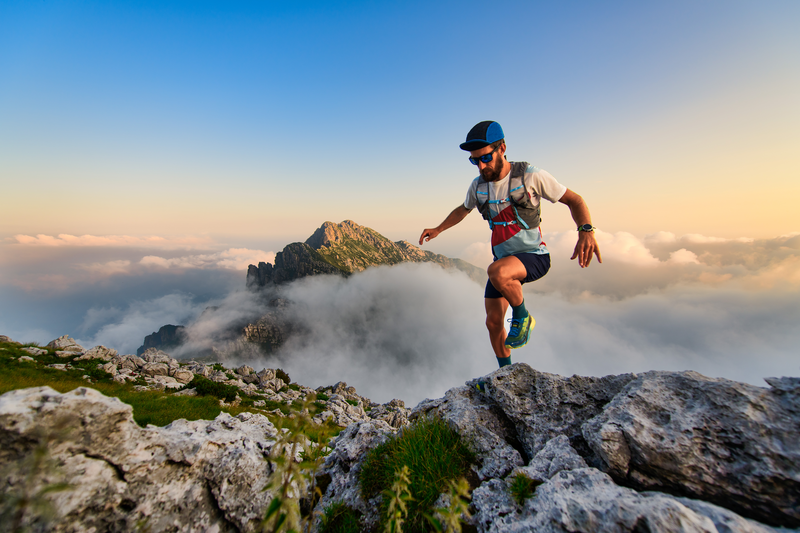
94% of researchers rate our articles as excellent or good
Learn more about the work of our research integrity team to safeguard the quality of each article we publish.
Find out more
REVIEW article
Front. Cardiovasc. Med.
Sec. Coronary Artery Disease
Volume 12 - 2025 | doi: 10.3389/fcvm.2025.1507665
The final, formatted version of the article will be published soon.
You have multiple emails registered with Frontiers:
Please enter your email address:
If you already have an account, please login
You don't have a Frontiers account ? You can register here
Cardio-cerebral infarction (CCI) is a rare clinical syndrome characterized by the simultaneous or sequential occurrence of acute myocardial infarction (AMI) and acute ischemic stroke (AIS). Despite its complex pathogenesis and more severe prognosis compared to isolated AMI or AIS, no consensus has been established regarding its definition, classification, epidemiology, treatment protocols, or prognostic management. Current research is largely confined to case reports or small case series, and there are no unified diagnostic or treatment guidelines, nor any expert consensus. Consequently, clinicians often rely on single-disease guidelines for AMI or AIS, or personal experience, when managing CCI cases. This approach complicates treatment decisions and may result in missed opportunities for optimal interventions, thereby adversely affecting long-term patient outcomes. This narrative review aimed to systematically summarize the definition, classification, epidemiological features, pathogenesis, diagnostic and therapeutic strategies, and prognostic aspects of CCI while thoroughly examining the progress and limitations of existing studies to guide future research and clinical practice. By offering a detailed analysis of reperfusion strategies, antiplatelet therapy, and anticoagulation in CCI patients, this review highlights the safety and efficacy differences among current treatments and explores methods for optimizing individualized management to improve clinical outcomes. Furthermore, This article aimed to enhance clinicians' understanding of CCI, provide evidence-based recommendations for patient care, and outline directions for future research. Ultimately, by refining diagnostic and therapeutic strategies, we aimed to reduce CCI-related mortality and improve long-term prognoses for affected patients.
Keywords: Cardio-cerebral infarction1, acute myocardial infarction2, acute ischemic stroke3, narrative review4, Pathophysiology5, Treatment strategies6, prognosis7
Received: 08 Oct 2024; Accepted: 12 Mar 2025.
Copyright: © 2025 Gao, Yu, She, Sun, Jin, Fang, Chen and Zhu. This is an open-access article distributed under the terms of the Creative Commons Attribution License (CC BY). The use, distribution or reproduction in other forums is permitted, provided the original author(s) or licensor are credited and that the original publication in this journal is cited, in accordance with accepted academic practice. No use, distribution or reproduction is permitted which does not comply with these terms.
* Correspondence:
Xingyu Chen, Zhongshan Hospital, Xiamen University, Xiamen, China
Renjing Zhu, Zhongshan Hospital, Xiamen University, Xiamen, China
Disclaimer: All claims expressed in this article are solely those of the authors and do not necessarily represent those of their affiliated organizations, or those of the publisher, the editors and the reviewers. Any product that may be evaluated in this article or claim that may be made by its manufacturer is not guaranteed or endorsed by the publisher.
Research integrity at Frontiers
Learn more about the work of our research integrity team to safeguard the quality of each article we publish.