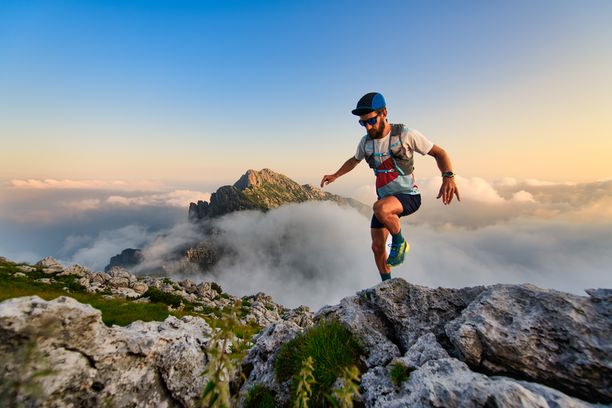
94% of researchers rate our articles as excellent or good
Learn more about the work of our research integrity team to safeguard the quality of each article we publish.
Find out more
ORIGINAL RESEARCH article
Front. Cardiovasc. Med.
Sec. General Cardiovascular Medicine
Volume 12 - 2025 | doi: 10.3389/fcvm.2025.1514564
This article is part of the Research TopicNovel Role and Mechanisms of Inflammation in Myocardial InfarctionView all articles
The final, formatted version of the article will be published soon.
Select one of your emails
You have multiple emails registered with Frontiers:
Notify me on publication
Please enter your email address:
If you already have an account, please login
You don't have a Frontiers account ? You can register here
Background: In-hospital cardiac arrest (IHCA) refers to the occurrence of cardiac arrest in hospitalized patients requiring chest compressions and/or defibrillation, with only about one-third of patients achieving return of spontaneous circulation (ROSC) after cardiopulmonary resuscitation. Pan-immuneinflammation value (PIIV) is an indicator assessing the overall inflammatory status within the body, but the relationship between PIIV and ROSC remains unclear. Objective: This study aims to analyze the occurrence of ROSC and its influencing factors, and investigate the predictive value of PIIV, in order to provide insights for clinical prevention and treatment. Methods: Clinical data of IHCA patients admitted to our hospital were retrospectively collected. Patients were divided into the ROSC group and non-ROSC group based on whether spontaneous circulation was restored after cardiopulmonary resuscitation. Multivariate logistic regression was used to analyze factors affecting ROSC, and the receiver operating characteristic (ROC) curve was employed to calculate the area under the curve (AUC) to evaluate the predictive value of PIIV. Results: 168 patients' clinical data were collected, including 62 patients with ROSC and 106 with non-ROSC. The results of multivariate logistic regression analysis showed that the duration of cardiopulmonary resuscitation, adrenaline dosage, blood lactate (Lac), and PIIV were independent influencing factors for ROSC in IHCA patients (P < 0.05). The ROC curve analysis revealed that the AUC of PIIV for predicting ROSC in IHCA patients was 0.805 (95% CI: 0.720-0.891), with an optimal cutoff value of 395.3, sensitivity of 83.33%, and specificity of 70.37%. Conclusion:PIIV demonstrates valuable application in predicting ROSC in IHCA patients.
Keywords: In-hospital cardiac arrest, Cardiopulmonary Resuscitation, Return of spontaneous circulation, panimmune-inflammation value, Influencing factors
Received: 21 Oct 2024; Accepted: 18 Mar 2025.
Copyright: © 2025 Wang and Kong. This is an open-access article distributed under the terms of the Creative Commons Attribution License (CC BY). The use, distribution or reproduction in other forums is permitted, provided the original author(s) or licensor are credited and that the original publication in this journal is cited, in accordance with accepted academic practice. No use, distribution or reproduction is permitted which does not comply with these terms.
* Correspondence:
Xiao Wang, Zhengzhou University, Zhengzhou, China
Tao Kong, First Affiliated Hospital of Zhengzhou University, Zhengzhou, 450052, Henan Province, China
Disclaimer: All claims expressed in this article are solely those of the authors and do not necessarily represent those of their affiliated organizations, or those of the publisher, the editors and the reviewers. Any product that may be evaluated in this article or claim that may be made by its manufacturer is not guaranteed or endorsed by the publisher.
Supplementary Material
Research integrity at Frontiers
Learn more about the work of our research integrity team to safeguard the quality of each article we publish.