstein-Barr virus-associated lymphomas. Philos. Trans. R. Soc. Lond. B Biol. Sci. 372:20160271. doi: 10.1098/rstb.2016.0271
Shichiri, M., Fukai, N., Kono, Y., and Tanaka, Y. (2009). Rifampicin as an oral angiogenesis inhibitor targeting Hepatic cancers. Cancer Res. 69, 4760–4768. doi: 10.1158/0008-5472.CAN-08-3417
Shichiri, M., and Tanaka, Y. (2010). Inhibition of cancer progression by rifampicin: involvement of antiangiogenic and anti-tumor effects. Cell Cycle 9, 64–68. doi: 10.4161/cc.9.1.10354
Shuda, M., Feng, H., Kwun, H. J., Rosen, S. T., Gjoerup, O., Moore, P. S., et al. (2008). T antigen mutations are a human tumor-specific signature for Merkel cell polyomavirus. Proc. Natl. Acad. Sci. U.S.A. 105, 16272–16277. doi: 10.1073/pnas.0806526105
Siebold, C., Hansen, B. E., Wyer, J. R., Harlos, K., Esnouf, R. E., and Svejgaard, A. (2004). Crystal structure of HLA-DQ0602 that protects against type 1 diabetes and confers strong susceptibility to narcolepsy. Proc. Natl. Acad. Sci. U.S.A. 101, 1999–2004. doi: 10.1073/pnas.0308458100
Simoni, Y., Becht, E., Fehlings, M., Loh, C. Y., Koo, S. L., and Teng, K. W. W. (2018). Bystander CD8(+) T cells are abundant and phenotypically distinct in human tumour infiltrates. Nature 557, 575–579. doi: 10.1038/s41586-018-0130-2
Sistigu, A., Viaud, S., Chaput, N., Bracci, L., Proietti, E., and Zitvogel, L. (2011). Immunomodulatory effects of cyclophosphamide and implementations for vaccine design. Semin. Immunopathol. 33, 369–383. doi: 10.1007/s00281-011-0245-0
Sripa, B., Brindley, P. J., Mulvenna, J., Laha, T., Smout, M. J., and Mairiang, E. (2012). The tumorigenic liver fluke Opisthorchis viverrini–multiple pathways to cancer. Trends Parasitol. 28, 395–407. doi: 10.1016/j.pt.2012.07.006
Sripa, B., Kaewkes, S., Sithithaworn, P., Mairiang, E., Laha, T., and Smout, M. (2007). Liver fluke induces cholangiocarcinoma. PLoS Med. 4:e201. doi: 10.1371/journal.pmed.0040201
Starrett, G. J., Marcelus, C., Cantalupo, P. G., Katz, J. P., Cheng, J., and Akagi, K. (2017). merkel cell polyomavirus exhibits dominant control of the tumor genome and transcriptome in virus-associated Merkel cell carcinoma. mBio 8:02079-2016. doi: 10.1128/mBio.02079-2016
Stecher, C. W., Fofana, H. K. M., Madsen, H., Wilson, S., Keita, A. D., and Landoure, A. (2017). Inflammation dynamics after praziquantel treatment of Schistosoma haematobium reflected by urinary eosinophil cationic protein. Trans. R. Soc. Trop. Med. Hyg. 111, 316–324. doi: 10.1093/trstmh/trx057
Stevanovic, S., Draper, L. M., Langhan, M. M., Campbell, T. E., Kwong, M. L., and Wunderlich, J. R. (2015). Complete regression of metastatic cervical cancer after treatment with human papillomavirus-targeted tumor-infiltrating T cells. J. Clin. Oncol. 33, 1543–1550. doi: 10.1200/JCO.2014.58.9093
Strickler, H. D., Goedert, J. J., Bethke, F. R., Trubey, C. M., Palefsky, J., and Whitman, J. E. (1999). Human herpesvirus 8 cellular immune responses in homosexual men. J. Infect. Dis. 180, 1682–1685. doi: 10.1086/315056
Sun, B., and Karin, M. (2012). Obesity, inflammation, and liver cancer. J. Hepatol. 56, 704–713. doi: 10.1016/j.jhep.2011.09.020
Szender, J. B., Eng, K. H., Matsuzaki, J., Miliotto, A., Gnjatic, S., and Tsuji, T. (2016). HLA superfamily assignment is a predictor of immune response to cancer testis antigens and survival in ovarian cancer. Gynecol. Oncol. 142, 158–162. doi: 10.1016/j.ygyno.2016.04.017
Tadmor, T., Aviv, A., and Polliack, A. (2011). Merkel cell carcinoma, chronic lymphocytic leukemia and other lymphoproliferative disorders: an old bond with possible new viral ties. Ann. Oncol. 22, 250–256. doi: 10.1093/annonc/mdq308
Tafti, M., Lammers, G. J., Dauvilliers, Y., Overeem, S., Mayer, G., and Nowak, J. (2016). Narcolepsy-associated HLA class i alleles implicate cell-mediated cytotoxicity. Sleep 39, 581–587. doi: 10.5665/sleep.5532
Takahashi, A., Tokita, H., Takahashi, K., Takeoka, T., Murayama, K., and Tomotsune, D. (2011). A novel potent tumour promoter aberrantly overexpressed in most human cancers. Sci. Rep. 1:15. doi: 10.1038/srep00015
Teng, G. G., Wang, W. H., Dai, Y., Wang, S. J., Chu, Y. X., and Li, J. (2013). Let-7b is involved in the inflammation and immune responses associated with Helicobacter pylori infection by targeting toll-like receptor 4. PLoS One 8:e56709. doi: 10.1371/journal.pone.0056709
Theiss, J. M., Gunther, T., Alawi, M., Neumann, F., Tessmer, U., and Fischer, N. (2015). A comprehensive analysis of replicating merkel cell Polyomavirus genomes delineates the viral transcription program and suggests a role for mcv-miR-M1 in episomal persistence. PLoS Pathog. 11:e1004974. doi: 10.1371/journal.ppat.1004974
Tian, C., Hromatka, B. S., Kiefer, A. K., Eriksson, N., Noble, S. M., and Tung, J. Y. (2017). Genome-wide association and HLA region fine-mapping studies identify susceptibility loci for multiple common infections. Nat. Commun. 8:599. doi: 10.1038/s41467-017-00257-255
Trabert, B., Waterboer, T., Idahl, A., Brenner, N., Brinton, L. A., and Butt, J. (2019). Antibodies against Chlamydia trachomatis and ovarian cancer risk in two independent populations. J. Natl. Cancer Inst. 111, 129–136. doi: 10.1093/jnci/djy084
Triozzi, P. L., and Fernandez, A. P. (2013). The role of the immune response in merkel cell carcinoma. Cancers 5, 234–254. doi: 10.3390/cancers5010234
Tsao, S. W., Tsang, C. M., and Lo, K. W. (2017). Epstein-Barr virus infection and nasopharyngeal carcinoma. Philos. Trans. R. Soc. Lond. B Biol. Sci. 372, 20160270. doi: 10.1098/rstb.2016.0270
Tsukerman, P., Stern-Ginossar, N., Gur, C., Glasner, A., Nachmani, D., and Bauman, Y. (2012). MiR-10b downregulates the stress-induced cell surface molecule MICB, a critical ligand for cancer cell recognition by natural killer cells. Cancer Res. 72, 5463–5472. doi: 10.1158/0008-5472.CAN-11-2671
Tynan, F. E., Elhassen, D., Purcell, A. W., Burrows, J. M., Borg, N. A., and Miles, J. J. (2005). The immunogenicity of a viral cytotoxic T cell epitope is controlled by its MHC-bound conformation. J. Exp. Med. 202, 1249–1260. doi: 10.1084/jem.20050864
Tzellos, S., and Farrell, P. J. (2012). Epstein-barr virus sequence variation-biology and disease. Pathogens 1, 156–174. doi: 10.3390/pathogens1020156
Vase, M. Ø, Maksten, E. F., Strandhave, C., Søndergaard, E., Bendix, K., and Hamilton-Dutoit, S. (2015). HLA associations and risk of posttransplant lymphoproliferative disorder in a Danish population-based cohort. Transpl. Direct 1:e25. doi: 10.1097/TXD.0000000000000534
Victora, C. G., Bahl, R., Barros, A. J. D., Horton, S., Krasevec, J., and Murch, S. (2016). Breastfeeding in the 21st century: epidemiology, mechanisms, and lifelong effect. Lancet 387, 475–490. doi: 10.1016/S0140-6736(15)01024-7
Wan, Y. Y. (2014). GATA3: a master of many trades in immune regulation. Trends Immunol. 35, 233–242. doi: 10.1016/j.it.2014.04.002
Wong, S. Q., Waldeck, K., Vergara, I. A., Schroder, J., Madore, J., and Wilmott, J. S. (2015). UV-associated mutations underlie the etiology of MCV-negative merkel cell carcinomas. Cancer Res. 75, 5228–5234. doi: 10.1158/0008-5472.CAN-15-1877
Wroblewski, L. E., Peek, R. M. Jr., and Wilson, K. T. (2010). Helicobacter pylori and gastric cancer: factors that modulate disease risk. Clin. Microbiol. Rev. 23, 713–739. doi: 10.1128/CMR.00011-10
Yang, D., Chen, X., Wang, J., Lou, Q., Lou, Y., and Li, L. (2019). Dysregulated lung commensal bacteria drive Interleukin-17B production to promote pulmonary Fibrosis through their outer membrane vesicles. Immunity 50, 692–706. doi: 10.1016/j.immuni.2019.02.001
Yap, G. C., Chee, K. K., Hong, P. Y., Lay, C., Satria, C. D., and Shek, L. P. C. (2011). Evaluation of stool microbiota signatures in two cohorts of Asian (Singapore and Indonesia) newborns at risk of atopy. BMC Microbiol. 11:193. doi: 10.1186/1471-2180-11-193
Yatsunenko, T., Rey, F. E., Manary, M. J., Trehan, I., Contreras, M., and Magris, M. (2012). Human gut microbiome viewed across age and geography. Nature 486, 222–227. doi: 10.1038/nature11053
Yoon, S. I., Jones, B. C., Logsdon, N. J., Harris, B. D., Kuruganti, S., and Walter, M. R. (2012). Epstein-Barr virus IL-10 engages IL-10R1 by a two-step mechanism leading to altered signaling properties. J. Biol. Chem. 287, 26586–26595. doi: 10.1074/jbc.M112.376707
Yoshimoto, S., Loo, T. M., Atarashi, K., Kanda, H., Sato, S., and Oyadomari, S. (2013). Obesity-induced gut microbial metabolite promotes liver cancer through senescence secretome. Nature 499, 97–101. doi: 10.1038/nature12347
Yost, K. E., Satpathy, A. T., Wells, D. K., Qi, Y., Wang, C., and Kageyama, R. (2019). Clonal replacement of tumor-specific T cells following PD-1 blockade. Nat. Med. 25, 1251–1259. doi: 10.1038/s41591-019-0522-3
Yue, J., Shukla, R., Accardi, R., Siouda, M., Cros, M. P., and Krutovskikh, V. (2011). Cutaneous human papillomavirus type 38 E7 regulates actin cytoskeleton structure for increasing cell proliferation through CK2 and the eukaryotic elongation factor 1A. J. Virol. 85, 8477–8494. doi: 10.1128/JVI.02561-10
Zehbe, I., Hohn, H., Pilch, H., Neukirch, C., Freitag, K., and Maeurer, M. J. (2005). Differential MHC class II component expression in HPV-positive cervical cancer cells: implication for immune surveillance. Int. J. Cancer 117, 807–815. doi: 10.1002/ijc.21226
Zheng, L., Kelly, C. J., Battista, K. D., Schaefer, R., Lanis, J. M., and Alexeev, E. E. (2017). Microbial-derived butyrate promotes epithelial barrier function through IL-10 receptor-dependent repression of Claudin-2. J. Immunol. 199, 2976–2984. doi: 10.4049/jimmunol.1700105
Zhou, Y., Cui, Z., Zhou, X., Chen, C., Jiang, S., and Hu, Z. (2013). The presence of old pulmonary tuberculosis is an independent prognostic factor for squamous cell lung cancer survival. J. Cardiothorac. Surg. 8:123. doi: 10.1186/1749-8090-8-123
Zimmermann, M., Zimmermann-Kogadeeva, M., Wegmann, R., and Goodman, A. L. (2019). Separating host and microbiome contributions to drug pharmacokinetics and toxicity. Science 363:eaat9931. doi: 10.1126/science.aat9931
Keywords: pathogens, microbiota, inflammation, neoplasia, immune responses, antibodies, cancer, immunotherapy
Citation: Lérias JR, Paraschoudi G, de Sousa E, Martins J, Condeço C, Figueiredo N, Carvalho C, Dodoo E, Castillo-Martin M, Beltrán A, Ligeiro D, Rao M, Zumla A and Maeurer M (2020) Microbes as Master Immunomodulators: Immunopathology, Cancer and Personalized Immunotherapies. Front. Cell Dev. Biol. 7:362. doi: 10.3389/fcell.2019.00362
Received: 06 April 2019; Accepted: 12 December 2019;
Published: 23 January 2020.
Edited by:
Giulia De Falco, Queen Mary University of London, United KingdomReviewed by:
Deyin Xing, School of Medicine, Johns Hopkins University, United StatesYoshiyuki Goto, Chiba University, Japan
Copyright © 2020 Lérias, Paraschoudi, de Sousa, Martins, Condeço, Figueiredo, Carvalho, Dodoo, Castillo-Martin, Beltrán, Ligeiro, Rao, Zumla and Maeurer. This is an open-access article distributed under the terms of the Creative Commons Attribution License (CC BY). The use, distribution or reproduction in other forums is permitted, provided the original author(s) and the copyright owner(s) are credited and that the original publication in this journal is cited, in accordance with accepted academic practice. No use, distribution or reproduction is permitted which does not comply with these terms.
*Correspondence: Markus Maeurer, markus.maeurer@fundacaochampalimaud.pt
†These authors share senior authorship