- 1Laboratory of Molecular and Cellular Ophthalmology, Centre for Integrative Biology, University of Trento, Trento, Italy
- 2Laboratory of Neural Development and Regeneration, Centre for Integrative Biology, University of Trento, Trento, Italy
- 3Laboratory of Translational Neurogenetics, Centre for Integrative Biology, University of Trento, Trento, Italy
The coordinated interplay between extrinsic activating and repressing cell signaling molecules is pivotal for embryonic development and subsequent tissue homeostasis. This is well exemplified by studies on the evolutionarily conserved Wnt signaling pathways. Tight temporal and spatial regulation of Wnt signaling activity is required throughout lifetime, from maternal stages before gastrulation until and throughout adulthood. Outside cells, the action of numerous Wnt ligands is counteracted and fine-tuned by only a handful of well characterized secreted inhibitors, such as for instance Dickkopf, secreted Frizzled Related Proteins and Cerberus. Here, we give an overview of our current understanding of another secreted Wnt signaling antagonist, the Wnt inhibitory factor Wif1. Wif1 can directly interact with various Wnt ligands and inhibits their binding to membrane bound receptors. Epigenetic promoter methylation of Wif1, leading to silencing of its transcription and concomitant up-regulation of Wnt signaling, is a common feature during cancer progression. Furthermore, an increasing number of reports describe Wif1 involvement in regulating processes during embryonic development, which so far has not received as much attention. We will summarize our knowledge on Wif1 function and its mode of action with a particular focus on the zebrafish (Danio rerio). In addition, we highlight the potential of Wif1 research to understand and possibly influence mechanisms underlying eye diseases and regeneration.
Introduction
Gene regulatory mechanisms facilitate the fundamental necessity that not all cells express and activate all genes and signaling pathways at the same time. Even in the presence of pathway components within a cell, the temporal control of signaling activity needs to be tightly controlled during development and tissue homeostasis. This can occur outside the cells such that ligands are prevented to bind receptors by secreted inhibitory molecules. These repressor proteins can act by directly binding to the ligand or by interacting with their receptor. Studies of the Wnt signaling cascades have been particularly informative as to the mechanisms of extrinsic control of signal activation by either of the nineteen Wnt ligands in mammals and twenty-six in teleosts (Kawano and Kypta, 2003; Beretta et al., 2011; Cruciat and Niehrs, 2013). The secreted repressors interacting with Wnt ligand receptors that have been identified so far include Dickkopf (Dkk) (Glinka et al., 1998; Niehrs, 2006), Sclerostin (Sost)/Wise (Sostdc1) (Itasaki et al., 2003; Li et al., 2005; Semenov et al., 2005) and Insulin growth factor binding protein 4 (Igfbp4) (Zhu et al., 2008). Conversely, those that directly bind to Wnt ligands comprise secreted Frizzled Related Proteins (sFRPs) (Hoang et al., 1996), Cerberus (Bouwmeester et al., 1996) and Wnt inhibitory factor 1 (Wif1) (Hsieh et al., 1999). Among these, Wif1 has perhaps received the least recognition. Its function is, however, implicated in various crucial processes during eye development and homeostasis (Hunter et al., 2004; Park et al., 2014), neurogenesis and axon extension (Hunter et al., 2004; Nakaya et al., 2008), lung and anorectal development (Xu et al., 2011; Ng et al., 2014), tooth morphogenesis (Lee et al., 2015), chondrogenesis (Surmann-Schmitt et al., 2009), stem cell maintenance (Ding et al., 2008; Nakatsu et al., 2011), regeneration and cancer (Wissmann et al., 2003; Lim et al., 2017; Figure 1). The Wif1 protein structure exhibits intriguing features suggesting that its full spectrum of action and importance has yet to be uncovered. Its expression is largely conserved from teleosts to human. This is a prerequisite for using genetically amenable animal models such as the zebrafish for studying Wif1 function in vivo to better understand the pathophysiology of Wif1 linked human diseases needed for therapy development.
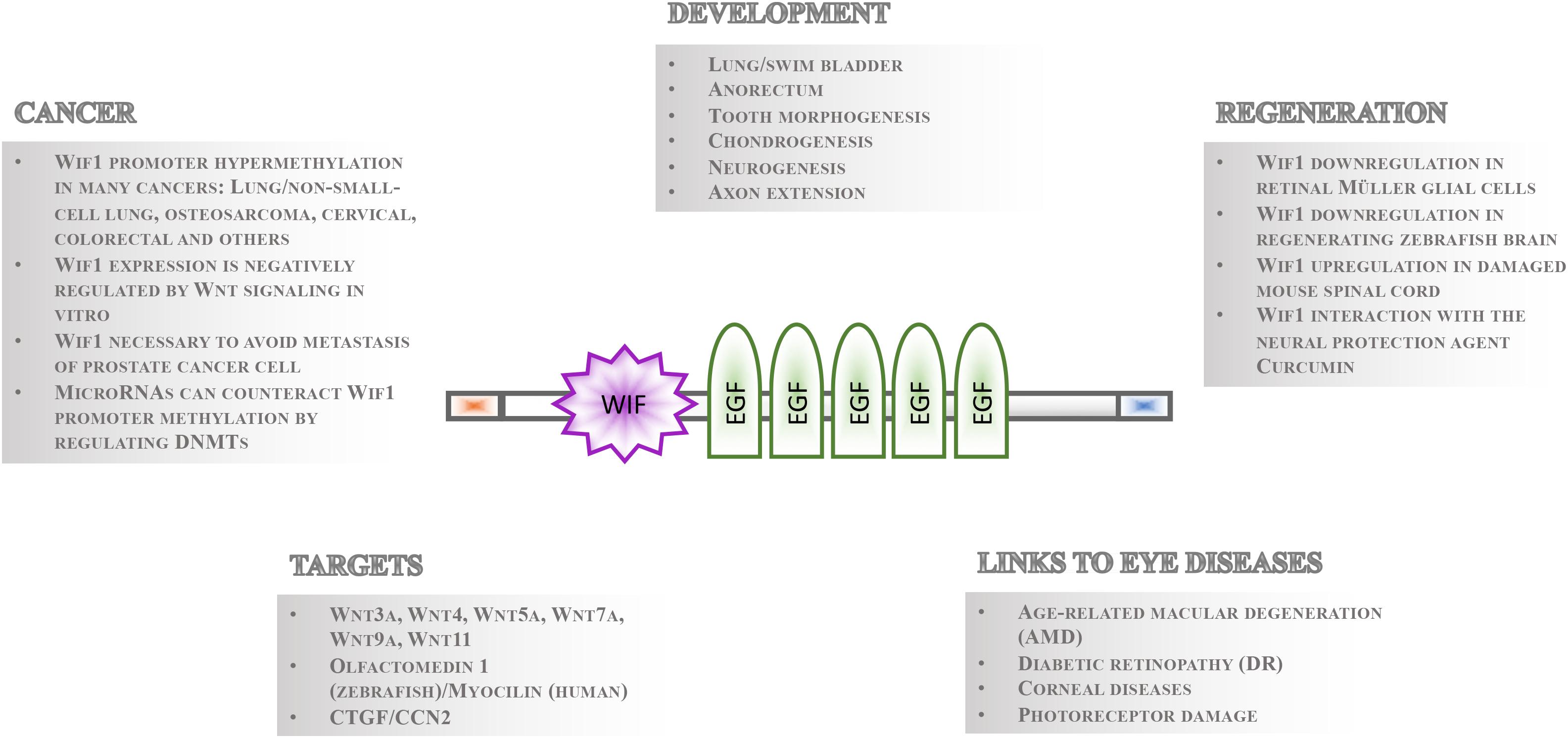
Figure 1. Overview of Wif1 structure and function. The 379 aa Wif1 protein comprises an N-terminal signal sequence (orange box), a 150 aa Wif-domain, five epidermal growth factor (EGF)-like repeats, and a 45 aa long hydrophilic tail (blue box) at the C-terminus (Hsieh et al., 1999). Wif1 interacts with a number of proteins and has been linked to various processes in embryonic development, regeneration, cancer, and eye diseases as indicated.
Wif1 Characteristics
Wif1 was identified as an expressed sequence tag in the human retina and was functionally described as a negative regulator of canonical or Wnt/beta-catenin signaling in 1999 (Hsieh et al., 1999). However, Wif1 is able to physically interact with both canonical and non-canonical Wnt ligands such as Drosophila wingless, and vertebrate Wnt3a, Wnt4, Wnt5a, Wnt7a, Wnt9a, and Wnt11 (Hsieh et al., 1999; Surmann-Schmitt et al., 2009). In addition, Wif1 binding to zebrafish glycoprotein Olfactomedin1 (Nakaya et al., 2008) and to connective tissue growth factor (CTGF/CCN2) was reported in vitro (Surmann-Schmitt et al., 2012). The 379 amino acids (aa) Wif1 protein comprises an N-terminal signal sequence, a unique and conserved 150 aa Wif-domain, five epidermal growth factor (EGF)-like repeats, and a 45 aa long hydrophilic tail (Hsieh et al., 1999; Figure 1). Crystal structure analysis of the Wnt ligand binding N-terminal part of the Wif1 domain (Wif1WD) and its interaction with Wnt3a revealed that the EGF domains of Wif1 are required for Wnt ligand binding (Malinauskas et al., 2011). In addition, EGF domains II–V have heparan sulfate proteoglycan (HSPG) binding properties. HSPGs strengthen the interaction between Wif1 and Wnt ligands (Avanesov et al., 2012), but they are also known to modulate diffusion of morphogens with which they interact such as Wnt proteins (Panakova et al., 2005; Yan and Lin, 2009). Thus, in addition to Wnt ligand binding, Wif1 might well influence the generation of morphogen gradients and/or protect cells within such gradients from signal cascade activation. This feature might not be restricted to Wnt ligands. The Drosophila Wif1 ortholog “Shifted” promotes hedgehog signaling (Glise et al., 2005; Gorfinkiel et al., 2005), and this was hypothesized to potentially occur also in vertebrates (Avanesov et al., 2012).
Intriguingly, a pocket for phospholipids was identified in the Wif1WD domain (Malinauskas et al., 2011) binding 1,2-dipalmitoyl-phosphatidylcholine (DPPC). This suggested that Wif1 shares similarities with lipoprotein particles that can sequester Wnt3a-linked lipids (Willert et al., 2003; Panakova et al., 2005; Malinauskas et al., 2011). However, since the Wif1WD pocket already binds DPPC, the exchange with a Wnt ligand-bound palmitoleoyl moiety would require energy in the aqueous environment and would therefore rather be unlikely. It was hypothesized that the lipid containing pocket in the Wif-domain may provide conformational flexibility and thereby indirectly contribute to expose the appropriate surface for ligand binding (Malinauskas et al., 2011). Interestingly, also RYK-receptors involved in non-canonical Wnt signaling contain Wif-domains, raising the possibility that the lipid pocket could also be used directly for the binding of moieties attached to Wnt ligands (Kawano and Kypta, 2003; Malinauskas and Jones, 2014).
Wif1 Epigenetics and Cancer
The Wnt pathway is well known for regulating cell stemness in many organs and tissues including bone, intestine and skin (Steinhart and Angers, 2018). In these and several other tissues, deregulation of various Wnt pathway components has been implicated in cancer occurrence and/or recurrence (Tai et al., 2015; Nusse and Clevers, 2017). One of these components is Wif1, which is downregulated in prostate, breast, lung and bladder cancers, as shown by RNA microarray analysis (Wissmann et al., 2003). Genomic studies identified a CpG island in the human Wif1 promoter 1,5 kb upstream of the Wif1 gene (Reguart et al., 2004). Methylation of CpG islands is one of the major modes of inactivating tumor suppressor genes in cancer (Herman and Baylin, 2003). Indeed, hypermethylation of the Wif1 promoter, leading to Wif1 silencing (and thus activation of Wnt/beta-catenin signaling), was shown to be associated with various types of cancers such as lung cancer, in particular non-small cell lung cancer (NSCLC) (Wissmann et al., 2003; Tan et al., 2013; Zheng et al., 2016; Guo et al., 2017), osteosarcoma formation (Kansara et al., 2009), colorectal cancer (Hu et al., 2018), cervical cancer (Ramachandran et al., 2012) and others (Figure 1). In NSCLC, Wif1 promoter hypermethylation can be counteracted by microRNAs, which negatively regulate DNA methyltransferases in a regulatory feedback loop (Tan et al., 2013). The epigenetic silencing of secreted Wnt pathway inhibitors related to cancer appears not restricted to Wif1 but holds true also for most other members such as Dkk1-3, Sost, Igfbp4 or sFRP1-5 (Mazieres et al., 2004; Aguilera et al., 2006; Roman-Gomez et al., 2007; Sato et al., 2007; Elston et al., 2008; Kongkham et al., 2010; Fellenberg et al., 2013; Gopal et al., 2013). In addition, Wif1 function is required to prevent metastasis of cancer cells. Prostate cancer (PCa) cells can invade bone tissue. In PCa cell lines, Wif1 expression is spontaneously downregulated by promoter hypermethylation. Restoring Wif1 expression, however, leads to a reduction in cell invasiveness and motility by upregulation of epithelial markers (Yee et al., 2010). These studies are encouraging for the development of cancer therapies. For instance, targeted disruption or addition of CpG islands in the Wif1 promoter using genome editing techniques would be informative with respect to the resulting cell behaviors in established Wnt cancer models. In parallel, effects at the developmental level can be analyzed in vivo in the physiological environment of the zebrafish. Such complementing studies in zebrafish would not only be interesting regarding the epigenetic regulation of genes in general, but would at the same time give important insights into potential side-effects when developing therapies.
Expression profiling experiments have revealed that Wif1 is a downstream target of Wnt/beta-catenin signaling suggesting that Wif1 may act as a feedback inhibitor (Wissmann et al., 2003; Reguart et al., 2004; Vaes et al., 2005; Boerboom et al., 2006; Zirn et al., 2006; Kansara et al., 2009). Thus, Wif1 could be a central player in the dynamic control of Wnt signaling through a regulatory feedback mechanism. Wif1 also plays roles during embryonic development and some evidences collected mainly in mice and zebrafish implicate that Wif1 is similarly self-regulating its own expression during developmental processes (Diep et al., 2004; Yin et al., 2012). Such Wif1 regulatory feedback loops can involve hedgehog (Hh) such that Hh positively regulates Wif1 expression to inhibit Wnt signaling. In turn, Wnt signaling maintains Hh expression. This mechanism is important for swim bladder development in the zebrafish (Yin et al., 2012; Figure 1). Wif1 morpholino knockdown reduces cell proliferation resulting in defective swim bladder development such that epithelium and mesenchyme growth are inhibited, smooth muscle differentiation is abolished and the organization of mesothelium is perturbed.
Wif1 Expression and Function in Embryonic Development
Zebrafish Wif1 starts to be expressed in the presumptive paraxial mesoderm during late gastrulation (Thisse and Thisse, 2005). During subsequent neurulation stages Wif1 expression appears largely similar in Xenopus and zebrafish in the notochord, visceral arches, nasal placodes, swim bladder/lung, otic vesicles, somites (Xenopus), lateral line and corneal epithelium (zebrafish) and discrete domains of the brain (Hsieh et al., 1999; Thisse and Thisse, 2005; Yin et al., 2012; Lush and Piotrowski, 2014). In zebrafish, the latter comprise the ventral midbrain and developing dorsal diencephalon (Thisse and Thisse, 2005).
Similarly, in mammals and birds Wif1 expression initiates relatively late during development and is mainly restricted to the brain, lung, retina, and cartilage (Hsieh et al., 1999; Hunter et al., 2004; Hu et al., 2008; Surmann-Schmitt et al., 2009). In adult mice, Wif1 expression is retained in the heart and lung and also in the brain and eye, albeit at lower levels (Hsieh et al., 1999). The rather late onset of Wif1 expression might explain the subtle effects observed in Wif1 knock out mice, which exhibit accelerated development of radiation-induced osteosarcomas but no recognizable morphological malformations (Kansara et al., 2009). Only in more recent years, mammalian Wif1 was additionally implicated in lung development (Xu et al., 2011), tooth morphogenesis (Lee et al., 2015) and anorectal development (Ng et al., 2014; Figure 1).
The subtle impact on embryonic development and/or maintenance of embryonic structures caused by loss of Wif1 may rather be counterintuitive given its direct interaction with at least six different canonical and non-canonical Wnt ligands and several other proteins (Nakaya et al., 2008; Surmann-Schmitt et al., 2009, 2012). Indeed, forced early ectopic expression of Wif1 mRNA in the ventral blastomeres of the Xenopus embryo causes secondary axes typical for early inhibition of the canonical Wnt signaling cascade (Hsieh et al., 1999). However, Wif1 DNA overexpression leading to ectopic activation at later developmental stages after mid-blastula-transition induces a mild somite phenotype. Furthermore, so far no work has reported a role for Wif1 function in non-canonical Wnt signaling during embryonic development. Thus, Wif1 has the potential to regulate fundamental early processes during axis formation similar to, for instance, Dkk1 (Glinka et al., 1998). However, the onset of its expression mainly after gastrulation implicates that the Wif1/Wnt interaction may only fine-tune the spatial and temporal patterns of Wnt activity (Hsieh et al., 1999). Wif1 is discretely expressed in cells of tissues, in which Wnt morphogen gradients are at work. For instance, developing neuronal cells in the zebrafish dorsal diencephalon show Wif1 expression at developmental times, when Wnt3a is active in and around the adjacent mid-diencephalic organizing center to pattern the zebrafish thalamus (Thisse and Thisse, 2005; Mattes et al., 2012). In contrast, cells in the dorsal diencephalon anterior to the thalamus show Wnt activity only later during development (Hüsken et al., 2014). Thus, it is conceivable that particular cells in close vicinity to the Wnt source are protected from premature Wnt signaling – at least for a certain period of time. A Wif1/Wnt feedback regulation for the temporal control of Wnt signaling activity during neurogenesis would be a favorable mechanism to react to dynamic changes within a morphogen gradient.
Wif1 in Stem Cell Plasticity and as Therapeutic Target in the Eye
Enriched expression of Wif1 is reported in the cornea of the zebrafish (Thisse and Thisse, 2005), mouse (Davis and Piatigorsky, 2011), monkey (Ding et al., 2008) and human (Nakatsu et al., 2011) eye. Notably, Wif1 is predominantly expressed in the limbus of the cornea where limbal epithelial stem cells (LESCs) are located (Ding et al., 2008; Nakatsu et al., 2011). LESCs are important for the homeostasis and wound healing capability of the corneal epithelium and hence there is great interest in restoring LESC function in presently untreatable pathologic conditions where corneal healing is impaired (Yazdanpanah et al., 2017). The strong Wif1 expression in the limbal niche suggests a function in controlling the quiescent state of LESCs under normal physiological conditions (Nakatsu et al., 2011). Removal of Wif1 would allow activation of Wnt signaling associated with the high proliferative behavior of LESCs observed during wound healing and corneal regeneration (Nakatsu et al., 2011). Despite the evolutionarily conserved Wif1 expression in the cornea and lens (Thisse and Thisse, 2005), surprisingly no studies on the role of zebrafish Wif1 have been reported. However, the combination of transgenes, genetic mutants and in vivo time-lapse imaging to visualize dynamic processes under physiological conditions would almost certainly help understanding more about the pathophysiology of LESCs/Wif1/Wnt related diseases. For instance, inducible systems to transiently activate Wif1 in injured wild type or Wif1 mutant embryos, which carry additional transgenes like anillin and signaling reporter to simultaneously highlight cell proliferation (Paolini et al., 2015; Cepero Malo et al., 2017) and Wnt signaling activity (Moro et al., 2012) would be an excellent tool to study Wif1 dependent regeneration processes in vivo. The fact that cornea structure, development and maintenance appear comparable between zebrafish and mammals (Pan et al., 2013; Takamiya et al., 2015) should encourage to exploit the zebrafish as in vivo model to understand the role of Wif1 in corneal regeneration and homeostasis.
Wif1 is also expressed in early developing rod photoreceptors, and in the interphotoreceptor matrix of the mature retina (Hsieh et al., 1999; Hunter et al., 2004). Studies from Hsieh et al. suggest that Wif1 plays a role as opposing binding partner of Wnt4, providing a fine-tuning system for the regulation of rod photoreceptor production during development (Hsieh et al., 1999; Hunter et al., 2004). Dysregulated Wnt signaling has been linked to the pathophysiology of neovascular age-related macular degeneration (AMD) as well as diabetic retinopathy (DR) – leading causes of blindness in adults (Chen and Ma, 2017). Intriguingly, increased levels of Wif1 were found in the aqueous humor of patients with AMD (Park et al., 2014) as well as DR (Kim et al., 2007), suggesting that Wif1 provides an attractive candidate drug target to treat these eye disorders (Figure 1). Furthermore, increasing levels of Wif1 expression in the vitreous humor correlate with degrees of photoreceptor damage (Park et al., 2014). Alongside being a potential therapeutic target, these studies highlight Wif1 as candidate biomarker of retinal photoreceptor health and disease states.
Other Wif1 functions in the vertebrate eye involve its interaction with members of the Olfactomedin protein family. Within the zebrafish retina, the antagonistic interaction between Wif1 and Olfactomedin 1 in the extracellular space may fine tune retinal ganglion cell axon growth through modulation of Wnt signaling (Nakaya et al., 2008). In the human eye, Wif1 interaction with the Olfactomedin protein family member myocilin appears critical for the regulation of the intraocular pressure (IOP). High IOP in turn is a risk factor for glaucoma and myocilin has been linked to more than 10% of juvenile onset glaucoma cases, which result in the progressive degeneration of the optic nerve (Ortego et al., 1997; Kwon et al., 2009; Menaa et al., 2011).
Wif1 Regulation During Regeneration
Dynamic changes in Wif1 expression appear tightly associated to regenerative events in the brain and retina (Gonzalez-Fernandez et al., 2014; Lambert et al., 2016; Yao et al., 2016; Lim et al., 2017). The capability to regenerate lost or damaged neurons in response to injury is a key feature of the fish central nervous system. In the retina, this is achieved through activation and transient asymmetric proliferation of retinal Müller glial cells - the potential stem cells of the retina (Nagashima et al., 2013; Lust et al., 2016). Intriguingly, significant transcriptional down-regulation of Wif1 and concomitant activation of canonical Wnt signaling was observed in transiently proliferating Müller glial cells (Yao et al., 2016) and also during early stages of zebrafish brain regeneration (Lim et al., 2017; Figure 1). In contrast, damaging the mouse spinal cord appears to induce up-regulation of Wif1 and other Wnt antagonists (Gonzalez-Fernandez et al., 2014). These data suggest that Wnt signaling after injury might be the key for de novo neurogenesis, which is inhibited in the mammalian central nervous system with its limited regenerative capability (Gonzalez-Fernandez et al., 2014 and reviewed in Lambert et al., 2016). The different molecular responses to injury in zebrafish and mammals with respect to Wif1/Wnt signaling and regeneration open exciting possibilities for the development of novel therapeutic approaches to treat central nervous system injuries and possibly also neurodegenerative disorders. Recently, Wif1 has been implicated as potential molecular target of curcumin (Tiwari et al., 2016). Curcumin is a natural polyphenol product derived from the rhizome of the Indian spice turmeric (Curcuma longa) that appears to provide neuroprotection in cellular and animal models of neurodegenerative and neurological disorders (Pluta et al., 2015). The capability of curcumin to promote adult neurogenesis, neurite outgrowth and proliferation appears to occur through the interaction with Wif1 and concomitant activation of canonical Wnt signaling (Tiwari et al., 2015).
Concluding Remarks/Outlook
In summary, the structure and mechanistic underlying Wif1 function is rather well described. Epigenetic silencing of the Wif1 promoter is a common feature, frequently resulting in cancer progression when uncontrolled. Wif1 appears to fine-tune cellular processes, perhaps also temporally controlling them, by dynamically regulating the delicate balance of Wnt signaling via feedback loop activation. Indeed, an increasing number of studies link Wif1/Wnt signaling to different comparably subtle, yet important processes in development and disease, particularly in the eye. Furthermore, Wif1 downregulation and concomitant upregulation of Wnt signaling has been connected to regeneration processes in the zebrafish CNS, while spinal cord lesions in mammals with limited regeneration potential has the opposing effect on Wif1 expression. Combining the knowledge of the genetic and epigenetic feedback regulation of Wif1 with the regeneration capacities of the zebrafish nervous system has great potential to complement and aid research progress on neural regeneration in mammals.
Author Contributions
LP and MC had the original idea and outlined the review. All authors contributed to writing the article.
Conflict of Interest Statement
The authors declare that the research was conducted in the absence of any commercial or financial relationships that could be construed as a potential conflict of interest.
Acknowledgments
We would like to thank L. Guglielmi, A. Bühler and G. Covello for their work on Wif1. We are grateful to the Centre for Integrative Biology (CIBIO), University of Trento for financial support.
References
Aguilera, O., Fraga, M. F., Ballestar, E., Paz, M. F., Herranz, M., Espada, J., et al. (2006). Epigenetic inactivation of the Wnt antagonist DICKKOPF-1 (DKK-1) gene in human colorectal cancer. Oncogene 25, 4116–4121. doi: 10.1038/sj.onc.1209439
Avanesov, A., Honeyager, S. M., Malicki, J., and Blair, S. S. (2012). The role of glypicans in Wnt inhibitory factor-1 activity and the structural basis of Wif1’s effects on Wnt and hedgehog signaling. PLoS Genet. 8:e1002503. doi: 10.1371/journal.pgen.1002503
Beretta, C. A., Brinkmann, I., and Carl, M. (2011). All four zebrafish Wnt7 genes are expressed during early brain development. Gene Expr. Patterns 11, 277–284. doi: 10.1016/j.gep.2011.01.004
Boerboom, D., White, L. D., Dalle, S., Courty, J., and Richards, J. S. (2006). Dominant-stable beta-catenin expression causes cell fate alterations and Wnt signaling antagonist expression in a murine granulosa cell tumor model. Cancer Res. 66, 1964–1973. doi: 10.1158/0008-5472.CAN-05-3493
Bouwmeester, T., Kim, S., Sasai, Y., Lu, B., and De Robertis, E. M. (1996). Cerberus is a head-inducing secreted factor expressed in the anterior endoderm of Spemann’s organizer. Nature 382, 595–601. doi: 10.1038/382595a0
Cepero Malo, M., Duchemin, A. L., Guglielmi, L., Patzel, E., Sel, S., Auffarth, G. U., et al. (2017). The zebrafish anillin-eGFP reporter marks late dividing retinal precursors and stem cells entering neuronal lineages. PLoS One 12:e0170356. doi: 10.1371/journal.pone.0170356
Chen, Q., and Ma, J. X. (2017). Canonical Wnt signaling in diabetic retinopathy. Vis. Res. 139, 47–58. doi: 10.1016/j.visres.2017.02.007
Cruciat, C. M., and Niehrs, C. (2013). Secreted and transmembrane wnt inhibitors and activators. Cold Spring Harb. Perspect. Biol. 5:a015081. doi: 10.1101/cshperspect.a015081
Davis, J., and Piatigorsky, J. (2011). Overexpression of Pax6 in mouse cornea directly alters corneal epithelial cells: changes in immune function, vascularization, and differentiation. Invest. Ophthalmol. Vis. Sci. 52, 4158–4168. doi: 10.1167/iovs.10-6726
Diep, D. B., Hoen, N., Backman, M., Machon, O., and Krauss, S. (2004). Characterisation of the Wnt antagonists and their response to conditionally activated Wnt signalling in the developing mouse forebrain. Brain Res. Dev. Brain Res. 153, 261–270. doi: 10.1016/j.devbrainres.2004.09.008
Ding, Z., Dong, J., Liu, J., and Deng, S. X. (2008). Preferential gene expression in the limbus of the vervet monkey. Mol. Vis. 14, 2031–2041.
Elston, M. S., Gill, A. J., Conaglen, J. V., Clarkson, A., Shaw, J. M., Law, A. J., et al. (2008). Wnt pathway inhibitors are strongly down-regulated in pituitary tumors. Endocrinology 149, 1235–1242. doi: 10.1210/en.2007-0542
Fellenberg, J., Sahr, H., Liu, L., Schonsiegel, F., Depeweg, D., Lehner, B., et al. (2013). Rescue of silenced UCHL1 and IGFBP4 expression suppresses clonogenicity of giant cell tumor-derived stromal cells. Cancer Lett. 336, 61–67. doi: 10.1016/j.canlet.2013.04.011
Glinka, A., Wu, W., Delius, H., Monaghan, A. P., Blumenstock, C., and Niehrs, C. (1998). Dickkopf-1 is a member of a new family of secreted proteins and functions in head induction. Nature 391, 357–362. doi: 10.1038/34848
Glise, B., Miller, C. A., Crozatier, M., Halbisen, M. A., Wise, S., Olson, D. J., et al. (2005). Shifted, the Drosophila ortholog of Wnt inhibitory factor-1, controls the distribution and movement of Hedgehog. Dev. Cell 8, 255–266. doi: 10.1016/j.devcel.2005.01.003
Gonzalez-Fernandez, C., Fernandez-Martos, C. M., Shields, S. D., Arenas, E., and Javier Rodriguez, F. (2014). Wnts are expressed in the spinal cord of adult mice and are differentially induced after injury. J. Neurotrauma 31, 565–581. doi: 10.1089/neu.2013.3067
Gopal, G., Raja, U. M., Shirley, S., Rajalekshmi, K. R., and Rajkumar, T. (2013). SOSTDC1 down-regulation of expression involves CpG methylation and is a potential prognostic marker in gastric cancer. Cancer Genet. 206, 174–182. doi: 10.1016/j.cancergen.2013.04.005
Gorfinkiel, N., Sierra, J., Callejo, A., Ibanez, C., and Guerrero, I. (2005). The Drosophila ortholog of the human Wnt inhibitor factor Shifted controls the diffusion of lipid-modified Hedgehog. Dev. Cell 8, 241–253. doi: 10.1016/j.devcel.2004.12.018
Guo, H., Zhou, S., Tan, L., Wu, X., Wu, Z., and Ran, R. (2017). Clinicopathological significance of WIF1 hypermethylation in NSCLC, a meta-analysis and literature review. Oncotarget 8, 2550–2557. doi: 10.18632/oncotarget.13707
Herman, J. G., and Baylin, S. B. (2003). Gene silencing in cancer in association with promoter hypermethylation. N. Engl. J. Med. 349, 2042–2054. doi: 10.1056/NEJMra023075
Hoang, B., Moos, M. Jr., Vukicevic, S., and Luyten, F. P. (1996). Primary structure and tissue distribution of FRZB, a novel protein related to Drosophila frizzled, suggest a role in skeletal morphogenesis. J. Biol. Chem. 271, 26131–26137. doi: 10.1074/jbc.271.42.26131
Hsieh, J.-C., Kodjabachian, L., Rebbert, M. L., Rattner, A., Smallwood, P. M., Harryman Samos, C., et al. (1999). A new secreted protein that binds to Wnt proteins and inhibits their activites. Nature 398, 431–436. doi: 10.1038/18899
Hu, H., Li, B., Zhou, C., Ying, X., Chen, M., Huang, T., et al. (2018). Diagnostic value of WIF1 methylation for colorectal cancer: a meta-analysis. Oncotarget 9, 5378–5386. doi: 10.18632/oncotarget.23870
Hu, Y. A., Gu, X., Liu, J., Yang, Y., Yan, Y., and Zhao, C. (2008). Expression pattern of Wnt inhibitor factor 1(Wif1) during the development in mouse CNS. Gene Expr. Patterns 8, 515–522. doi: 10.1016/j.gep.2008.06.001
Hunter, D. D., Zhang, M., Ferguson, J. W., Koch, M., and Brunken, W. J. (2004). The extracellular matrix component WIF-1 is expressed during, and can modulate, retinal development. Mol. Cell. Neurosci. 27, 477–488. doi: 10.1016/j.mcn.2004.08.003
Hüsken, U., Stickney, H. L., Gestri, G., Bianco, I. H., Faro, A., Young, R. M., et al. (2014). Tcf7l2 is required for left-right asymmetric differentiation of habenular neurons. Curr. Biol. 24, 2217–2227. doi: 10.1016/j.cub.2014.08.006
Itasaki, N., Jones, C. M., Mercurio, S., Rowe, A., Domingos, P. M., Smith, J. C., et al. (2003). Wise, a context-dependent activator and inhibitor of Wnt signalling. Development 130, 4295–4305. doi: 10.1242/dev.00674
Kansara, M., Tsang, M., Kodjabachian, L., Sims, N. A., Trivett, M. K., Ehrich, M., et al. (2009). Wnt inhibitory factor 1 is epigenetically silenced in human osteosarcoma, and targeted disruption accelerates osteosarcomagenesis in mice. J. Clin. Invest. 119, 837–851. doi: 10.1172/JCI37175
Kawano, Y., and Kypta, R. (2003). Secreted antagonists of the Wnt signalling pathway. J. Cell Sci. 116, 2627–2634. doi: 10.1242/jcs.00623
Kim, T., Kim, S. J., Kim, K., Kang, U. B., Lee, C., Park, K. S., et al. (2007). Profiling of vitreous proteomes from proliferative diabetic retinopathy and nondiabetic patients. Proteomics 7, 4203–4215. doi: 10.1002/pmic.200700745
Kongkham, P. N., Northcott, P. A., Croul, S. E., Smith, C. A., Taylor, M. D., and Rutka, J. T. (2010). The SFRP family of WNT inhibitors function as novel tumor suppressor genes epigenetically silenced in medulloblastoma. Oncogene 29, 3017–3024. doi: 10.1038/onc.2010.32
Kwon, H. S., Lee, H. S., Ji, Y., Rubin, J. S., and Tomarev, S. I. (2009). Myocilin is a modulator of Wnt signaling. Mol. Cell. Biol. 29, 2139–2154. doi: 10.1128/mcb.01274-08
Lambert, C., Cisternas, P., and Inestrosa, N. C. (2016). Role of Wnt signaling in central nervous system injury. Mol. Neurobiol. 53, 2297–2311. doi: 10.1007/s12035-015-9138-x
Lee, M. J., Kim, E. J., Li, L., and Jung, H. S. (2015). Roles of Wnt inhibitory factor 1 during tooth morphogenesis. Cell Tissue Res. 362, 61–68. doi: 10.1007/s00441-015-2170-3
Li, X., Zhang, Y., Kang, H., Liu, W., Liu, P., Zhang, J., et al. (2005). Sclerostin binds to LRP5/6 and antagonizes canonical Wnt signaling. J. Biol. Chem. 280, 19883–19887. doi: 10.1074/jbc.M413274200
Lim, F. T., Ogawa, S., Smith, A. I., and Parhar, I. S. (2017). Proteomics identification of potential candidates involved in cell proliferation for early stage of brain regeneration in the adult zebrafish. Zebrafish 14, 10–22. doi: 10.1089/zeb.2016.1319
Lush, M. E., and Piotrowski, T. (2014). ErbB expressing Schwann cells control lateral line progenitor cells via non-cell-autonomous regulation of Wnt/beta-catenin. eLife 3:e01832. doi: 10.7554/eLife.01832
Lust, K., Sinn, R., Perez Saturnino, A., Centanin, L., and Wittbrodt, J. (2016). De novo neurogenesis by targeted expression of atoh7 to Muller glia cells. Development 143, 1874–1883. doi: 10.1242/dev.135905
Malinauskas, T., Aricescu, A. R., Lu, W., Siebold, C., and Jones, E. Y. (2011). Modular mechanism of Wnt signaling inhibition by Wnt inhibitory factor 1. Nat. Struct. Mol. Biol. 18, 886–893. doi: 10.1038/nsmb.2081
Malinauskas, T., and Jones, E. Y. (2014). Extracellular modulators of Wnt signalling. Curr. Opin. Struct. Biol. 29, 77–84. doi: 10.1016/j.sbi.2014.10.003
Mattes, B., Weber, S., Peres, J., Chen, Q., Davidson, G., Houart, C., et al. (2012). Wnt3 and Wnt3a are required for induction of the mid-diencephalic organizer in the caudal forebrain. Neural Dev. 7:12. doi: 10.1186/1749-8104-7-12
Mazieres, J., He, B., You, L., Xu, Z., Lee, A. Y., Mikami, I., et al. (2004). Wnt inhibitory factor-1 is silenced by promoter hypermethylation in human lung cancer. Cancer Res. 64, 4717–4720. doi: 10.1158/0008-5472.can-04-1389
Menaa, F., Braghini, C. A., Vasconcellos, J. P., Menaa, B., Costa, V. P., Figueiredo, E. S., et al. (2011). Keeping an eye on myocilin: a complex molecule associated with primary open-angle glaucoma susceptibility. Molecules 16, 5402–5421. doi: 10.3390/molecules16075402
Moro, E., Ozhan-Kizil, G., Mongera, A., Beis, D., Wierzbicki, C., Young, R. M., et al. (2012). In vivo Wnt signaling tracing through a transgenic biosensor fish reveals novel activity domains. Dev. Biol. 366, 327–340. doi: 10.1016/j.ydbio.2012.03.023
Nagashima, M., Barthel, L. K., and Raymond, P. A. (2013). A self-renewing division of zebrafish muller glial cells generates neuronal progenitors that require N-cadherin to regenerate retinal neurons. Development 140, 4510–4521. doi: 10.1242/dev.090738
Nakatsu, M. N., Ding, Z., Ng, M. Y., Truong, T. T., Yu, F., and Deng, S. X. (2011). Wnt/beta-catenin signaling regulates proliferation of human cornea epithelial stem/progenitor cells. Invest. Ophthalmol. Vis. Sci. 52, 4734–4741. doi: 10.1167/iovs.10-6486
Nakaya, N., Lee, H. S., Takada, Y., Tzchori, I., and Tomarev, S. I. (2008). Zebrafish olfactomedin 1 regulates retinal axon elongation in vivo and is a modulator of Wnt signaling pathway. J. Neurosci. 28, 7900–7910. doi: 10.1523/jneurosci.0617-08.2008
Ng, R. C., Matsumaru, D., Ho, A. S., Garcia-Barcelo, M. M., Yuan, Z. W., Smith, D., et al. (2014). Dysregulation of Wnt inhibitory factor 1 (Wif1) expression resulted in aberrant Wnt-beta-catenin signaling and cell death of the cloaca endoderm, and anorectal malformations. Cell Death Differ. 21, 978–989. doi: 10.1038/cdd.2014.20
Niehrs, C. (2006). Function and biological roles of the Dickkopf family of Wnt modulators. Oncogene 25, 7469–7481. doi: 10.1038/sj.onc.1210054
Nusse, R., and Clevers, H. (2017). Wnt/beta-catenin signaling, disease, and emerging therapeutic modalities. Cell 169, 985–999. doi: 10.1016/j.cell.2017.05.016
Ortego, J., Escribano, J., and Coca-Prados, M. (1997). Cloning and characterization of subtracted cDNAs from a human ciliary body library encoding TIGR, a protein involved in juvenile open angle glaucoma with homology to myosin and olfactomedin. FEBS Lett. 413, 349–353. doi: 10.1016/S0014-5793(97)00934-4
Pan, Y. A., Freundlich, T., Weissman, T. A., Schoppik, D., Wang, X. C., Zimmerman, S., et al. (2013). Zebrabow: multispectral cell labeling for cell tracing and lineage analysis in zebrafish. Development 140, 2835–2846. doi: 10.1242/dev.094631
Panakova, D., Sprong, H., Marois, E., Thiele, C., and Eaton, S. (2005). Lipoprotein particles are required for Hedgehog and Wingless signalling. Nature 435, 58–65. doi: 10.1038/nature03504
Paolini, A., Duchemin, A. L., Albadri, S., Patzel, E., Bornhorst, D., Gonzalez Avalos, P., et al. (2015). Asymmetric inheritance of the apical domain and self-renewal of retinal ganglion cell progenitors depend on Anillin function. Development 142, 832–839. doi: 10.1242/dev.118612
Park, K. H., Choi, A. J., Yoon, J., Lim, D., Woo, S. J., Park, S. J., et al. (2014). Wnt modulators in the aqueous humor are associated with outer retinal damage severity in patients with neovascular age-related macular degeneration. Invest. Ophthalmol. Vis. Sci. 55, 5522–5530. doi: 10.1167/iovs.14-14566
Pluta, R., Bogucka-Kocka, A., Ulamek-Koziol, M., Furmaga-Jablonska, W., Januszewski, S., Brzozowska, J., et al. (2015). Neurogenesis and neuroprotection in postischemic brain neurodegeneration with Alzheimer phenotype: is there a role for curcumin? Folia Neuropathol. 53, 89–99.
Ramachandran, I., Thavathiru, E., Ramalingam, S., Natarajan, G., Mills, W. K., Benbrook, D. M., et al. (2012). Wnt inhibitory factor 1 induces apoptosis and inhibits cervical cancer growth, invasion and angiogenesis in vivo. Oncogene 31, 2725–2737. doi: 10.1038/onc.2011.455
Reguart, N., He, B., Xu, Z., You, L., Lee, A. Y., Mazieres, J., et al. (2004). Cloning and characterization of the promoter of human Wnt inhibitory factor-1. Biochem. Biophys. Res. Commun. 323, 229–234. doi: 10.1016/j.bbrc.2004.08.075
Roman-Gomez, J., Jimenez-Velasco, A., Barrios, M., Prosper, F., Heiniger, A., Torres, A., et al. (2007). Poor prognosis in acute lymphoblastic leukemia may relate to promoter hypermethylation of cancer-related genes. Leuk. Lymphoma 48, 1269–1282. doi: 10.1080/10428190701344899
Sato, H., Suzuki, H., Toyota, M., Nojima, M., Maruyama, R., Sasaki, S., et al. (2007). Frequent epigenetic inactivation of DICKKOPF family genes in human gastrointestinal tumors. Carcinogenesis 28, 2459–2466. doi: 10.1093/carcin/bgm178
Semenov, M., Tamai, K., and He, X. (2005). SOST is a ligand for LRP5/LRP6 and a Wnt signaling inhibitor. J. Biol. Chem. 280, 26770–26775. doi: 10.1074/jbc.M504308200
Steinhart, Z., and Angers, S. (2018). Wnt signaling in development and tissue homeostasis. Development 145:dev146589. doi: 10.1242/dev.146589
Surmann-Schmitt, C., Sasaki, T., Hattori, T., Eitzinger, N., Schett, G., von der Mark, K., et al. (2012). The Wnt antagonist Wif-1 interacts with CTGF and inhibits CTGF activity. J. Cell. Physiol. 227, 2207–2216. doi: 10.1002/jcp.22957
Surmann-Schmitt, C., Widmann, N., Dietz, U., Saeger, B., Eitzinger, N., Nakamura, Y., et al. (2009). Wif-1 is expressed at cartilage-mesenchyme interfaces and impedes Wnt3a-mediated inhibition of chondrogenesis. J. Cell Sci. 122(Pt 20), 3627–3637. doi: 10.1242/jcs.048926
Tai, D., Wells, K., Arcaroli, J., Vanderbilt, C., Aisner, D. L., Messersmith, W. A., et al. (2015). Targeting the WNT signaling pathway in cancer therapeutics. Oncologist 20, 1189–1198. doi: 10.1634/theoncologist.2015-0057
Takamiya, M., Weger, B. D., Schindler, S., Beil, T., Yang, L., Armant, O., et al. (2015). Molecular description of eye defects in the zebrafish Pax6b mutant, sunrise, reveals a Pax6b-dependent genetic network in the developing anterior chamber. PLoS One 10:e0117645. doi: 10.1371/journal.pone.0117645
Tan, M., Wu, J., and Cai, Y. (2013). Suppression of Wnt signaling by the miR-29 family is mediated by demethylation of WIF-1 in non-small-cell lung cancer. Biochem. Biophys. Res. Commun. 438, 673–679. doi: 10.1016/j.bbrc.2013.07.123
Thisse, C., and Thisse, B. (2005). High Throughput Expression Analysis of ZF-Models Consortium Clones. ZFIN Direct Data Submission. Available at: http://zfin.org
Tiwari, S. K., Agarwal, S., Seth, B., Yadav, A., Ray, R. S., Mishra, V. N., et al. (2015). Inhibitory effects of Bisphenol-A on neural stem cells proliferation and differentiation in the rat brain are dependent on Wnt/beta-Catenin pathway. Mol. Neurobiol. 52, 1735–1757. doi: 10.1007/s12035-014-8940-1
Tiwari, S. K., Agarwal, S., Tripathi, A., and Chaturvedi, R. K. (2016). Bisphenol-A mediated inhibition of hippocampal neurogenesis attenuated by curcumin via canonical wnt pathway. Mol. Neurobiol. 53, 3010–3029. doi: 10.1007/s12035-015-9197-z
Vaes, B. L., Dechering, K. J., van Someren, E. P., Hendriks, J. M., van de Ven, C. J., Feijen, A., et al. (2005). Microarray analysis reveals expression regulation of Wnt antagonists in differentiating osteoblasts. Bone 36, 803–811. doi: 10.1016/j.bone.2005.02.001
Willert, K., Brown, J. D., Danenberg, E., Duncan, A. W., Weissman, I. L., Reya, T., et al. (2003). Wnt proteins are lipid-modified and can act as stem cell growth factors. Nature 423, 448–452. doi: 10.1038/nature01611
Wissmann, C., Wild, P. J., Kaiser, S., Roepcke, S., Stoehr, R., Woenckhaus, M., et al. (2003). WIF1, a component of the Wnt pathway, is down-regulated in prostate, breast, lung, and bladder cancer. J. Pathol. 201, 204–212. doi: 10.1002/path.1449
Xu, B., Chen, C., Chen, H., Zheng, S. G., Bringas, P. J., Xu, M., et al. (2011). Smad1 and its target gene Wif1 coordinate BMP and Wnt signaling activities to regulate fetal lung development. Development 138, 925–935. doi: 10.1242/dev.062687
Yan, D., and Lin, X. (2009). Shaping morphogen gradients by proteoglycans. Cold Spring Harb. Perspect. Biol. 1:a002493. doi: 10.1101/cshperspect.a002493
Yao, K., Qiu, S., Tian, L., Snider, W. D., Flannery, J. G., Schaffer, D. V., et al. (2016). Wnt regulates proliferation and neurogenic potential of muller glial cells via a Lin28/let-7 miRNA-dependent pathway in adult mammalian retinas. Cell Rep. 17, 165–178. doi: 10.1016/j.celrep.2016.08.078
Yazdanpanah, G., Jabbehdari, S., and Djalilian, A. R. (2017). Limbal and corneal epithelial homeostasis. Curr. Opin. Ophthalmol. 28, 348–354. doi: 10.1097/icu.0000000000000378
Yee, D. S., Tang, Y., Li, X., Liu, Z., Guo, Y., Ghaffar, S., et al. (2010). The Wnt inhibitory factor 1 restoration in prostate cancer cells was associated with reduced tumor growth, decreased capacity of cell migration and invasion and a reversal of epithelial to mesenchymal transition. Mol. Cancer 9:162. doi: 10.1186/1476-4598-9-162
Yin, A., Korzh, V., and Gong, Z. (2012). Perturbation of zebrafish swimbladder development by enhancing Wnt signaling in Wif1 morphants. BBA Mol. Cell Res. 1832, 236–244. doi: 10.1016/j.bbamcr.2011.09.018
Zheng, Y., Li, X., Jiang, Y., Xu, Y., Song, B., Zhou, Q., et al. (2016). Promoter hypermethylation of Wnt inhibitory factor-1 in patients with lung cancer: a systematic meta-analysis. Medicine 95:e5433. doi: 10.1097/md.0000000000005433
Zhu, W., Shiojima, I., Ito, Y., Li, Z., Ikeda, H., Yoshida, M., et al. (2008). IGFBP-4 is an inhibitor of canonical Wnt signalling required for cardiogenesis. Nature 454, 345–349. doi: 10.1038/nature07027
Keywords: Wif1, Wnt signaling, zebrafish, eye, retina, cancer
Citation: Poggi L, Casarosa S and Carl M (2018) An Eye on the Wnt Inhibitory Factor Wif1. Front. Cell Dev. Biol. 6:167. doi: 10.3389/fcell.2018.00167
Received: 28 September 2018; Accepted: 23 November 2018;
Published: 06 December 2018.
Edited by:
Isaac Henry Bianco, University College London, United KingdomReviewed by:
Filippo Del Bene, Institut Curie, FranceQueeLim Ch’ng, King’s College London, United Kingdom
Copyright © 2018 Poggi, Casarosa and Carl. This is an open-access article distributed under the terms of the Creative Commons Attribution License (CC BY). The use, distribution or reproduction in other forums is permitted, provided the original author(s) and the copyright owner(s) are credited and that the original publication in this journal is cited, in accordance with accepted academic practice. No use, distribution or reproduction is permitted which does not comply with these terms.
*Correspondence: Lucia Poggi, bHVjaWEucG9nZ2lAdW5pdG4uaXQ= Matthias Carl, bWF0dGhpYXMuY2FybEB1bml0bi5pdA==