- 1Department of Medicine, Christchurch Heart Institute, University of Otago Christchurch, Christchurch, New Zealand
- 2Department of Surgery, University of Otago Christchurch, Christchurch, New Zealand
Cardiovascular disease (CVD) remains a global health burden despite advances in prevention and treatment. Conventional biomarkers, while effective for a number of patient groups, fail to provide personalized diagnosis and prognosis, necessitating the exploration of novel markers. Advancements in sequencing technology have unveiled the role of cell-free DNA (cfDNA) as a reservoir of genetic information from all cells within the body, and associations between elevated cfDNA levels and CVD risk factors and status have been reported. Recent attention has turned to a subset of cfDNA, circulating bacterial DNA (cbDNA), derived from gut microbiota, as a potential biomarker. Investigations into microbial translocation from the gut, particularly the phenomenon of ‘leaky gut,’ reveal its association with CVD and provide a potential source for cbDNA. Here, we review the existing literature on cbDNA in CVD, highlighting its potential diagnostic and prognostic value. Current studies have largely been carried out in small, disparate cohorts, using different sample types and a range of methodologies. While cbDNA shows potential as a diagnostic and prognostic biomarker, the lack of consensus in methodologies and populations studied calls for standardized approaches and large cohorts to establish cbDNA as a reliable CVD biomarker. Future research should focus on identifying the source of cbDNA and its pathological relevance, utilizing advanced sequencing techniques and standardized cohorts for conclusive findings.
1 Introduction
Despite medical advances, cardiovascular disease (CVD), remains a leading cause of morbidity and mortality worldwide, consuming a significant amount of health-care resources (1). The complexity of the disease means that although a number of patients benefit from the current biomarkers, some are being disadvantaged by this ‘one size fits all’ testing. Accordingly, the identification of novel biomarkers is required to complement or replace current markers to allow for timely diagnosis and improve clinical outcomes for patients and reduce the large numbers of people affected by the disease. Recent advances in sequencing technology have led to the explosion of knowledge in the field of circulating cell-free DNA (cfDNA). cfDNA is found in bodily fluids and acts like a genetic reservoir that carries genetic information from all cells within the body. The amount of cfDNA is minimal in healthy individuals but concentrations have been shown to increase with age, pregnancy, exercise and disease (2), thus, making it an attractive biomarker target for clinical applications. Several recent studies have shown a strong association between the presence of cfDNA with both the risk factors and status of CVD (3, 4). Although research into cfDNA is increasing, there are very few studies identifying and characterizing circulating bacterial DNA (cbDNA), a subset of cfDNA derived from gut bacteria. As a result, cbDNA in CVD is not well understood, nor is its biomarker utility. Here, we review the current literature on cbDNA in CVD (Figure 1 and Table 1).
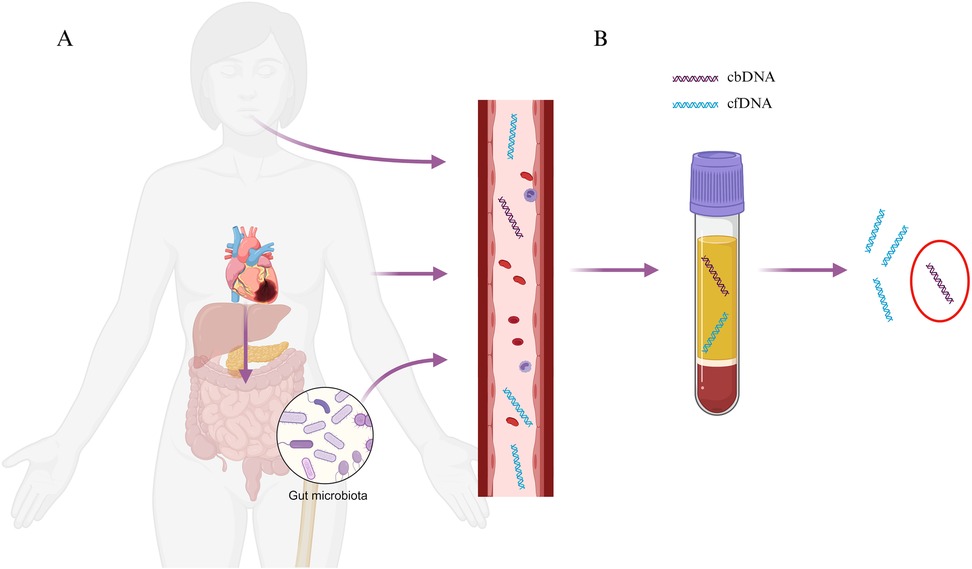
Figure 1. Graphical depiction of (A) potential routes of translocation of bacterial DNA into the bloodstream including oral, skin, gut and (B) subsequent detection in plasma samples. Created with BioRender.com.
2 Gut-heart axis
In addition to established risk factors, there is an expanding body of evidence to support the role of the human microbiome and the gut-heart axis in the establishment of cardiovascular disease (CVD) (5). The gut microbiome consists of trillions of microbes, including bacteria, viruses and eukaryotes, which has the ability to modify or influence numerous biological functions in the host, including contributing to the pathogenesis of multiple immune-mediated diseases and metabolic conditions (6). There is increasing evidence to show that gut dysbiosis, the adverse changes in the gut microbiome present, can induce inflammation, leading to the onset of chronic disease (7). In the context of CVD, products of microbial metabolism, for example trimethylamine-N-oxide (TMAO), have been shown to promote atherogenic activity (8). Research into the gut-heart axis has led to growing interest in the potential of gut health interventions, such as dietary modifications, prebiotics and probiotics as a means of improving cardiovascular health (5).
3 Leaky gut
Historically, blood was presumed to be sterile and the identification of microbes in the circulatory system was only thought to occur in infectious disease and sepsis (9, 10), but of late both microbes and cbDNA has been found in both healthy individuals and patients with various diseases (11–14). Unlike the proposed mechanisms of active release of cfDNA into the circulation from human cells, namely, apoptosis or necrosis, an alternate hypothesis has been proposed for the origin of cbDNA. There are several suggested routes for microbial ingress into the circulation including oral (15) and skin, however, microbial translocation from the gut has been proposed as the major contributor of cbDNA in the circulation (16). Here, failure of the gut barrier, termed “leaky gut”, enables bacteria and their endotoxins to cross the intestinal barrier into the circulatory system. Increased intestinal permeability has been observed in animal models of myocardial infarction (MI) (17, 18) and atherosclerosis (19), as well as human studies of CVD including chronic heart failure (20), MI (18), atherosclerosis and coronary artery disease (21, 22). Markers of gut bacterial translocation into the circulatory system, namely lipopolysaccharide (LPS) and D-lactate, have also been linked to CVD prognosis. Zhou et al. provided the first evidence that CVD outcomes post-MI are associated with intestinal microbiota translocation into the systemic circulation (18). They reported that the plasma markers LPS and D-lactate, were significantly increased and correlated with systemic inflammation and predicted the occurrence of a first major adverse cardiovascular event.
4 Methodological differences
Initial studies identifying circulating microbes in CVD focused on single bacterial species detected using PCR (23, 24). With the advent of microbiome analysis by 16S rRNA gene amplicon sequencing and/or metagenomic analysis, the detection of all microbes/microbial DNA in the circulation could be identified, allowing for microbial diversity and taxa abundance to be assessed. A number of studies have now been published that utilize 16S rRNA gene amplicon sequencing; the 16S rRNA gene contains highly conserved regions interspersed with hypervariable regions that provide genus/species-specific signatures that are used for bacterial taxonomic profiling. Utilizing metagenomic sequencing or direct DNA sequencing increases the taxonomic resolution. However, unlike culture-based techniques, these methods do not differentiate between live and dead bacteria, making it difficult to infer cause or consequence. Further, the choice of whole blood, plasma or serum when analyzing circulating bacterial DNA generates different results.
5 cbDNA in whole blood
Utilizing 16S rRNA sequencing of whole blood, Amar et al. were the first to show that microbial dysbiosis in the circulation may play a role in the pathological processes in CVD (25), in a large (n = 3,936) longitudinal study of the general population, free of obesity and diabetes. They found that baseline Eubacteria was significantly lower and Proteobacteria higher in peripheral blood leucocytes of patients that went on to have an acute cardiovascular event comprising MI, myocardial ischemia and/or significant coronary stenosis. Using multivariate Cox model analysis, they found a 3.7-fold increase in the risk of CVD events in participants who had the lowest levels of Eubacteria and the highest levels of Proteobacteria (p = 0.003) (25). Similarly, an increase in Proteobacteria has also been observed in the whole blood of CVD patients (mix of valvular, ischemic and congenital heart disease) compared to healthy controls in a small study in an Indian population (26).
Elevated bacterial abundance and specific bacterial signatures have been observed in the whole blood of patients following an MI. In ST-segment elevation myocardial infarction (STEMI, n = 100) patients, one study found greater microbial diversity when compared to patients with stable coronary heart disease (CHD, n = 50) and healthy individuals (n = 49) (18). Blood bacterial characteristics were distinct for each group, with an increased abundance of gut specific bacteria (Lactobacillus, Bacteroides, and Streptococcus) found in STEMI patients (18). This finding was not replicated by Amar et al., who reported that although acute MI patients (n = 99) had higher abundance and a specific bacterial signature, they had lower blood bacterial diversity compared to non-MI individuals at high CVD risk but free of coronary disease (n = 103) (27).
6 cbDNA in plasma
Few studies have analyzed cbDNA in plasma, with only one comparison being made between whole blood (26) and plasma (28). Here circulating bacterial DNA was analyzed in the same group of CVD patients (valvular, ischemic and congenital heart disease). In plasma, patients with CVD were found to have higher circulating bacterial diversity compared to healthy individuals. Actinobacteria and Proteobacteria were the two major phyla represented in both CVD and control samples, followed by a smaller fraction of Firmicutes. Actinobacteria was dominant in CVD patients, followed by Proteobacteria, whereas the opposite was observed in the controls (28). Although these results are based on a small subset of the cohort with metagenomic shotgun sequencing with low depth. These results were in contrast to what was reported by the same group in whole blood (26) where patients had an increased amount of Proteobacteria and a reduction in Firmicutes in comparison to healthy controls. Analysis of the circulating bacteria in whole blood was performed using 16S rRNA amplicon sequencing, whereas only relative abundance was assessed in the plasma of patients by PCR. These methodological differences may account for some of the differences seen. Further, Dinakaran et al. were the first to report the use of bacterial abundance in plasma to investigate the diagnostic utility of cbDNA in CVD. The authors showed that increased bacterial abundance was able to discriminate ischemic heart disease (p < 0.0001) and CHD patients (p < 0.0001) from healthy controls, but the sample size was small and highly selected.
7 cbDNA as a prognostic marker
The prognostic utility of cbDNA has been investigated in patients with kidney disease [end stage renal disease (29) and peritoneal dialysis patients (30)], a group who have an elevated risk of developing CVD or having a CVD event. A small pilot study of 17 hemodialysis patients who subsequently died from a CVD event and matched controls, reported that the proportion of cbDNA from Actinobacteria and Proteobacteria present in serum was slightly associated with risk of cardiovascular death [adjusted odds ratios [95% confidence intervals] = 1.12 [0.98–1.29] and 0.88 [0.76–1.02] for 1% increase, respectively] (29). Another study of 191 new peritoneal dialysis patients (30) showed a weak negative correlation between cbDNA abundance and the number of hospital admissions and duration of hospitalization for cardiovascular reasons. They reported that each doubling in plasma cbDNA concentration confers a 26.9% excess in risk of developing a composite cardiovascular end point comprising a number of outcomes for example, cardiovascular death, non-fatal MI or stroke and hospital admission for unstable angina. When cbDNA level was split into quartiles, the event-free survival for those with the lowest cbDNA concentration was 86.1% whereas those with the highest was 30.8% (30).
8 Ethnic variation in cbDNA
While some studies suggest that cfDNA abundance and characteristics might vary across different ethnic groups (31, 32), there has been no research to date exploring these differences in cbDNA. Diet, lifestyle, genetic lineage, socioeconomic status, and metabolic profiles are all factors that may differ between ethnic groups and could potentially contribute to varying cbDNA profiles. Among the studies reviewed here, only one provided demographic information regarding the ethnic composition of the studied population. Sumida et al. reported that their population was predominantly African American (70.6%), with 23.5% White and 5.9% other. No formal comparisons were made, but they only had a small number of total participants (n = 34). Understanding ethnic differences in cbDNA profiles would provide valuable insights into how genetic and environmental interactions within the gut-heart axis influence CVD, and future studies should aim to include a wide range of ethnic populations in order to ensure any findings are widely applicable.
9 Discussion
Currently, there are very few studies investigating cbDNA in CVD. Each study utilizes different methodologies and sample types across ethnically diverse populations with varying CVD profiles, making comparisons difficult. As such, there is no consensus as to abundance, diversity, origin and specific bacterial signatures when comparing CVD patients to healthy individuals. The use of PCR to assess cbDNA, particularly without adequate controls, is not a gold standard method of analyzing the microbiome and studies using this method are not reliable. Further, the accuracy of 16S rRNA amplicon sequencing in plasma remains to be determined. cbDNA in plasma is highly fragmented and 16S rRNA primer binding sites may not be available. This will vastly underestimate bacterial DNA abundance and potentially the diversity in a sample. Future studies should employ DNA sequencing techniques to assess cbDNA in the plasma by either whole metagenomic or direct sequencing. There is an accumulating amount of research showing that a major source of cbDNA in the circulation is arising from leaky gut. Future research should identify the source of the cbDNA and the pathological relevance. While there is potential in investigating cbDNA as a biomarker in CVD, more research using large standardized cohorts and methodologies needs to be performed.
Author contributions
SA: Writing – original draft, Writing – review & editing. RP: Writing – original draft, Writing – review & editing.
Funding
The author(s) declare that financial support was received for the research and/or publication of this article. This work was supported by the Health Research Council of New Zealand (21/786).
Conflict of interest
The authors declare that the research was conducted in the absence of any commercial or financial relationships that could be construed as a potential conflict of interest.
Publisher's note
All claims expressed in this article are solely those of the authors and do not necessarily represent those of their affiliated organizations, or those of the publisher, the editors and the reviewers. Any product that may be evaluated in this article, or claim that may be made by its manufacturer, is not guaranteed or endorsed by the publisher.
References
1. Vaduganathan M, Mensah GA, Turco JV, Fuster V, Roth GA. The global burden of cardiovascular diseases and risk. JACC. (2022) 80(25):2361–71. doi: 10.1016/j.jacc.2022.11.005
2. Bronkhorst AJ, Ungerer V, Holdenrieder S. The emerging role of cell-free DNA as a molecular marker for cancer management. Biomol Detect Quantif. (2019) 17:100087. doi: 10.1016/j.bdq.2019.100087
3. Zemmour H, Planer D, Magenheim J, Moss J, Neiman D, Gilon D, et al. Non-invasive detection of human cardiomyocyte death using methylation patterns of circulating DNA. Nat Commun. (2018) 9(1):1443. doi: 10.1038/s41467-018-03961-y
4. Jylhävä J, Lehtimäki T, Jula A, Moilanen L, Kesäniemi YA, Nieminen MS, et al. Circulating cell-free DNA is associated with cardiometabolic risk factors: the health 2000 survey. Atherosclerosis. (2014) 233(1):268–71. doi: 10.1016/j.atherosclerosis.2013.12.022
5. Kazemian N, Mahmoudi M, Halperin F, Wu JC, Pakpour S. Gut microbiota and cardiovascular disease: opportunities and challenges. Microbiome. (2020) 8(1):36. doi: 10.1186/s40168-020-00821-0
6. Turnbaugh PJ, Ley RE, Hamady M, Fraser-Liggett CM, Knight R, Gordon JI. The human microbiome project. Nature. (2007) 449(7164):804–10. doi: 10.1038/nature06244
7. Wilkins LJ, Monga M, Miller AW. Defining dysbiosis for a cluster of chronic diseases. Sci Rep. (2019) 9(1):12918. doi: 10.1038/s41598-019-49452-y
8. Wang Z, Klipfell E, Bennett BJ, Koeth R, Levison BS, DuGar B, et al. Gut flora metabolism of phosphatidylcholine promotes cardiovascular disease. Nature. (2011) 472(7341):57–63. doi: 10.1038/nature09922
9. De Vlaminck I, Martin L, Kertesz M, Patel K, Kowarsky M, Strehl C, et al. Noninvasive monitoring of infection and rejection after lung transplantation. PNAS. (2015) 112(43):13336–41. doi: 10.1073/pnas.1517494112
10. Grumaz S, Stevens P, Grumaz C, Decker SO, Weigand MA, Hofer S, et al. Next-generation sequencing diagnostics of bacteremia in septic patients. Genome Med. (2016) 8(1):73. doi: 10.1186/s13073-016-0326-8
11. Glyn T, Purcell R. Circulating bacterial DNA: a new paradigm for cancer diagnostics. Front Med. (2022) 9:831096. doi: 10.3389/fmed.2022.831096
12. Nikkari S, McLaughlin IJ, Bi W, Dodge DE, Relman DA. Does blood of healthy subjects contain bacterial ribosomal DNA? J Clin Microbiol. (2001) 39(5):1956–9. doi: 10.1128/JCM.39.5.1956-1959.2001
13. Chakaroun RM, Massier L, Heintz-Buschart A, Said N, Fallmann J, Crane A, et al. Circulating bacterial signature is linked to metabolic disease and shifts with metabolic alleviation after bariatric surgery. Genome Med. (2021) 13(1):105. doi: 10.1186/s13073-021-00919-6
14. Lelouvier B, Servant F, Païssé S, Brunet AC, Benyahya S, Serino M, et al. Changes in blood microbiota profiles associated with liver fibrosis in obese patients: a pilot analysis. Hepatology. (2016) 64(6):2015–27. doi: 10.1002/hep.28829
15. Chopra A, Franco-Duarte R, Rajagopal A, Choowong P, Soares P, Rito T, et al. Exploring the presence of oral bacteria in non-oral sites of patients with cardiovascular diseases using whole metagenomic data. Sci Rep. (2024) 14(1):1476. doi: 10.1038/s41598-023-50891-x
16. Brenchley JM, Douek DC. Microbial translocation across the GI tract. Annu Rev Immunol. (2012) 30:149–73. doi: 10.1146/annurev-immunol-020711-075001
17. Arseneault-Bréard J, Rondeau I, Gilbert K, Girard SA, Tompkins TA, Godbout R, et al. Combination of Lactobacillus helveticus R0052 and Bifidobacterium longum R0175 reduces post-myocardial infarction depression symptoms and restores intestinal permeability in a rat model. Br J Nutr. (2012) 107(12):1793–9. doi: 10.1017/S0007114511005137
18. Zhou X, Li J, Guo J, Geng B, Ji W, Zhao Q, et al. Gut-dependent microbial translocation induces inflammation and cardiovascular events after ST-elevation myocardial infarction. Microbiome. (2018) 6(1):1–17. doi: 10.1186/s40168-017-0383-2
19. Arakawa K, Ishigami T, Nakai-Sugiyama M, Chen L, Doi H, Kino T, et al. Lubiprostone as a potential therapeutic agent to improve intestinal permeability and prevent the development of atherosclerosis in apolipoprotein E-deficient mice. PLoS One. (2019) 14(6):e0218096. doi: 10.1371/journal.pone.0218096
20. Sandek A, Bauditz J, Swidsinski A, Buhner S, Weber-Eibel J, von Haehling S, et al. Altered intestinal function in patients with chronic heart failure. J Am Coll Cardiol. (2007) 50(16):1561–9. doi: 10.1016/j.jacc.2007.07.016
21. Li C, Gao M, Zhang W, Chen C, Zhou F, Hu Z, et al. Zonulin regulates intestinal permeability and facilitates enteric Bacteria permeation in coronary artery disease. Sci Rep. (2016) 6:29142. doi: 10.1038/srep29142
22. Sanchez-Alcoholado L, Castellano-Castillo D, Jordán-Martínez L, Moreno-Indias I, Cardila-Cruz P, Elena D, et al. Role of gut microbiota on cardio-metabolic parameters and immunity in coronary artery disease patients with and without type-2 diabetes mellitus. Front Microbiol. (2017) 8:1936. doi: 10.3389/fmicb.2017.01936
23. Boman J, Söderberg S, Forsberg J, Birgander LS, Allard A, Persson K, et al. High prevalence of Chlamydia pneumoniae DNA in peripheral blood mononuclear cells in patients with cardiovascular disease and in middle-aged blood donors. J Infect Dis. (1998) 178(1):274–7. doi: 10.1086/517452
24. Wong Y-k, Dawkins KD, Ward ME. Circulating chlamydia pneumoniaeDNA as a predictor of coronary artery disease. J Am Coll Cardiol. (1999) 34(5):1435–9. doi: 10.1016/S0735-1097(99)00391-5
25. Amar J, Lange C, Payros G, Garret C, Chabo C, Lantieri O, et al. Blood microbiota dysbiosis is associated with the onset of cardiovascular events in a large general population: the D.E.S.I.R. Study. PLoS One. (2013) 8(1):e54461. doi: 10.1371/journal.pone.0054461
26. Rajendhran J, Shankar M, Dinakaran V, Rathinavel A, Gunasekaran P. Contrasting circulating microbiome in cardiovascular disease patients and healthy individuals. Int J Cardiol. (2013) 168(5):5118–20. doi: 10.1016/j.ijcard.2013.07.232
27. Amar J, Lelouvier B, Servant F, Lluch J, Burcelin R, Bongard V, et al. Blood microbiota modification after myocardial infarction depends upon low-density lipoprotein cholesterol levels. J Am Heart Assoc. (2019) 8(19):e011797. doi: 10.1161/JAHA.118.011797
28. Dinakaran V, Rathinavel A, Pushpanathan M, Sivakumar R, Gunasekaran P, Rajendhran J. Elevated levels of circulating DNA in cardiovascular disease patients: metagenomic profiling of microbiome in the circulation. PLoS One. (2014) 9(8):e105221. doi: 10.1371/journal.pone.0105221
29. Sumida K, Pierre JF, Han Z, Mims TS, Potukuchi PK, Yuzefpolskaya M, et al. Circulating microbial signatures and cardiovascular death in patients with ESRD. Kidney Int Rep. (2021) 6(10):2617–28. doi: 10.1016/j.ekir.2021.07.023
30. Szeto C-C, Kwan BC-H, Chow K-M, Kwok JS-S, Lai K-B, Cheng PM-S, et al. Circulating bacterial-derived DNA fragment level is a strong predictor of cardiovascular disease in peritoneal dialysis patients. PLoS One. (2015) 10(5):e0125162. doi: 10.1371/journal.pone.0125162
31. Silver RM, Myatt L, Hauth JC, Leveno KJ, Peaceman AM, Ramin SM, et al. Cell-free total and fetal DNA in first trimester maternal serum and subsequent development of preeclampsia. Am J Perinatol. (2017) 34(2):191–8. doi: 10.1055/s-0035-1570383
Keywords: circulating bacterial DNA, cbDNA, cell-free DNA, cardiovascular disease, biomarker, liquid biopsy
Citation: Appleby S and Purcell R (2025) Circulating bacterial DNA in cardiovascular disease. Front. Cardiovasc. Med. 12:1476165. doi: 10.3389/fcvm.2025.1476165
Received: 6 August 2024; Accepted: 1 April 2025;
Published: 15 April 2025.
Edited by:
Dexter Canoy, Newcastle University, United KingdomReviewed by:
Dragos Cretoiu, Carol Davila University of Medicine and Pharmacy, RomaniaCopyright: © 2025 Appleby and Purcell. This is an open-access article distributed under the terms of the Creative Commons Attribution License (CC BY). The use, distribution or reproduction in other forums is permitted, provided the original author(s) and the copyright owner(s) are credited and that the original publication in this journal is cited, in accordance with accepted academic practice. No use, distribution or reproduction is permitted which does not comply with these terms.
*Correspondence: Sarah Appleby, c2FyYWguYXBwbGVieUBvdGFnby5hYy5ueg==