- Division of Cardiology, The Second Hospital of Hebei Medical University, Shijiazhuang, Hebei, China
Objective: This study aims to systematically evaluate and perform a meta-analysis on the effects of LBBAP on right ventricular (RV) function by collecting data on Right Ventricular Fractional Area Change (RV-FAC), Tricuspid Annular Plane Systolic Excursion (TAPSE), Interventricular Mechanical Delay (IVMD), and the incidence of tricuspid regurgitation (TR) worsening in Left bundle branch area pacing (LBBAP) patients.
Methods: A comprehensive search was conducted for studies published from the establishment of the respective databases until October 2024 in PubMed, Embase, Web of Science, and the Cochrane Library. After screening and data extraction, the Newcastle-Ottawa Scale was used for the quality assessment of the included cohort studies, and meta-analysis was performed using R software. The effect size was estimated using either a random-effect model or a fixed-effect model, with odds ratio (OR) and mean difference (MD).
Results: A total of 14 studies were included, analyzing 1,555 LBBAP patients. The meta-analysis revealed that compared with intrinsic conduction, LBBAP implantation significantly improved RV-FAC (MD = 1.93; 95% CI: 0.64–3.23, P = 0.0034) and TAPSE (MD = 1.57; 95% CI: 1.07–2.06, P < 0.0001). Compared to the RVP group, LBBAP implantation significantly shortened IVMD (MD = −21.27; 95% CI: −31.33 to −11.22, P < 0.0001). For patients with RV dysfunction or right bundle branch block (RBBB), LBBAP implantation also significantly reduced IVMD (MD = −31.31; 95% CI: −37.10 to −25.52, P < 0.0001). The incidence of TR worsening within one year after LBBAP was approximately 8%, increasing to 23% beyond one year.
Conclusion: This meta-analysis demonstrates the superiority of LBBAP over intrinsic conduction in improving RV systolic function. Compared to RVP, LBBAP significantly enhances biventricular synchronization. Furthermore, LBBAP also improves ventricular synchronization in patients with RV dysfunction or RBBB.
1 Introduction
As a traditional pacing modality for the treatment of bradyarrhythmias, right ventricular pacing (RVP) has been successfully used in clinical practice for several decades. However, in patients who are dependent on ventricular pacing, long-term RVP can lead to dyssynchronous ventricular activation and impaired left ventricular function, ultimately resulting in heart failure (1). His bundle pacing, which stimulates the intrinsic conduction system to pace the myocardium, offers significant clinical benefits for patients with bradyarrhythmias or heart failure. However, the application of His bundle pacing is still limited by several factors, such as the complexity of the procedure, higher pacing thresholds, and lower R-wave amplitudes (2).
In recent years, left bundle branch area pacing (LBBAP) has emerged as a novel approach to achieving physiological pacing. By capturing the left bundle branch either partially or fully, it achieves left ventricular activation comparable to that of His bundle pacing. Compared to His bundle pacing, LBBAP offers superior pacing characteristics, including lower pacing thresholds and higher R-wave amplitudes (3). Due to these advantages, LBBAP has been widely adopted in clinical practice.
Despite the numerous advantages of left bundle branch area pacing (LBBAP), it also has certain limitations. While LBBAP achieves rapid left ventricular activation, it often results in delayed activation of the right ventricle, with the pacing electrocardiogram typically showing a right bundle branch block (RBBB) pattern (4). Although RBBB is generally considered benign, some clinical studies have suggested that patients with complete right bundle branch block and concomitant cardiopulmonary disease tend to have a higher incidence of adverse events (5, 6). Unlike the left heart system, clinical studies on the effects of LBBAP on the right heart system are primarily based on small cohort studies, and large-scale data comparisons are lacking. Therefore, a systematic review of the impact of LBBAP on right ventricular (RV) function is particularly important.
The article performs a systematic review and meta-analysis by collecting data on right ventricle fractional area change (RV-FAC), tricuspid annular systolic excursion (TAPSE), interventricular mechanical delay (IVMD), and the rate of tricuspid regurgitation (TR) deterioration in LBBAP patients, with the aim of investigating the impact of LBBAP on RV function.
2 Methods
2.1 Data sources and searches
We systematically searched the PubMed, Cochrane Library, Web of Science, and Embase databases for studies published from their inception through October 14, 2024, focusing on the impact of LBBAP on RV function. The search strategy for the databases, using PubMed as an example, was as follows: [left bundle branch pacing(Title/Abstract)] OR [left bundle branch area pacing(Title/Abstract)]. This study synthesized data from previously published research, and therefore, ethical approval was not required.
2.2 Study design
This study followed the Preferred Reporting Items for Systematic Reviews and Meta-Analyses (PRISMA) guidelines for reporting systematic reviews and meta-analyses (7). Clinical studies were considered eligible for inclusion if they met the following criteria: (1) Randomized controlled trials, cohort studies, case-control studies, or single-arm studies. (2) The study investigated the impact of LBBAP on RV function (non-acute hemodynamic changes). (3) Full-text articles published in English in peer-reviewed journals. (4) For studies with multiple publications from the same research, only the study with the largest population was included. Studies without original data, reviews, case reports, editorials, and animal studies were excluded.
2.3 Data extraction and quality appraisal
Two independent reviewers (Lei Yin and Lianxia Wang) conducted the search and reviewed the titles, abstracts, and full texts to determine eligible studies. Any disagreements regarding the decisions were resolved through consultation with a third-party reviewer (Ling You). The data extracted primarily included: (1) Baseline information: authors, publication year, study design, number of patients, and follow-up duration. (2) Primary clinical outcome measures: RV-FAC%, TAPSE. Secondary outcome measures: IVMD, TR deterioration rate. The quality of the included studies was assessed using the Newcastle-Ottawa Scale for cohort studies.
2.4 Statistical analysis
Data were analyzed using R version 4.2.1 statistical software (https://www.r-project.org, The R Foundation). The primary outcomes analyzed were RV-FAC, TAPSE, IVMD, and the TR deterioration rate. Descriptive statistics for continuous variables were expressed as means and standard deviations (SD), while categorical variables were presented as counts (n) and percentages (%). Values expressed as medians (interquartile range, IQR) were converted to means ± standard deviation for statistical analysis. For continuous variables, the difference in means (MD) with 95% confidence intervals (CI) was used for statistical description. For categorical variables, odds ratios (OR) with 95% CI were used for statistical description. Heterogeneity across studies was assessed using the I2 statistic. If I2 > 50%, significant heterogeneity was assumed, and a random-effects model was applied; otherwise, a fixed-effects model was used. In the presence of significant heterogeneity, sensitivity analyses were performed by sequentially omitting one study at a time to assess the impact of each individual study on the overall risk estimate. Potential publication bias was evaluated using Egger's test. Statistical significance was set at p < 0.05.
3 Results
3.1 Study selection and quality assessment
A total of 3,397 articles were retrieved from the following databases: PubMed (894), Cochrane Library (324), Web of Science (952), and Embase (1,227). After removing duplicates, 1,855 articles remained. These articles were screened by reviewing the titles, abstracts, and full texts, resulting in 23 clinical studies for further evaluation (see Figure 1). Among these, two studies originated from the same center, with patient enrollment occurring from January 2018 to August 2020. Since some patients were overlapping in these studies, we ultimately selected the study with the largest sample size (8, 9). Additionally, two clinical studies, which focused on acute-phase right ventricular hemodynamic parameters, were excluded from the analysis (10, 11). In the end, 14 clinical studies met our inclusion criteria. All the included studies were cohort studies, comprising a total of 1,555 LBBAP patients. The demographic and clinical characteristics of the included studies are presented in Table 1.
3.2 Effect of LBBAP on right ventricular fractional area change (RV-FAC)
A total of four studies were included, involving 275 LBBAP patients. The analysis showed no significant heterogeneity between the two groups (P = 0.4002, I2 = 2.5%), so a fixed-effects model was used. Sensitivity analysis revealed that the combined interval did not show significant variation, ranging from 1.5759 [0.2119; 2.9400] to 2.5655 [1.0641; 4.0668]. Although the Egger test indicated the presence of publication bias (P = 0.0271), the trim-and-fill method did not reveal any significant changes in the results, suggesting the findings were robust (MD = 1.37; 95% CI: 0.19–2.56, P = 0.0233). When compared with intrinsic conduction, the implantation of LBBAP significantly improved RV-FAC in patients (MD = 1.93; 95% CI: 0.64–3.23, P = 0.0034) (see Figure 2).
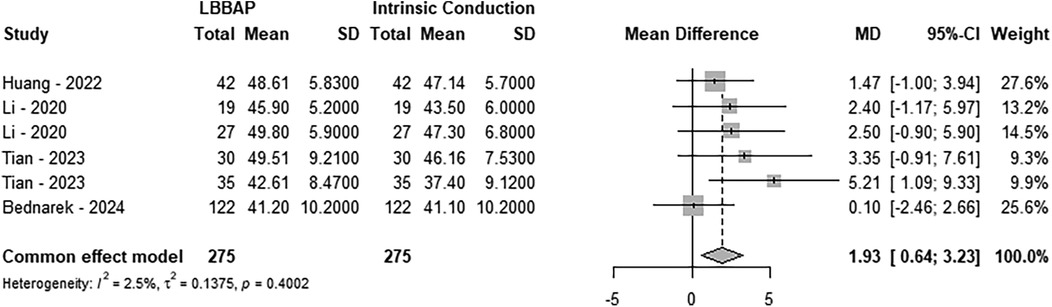
Figure 2. Forest plot of the right ventricle fractional area change. Comparison of the right ventricle fractional area change between LBBAP and intrinsic conduction.
3.3 Impact of LBBAP on tricuspid annular plane systolic excursion (TAPSE)
A total of four studies, comprising 260 LBBAP patients, were included in the analysis. The results indicated no significant heterogeneity between the two groups (P = 0.1761, I2 = 34.7%), and a fixed-effect model was applied. Sensitivity analysis demonstrated that the combined interval did not exhibit significant variation, with a range from 1.3023 [0.7631; 1.8415] to 1.7340 [1.1860; 2.2821]. Furthermore, the Egger test revealed no publication bias (P = 0.67). Compared to intrinsic conduction, LBBAP implantation significantly improved patients’ TAPSE (MD = 1.57; 95% CI: 1.07–2.06, P < 0.0001), as shown in Figure 3.
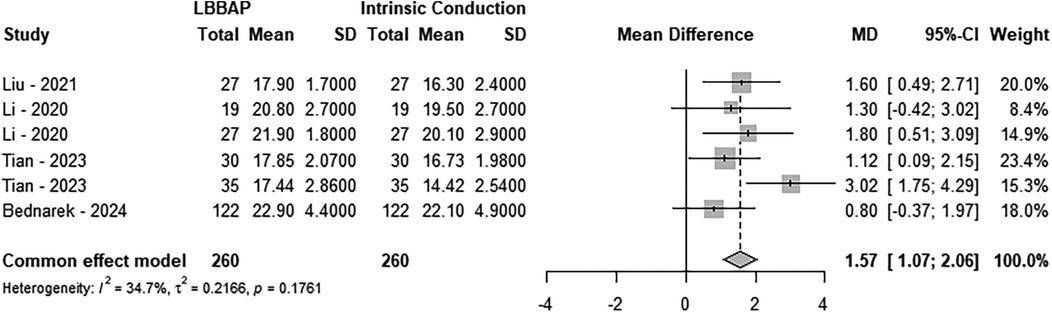
Figure 3. Forest plot of the tricuspid annular plane systolic excusion. Comparison of the tricuspid annular plane systolic excusion between LBBAP and intrinsic conduction.
3.4 Impact of LBBAP on interventricular mechanical delay (IVMD)
A total of 4 studies involving 199 patients were included in this analysis, with 107 patients in the LBBAP group and 92 patients in the RVP group. The results revealed significant heterogeneity between the two groups (P < 0.0001, I2 = 87.3%). Consequently, a random-effects model was applied. Sensitivity analysis demonstrated no substantial variation in the combined confidence intervals, which ranged from −18.0179 [−29.2156; −6.8202] to −25.6888 [−35.2327; −16.1450]. Furthermore, the Egger test indicated no evidence of publication bias (P = 0.3180). When comparing the two groups, LBBAP implantation significantly reduced IVMD compared to RVP (MD = −21.27; 95% CI: −31.33 to −11.22, P < 0.0001) (Figure 4).
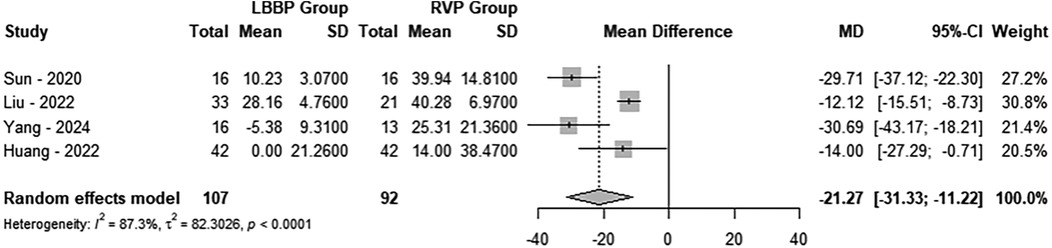
Figure 4. Forest plot of the interventricular mechanical delay. Comparison of the interventricular mechanical delay between LBBAP group and RVP group.
To further investigate the impact of LBBAP on RV function, we included two studies focused on patients with RV dysfunction or RBBB, comprising a total of 68 patients who received LBBAP. The results showed no significant heterogeneity between the two groups (P = 0.4488, I2 = 0.0%), allowing for the use of a fixed-effects model. Compared to native conduction, LBBAP implantation significantly reduced the IVMD in patients with RV dysfunction or RBBB (MD = −31.31; 95% CI: −37.10 to −25.52, P < 0.0001) (Figure 5).

Figure 5. Forest plot of the interventricular mechanical delay. Comparison of the interventricular mechanical delay between LBBAP group and intrinsic conduction.
3.5 Incidence of tricuspid regurgitation worsening in LBBAP patients
A total of 7 studies were included, comprising 1,226 LBBAP patients. Based on the follow-up duration, the studies were divided into two groups. In the group with a follow-up duration ≤12 months, 5 studies were included, totaling 1,013 LBBAP patients. The incidence of TR worsening was found to follow a normal distribution and was analyzed using the raw rate for meta-analysis (Shapiro-Wilk normality test = 0.6923). The results showed significant heterogeneity between the two groups (P < 0.0001, I2 = 94.7%), and a random-effects model was applied. Sensitivity analysis demonstrated no significant changes in the combined interval, ranging from 0.06 [0.0000; 0.1294] to 0.10 [0.0258; 0.1745]. Moreover, Egger's test revealed no publication bias (P = 0.5558). In the group with follow-up ≤12 months, 8% of the population experienced worsening of TR (Figure 6).
A total of 3 studies were included, comprising 482 LBBAP patients, in the group with follow-up duration >12 months. The incidence of TR worsening followed a normal distribution and was analyzed using the raw rate for meta-analysis (Shapiro–Wilk normality test = 0.6923). The results revealed significant heterogeneity between the two groups (P = 0.0014, I2 = 84.8%), and a random-effects model was applied. Sensitivity analysis showed that the combined interval ranged from 0.1798 [0.1052; 0.2544] to 0.2767 [0.1441; 0.4094]. Furthermore, Egger's test indicated no evidence of publication bias (P = 0.5788). In the group with follow-up >12 months, 23% of patients experienced worsening of TR (Figure 7).
4 Discussion
The main findings of this study are as follows:
1. LBBAP significantly improves right ventricular systolic function in patients with pacing indications.
2. Compared to RVP, LBBAP significantly improves left and right ventricular synchrony. Additionally, LBBAP also enhances interventricular synchrony in patients with right ventricular dysfunction or right bundle branch block.
3. Meta-analysis results indicate that the incidence of tricuspid regurgitation worsening within the first year after LBBAP is approximately 8%, whereas the TR worsening rate increases to 23% after one year.
4.1 Right ventricular systolic function in LBBAP patients
Although LBBAP patients often present with RBBB patterns on their electrocardiogram (ECG), this does not appear to significantly impact RV systolic function. In the current study, RV-FAC and TAPSE were used to assess post-operative RV systolic function in LBBAP patients. RV-FAC typically reflects overall RV systolic function, while TAPSE assesses longitudinal RV systolic function. The results showed that both RV-FAC and TAPSE were significantly improved in LBBAP patients compared to those with intrinsic conduction. These findings are generally consistent with previous clinical studies (12–14). Additionally, Bednarek et al. (14) conducted a follow-up study of 122 LBBAP patients, with a median duration of 21 months. Through multivariate analysis, they identified that the improvement in left ventricular ejection fraction was the main independent predictor of improved RV systolic function in LBBAP patients. The interval or amplitude of the terminal R wave in lead V1 showed no statistical significance in relation to RV systolic function improvement, suggesting that the RBBB pattern in LBBAP patients may differ from conventional RBBB. Previous studies have also indicated that 20%–40% of RV systolic pressure and flow are influenced by left ventricular contraction (15). Considering that LBBAP significantly improves left ventricular electromechanical synchrony and reduces mitral regurgitation (16, 17), the interaction between the left and right ventricles may be a key mechanism driving the observed improvement in RV function following LBBAP. Furthermore, LBBAP may improve RV function by preferentially activating the left ventricle (13).
4.2 Synchronous function of the left and right ventricles
Ozpak et al. evaluated right ventricular activation by measuring the time from the QRS onset to the peak of the R wave in lead V1 (V1RWPT). They found that the V1RWPT in LBBAP patients was between that of complete RBBB and incomplete RBBB, with a tendency toward incomplete RBBB [42 ms (28, 55) vs. 42 ms (35, 49), P = 0.49] (18). This suggests that the RBBB induced by LBBAP may be improved, or even corrected, through mechanisms such as lateral connections between the left and right bundle branches, retrograde conduction of the left bundle branch signal, and intermyocardial electrical conduction (4). Our meta-analysis results also demonstrate that LBBAP patients, compared to those undergoing RVP, have a significantly shorter IVMD. Additionally, the findings suggest that LBBAP improves interventricular synchrony in patients with RV dysfunction or RBBB. Some studies also examined the effects of LBBAP in both non-RBBB and RBBB patients. The results revealed no significant differences between the two groups in right ventricular free wall strain, the standard deviation of peak time across three segments of the right ventricular free wall, TAPSE, or IVMD. However, in the RBBB group, there was a significant delay in the peak time difference during systole between the tricuspid and mitral valves (△TSTV-MV) and the displacement peak time difference (△PTTV-MV) when compared to the non-RBBB group (47.29 ± 58.45 vs. −12.00 ± 49.91, P = 0.02; 28.14 ± 39.04 vs. −28.00 ± 48.26, P = 0.01) (19). These findings suggest that LBBAP may be a viable option for patients with right bundle branch block who require pacing intervention.
In addition, recently proposed techniques such as anodal capture and bilateral bundle capture have provided alternative feasible options for patients with right bundle branch block. Ali et al (20). demonstrated that anodal capture significantly shortened both the QRS duration and total ventricular activation time (116 ± 12 ms vs. 129 ± 14 ms, P < 0.01 and 83 ± 18 ms vs. 90 ± 15 ms, P = 0.01). Lin et al. (21) achieved favorable pacing electrocardiographic parameters by simultaneously capturing both the left and right bundle branches using the tip and ring electrodes of the lead. As implantation techniques mature and lead technology improves, these approaches may have broader clinical applications in the future (22).
4.3 Incidence of tricuspid regurgitation (TR) deterioration
Due to the implantation of the LBBAP electrode in the right ventricular septum, improper handling during the procedure may potentially damage surrounding structures, thereby affecting RV function. Our meta-analysis results indicate that in the population with a follow-up time of ≤12 months, approximately 8% of LBBAP patients experience deterioration of tricuspid regurgitation. In the population with a follow-up time of >12 months, the incidence of TR deterioration in LBBAP patients rises to 23%. The comparison of TR deterioration rates between the RVP and LBBAP groups is currently a topic of interest. However, given that the meta-analysis results for the RVP and LBBAP groups in the included studies were not robust, we did not perform further analysis. Although the original research team of LBBAP suggested that approximately 30% of LBBAP patients experience improvement in TR, it remains critical to avoid damage to tricuspid valve structures during the surgical procedure (3). A recent meta-analysis identified the distance between the lead-implant site and the tricuspid valve annulus (lead-TA distance) as an important predictor of TR deterioration. A longer lead-TA distance significantly reduces the likelihood of TR deterioration (23). Two related studies from Fuwai Hospital, which used intraoperative mapping of the left bundle branch potential, suggested that the left bundle branch trunk is primarily located 15–35 mm from the tricuspid valve annulus. Based on these findings, they recommend that the LBBAP electrode be implanted at a distance greater than 19 mm from the tricuspid valve annulus to reduce the risk of TR deterioration (24, 25). In addition, the use of intracardiac echocardiogram during the procedure can further reduce the occurrence of such complications (26).
This study has several limitations: ① All the studies included in this research are cohort studies, and the lack of randomized controlled trials means that the findings are not corroborated by more rigorous study designs. ② The definition of left bundle branch pacing in the existing literature is inconsistent, and we did not clearly distinguish between left bundle branch pacing and left ventricular septal pacing. This lack of differentiation may have impacted the accuracy of our results to some extent. ③ The literature on evaluating right heart function in LBBAP patients is relatively limited, and the methods used are somewhat homogeneous. As a result, this study was unable to provide a comprehensive and detailed assessment of the effects of LBBAP on the right heart system. Research on Doppler tissue and strain assessment will provide more information on RV function. ④ Given that LBBAP may influence right heart function through improvement in left ventricular function, the improvement in RV function might be more significant in populations with reduced ejection fraction. However, due to limitations in the included studies, we were unable to conduct further subgroup analyses based on ejection fraction. ⑤ The studies included in this research all used the Medtronic 3,830 lead, and did not further explore the impact of Stylet-driven leads on right ventricular function.
5 Conclusion
This meta-analysis demonstrates the superiority of LBBAP over intrinsic conduction in improving RV systolic function. Compared to RVP, LBBAP significantly enhances biventricular synchronization. Furthermore, LBBAP also improves ventricular synchronization in patients with RV dysfunction or right bundle branch block (RBBB).
Author contributions
LY: Software, Writing – original draft. LW: Methodology, Writing – review & editing. JM: Investigation, Writing – review & editing. QL: Methodology, Writing – review & editing. YZhan: Validation, Writing – review & editing. YZhao: Formal Analysis, Writing – review & editing. ML: Data curation, Methodology, Writing – review & editing. LY: Funding acquisition, Resources, Writing – review & editing.
Funding
The author(s) declare that no financial support was received for the research, authorship, and/or publication of this article.
Conflict of interest
The authors declare that the research was conducted in the absence of any commercial or financial relationships that could be construed as a potential conflict of interest.
Generative AI statement
The author(s) declare that no Generative AI was used in the creation of this manuscript.
Publisher's note
All claims expressed in this article are solely those of the authors and do not necessarily represent those of their affiliated organizations, or those of the publisher, the editors and the reviewers. Any product that may be evaluated in this article, or claim that may be made by its manufacturer, is not guaranteed or endorsed by the publisher.
References
1. Somma V, Ha FJ, Palmer S, Mohamed U, Agarwal S. Pacing-induced cardiomyopathy: a systematic review and meta-analysis of definition, prevalence, risk factors, and management. Heart Rhythm. (2023) 20(2):282–90. doi: 10.1016/j.hrthm.2022.09.019
2. Kuang X, Zhang X, Cui Y, Wei F, Wu P, Gao X, et al. Intracardiac echocardiography-guided implantation for proximal left bundle branch pacing. Circ Arrhythm Electrophysiol. (2023) 16(4):e011408. doi: 10.1161/CIRCEP.122.011408
3. Su L, Wang S, Wu S, Xu L, Huang Z, Chen X, et al. Long-term safety and feasibility of left bundle branch pacing in a large single-center study. Circ Arrhythm Electrophysiol. (2021) 14(2):e009261. doi: 10.1161/CIRCEP.120.009261
4. Zhu K, Sun Y, Lin M, Deng Y, Li L, Li G, et al. The physiologic mechanisms of paced QRS narrowing during left bundle branch pacing in right bundle branch block patients. Front Cardiovasc Med. (2022) 9:835493. doi: 10.3389/fcvm.2022.835493
5. Houston BA, Sturdivant JL, Yu Y, Gold MR. Acute biventricular hemodynamic effects of cardiac resynchronization therapy in right bundle branch block. Heart Rhythm. (2018) 15(10):1525–32. doi: 10.1016/j.hrthm.2018.05.017
6. Bussink BE, Holst AG, Jespersen L, Deckers JW, Jensen GB, Prescott E. Right bundle branch block: prevalence, risk factors, and outcome in the general population: results from the Copenhagen city heart study. Eur Heart J. (2013) 34(2):138–46. doi: 10.1093/eurheartj/ehs291
7. Page MJ, McKenzie JE, Bossuyt PM, Boutron I, Hoffmann TC, Mulrow CD, et al. The PRISMA 2020 statement: an updated guideline for reporting systematic reviews. J Clin Epidemiol. (2021) 134:178–89. doi: 10.1016/j.jclinepi.2021.03.001
8. Hu Q, Lu W, Chen K, Dai Y, Lin J, Xu N, et al. Long-term follow-up results of patients with left bundle branch pacing and exploration for potential factors affecting cardiac function. Front Physiol. (2022) 13:996640. doi: 10.3389/fphys.2022.996640
9. Hu Q, You H, Chen K, Dai Y, Lu W, Li Y, et al. Distance between the lead-implanted site and tricuspid valve annulus in patients with left bundle branch pacing: effects on postoperative tricuspid regurgitation deterioration. Heart Rhythm. (2023) 20(2):217–23. doi: 10.1016/j.hrthm.2022.10.027
10. Xie H, Chen X, Wang Y, Cheng Y, Zhao Y, Liu Y, et al. Comparison of the acute effects of different pacing sites on cardiac synchrony and contraction using speckle-tracking echocardiography. Front Cardiovasc Med. (2021) 8:758500. doi: 10.3389/fcvm.2021.758500
11. Chen X, Zhou X, Wang Y, Jin Q, Chen Y, Wang J, et al. Evaluation of electrophysiological characteristics and ventricular synchrony: an intrapatient-controlled study during his-purkinje conduction system pacing versus right ventricular pacing. Clin Cardiol. (2022) 45(7):723–32. doi: 10.1002/clc.23837
12. Huang X, Lin M, Huang S, Guo J, Li L, Chen S, et al. Impact on right ventricular performance in patients undergoing permanent pacemaker implantation: left bundle branch pacing versus right ventricular septum pacing. J Cardiovasc Electrophysiol. (2022) 33(12):2614–24. doi: 10.1111/jce.15675
13. Tian F, Weng H, Liu A, Liu W, Zhang B, Wang Y, et al. Effect of left bundle branch pacing on right ventricular function: a 3-dimensional echocardiography study. Heart Rhythm. (2024) 21(4):445–53. doi: 10.1016/j.hrthm.2023.12.012
14. Bednarek A, Kiełbasa G, Moskal P, Ostrowska A, Bednarski A, Sondej T, et al. Left bundle branch area pacing improves right ventricular function and synchrony. Heart Rhythm. (2024) 21(11):2234–41. doi: 10.1016/j.hrthm.2024.05.019
15. Sheehan F, Redington A. The right ventricle: anatomy, physiology and clinical imaging. Heart. (2008) 94(11):1510–5. doi: 10.1136/hrt.2007.132779
16. Leventopoulos G, Travlos CK, Aronis KN, Anagnostopoulou V, Patrinos P, Papageorgiou A, et al. Safety and efficacy of left bundle branch area pacing compared with right ventricular pacing in patients with bradyarrhythmia and conduction system disorders: systematic review and meta-analysis. Int J Cardiol. (2023) 390:131230. doi: 10.1016/j.ijcard.2023.131230
17. Ponnusamy SS, Syed T, Vijayaraman P. Response of functional mitral regurgitation in nonischemic cardiomyopathy to left bundle branch pacing. Heart Rhythm. (2022) 19(5):737–45. doi: 10.1016/j.hrthm.2022.01.019
18. Ozpak E, Demolder A, Kizilkilic S, Calle S, Timmermans F, De Pooter J. An electrocardiographic characterization of left bundle branch area pacing-induced right ventricular activation delay: a comparison with native right bundle branch block. Front Cardiovasc Med. (2022) 9:885201. doi: 10.3389/fcvm.2022.885201
19. Zhao R, Xiong F, Deng X, Wang S, Liu C, Xu M, et al. Early assessment of ventricular synchronization and function after left bundle-branch-area pacing with right bundle-branch block. BMC Cardiovasc Disord. (2022) 22(1):380. doi: 10.1186/s12872-022-02818-z
20. Ali N, Saqi K, Arnold AD, Miyazawa AA, Keene D, Chow JJ, et al. Left bundle branch pacing with and without anodal capture: impact on ventricular activation pattern and acute haemodynamics. Europace. (2023) 25(10):euad264. doi: 10.1093/europace/euad264
21. Lin J, Chen K, Dai Y, Sun Q, Li Y, Jiang Y, et al. Bilateral bundle branch area pacing to achieve physiological conduction system activation. Circ Arrhythm Electrophysiol. (2020) 13(8):e008267. doi: 10.1161/CIRCEP.119.008267
22. Lu W, Lin J, Li Y, Hu Q, Cheng C, Chen R, et al. Quantitative analysis reveals influencing factors to facilitate successful anodal-ring capture in left bundle branch pacing. Europace. (2023) 25(6):euad172. doi: 10.1093/europace/euad172
23. Karwiky G, Kamarullah W, Pranata R, Achmad C, Iqbal M. A meta-analysis of the distance between lead-implanted site and tricuspid valve annulus with postoperative tricuspid regurgitation deterioration in patients with left bundle branch area pacing. J Cardiovasc Electrophysiol. (2024) 35(11):2220–9. doi: 10.1111/jce.16444
24. Li X, Zhu H, Fan X, Wang Q, Wang Z, Li H, et al. Tricuspid regurgitation outcomes in left bundle branch area pacing and comparison with right ventricular septal pacing. Heart Rhythm. (2022) 19(7):1202–3. doi: 10.1016/j.hrthm.2022.03.005
25. Liu X, Niu HX, Gu M, Chen X, Hu Y, Cai M, et al. Contrast-enhanced image-guided lead deployment for left bundle branch pacing. Heart Rhythm. (2021) 18(8):1318–25. doi: 10.1016/j.hrthm.2021.04.015
Keywords: left bundle branch area pacing, right ventricular function, meta-analysis, right ventricular fractional area change, interventricular mechanical delay
Citation: Yin L, Wang L, Meng J, Liu Q, Zhang Y, Zhao Y, Li M and You L (2025) A systematic review and meta-analysis of the impact of left bundle branch area pacing on right ventricular function. Front. Cardiovasc. Med. 12:1545757. doi: 10.3389/fcvm.2025.1545757
Received: 15 December 2024; Accepted: 20 January 2025;
Published: 30 January 2025.
Edited by:
Vassil Traykov, Acibadem City Clinic Tokuda Hospital, BulgariaReviewed by:
Sandro Brusich, Clinical Hospital Centre Rijeka, CroatiaEnes Gul, Necmettin Erbakan University, Türkiye
Georgios Leventopoulos, General University Hospital of Patras, Greece
Copyright: © 2025 Yin, Wang, Meng, Liu, Zhang, Zhao, Li and You. This is an open-access article distributed under the terms of the Creative Commons Attribution License (CC BY). The use, distribution or reproduction in other forums is permitted, provided the original author(s) and the copyright owner(s) are credited and that the original publication in this journal is cited, in accordance with accepted academic practice. No use, distribution or reproduction is permitted which does not comply with these terms.
*Correspondence: Ling You, eW91bGluZ0BoZWJtdS5lZHUuY24=
†These authors have contributed equally to this work