- 1Departamento de Anatomía y Biología Celular and IDIVAL, Universidad de Cantabria, Santander, Spain
- 2Departamento de Anatomía, Biología Celular, y Zoología, Universidad de Extremadura, Badajoz, Spain
- 3Departamento de Medicina Genómica y Toxicología Ambiental, Instituto de Investigaciones Biomédicas, Universidad Nacional Autónoma de México, Mexico City, Mexico
In tetrapods the digit pattern has evolved to adapt to distinct locomotive strategies. The number of digits varies between species or even between hindlimb and forelimb within the same species. These facts illustrate the plasticity of embryonic limb autopods. Sox9 is a precocious marker of skeletal differentiation of limb mesenchymal cells. Its pattern of expression in the developing limb has been widely studied and reflects the activity of signaling cascades responsible for skeletogenesis. In this assay we stress previously overlooked differences in the pattern of expression of Sox9 in limbs of avian, mouse and turtle embryos which may reflect signaling differences associated with distinct limb skeletal morphologies observed in these species. Furthermore, we show that Sox9 gene expression is higher and maintained in the interdigital region in species with webbed digits in comparison with free digit animals.
The limb is an excellent model system to study the molecular basis of morphogenesis (Hinchliffe, 2002; Fabrezi et al., 2007). The skeletal pattern of the limb is conserved in tetrapods, yet differences in bone morphology are remarkable among different species (Kavanagh et al., 2013). Interpretations of skeletal limb diversification has been largely based on comparative developmental studies using histochemical or radiolabeling markers of initial stages of cartilage differentiation. From these approaches it has been proposed that the limb skeleton in tetrapods is generated by sequential branching and segmentation of a basic pattern representative of the distal segment of the fish fins, termed the “metapterygial axis.” The advent and progress of molecular biology has provided new insights about the diversification of the limb skeletal morphology. For example, it has been shown that activation of signals responsible for skeletogenesis may be differentially regulated by transcriptional enhancer DNA sequences that are species-specific (Kvon et al., 2016). These studies explain major skeletal differences in evolutionary distant species such as the absence of limbs in snakes. However, differences between the fore- and the hind-limb in the same species or skeletal differences observed among closely related tetrapods might be regulated in a different fashion, such as timing differences in the expression of signaling molecules (Richardson et al., 2009; Moore et al., 2015; Zuniga, 2015).
Sox9 is a well known marker of the skeleton that precedes the appearance of cartilage blastemas (Wright et al., 1995; Healy et al., 1999; Chimal-Monroy et al., 2003; Kawakami et al., 2005; Lorda-Diez et al., 2011; Sensiate et al., 2014). Hence, Sox9 is expressed even in domains that represent skeletal pieces lost in the course of evolution of specialized species (de Bakker et al., 2013). Silencing Sox9 in mouse embryos causes loss of appendicular skeleton and increases programmed cell death (Akiyama et al., 2002). Sox9 overexpression promotes polydactyly (Akiyama et al., 2007). Furthermore, Sox9 along with BMP and WNT signaling are considered key regulators of digits formation through a self-organizing Turing mechanism (Raspopovic et al., 2014). Overall, such findings make Sox9 an excellent marker to detect signaling differences, later transduced into specific patterns of chondrification (Richardson et al., 2009), responsible for variations in the morphology of the appendicular skeleton. Based on the observation of in situ hybridizations, we have revised the pattern of Sox9 gene expression during digit development in reptilian (Mauremys turtle), avian (chick and duck), and mammal (mouse) species with different autopodial morphology to uncover signaling differences of potential interest to explain digit morphogenesis.
In chick embryos the expression of Sox9 shows differences between the wing (Figures 1A–G) and the leg bud (Figures 1H–M). In wing buds at stage HH22 (3.5 id) the expression of Sox9 marks the primary axis of the appendicular skeleton. In next stages, the initial domain extends proximally and distally (Figures 1A–C). Proximally, the domain forms the humerus primordium, and distally it shows a branching that establishes the primordium of the radius (Figures 1C,D). By stage HH24 (4 id) the primary axis is continued distally by the digital arch oriented toward the anterior margin of the bud. Between stages HH26-HH28 the digital arch undergoes a branching process to form each digit (Figures 1E–G). First branching forms digit 3 and a common branch that bifurcates to form digit 4, and a reduced domain reminiscent of a digit 5. The latest, is progressively reduced in size and expression intensity. The most anterior digit, is formed distally and aligned with the radial domain (Figures 1F,G).
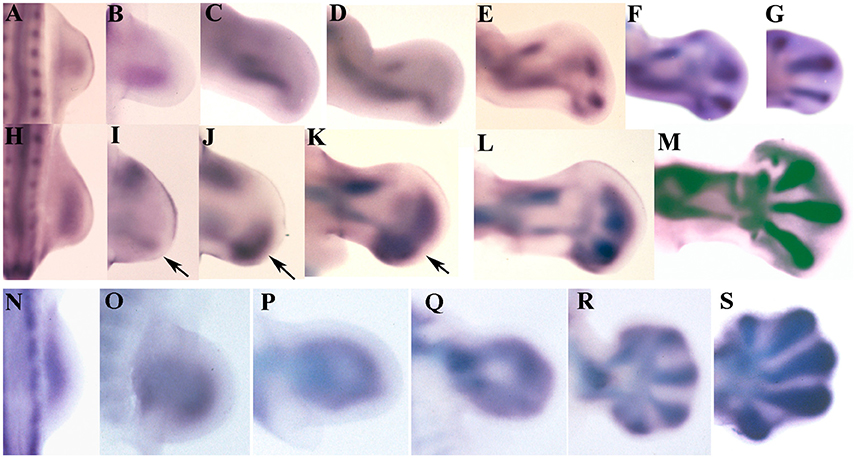
Figure 1. Sox9 expression during limb development of chicken and mouse embryos. (A–G) Embryonic chicken wing buds at stages HH20 (A), HH22 (B), HH24 (C), HH 25 (D), HH26 (E), HH27 (F), and HH28 (G). (H–M) Chicken leg buds at stages HH20 (H), HH22 (I), HH23 (J), HH25 (K), HH27 (L), HH28 (M). Arrows indicate the position of the digital arch domain. (N–S) Mouse forelimbs illustrating the sequence of Sox9 expression at stages E9,5 (N), E10 (O), E10,5 (P), E11 (Q), E12 (R), and E13 (S). Anterior is to the top and distal to the right in all images.
In the leg bud the initial expression of Sox9 at stage HH22 appears divided into a posterior (primary axis) and an anterior domain for the tibia (Figures 1H,I). The femur is identifiable at stage HH25 coupled between the proximal end of the fibular and tibial domains (Figure 1K). The appearance of these skeletal domains at stage HH23 is accompanied by the formation of a nascent digital arch that occupies a posterior and distal position (Figures 1K,L). Initially, the expression is uniform and limited to the posterior half of the autopod but, in the following stages (HH25 and HH26), the expression progresses anteriorly and digits became identifiable as patches of higher expression (Figures 1J–M). Digits 3 and 4 are the most prominent at these stages while digits 2 and 5 are poorly defined areas where the expression of Sox9 is not very intense. Interestingly, the most anterior part of the autopod lacks Sox9 transcripts until stage HH26-HH27.
Both in the wing and in the leg bud, concomitantly with the intensification of Sox9 expression at stage HH26 in the digit blastemas, a carpal/tarsal arch of lower Sox9 expression level is formed. Carpal and tarsal pre-cartilages are individualized when digit blastemas are defined.
Expression of Sox9 in the mouse is similar in fore- and hind-limbs (Figures 1N–S). Initial expression of Sox9 occupies the whole central region of the early bud (Figures 1N,O). Regionalization of this domain in stylopod, zeugopod and digital arch is due to the loss of transcripts from the central region at 10.5 pc (Figures 1P,Q). Due to this process, expression of Sox9 appears as a loop where the distal curved region constitutes the digital arch. The proximal part of the loop lengthens marking the position of the stylopod. The zeugopodial elements are identified as the lateral regions of the loop. In next stages the digit primordia appear as elongated domains of intensified Sox9 gene expression (Figures 1R,S). Digits 3 and 4 are the first to appear.
The skeletal domains of Sox9 in the Mauremys turtle are similar in fore and hind-limbs (Figure 2). At the beginning, a central ill-defined domain is transformed into a triangular domain with a posterior elongated vertex, which marks the stylopod (Figure 2C). The sides of the triangle form the zeugopodial domains, and the base corresponds with the digital arch. The expression of Sox9 in the digital arch becomes progressively intensified at discrete regions to form digit primordia (Figures 2D,E). Digits 3 and 4 are the most precociously identifiable while digit 1 is the last to appear, preceded by digit 5 (Figures 2E–I). In the course of digit development, the expression of Sox9 is progressively restricted to the digit tip and to the developing joints (Figures 2E–I).
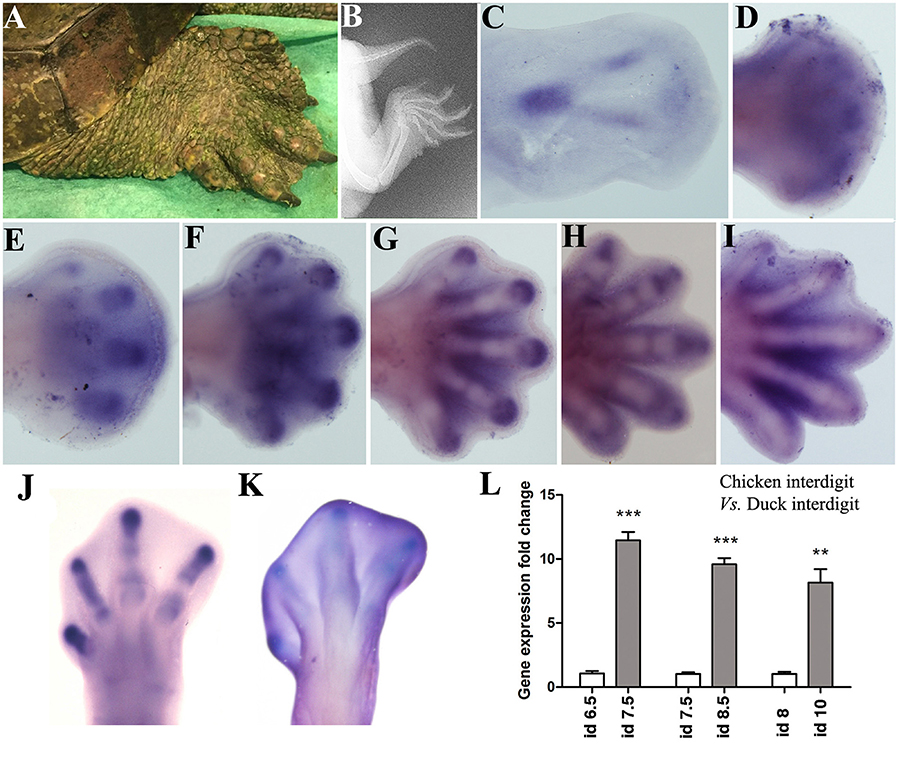
Figure 2. Sox9 expression during limb development in the turtle (Mauremys Leprosa). Stages were established according to the developmental series of Yntema (1968). (A,B) adult limb in a live picture (A) and a radiographic image (B) to illustrate the presence of interdigital membranes in this species. (C–I) Sox9 expression at stages Y14 (C), Y15 (D), Y16 (E), Y17 (F), Y18 (G), Y19 (H), and Y20 (I). (J–L) Comparative analysis of Sox9 expression in chick and duck interdigits. (J,K) Sox9 expression in the chicken and duck autopod at day 6.5 and 8 of incubation respectively. (L) QPCR comparison of Sox9 expression level in the developing third interdigit of the leg bud of chicken (white bars) and duck (gray bars) embryos at equivalent developmental stages. Each value represents the mean of three samples of 12 interdigits and statistical significance was set at P < 0.05. Incubation days (id) from left to right: chicken id 6.5 vs. duck id 7.5; chicken id 7.5 vs. duck 8.5; and chicken id 8 vs. duck id 10. Q-PCR specific primers were designed searching for identical homologous sequences in the duck and chicken for Sox9 and GAPDH genes. **p ≤ 0.01; ***p ≤ 0.001.
Remarkably, the Mauremys turtle interdigital regions retain considerable levels of Sox9 expression not observed in chick and mouse embryos (Figures 2F–I). To ascertain if interdigital expression of Sox9 associates with the absence of interdigit remodeling in the Mauremys turtle (Figure 2A), we compared the level of expression of Sox9 in the third interdigit of the leg bud of chick and duck embryos, as characteristic models of species with free and webbed digits respectively. As shown in Figures 2J–L, expression of Sox9 in the non-regressing interdigit of the duck was much higher than that of the chick embryo.
Detailed analysis of phylogenetically related but phenotypically different species have provided important cues about the mechanisms accounting for limb morphogenesis (Moore et al., 2015). Gene expression computational modeling have also provided insights on the molecular bases responsible for differences in limb skeletogenesis among vertebrates (Uzkudun et al., 2015). The consideration of the subtle Sox9 expression differences highlighted in this “perspective” assay, are consistent with heterochrony detected in the stages of chondrification (Richardson et al., 2009), and may help to improve our understanding of how digits differ in morphology. In all species, the expression of Sox9 marks the successive appearance of the stylopod, zeugopod, and autopod along the proximo-distal axis of the limb. The autopod includes the mesopodium (carpi/tarsi) and the acropod (digits); the specification of zeugopod and the acropod has been proposed to determine the mesopodial intermediate domain in between (Woltering and Duboule, 2010). Consistent with this hypothesis, the appearance of intensified expression of Sox9 marking the nascent digits precedes that of the carpal/tarsal domains that lie in the concavity of the digit arch.
The formation and expansion of the digit arch in the chick embryo is clearly distinct from that observed in mouse and turtle embryos. Consistent with the evolutionary model proposed by Shubin and Alberch (1986), in the avian limb the progressive appearance of the digit expression domains follows a polarized sequence from posterior to anterior, which is more accentuated in the wing bud. In contrast, the digital arch domain in mouse and turtle limbs appears occupying a central position in the autopod, and in the course of development expands uniformly to the margins of the bud. These differences raise doubts about the validity of current thought, which considers independent identities for each of the digits in the hand/foot of vertebrates. The consideration of such identities have implications for evolutionary hypothesis that consider digit 1, as the most distal element of a conserved skeletal axis modified in the course of evolution through branching and segmentation processes (see Cohn et al., 2002, for discussion). Sox9 expression domains precede the appearance of prechondrogenic blastemas that were formerly employed in traditional comparative embryonic studies. Hence, the differences among species observed here, support mechanisms of skeletal diversification based on the combination of a distinct distribution of signals with differences in the intrinsic properties of the skeletal progenitors of the autopod, likely associated with differential epigenetic signatures (Sheth et al., 2016). Both in the wing and in the leg of avian embryos, digits are different along the antero-posterior axis justifying the consideration of different digit identities according to their position and number of phalanxes. In contrast, digits of mouse and turtle embryos, expands from the centrally located digital arch toward the margins of the limb bud. In this model of digit arch expansion, there are not morphological landmarks that allow to assign specific identities to the central digits (2,3,4). It must be taken into account that the carpal/tarsal domains of Sox9 appear when the digit arch shows independent digit domains. Therefore, at these embryonic stages mesopodial domains cannot be taken as a primary reference to establish the identity of the digits. The only morphological differences observed among the pre-cartilaginous blastemas are located in the marginal digits (digits 1 and 5) where Sox9 domains exhibit a reduced size and appear at more advanced stages than the central digits.
The growth of the limb bud is regulated by a complex signaling network (Uzkudun et al., 2015), where Shh and Gremlin1 genes play an important role in digit specification (Sanz-Ezquerro and Tickle, 2003a; Zhu et al., 2008). Evolutionary or genetic deregulations of the Shh/Gremlin loop causes polydactylous (Norrie et al., 2014) or oligodactylous (Lopez-Rios et al., 2014) autopods. Consistent with our interpretation, central digits in these mutants, regardless of its number, are identical and indistinguishable from each other (Norrie et al., 2014). These findings make plausible that digit formation result of the self-organization of the limb mesenchyme (Cooper, 2015), within an autopod of dimensions and shape finely tuned by regulatory genes responsible for growth (Zhu et al., 2008).
Sox9 is target of signals controlling proliferation and differentiation of the skeletal progenitors, including FGFs, BMPs, TGFbetas, and Retinoic acid (RA). These signals are themselves closely regulated by the AER and the ZPA, to establish the pattern of limb skeletogenesis as well as the number of digits in the autopod. BMPs up-regulate the expression of Sox9 and promote differentiation of progenitors (Lorda-Diez et al., 2014; Norrie et al., 2014) and in conjunction with TGFβs and Activins induce the formation of extra-digits in the avian limb (Chimal-Monroy et al., 2003; Montero et al., 2008). FGFs are major determinants of digit size (Sanz-Ezquerro and Tickle, 2003b; Seki et al., 2015). FGFs inhibit chondrogenesis but expand the amount of Sox 9 positive skeletal progenitors and its overexpression in the limb results in the formation of extra cartilages, including extra-digits (Montero et al., 2001; Norrie et al., 2014), or extra-phalanxes (Sanz-Ezquerro and Tickle, 2003b). RA is a potent inhibitor of Sox9 gene expression (Weston et al., 2002) and RA inhibition in the autopod causes the formation of extra digits (Rodriguez-Leon et al., 1999). Hence, the pattern of Sox9 gene expression may reflect differences in the spatial distribution of signals within the limb bud mesoderm. According with this interpretation, avian digits may represent an evolutionary specialization of digit development consequence of a posterior polarization of signals responsible for limb outgrowth. In contrast, the pentadactyl autopod of mouse and turtle embryos may result from the uniform expansion (like opening a fan) of the signals that coordinate proliferation and differentiation of the skeletal progenitors.
Ethics Statement
The animal care and handling, and all the experimental procedures were in accordance with the guidelines of the European Communities Council and the Spanish legislation and they were approved by the Service of Animal Health and Welfare of the Regional Government of Cantabria (Reference No. PI-10-15).
Author Contributions
Conceived and designed the experiments: CL, JF, JG, JC, and JH. Performed the experiments: CL, JM, JF, JC, and JH. Analyzed the data: CL, JM, JG, JC, and JH. Writing of the manuscript: JM and JH.
Conflict of Interest Statement
The authors declare that the research was conducted in the absence of any commercial or financial relationships that could be construed as a potential conflict of interest.
Acknowledgments
Thanks are due to Prof. Virginio García-Martínez for help and suggestions. Supported by Grants BFU2014-54026P from the Spanish Science and Innovation Ministry to JH and NextVal NVAL15-07 from the IDIVAL to CL. Figure 1L has been previously published by Chimal-Monroy et al. (2003).
References
Akiyama, H., Chaboissier, M. C., Martin, J. F., Schedl, A., and de Crombrugghe, B. (2002). The transcription factor Sox9 has essential roles in successive steps of the chondrocyte differentiation pathway and is required for expression of Sox5 and Sox6. Genes Dev. 16, 2813–2828. doi: 10.1101/gad.1017802
Akiyama, H., Stadler, H. S., Martin, J. F., Ishii, T. M., Beachy, P. A., Nakamura, T., et al. (2007). Misexpression of Sox9 in mouse limb bud mesenchyme induces polydactyly and rescues hypodactyly mice. Matrix Biol. 26, 224–233. doi: 10.1016/j.matbio.2006.12.002
Chimal-Monroy, J., Rodriguez-Leon, J., Montero, J. A., Gañan, Y., Macias, D., Merino, R., et al. (2003). Analysis of the molecular cascade responsible for mesodermal limb chondrogenesis: sox genes and BMP signaling. Dev. Biol. 257, 292–301. doi: 10.1016/S0012-1606(03)00066-6
Cohn, M. J., Lovejoy, C. O., Wolpert, L., and Coates, M. I. (2002). Branching, segmentation and the metapterygial axis: pattern versus process in the vertebrate limb. Bioessays 24, 460–465. doi: 10.1002/bies.10088
Cooper, K. L. (2015). Self-organization in the limb: a turing mechanism for digit development. Curr. Opin. Genet. Dev. 32, 92–97. doi: 10.1016/j.gde.2015.02.001
de Bakker, M. A., Fowler, D. A., den Oude, K., Dondorp, E. M., Navas, M. C., Horbanczuk, J. O., et al. (2013). Digit loss in archosaur evolution and the interplay between selection and constraints. Nature 500, 445–448. doi: 10.1038/nature12336
Fabrezi, M., Abdala, V., and Oliver, M. I. (2007). Developmental basis of limb homology in lizards. Anat. Rec. (Hoboken) 290, 900–912. doi: 10.1002/ar.20522
Healy, C., Uwanogho, D., and Sharpe, P. T. (1999). Regulation and role of Sox9 in cartilage formation. Dev. Dyn. 215, 69–78. doi: 10.1002/(SICI)1097-0177(199905)215:1<69::AID-DVDY8>3.0.CO;2-N
Kavanagh, K. D., Shoval, O., Winslow, B. B., Alon, U., Leary, B. P., Kan, A., et al. (2013). Developmental bias in the evolution of phalanges. Proc. Natl. Acad. Sci. U.S.A. 110, 18190–18195. doi: 10.1073/pnas.1315213110
Kawakami, Y., Tsuda, M., Takahashi, S., Taniguchi, N., Esteban, C. R., Zemmyo, M., et al. (2005). Transcriptional coactivator PGC-1alpha regulates chondrogenesis via association with Sox9. Proc. Natl. Acad. Sci. U. S. A. 102, 2414–2419. doi: 10.1073/pnas.0407510102
Kvon, E. Z., Kamneva, O. K., Melo, U. S., Barozzi, I., Osterwalder, M., Mannion, B. J., et al. (2016). Progressive loss of function in a limb enhancer during snake evolution. Cell 167, 633.e11–642.e11. doi: 10.1016/j.cell.2016.09.028
Lopez-Rios, J., Duchesne, A., Speziale, D., Andrey, G., Peterson, K. A., Germann, P., et al. (2014). Attenuated sensing of SHH by Ptch1 underlies evolution of bovine limbs. Nature 511, 46–51. doi: 10.1038/nature13289
Lorda-Diez, C. I., Montero, J. A., Choe, S., Garcia-Porrero, J. A., and Hurle, J. M. (2014). Ligand- and stage-dependent divergent functions of BMP signaling in the differentiation of embryonic skeletogenic progenitors in vitro. J. Bone Miner. Res. 29, 735–748. doi: 10.1002/jbmr.2077
Lorda-Diez, C. I., Montero, J. A., Diaz-Mendoza, M. J., Garcia-Porrero, J. A., and Hurle, J. M. (2011). Defining the earliest transcriptional steps of chondrogenic progenitor specification during the formation of the digits in the embryonic limb. PLoS ONE 6:e24546. doi: 10.1371/journal.pone.0024546
Montero, J. A., Gañan, Y., Macias, D., Rodriguez-Leon, J., Sanz-Ezquerro, J. J., Merino, R., et al. (2001). Role of FGFs in the control of programmed cell death during limb development. Development 128, 2075–2084.
Montero, J. A., Lorda-Diez, C. I., Gañan, Y., Macias, D., and Hurle, J. M. (2008). Activin/TGFbeta and BMP crosstalk determines digit chondrogenesis. Dev. Biol. 321, 343–356. doi: 10.1016/j.ydbio.2008.06.022
Moore, T. Y., Organ, C. L., Edwards, S. V., Biewener, A. A., Tabin, C. J., Jenkins, F. A. Jr., et al. (2015). Multiple phylogenetically distinct events shaped the evolution of limb skeletal morphologies associated with bipedalism in the jerboas. Curr. Biol. 25, 2785–2794. doi: 10.1016/j.cub.2015.09.037
Norrie, J. L., Lewandowski, J. P., Bouldin, C. M., Amarnath, S., Li, Q., Vokes, M. S., et al. (2014). Dynamics of BMP signaling in limb bud mesenchyme and polydactyly. Dev. Biol. 393, 270–281. doi: 10.1016/j.ydbio.2014.07.003
Raspopovic, J., Marcon, L., Russo, L., and Sharpe, J. (2014). Modeling digits. Digit patterning is controlled by a Bmp-Sox9-Wnt Turing network modulated by morphogen gradients. Science 345, 566–570. doi: 10.1126/science.1252960
Richardson, M. K., Gobes, S. M., van Leeuwen, A. C., Polman, J. A., Pieau, C., and Sánchez-Villagra, M. R. (2009). Heterochrony in limb evolution: developmental mechanisms and natural selection. J. Exp. Zool. B Mol. Dev. Evol. 312, 639–364. doi: 10.1002/jez.b.21250
Rodriguez-Leon, J., Merino, R., Macias, D., Gañan, Y., Santesteban, E., and Hurle, J. M. (1999). Retinoic acid regulates programmed cell death through BMP signalling. Nat. Cell Biol. 1, 125–126.
Sanz-Ezquerro, J. J., and Tickle, C. (2003b). Fgf signaling controls the number of phalanges and tip formation in developing digits. 13, 1830–1836. doi: 10.1016/j.cub.2003.09.040
Sanz-Ezquerro, J. J., and Tickle, C. (2003a). Digital development and morphogenesis. J. Anat. 202, 51–58. doi: 10.1046/j.1469-7580.2003.00134.x
Seki, R., Kitajima, K., Matsubara, H., Suzuki, T., Saito, D., Yokoyama, H., et al. (2015). AP-2β is a transcriptional regulator for determination of digit length in tetrapods. Dev. Biol. 407, 75–89. doi: 10.1016/j.ydbio.2015.08.006
Sensiate, L. A., Sobreira, D. R., Da Veiga, F. C., Peterlini, D. J., Pedrosa, A. V., Rirsch, T., et al. (2014). Dact gene expression profiles suggest a role for this gene family in integrating Wnt and TGF-β signaling pathways during chicken limb development. Dev. Dyn. 243, 428–439. doi: 10.1002/dvdy.23948
Sheth, R., Barozzi, I., Langlais, D., Osterwalder, M., Nemec, S., Carlson, H. L., et al. (2016). Distal limb patterning requires modulation of cis-regulatory activities by HOX13. Cell Rep. 17, 2913–2926. doi: 10.1016/j.celrep.2016.11.039
Shubin, N. H., and Alberch, P. (1986). A morphogenetic approach to the origin and basic origanization of the tetrapod limb. Evol. Biol. 20, 319–387.
Uzkudun, M., Marcon, L., and Sharpe, J. (2015). Data-driven modelling of a gene regulatory network for cell fate decisions in the growing limb bud. Mol. Syst. Biol. 11:815. doi: 10.15252/msb.20145882
Weston, A. D., Chandraratna, R. A., Torchia, J., and Underhill, T. M. (2002). Requirement for RAR-mediated gene repression in skeletal progenitor differentiation. J. Cell Biol. 158, 39–51. doi: 10.1083/jcb.200112029
Woltering, J. M., and Duboule, D. (2010). The origin of digits: expression patterns versus regulatory mechanisms. Dev. Cell 18, 526–532. doi: 10.1016/j.devcel.2010.04.002
Wright, E., Hargrave, M. R., Christiansen, J., Cooper, L., Kun, J., Evans, T., et al. (1995). The Sry-related gene Sox9 is expressed during chondrogenesis in mouse embryos. Nat. Genet. 9, 15–20. doi: 10.1038/ng0195-15
Yntema, C. L. (1968). A series of stages in the embryonic development of chelydra serpentina. J. Morphol. 125, 219–251. doi: 10.1002/jmor.1051250207
Zhu, J., Nakamura, E., Nguyen, M. T., Bao, X., Akiyama, H., and Mackem, S. (2008). Uncoupling sonic hedgehog control of pattern and expansion of the developing limb bud. Dev. Cell 14, 624–632. doi: 10.1016/j.devcel.2008.01.008
Keywords: limb development, interdigit regression, chondrogenesis, skeletal progenitors, SOX9 transcription factor
Citation: Montero JA, Lorda-Diez CI, Francisco-Morcillo J, Chimal-Monroy J, Garcia-Porrero JA and Hurle JM (2017) Sox9 Expression in Amniotes: Species-Specific Differences in the Formation of Digits. Front. Cell Dev. Biol. 5:23. doi: 10.3389/fcell.2017.00023
Received: 03 January 2017; Accepted: 07 March 2017;
Published: 23 March 2017.
Edited by:
Juan Jose Sanz-Ezquerro, Consejo Superior de Investigaciones Científicas, SpainReviewed by:
Juan Carlos Izpisua Belmonte, Salk Institute, USAAlexander Omar Vargas, Universidad de Chile, Chile
Karen Sears, University of Illinois at Urbana–Champaign, USA
Copyright © 2017 Montero, Lorda-Diez, Francisco-Morcillo, Chimal-Monroy, Garcia-Porrero and Hurle. This is an open-access article distributed under the terms of the Creative Commons Attribution License (CC BY). The use, distribution or reproduction in other forums is permitted, provided the original author(s) or licensor are credited and that the original publication in this journal is cited, in accordance with accepted academic practice. No use, distribution or reproduction is permitted which does not comply with these terms.
*Correspondence: Juan M. Hurle, hurlej@unican.es