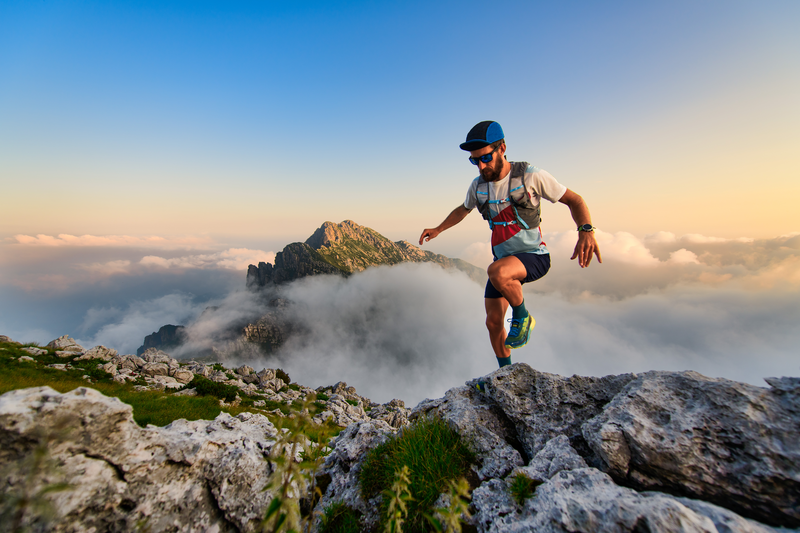
94% of researchers rate our articles as excellent or good
Learn more about the work of our research integrity team to safeguard the quality of each article we publish.
Find out more
SYSTEMATIC REVIEW article
Front. Cardiovasc. Med.
Sec. Coronary Artery Disease
Volume 12 - 2025 | doi: 10.3389/fcvm.2025.1452006
The final, formatted version of the article will be published soon.
You have multiple emails registered with Frontiers:
Please enter your email address:
If you already have an account, please login
You don't have a Frontiers account ? You can register here
Objective: The influence of extreme sleep duration on coronary heart disease (CHD) risk across genders remains a debated topic.Methods: This analysis gathers observational studies that explore association between varying sleep durations and CHD risks. Trend estimation employs generalized least squares, converting specific category risk estimates into relative risks (RR) per hour of sleep increase. A two-stage hierarchical regression model evaluates potential linear dose-response relationships. Data analysis utilizes random-effects restricted cubic spline models with four knots.Results: Involving 17 studies and 906,908 participants, this meta-analysis identifies a pronounced U-shaped nonlinear relationship between sleep duration and CHD risk applicable to both genders (P < 0.01). Notably, shorter sleep durations are linked to higher CHD risks in women, whereas longer durations are more consequential for men. The optimal sleep duration for minimizing CHD risk is between 7.0-8.0 hours daily for men and 7.5-8.5 hours for women.Conclusion: The influence of sleep duration on CHD risk differs significantly between genders.
Keywords: extreme sleep duration, coronary heart disease, gender, BMI, continent
Received: 20 Jun 2024; Accepted: 07 Mar 2025.
Copyright: © 2025 Li, Luo, Liang, Song and Fu. This is an open-access article distributed under the terms of the Creative Commons Attribution License (CC BY). The use, distribution or reproduction in other forums is permitted, provided the original author(s) or licensor are credited and that the original publication in this journal is cited, in accordance with accepted academic practice. No use, distribution or reproduction is permitted which does not comply with these terms.
* Correspondence:
Jin-Xiao Fu, affiliated hospital of Yunnan University, Kunming, China
Disclaimer: All claims expressed in this article are solely those of the authors and do not necessarily represent those of their affiliated organizations, or those of the publisher, the editors and the reviewers. Any product that may be evaluated in this article or claim that may be made by its manufacturer is not guaranteed or endorsed by the publisher.
Research integrity at Frontiers
Learn more about the work of our research integrity team to safeguard the quality of each article we publish.