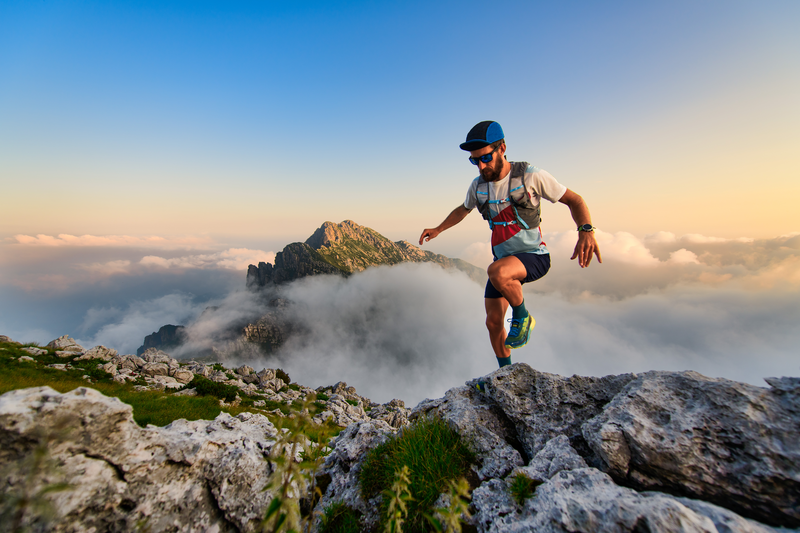
95% of researchers rate our articles as excellent or good
Learn more about the work of our research integrity team to safeguard the quality of each article we publish.
Find out more
MINI REVIEW article
Front. Cell Dev. Biol. , 21 September 2016
Sec. Molecular and Cellular Pathology
Volume 4 - 2016 | https://doi.org/10.3389/fcell.2016.00103
Colorectal cancer is the third most common cancer worldwide and is associated with significant morbidity and mortality. Current pharmacotherapy options include cytotoxic chemotherapy, anti-VEGF, and anti-EGFR targeting drugs, but these are limited by toxic side effects, limited responses and ultimately resistance. Cysteinyl leukotriene (CysLT) signaling regulates intestinal homeostasis with mounting evidence suggesting that CysLT signaling also plays a role in the pathogenesis of colorectal cancer. Therefore, CysLT signaling represents a novel target for this malignancy. This review evaluates reported links between CysLT signaling and established hallmarks of cancer in addition to its pharmacological potential as a new therapeutic target.
Each year, ~1.4 million new cases of colorectal cancer (CRC) are diagnosed, with almost 700,000 cancer-related deaths, making it the third most common cancer worldwide and the fourth most common cause of cancer death (Torre et al., 2015). Surgical intervention is the cornerstone of treatment but adjuvant chemotherapy and targeted therapies play significant roles in improving survival. Treatment decision is guided by clinical factors (e.g., co-morbid conditions, performance status) and pathological factors (e.g., KRAS mutation status, microsatellite stability status). 5-fluorouracil-based regimens such as FOLFOX or FOLFIRI form the backbone of cytotoxic chemotherapy in the treatment of metastatic disease but are limited by chemoresistance and toxic effects on non-neoplastic healthy tissue (Longley and Johnston, 2005; Fuchs et al., 2007).
Targeted therapies include the anti-angiogenic drug bevacizumab (Avastin®), a humanized monoclonal antibody that binds directly to vascular endothelial growth factor A (VEGF-A; Ferrara et al., 2004). Blocking VEGF inhibits tumor angiogenesis, a pathophysiological process upon which solid tumors depend for growth, survival, and metastasis (Folkman, 1971). Other targeted therapies include anti-epidermal growth factor receptor (EGFR) antibodies cetuximab and panitumumab, the major advantage of which is the availability of a validated biomarker that reliably predicts patient response—patients with activating KRAS mutations have poorer progression-free and overall survival (Martinelli et al., 2009; Yen et al., 2010). Immune checkpoint inhibition is emerging as a promising treatment strategy in microsatellite instability-high (MSI-H) colorectal tumors, a phenotype resulting from defective DNA mismatch repair and accounting for up to 15% of all CRCs (Boland and Goel, 2010; Le et al., 2015). Pembrolizumab, an anti-programmed cell death protein 1 (anti-PD-1) antibody potentiates T-cell immune responses, circumventing tumor immune evasion, and significantly prolonging progression-free survival in patients with MSI-H tumors (McDermott and Atkins, 2013; Le et al., 2015). Numerous other immunotherapies are currently being evaluated in clinical trials in combination with chemotherapy regimens and/or targeted therapies.
While CRC survival rates are improving, there is a need for more effective therapies as the survival benefit associated with targeted therapies is only ~4–5 months (McCormack and Keam, 2008; Bokemeyer et al., 2012). Many novel strategies are currently under investigation, one of which is to target cysteinyl leukotriene (CysLT) signaling. The focus of this mini-review is to evaluate the link between CysLTs and the hallmarks of cancer.
Cysteinyl leukotrienes (CysLTs) are a subfamily of eicosanoids, lipophilic signaling molecules that regulate both acute and chronic inflammation (Henderson, 1994). These potent bioactive lipids are rapidly generated de novo from cell membrane-associated arachidonic acid (AA), an essential polyunsaturated fatty acid in response to cell activation (Clark et al., 1991). Once mobilized to the cytosol, AA is metabolized by 5-lipoxygenase (5-LOX) in conjunction with 5-lipoxygenase-activating protein (FLAP) to yield leukotriene A4 (LTA4), which undergoes further metabolism to form leukotrienes B4 (LTB4) and C4 (LTC4) (Kanaoka and Boyce, 2004). Subsequently LTC4 is exported from the cell via multi-drug resistance-associated proteins 1 and 4, and metabolized to LTD4 and LTE4 (Lam et al., 1989). LTC4, LTD4, and LTE4, referred to collectively as CysLTs, are structurally similar but exhibit functional diversity (Laidlaw and Boyce, 2012).
The biological actions of CysLTs are mediated via ligation of the widely distributed G-protein-coupled receptors, CysLT1 and CysLT2 (Figure 1; Peters-Golden et al., 2006). The role of newly-identified CysLT receptors GPR17 and GPR99 remains to be established. GPR17 is an orphan P2Y-like receptor with dual specificity for uracil nucleotides and CysLTs, while GPR99 has been proposed as a potential LTE4-selective CysLT receptor (Ciana et al., 2006; Kanaoka et al., 2013). Cross-regulation occurs between CysLT receptors—CysLT2 negatively regulates CysLT1 signaling via receptor heterodimerization and GPR17 has been reported as a ligand-independent negative regulator of CysLT1 (Lynch et al., 1999; Jiang et al., 2007; Maekawa et al., 2009).
Figure 1. Cysteinyl leukotriene receptors: pharmacology, distribution, function and signaling pathways. Upon binding of ligand to the CysLT receptor various downstream signaling pathways are activated. CysLT1 induces PI3K-Akt signaling, resulting in β-catenin nuclear translocation and activation of target genes including cyclin D1, COX-2, and c-Myc (Savari et al., 2014). Akt/PKB activates IKK complex with subsequent degradation of IκB protein leading the NF-kB translocation and activation (Madrid et al., 2001). CysLT1, CysLT2, and GPR17 signaling can also activate phospholipase C (PLC) and Ras-Raf-MEK-ERK pathway (Thompson et al., 2008; Hennen et al., 2013; Savari et al., 2014). This lead to nuclear translocation of Erk 1/2 resulting in activation of genes involved in proliferation, migration, survival. Alternatively, CysLT receptor ligation can activate PKC and the transcription factor cAMP response element-binding protein (CREB; Savari et al., 2014). Crosstalk occurs between CysLT and EGF signaling pathways – EGF signaling also activates Rac and the Ras-Raf-MEK-ERK pathway (Magi et al., 2014). EGF signaling induces production of 5-LOX, resulting in leukotriene synthesis and consequential potentiation of CysLT receptor signaling, while LOX/CysLT1 also regulate EGF-induced migration (Magi et al., 2014). PLC, phospholipase C; PKC, protein kinase C; p90RSK, p90 ribosomal S6 kinase; CREB, cAMP response element binding; NF-κB, nuclear factor kappa b; IKK, IkB kinase; TCF/LEF, T-cell factor/lymphoid enhancer factor; β-cat, β-catenin; APC adenomatous polyposis coli; GSK-3β glycogen synthase kinase-3 beta; PKB protein kinase B; PI3K, phosphoinositide 3-kinase; 5-LOX, 5-lipoxygenase; EGF, epidermal growth factor; EGFR, epidermal growth factor receptor.
CysLTs play recognized roles in promoting the inflammatory response, bronchoconstriction and vascular permeability (Davidson et al., 1987; Lee et al., 2004). CysLTs have also recently emerged as important regulators of intestinal homeostasis, with endogenous CysLT production mediating the survival and proliferation of intestinal epithelial cells (Paruchuri et al., 2006). Dysregulated CysLT signaling has been implicated in colorectal adenocarcinomas with increased CysLT1 and decreased CysLT2 levels in patient tumor samples compared with surrounding normal tissue (Magnusson et al., 2007). Interestingly, unlike the majority of G-protein coupled receptors, CysLT1 and CysLT2 are located both at the plasma membrane and the nuclear membrane (Magnusson et al., 2010). This subcellular receptor localization is critically important for CRC patient survival - patients with high nuclear CysLT1 expression have a poorer prognosis than patients with high cytoplasmic expression (Magnusson et al., 2010). In contrast, patients with high nuclear CysLT2 expression have a better overall survival expectancy, indicating the existence of an inverse relationship between nuclear CysLT1 and CysLT2 expression, and suggesting that CysLT2 has a protective role in CRC (Magnusson et al., 2010).
In vitro data corroborate these findings as malignant intestinal cell lines Caco-2 and SW480 demonstrate higher CysLT1 but lower CysLT2 expression levels compared to non-cancerous Int 407 intestinal epithelial cells (Magnusson et al., 2007). CysLT2 signaling also results in terminal differentiation of Caco-2 cells and growth inhibition (Magnusson et al., 2007). All-trans retinoic acid (ATRA), an anti-cancer agent promoting cell differentiation, acts in part by upregulating CysLT2 mRNA expression and LTC4 synthase (Bengtsson et al., 2013).
Interestingly, CysLT1 expression increases CRC tumor burden in a gender-specific manner in vivo—In the ApcMin/+ mouse model of familial adenomatous polyposis/sporadic CRC, female mice lacking the CysLT1 gene (Cysltr1 −/− ApcMin/+) develop significantly less polyps, less systemic inflammation, and increased regulatory T-cell tumor infiltration, a negative prognostic factor in CRC, compared to Cysltr1 +/+ ApcMin/+ mice.
Analysis of The Cancer Genome Atlas (TCGA) RNA-sequencing dataset reveal that GPR17 and GPR99 are expressed in CRC patient samples, with GPR99 more highly expressed relative to GPR17 based on FPKM relative expression values. Kaplan-Meier survival analysis was conducted using a log-rank test with the “survival” package in R and grouping 41 tumor samples based on median expression. We found no significant correlation of either gene with overall survival. While both receptors have been identified in the colon, further investigation in a larger cohort of CRC patients is required to confirm these findings.
CysLTs have multiple roles in many hallmarks of cancer (Figure 2).
Figure 2. Cysteinyl leukotriene signaling modulates many of the hallmarks of cancer in colorectal cancer. Mounting evidence indicates CysLTs play an important role in the tumorigenesis of colorectal cancer. CysLT signaling modifies 7 out of the 10 Hanahan and Weinberg hallmarks of cancer (Hanahan and Weinberg, 2011). CysLTs sustain proliferative signaling, activate migration and invasion, induce angiogenesis, contribute to genome instability and mutation, deregulate cellular energetics, and resist cell death. No evidence to date supports a role for CysLT in enabling replicative immortality, evading immune destruction or evading growth suppressors. PI3K, phosphoinositide 3-kinase; EGF, epidermal growth factor; MAPK, mitogen-activated protein kinase; NF-κB, nuclear factor kappa b; TNFα, tumor necrosis factor alpha; CREB, cAMP response element binding; NOX4, NADPH oxidase 4; ROS, reactive oxygen species; MGST2, microsomal glutathione S-transferase 2; EC, endothelial cell.
CysLTs induce cell proliferation in various cell types including bone marrow cells, smooth muscle cells, endothelial cells, and intestinal cells and it is suggested that dysregulated CysLT signaling contributes to uncontrolled proliferation (Lindgren et al., 1993; Porreca et al., 1996; Paruchuri and Sjölander, 2003; Duah et al., 2013). LTD4 induces intestinal cell proliferation in Int 407 cells via stimulation of the Erk 1/2 pathway and subsequent activation of p90 ribosomal S6 kinase (p90RSK; Paruchuri et al., 2002). LTD4 also reduces G0/G1 and increases S+G2/M phases of Int 407 cell cycle, further supporting a role in intestinal cell proliferation. CysLT1 signaling via the GSK-3β/β-catenin pathway increases transcription of target genes COX-2, c-Myc and Cyclin D1, well-known modulators of cell proliferation (Savari et al., 2014). The β-catenin pathway is negatively regulated by the APC tumor-suppressor protein and is critical in colorectal carcinogenesis—over 80% of sporadic CRCs carry a somatic mutation of the APC gene, while a germline mutation carries an almost 100% lifetime risk of CRC (Kinzler and Vogelstein, 1996; Jasperson et al., 2010).
The proliferative effects of CysLTs in the intestine depend on the receptor subtype. CysLT1 signaling induces proliferation of Int 407 epithelial cells in an autocrine manner, whereas CysLT2 signaling promotes terminal cell differentiation of Caco-2 cells, inhibiting growth (Paruchuri et al., 2002; Magnusson et al., 2007). CysLT2 expression is significantly higher during the quiescent G0 phase of the intestinal cell cycle compared to actively proliferating phases (Magnusson et al., 2007). In HCT-116 colorectal xenograft mouse models, CysLT1 antagonists reduce proliferation as determined by Ki-67 levels and significantly reduce tumor size (Savari et al., 2013). The role of non-classical CysLT receptors in cell proliferation is unknown—GPR17+ cells demonstrate proliferative activity in the central nervous system but have not been investigated in a CRC context (Ceruti et al., 2009).
Cell migration, facilitated by the epithelial-mesenchymal transition (EMT) process, is essential for tumor invasion, angiogenesis, and metastasis. CysLT1 signaling stimulates the migration of Int 407 intestinal epithelial cells via a phosphatidylinositol 3-kinase and Rac-dependent mechanism (Paruchuri et al., 2005). LTD4 also stimulates HCT-116 colon cancer cell migration and decreases expression of adhesion molecule E-cadherin, a key mediator of the EMT process (Hirohashi, 1998; Salim et al., 2014).
Crosstalk between EGFR signaling and CysLT1 signaling is essential for epithelial cancer migration and invasion—the LOX/LTC4/CysLT1 signaling pathway regulates EGF-induced cell migration via Rac1 activation in A431 human epidermoid carcinoma cells (Magi et al., 2014). Conversely CysLT2 signaling has been shown to reduce cell migration in MCF-7 breast cancer cell lines, further substantiating many reports that CysLT receptors have opposing effects (Jiang et al., 2007; Magnusson et al., 2007, 2011a; Bengtsson et al., 2013). GPR17 signaling stimulates migration in oligodendrocytes and cardiac stromal cells but its effect on intestinal cells have not been investigated (Coppi et al., 2013; Cosentino et al., 2014).
In order to sustain growth, a tumor must obtain a blood supply by tilting the balance of angiogenic mediators in favor of angiogenesis (Bergers and Benjamin, 2003). CysLT1 signaling activates production of the pro-angiogenic mediator VEGF-A in vitro, while CysLT antagonists modulate vascular permeability and reduce VEGF levels in murine models of allergic asthma and in asthmatic patients (Kanazawa et al., 2004; Lee et al., 2004; Poulin et al., 2011).
CysLT1 and CysLT2 receptors are both expressed in vascular endothelial cells (ECs) and vascular smooth muscle cells, either constitutively or inducibly—although CysLT2 is the dominant receptor type (Gronert et al., 2001; Sjöström et al., 2003; Kaetsu et al., 2007). Transgenic mice overexpressing endothelial CysLT2 exhibit vascular hyperpermeability and upregulate ICAM-1 and VCAM-1 expression, adhesion molecules which regulate angiogenesis (Jiang et al., 2008; Ni et al., 2014). Conflicting reports surround the regulation of EC function by CysLTs—CysLT stimulation has been shown to increase human umbilical vein endothelial cell proliferation in vitro (Duah et al., 2013). Yuan et al. (2009) demonstrated no proliferative effect in EA.hy926 macrovascular endothelial cells upon CysLT stimulation, solely induction of endothelial cell migration. In vivo, CysLTs significantly enhance microvessel growth in rat thoracic aortic ring and chick chorioallantoic membrane assays in a dose-dependent manner, an effect abrogated by both CysLT1 and CysLT2 antagonists (Tsopanoglou et al., 1994; Xu et al., 2010).
Furthermore, GPR99 and its ligand α-ketoglutarate upregulate EC proliferation and promote vessel sprouting and neovascularization using in vivo models of retinal angiogenesis (Sirinyan et al., 2007). GPR99 also mediates cutaneous vascular permeability in mouse respiratory models (Bankova et al., 2016).
DNA damage and genomic instability is a primary driver of carcinogenesis (Shen, 2011). Recent evidence highlights the role of LTC4 in promoting oxidative DNA damage, which if not sufficiently repaired may contribute to genomic instability and increased mutation rates (Dvash et al., 2015). A previously unrecognized microsomal glutathione-S-transferase 2 (MGST2)-LTC4 signaling pathway elicits nuclear translocation of NADPH oxidase 4 (NOX4) and generation of reactive oxygen species (ROS) when triggered by endoplasmic reticulum (ER) stress and chemotherapy (Dvash et al., 2015). Activation of this pathway upregulates MGST2, CysLT production enzymes and CysLT1 and CysLT2 expression in human amniotic WISH epithelial cells. LTC4 antagonists reduce NOX4 levels, inhibit ROS accumulation and significantly attenuate nuclear DNA damage induced by the chemotherapeutic agent doxorubicin (Dvash et al., 2015). Therefore, LTC4 antagonism may have potential in combination with chemotherapy to reduce toxicities.
Cancer cells often exhibit ER stress due to high proliferation rates (Wang et al., 2010). Therefore, while this pathway may not be responsible for the initiation of tumorigenesis, it may contribute to tumor progression via an ER-stress triggered mechanism of leukotriene production.
CysLT signaling may induce intestinal cell resistance to apoptosis, as evidenced by the increase in cell survival of Int 407 intestinal cells exposed to LTD4 (Ohd et al., 2000). CysLT1 signaling increases COX-2 expression and subsequent expression of the anti-apoptotic protein Bcl-2 by activating the MEK/Erk signaling pathway in the Caco-2 cell line (Wikström et al., 2003). LTD4 promotes mitochondrial translocation of β-catenin in Int 407 intestinal cells where it induces Bcl-2 expression, thereby protecting cells from apoptosis and enhancing cell survival (Mezhybovska et al., 2006). The mechanism by which the CysLT1 signaling exerts this pro-survival effect has been attributed to cAMP response element-binding protein (CREB), a transcription factor implicated in the pathophysiology of a number of cancers (Paruchuri and Sjölander, 2003; Sakamoto and Frank, 2009). While CysLT1 signaling can inhibit intestinal cell apoptosis, CysLT2 signaling appears to have no effect on the levels of apoptosis in a MCF-7 breast cancer cell line (Magnusson et al., 2011b).
The glycolytic metabolic switch adapted by tumor cells is necessary to support the demands of rapid cell proliferation, and is critically mediated by the “master regulator” c-Myc oncogene (Miller et al., 2012). CysLT1 signaling activates transcription of c-Myc via induction of β-catenin nuclear translocation in Int 407 intestinal epithelial cells (He et al., 1998; Ohd et al., 2000). CysLT1 activation also induces the PI3K-Akt signaling pathway in Int 407 cells, constitutive activation of which stimulates glycolysis (Elstrom et al., 2004; Mezhybovska et al., 2005). LTD4 stimulation increases metabolic activity in both non-transformed Int 407 epithelial cells and Caco-2 colon cancer cells with observed increases in ATP/ADP ratios. LTD4 also triggers significant increases in mitochondrial gene activity, changes in which have been identified in primary tumors of CRC patients (Polyak et al., 1998; Mezhybovska et al., 2009).
Interestingly, a key citric acid cycle intermediate α -ketoglutarate has been identified as a ligand GPR99 (He et al., 2004). α-ketoglutarate is also a substrate for prolyl hydroxylases which regulate hypoxia inducible factor 1α, a key player in the reprogramming of cancer metabolism (MacKenzie et al., 2007).
Chronic inflammation is a risk factor for the development of cancer, as illustrated in inflammatory bowel disease (IBD) patients (Bernstein et al., 2001). IBD patients exhibit a 3 to seven-fold higher expression of leukotriene pathway enzymes—it is currently unknown if this correlates with an increased risk of cancer development (Jupp et al., 2007). LTD4 antagonists reduce colonic inflammation in a rat model of acute colitis induced by intracolonic administration of trinitrobenzene sulfonic acid and may have potential in the prevention of inflammation-associated CRC (Nishikawa et al., 1995).
CysLTs act as leukocyte chemoattractants—CysLT1 mediates Th17 cell migration, accumulation of which correlates with the progression of inflammation-associated cancer (Kim and Lee, 2015). Tumor-associated macrophages (TAMs) within the tumor microenvironment also secrete high levels of LTD4 which may further promote immune cell infiltration (Zhang, 2013). Tumor necrosis factor-α (TNF-α), a pro-inflammatory cytokine involved in the initiation and propagation of CRC, upregulates 5-LOX, LTC4 synthase and CysLT1 while downregulating CysLT2 expression in SW-480, HCT-116, HT-29, and Caco-2 colon cancer cells (Yudina et al., 2008). CysLTs also activate NF-κB, a transcriptional regulator of numerous inflammatory genes, constitutively active in up to 80% of colorectal tumors (Lind et al., 2001; Kawano et al., 2003; Hashimoto et al., 2009).
GPR17 negatively regulates CysLT1-mediated immune cell accumulation in the lungs, while GPR99 is proposed to elicit a pro-inflammatory response upon binding of LTE4, the predominant CysLT in inflamed tissue (Kanaoka et al., 2013; Akiko Maekawa et al., 2016). Autocrine CysLT1 signaling loops could allow intestinal epithelial cells to maintain chronic inflammation, thereby increasing the risk of inflammation-associated CRC (Paruchuri et al., 2006).
Overall, targeting CysLT signaling is a promising option for anti-cancer therapy due to its effects on multiple oncogenic pathways described above (Figure 2). Commercially available anti-asthmatic drugs which target the CysLT1, have demonstrated notable in vivo potential as anti-cancer agents. Montelukast, a CysLT1-selective antagonist significantly reduces tumor volume in a HCT-116 CRC-specific murine xenograft model, via a combination of anti-proliferative and pro-apoptotic effects (Savari et al., 2013). CysLT1 antagonism also exhibits an anti-angiogenic effect, with reduced blood vessel formation and VEGF expression levels in colorectal tumors (Savari et al., 2013). Furthermore, pre-treatment of HCT-116 cells with montelukast prior to inoculation completely inhibited tumor initiation in BALB/c nu/nu mice. Montelukast also has antagonistic actions at GPR17—it is unknown if this receptor is important for the drug's anti-neoplastic effect (Ciana et al., 2006). Pranlukast significantly attenuates chemotherapy-triggered morbidity in mice via a MGST2-LTC4 pathway, and so may have therapeutic potential in combination with chemotherapy to reduce toxic side effects or to reduce the risk of metastases (Dvash et al., 2015). Furthermore, CysLT1 antagonists potently inhibit the growth of HCT-116 colon cancer cells, in addition to prostate, urothelial, and neuroblastoma cancer cell lines (Matsuyama et al., 2007, 2009; Sveinbjörnsson et al., 2008; Savari et al., 2013).
No studies to date have investigated the effects of specific CysLT2 agonists or antagonists on tumor progression specifically. In the case of CRC, selective CysLT2 agonism would appear to be the desired approach, but this needs potent selectivity in order to avoid negative effects of CysLT1 agonism on the intestine. Due to cross regulation between CysLT receptors, such a targeting strategy would need to be approached with caution (Laidlaw and Boyce, 2012).
Targeting 5-LOX or FLAP to inhibit endogenous production of CysLTs is an alternative strategy that would circumvent the increasing number of CysLT isoreceptors. Zileuton, a 5-LOX inhibitor, prevents colonic polyp formation in an APCΔ468 mouse model of polyposis and significantly decreases tumor burden in LoVo and HT29 colon cancer murine xenograft models (Melstrom et al., 2008; Gounaris et al., 2015). MK-866, a FLAP inhibitor, reduces proliferation of Caco-2 and HT29 colon cancer cell lines (Ford-Hutchinson, 1991; Cianchi et al., 2006).
These studies highlight the importance of CysLT signaling in intestinal biology, and its potential role in the tumorigenesis of colorectal adenocarcinoma. CysLT signaling is linked with many hallmarks of cancer—cell proliferation and survival, cell migration, angiogenesis, genomic instability, glycolytic metabolic switch, and tumor-promoting inflammation. Data largely centers on CysLT1 and CysLT2 with further investigation necessary to establish the importance of GPR17 and GPR99 in this context.
Targeting CysLT signaling is a promising pharmacological strategy, as evidenced by the numerous CysLT antagonists which have anti-tumor efficacy in in vitro and in vivo CRC models. In particular, blockade of LTC4 has recently emerged as a promising new therapeutic opportunity in limiting toxicity to current chemotherapy drugs. A notable advantage of many CysLT receptor antagonists is their favorable safety profile. CysLT signaling modulators may have potential to synergize with other targeted therapies to benefit survival rates. In summary, CysLT signaling is gaining increasing recognition as a mediator of colorectal tumorigenesis and pharmacological manipulation of this signaling pathway represents an exciting opportunity that merits further translational research work.
LB conducted the literature review and wrote the paper. CB revised and edited the paper. AM revised and edited the paper. BM provided analysis of RNA sequencing dataset. WG provided analysis of RNA sequencing dataset. JO revised and edited the paper. BK revised and edited the paper.
This work is supported by Irish Cancer Society Research Scholarship CRS13BUT.
The authors declare that the research was conducted in the absence of any commercial or financial relationships that could be construed as a potential conflict of interest.
Akiko Maekawa, K., Xing, W., Frank Austen, K., Maekawa, A., and Kanaoka, Y. (2016). Inflammation induced by house dust mites GPR17 regulates immune pulmonary GPR17 regulates immune pulmonary inflammation induced by house dust mites. J. Immunol. 185, 1846–1854. doi: 10.4049/jimmunol.1001131
Bäck, M., Rovati, G. E., Dahlén, S.-E., Drazen, J., Evans, J. F., Shimizu, T., et al. (2015). Leukotriene receptors: CysLT1 receptor. IUPHAR/BPS Guid. to Pharmacol. Available online at: http://www.guidetopharmacology.org/GRAC/ObjectDisplayForward?objectId=269 [Accessed June 28, 2016].
Bankova, L. G., Lai, J., Yoshimoto, E., Boyce, J. A., Austen, K. F., Kanaoka, Y., et al. (2016). Leukotriene E4 elicits respiratory epithelial cell mucin release through the G-protein–coupled receptor, GPR99. Proc. Natl. Acad. Sci. U.S.A. 113, 6242–6247. doi: 10.1073/pnas.1605957113
Bengtsson, A. M., Jönsson, G., Magnusson, C., Salim, T., Axelsson, C., and Sjölander, A. (2013). The cysteinyl leukotriene 2 receptor contributes to all-trans retinoic acid-induced differentiation of colon cancer cells. BMC Cancer 13:336. doi: 10.1186/1471-2407-13-336
Bergers, G., and Benjamin, L. E. (2003). Tumorigenesis and the angiogenic switch. Nat. Rev. Cancer 3, 401–410. doi: 10.1038/nrc1093
Bernstein, C. N., Blanchard, J. F., Kliewer, E., and Wajda, A. (2001). Cancer risk in patients with inflammatory bowel disease: a population-based study. Cancer 91, 854–862. doi: 10.1002/1097-0142(20010215)91:4<854::AID-CNCR1073>3.0.CO;2-Z
Bokemeyer, C., Van Cutsem, E., Rougier, P., Ciardiello, F., Heeger, S., Schlichting, M., et al. (2012). Addition of cetuximab to chemotherapy as first-line treatment for KRAS wild-type metastatic colorectal cancer: pooled analysis of the CRYSTAL and OPUS randomised clinical trials. Eur. J. Cancer 48, 1466–1475. doi: 10.1016/j.ejca.2012.02.057
Boland, C. R., and Goel, A. (2010). Microsatellite instability in colorectal cancer. Gastroenterology 138, 2073.e3–2087.e3. doi: 10.1053/j.gastro.2009.12.064
Ceruti, S., Villa, G., Genovese, T., Mazzon, E., Longhi, R., Rosa, P., et al. (2009). The P2Y-like receptor GPR17 as a sensor of damage and a new potential target in spinal cord injury. Brain 132, 2206–2218. doi: 10.1093/brain/awp147
Ciana, P., Fumagalli, M., Trincavelli, M. L., Verderio, C., Rosa, P., Lecca, D., et al. (2006). The orphan receptor GPR17 identified as a new dual uracil nucleotides/cysteinyl-leukotrienes receptor. EMBO J. 25, 4615–4627. doi: 10.1038/sj.emboj.7601341
Cianchi, F., Cortesini, C., Magnelli, L., Fanti, E., Papucci, L., Schiavone, N., et al. (2006). Inhibition of 5-lipoxygenase by MK886 augments the antitumor activity of celecoxib in human colon cancer cells. Mol. Cancer Ther. 5, 2716–2726. doi: 10.1158/1535-7163.MCT-06-0318
Clark, J. D., Lin, L. L., Kriz, R. W., Ramesha, C. S., Sultzman, L. A., Lin, A. Y., et al. (1991). A novel arachidonic acid-selective cytosolic PLA2 contains a Ca(2+)-dependent translocation domain with homology to PKC and GAP. Cell 65, 1043–1051. doi: 10.1016/0092-8674(91)90556-E
Coppi, E., Maraula, G., Fumagalli, M., Failli, P., Cellai, L., Bonfanti, E., et al. (2013). UDP-glucose enhances outward K+ currents necessary for cell differentiation and stimulates cell migration by activating the GPR17 receptor in oligodendrocyte precursors. Glia 61, 1155–1171. doi: 10.1002/glia.22506
Cosentino, S., Castiglioni, L., Colazzo, F., Nobili, E., Tremoli, E., Rosa, P., et al. (2014). Expression of dual Nucleotides/Cysteinyl-Leukotrienes receptor GPR17 in early trafficking of cardiac stromal cells after myocardial infarction. J. Cell. Mol. Med. 18, 1785–1796. doi: 10.1111/jcmm.12305
Davenport, A. P., Alexander, S., Sharman, J. L., Pawson, A. J., Benson, H. E., Monaghan, A. E., et al. (2015). Class A Orphans: GPR17. IUPHAR/BPS Guid. to Pharmacol. Available online at: http://www.guidetopharmacology.org/GRAC/ObjectDisplayForward?objectId=88 [Accessed June 28, 2016].
Davidson, A. B., Lee, T. H., Scanlon, P. D., Solway, J., McFadden, E. R., Ingram, R. H., et al. (1987). Bronchoconstrictor effects of leukotriene E4 in normal and asthmatic subjects. Am. Rev. Respir. Dis. 135, 333–337.
Duah, E., Adapala, R. K., Al-Azzam, N., Kondeti, V., Gombedza, F., Thodeti, C. K., et al. (2013). Cysteinyl leukotrienes regulate endothelial cell inflammatory and proliferative signals through CysLT2 and CysLT1 receptors. Sci. Rep. 3, 63–71. doi: 10.1038/srep03274
Dvash, E., Har-Tal, M., Barak, S., Meir, O., and Rubinstein, M. (2015). Leukotriene C4 is the major trigger of stress-induced oxidative DNA damage. Nat. Commun. 6:10112. doi: 10.1038/ncomms10112
Elstrom, R. L., Bauer, D. E., Buzzai, M., Karnauskas, R., Harris, M. H., Plas, D. R., et al. (2004). Akt stimulates aerobic glycolysis in cancer cells. Cancer Res. 64, 3892–3899. doi: 10.1158/0008-5472.CAN-03-2904
Ferrara, N., Hillan, K. J., Gerber, H.-P., and Novotny, W. (2004). Case history: discovery and development of bevacizumab, an anti-VEGF antibody for treating cancer. Nat. Rev. Drug Discov. 3, 391–400. doi: 10.1038/nrd1381
Folkman, J. (1971). Tumor angiogenesis: therapeutic implications. N. Engl. J. Med. 285, 1182–1186. doi: 10.1056/NEJM197111182852108
Ford-Hutchinson, A. W. (1991). FLAP: a novel drug target for inhibiting the synthesis of leukotrienes. Trends Pharmacol. Sci. 12, 68–70. doi: 10.1016/0165-6147(91)90500-R
Fuchs, C. S., Marshall, J., Mitchell, E., Wierzbicki, R., Ganju, V., Jeffery, M., et al. (2007). Randomized, controlled trial of irinotecan plus infusional, bolus, or oral fluoropyrimidines in first-line treatment of metastatic colorectal cancer: results from the BICC-C Study. J. Clin. Oncol. 25, 4779–4786. doi: 10.1200/JCO.2007.11.3357
Gounaris, E., Heiferman, M. J., Heiferman, J. R., Shrivastav, M., Vitello, D., Blatner, N. R., et al. (2015). Zileuton, 5-lipoxygenase inhibitor, acts as a chemopreventive agent in intestinal polyposis, by modulating polyp and systemic inflammation. PLoS ONE 10:e0121402. doi: 10.1371/journal.pone.0121402
Gronert, K., Martinsson-Niskanen, T., Ravasi, S., Chiang, N., and Serhan, C. N. (2001). Selectivity of recombinant human leukotriene D(4), leukotriene B(4), and lipoxin A(4) receptors with aspirin-triggered 15-epi-LXA(4) and regulation of vascular and inflammatory responses. Am. J. Pathol. 158, 3–9. doi: 10.1016/S0002-9440(10)63937-5
Hanahan, D., and Weinberg, R. A. (2011). Hallmarks of cancer: the next generation. Cell 144, 646–674. doi: 10.1016/j.cell.2011.02.013
Hashimoto, K., Ichiyama, T., Hasegawa, M., Hasegawa, S., Matsubara, T., and Furukawa, S. (2009). Cysteinyl leukotrienes induce monocyte chemoattractant protein-1 in human monocyte/macrophages via mitogen-activated protein kinase and nuclear factor-kappaB pathways. Int. Arch. Allergy Immunol. 149, 275–282. doi: 10.1159/000199724
He, T. C., Sparks, A. B., Rago, C., Hermeking, H., Zawel, L., da Costa, L. T., et al. (1998). Identification of c-MYC as a target of the APC pathway. Science 281, 1509–1512. doi: 10.1126/science.281.5382.1509
He, W., Miao, F. J.-P., Lin, D. C.-H., Schwandner, R. T., Wang, Z., Gao, J., et al. (2004). Citric acid cycle intermediates as ligands for orphan G-protein-coupled receptors. Nature 429, 188–193. doi: 10.1038/nature02488
Henderson, W. R. (1994). The role of leukotrienes in inflammation. Ann. Intern. Med. 121, 684–697. doi: 10.7326/0003-4819-121-9-199411010-00010
Hennen, S., Wang, H., Peters, L., Merten, N., Simon, K., Spinrath, A., et al. (2013). Decoding signaling and function of the orphan G protein-coupled receptor GPR17 with a small-molecule agonist. Sci. Signal. 6:ra93. doi: 10.1126/scisignal.2004350
Hirohashi, S. (1998). Inactivation of the E-cadherin-mediated cell adhesion system in human cancers. Am. J. Pathol. 153, 333–339. doi: 10.1016/S0002-9440(10)65575-7
Jasperson, K. W., Tuohy, T. M., Neklason, D. W., and Burt, R. W. (2010). Hereditary and familial colon cancer. Gastroenterology 138, 2044–2058. doi: 10.1053/j.gastro.2010.01.054
Jiang, W., Hall, S. R., Moos, M. P. W., Cao, R. Y., Ishii, S., Ogunyankin, K. O., et al. (2008). Endothelial cysteinyl leukotriene 2 receptor expression mediates myocardial ischemia-reperfusion injury. Am. J. Pathol. 172, 592–602. doi: 10.2353/ajpath.2008.070834
Jiang, Y., Borrelli, L. A., Kanaoka, Y., Bacskai, B. J., and Boyce, J. A. (2007). CysLT2 receptors interact with CysLT1 receptors and down-modulate cysteinyl leukotriene dependent mitogenic responses of mast cells. Blood 110, 3263–3270. doi: 10.1182/blood-2007-07-100453
Jupp, J., Hillier, K., Elliott, D. H., Fine, D. R., Bateman, A. C., Johnson, P. A., et al. (2007). Colonic expression of leukotriene-pathway enzymes in inflammatory bowel diseases. Inflamm. Bowel Dis. 13, 537–546. doi: 10.1002/ibd.20094
Kaetsu, Y., Yamamoto, Y., Sugihara, S., Matsuura, T., Igawa, G., Matsubara, K., et al. (2007). Role of cysteinyl leukotrienes in the proliferation and the migration of murine vascular smooth muscle cells in vivo and in vitro. Cardiovasc. Res. 76, 160–166. doi: 10.1016/j.cardiores.2007.05.018
Kanaoka, Y., and Boyce, J. A. (2004). Cysteinyl leukotrienes and their receptors: cellular distribution and function in immune and inflammatory responses. J. Immunol. 173, 1503–1510. doi: 10.4049/jimmunol.173.3.1503
Kanaoka, Y., Maekawa, A., and Austen, K. F. (2013). Identification of GPR99 protein as a potential third cysteinyl leukotriene receptor with a preference for leukotriene E4 ligand. J. Biol. Chem. 288, 10967–10972. doi: 10.1074/jbc.C113.453704
Kanazawa, H., Yoshikawa, T., Hirata, K., and Yoshikawa, J. (2004). Effects of pranlukast administration on vascular endothelial growth factor levels in asthmatic patients. Chest 125, 1700–1705. doi: 10.1378/chest.125.5.1700
Kawano, T., Matsuse, H., Kondo, Y., Machida, I., Saeki, S., Tomari, S., et al. (2003). Cysteinyl leukotrienes induce nuclear factor kappa b activation and RANTES production in a murine model of asthma. J. Allergy Clin. Immunol. 112, 369–374. doi: 10.1067/mai.2003.1636
Kim, H. S., and Lee, G. (2015). The cysteinyl leukotriene receptor CysLTR1 mediates Th17 cell migration (CAM4P.156). J. Immunol. 194, 185.14.
Kinzler, K. W., and Vogelstein, B. (1996). Lessons from hereditary colorectal cancer. Cell 87, 159–170. doi: 10.1016/S0092-8674(00)81333-1
Laidlaw, T. M., and Boyce, J. A. (2012). Cysteinyl leukotriene receptors, old and new; implications for asthma. Clin. Exp. Allergy 42, 1313–1320. doi: 10.1111/j.1365-2222.2012.03982.x
Lam, B. K., Owen, W. F., Austen, K. F., and Soberman, R. J. (1989). The identification of a distinct export step following the biosynthesis of leukotriene C4 by human eosinophils. J. Biol. Chem. 264, 12885–12889.
Le, D. T., Uram, J. N., Wang, H., Bartlett, B. R., Kemberling, H., Eyring, A. D., et al. (2015). PD-1 blockade in tumors with mismatch-repair deficiency. N. Engl. J. Med. 372, 2509–2520. doi: 10.1056/NEJMoa1500596
Lee, K. S., Kim, S. R., Park, H. S., Jin, G. Y., Lee, Y. C., Bousquet, J., et al. (2004). Cysteinyl leukotriene receptor antagonist regulates vascular permeability by reducing vascular endothelial growth factor expression. J. Allergy Clin. Immunol. 114, 1093–1099. doi: 10.1016/j.jaci.2004.07.039
Liew W. C. (2015). Oxoglutarate receptor: oxoglutarate receptor. IUPHAR/BPS Guid. to Pharmacol. Available online at: http://www.guidetopharmacology.org/GRAC/ObjectDisplayForward?objectId=162. [Accessed June 28, 2016].
Lind, D. S., Hochwald, S. N., Malaty, J., Rekkas, S., Hebig, P., Mishra, G., et al. (2001). Nuclear factor-kappa B is upregulated in colorectal cancer. Surgery 130, 363–369. doi: 10.1067/msy.2001.116672
Lindgren, J. A., Stenke, L., Mansour, M., Edenius, C., Laurén, L., Näsman-Glaser, B., et al. (1993). Formation and effects of leukotrienes and lipoxins in human bone marrow. J. Lipid Mediat. 6, 313–320.
Longley, D. B., and Johnston, P. G. (2005). Molecular mechanisms of drug resistance. J. Pathol. 205, 275–292. doi: 10.1002/path.1706
Lynch, K. R., O'Neill, G. P., Liu, Q., Im, D. S., Sawyer, N., Metters, K. M., et al. (1999). Characterization of the human cysteinyl leukotriene CysLT1 receptor. Nature 399, 789–793.
MacKenzie, E. D., Selak, M. A., Tennant, D. A., Payne, L. J., Crosby, S., Frederiksen, C. M., et al. (2007). Cell-permeating alpha-ketoglutarate derivatives alleviate pseudohypoxia in succinate dehydrogenase-deficient cells. Mol. Cell. Biol. 27, 3282–3289. doi: 10.1128/MCB.01927-06
Madrid, L. V., Mayo, M. W., Reuther, J. Y., and Baldwin, A. S. (2001). Akt stimulates the transactivation potential of the RelA/p65 Subunit of NF-kappa B through utilization of the Ikappa B kinase and activation of the mitogen-activated protein kinase p38. J. Biol. Chem. 276, 18934–18940. doi: 10.1074/jbc.M101103200
Maekawa, A., Balestrieri, B., Austen, K. F., and Kanaoka, Y. (2009). GPR17 is a negative regulator of the cysteinyl leukotriene 1 receptor response to leukotriene D4. Proc. Natl. Acad. Sci. U.S.A. 106, 11685–11690. doi: 10.1073/pnas.0905364106
Magi, S., Takemoto, Y., Kobayashi, H., Kasamatsu, M., Akita, T., Tanaka, A., et al. (2014). 5-Lipoxygenase and cysteinyl leukotriene receptor 1 regulate epidermal growth factor-induced cell migration through Tiam1 upregulation and Rac1 activation. Cancer Sci. 105, 290–296. doi: 10.1111/cas.12340
Magnusson, C., Bengtsson, A. M., Liu, M., Liu, J., Ceder, Y., Ehrnström, R., et al. (2011a). Regulation of cysteinyl leukotriene receptor 2 expression–a potential anti-tumor mechanism. PLoS ONE 6:e29060. doi: 10.1371/journal.pone.0029060
Magnusson, C., Ehrnström, R., Olsen, J., and Sjölander, A. (2007). An increased expression of cysteinyl leukotriene 2 receptor in colorectal adenocarcinomas correlates with high differentiation. Cancer Res. 67, 9190–9198. doi: 10.1158/0008-5472.CAN-07-0771
Magnusson, C., Liu, J., Ehrnström, R., Manjer, J., Jirström, K., Andersson, T., et al. (2011b). Cysteinyl leukotriene receptor expression pattern affects migration of breast cancer cells and survival of breast cancer patients. Int. J. Cancer 129, 9–22. doi: 10.1002/ijc.25648
Magnusson, C., Mezhybovska, M., Lörinc, E., Fernebro, E., Nilbert, M., and Sjölander, A. (2010). Low expression of CysLT1R and high expression of CysLT2R mediate good prognosis in colorectal cancer. Eur. J. Cancer 46, 826–835. doi: 10.1016/j.ejca.2009.12.022
Martinelli, E., De Palma, R., Orditura, M., De Vita, F., and Ciardiello, F. (2009). Anti-epidermal growth factor receptor monoclonal antibodies in cancer therapy. Clin. Exp. Immunol. 158, 1–9. doi: 10.1111/j.1365-2249.2009.03992.x
Matsuyama, M., Funao, K., Hayama, T., Tanaka, T., Kawahito, Y., Sano, H., et al. (2009). Relationship between Cysteinyl-Leukotriene-1 receptor and human transitional cell carcinoma in bladder. Urology 73, 916–921. doi: 10.1016/j.urology.2008.11.005
Matsuyama, M., Hayama, T., Funao, K., Kawahito, Y., Sano, H., Takemoto, Y., et al. (2007). Overexpression of cysteinyl LT1 receptor in prostate cancer and CysLT1R antagonist inhibits prostate cancer cell growth through apoptosis. Oncol. Rep. 18, 99–104. doi: 10.3892/or.18.1.99
McCormack, P. L., and Keam, S. J. (2008). Bevacizumab: a review of its use in metastatic colorectal cancer. Drugs 68, 487–506. doi: 10.2165/00003495-200868040-00009
McDermott, D. F., and Atkins, M. B. (2013). PD-1 as a potential target in cancer therapy. Cancer Med. 2, 662–673. doi: 10.1002/cam4.106
Melstrom, L. G., Bentrem, D. J., Salabat, M. R., Kennedy, T. J., Ding, X.-Z., Strouch, M., et al. (2008). Overexpression of 5-lipoxygenase in colon polyps and cancer and the effect of 5-LOX inhibitors in vitro and in a murine model. Clin. Cancer Res. 14, 6525–6530. doi: 10.1158/1078-0432.CCR-07-4631
Mezhybovska, M., Wikström, K., Ohd, J. F., and Sjölander, A. (2006). The inflammatory mediator leukotriene D4 induces beta-catenin signaling and its association with antiapoptotic Bcl-2 in intestinal epithelial cells. J. Biol. Chem. 281, 6776–6784. doi: 10.1074/jbc.M509999200
Mezhybovska, M., Wikström, K., Ohd, J. F., Sjölander, A., Lynch, K. R., O'Neill, G. P., et al. (2005). Pro-inflammatory mediator leukotriene D4 induces transcriptional activity of potentially oncogenic genes. Biochem. Soc. Trans. 33, 698–700. doi: 10.1042/BST0330698
Mezhybovska, M., Yudina, Y., Abhyankar, A., and Sjölander, A. (2009). Beta-catenin is involved in alterations in mitochondrial activity in non-transformed intestinal epithelial and colon cancer cells. Br. J. Cancer 101, 1596–1605. doi: 10.1038/sj.bjc.6605342
Miller, D. M., Thomas, S. D., Islam, A., Muench, D., and Sedoris, K. (2012). c-Myc and cancer metabolism. Clin. Cancer Res. 18, 5546–5553. doi: 10.1158/1078-0432.CCR-12-0977
Ni, N. C., Ballantyne, L. L., Mewburn, J. D., and Funk, C. D. (2014). Multiple-site activation of the cysteinyl leukotriene receptor 2 is required for exacerbation of ischemia/reperfusion injury. Arterioscler. Thromb. Vasc. Biol. 34, 321–330. doi: 10.1161/ATVBAHA.113.302536
Nishikawa, M., Hikasa, Y., Hori, K., Tanida, N., and Shimoyama, T. (1995). Effect of leukotriene C4D4 antagonist on colonic damage induced by intracolonic administration of trinitrobenzene sulfonic acid in rats. J. Gastroenterol. 30, 34–40. doi: 10.1007/BF01211372
Ohd, J. F., Wikström, K., and Sjölander, A. (2000). Leukotrienes induce cell-survival signaling in intestinal epithelial cells. Gastroenterology 119, 1007–1018. doi: 10.1053/gast.2000.18141
Omede, A., Oceandy, D., Mamas, M., Cartwright, E., Zi, M., Prehar, S., et al. (2014). 177 The alpha-ketoglutarate receptor gpr99 regulates pathological cardiac hypertrophy. Heart 100, A100.1–A100. doi: 10.1136/heartjnl-2014-306118.177
Paruchuri, S., Broom, O., Dib, K., and Sjölander, A. (2005). The pro-inflammatory mediator leukotriene D4 induces phosphatidylinositol 3-kinase and Rac-dependent migration of intestinal epithelial cells. J. Biol. Chem. 280, 13538–13544. doi: 10.1074/jbc.M409811200
Paruchuri, S., Hallberg, B., Juhas, M., Larsson, C., and Sjölander, A. (2002). Leukotriene D(4) activates MAPK through a Ras-independent but PKCepsilon-dependent pathway in intestinal epithelial cells. J. Cell Sci. 115, 1883–1893.
Paruchuri, S., Mezhybovska, M., Juhas, M., and Sjölander, A. (2006). Endogenous production of leukotriene D4 mediates autocrine survival and proliferation via CysLT1 receptor signalling in intestinal epithelial cells. Oncogene 25, 6660–6665. doi: 10.1038/sj.onc.1209666
Paruchuri, S., and Sjölander, A. (2003). Leukotriene D4 mediates survival and proliferation via separate but parallel pathways in the human intestinal epithelial cell line Int 407. J. Biol. Chem. 278, 45577–45585. doi: 10.1074/jbc.M302881200
Peters-Golden, M., Gleason, M. M., and Togias, A. (2006). Cysteinyl leukotrienes: multi-functional mediators in allergic rhinitis. Clin. Exp. Allergy 36, 689–703. doi: 10.1111/j.1365-2222.2006.02498.x
Polyak, K., Li, Y., Zhu, H., Lengauer, C., Willson, J. K., Markowitz, S. D., et al. (1998). Somatic mutations of the mitochondrial genome in human colorectal tumours. Nat. Genet. 20, 291–293. doi: 10.1038/3108
Porreca, E., Di Febbo, C., Di Sciullo, A., Angelucci, D., Nasuti, M., Vitullo, P., et al. (1996). Cysteinyl leukotriene D4 induced vascular smooth muscle cell proliferation: a possible role in myointimal hyperplasia. Thromb. Haemost. 76, 99–104.
Poulin, S., Thompson, C., Thivierge, M., Véronneau, S., McMahon, S., Dubois, C. M., et al. (2011). Cysteinyl-leukotrienes induce vascular endothelial growth factor production in human monocytes and bronchial smooth muscle cells. Clin. Exp. Allergy 41, 204–217. doi: 10.1111/j.1365-2222.2010.03653.x
Reynolds, A. L., Alvarez, Y., Sasore, T., Waghorne, N., Butler, C. T., Kilty, C., et al. (2016). Phenotype-based discovery of 2-[(E)-2-(Quinolin-2-yl)vinyl]phenol as a novel regulator of ocular angiogenesis. J. Biol. Chem. 291, 7242–7255. doi: 10.1074/jbc.M115.710665
Rovati, G. E., Bäck, M., Dahlén, S.-E., Drazen, J., Evans, J. F., Shimizu, T., et al. (2015). Leukotriene receptors: CysLT2 receptor. IUPHAR/BPS Guid. to Pharmacol. Available online at: http://www.guidetopharmacology.org/GRAC/ObjectDisplayForward?objectId=270. [Accessed June 28, 2016].
Sakamoto, K. M., and Frank, D. A. (2009). CREB in the pathophysiology of cancer: implications for targeting transcription factors for cancer therapy. Clin. Cancer Res. 15, 2583–2587. doi: 10.1158/1078-0432.CCR-08-1137
Salim, T., Sand-Dejmek, J., and Sjölander, A. (2014). The inflammatory mediator leukotriene D4 induces subcellular β-catenin translocation and migration of colon cancer cells. Exp. Cell Res. 321, 255–266. doi: 10.1016/j.yexcr.2013.10.021
Savari, S., Liu, M., Zhang, Y., Sime, W., Sjölander, A., Wang, D., et al. (2013). CysLT1R antagonists inhibit tumor growth in a xenograft model of colon cancer. PLoS ONE 8:e73466. doi: 10.1371/journal.pone.0073466
Savari, S., Vinnakota, K., Zhang, Y., and Sjölander, A. (2014). Cysteinyl leukotrienes and their receptors: bridging inflammation and colorectal cancer. World J. Gastroenterol. 20, 968–977. doi: 10.3748/wjg.v20.i4.968
Shen, Z. (2011). Genomic instability and cancer: an introduction. J. Mol. Cell Biol. 3, 1–3. doi: 10.1093/jmcb/mjq057
Sirinyan, M., Sennlaub, F., Sapieha, P., and Chemtob, S. (2007). Succinate and -ketoglutarate act as sensors of hypoxia and mediate retinal angiogenesis. Invest. Ophthalmol. Vis. Sci. 48, 1755–1755. doi: 10.1038/nm.1873
Sjöström, M., Johansson, A.-S., Schröder, O., Qiu, H., Palmblad, J., and Haeggström, J. Z. (2003). Dominant expression of the CysLT2 receptor accounts for calcium signaling by cysteinyl leukotrienes in human umbilical vein endothelial cells. Arterioscler. Thromb. Vasc. Biol. 23, e37–e41. doi: 10.1161/01.ATV.0000082689.46538.DF
Sveinbjörnsson, B., Rasmuson, A., Baryawno, N., Wan, M., Pettersen, I., Ponthan, F., et al. (2008). Expression of enzymes and receptors of the leukotriene pathway in human neuroblastoma promotes tumor survival and provides a target for therapy. FASEB J. 22, 3525–3536. doi: 10.1096/fj.07-103457
Thompson, C., Cloutier, A., Bossé, Y., Poisson, C., Larivée, P., McDonald, P. P., et al. (2008). Signaling by the cysteinyl-leukotriene receptor 2. Involvement in chemokine gene transcription. J. Biol. Chem. 283, 1974–1984. doi: 10.1074/jbc.M608197200
Torre, L. A., Bray, F., Siegel, R. L., Ferlay, J., Lortet-Tieulent, J., and Jemal, A. (2015). Global cancer statistics, 2012. CA. Cancer J. Clin. 65, 87–108. doi: 10.3322/caac.21262
Tsopanoglou, N. E., Pipili-Synetos, E., and Maragoudakis, M. E. (1994). Leukotrienes C4 and D4 promote angiogenesis via a receptor-mediated interaction. Eur. J. Pharmacol. 258, 151–154. doi: 10.1016/0014-2999(94)90068-X
Wang, G., Yang, Z.-Q., and Zhang, K. (2010). Endoplasmic reticulum stress response in cancer: molecular mechanism and therapeutic potential. Am. J. Transl. Res. 2, 65–74.
Wikström, K., Ohd, J. F., and Sjölander, A. (2003). Regulation of leukotriene-dependent induction of cyclooxygenase-2 and Bcl-2. Biochem. Biophys. Res. Commun. 302, 330–335. doi: 10.1016/S0006-291X(03)00187-6
Xu, L., Zhang, L., Liu, L., Fang, S., Lu, Y., Wei, E., et al. (2010). Involvement of cysteinyl leukotriene receptors in angiogenesis in rat thoracic aortic rings. Pharmazie 65, 750–754. doi: 10.1691/ph.2010.9810
Yen, L.-C., Uen, Y.-H., Wu, D.-C., Lu, C.-Y., Yu, F.-J., Wu, I.-C., et al. (2010). Activating KRAS mutations and overexpression of epidermal growth factor receptor as independent predictors in metastatic colorectal cancer patients treated with cetuximab. Ann. Surg. 251, 254–260. doi: 10.1097/SLA.0b013e3181bc9d96
Yuan, Y.-M., Fang, S.-H., Qian, X.-D., Liu, L.-Y., Xu, L.-H., Shi, W.-Z., et al. (2009). Leukotriene D4 stimulates the migration but not proliferation of endothelial cells mediated by the cysteinyl leukotriene CysLT1 receptor via the extracellular signal-regulated kinase pathway. J. Pharmacol. Sci. 109, 285–292. doi: 10.1254/jphs.08321FP
Yudina, Y., Parhamifar, L., Bengtsson, A. M.-L., Juhas, M., Sjölander, A., Romano, M., et al. (2008). Regulation of the eicosanoid pathway by tumour necrosis factor alpha and leukotriene D4 in intestinal epithelial cells. Prostaglandins Leukot. Essent. Fatty Acids 79, 223–231. doi: 10.1016/j.plefa.2008.09.024
Keywords: cysteinyl leukotriene, eicosanoid, colorectal cancer, tumorigenesis, hallmarks of cancer, cysteinyl leukotriene receptor antagonist
Citation: Burke L, Butler CT, Murphy A, Moran B, Gallagher WM, O'Sullivan J and Kennedy BN (2016) Evaluation of Cysteinyl Leukotriene Signaling as a Therapeutic Target for Colorectal Cancer. Front. Cell Dev. Biol. 4:103. doi: 10.3389/fcell.2016.00103
Received: 14 July 2016; Accepted: 30 August 2016;
Published: 21 September 2016.
Edited by:
Hasan Korkaya, Augusta University, USAReviewed by:
Caroline Saucier, Université de Sherbrooke, CanadaCopyright © 2016 Burke, Butler, Murphy, Moran, Gallagher, O'Sullivan and Kennedy. This is an open-access article distributed under the terms of the Creative Commons Attribution License (CC BY). The use, distribution or reproduction in other forums is permitted, provided the original author(s) or licensor are credited and that the original publication in this journal is cited, in accordance with accepted academic practice. No use, distribution or reproduction is permitted which does not comply with these terms.
*Correspondence: Breandán N. Kennedy, YnJlbmRhbi5rZW5uZWR5QHVjZC5pZQ==
Disclaimer: All claims expressed in this article are solely those of the authors and do not necessarily represent those of their affiliated organizations, or those of the publisher, the editors and the reviewers. Any product that may be evaluated in this article or claim that may be made by its manufacturer is not guaranteed or endorsed by the publisher.
Research integrity at Frontiers
Learn more about the work of our research integrity team to safeguard the quality of each article we publish.