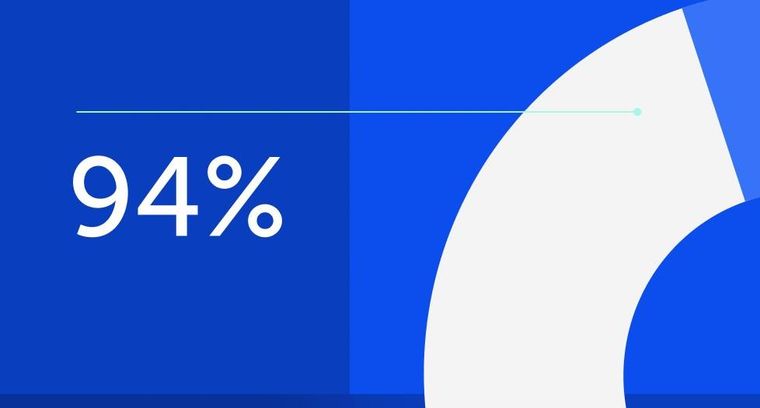
94% of researchers rate our articles as excellent or good
Learn more about the work of our research integrity team to safeguard the quality of each article we publish.
Find out more
REVIEW article
Front. Cardiovasc. Med., 20 March 2025
Sec. Clinical and Translational Cardiovascular Medicine
Volume 12 - 2025 | https://doi.org/10.3389/fcvm.2025.1482918
SGLT-2 inhibitors, initially developed for type 2 diabetes, demonstrate profound cardiorenal and metabolic benefits. This review synthesizes evidence from clinical trials and mechanistic studies to elucidate their roles in cardiovascular diseases, chronic kidney disease, and non-alcoholic fatty liver disease. Key findings include a notable reduction in cardiovascular death/heart failure hospitalization, a marked decrease in heart failure hospitalization risk, and significant improvements in renal and hepatic outcomes. Emerging mechanisms, such as autophagy induction, ketone utilization, and anti-inflammatory effects, underpin these benefits. Ongoing trials explore their potential in non-diabetic populations, positioning SGLT-2 inhibitors as transformative agents in multisystem disease management.
Sodium-glucose cotransporter 2 inhibitors (SGLT-2i), initially developed for managing type 2 diabetes mellitus (T2DM), have garnered significant attention for their multifaceted therapeutic benefits extending beyond glycemic control (1). These inhibitors, including canagliflozin, dapagliflozin, and empagliflozin, function by inhibiting the SGLT-2 protein in the proximal tubules of the kidney, thereby promoting glucose excretion through urine. This mechanism not only lowers blood glucose levels but also imparts several cardiovascular, renal, and metabolic benefits (2).
The journey of SGLT-2i dates back to the late 19th century with the discovery of phlorizin, a natural compound with glucose-lowering properties (3). Despite its initial promise, phlorizin's non-selective nature and gastrointestinal side effects hindered its clinical application (4). Advances in medicinal chemistry in the late 20th and early 21st centuries led to the development of selective SGLT-2i, revolutionizing the treatment landscape for T2DM and paving the way for their expanded use in cardiovascular and renal disease management (5, 6). Beyond T2DM, the current clinical indications for SGLT-2i mainly include heart failure (HF) and chronic kidney disease (CKD).
This review innovatively summarizes the potential clinical applications and mechanisms of SGLT-2i beyond T2DM, including HF, and CKD, myocardial infarction (MI), hypertension, arrhythmias, and non-alcoholic fatty liver disease (NAFLD). By examining the clinical evidence and exploring these mechanisms, we seek to highlight the transformative potential of SGLT-2i in managing these diseases and improving patient outcomes.
SGLT-2 protein is expressed in the proximal tubules of the kidney, where it is responsible for reabsorbing approximately 90% of filtered glucose. In patients with T2DM, SGLT-2 expression may be upregulated, exacerbating hyperglycemia (7). SGLT-2i are a class of medications initially developed for the treatment of T2DM (8). These drugs function by inhibiting the SGLT-2 protein in the proximal tubules of the kidney, thereby reducing the reabsorption of glucose and sodium (9). This action promotes the excretion of glucose through urine, leading to lower blood glucose levels (10).
The origins of SGLT-2i date back to the 1980s, with the study of the natural compound phlorizin, which inhibits both SGLT1 and SGLT2. However, due to its non-selective inhibition, phlorizin exhibited gastrointestinal instability and undesirable side effects, limiting its therapeutic potential. In the 1990s, as the understanding of glucose transport in the kidneys deepened, SGLT2 was identified as the key protein responsible for reabsorbing most of the glucose in the renal proximal tubules. This discovery laid the foundation for the development of selective SGLT-2i (11).
In the early 2000s, pharmaceutical chemists began creating selective SGLT-2i with the goal of reducing side effects and enhancing efficacy. This effort led to the development of drugs such as dapagliflozin, empagliflozin, and canagliflozin. Dapagliflozin became the first SGLT-2i to receive approval, launching in Europe in 2012 and subsequently being approved by the Food and Drug Administration in 2013 for the treatment of T2DM (12). Empagliflozin and canagliflozin followed, and clinical trials demonstrated their effectiveness not only in lowering blood glucose but also in promoting weight loss and reducing blood pressure (BP) (8).
Further clinical studies in the late 2010s revealed that SGLT-2i offered significant cardiovascular and renal protective effects, particularly in diabetic patients with existing cardiovascular disease or at high risk. This led to the expansion of their indications to include HF and CKD (13). With a growing understanding of their mechanisms, SGLT-2i are now being explored for use in non-diabetic patients, especially in the areas of cardiovascular and renal diseases. Additionally, new SGLT-2i are being developed to further improve efficacy and reduce side effects. Figure 1 presents a timeline of the development of SGLT-2i and key milestones.
Figure 1. Timeline of SGLT-2i development and key milestones. The development of SGLT-2i began in the 1980s with phlorizin, a compound that inhibited both SGLT-1 and SGLT-2 but had unsuitable gastrointestinal side effects. The 1990s discovery of the SGLT-2 protein, key to glucose reabsorption in the kidney, led to selective inhibitors like dapagliflozin and canagliflozin, approved in the early 2010s. Clinical studies later showed these drugs not only lower blood glucose but also offer cardiovascular and renal protection, extending their use to HF and CKD.
SGLT-2i have significantly impacted the landscape of CVDs management, extending their benefits far beyond glycemic control in diabetes. These inhibitors, including empagliflozin, dapagliflozin, canagliflozin, and sotagliflozin, have demonstrated profound cardioprotective effects, notably in HF management. Clinical trials have consistently shown that SGLT-2i reduce HF-related morbidity and mortality, improve cardiac outcomes, and enhance the quality of life for patients with HF (14). Moreover, these drugs also offer benefits in the context of MI, hypertension, and arrhythmias, highlighting their multifaceted role in CVDs treatment.
SGLT-2i offer cardiovascular benefits through a range of mechanisms, despite SGLT-2 not being significantly expressed in cardiomyocytes. They indirectly modulate cardiac function via systemic effects, such as improved myocardial energetics through ketone utilization, reduced oxidative stress, and inhibition of the Na+/H+ exchanger 1, which mitigates intracellular Na+ overload, calcium dysregulation, and calcineurin-driven cell death (15). SGLT-2i induce glycosuria, lowering the portal insulin-to-glucagon ratio and mimicking prolonged fasting, which stimulates lipolysis, hepatic ketogenesis, and increased circulating ketones (16). This shift in myocardial metabolism from glucose to more energy-efficient substrates, like ketone bodies, fatty acids, and branched-chain amino acids, is supported by altered enzyme activity favoring fatty acids/ketone/branched-chain amino acids oxidation while reducing glucose utilization (17). Additionally, SGLT-2i attenuate cardiac fibrosis by suppressing oxidative stress, collagen synthesis, and fibroblast activation (18). They also activate AMPK to enhance bioenergetics, promote macrophage polarization toward anti-inflammatory M2 phenotypes, and reduce CaMKII-mediated sarcoplasmic reticulum Ca2+ leaks (19). These effects are independent of glycemic control, as cardiac SGLT2 receptors are absent, highlighting the pleiotropic benefits of SGLT-2i through metabolic reprogramming, anti-fibrotic actions, and improved cardiomyocyte Ca2+/Na+ homeostasis. Furthermore, SGLT-2i promote a fasting-like response by stimulating autophagy, reducing visceral and epicardial fat, lowering BP, and aiding in HF management through osmotic diuresis and natriuresis (20). They also stimulate erythropoietin production to enhance hematocrit and oxygen delivery, improve cardiac energetics by increasing ketone bodies, inhibit the sympathetic nervous system, modulate cardiac inflammation and fibrosis, and promote reverse cardiac remodeling, all contributing to improved heart health (2). Figure 2 illustrates the mechanisms through which SGLT-2i provide cardiovascular benefits.
Figure 2. Cardiovascular benefits of SGLT-2i. SGLT-2i offer cardiovascular benefits through various mechanisms. They induce autophagy, reduce oxidative stress and inflammation, and decrease visceral and epicardial fat. They also promote osmotic diuresis and natriuresis, reducing BP and sodium levels, aiding HF management. Additionally, SGLT-2i enhance erythropoietin production, improving hematocrit and oxygen delivery, and increase ketone bodies for better cardiac energetics. They inhibit the sympathetic nervous system, modulate cardiac inflammation and fibrosis, promote reverse cardiac remodeling, and improve vascular function, collectively enhancing heart health.
Given these wide-ranging benefits, SGLT-2i have become a cornerstone in the therapeutic arsenal for managing various cardiovascular conditions. Future research is poised to explore additional indications and long-term benefits of these inhibitors, potentially broadening their application in CVD management.
HF is the terminal manifestation of almost all CVDs. HF is a complex clinical syndrome characterized by any structural or functional impairment of ventricular filling or blood ejection (21). Ischemic heart disease, the leading cause of death worldwide, is also the primary cause of HF (22). HF is a common global disease with high morbidity and mortality rates (23). It is estimated that 26 million people worldwide suffer from HF, which increases healthcare costs, reduces functional capacity, and severely impacts quality of life (24). HF is characterized by impaired myocardial function leading to inadequate blood supply to the body. Patients typically present with fatigue, dyspnea, reduced exercise tolerance, and congestion in the systemic or pulmonary circulation. General treatment aims to relieve systemic and pulmonary congestion and stabilize hemodynamic status (25).
The cardioprotective effects of SGLT-2i in HF are multifaceted. These drugs promote glucosuria, leading to reduced blood glucose levels and consequently lower insulin resistance and weight reduction, which alleviates the cardiac workload (26). SGLT-2i also enhance natriuresis and diuresis, which help to reduce BP and decrease the volume overload on the heart (27). Additionally, these inhibitors improve myocardial energy metabolism by increasing ketone body utilization and reducing myocardial inflammation and oxidative stress, thereby preserving cardiac function and structure (28). The modulation of neurohormonal pathways, including the reduction of sympathetic nervous system activity and improvement in renal function, further contributes to their therapeutic benefits in HF (29).
SGLT-2i have demonstrated significant benefits in the management of HF, addressing various patient populations with differing HF characteristics. The effects and mechanisms of SGLT-2i vary depending on the ejection fraction (EF) of HF patients, leading to differential therapeutic outcomes across HF subtypes. In HF with reduced EF (HFrEF), dapagliflozin improved Kansas City Cardiomyopathy Questionnaire (KCCQ) scores and significantly reduced the risk of worsening HF or cardiovascular death, while empagliflozin also lowered the risk of cardiovascular death or HF hospitalization but did not improve cardiac energetics or circulating serum metabolites related to energy metabolism (30–32). In HFrEF patients without diabetes, empagliflozin improved adiposity, interstitial myocardial fibrosis, aortic stiffness, and inflammatory markers (33). For HF with preserved EF (HFpEF), dapagliflozin did not enhance KCCQ scores or six-minute walking distance but significantly improved patient-reported symptoms, physical limitations, and exercise function, while also reducing resting and exercise pulmonary capillary wedge pressure, plasma volume, and body weight. In HFpEF or HF with mid-range EF (HFmrEF), both dapagliflozin and empagliflozin reduced the combined risk of worsening HF or cardiovascular death (34, 35).
The therapeutic effects of SGLT-2i also vary depending on the progression of HF. In patients with acute HF, dapagliflozin initiation has been shown to be safe and fulfills a component of guideline-directed medical therapy optimization (36). Empagliflozin, during acute decompensated HF, resulted in higher circulating ketone body concentrations, particularly acetone, compared to stabilized phases (37). It also produced clinical benefits and was well tolerated regardless of background mineralocorticoid receptor antagonist use (38). For patients with chronic HF and T2DM, empagliflozin increased 24 h urine volume without an increase in urinary sodium when combined with loop diuretics, highlighting its renal and cardiovascular effects (39). More broadly, SGLT-2i significantly reduced the risk of cardiovascular death or hospitalization for HF by 23%, and hospitalization for HF by 31% (40). Table 1 reviews clinical trials evaluating the effects of SGLT-2i in HF.
In summary, SGLT-2i have shown diverse and significant clinical benefits in various HF scenarios. These benefits span improvements in symptoms, reduction in cardiovascular events, and optimization of HF management strategies, making them a crucial component in contemporary HF treatment regimens.
MI, commonly known as a “heart attack”, is caused by the partial or complete cessation of blood flow to a part of the heart muscle (41). MI can range from being asymptomatic to causing catastrophic events like hemodynamic deterioration and sudden death. Most MIs are triggered by underlying coronary artery disease, where the occlusion of a coronary artery leads to myocardial hypoxia. Prolonged oxygen deprivation results in the death and necrosis of myocardial cells. Patients may experience chest discomfort or pressure that can radiate to the neck, jaw, shoulders, or arms (42). In addition to the patient's medical history and physical examination, myocardial ischemia is evidenced by changes on an electrocardiogram and elevated biochemical markers such as cardiac troponins (43).
SGLT-2i offer cardioprotective effects against MI through multiple mechanisms. These include the suppression of autophagic cell death by inhibiting the Na+/H+ exchanger 1, reducing infarct size and myocardial fibrosis (44). Furthermore, these inhibitors help normalize mitochondrial size and number, and prevent excessive reduction in mitochondrial size after MI, leading to improved structural and functional heart outcomes post-MI (45). In addition, they have been shown to attenuate cardiac fibrosis by regulating macrophage polarization through a STAT3-dependent pathway, which helps in reducing adverse ventricular remodeling post-MI (46). They also exhibit significant anti-inflammatory effects, lowering levels of inflammatory markers and contributing to smaller infarct sizes (47). Additionally, SGLT-2i reduce the risk of new-onset cardiac arrhythmias, such as atrial fibrillation (AF) and ventricular arrhythmias, during hospitalization for acute MI (48).
SGLT-2i have shown considerable promise in the context of MI, addressing various patient populations and clinical scenarios. In patients with acute MI, the EMMY trial demonstrated that empagliflozin was associated with a significantly greater N-terminal pro-hormone of brain natriuretic peptide (NT-proBNP) reduction over 26 weeks, alongside notable improvements in echocardiographic functional and structural parameters (49). For patients without diabetes or established HF presenting with MI and impaired left ventricular (LV) systolic function or Q-wave MI, the DAPA-MI trial explores the potential of dapagliflozin to further improve outcomes in these patients (50). Similarly, dapagliflozin showed significant benefits in cardiometabolic outcomes in patients with acute MI without prior diabetes or chronic HF. In patients with T2DM and a history of MI, dapagliflozin robustly reduced the risk of major adverse cardiovascular events (MACE) and cardiovascular death or hospitalization for HF (51). Additionally, in patients with acute MI and newly reduced LVEF below 45% or congestion, empagliflozin reduced first and total HF hospitalizations across the range of LVEF with or without congestion (52).
Empagliflozin also showed promise in reducing the risk of first and recurrent HF events in patients with acute MI at risk for HF due to newly developed LVEF below 45% or signs of congestion, as seen in the EMPACT-MI trial (53). Early administration of SGLT-2i in acute MI patients with T2DM, as investigated in the EMBODY trial, might effectively improve cardiac nerve activity without adverse events (AEs) (54). For non-diabetic patients with anterior ST-elevation MI and LVEF below 50%, dapagliflozin appears to play a role in preventing LV dysfunction and maintaining cardiac function, as demonstrated in the DACAMI trial (55). Furthermore, in patients with T2DM and CVD, empagliflozin reduced the risk of total MI events by 21%, driven by effects on both type 1 and type 2 MIs, according to insights from the EMPA-REG OUTCOME trial (56). Table 2 presents clinical trials investigating the use of SGLT-2i in MI.
In brief, SGLT-2i like empagliflozin and dapagliflozin have shown diverse benefits in managing MI, particularly in improving cardiac function, reducing cardiovascular events, and optimizing HF outcomes in various patient populations.
Hypertension, defined as BP consistently at or above 140/90 mmHg, affects an estimated 1.28 billion adults worldwide, with the two-thirds residing in low- and middle-income countries (57). It is one of the most significant risk factors for ischemic heart disease, stroke, other CVDs, CKD, and dementia. Key risk factors for hypertension include advanced age, genetics, obesity, physical inactivity, high-salt diet, and excessive alcohol consumption (58). While most individuals with hypertension exhibit no symptoms, severely elevated BP can cause headaches, blurred vision, and chest pain. Hypertension remains the leading preventable cause of CVD-related deaths and disease burden globally and in most regions of the world (59).
The antihypertensive effects of SGLT-2i are mediated through several mechanisms. These drugs induce osmotic diuresis and natriuresis, leading to reduced blood volume and decreased preload on the heart (60). By inhibiting sodium reabsorption in the proximal tubules, SGLT-2i promote sodium excretion, which helps lower BP (61). Additionally, these inhibitors improve endothelial function and reduce arterial stiffness, which are critical factors in the pathogenesis of hypertension (62). Furthermore, they inhibit the renin-angiotensin-aldosterone system (RAAS) and decrease oxidative stress and inflammation in renal tissues, mitigating hypertension and protecting against renal and cardiovascular damage (61, 63).
SGLT-2i have demonstrated considerable efficacy in managing hypertension, especially in patients with T2DM. Clinical trials underscore their significant impact on BP and broader cardiovascular outcomes. For instance, empagliflozin has yielded substantial and clinically meaningful reductions in both BP and HbA1c levels among T2DM patients with hypertension, while maintaining a good tolerance profile (64). This highlights its dual benefit in simultaneously controlling glucose levels and BP. Over periods ranging from 12 to 24 weeks, empagliflozin consistently decreased glycohemoglobin, body weight, and BP, marking its prolonged efficacy in this patient group (65). Additionally, it has significantly lowered mean 24 h systolic BP (SBP) in both dippers and non-dippers, demonstrating versatility across different BP profiles (66). Empagliflozin also benefits T2DM patients on various antihypertensive treatments, including those on multiple drugs, diuretics, or ACE inhibitors/ARBs, effectively reducing both SBP and diastolic BP (DBP) (67). Notably, in elderly patients with T2DM and hypertension, it has notably decreased BP, improved endothelial function, and reduced arterial stiffness, suggesting additional cardiovascular protective effects (68). For those receiving renin-angiotensin blockers, dapagliflozin has significantly reduced albuminuria, a critical marker of kidney damage, with these benefits persisting even after adjusting for changes in HbA1c, BP, body weight, and estimated glomerular filtration rate (eGFR), underlining its renal protective properties in hypertensive diabetic patients (69). Table 3 covers clinical trials examining the role of SGLT-2i in hypertension.
To summarize, SGLT-2i such as empagliflozin and dapagliflozin provide significant benefits in managing hypertension in patients with T2DM. These benefits extend beyond BP control to include improvements in glycemic control, cardiovascular health, and renal protection, making them a valuable addition to the therapeutic arsenal for these patients.
Arrhythmia, also known as an irregular heartbeat, is a condition where the heart beats too fast, too slow, or with an irregular rhythm. Arrhythmias can originate from different parts of the heart, disrupting the normal organized and coordinated heartbeat (70). Frequent arrhythmias may indicate that the heart cannot pump enough blood to the body, which is crucial as the heart supplies nutrients and oxygen through the blood. This can lead to symptoms such as dizziness, fainting, or other signs of inadequate blood flow (71). Arrhythmias can be treated with medications or surgical procedures to control the irregular rhythm. AF is the most common type of treated heart arrhythmia. Without treatment, arrhythmias can damage the heart, brain, or other organs, potentially leading to life-threatening conditions like stroke, HF, or sudden cardiac arrest (72).
The anti-arrhythmic effects of SGLT-2i are mediated through various mechanisms. Primarily, these drugs reduce intracellular sodium and calcium overload, which helps stabilize cardiac myocytes and prevent arrhythmogenic events (73). SGLT-2i also promote glucosuria and natriuresis, leading to lower BP and reduced myocardial stress (74). Furthermore, these inhibitors improve myocardial energy metabolism, enhance mitochondrial function, and reduce oxidative stress and inflammation, all of which contribute to their anti-arrhythmic benefits (75). Additionally, SGLT-2i suppress sympathetic overactivity and RAAS activation, which further aids in preventing arrhythmias (76).
SGLT-2i have demonstrated significant potential in managing arrhythmias, particularly in patients with HF and T2DM. In patients with T2DM and arrhythmias, SGLT-2i have shown promising results. For example, in patients with T2DM and established CVD, empagliflozin significantly reduced cardiovascular death, HF hospitalizations, and renal events. The absolute reduction in these outcomes was greater in those with AF (77). For patients with HF, SGLT-2i have shown significant benefits in reducing arrhythmic events and improving outcomes. In HFrEF, dapagliflozin significantly reduced the risk of serious ventricular arrhythmias, cardiac arrest, or sudden death when added to conventional therapy (78). Dapagliflozin demonstrated consistent benefits across HFpEF and HFrEF, significantly reducing the risk of cardiovascular death or worsening HF, especially in patients with paroxysmal AF (79).
In addition, in patients treated with implantable cardioverter-defibrillator or cardiac resynchronization therapy-defibrillator, empagliflozin reduced the number of ventricular arrhythmias compared with placebo, indicating its efficacy in managing arrhythmic events in T2DM patients with implanted devices (80). Additionally, in T2DM patients with CKD, canagliflozin showed benefits in preventing hemorrhagic stroke and atrial fibrillation/flutter (AF/AFL), with significant protection observed in those with the lowest baseline eGFR (81). Table 4 summarizes clinical trials focused on SGLT-2i in arrhythmia.
In summary, SGLT-2i like ertugliflozin, dapagliflozin, and empagliflozin provide significant benefits in managing arrhythmias and improving cardiovascular outcomes in various patient populations, particularly those with HF and diabetes.
CKD, also known as chronic renal failure, involves the gradual loss of kidney function. The kidneys filter waste and excess fluids from the blood, which are then excreted in the urine. In the early stages of CKD, there are usually few signs or symptoms. However, advanced CKD can lead to dangerous levels of fluid, electrolytes, and waste buildup in the body (82). Treatment for CKD focuses on slowing the progression of kidney damage, typically through managing the underlying cause (83). However, even with control of the underlying cause, kidney damage may continue to progress. CKD can eventually progress to end-stage renal disease, which can be life-threatening without dialysis or a kidney transplant (84).
SGLT2, located in the proximal convoluted tubule (S1 segment) of the nephron, is responsible for reabsorbing approximately 90% of the filtered glucose from the urine back into the bloodstream. It functions by coupling sodium (Na+) transport with glucose, utilizing the sodium gradient created by the Na+/K+ ATPase pump on the basolateral membrane, with glucose exiting the tubular cells into the bloodstream via GLUT2 transporters (85). SGLT-2i exert kidney-protective effects through multifaceted mechanisms that go beyond tubuloglomerular feedback-mediated reduction of intraglomerular pressure and hyperfiltration, a process pivotal in diabetic conditions through increased distal solute delivery to the macula densa, afferent arteriolar vasoconstriction, and efferent vasodilation. However, tubuloglomerular feedback's contribution varies with factors like salt intake, dietary protein, and underlying disease (e.g., weaker in non-diabetic CKD) (86). Additional mechanisms include optimization of energy metabolism, where glycosuria-induced caloric deficit promotes lipolysis, ketogenesis (β-hydroxybutyrate), and reduced proximal tubular oxygen consumption, mitigating hypoxia (87). SGLT-2i also enhance autophagy and cellular homeostasis by inhibiting mTORC1, activating AMPK/SIRT1 pathways, and balancing HIF-1α/HIF-2α signaling, reducing oxidative stress, inflammation, and fibrosis (88). They attenuate sympathetic hyperactivity via natriuresis, reduced Na+/H+ exchanger activity, and suppression of neurohormonal pathways, thereby lowering BP and cardiac strain (89). Furthermore, SGLT-2i improve vascular health by upregulating endothelial nitric oxide, reducing arterial stiffness, and mobilizing pro-vascular progenitor cells (90). These pleiotropic effects—alongside glycemic control, BP reduction, weight loss, and improved lipid profiles—contribute to a significant reduction in CKD progression, decrease albuminuria, and offer anti-inflammatory and antifibrotic benefits, highlighting SGLT-2 inhibitors' role beyond glucose-lowering. Figure 3 details how SGLT-2i help reduce the risk of CKD.
Figure 3. Mechanistic overview of SGLT-2i in reducing the risk of CKD. Key pathways by which SGLT-2i reduce the risk of CKD include enhanced natriuresis and improved tissue sodium handling that lower intraglomerular pressure and mitigate hyperfiltration; decreased cardiac preload and BP; and metabolic benefits such as increased ketogenesis and fatty acid oxidation. Additionally, these inhibitors reduce oxidative stress and inflammatory mediators, contributing to the prevention of kidney tissue damage and CKD progression.
SGLT-2i have shown significant promise in managing CKD, particularly in patients with T2DM and those at high cardiovascular risk. Various clinical trials have demonstrated the renal and cardiovascular benefits of these inhibitors across different patient populations. Dapagliflozin therapy in patients with CKD, irrespective of T2DM status, led to a lower risk of kidney disease progression or death from cardiovascular causes (91). For patients with T2DM and chronic HF taking regular loop diuretics, empagliflozin caused a significant increase in 24 h urine volume without increasing urinary sodium. This combination therapy showed positive renal and cardiovascular effects, emphasizing the benefit of SGLT2 inhibition in conjunction with loop diuretics (39). Ertugliflozin was effective in reducing HbA1c, body weight, and SBP while maintaining eGFR in patients with T2DM and stage 3 CKD, proving to be well tolerated even in moderate-to-severe CKD (92). In patients without diabetes and with CKD, dapagliflozin exhibited a plasma concentration-time profile similar to that in diabetic kidney disease, with changes in risk markers for kidney disease, indicating its potential utility across different types of CKD (93).
Dapagliflozin has significantly reduced the risk of a composite of sustained decline in eGFRF, end-stage kidney disease, or death from renal or cardiovascular causes in patients with CKD (91). The DELIVER trial showed that dapagliflozin slowed the rate of decline in eGFR in patients with HFrEF or HFpEF (94). In a unique trial combining dapagliflozin with eplerenone, a robust additive HbA1c and urine albumin-creatinine ratio (UACR)-lowering effect was observed, suggesting potential synergistic benefits in CKD management (95). These findings highlight its role in mitigating disease progression and reducing severe renal and cardiovascular events. Canagliflozin has demonstrated a significant reduction in the risk of kidney failure and cardiovascular events in patients with T2DM and albuminuric CKD, making it an effective therapeutic option for protecting kidney function and cardiovascular health (96). Sotagliflozin, used in patients with diabetes and CKD, resulted in a lower risk of deaths from cardiovascular causes, hospitalizations for HF, and urgent HF visits, although it was associated with AEs (97). It also showed efficacy in reducing urine albumin-creatinine ratio (UACR) in patients with stage 3 and stage 4 CKD (98, 99). Table 5 highlights clinical trials assessing the impact of SGLT-2i in CKD.
Collectively, SGLT-2i like empagliflozin, dapagliflozin, canagliflozin, and sotagliflozin offer significant benefits in managing CKD, from improving renal outcomes to reducing cardiovascular risks. Their application extends across various CKD patient profiles, highlighting their versatility and effectiveness in CKD management.
NAFLD is a condition characterized by the accumulation of fat in the liver. It is most commonly found in individuals who are overweight or obese. With the rising prevalence of obesity, NAFLD is becoming increasingly common, especially in the Middle East and Western countries. It is now the most prevalent form of liver disease globally (100). The severity of NAFLD varies, ranging from steatosis to a more severe condition called non-alcoholic steatohepatitis (NASH). NASH causes liver inflammation and damage due to fat buildup in the liver. It can progress, leading to severe cirrhosis and even liver cancer. This damage is similar to that caused by heavy alcohol consumption (101).
Hepatic SGLT-2 expression is minimal, but SGLT-2i ameliorate NAFLD and NASH through indirect mechanisms, including reduced hepatic gluconeogenesis, improved insulin sensitivity, and modulation of adipokine signaling, such as increased adiponectin. These drugs primarily exert their effects in liver disease by improving glucose and lipid metabolism, reducing hepatic fat accumulation, and modulating inflammatory pathways (102). By inhibiting SGLT2 in the kidneys, SGLT-2i promote glucosuria, lowering blood glucose levels and insulin resistance, which can mitigate NAFLD. Additionally, SGLT-2i enhance ketogenesis, shifting the liver's energy metabolism from glucose to ketone bodies, potentially reducing hepatic steatosis. They also exert anti-inflammatory and antifibrotic effects by lowering pro-inflammatory cytokines and reducing oxidative stress, which further benefits liver function in chronic liver diseases. Furthermore, these inhibitors improve adiponectin levels, reduce inflammation, and enhance insulin sensitivity, all contributing to hepatoprotection (103). Overall, SGLT-2 inhibitors improve both metabolic and inflammatory parameters in the liver, offering potential therapeutic benefits for conditions such as NAFLD and NASH. Figure 4 describes the mechanisms by which SGLT-2i affect NAFLD.
Figure 4. SGLT-2i improve insulin sensitivity by reducing blood glucose levels and promoting a lower insulin demand in the body. This improvement helps decrease hepatic glucose production and reduces the deposition of triglycerides in the liver. These drugs reduce the reabsorption of filtered glucose in the kidneys, leading to increased urinary glucose excretion and a consequent caloric loss, which promotes a reduction in the liver fat content. This reduction in hepatic steatosis is vital for improving liver function and reversing NAFLD. By reducing liver fat content, SGLT-2i also help lower the hepatic inflammation and fibrosis that characterize NASH, an advanced form of fatty liver disease.
SGLT-2i have shown significant potential in managing NAFLD. Various clinical trials have demonstrated the hepatic and metabolic benefits of these inhibitors across different patient populations. Empagliflozin and dapagliflozin have both shown efficacy in reducing liver fat and improving liver function. For instance, a trial involving dapagliflozin demonstrated improvements in liver dysfunction associated with a decrease in serum soluble dipeptidyl peptidase-4 (sDPP-4), suggesting a therapeutic strategy independent of glucose lowering or weight loss (104). Moreover, another study found dapagliflozin more beneficial than pioglitazone in patients with NAFLD, with improvements in fatty liver index closely related to glycemic control (105). Similarly, tofogliflozin was effective in reducing hepatic steatosis compared to pioglitazone, as indicated by lower MRI-proton density fat fraction (MRI-PDFF) levels (106). Additionally, lower serum high-molecular-weight (HMW) adiponectin was associated with a better response to dapagliflozin, indicating a potential biomarker for treatment efficacy (107). Furthermore, a study combining dapagliflozin and OM-3CA significantly reduced liver fat content, with dapagliflozin monotherapy reducing hepatocyte injury biomarkers and FGF21 (108). Canagliflozin also reduced body mass, fat mass, and hepatic fat content without significantly reducing muscle mass, demonstrating its effectiveness in altering body composition favorably (109).
In patients with T2DM and NAFLD, empagliflozin significantly improved hepatic steatosis compared with sitagliptin, potentially protecting against hepatic insulin resistance (110). Similarly, dapagliflozin improved liver steatosis and attenuated liver fibrosis in patients with significant liver fibrosis, highlighting its potential for treating liver conditions associated with NAFLD (111). Dapagliflozin also decreased liver fat content and pancreatic fat content while improving serum ALT, TNF-α, and IL-6 levels, indicating broad metabolic benefits (112). Furthermore, in non-insulin-dependent T2DM patients with NAFLD, dapagliflozin significantly decreased intrahepatic lipid content, body weight, body fat, and visceral fat/subcutaneous fat ratio, along with improvements in HbA1c and ALT levels (113). Additionally, in patients with NAFLD without diabetes, empagliflozin improved liver steatosis and measures of liver fibrosis, suggesting its applicability in non-diabetic populations as well (114). Table 6 discusses clinical trials exploring the effects of SGLT-2i on NAFLD.
In conclusion, SGLT-2i like empagliflozin, dapagliflozin, tofogliflozin, and canagliflozin provide significant benefits in managing NAFLD by improving liver steatosis, reducing liver fibrosis, and enhancing metabolic health, particularly in patients with T2DM. These findings highlight their potential as effective therapeutic options for NAFLD management.
Most trials show consistent benefits. For example, the DAPA-MI trial (NCT04564742) investigates dapagliflozin in post-MI patients without diabetes, while EMBODY (NCT04193557) explores empagliflozin's effects on cardiac nerve activity. Upcoming trials like ZENITH-CKD (NCT04724837) evaluate combination therapies (SGLT-2i + endothelin antagonists) for advanced CKD. However, some studies report neutral effects on arrhythmias or variable efficacy in non-diabetic NAFLD. For instance, dapagliflozin improved liver steatosis in diabetic patients but showed modest effects in non-diabetic cohorts (115). Despite robust cardiorenal benefits, SGLT-2i are not universally effective. Genitourinary infections, volume depletion risks, and incomplete responses in high-risk subgroups (e.g., advanced CKD) necessitate personalized approaches. Therefore, further research is needed to overcome the therapeutic limitations of SGLT-2i.
Firstly, while the cardiovascular and renal benefits of these inhibitors are well-documented, their long-term safety and efficacy across diverse patient populations require continued scrutiny. Clinical trials have predominantly focused on patients with T2DM, CVD, CKD, and NAFLD necessitating studies in broader cohorts to validate their utility in other conditions.
Additionally, the mechanisms underpinning the multifaceted effects of SGLT-2i are complex and not fully understood. While current evidence suggests roles in modulating glucose metabolism, reducing inflammation, and improving cardiovascular and renal function, further research is needed to elucidate these pathways and identify potential biomarkers for patient stratification and treatment optimization.
Another limitation is the potential for adverse effects, including genitourinary infections and dehydration, particularly in elderly patients or those with compromised renal function (116). To mitigate these risks, careful patient selection and individualized treatment strategies are essential. Baseline renal function, fluid status, and infection history should be evaluated before initiating therapy. Patient education on maintaining proper hydration, recognizing early signs of dehydration or infection, and practicing good hygiene can further minimize complications. Close monitoring of renal function, electrolyte balance, and hemodynamic status, particularly in those on concomitant diuretics or RAAS inhibitors, is recommended to prevent excessive volume depletion.
While SGLT-2i provide significant cardiovascular and renal protection, these benefits may not be sufficient for all patients, especially those with high cardiovascular risk profiles. In such patients, the protective effects of SGLT-2i alone might not fully address the complex cardiovascular challenges they face. Therefore, combining SGLT-2i with other guideline-directed medical therapies (GDMT) is recommended. GDMT typically includes medications such as ACE inhibitors, angiotensin II receptor blockers, beta-blockers, and mineralocorticoid receptor antagonists, which have been proven to improve HF outcomes and reduce cardiovascular events. This combination approach, by targeting multiple disease pathways, can offer more robust protection against heart attacks, strokes, and other complications, thereby optimizing cardiovascular outcomes. Thus, while SGLT-2i are an essential part of managing HF and CKD, their limitations highlight the need for their use within the broader context of GDMT.
SGLT-2i do not elicit a complete response in all patients, and the therapeutic efficacy can vary significantly between individuals. This variability underscores the need for more personalized approaches to treatment. The integration of precision medicine strategies, such as genetic and biomarker profiling, holds promise for tailoring SGLT-2i therapy to the specific needs of individual patients. By identifying genetic factors and biomarkers that predict response, healthcare providers can better optimize treatment plans, potentially improving therapeutic outcomes and minimizing the risks of adverse effects. This personalized approach could enhance the overall effectiveness of SGLT-2i across diverse patient populations. Figure 5 outlines the limitations and future perspectives of SGLT-2i.
Figure 5. Limitations and future perspectives of SGLT-2i. The key challenges and future directions in the clinical application of SGLT2 inhibitors includes long-term safety and efficacy across diverse patient populations, further research for potential pathways and biomarkers, variable efficacy and incomplete responses, combination with cardioprotective and renoprotective agents, adverse effects and careful monitoring.
In the future, the clinical significance of SGLT-2 inhibitors is expected to expand, particularly in the management of cardiovascular, renal, and metabolic diseases such as NAFLD. As ongoing trials explore their potential in non-diabetic populations, SGLT-2 inhibitors may become a key treatment option for multi-system diseases. Additionally, the development of personalized medicine, through genetic profiling and biomarker identification, will likely enhance their efficacy across diverse patient groups. Long-term studies will further evaluate their enduring benefits in reducing cardiovascular and renal events, solidifying their role in improving patient outcomes and quality of life.
SGLT-2i have revolutionized the management of not only T2DM but also HF and CKD, among other conditions. This review underscores their broad therapeutic potential, highlighting their beneficial effects in MI, hypertension, arrhythmias, and NAFLD. The multifaceted mechanisms of SGLT-2i, including glucose excretion, reduction of oxidative stress and inflammation, improved myocardial and renal function, and modulation of neurohormonal pathways, contribute to their efficacy. As we continue to explore the full spectrum of SGLT-2i applications, these inhibitors hold promise for significantly improving patient outcomes across a range of cardiovascular, renal, and metabolic diseases.
QF: Conceptualization, Writing – original draft. MW: Investigation, Writing – original draft. ZM: Supervision, Validation, Writing – review & editing.
The author(s) declare that no financial support was received for the research and/or publication of this article.
The authors declare that the research was conducted in the absence of any commercial or financial relationships that could be construed as a potential conflict of interest.
The author(s) declare that no Generative AI was used in the creation of this manuscript.
All claims expressed in this article are solely those of the authors and do not necessarily represent those of their affiliated organizations, or those of the publisher, the editors and the reviewers. Any product that may be evaluated in this article, or claim that may be made by its manufacturer, is not guaranteed or endorsed by the publisher.
AE, adverse event; AF, atrial fibrillation; AF/AFL, atrial fibrillation/flutter; BP, blood pressure; CKD, chronic kidney disease; CVD, cardiovascular disease; DBP, diastolic BP; EF, ejection fraction; eGFR, estimated glomerular filtration rate; HF, heart failure; HFmrEF, HF with mid-range EF; HFpEF, HF with preserved EF; HFrEF, HF with reduced EF; HMW, High-molecular-weight; KCCQ, Kansas City cardiomyopathy questionnaire; LV, left ventricular; MACE, major adverse cardiovascular event; MI, myocardial infarction; MRI-PDFF, MRI-proton density fat fraction; NAFLD, non-alcoholic fatty liver disease; NASH, non-alcoholic steatohepatitis; NT-proBNP, N-terminal pro-hormone of brain natriuretic peptide; RAAS, renin-angiotensin-aldosterone system; SBP, systolic BP; sDPP-4, soluble dipeptidyl peptidase-4; SGLT-2i, sodium-glucose cotransporter 2 inhibitors; T2DM, type 2 diabetes mellitus; UACR, urine albumin-creatinine ratio.
1. Baruah MP, Makkar BM, Ghatnatti V, Mandal K. Sodium glucose co-transporter-2 inhibitor: benefits beyond glycemic control. Indian J Endocrinol Metab. (2019) 23(1):140–9. doi: 10.4103/ijem.IJEM_160_17
2. Zelniker TA, Braunwald E. Mechanisms of cardiorenal effects of sodium-glucose cotransporter 2 inhibitors: JACC state-of-the-art review. J Am Coll Cardiol. (2020) 75(4):422–34. doi: 10.1016/j.jacc.2019.11.031
3. Choi CI. Sodium-Glucose cotransporter 2 (SGLT2) inhibitors from natural products: discovery of next-generation antihyperglycemic agents. Molecules. (2016) 21(9):1136. doi: 10.3390/molecules21091136
4. Feijoo-Bandin S, Aragón-Herrera A, Otero-Santiago M, Anido-Varela L, Moraña-Fernández S, Tarazón E, et al. Role of sodium-glucose co-transporter 2 inhibitors in the regulation of inflammatory processes in animal models. Int J Mol Sci. (2022) 23(10):5634. doi: 10.3390/ijms23105634
5. Udell JA, Jones WS, Petrie MC, Harrington J, Anker SD, Bhatt DL, et al. Sodium glucose cotransporter-2 inhibition for acute myocardial infarction: JACC review topic of the week. J Am Coll Cardiol. (2022) 79(20):2058–68. doi: 10.1016/j.jacc.2022.03.353
6. Heerspink HJL, Kosiborod M, Inzucchi SE, Cherney DZI. Renoprotective effects of sodium-glucose cotransporter-2 inhibitors. Kidney Int. (2018) 94(1):26–39. doi: 10.1016/j.kint.2017.12.027
7. Scheen AJ. Pharmacodynamics, efficacy and safety of sodium-glucose co-transporter type 2 (SGLT2) inhibitors for the treatment of type 2 diabetes mellitus. Drugs. (2015) 75(1):33–59. doi: 10.1007/s40265-014-0337-y
8. Hsia DS, Grove O, Cefalu WT. An update on sodium-glucose co-transporter-2 inhibitors for the treatment of diabetes mellitus. Curr Opin Endocrinol Diabetes Obes. (2017) 24(1):73–9. doi: 10.1097/MED.0000000000000311
9. Sen T, Heerspink HJL. A kidney perspective on the mechanism of action of sodium glucose co-transporter 2 inhibitors. Cell Metab. (2021) 33(4):732–9. doi: 10.1016/j.cmet.2021.02.016
10. List JF, Whaley JM. Glucose dynamics and mechanistic implications of SGLT2 inhibitors in animals and humans. Kidney Int Suppl. (2011) 120:S20–7. doi: 10.1038/ki.2010.512
11. Blaschek W. Natural products as lead compounds for sodium glucose cotransporter (SGLT) inhibitors. Planta Med. (2017) 83(12–13):985–93. doi: 10.1055/s-0043-106050
12. American Diabetes Association Professional Practice, C. 9. Pharmacologic approaches to glycemic treatment: standards of medical care in diabetes-2022. Diabetes Care. (2022) 45(1):S125–43. doi: 10.2337/dc22-S009
13. Mark PB, Sarafidis P, Ekart R, Ferro CJ, Balafa O, Fernandez-Fernandez B, et al. SGLT2i For evidence-based cardiorenal protection in diabetic and non-diabetic chronic kidney disease: a comprehensive review by EURECA-m and ERBP working groups of ERA. Nephrol Dial Transplant. (2023) 38(11):2444–55. doi: 10.1093/ndt/gfad112
14. Spertus JA, Birmingham MC, Nassif M, Damaraju CV, Abbate A, Butler J, et al. The SGLT2 inhibitor canagliflozin in heart failure: the CHIEF-HF remote, patient-centered randomized trial. Nat Med. (2022) 28(4):809–13. doi: 10.1038/s41591-022-01703-8
15. Dyck JRB, Sossalla S, Hamdani N, Coronel R, Weber NC, Light PE, et al. Cardiac mechanisms of the beneficial effects of SGLT2 inhibitors in heart failure: evidence for potential off-target effects. J Mol Cell Cardiol. (2022) 167:17–31. doi: 10.1016/j.yjmcc.2022.03.005
16. Bobrowski D, Kumar R, Wu PE, Lapointe-Shaw L. Prolonged ketosis and glycosuria secondary to SGLT2 inhibitor therapy. Clin Case Rep. (2021) 9(11):e05057. doi: 10.1002/ccr3.5057
17. Su S, Ji X, Li T, Teng Y, Wang B, Han X, et al. The changes of cardiac energy metabolism with sodium-glucose transporter 2 inhibitor therapy. Front Cardiovasc Med. (2023) 10:1291450. doi: 10.3389/fcvm.2023.1291450
18. Tian J, Zhang M, Suo M, Liu D, Wang X, Liu M, et al. Dapagliflozin alleviates cardiac fibrosis through suppressing EndMT and fibroblast activation via AMPKalpha/TGF-beta/Smad signalling in type 2 diabetic rats. J Cell Mol Med. (2021) 25(16):7642–59. doi: 10.1111/jcmm.16601
19. Garcia-Ropero A, Vargas-Delgado A, Santos-Gallego C, Badimon J. Inhibition of sodium glucose cotransporters improves cardiac performance. Int J Mol Sci. (2019) 20(13):3289. doi: 10.3390/ijms20133289
20. Lopaschuk GD, Verma S. Mechanisms of cardiovascular benefits of sodium glucose co-transporter 2 (SGLT2) inhibitors: a state-of-the-art review. JACC Basic Transl Sci. (2020) 5(6):632–44. doi: 10.1016/j.jacbts.2020.02.004
21. Kubota Y, Shimizu W. Clinical benefits of sodium-glucose cotransporter 2 inhibitors and the mechanisms underlying their cardiovascular effects. JACC Asia. (2022) 2(3):287–93. doi: 10.1016/j.jacasi.2022.03.009
22. Katz D, Gavin MC. Stable ischemic heart disease. Ann Intern Med. (2019) 171(3):ITC17–32. doi: 10.7326/AITC201908060
23. McMurray JJ, Pfeffer MA. Heart failure. Lancet. (2005) 365(9474):1877–89. doi: 10.1016/S0140-6736(05)66621-4
24. Savarese G, Lund LH. Global public health burden of heart failure. Card Fail Rev. (2017) 3(1):7–11. doi: 10.15420/cfr.2016:25:2
25. Borlaug BA. Evaluation and management of heart failure with preserved ejection fraction. Nat Rev Cardiol. (2020) 17(9):559–73. doi: 10.1038/s41569-020-0363-2
26. Fatima A, Rasool S, Devi S, Talha M, Waqar F, Nasir M, et al. Exploring the cardiovascular benefits of sodium-glucose cotransporter-2 (SGLT2) inhibitors: expanding horizons beyond diabetes management. Cureus. (2023) 15(9):e46243. doi: 10.7759/cureus.46243
27. Packer M, Wilcox CS, Testani JM. Critical analysis of the effects of SGLT2 inhibitors on renal tubular sodium, water and chloride homeostasis and their role in influencing heart failure outcomes. Circulation. (2023) 148(4):354–72. doi: 10.1161/CIRCULATIONAHA.123.064346
28. Honka H, Solis-Herrera C, Triplitt C, Norton L, Butler J, DeFronzo RA. Therapeutic manipulation of myocardial metabolism: JACC state-of-the-art review. J Am Coll Cardiol. (2021) 77(16):2022–39. doi: 10.1016/j.jacc.2021.02.057
29. Packer M. Molecular, cellular, clinical evidence that sodium-glucose cotransporter 2 inhibitors act as neurohormonal antagonists when used for the treatment of chronic heart failure. J Am Heart Assoc. (2020) 9(16):e016270. doi: 10.1161/JAHA.120.016270
30. McMurray JJV, Docherty KF, de Boer RA, Hammarstedt A, Kitzman DW, Kosiborod MN, et al. Effect of dapagliflozin versus placebo on symptoms and 6-minute walk distance in patients with heart failure: the DETERMINE randomized clinical trials. Circulation. (2024) 149(11):825–38. doi: 10.1161/CIRCULATIONAHA.123.065061
31. Packer M, Anker SD, Butler J, Filippatos G, Pocock SJ, Carson P, et al. Cardiovascular and renal outcomes with empagliflozin in heart failure. N Engl J Med. (2020) 383(15):1413–24. doi: 10.1056/NEJMoa2022190
32. McMurray JJV, Solomon SD, Inzucchi SE, Køber L, Kosiborod MN, Martinez FA, et al. Dapagliflozin in patients with heart failure and reduced ejection fraction. N Engl J Med. (2019) 381(21):1995–2008. doi: 10.1056/NEJMoa1911303
33. Requena-Ibanez JA, Santos-Gallego CG, Rodriguez-Cordero A, Vargas-Delgado AP, Mancini D, Sartori S, et al. Mechanistic insights of empagliflozin in nondiabetic patients with HFrEF: from the EMPA-TROPISM study. JACC Heart Fail. (2021) 9(8):578–89. doi: 10.1016/j.jchf.2021.04.014
34. Anker SD, Butler J, Filippatos G, Ferreira JP, Bocchi E, Böhm M, et al. Empagliflozin in heart failure with a preserved ejection fraction. N Engl J Med. (2021) 385(16):1451–61. doi: 10.1056/NEJMoa2107038
35. Solomon SD, McMurray JJV, Claggett B, de Boer RA, DeMets D, Hernandez AF, et al. Dapagliflozin in heart failure with mildly reduced or preserved ejection fraction. N Engl J Med. (2022) 387(12):1089–98. doi: 10.1056/NEJMoa2206286
36. Cox ZL, Collins SP, Hernandez GA, McRae AT, Davidson BT, Adams K, et al. Efficacy and safety of dapagliflozin in patients with acute heart failure. J Am Coll Cardiol. (2024) 83(14):1295–306. doi: 10.1016/j.jacc.2024.02.009
37. Voorrips SN, Boorsma EM, Beusekamp JC, De-Boer RA, Connelly MA, Dullaart RPF, et al. Longitudinal changes in circulating ketone body levels in patients with acute heart failure: a post hoc analysis of the EMPA-response-AHF trial. J Card Fail. (2023) 29(1):33–41. doi: 10.1016/j.cardfail.2022.09.009
38. Ferreira JP, Blatchford JP, Teerlink JR, Kosiborod MN, Angermann CE, Biegus J, et al. Mineralocorticoid receptor antagonist use and the effects of empagliflozin on clinical outcomes in patients admitted for acute heart failure: findings from EMPULSE. Eur J Heart Fail. (2023) 25(10):1797–805. doi: 10.1002/ejhf.2982
39. Mordi NA, Mordi IR, Singh JS, McCrimmon RJ, Struthers AD, Lang CC. Renal and cardiovascular effects of SGLT2 inhibition in combination with loop diuretics in patients with type 2 diabetes and chronic heart failure: the RECEDE-CHF trial. Circulation. (2020) 142(18):1713–24. doi: 10.1161/CIRCULATIONAHA.120.048739
40. Nassif ME, Kosiborod M. Effects of sodium glucose cotransporter type 2 inhibitors on heart failure. Diabetes Obes Metab. (2019) 21(2):19–23. doi: 10.1111/dom.13678
41. Thygesen K, White HD. Universal definition of myocardial infarction. J Am Coll Cardiol. (2007) 50(22):2173–95. doi: 10.1016/j.jacc.2007.09.011
42. Truesdell AG, Mehta A, Cilia LA. Myocardial infarction, cardiogenic shock, and cardiac arrest: management made simple, but not too simple. J Am Coll Cardiol. (2023) 81(12):1177–80. doi: 10.1016/j.jacc.2023.02.001
43. Sgarbossa EB. Electrocardiogram in myocardial infarction: what is most relevant? J Am Coll Cardiol. (2000) 35(1):257–8. doi: 10.1016/S0735-1097(99)00511-2
44. Jiang K, Xu Y, Wang D, Chen F, Tu Z, Qian J, et al. Cardioprotective mechanism of SGLT2 inhibitor against myocardial infarction is through reduction of autosis. Protein Cell. (2022) 13(5):336–59. doi: 10.1007/s13238-020-00809-4
45. Mizuno M, Kuno A, Yano T, Miki T, Oshima H, Sato T, et al. Empagliflozin normalizes the size and number of mitochondria and prevents reduction in mitochondrial size after myocardial infarction in diabetic hearts. Physiol Rep. (2018) 6(12):e13741. doi: 10.14814/phy2.13741
46. Lee TM, Chang NC, Lin SZ. Dapagliflozin, a selective SGLT2 inhibitor, attenuated cardiac fibrosis by regulating the macrophage polarization via STAT3 signaling in infarcted rat hearts. Free Radic Biol Med. (2017) 104:298–310. doi: 10.1016/j.freeradbiomed.2017.01.035
47. Paolisso P, Bergamaschi L, Santulli G, Gallinoro E, Cesaro A, Gragnano F, et al. Infarct size, inflammatory burden, and admission hyperglycemia in diabetic patients with acute myocardial infarction treated with SGLT2-inhibitors: a multicenter international registry. Cardiovasc Diabetol. (2022) 21(1):77. doi: 10.1186/s12933-022-01506-8
48. Cesaro A, Gragnano F, Paolisso P, Bergamaschi L, Gallinoro E, Sardu C, et al. In-hospital arrhythmic burden reduction in diabetic patients with acute myocardial infarction treated with SGLT2-inhibitors: insights from the SGLT2-I AMI PROTECT study. Front Cardiovasc Med. (2022) 9:1012220. doi: 10.3389/fcvm.2022.1012220
49. von Lewinski D, Kolesnik E, Tripolt NJ, Pferschy PN, Benedikt M, Wallner M, et al. Empagliflozin in acute myocardial infarction: the EMMY trial. Eur Heart J. (2022) 43(41):4421–32. doi: 10.1093/eurheartj/ehac494
50. James S, Erlinge D, Storey RF, McGuire DK, de Belder M, Björkgren I, et al. Rationale and design of the DAPA-MI trial: dapagliflozin in patients without diabetes mellitus with acute myocardial infarction. Am Heart J. (2023) 266:188–97. doi: 10.1016/j.ahj.2023.08.008
51. Furtado RHM, Bonaca MP, Raz I, Zelniker TA, Mosenzon O, Cahn A, et al. Dapagliflozin and cardiovascular outcomes in patients with type 2 diabetes Mellitus and previous myocardial infarction. Circulation. (2019) 139(22):2516–27. doi: 10.1161/CIRCULATIONAHA.119.039996
52. Udell JA, Petrie MC, Jones WS, Anker SD, Harrington J, Mattheus M, et al. Left ventricular function, congestion, and effect of empagliflozin on heart failure risk after myocardial infarction. J Am Coll Cardiol. (2024) 83(23):2233–46. doi: 10.1016/j.jacc.2024.03.405
53. Hernandez AF, Udell JA, Jones WS, Anker SD, Petrie MC, Harrington J, et al. Effect of empagliflozin on heart failure outcomes after acute myocardial infarction: insights from the EMPACT-MI trial. Circulation. (2024) 149(21):1627–38. doi: 10.1161/CIRCULATIONAHA.124.069217
54. Shimizu W, Kubota Y, Hoshika Y, Mozawa K, Tara S, Tokita Y, et al. Effects of empagliflozin versus placebo on cardiac sympathetic activity in acute myocardial infarction patients with type 2 diabetes mellitus: the EMBODY trial. Cardiovasc Diabetol. (2020) 19(1):148. doi: 10.1186/s12933-020-01127-z
55. Dayem KA, Younis O, Zarif B, Attia S, AbdelSalam A. Impact of dapagliflozin on cardiac function following anterior myocardial infarction in non-diabetic patients—DACAMI (a randomized controlled clinical trial). Int J Cardiol. (2023) 379:9–14. doi: 10.1016/j.ijcard.2023.03.002
56. Fitchett D, Zinman B, Inzucchi SE, Wanner C, Anker SD, Pocock S, et al. Effect of empagliflozin on total myocardial infarction events by type and additional coronary outcomes: insights from the randomized EMPA-REG OUTCOME trial. Cardiovasc Diabetol. (2024) 23(1):248. doi: 10.1186/s12933-024-02328-6
57. Arnett DK, Claas SA. Omics of blood pressure and hypertension. Circ Res. (2018) 122(10):1409–19. doi: 10.1161/CIRCRESAHA.118.311342
58. Zhou B, Perel P, Mensah GA, Ezzati M. Global epidemiology, health burden and effective interventions for elevated blood pressure and hypertension. Nat Rev Cardiol. (2021) 18(11):785–802. doi: 10.1038/s41569-021-00559-8
59. Cohen DL, Townsend RR. Hypertension in 2016: blood pressure goals, variability and SGLT2 blockade in CKD. Nat Rev Nephrol. (2017) 13(2):75–6. doi: 10.1038/nrneph.2016.189
60. Kawasoe S, Maruguchi Y, Kajiya S, Uenomachi H, Miyata M, Kawasoe M, et al. Mechanism of the blood pressure-lowering effect of sodium-glucose cotransporter 2 inhibitors in obese patients with type 2 diabetes. BMC Pharmacol Toxicol. (2017) 18(1):23. doi: 10.1186/s40360-017-0125-x
61. Woods TC, Satou R, Miyata K, Katsurada A, Dugas CM, Klingenberg NC, et al. Canagliflozin prevents intrarenal angiotensinogen augmentation and mitigates kidney injury and hypertension in mouse model of type 2 diabetes Mellitus. Am J Nephrol. (2019) 49(4):331–42. doi: 10.1159/000499597
62. Dabravolski SA, Zhuravlev AD, Kartuesov AG, Borisov EE, Sukhorukov VN, Orekhov AN. Mitochondria-mediated cardiovascular benefits of sodium-glucose co-transporter 2 inhibitors. Int J Mol Sci. (2022) 23(10):5371. doi: 10.3390/ijms23105371
63. Kravtsova O, Bohovyk R, Levchenko V, Palygin O, Klemens CA, Rieg T, et al. SGLT2 Inhibition effect on salt-induced hypertension, RAAS, and Na(+) transport in Dahl SS rats. Am J Physiol Renal Physiol. (2022) 322(6):F692–707. doi: 10.1152/ajprenal.00053.2022
64. Tikkanen I, Narko K, Zeller C, Green A, Salsali A, Broedl UC, et al. Empagliflozin reduces blood pressure in patients with type 2 diabetes and hypertension. Diabetes Care. (2015) 38(3):420–8. doi: 10.2337/dc14-1096
65. Ferdinand KC, Izzo JL, Lee J, Meng L, George J, Salsali A, et al. Antihyperglycemic and blood pressure effects of empagliflozin in black patients with type 2 diabetes mellitus and hypertension. Circulation. (2019) 139(18):2098–109. doi: 10.1161/CIRCULATIONAHA.118.036568
66. Chilton R, Tikkanen I, Hehnke U, Woerle HJ, Johansen OE. Impact of empagliflozin on blood pressure in dipper and non-dipper patients with type 2 diabetes mellitus and hypertension. Diabetes Obes Metab. (2017) 19(11):1620–4. doi: 10.1111/dom.12962
67. Mancia G, Cannon CP, Tikkanen I, Zeller C, Ley L, Woerle HJ, et al. Impact of empagliflozin on blood pressure in patients with type 2 diabetes mellitus and hypertension by background antihypertensive medication. Hypertension. (2016) 68(6):1355–64. doi: 10.1161/HYPERTENSIONAHA.116.07703
68. Cheng L, Fu Q, Zhou L, Fan Y, Liu F, Fan Y, et al. Effect of SGLT-2 inhibitor, empagliflozin, on blood pressure reduction in Chinese elderly hypertension patients with type 2 diabetes and its possible mechanisms. Sci Rep. (2022) 12(1):3525. doi: 10.1038/s41598-022-07395-x
69. Heerspink HJ, Johnsson E, Gause-Nilsson I, Cain VA, Sjöström CD. Dapagliflozin reduces albuminuria in patients with diabetes and hypertension receiving renin-angiotensin blockers. Diabetes Obes Metab. (2016) 18(6):590–7. doi: 10.1111/dom.12654
70. Curtis AB, Karki R, Hattoum A, Sharma UC. Arrhythmias in patients ≥80 years of age: pathophysiology, management, and outcomes. J Am Coll Cardiol. (2018) 71(18):2041–57. doi: 10.1016/j.jacc.2018.03.019
71. John RM, Kumar S. Sinus node and atrial arrhythmias. Circulation. (2016) 133(19):1892–900. doi: 10.1161/CIRCULATIONAHA.116.018011
72. Giudicessi JR, Ackerman MJ, Fatkin D, Kovacic JC. Precision medicine approaches to cardiac arrhythmias: JACC focus seminar 4/5. J Am Coll Cardiol. (2021) 77(20):2573–91. doi: 10.1016/j.jacc.2021.03.325
73. Jhuo SJ, Lin Y-H, Liu I-H, Lin T-H, Wu B-N, Lee K-T, et al. Sodium glucose cotransporter 2 (SGLT2) inhibitor ameliorate metabolic disorder and obesity induced cardiomyocyte injury and mitochondrial remodeling. Int J Mol Sci. (2023) 24(7):6842. doi: 10.3390/ijms24076842
74. Palmer BF, Clegg DJ. Kidney-protective effects of SGLT2 inhibitors. Clin J Am Soc Nephrol. (2023) 18(2):279–89. doi: 10.2215/CJN.09380822
75. Santos-Gallego CG, Requena-Ibanez JA, San Antonio R, Ishikawa K, Watanabe S, Picatoste B, et al. Empagliflozin ameliorates adverse left ventricular remodeling in nondiabetic heart failure by enhancing myocardial energetics. J Am Coll Cardiol. (2019) 73(15):1931–44. doi: 10.1016/j.jacc.2019.01.056
76. Sardu C, Massimo Massetti M, Rambaldi P, Gatta G, Cappabianca S, Sasso FC, et al. SGLT2-inhibitors Reduce the cardiac autonomic neuropathy dysfunction and vaso-vagal syncope recurrence in patients with type 2 diabetes mellitus: the SCAN study. Metab Clin Exp. (2022) 137:155243. doi: 10.1016/j.metabol.2022.155243
77. Bohm M, Slawik J, Brueckmann M, Mattheus M, George JT, Ofstad AP, et al. Efficacy of empagliflozin on heart failure and renal outcomes in patients with atrial fibrillation: data from the EMPA-REG OUTCOME trial. Eur J Heart Fail. (2020) 22(1):126–35. doi: 10.1002/ejhf.1663
78. Curtain JP, Docherty KF, Jhund PS, Petrie MC, Inzucchi SE, Køber L, et al. Effect of dapagliflozin on ventricular arrhythmias, resuscitated cardiac arrest, or sudden death in DAPA-HF. Eur Heart J. (2021) 42(36):3727–38. doi: 10.1093/eurheartj/ehab560
79. Butt JH, Kondo T, Jhund PS, Comin-Colet J, de Boer RA, Desai AS, et al. Atrial fibrillation and dapagliflozin efficacy in patients with preserved or mildly reduced ejection fraction. J Am Coll Cardiol. (2022) 80(18):1705–17. doi: 10.1016/j.jacc.2022.08.718
80. Fujiki S, Iijima K, Nakagawa Y, Takahashi K, Okabe M, Kusano K, et al. Effect of empagliflozin on ventricular arrhythmias in patients with type 2 diabetes treated with an implantable cardioverter-defibrillator: the EMPA-ICD trial. Cardiovasc Diabetol. (2024) 23(1):224. doi: 10.1186/s12933-024-02309-9
81. Zhou Z, Jardine MJ, Li Q, Neuen BL, Cannon CP, de Zeeuw D, et al. Effect of SGLT2 inhibitors on stroke and atrial fibrillation in diabetic kidney disease: results from the CREDENCE trial and meta-analysis. Stroke. (2021) 52(5):1545–56. doi: 10.1161/STROKEAHA.120.031623
82. Webster AC, Nagler EV, Morton RL, Masson P. Chronic kidney disease. Lancet. (2017) 389(10075):1238–52. doi: 10.1016/S0140-6736(16)32064-5
83. Kalantar-Zadeh K, Fouque D. Nutritional management of chronic kidney disease. N Engl J Med. (2017) 377(18):1765–76. doi: 10.1056/NEJMra1700312
84. Breyer MD, Susztak K. The next generation of therapeutics for chronic kidney disease. Nat Rev Drug Discov. (2016) 15(8):568–88. doi: 10.1038/nrd.2016.67
85. Abdul-Ghani MA, Norton L, DeFronzo RA. Renal sodium-glucose cotransporter inhibition in the management of type 2 diabetes mellitus. Am J Physiol Renal Physiol. (2015) 309(11):F889–900. doi: 10.1152/ajprenal.00267.2015
86. Upadhyay A. SGLT2 Inhibitors and kidney protection: mechanisms beyond tubuloglomerular feedback. Kidney360. (2024) 5(5):771–82. doi: 10.34067/KID.0000000000000425
87. Kim JH, Lee M, Kim SH, Kim SR, Lee B, Kang ES, et al. Sodium-glucose cotransporter 2 inhibitors regulate ketone body metabolism via inter-organ crosstalk. Diabetes Obes Metab. (2019) 21(4):801–11. doi: 10.1111/dom.13577
88. Yaribeygi H, Maleki M, Atkin SL, Jamialahmadi T, Sahebkar A. SGLT2 Inhibitors and autophagy in diabetes. Cell Biochem Funct. (2023) 41(4):392–8. doi: 10.1002/cbf.3792
89. Onishi A, Fu Y, Patel R, Darshi M, Crespo-Masip M, Huang W, et al. A role for tubular Na(+)/H(+) exchanger NHE3 in the natriuretic effect of the SGLT2 inhibitor empagliflozin. Am J Physiol Renal Physiol. (2020) 319(4):F712–28. doi: 10.1152/ajprenal.00264.2020
90. Sridharan K, Sivaramakrishnan G. Sodium glucose cotransporter-2 inhibitors improve endothelial function and arterial stiffness in diabetic individuals: a systematic review and network meta-analysis. Curr Vasc Pharmacol. (2025). doi: 10.2174/0115701611337138241226101956
91. Heerspink HJL, Stefánsson BV, Correa-Rotter R, Chertow GM, Greene T, Hou F-F, et al. Dapagliflozin in patients with chronic kidney disease. N Engl J Med. (2020) 383(15):1436–46. doi: 10.1056/NEJMoa2024816
92. Dagogo-Jack S, Pratley RE, Cherney DZI, McGuire DK, Cosentino F, Shih WJ, et al. Glycemic efficacy and safety of the SGLT2 inhibitor ertugliflozin in patients with type 2 diabetes and stage 3 chronic kidney disease: an analysis from the VERTIS CV randomized trial. BMJ Open Diabetes Res Care. (2021) 9(1):e002484. doi: 10.1136/bmjdrc-2021-002484
93. van der Aart-van der Beek AB, Koomen JV, Dekkers CCJ, Barbour SJ, Boulton DW, Gansevoort RT, et al. Evaluation of the pharmacokinetics and exposure-response relationship of dapagliflozin in patients without diabetes and with chronic kidney disease. Clin Pharmacokinet. (2021) 60(4):517–25. doi: 10.1007/s40262-020-00956-1
94. Mc Causland FR, Claggett BL, Vaduganathan M, Desai AS, Jhund P, de Boer RA, et al. Dapagliflozin and kidney outcomes in patients with heart failure with mildly reduced or preserved ejection fraction: a prespecified analysis of the DELIVER randomized clinical trial. JAMA Cardiol. (2023) 8(1):56–65. doi: 10.1001/jamacardio.2022.4210
95. Provenzano M, Puchades MJ, Garofalo C, Jongs N, D’Marco L, Andreucci M, et al. Albuminuria-lowering effect of dapagliflozin, eplerenone, and their combination in patients with chronic kidney disease: a randomized crossover clinical trial. J Am Soc Nephrol. (2022) 33(8):1569–80. doi: 10.1681/ASN.2022020207
96. Perkovic V, Jardine MJ, Neal B, Bompoint S, Heerspink HJL, Charytan DM, et al. Canagliflozin and renal outcomes in type 2 diabetes and nephropathy. N Engl J Med. (2019) 380(24):2295–306. doi: 10.1056/NEJMoa1811744
97. Bhatt DL, Szarek M, Pitt B, Cannon CP, Leiter LA, McGuire DK, et al. Sotagliflozin in patients with diabetes and chronic kidney disease. N Engl J Med. (2021) 384(2):129–39. doi: 10.1056/NEJMoa2030186
98. Cherney DZI, Ferrannini E, Umpierrez GE, Peters AL, Rosenstock J, Powell DR, et al. Efficacy and safety of sotagliflozin in patients with type 2 diabetes and stage 3 chronic kidney disease. Diabetes Obes Metab. (2023) 25(6):1646–57. doi: 10.1111/dom.15019
99. Cherney DZI, Ferrannini E, Umpierrez GE, Peters AL, Rosenstock J, Carroll AK, et al. Efficacy and safety of sotagliflozin in patients with type 2 diabetes and severe renal impairment. Diabetes Obes Metab. (2021) 23(12):2632–42. doi: 10.1111/dom.14513
100. Powell EE, Wong VW, Rinella M. Non-alcoholic fatty liver disease. Lancet. (2021) 397(10290):2212–24. doi: 10.1016/S0140-6736(20)32511-3
101. Ibrahim SH, Hirsova P, Gores GJ. Non-alcoholic steatohepatitis pathogenesis: sublethal hepatocyte injury as a driver of liver inflammation. Gut. (2018) 67(5):963–72. doi: 10.1136/gutjnl-2017-315691
102. Yaribeygi H, Maleki M, Jamialahmadi T, Moallem SA, Sahebkar A. Hepatic benefits of sodium-glucose cotransporter 2 inhibitors in liver disorders. EXCLI J. (2023) 22:403–14. doi: 10.17179/excli2023-6022
103. Androutsakos T, Nasiri-Ansari N, Bakasis A-D, Kyrou I, Efstathopoulos E, Randeva HS, et al. SGLT-2 Inhibitors in NAFLD: expanding their role beyond diabetes and cardioprotection. Int J Mol Sci. (2022) 23(6):3107. doi: 10.3390/ijms23063107
104. Aso Y, Kato K, Sakurai S, Kishi H, Shimizu M, Jojima T, et al. Impact of dapagliflozin, an SGLT2 inhibitor, on serum levels of soluble dipeptidyl peptidase-4 in patients with type 2 diabetes and non-alcoholic fatty liver disease. Int J Clin Pract. (2019) 73(5):e13335. doi: 10.1111/ijcp.13335
105. Cho KY, Nakamura A, Omori K, Takase T, Miya A, Yamamoto K, et al. Favorable effect of sodium-glucose cotransporter 2 inhibitor, dapagliflozin, on non-alcoholic fatty liver disease compared with pioglitazone. J Diabetes Investig. (2021) 12(7):1272–7. doi: 10.1111/jdi.13457
106. Yoneda M, Honda Y, Ogawa Y, Kessoku T, Kobayashi T, Imajo K, et al. Comparing the effects of tofogliflozin and pioglitazone in non-alcoholic fatty liver disease patients with type 2 diabetes mellitus (ToPiND study): a randomized prospective open-label controlled trial. BMJ Open Diabetes Res Care. (2021) 9(1):e001990. doi: 10.1136/bmjdrc-2020-001990
107. Aso Y, Sagara M, Niitani T, Kato K, Iijima T, Tomaru T, et al. Serum high-molecular-weight adiponectin and response to dapagliflozin in patients with type 2 diabetes and non-alcoholic fatty liver disease. J Investig Med. (2021) 69(7):1324–9. doi: 10.1136/jim-2020-001621
108. Eriksson JW, Lundkvist P, Jansson P-A, Johansson L, Kvarnström M, Moris L, et al. Effects of dapagliflozin and n-3 carboxylic acids on non-alcoholic fatty liver disease in people with type 2 diabetes: a double-blind randomised placebo-controlled study. Diabetologia. (2018) 61(9):1923–34. doi: 10.1007/s00125-018-4675-2
109. Inoue M, Hayashi A, Taguchi T, Arai R, Sasaki S, Takano K, et al. Effects of canagliflozin on body composition and hepatic fat content in type 2 diabetes patients with non-alcoholic fatty liver disease. J Diabetes Investig. (2019) 10(4):1004–11. doi: 10.1111/jdi.12980
110. Hiruma S, Shigiyama F, Kumashiro N. Empagliflozin versus sitagliptin for ameliorating intrahepatic lipid content and tissue-specific insulin sensitivity in patients with early-stage type 2 diabetes with non-alcoholic fatty liver disease: a prospective randomized study. Diabetes Obes Metab. (2023) 25(6):1576–88. doi: 10.1111/dom.15006
111. Shimizu M, Suzuki K, Kato K, Jojima T, Iijima T, Murohisa T, et al. Evaluation of the effects of dapagliflozin, a sodium-glucose co-transporter-2 inhibitor, on hepatic steatosis and fibrosis using transient elastography in patients with type 2 diabetes and non-alcoholic fatty liver disease. Diabetes Obes Metab. (2019) 21(2):285–92. doi: 10.1111/dom.13520
112. Shi M, Zhang H, Wang W, Zhang X, Liu J, Wang Q, et al. Effect of dapagliflozin on liver and pancreatic fat in patients with type 2 diabetes and non-alcoholic fatty liver disease. J Diabetes Complications. (2023) 37(10):108610. doi: 10.1016/j.jdiacomp.2023.108610
113. Phrueksotsai S, Pinyopornpanish K, Euathrongchit J, Leerapun A, Phrommintikul A, Buranapin S, et al. The effects of dapagliflozin on hepatic and visceral fat in type 2 diabetes patients with non-alcoholic fatty liver disease. J Gastroenterol Hepatol. (2021) 36(10):2952–9. doi: 10.1111/jgh.15580
114. Taheri H, Malek M, Ismail-Beigi F, Zamani F, Sohrabi M, Reza babaei M, et al. Effect of empagliflozin on liver steatosis and fibrosis in patients with non-alcoholic fatty liver disease without diabetes: a randomized, double-blind, placebo-controlled trial. Adv Ther. (2020) 37(11):4697–708. doi: 10.1007/s12325-020-01498-5
115. Gao X, Zhu C, Zhu W, Wang L. Dapagliflozin treatment alleviates fatty liver in patients with type 2 diabetes. Biomed Rep. (2025) 22(2):26. doi: 10.3892/br.2024.1904
116. Kittipibul V, Cox ZL, Chesdachai S, Fiuzat M, Lindenfeld J, Mentz RJ. Genitourinary tract infections in patients taking SGLT2 inhibitors: JACC review topic of the week. J Am Coll Cardiol. (2024) 83(16):1568–78. doi: 10.1016/j.jacc.2024.01.040
117. Nassif ME, Qintar M, Windsor SL, Jermyn R, Shavelle DM, Tang F, et al. Empagliflozin effects on pulmonary artery pressure in patients with heart failure: results from the EMBRACE-HF trial. Circulation. (2021) 143(17):1673–86. doi: 10.1161/CIRCULATIONAHA.120.052503
118. Hundertmark MJ, Adler A, Antoniades C, Coleman R, Griffin JL, Holman RR, et al. Assessment of cardiac energy metabolism, function, and physiology in patients with heart failure taking empagliflozin: the randomized, controlled EMPA-VISION trial. Circulation. (2023) 147(22):1654–69. doi: 10.1161/CIRCULATIONAHA.122.062021
119. Nassif ME, Windsor SL, Borlaug BA, Kitzman DW, Shah SJ, Tang F, et al. The SGLT2 inhibitor dapagliflozin in heart failure with preserved ejection fraction: a multicenter randomized trial. Nat Med. (2021) 27(11):1954–60. doi: 10.1038/s41591-021-01536-x
120. Borlaug BA, Reddy YNV, Braun A, Sorimachi H, Omar M, Popovic D, et al. Cardiac and metabolic effects of dapagliflozin in heart failure with preserved ejection fraction: the CAMEO-DAPA trial. Circulation. (2023) 148(10):834–44. doi: 10.1161/CIRCULATIONAHA.123.065134
121. James S, Erlinge D, Storey RF, McGuire DK, de Belder M, Eriksson N, et al. Dapagliflozin in myocardial infarction without diabetes or heart failure. NEJM Evid. (2024) 3(2):EVIDoa2300286. doi: 10.1056/EVIDoa2300286
122. Butler J, Jones WS, Udell JA, Anker SD, Petrie MC, Harrington J, et al. Empagliflozin after acute myocardial infarction. N Engl J Med. (2024) 390(16):1455–66. doi: 10.1056/NEJMoa2314051
123. Filippatos G, Farmakis D, Butler J, Zannad F, Ferreira JP, Ofstad AP, et al. Empagliflozin in heart failure with preserved ejection fraction with and without atrial fibrillation. Eur J Heart Fail. (2023) 25(7):970–7. doi: 10.1002/ejhf.2861
124. Butt JH, Docherty KF, Jhund PS, de Boer RA, Böhm M, Desai AS, et al. Dapagliflozin and atrial fibrillation in heart failure with reduced ejection fraction: insights from DAPA-HF. Eur J Heart Fail. (2022) 24(3):513–25. doi: 10.1002/ejhf.2381
125. The EMPA-KIDNEY Collaborative Group, Herrington WG, Staplin N, Wanner C, Green JB, Hauske SJ, et al. Empagliflozin in patients with chronic kidney disease. N Engl J Med. (2023) 388(2):117–27. doi: 10.1056/NEJMoa2204233
126. Tuttle KR, Hauske SJ, Canziani ME, Caramori ML, Cherney D, Cronin L, et al. Efficacy and safety of aldosterone synthase inhibition with and without empagliflozin for chronic kidney disease: a randomised, controlled, phase 2 trial. Lancet. (2024) 403(10424):379–90. doi: 10.1016/S0140-6736(23)02408-X
127. Heerspink HJL, Kiyosue A, Wheeler DC, Lin M, Wijkmark E, Carlson G, et al. Zibotentan in combination with dapagliflozin compared with dapagliflozin in patients with chronic kidney disease (ZENITH-CKD): a multicentre, randomised, active-controlled, phase 2b, clinical trial. Lancet. (2023) 402(10416):2004–17. doi: 10.1016/S0140-6736(23)02230-4
128. Pollock C, Stefánsson B, Reyner D, Rossing P, Sjöström CD, Wheeler DC, et al. Albuminuria-lowering effect of dapagliflozin alone and in combination with saxagliptin and effect of dapagliflozin and saxagliptin on glycaemic control in patients with type 2 diabetes and chronic kidney disease (DELIGHT): a randomised, double-blind, placebo-controlled trial. Lancet Diabetes Endocrinol. (2019) 7(6):429–41. doi: 10.1016/S2213-8587(19)30086-5
129. Chertow GM, Vart P, Jongs N, Toto RD, Gorriz JL, Hou FF, et al. Effects of dapagliflozin in stage 4 chronic kidney disease. J Am Soc Nephrol. (2021) 32(9):2352–61. doi: 10.1681/ASN.2021020167
130. Mosenzon O, Raz I, Wiviott SD, Schechter M, Goodrich EL, Yanuv I, et al. Dapagliflozin and prevention of kidney disease among patients with type 2 diabetes: post hoc analyses from the DECLARE-TIMI 58 trial. Diabetes Care. (2022) 45(10):2350–9. doi: 10.2337/dc22-0382
131. Wanner C, Lachin JM, Inzucchi SE, Fitchett D, Mattheus M, George J, et al. Empagliflozin and clinical outcomes in patients with type 2 diabetes mellitus, established cardiovascular disease, and chronic kidney disease. Circulation. (2018) 137(2):119–29. doi: 10.1161/CIRCULATIONAHA.117.028268
132. Adamson C, Docherty KF, Heerspink HJL, de Boer RA, Damman K, Inzucchi SE, et al. Initial decline (dip) in estimated glomerular filtration rate after initiation of dapagliflozin in patients with heart failure and reduced ejection fraction: insights from DAPA-HF. Circulation. (2022) 146(6):438–49. doi: 10.1161/CIRCULATIONAHA.121.058910
133. Kinoshita T, Shimoda M, Nakashima K, Fushimi Y, Hirata Y, Tanabe A, et al. Comparison of the effects of three kinds of glucose-lowering drugs on non-alcoholic fatty liver disease in patients with type 2 diabetes: a randomized, open-label, three-arm, active control study. J Diabetes Investig. (2020) 11(6):1612–22. doi: 10.1111/jdi.13279
Keywords: SGLT-2 inhibitors, cardiovascular diseases, heart failure, chronic kidney disease, nonalcoholic fatty liver disease
Citation: Feng Q, Wu M and Mai Z (2025) Emerging horizons: clinical applications and multifaceted benefits of SGLT-2 inhibitors beyond diabetes. Front. Cardiovasc. Med. 12:1482918. doi: 10.3389/fcvm.2025.1482918
Received: 19 January 2025; Accepted: 10 March 2025;
Published: 20 March 2025.
Edited by:
DeLisa Fairweather, Mayo Clinic Florida, United StatesReviewed by:
Lizhu Han, Sichuan Academy of Medical Sciences and Sichuan Provincial People's Hospital, ChinaCopyright: © 2025 Feng, Wu and Mai. This is an open-access article distributed under the terms of the Creative Commons Attribution License (CC BY). The use, distribution or reproduction in other forums is permitted, provided the original author(s) and the copyright owner(s) are credited and that the original publication in this journal is cited, in accordance with accepted academic practice. No use, distribution or reproduction is permitted which does not comply with these terms.
*Correspondence: Zizhao Mai, bWFpeml6aGFvQHNtdS5lZHUuY24=
Disclaimer: All claims expressed in this article are solely those of the authors and do not necessarily represent those of their affiliated organizations, or those of the publisher, the editors and the reviewers. Any product that may be evaluated in this article or claim that may be made by its manufacturer is not guaranteed or endorsed by the publisher.
Research integrity at Frontiers
Learn more about the work of our research integrity team to safeguard the quality of each article we publish.