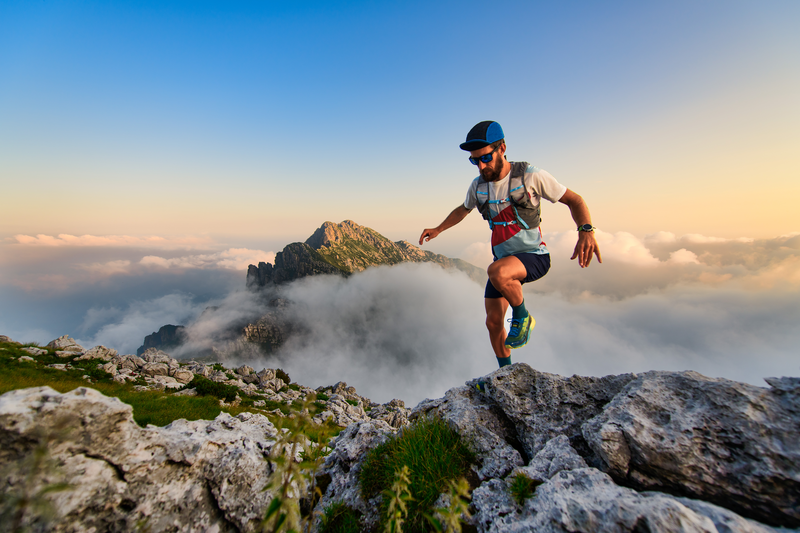
95% of researchers rate our articles as excellent or good
Learn more about the work of our research integrity team to safeguard the quality of each article we publish.
Find out more
SPECIALTY GRAND CHALLENGE article
Front. Catal. , 22 February 2021
Sec. Biocatalysis
Volume 1 - 2021 | https://doi.org/10.3389/fctls.2021.633893
Biocatalysis comprises the use of nature’s catalytic repertoire to facilitate chemical reactions (Sheldon and Woodley 2018; Sheldon and Brady 2019).
Enzymes catalyze a broad range of chemical transformations, generally under very mild reaction conditions and with high selectivity. These features make enzymes attractive catalysts for industrial chemical transformations, enabling less resource-consuming and waste-generating synthesis routes. Therefore, biocatalysis is already today an important pillar of chemistry and continues gaining relevance in academic research and in industrial application.
The last two decades have seen an exponential expansion of biocatalytic tools ranging from new catalysts with tailored properties to new reaction engineering concepts. As a result, the relevance of biocatalysis in the chemical industry has been also increasing steadily.
Today’s grand challenge in biocatalysis is to keep this impetus up and further consolidate and expand the toolbox of biocatalysis.
A few aspects will be highlighted in the following:
Although we already have access to a broad range of biocatalysts, there is still an urgent need for more enzymes/biocatalysts to cover an even larger space of chemical reactions and reaction conditions. Natural diversity represents a rich source of new enzymes obtained, for example, from metagenomic libraries and from sequence libraries (Lorenz and Eck 2005). Naturally occurring (wild-type) enzymes, however, have evolved to suit their host organisms’ needs and not the needs of the organic chemist. Fortunately, we now have tools of enzyme engineering at hand with which the properties of wild-type enzymes can be modified to suit the needs of chemical transformations (Bornscheuer et al. 2012; Arnold 2018; Qu et al. 2020). In principle, enzyme variants with tailored selectivity, substrate specificity, activity, and stability under “unnatural” reaction conditions can be generated using the tools of enzyme engineering (directed evolution, semi-rational design, and in silico design).
Nevertheless, enzyme engineering can be a tedious, time- and resource-consuming process, especially screening large libraries. Therefore, further improvements are urgently needed not only to generate new, tailored catalysts but also to expand our understanding of structure–property relationships. New high-throughput screening methods to assay huge libraries will allow for covering a larger sequence space and, maybe, also discovering “needles in the haystack” such as enzymes with unforeseen properties. Machine learning algorithms may also play a central role here. Likewise, new algorithms for enzyme modeling will enable us to more efficiently design enzyme variants.
While wild-type enzymes catalyze a broad range of useful chemical transformations, there are still many “white spots” in the reactivity landscape of biocatalysis. In the past years, we have seen how chemistry-inspired enzyme engineering resulted in new enzymes catalyzing bond formation reactions such as C-Si-, C-B-, C-C-, or C-N-bonds previously not conceivable in biocatalysis (Arnold 2018). Likewise, utilizing chemical concepts such as photochemical activation have resulted in new, “nonnatural” transformations using enzymes.
Chemically modified protein scaffolds, so-called hybrid enzymes, continue receiving considerable attention. Hybrid enzymes combine the chemical versatility of transition metal catalysis with the selectivity and specificity of enzymes exceeded by the well-defined three-dimensional structure of protein active sites (Steinreiber and Ward 2008).
Also, so-called Plurizymes, i.e., engineered enzymes exhibiting multiple active sites (Santiago et al. 2018) and catalyzing several transformations, represent an interesting next-generation approach for cascade reactions (vide infra) (Alonso et al. 2020).
We believe that we have just scratched the surface here and expect that further smart applications of chemical principles to enzyme reactions will result in more exciting transformations. But also nature still has a lot of surprises for us in the chest. New cofactors are constantly being discovered, enabling new chemical reactivities; but also the existing natural cofactor repertoire is waiting to be fully elucidated.
Today, biocatalysis research too often is stuck at the proof-of-concept level. Biocatalysis publications rarely surpass millimolar substrate concentrations. Chemical reactions, in contrast, generally entail reagent concentrations orders of magnitude higher or even neat (i.e., solvent-free) conditions. Bridging the “concentration gap” will be of utmost importance to further increase the acceptance of biocatalysis among organic chemists.
En route to this goal, we should reconsider the role of water as solvent of choice for biocatalytic reactions. For a large part of the reagents of interest, water is a suboptimal solvent and does not support the high reagent concentrations required. Water-soluble cosolvents can only partially compensate. Promising concepts comprise nonaqueous reaction media or two-phase reaction setups. Also new, tailored solvents may play a significant role here (Lozano et al. 2015; Lozano et al. 2017).
For a range of industrial applications, immobilized, recyclable biocatalysts are imperative (Sheldon and van Pelt 2013). Next to heterogenization and recovery of biocatalysts, enzyme immobilization offers further possibilities to tailor the properties of given enzymes. Multipoint and multisubunit immobilization can result in more robust enzymes; engineering of the microenvironment of the immobilized enzyme offers the possibility to tune enzyme activity (Mateo et al. 2007; Garcia-Galan et al. 2011). Co-immobilization of several enzymes enables exciting possibilities for multistep cascade reactions, but the pros and cons must be carefully considered (Arana-Peña et al. 2020). Tailoring of supports and enzymes may enable the one-step purification–immobilization of the target enzyme (Barbosa et al. 2015). Despite the progress and impressive examples of enzyme immobilization, this process remains largely trial and error. Rational approaches to design enzyme immobilization and maximize the residual activity and stability of the immobilisate are urgently needed.
“Environmental benignity” is a reoccurring claim in biocatalysis publications. Arguments used to substantiate this are mild reaction conditions, water as solvent, and the biobased-ness and biodegradability of enzymes. While we certainly agree that these features are likely to contribute to a low environmental impact of biocatalysis, we also emphasize that biocatalysis also has an environmental impact. Resources are consumed and wastes are generated during the preparation of the catalysts, during the reaction, and in downstream processing, overall placing a burden on the environment. We therefore urge all authors to use “green claims” very cautiously, ideally quantifying the resources consumed and wastes generated and comparing the environmental impact with existing reaction procedures.
All authors listed have made a substantial, direct, and intellectual contribution to the work and approved it for publication.
The authors declare that the research was conducted in the absence of any commercial or financial relationships that could be construed as a potential conflict of interest.
Alonso, S., Santiago, G., Cea-Rama, I., Fernandez-Lopez, L., Coscolín, C., Modregger, J., et al. (2020). Genetically engineered proteins with two active sites for enhanced biocatalysis and synergistic chemo- and biocatalysis. Nat. Catal. 3, 319–328. doi:10.1038/s41929-019-0394-4
Arana-Peña, S., Carballares, D., Morellon-Sterlling, R., Berenguer-Murcia, Á., Alcántara, A. R., Rodrigues, R. C., et al. (2020). Enzyme co-immobilization: always the biocatalyst designers’ choice…or not? Biotechnol. Adv., 107584. doi:10.1016/j.biotechadv.2020.107584
Arnold, F. H. (2018). Directed evolution: bringing new chemistry to life. Angew. Chem. Int. Ed. 57, 4143–4148. doi:10.1002/anie.201708408
Barbosa, O., Ortiz, C., Berenguer-Murciartiz, Á., Torres, R., Rodrigues, R. C., and Fernandez-Lafuente, R. (2015). Strategies for the one-step immobilization–purification of enzymes as industrial biocatalysts. Biotechnol. Adv. 33, 435–456. doi:10.1016/j.biotechadv.2015.03.006
Bornscheuer, U. T., Huisman, G. W., Kazlauskas, R. J., Lutz, S., Moore, J. C., and Robins, K. (2012). Engineering the third wave of biocatalysis. Nature 485, 185–194. doi:10.1038/nature11117
Garcia-Galan, C., Berenguer-Murcia, Á., Fernandez-Lafuente, R., and Rodrigues, R. C. (2011). Potential of different enzyme immobilization strategies to improve enzyme performance. Adv. Synth. Catal. 353, 2885–2904. doi:10.1002/adsc.201100534
Lorenz, P., and Eck, J. (2005). Metagenomics and industrial applications. Nat. Rev. Microbiol. 3, 510–516. doi:10.1038/nrmicro1161
Lozano, P., Bernal, J. M., Garcia-Verdugo, E., Sanchez-Gomez, G., Vaultier, M., Burguete, M. I., et al. (2015). Sponge-like ionic liquids: a new platform for green biocatalytic chemical processes. Green Chem. 17, 3706–3717. doi:10.1039/C5GC00894H
Lozano, P., Nieto, S., Serrano, J. L., Perez, J., Sánchez-Gomez, G., García-Verdugo, E., et al. (2017). Flow biocatalytic processes in ionic liquids and supercritical fluids. Mini-Rev. Org. Chem. 14, 65–74. doi:10.2174/1570193X13666161103145723
Mateo, C., Palomo, J. M., Fernandez-Lorente, G., Guisan, J. M., and Fernandez-Lafuente, R. (2007). Improvement of enzyme activity, stability and selectivity via immobilization techniques. Enz. Microb. Technol. 40, 1451–1463. doi:10.1016/j.enzmictec.2007.01.018
Qu, G., Li, A., Acevedo-Rocha, C. G., Sun, Z., and Reetz, M. T. (2020). The crucial role of methodology development in directed evolution of selective enzymes. Angew. Chem. Int. Ed. 59, 13204–13231. doi:10.1002/anie.201901491
Santiago, G., Martínez-Martínez, M., Alonso, S., Bargiela, R., Coscolín, C., Golyshin, P. N., et al. (2018). Rational engineering of multiple active sites in an ester hydrolase. Biochemistry 57, 2245–2255. doi:10.1021/acs.biochem.8b00274
Sheldon, R. A., and Brady, D. (2019). Broadening the scope of biocatalysis in sustainable organic synthesis. ChemSusChem 12, 2859–2881. doi:10.1002/cssc.201900351
Sheldon, R. A., and van Pelt, S. (2013). Enzyme immobilisation in biocatalysis: why, what and how. Chem. Soc. Rev. 42, 6223–6235. doi:10.1039/c3cs60075k
Sheldon, R. A., and Woodley, J. M. (2018). Role of biocatalysis in sustainable chemistry. Chem. Rev. 118, 801–838. doi:10.1021/acs.chemrev.7b00203
Keywords: biocatalysis, green chemistry, enzyme catalysis, new enzymes, process intensification (PI), new reactions
Citation: Hollmann F and Fernandez‐Lafuente R (2021) Grand Challenges in Biocatalysis. Front. Catal. 1:633893. doi: 10.3389/fctls.2021.633893
Received: 26 November 2020; Accepted: 20 January 2021;
Published: 22 February 2021.
Edited by:
Francesca Paradisi, University of Bern, SwitzerlandReviewed by:
Martina Letizia Contente, University of Nottingham, United KingdomCopyright © 2021 Fernandez-Lafuente and Hollmann. This is an open-access article distributed under the terms of the Creative Commons Attribution License (CC BY). The use, distribution or reproduction in other forums is permitted, provided the original author(s) and the copyright owner(s) are credited and that the original publication in this journal is cited, in accordance with accepted academic practice. No use, distribution or reproduction is permitted which does not comply with these terms.
*Correspondence: Roberto Fernandez‐Lafuente, cmZsQGljcC5jc2ljLmVz; Frank Hollmann, Zi5ob2xsbWFubkB0dWRlbGZ0Lm5s
Disclaimer: All claims expressed in this article are solely those of the authors and do not necessarily represent those of their affiliated organizations, or those of the publisher, the editors and the reviewers. Any product that may be evaluated in this article or claim that may be made by its manufacturer is not guaranteed or endorsed by the publisher.
Research integrity at Frontiers
Learn more about the work of our research integrity team to safeguard the quality of each article we publish.