- 1Department of Acupuncture, Bao’an Authentic TCM Therapy Hospital, Shenzheng, China
- 2Graduate School, Jiangxi University of Chinese Medicine, Nanchang, China
- 3Fever Clinic, The 334 Affiliated Hospital of Nanchang University, Nanchang, China
Myocardial fibrosis (MF) is a common pathological manifestation of many cardiovascular diseases, such as myocardial infarction, myocardial ischemia, and sudden cardiac death. It is characterized by excessive proliferation and activation of fibroblasts, transformation into myofibroblasts, and, eventually, excessive deposition of the extracellular matrix, resulting in heart damage. Currently, modern drugs such as angiotensin-converting enzyme inhibitors, diuretics, and β-blockers can improve myocardial fibrosis in clinical treatment, but their therapeutic effect on this disease is limited, with obvious side effects and high cost. Traditional Chinese medicine (TCM) has the advantages of multiple targets, low cost, and few side effects. Traditional Chinese medicines, such as Salvia miltiorrhiza, Astragalus, and Angelica extracts, and patent Chinese medicines, such as Qiliqiangxin capsules, Shenqi Yiqi dropping pills, and Tongxinluo capsules, can improve myocardial fibrosis. In this review, current Chinese and Western medicine methods for treating myocardial fibrosis are discussed. The signaling pathways and targets of Chinese and Western medicine are involved in the treatment of myocardial fibrosis. This review aimed to provide valuable insights and ideas for both clinical treatment and basic research on myocardial fibrosis.
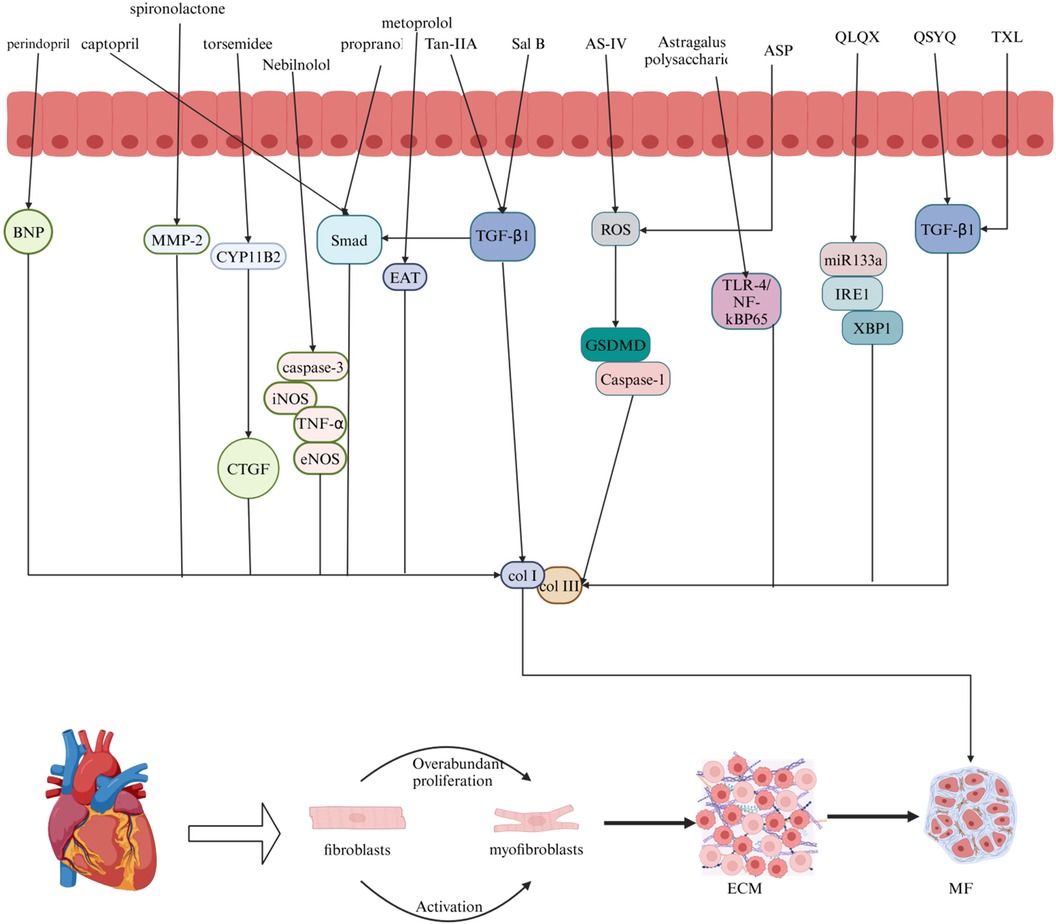
Graphical Abstract.
Figure 1 Graphical summary (Figure 1 created with Biorender.com).
1 Introduction
MF frequently appears as a pathological feature in a range of cardiovascular conditions, such as myocardial infarction, myocardial ischemia, and unexpected cardiac death (1). Following myocardial infarction, the accumulation of collagen I (Col I) and collagen III (Col III) leads to increased MF and the formation of non-contractile scar tissue. Additionally, myofibroblasts in the infarction scar contribute to excessive extracellular matrix (ECM) deposition, resulting in cardiac damage and eventual remodeling (2). When myocardial ischemia occurs, inflammatory cells activate the transforming growth factor-β receptor 1 (TGF-β1) pathway, leading to an increase in matrix protein accumulation and worsening of MF (2–4). In cases of sudden cardiac death, thickening of Col I and Col III within the heart tissue is observed, which leads to myocardial fibrosis and increased cardiac stiffness (5). Cardiovascular diseases affect millions of people globally, imposing a significant economic burden and ranking as a leading cause of human mortality (6). The main pathological characteristics of MF include the transformation and activation of cardiac fibroblasts (CFs) into myofibroblasts, the accumulation of excessive collagen, increased stiffness of the ECM, scar tissue formation, and structural and functional alterations in the heart, which ultimately lead to reduced cardiac function (7, 8). The mechanism of MF is intricate, with rapid onset and high mortality rates (9). Modern medicine offers limited therapeutic options for this disease with high treatment costs (1). As an alternative, traditional Chinese medicine is known for its multiple targets, minimal side effects, and low cost (10). Given the extensive research on MF treatment in traditional Chinese medicine, this article aims to review and summarize current advancements to serve as a foundation for future clinical and experimental studies (11).
When traditional Chinese medicinal materials are utilized for disease treatment, they are often subjected to multiple rounds of steaming and drying to enhance their therapeutic properties and mitigate potential toxicities and side effects (12). TCM has demonstrated promising outcomes, with a rich history of treating cardiovascular diseases (13, 14). Recent studies have increasingly indicated that traditional Chinese medicine could open novel avenues for MF treatment research (7). Owing to its diverse composition, ability to target multiple sites, and minimal adverse effects, traditional Chinese medicine allows its various chemical constituents to follow distinct therapeutic pathways, reaching multiple targets to address MF effectively (15). Nevertheless, the precise therapeutic mechanisms of traditional Chinese medicine remain unclear (16). Currently, most traditional Chinese medicine interventions are at the stage of animal experimentation, with limited clinical trials and reliance on singular research methodologies, resulting in a dearth of exploration of molecular mechanisms (17). Given the variability in patient symptoms, individualized syndrome differentiation and treatment are necessary, highlighting the lack of a standardized syndrome differentiation system (7). Furthermore, improvements in production techniques, processing methods, and environmental quality are needed to increase the efficacy of traditional Chinese medicine (18).
This article provides a summary of representative drugs and their mechanisms of action, serving as a reference for future research and experiments on MF treatment.
Contemporary medicine is primarily divided into ACE inhibitors, diuretics, and beta blockers. Examples of ACE inhibitors are perindopril and captopril; examples of diuretics are spironolactone and torsemide; and common beta-blocker medications include metoprolol and propranolol. Salvia miltiorrhiza, Astragalus membranaceus, and Angelica sinensis are the three main categories of traditional Chinese medicine. The active substances extracted from Salvia miltiorrhiza include tanshinone IIA and salvianolic acid B. Astragalus, which contain active substances such as astragaloside IV and total astragalus saponins. Angelica is known for its medicinal properties, and Angelica and Angelica polysaccharides are commonly used for its treatment.
2 Modern medicine
2.1 Angiotensin-converting enzyme inhibitors (ACEI)
Angiotensin II is a crucial factor in MF development. Increased angiotensin II activity can result in myocardial cell deficiency, hypertrophy, and inflammation, ultimately leading to myocardial fibrosis and cardiac remodeling (19, 20). Research has demonstrated that ACEIs can reduce myocardial fibrosis by blocking the generation of angiotensin II (21). Perindopril, a third-generation ACE inhibitor, has been extensively studied for its ability to reduce angiotensin I (ATI) activity by inhibiting ACE, thereby slowing the conversion of angiotensin II (ATII) (22, 23). By lowering the collagen volume fraction (CVF) and decreasing the protein levels of Col I and Col III, perindopril effectively decreased myocardial fibrosis in a rat model of diabetic cardiomyopathy (24). In a rat model of heart failure, perindopril was shown to decrease the levels of brain natriuretic peptide (BNP), COL I, and COL III, improve inflammatory cell infiltration, reduce collagen fibers, and ameliorate MF (25). In contrast, captopril, the most widely used ACE inhibitor, blocks the renin‒angiotensin system and prevents the conversion of ATI into ATII (26–29). In MI models, captopril has been shown to prevent the transformation of cardiac fibroblasts via the TGF-β1/Smad3 pathway. This action results in decreased collagen accumulation, enhanced extracellular matrix (ECM), and improved myocardial function (30). Captopril reduces ATII levels, thereby slowing the development of fibrous tissue and inhibiting collagen build-up, which ultimately results in an improvement in myocardial fibrosis (31).
The therapeutic target of ACE inhibitors is the cardiovascular system (32). However, they may lead to side effects such as hypotension (33), hyperkalemia (34), intestinal angioedema (35), and angioedema (36). However, the specific mechanism by which perindopril improves MF remains unclear (37).
2.2 Diuretics
Aldosterone, which is generated in the adrenal gland, significantly contributes to the progression of MF (19, 38). The activation of both the mineralocorticoid receptor (MR) and glucocorticoid receptor (GR) can induce MF and promote the differentiation of cardiac fibroblasts. Furthermore, aldosterone indirectly plays a role in the progression of MF by amplifying cardiomyocyte inflammation and inhibiting the expression of antifibrotic factors (39). Spironolactone, which acts as a mineralocorticoid receptor (MR) antagonist, reduces matrix metallopeptidase-2 (MMP-2), inhibits collagen production, and improves MF, ultimately reducing cardiac pre- and post-load and protecting the heart (40–43). Spironolactone has been demonstrated to reduce COL-I levels and collagen deposition in individuals with heart failure and preserved ejection fraction (HFpEF), resulting in the inhibition of MF (44). In contrast, torsemide, a widely used potent loop diuretic, inhibits aldosterone synthase (CYP11B2), reduces connective tissue growth factor (CTGF), and inhibits collagen accumulation, ultimately improving MF (45, 46). In rat models of heart failure, torsemide has been shown to upregulate gap junction proteins, enhance cardiomyocyte interactions, decrease myocardial collagen accumulation, improve MF, and prevent cardiac remodeling (47, 48).
Diuretics increase water and sodium excretion in the body, reduce fluid load, and improve the clinical symptoms of various diseases (49). However, they can lead to electrolyte disorders such as hyponatremia, hypokalemia, hyperkalemia, hypomagnesemia, and hyperuricemia (50). Spironolactone may have anti-androgenic side effects, but its mechanism of treating MF remains unclear (51, 52). Torsemide, which has poor water solubility, lacks a clear mechanism for MF (47, 53).
2.3 Beta-blockers
β-adrenergic receptors (β-ARs) can initially preserve cardiac function, but prolonged stimulation leads to the activation of cardiac fibroblasts, resulting in collagen accumulation and eventual MF (54). β-blockers are essential drugs for the treatment of cardiovascular diseases. In acute psychological failure, β-blockers can slow the resting heart rate, increase the filling pressure, and improve the survival rate of patients. In chronic heart failure, left ventricular function can be improved, thereby reducing the morbidity and mortality of patients (55–57). β-blockers can prevent renin‒angiotensin‒aldosterone system activation, sympathetic nerve activation, oxidative stress, inflammation, and other potential cardiac hazards, reduce myocardial fibrosis, improve myocardial pathological status, and prevent myocardial remodeling (58). Metoprolol, a beta-blocker, inhibits beta-adrenergic energy and reduces the levels of fibrotic adipocytokines produced by atrial adipose tissue (EAT). This inhibition suppresses cardiac fibroblast activity, decreases collagen accumulation, and improves MF (59, 60). Metoprolol decreased myocardial collagen deposition and alleviated MF (61). Propranolol, a non-selective beta-blocker, inhibits beta-adrenergic receptors, thereby neutralizing the effects of epinephrine and norepinephrine (62, 63). Propranolol also decreases fibroblast growth factor 23 (FGF-23) activity, inhibits myofibroblast function, reduces collagen accumulation, and ameliorates MF (64, 65). Propranolol reduces collagen build-up and enhances MF by regulating the TGF-β1/Smad signaling pathway (66). Nebilolol acts as a selective beta-1 adrenergic blocker and has beneficial effects on the central and peripheral vascular systems (67, 68). Nebiprolol reduces the collagen fiber area and alleviates MF by regulating caspase-3, eNOS, iNOS, and TNF-α (69).
The prognosis is poor when beta-blockers are administered to elderly patients with preserved ejection fraction heart failure (HFpEF) (70). Individuals with diabetes are more likely to experience adverse events while taking beta blockers (71). However, the precise mechanism of action of metoprolol in MF remains uncertain (58). Although propranolol is generally considered safe, it may lead to side effects, including hypoglycemia, hypotension, bradycardia, bronchospasm, and impairment of cardiovascular or respiratory function (72). Nebilolol may be associated with adverse drug events (73).
2.4 Other modern medicines
Other drugs, such as empagliflozin and atorvastatin, have been shown to be effective in treating MF (74). Empagliflozin reduces reactive oxygen species (ROS), decreases myocardial oxidative stress, and improves MF (75). Similarly, atorvastatin reduces myofibroblast content and MF by inhibiting oxidative stress (76).
2.5 Combined treatment
The combined use of drugs has a greater impact than the use of a single drug (77). When an angiotensin receptor neprilysin inhibitor (ARNI) is used in conjunction with an ACEI, it diminishes myocardial fibrosis by reducing TGF-β1 expression (78). Furthermore, the concurrent use of ivabradine HCl and trimetazidine decreased TGF-β1 and COL-L levels, resulting in decreased myocardial fibrosis (79) (Table 1).
3 Chinese medicine
3.1 Salvia miltiorrhiza
Salvia miltiorrhiza, a plant first documented in “Shenlong Materia Medica”, belongs to the Sage family of Lamiaceae (80). In China, Salvia miltiorrhiza is mainly used to treat angina pectoris, hyperlipidemia, and coronary heart disease and can also enhance human immunity (81). Tanshinone IIA (Tan-IIA) is a lipophilic active component of Salvia miltiorrhiza that inhibits fibrosis (82, 83). Tan-IIA inhibits fibroblast proliferation, reduces COL I and COL III accumulation, and mitigates MF (84, 85). In patients experiencing MI, Tan-IIA opposes the impact of TGF-β1 on heart fibroblasts, resulting in reduced concentrations of COL I and COL III and the mitigation of MF (86). Salvianolic acid B (Sal B), the main bioactive component of salvianolic acid, has the chemical formula C36H30O16 (87). It has been shown to be effective in suppressing fibroblast growth, lowering the levels of COL I and COL III, and improving fibrosis (88, 89). Sal B inhibits cardiac fibroblast (CF) growth, decreases collagen accumulation, and improves MF in diabetic mice by regulating TGF-β1/Smad7 expression (90). Danshen extract can significantly reduce the biochemical indices of patients with CHD, reduce the incidence of CHD, and thus protect the heart (91).
Tan IIA has a slow dissolution rate and low bioavailability, which hinders its clinical utility (85). Although its mechanism of action in treating MF remains incompletely understood (92), salvianolic acid is recognized as the most crucial active monomer component of Salvia miltiorrhiza. However, it targets only a single therapeutic pathway and does not align with the “holistic concept” in traditional Chinese medicine (93). Sal B is the most water-soluble active ingredient in Salvia miltiorrhiza; however, the precise mechanism for preventing and treating MF remains unclear (94).
3.2 Astragalus membranaceus
Astragalus membranaceus (AR) is a dry root obtained from the leguminous plant Bge. var. Mongolicus (Bge.) Hisao, and Astragalus membranaceus (Fisch.) Bge (95). AR is often used to regulate human immunity and cardiovascular diseases (96). It contains saponins, flavonoids, isoflavones, glycosides, flavonoids, polysaccharides, rosewood, and other active ingredients (97). Methyl glycosides, total saponins, and polysaccharides have been shown to effectively inhibit myocardial fibrosis (98). Astragaloside IV (AS-IV) is the main active ingredient (99). Astragaloside can reduce collagen I and III, inhibit oxidative stress and the p53 signaling pathway, and reduce MF (100). Moreover, AS-IV can reduce the content of COL-I and COL-III, collagen accumulation, and MI by reducing the activity of the ROS/caspase-1/GSDMD signaling pathway (101). Astragalus total saponin (ATS), the basic bioactive substance of astragalus, can reduce collagen deposition and MF by inhibiting the expression of tumor necrosis factor α and Fas ligands (102, 103). Astragalus polysaccharide is a water-soluble heteropolysaccharide (104). Astragalus polysaccharide can counteract myocardial injury, regulate the TLR-4/NF-kBp65 signaling pathway, reduce the inflammatory response, and improve MF (105). Astragalus injections are used in patients with coronary heart disease to reduce cardiovascular risk factors and protect the heart (106).
After oral administration of AS-IV, its bioavailability is relatively low, restricting its usefulness in clinical settings. Additional investigations are needed to improve the MF (101, 107, 108). The biological mechanism of astragaloside IV (AST) in MF treatment remains unclear (103). Clinical trials have only been conducted in China and have not been conducted outside the country (106).
3.3 Angelica sinensis
Initially, reported in “Shenlong Materia Medica”, Angelica sinensis is effective in treating cardiovascular diseases (109). Current pharmacological research has indicated that Angelica sinensis comprises a range of active constituents, such as phthalates, monoterpenes, sesquiterpenes, aromatic compounds, aliphatic hydrocarbons, derivatives, polysaccharides, and organic acids. Polysaccharides have demonstrated promising efficacy in the treatment of fibrosis (110). In an x-ray-induced MF rat model, the P13K/AKT/mTOR pathway reduced the accumulation of collagen fibers, lowered the content of COL-I and COL-III, and mitigated MF (111). Furthermore, in a study involving a rat model of myocardial infarction, Angelica sinensis inhibited macrophage proliferation, decreased TGF-β1 expression, prevented collagen deposition, and reduced myocardial fibrosis (112). Furthermore, in a hypertensive rat model, Angelica polysaccharide (ASP) mitigated MF by reducing oxidative stress, decreasing reactive oxygen species (ROS) accumulation, inhibiting cardiac fibroblast proliferation, and reducing collagen fiber accumulation (113). ASP inhibits ROS production in a dose-dependent manner, thereby reducing oxidative stress and alleviating MF (114).
Angelica sinensis, a Chinese herbal medicine, is commonly incorporated into formulas to increase medicinal efficacy (115). However, the specific mechanism by which Angelica sinensis treats MF remains unclear (111). Although ASP has significant cardioprotective properties, its specific mechanism for treating MF warrants further investigation (113, 116).
3.4 Other traditional Chinese medicines
Chinese medicines such as puerarin (117), triptolide (118) and ginsenoside (119) can also treat MF. Puerarin hinders the activity of heart fibroblasts, reduces the levels of COL-I and COL-III, and alleviates myocardial fibrosis by adjusting the HMGB1/TLR4-NF-kB pathway (120). Triptolide, the active compound found in Tripterygium wilfordii, decreases the number of collagen fibers, specifically COL-I and COL-III fibers, by inhibiting the Wnt/β-catenin pathway (β-catenin/c-myc/Cyclin D1). This leads to a reduction in cardiac fibroblast differentiation and alleviates myocardial fibrosis (121). The active compound RH4 in ginsenosides diminishes COL-I and COL-III content, decreases collagen accumulation, and alleviates MF by inhibiting the STAT3 and p38/MAPK signaling pathways (122) (Table 2).
4 Proprietary Chinese medicine
4.1 Qiliqiangxin capsule (QLQX)
Qiliqiangxin capsule, a Chinese herbal compound, is extracted from 11 different Chinese herbs, including astragalus and ginseng (123, 124). It is included in the Pharmacopoeia of the People's Republic of China and is commonly used to treat chronic heart failure (CHF) (125, 126). In a rat model of heart failure, QLQX reduced the collagen content in myocardial tissue by regulating the miR133a-endoplasmic reticulum stress-inositol-requiring enzyme 1/X-box binding protein 1 (miR133a-IRE1/XBP1) pathway (127). In a rat model of myocardial infarction, QLQX reduced type II and III collagen content, regulated collagen homeostasis, improved cardiac function, and alleviated MF (126). QLQX improves clinical symptoms and protects cardiac function in patients with chronic heart failure (123). QLQX can protect the heart by improving the clinical symptoms of patients with chronic heart failure and the levels of 6-min walking distance (6-MWD), brain natriuretic peptide (BNP), and N-terminal brain natriuretic peptide precursor (NT-proBNP) (128).
QLQX can reduce fibrosis; however, further studies and clinical trials are needed to support these findings (129). QLQX has not been fully explored for signaling pathways related to ventricular remodeling, and more high-quality RCTs are needed to improve the credibility of the evidence (128).
4.2 Shenqi yiqi dropping pills (QSYQ)
QSYQ is a traditional Chinese medicine (TCM). It is formed by Astragalus membranaceus Fisch. ex Bunge, Salvia miltiorrhiza Bge., Panax notoginseng (Burk.) F. H. Chen and Dalbergia odorifera T. Chen, which can be used to treat various heart diseases (130, 131). QSYQ can inhibit cardiomyocyte apoptosis, reduce type I and III collagen content, improve myocardial collagen metabolism, and reduce MF (132). QSYQ can inhibit TGF-β1, reduce type I and type III collagen, relieve myocardial collagen, and improve MF (133). QSYQ reduces extracellular matrix deposition and improves MF by regulating TGF-β1 (134). In clinical trials, QSYQ was shown to regulate the 6-minute walking distance, BNP level, and left ventricular ejection fraction in patients with ischemic heart failure (IHF), protect heart function, and improve patients’ quality of life (135). The data collected by Meta revealed that QSYQ can improve the clinical symptoms of heart failure patients with preserved ejection fraction (HFpEF), increase the 6-minute walking distance, reduce BNP, and achieve cardiac protection (136).
Although there are many pathways for the treatment of MF via QSYQ, the underlying mechanism of action requires further elucidation (132). In randomized controlled trials, the application of the QSYQ in traditional Chinese dialectical thinking has limitations (135). There is a lack of large-scale, multi-center, randomized, double-blind, and high-quality studies (137).
4.3 Tongxinluo capsule (TXL)
Tongxinluo capsules constitute an innovative Chinese medicine composed of 12 types of Chinese medicines, such as ginseng (138). TXL is often used to treat angina pectoris in patients with coronary heart disease (139). TXL can improve MF in the following four ways: (1) it inhibits the transition of endothelial cells to mesenchymal cells (EndMTs), activates the neuregulin-1/epithelial growth factor receptor 4-protein kinase B/protein kinase B (NRG-1/ErbB-PI3K/AKT) signaling pathway, inhibits type I and III collagen, reduces extracellular matrix deposition, and alleviates MF (140). (2) The PI3K/AKT signaling pathway is activated to reduce MF (141). (3) Inhibiting the TGF-β1 pathway, reducing collagen fiber accumulation, and improving MF (142); (4) Stress on the ventricular wall related to the MF should be reduced, the MF should be improved, the myocardium should be protected, and myocardial ischemia should be improved (143). In chronic coronary syndrome (CCS), TXL can effectively improve clinical symptoms and protect the heart (144).
The mechanism of action of TXL in improving MF is unclear, and further experimental studies are needed to determine whether it is accomplished by a single component or multiple compounds (142). Most clinical trial data on TXL are from China, and high-quality, large-scale, multi-center, and randomized controlled clinical trials are lacking (145).
4.4 Other proprietary Chinese medicines
Wenxin granules, Yixinshu capsules (YXS), Qifu yixin prescription (QFYX), and other proprietary Chinese medicines can also improve MF. Wenxin granules can improve MF, ventricular remodeling, and cardiac function by regulating the unfolded protein response (146). YXS regulates the retinoblastoma/histone deacetylase 1/GATA-binding protein 4 (RB/HDAC1/GATA4) pathway, improves MF, and restores cardiac function (147). QFYX improves MF and inhibits myocardial hypertrophy through the β-arrestin2 (β-arr2) pathway (Table 3) (148).
5 Conclusions and prospects
MF is the pathological basis of most cardiovascular diseases and is often closely related to myocardial infarction, myocardial ischemia, sudden cardiac death, and other cardiovascular diseases (149). Modern medicine is effective and fast, but it has potential side effects, such as hypotension and hyperkalemia (33, 34, 150). TCM can be used to treat patients according to their clinical symptoms and improve their quality of life. Owing to the advantages of multiple components, multiple approaches, and multiple targets, Chinese medicine has made progress in the study of MF; however, it also has limitations (151). For example, the cell model lacks a complex microenvironment and cannot completely replicate the pathogenesis in vivo; (2) the animal experimental period is long, has a high cost, and species differentiation; (3) Chinese medicine involves a variety of ingredients, making it difficult to clarify their mechanism of action; and (4) there is a lack of unified quality control standards and herbal standardization. In the analysis of TCM clinical trials, most studies were published in Chinese, the subjects were Chinese, and there were no overseas clinical studies.
Although the treatment of MF is challenging, the advantages of traditional Chinese medicine, such as good clinical efficacy, few toxic side effects, and low drug resistance, can become the focus of the treatment of myocardial fibrosis and a new research field. Therefore, TCM treatment of MF has broad research prospects. The promotion of Chinese medical treatment for myocardial fibrosis is more standardized. (1) Depending on the research purpose and experimental conditions, an appropriate method can be chosen to establish animal and cell models. (2) The relevant therapeutic drugs and mechanisms of action of traditional Chinese medicine in the treatment of myocardial fibrosis should be thoroughly and systematically explored, and the signaling pathways and core targets of this medicine should be understood. (3) As Chinese medicine has multiple components and targets, it is necessary to clarify the chemical composition of Chinese medicinal materials and compound preparations and establish a unified drug quality control standard. (4) The sample size should be increased to conduct large-scale, multi-center, randomized, double-blind, and high-quality controlled clinical trials on TCM for the treatment of MF. (5) Due to the popularity of traditional Chinese medicine in Western countries, its effects on populations in other countries should be observed to reduce sample bias. (6) The basic theories of traditional Chinese medicine should be combined with those of Western medicine and modern biological science. New traditional Chinese medicine compounds should be researched, and their clinical application should be actively promoted.
Author contributions
YZhu: Writing – original draft. FZ: Writing – original draft. ZL: Writing – original draft. YZho: Writing – original draft. YS: Writing – review & editing. JR: Writing – review & editing. GC: Writing – review & editing.
Funding
The author(s) declare financial support was received for the research, authorship, and/or publication of this article. This study was funded by the Sanming Project of Medicine in Shenzhen (Project Number: [SZZYSM202311015]).
Acknowledgments
We would like to express our gratitude to Yi Shu, Jian Ruan, and Guo Chen for their contributions in conceptualizing, editing, and finalizing the manuscript. Additionally, we extend our thanks to Yuxi Zhu, Fangmei Zhang, Zhongcheng Li, and Yu Zhou for their efforts in reviewing and revising the manuscript.
Conflict of interest
The authors declare that the research was conducted in the absence of any commercial or financial relationships that could be construed as a potential conflict of interest.
Publisher's note
All claims expressed in this article are solely those of the authors and do not necessarily represent those of their affiliated organizations, or those of the publisher, the editors and the reviewers. Any product that may be evaluated in this article, or claim that may be made by its manufacturer, is not guaranteed or endorsed by the publisher.
References
1. Ren Z, Zhang Z, Ling L, Liu X, Wang X. Drugs for treating myocardial fibrosis. Front Pharmacol. (2023) 14:1221881. doi: 10.3389/fphar.2023.1221881
2. Li F, Chen L, Zhong S, Chen J, Cao Y, Yu H, et al. Collagen-targeting self-assembled nanoprobes for multimodal molecular imaging and quantification of myocardial fibrosis in a rat model of myocardial infarction. ACS nano. (2024) 18(6):4886–902. doi: 10.1021/acsnano.3c09801
3. Flori L, Lazzarini G, Spezzini J, Pirone A, Calderone V, Testai L, et al. The isoproterenol-induced myocardial fibrosis: a biochemical and histological investigation. Biomed Pharmacother. (2024) 174:116534. doi: 10.1016/j.biopha.2024.116534
4. Jiang M, Zhang G, Li L, He Y, Li G, Yu J, et al. Case report: a case report of myocardial fibrosis activation assessment after unstable angina using 68Ga-FAPI-04 PET/CT. Front Cardiovasc Med. (2024) 11:1332307. doi: 10.3389/fcvm.2024.1332307
5. Fnon NF, Hassan HH, Shehata SA, Abdelrahman KM, Ibrahim MA. Endomyocardial fibrosis related sudden cardiac death; two autopsied case-reports from Egypt. Leg Med. (2023) 62:102221. doi: 10.1016/j.legalmed.2023.102221
6. Safdar B, Mangi AA. Survival of the fittest: impact of cardiorespiratory fitness on outcomes in men and women with cardiovascular disease. Clin Ther. (2020) 42(3):385–92. doi: 10.1016/j.clinthera.2020.01.014
7. Ren C, Liu K, Zhao X, Guo H, Luo Y, Chang J, et al. Research progress of traditional Chinese medicine in treatment of myocardial fibrosis. Front Pharmacol. (2022) 13:853289. doi: 10.3389/fphar.2022.853289
8. Wang M, Yan M, Tan L, Zhao X, Liu G, Zhang Z, et al. Non-coding RNAs: targets for Chinese herbal medicine in treating myocardial fibrosis. Front Pharmacol. (2024) 15:1337623. doi: 10.3389/fphar.2024.1337623
9. Scridon A, Balan AI. Targeting myocardial fibrosis—a magic pill in cardiovascular medicine? Pharmaceutics. (2022) 14(8):1599. doi: 10.3390/pharmaceutics14081599
10. Pan Y, Shao C, Zhang L, He Y, Yang J, Fu W, et al. The effect of Guanxin shutong capsule on alleviating the myocardial fibrosis in heart failure rats. J Ethnopharmacol. (2021) 275:114169. doi: 10.1016/j.jep.2021.114169
11. Sun S, Liu F, Fan F, Chen N, Pan X, Wei Z, et al. Exploring the mechanism of atherosclerosis and the intervention of traditional Chinese medicine combined with mesenchymal stem cells based on inflammatory targets. Heliyon. (2023) 9(11):e22005. doi: 10.1016/j.heliyon.2023.e22005
12. Liu J, Zhang Z, Huai X, Wei Y, Zhu J, Li X, et al. Development and application of the new integrated equipment and process of the nine-steam-nine-bask method in the processing of Polygonatum cyrtonema. Processes. (2022) 10(6):1044. doi: 10.3390/pr10061044
13. Liu M, Long X, Xu J, Chen M, Yang H, Guo X, et al. Hypertensive heart disease and myocardial fibrosis: how traditional Chinese medicine can help addressing unmet therapeutical needs. Pharmacol Res. (2022) 185:106515. doi: 10.1016/j.phrs.2022.106515
14. Li L, Ran Y, Wen J, Lu Y, Liu S, Li H, et al. Traditional Chinese medicine-based treatment in cardiovascular disease: potential mechanisms of action. Curr Pharm Biotechnol. (2024) 25(17):2186–99. doi: 10.2174/0113892010279151240116103917
15. Li X, Li L, Lei W, Chua HZ, Li Z, Huang X, et al. Traditional Chinese medicine as a therapeutic option for cardiac fibrosis: pharmacology and mechanisms. Biomed Pharmacother. (2021) 142:111979. doi: 10.1016/j.biopha.2021.111979
16. Zhang Z, Yang Z, Wang S, Wang X, Mao J. Targeting MAPK-ERK/JNK pathway: a potential intervention mechanism of myocardial fibrosis in heart failure. Biomed Pharmacother. (2024) 173:116413. doi: 10.1016/j.biopha.2024.116413
17. Liu C, Guo X, Zhou Y, Wang H. AMPK signalling pathway: a potential strategy for the treatment of heart failure with Chinese medicine. J Inflamm Res. (2023) 2023:5451–64. doi: 10.2147/JIR.S441597
18. Wang Z. Research on the development of Chinese medicine and its impact on the environment. SHS Web of Conferences; EDP Sciences (2022) 144. p. 01005. doi: 10.1051/shsconf/202214401005
19. Ksiazek SH, Hu L, Andò S, Pirklbauer M, Säemann MD, Ruotolo C, et al. Renin–angiotensin–aldosterone system: from history to practice of a secular topic. Int J Mol Sci. (2024) 25(7):4035. doi: 10.3390/ijms25074035
20. Balogh DB, Molnar A, Degi A, Toth A, Lenart L, Saeed A, et al. Cardioprotective and antifibrotic effects of low-dose renin–angiotensin–aldosterone system inhibitors in type 1 diabetic rat model. Int J Mol Sci. (2023) 24(23):17043. doi: 10.3390/ijms242317043
21. Shouji M, Hiroyuki T, Tetuya S, Hidenori M, Ikeuchi M, Keiko K, et al. Angiotensin-converting enzyme inhibitor attenuates myocardial fibrosis in diabetes mellitus by inhibiting connective tissue growth factor. J Card Fail. (2004) 10(5):S186. doi: 10.1016/j.cardfail.2004.08.127
22. Alfakih K, Hall AS. Perindopril. Expert Opin Pharmacother. (2006) 7(1):63–71. doi: 10.1517/14656566.7.1.63
23. Connolly K, Batacan R Jr, Jackson D, Vella R, Fenning A. Perindopril prevents development of obesity and hypertension in middle aged diet-induced obese rat models of metabolic syndrome. Life Sci. (2023) 314:121291. doi: 10.1016/j.lfs.2022.121291
24. Liu M, Ai J, Shuai Z, Tang K, Li Z, Huang Y. Adropin alleviates myocardial fibrosis in diabetic cardiomyopathy rats: a preliminary study. Front Cardiovasc Med. (2021) 8:688586. doi: 10.3389/fcvm.2021.688586
25. Liu Q, Qu HY, Zhou H, Rong JF, Yang TS, Xu JJ, et al. Luhong formula has a cardioprotective effect on left ventricular remodeling in pressure-overloaded rats. Evid Based Complement Alternat Med. (2020) 2020(1):4095967. doi: 10.1155/2020/4095967
26. Vasconcelos DLM, Silva CB, da Silva Filho JG, Façanha-Filho PF, Teixeira AMR, Lima JA Jr, et al. Raman spectra of captopril under high pressure. Vib Spectrosc. (2019) 102:116–24. doi: 10.1016/j.vibspec.2019.05.006
27. Tripathi PK, Singh S, Jadhav KR. Floating minitablets loaded with captopril encapsulated microparticles. J Drug Deliv Sci Technol. (2021) 63:102445. doi: 10.1016/j.jddst.2021.102445
28. Li M, Wang Z, Shao J, Li S, Xia H, Yu L, et al. Captopril attenuates the upregulated connexin 43 expression in artery calcification. Arch Med Res. (2020) 51(3):215–23. doi: 10.1016/j.arcmed.2020.02.002
29. Das U, Wadhwa P, Singh PK, Kalidindi DV, Nagpal K. The role of polymers and excipients for better gastric retention of captopril. Crit Rev Ther Drug Carrier Syst. (2022) 39(6):85–106. doi: 10.1615/CritRevTherDrugCarrierSyst.2022042122
30. Wang M, Wang M, Zhao J, Xu H, Xi Y, Yang H. Dengzhan shengmai capsule attenuates cardiac fibrosis in post-myocardial infarction rats by regulating LTBP2 and TGF-β1/Smad3 pathway. Phytomedicine. (2023) 116:154849. doi: 10.1016/j.phymed.2023.154849
31. Zhang L, Chen D, Peng M, Ma H. Effects of yixintai pills on myocardial cell apoptosis in rats with adriamycin-induced heart failure. Heart Surg Forum. (2020) 23(2):E234–8. doi: 10.1532/hsf.2941
32. Silva-Velasco DL, Cervantes-Pérez LG, Sánchez-Mendoza A. ACE inhibitors and their interaction with systems and molecules involved in metabolism. Heliyon. (2024) 10(2):e24655. doi: 10.1016/j.heliyon.2024.e24655
33. Zheng W, Tian E, Liu Z, Zhou C, Yang P, Tian K, et al. Small molecule angiotensin converting enzyme inhibitors: a medicinal chemistry perspective. Front Pharmacol. (2022) 13:968104. doi: 10.3389/fphar.2022.968104
34. Mae H, Fujimaru T, Shimoyama K, Kadota N, Konishi K, Itou Y, et al. Association of serum sodium minus chloride level at initiation of angiotensin-converting enzyme inhibitors or angiotensin receptor blockers and hyperkalemia in patients with CKD: a case control study. Renal Replacement Ther. (2024) 10(1):24. doi: 10.1186/s41100-024-00541-3
35. Arshad A, Yaqoob H. Angiotensin-converting enzyme inhibitor-induced intestinal angioedema: a rare side effect of a common drug. Chest. (2023) 164(4):A120. doi: 10.1016/j.chest.2023.07.132
36. Davin L, Marechal P, Lancellotti P, Martinez C, Pierard L, Radermecker R. Angioedema: a rare and sometimes delayed side effect of angiotensin-converting enzyme inhibitors. Acta Cardiol. (2019) 74(4):277–81. doi: 10.1080/00015385.2018.1507477
37. Li S, Li S, Hao X, Zhang Y, Deng W. Perindopril and a galectin-3 inhibitor improve ischemic heart failure in rabbits by reducing gal-3 expression and myocardial fibrosis. Front Physiol. (2019) 10:267. doi: 10.3389/fphys.2019.00267
38. Wang NP, Zhang WW, Zheng RH, Bai F, Sturdivant K, James EA, et al. Aldosterone mediates angiotensin II-induced myocardial fibrosis and hypertrophy via steroidogenic acute regulatory protein and aldosterone synthase. FASEB J. (2019) 33(S1):833.5–833.5. doi: 10.1096/fasebj.2019.33.1_supplement.833.5
39. Zhou F, Wu T, Wang W, Cheng W, Wan S, Tian H, et al. CMR-verified myocardial fibrosis is associated with subclinical diastolic dysfunction in primary aldosteronism patients. Front Endocrinol. (2021) 12:672557. doi: 10.3389/fendo.2021.672557
40. Wang WK, Wang B, Cao XH, Liu YS. Spironolactone alleviates myocardial fibrosis via inhibition of ets-1 in mice with experimental autoimmune myocarditis. Exp Ther Med. (2022) 23(6):1–10. doi: 10.3892/etm.2022.11296
41. Kobayashi M, Ferreira JP, Duarte K, Bresso E, Huttin O, Bozec E, et al. Proteomic profiles of left atrial volume and its influence on response to spironolactone: findings from the HOMAGE trial and STANISLAS cohort. Eur J Heart Fail. (2024) 26(5):1231–41. doi: 10.1002/ejhf.3202
42. Sacharczuk W, Dankowski R, Ożegowski S, Rojna M, Szyszka A. Evaluation of early left-sided cardiac reverse remodeling under combined therapy of sacubitril-valsartan and spironolactone compared with angiotensin-converting enzyme inhibitors and spironolactone. Front Cardiovasc Med. (2023) 10:1103688. doi: 10.3389/fcvm.2023.1103688
43. Chen X, Ge W, Dong T, Hu J, Chen L, Fan X, et al. Spironolactone inhibits endothelial-mesenchymal transition via the adenosine A2A receptor to reduce cardiorenal fibrosis in rats. Life Sci. (2019) 224:177–86. doi: 10.1016/j.lfs.2019.01.017
44. Ravassa S, Trippel T, Bach D, Bachran D, González A, López B, et al. Biomarker-based phenotyping of myocardial fibrosis identifies patients with heart failure with preserved ejection fraction resistant to the beneficial effects of spironolactone: results from the aldo-DHF trial. Eur J Heart Fail. (2018) 20(9):1290–9. doi: 10.1002/ejhf.1194
45. Sandré F, Moilleron R, Morin C, Garrigue-Antar L. Comprehensive analysis of a widely pharmaceutical, furosemide, and its degradation products in aquatic systems: occurrence, fate, and ecotoxicity. Environ Pollut. (2024) 348:123799. doi: 10.1016/j.envpol.2024.123799
46. Adam O, Zimmer C, Hanke N, Hartmann RW, Klemmer B, Böhm M, et al. Inhibition of aldosterone synthase (CYP11B2) by torasemide prevents atrial fibrosis and atrial fibrillation in mice. J Mol Cell Cardiol. (2015) 85:140–50. doi: 10.1016/j.yjmcc.2015.05.019
47. López B, González A, Beaumont J, Querejeta R, Larman M, Díez J. Identification of a potential cardiac antifibrotic mechanism of torasemide in patients with chronic heart failure. J Am Coll Cardiol. (2007) 50(9):859–67. doi: 10.1016/j.jacc.2007.04.080
48. Watanabe K, Sreedhar R, Thandavarayan RA, Karuppagounder V, Giridharan VV, Antony S, et al. Comparative effects of torasemide and furosemide on gap junction proteins and cardiac fibrosis in a rat model of dilated cardiomyopathy. Biofactors. (2017) 43(2):187–94. doi: 10.1002/biof.1332
49. Lava SAG, Zollinger C, Chehade H, Schaffner D, Sekarski N, Di Bernardo S. Diuretics in pediatrics. Eur J Pediatr. (2023) 182(5):2077–88. doi: 10.1007/s00431-022-04768-2
50. Sica DA. Diuretic-related side effects: development and treatment. J Clin Hypertens. (2004) 6(9):532–40. doi: 10.1111/j.1524-6175.2004.03789.x
51. Cohen JB, Bancos I, Brown JM, Sarathy H, Turcu AF, Cohen DL. Primary aldosteronism and the role of mineralocorticoid receptor antagonists for the heart and kidneys. Annu Rev Med. (2023) 74(1):217–30. doi: 10.1146/annurev-med-042921-100438
52. Leader CJ, Moharram M, Coffey S, Sammut IA, Wilkins GW, Walker RJ. Myocardial global longitudinal strain: an early indicator of cardiac interstitial fibrosis modified by spironolactone, in a unique hypertensive rat model. PLoS One. (2019) 14(8):e0220837. doi: 10.1371/journal.pone.0220837
53. Garg M, Singh MK, Koli SM, Sreedhar B, Ramakrishna S, Nanubolu JB. Crystalline salts of a diuretic drug torasemide with improved solubility and dissolution properties. CrystEngComm. (2022) 24(23):4235–50. doi: 10.1039/D2CE00383J
54. Nuamnaichati N, Sato VH, Moongkarndi P, Parichatikanond W, Mangmool S. Sustained β-AR stimulation induces synthesis and secretion of growth factors in cardiac myocytes that affect on cardiac fibroblast activation. Life Sci. (2018) 193:257–69. doi: 10.1016/j.lfs.2017.10.034
55. Masarone D, Vastarella R, Melillo E, Petraio A, Pacileo G. Beta-blocker therapy in heart transplant recipients: a review. Clin Transplant. (2020) 34(11):e14081. doi: 10.1111/ctr.14081
56. Ahn MS, Yoo BS, Son JW, Yu MH, Kang DR, Lee HY, et al. Beta-blocker therapy at discharge in patients with acute heart failure and atrial fibrillation. J Korean Med Sci. (2020) 35(33):e278. doi: 10.3346/jkms.2020.35.e278
57. Niriayo YL, Asgedom SW, Demoz GT, Gidey K. Treatment optimization of beta-blockers in chronic heart failure therapy. Sci Rep. (2020) 10(1):15903. doi: 10.1038/s41598-020-72836-4
58. Chinnappa S, Maqbool A, Viswambharan H, Mooney A, Denby L, Drinkhill M. Beta blockade prevents cardiac morphological and molecular remodelling in experimental uremia. Int J Mol Sci. (2023) 25(1):373. doi: 10.3390/ijms25010373
59. Robert S, Pilon MO, Oussaïd E, Meloche M, Leclair G, Jutras M, et al. Impact of amiodarone use on metoprolol concentrations, α-OH-metoprolol concentrations, metoprolol dosing and heart rate: a cross-sectional study. Pharmacol Res Perspect. (2023) 11(5):e01137. doi: 10.1002/prp2.1137
60. Dai H, Yuan Y, Yin S, Zhang Y, Han Y, Sun L, et al. Metoprolol inhibits profibrotic remodeling of epicardial adipose tissue in a canine model of chronic obstructive sleep apnea. J Am Heart Assoc. (2019) 8(3):e011155. doi: 10.1161/jaha.118.011155
61. Liu M, Liu J, Zhang L, Geng Q, Ge Y. Antidepressant-like effects of ginseng fruit saponin in myocardial infarction mice. Biomed Pharmacother. (2019) 115:108900. doi: 10.1016/j.biopha.2019.108900
62. Ranjan A, Jindal A, Maiwall R, Vashishtha C, Vijayaraghavan R, Arora V, et al. Midodrine plus propranolol versus propranolol alone in preventing first bleed in patients with cirrhosis and severe ascites: a randomized controlled trial. Hepatol Int. (2024) 18(4):1261–70. doi: 10.1007/s12072-024-10687-1
63. Chen T, Gudipudi R, Nguyen SA, Carroll W, Clemmens C. Should propranolol remain the gold standard for treatment of infantile hemangioma? A systematic review and meta-analysis of propranolol versus atenolol. Ann Otol Rhinol Laryngol. (2023) 132(3):332–40. doi: 10.1177/00034894221089758
64. Li L, Gan H. Intact fibroblast growth factor 23 regulates chronic kidney disease–induced myocardial fibrosis by activating the sonic hedgehog signaling pathway. J Am Heart Assoc. (2022) 11(18):e026365. doi: 10.1161/jaha.122.026365
65. Tsai CK, Chen BH, Chen HH, Hsieh RJ, Lee JC, Chu YT, et al. Low-dose propranolol prevents functional decline in catecholamine-induced acute heart failure in rats. Toxics. (2022) 10(5):238. doi: 10.3390/toxics10050238
66. Li JS, Zhu XY, Lu ML, Gao JH, Wang HX, Yu XC. Effect of combined intervention of electroacupuncture and astragaloside IV on myocardial hypertrophy and TGF-β 1/smad signaling in rats with myocardial fibrosis. Zhen Ci Yan Jiu. (2017) 42(6):477–81. doi: 10.13702/j.1000-0607.2017.06.002
67. Susic D, Fares H, Frohlich ED. Nebivolol prevents myocardial fibrosis and diastolic dysfunction in salt-loaded spontaneously hypertensive rats. J Am Soc Hypertens. (2012) 6(5):316–23. doi: 10.1016/j.jash.2012.06.001
68. Huck DM, Rosenberg MA, Stauffer BL. Nebivolol and incident cardiovascular events in hypertensive patients compared with nonvasodilatory beta blockers. J Hypertens. (2022) 40(5):1019–29. doi: 10.1097/HJH.0000000000003109
69. Mohamed EA, Kassem HH. Protective effect of nebivolol on doxorubicin-induced cardiotoxicity in rats. Arch Med Sci. (2018) 14(6):1450–8. doi: 10.5114/aoms.2018.79008
70. Hikoso S, Kida H, Sunaga A, Nakatani D, Okada K, Dohi T, et al. β-blockers may be detrimental in frail patients with heart failure with preserved ejection fraction. Clin Res Cardiol. (2024) 113(6):842–55. doi: 10.1007/s00392-023-02301-5
71. Dar JA, Jacob JR. Beta blockers in contemporary cardiology: is it better to cast them out? Korean Circ J. (2024) 54(4):165. doi: 10.4070/kcj.2023.0209
72. Huang CY, Perman MJ, Yan AC. Regimen for accelerated propranolol initial dosing (RAPID). Pediatr Dermatol. (2024) 41(4):621–7. doi: 10.1111/pde.15623
73. Hanif N, Zamir A, Imran I, Saeed H, Majeed A, Rehman AU, et al. Clinical pharmacokinetics of nebivolol: a systematic review. Drug Metab Rev. (2023) 55(4):428–40. doi: 10.1080/03602532.2023.2271195
74. Lyu YT, Huo JY, Jiang W, Yang W, Wang S, Zhang S, et al. Empagliflozin ameliorates cardiac dysfunction in heart failure mice via regulating mitochondrial dynamics. Eur J Pharmacol. (2023) 942:175531. doi: 10.1016/j.ejphar.2023.175531
75. Wang Z, Liu Q, Wang X, Wang P, Wang Z, Zhang F. Empagliflozin improves cardiac function in rats with chronic heart failure. Naunyn Schmiedebergs Arch Pharmacol. (2024) 397(2):1037–44. doi: 10.1007/s00210-023-02655-7
76. Song XM, Zhao MN, Li GZ, Li N, Wang T, Zhou H. Atorvastatin ameliorated myocardial fibrosis in db/db mice by inhibiting oxidative stress and modulating macrophage polarization. World J Diabetes. (2023) 14(12):1849. doi: 10.4239/wjd.v14.i12.1849
77. Zhang T, Wang X, Wang Z, Zhai J, He L, Wang Y, et al. Canagliflozin ameliorates ventricular remodeling through apelin/angiotensin-converting enzyme 2 signaling in heart failure with preserved ejection fraction rats. Pharmacology. (2023) 108(5):478–91. doi: 10.1159/000533277
78. Liu Y, Fan Y, Li J, Chen M, Chen A, Yang D, et al. Combination of LCZ696 and ACEI further improves heart failure and myocardial fibrosis after acute myocardial infarction in mice. Biomed Pharmacother. (2021) 133:110824. doi: 10.1016/j.biopha.2020.110824
79. Ma D, Xu T, Cai G, Wu X, Lei Z, Liu X, et al. Effects of ivabradine hydrochloride combined with trimetazidine on myocardial fibrosis in rats with chronic heart failure. Exp Ther Med. (2019) 18(3):1639–44. doi: 10.3892/etm.2019.7730
80. Tang J, Zhao X. Research progress on regulation of immune response by tanshinones and salvianolic acids of danshen (salvia miltiorrhiza bunge). Molecules. (2024) 29(6):1201. doi: 10.3390/molecules29061201
81. Ayvazyan A, Deutsch L, Zidorn C, Kircher B, Çiçek SS. Cytotoxic diterpenoids from salvia glutinosa and comparison with the tanshinone profile of danshen (salvia miltiorrhiza). Front Plant Sci. (2023) 14:1269710. doi: 10.3389/fpls.2023.1269710
82. Zhu PC, Shen J, Qian RY, Xu J, Liu C, Hu WM. Effect of tanshinone IIA for myocardial ischemia/reperfusion injury in animal model: preclinical evidence and possible mechanisms. Front Pharmacol. (2023) 14:1165212. doi: 10.3389/fphar.2023.1165212
83. Wu M, Li H, Zhai R, Shan B, Guo C, Chen J. Tanshinone IIA positively regulates the Keap1-Nrf2 system to alleviate pulmonary fibrosis via the sestrin2-sqstm1 signaling axis-mediated autophagy. Phytomedicine. (2024) 129:155620. doi: 10.1016/j.phymed.2024.155620
84. Shan B, Guo C, Zhou H, Chen J. Tanshinone IIA alleviates pulmonary fibrosis by modulating glutamine metabolic reprogramming based on [U-13C5]-glutamine metabolic flux analysis. J Adv Res. (2024). doi: 10.1016/j.jare.2024.04.029
85. Bi Z, Wang Y, Zhang W. A comprehensive review of tanshinone IIA and its derivatives in fibrosis treatment. Biomed Pharmacother. (2021) 137:111404. doi: 10.1016/j.biopha.2021.111404
86. Qiao P, Xu J, Liu X, Li X. Tanshinone IIA improves ventricular remodeling following cardiac infarction by regulating miR-205-3p. Dis Markers. (2021) 2021(1):8740831. doi: 10.1155/2021/8740831
87. Hu S, Yang Z, Li L, Yan Q, Hu Y, Zhou F, et al. Salvianolic acid B alleviates liver injury by regulating lactate-mediated histone lactylation in macrophages. Molecules. (2024) 29(1):236. doi: 10.3390/molecules29010236
88. He G, Chen G, Liu W, Ye D, Liu X, Liang X, et al. Salvianolic acid B: a review of pharmacological effects, safety, combination therapy, new dosage forms, and novel drug delivery routes. Pharmaceutics. (2023) 15(9):2235. doi: 10.3390/pharmaceutics15092235
89. Chong CH, Sun JM, Liu YX, Tsai YT, Zheng DN, Zhang YF, et al. Salvianolic acid B attenuates hypertrophic scar formation in vivo and in vitro. Aesthetic Plast Surg. (2023) 47(4):1587–97. doi: 10.1007/s00266-023-03279-1
90. Luo H, Fu L, Wang X, Xu Y, Tao L, Shen X. Salvianolic acid B ameliorates myocardial fibrosis in diabetic cardiomyopathy by deubiquitinating Smad7. Chin Med. (2023) 18(1):161. doi: 10.1186/s13020-023-00868-9
91. Liu B, Du Y, Cong L, Jia X, Yang G. Danshen (Salvia miltiorrhiza) compounds improve the biochemical indices of the patients with coronary heart disease. Evid Based Complement Alternat Med. (2016) 2016(1):9781715. doi: 10.1155/2016/9781715
92. Huang L, Zhu J, Zheng M, Zou R, Zhou Y, Zhu M. Tanshinone IIA protects against subclinical lipopolysaccharide induced cardiac fibrosis in mice through inhibition of NADPH oxidase. Int Immunopharmacol. (2018) 60:59–63. doi: 10.1016/j.intimp.2018.04.036
93. Li W, Liu K, Tao X, Zhao S, Mu F, Chen S, et al. Application of mixture design for optimum cardio protection efficacy of mixtures of salvianolic acid A, salvianolic acid B and danshensu from salvia miltiorrhiza. Ind Crops Prod. (2023) 192:116095. doi: 10.1016/j.indcrop.2022.116095
94. Gao H, Bo Z, Wang Q, Luo L, Zhu H, Ren Y. Salvanic acid B inhibits myocardial fibrosis through regulating TGF-β1/smad signaling pathway. Biomed Pharmacother. (2019) 110:685–91. doi: 10.1016/j.biopha.2018.11.098
95. Yao J, Peng T, Shao C, Liu Y, Lin H, Liu Y. The antioxidant action of astragali radix: its active components and molecular basis. Molecules. (2024) 29(8):1691. doi: 10.3390/molecules29081691
96. Li M, Han B, Zhao H, Xu C, Xu D, Sieniawska E, et al. Biological active ingredients of astragali radix and its mechanisms in treating cardiovascular and cerebrovascular diseases. Phytomedicine. (2022) 98:153918. doi: 10.1016/j.phymed.2021.153918
97. Chien MY, Yang CM, Chen CH. Effects of physical properties and processing methods on astragaloside IV and flavonoids content in astragali radix. Molecules. (2022) 27(2):575. doi: 10.3390/molecules27020575
98. Gong F, Qu R, Li Y, Lv Y, Dai J. Astragalus mongholicus: a review of its anti-fibrosis properties. Front Pharmacol. (2022) 13:976561. doi: 10.3389/fphar.2022.976561
99. Shan Y, Yu M, Dai H, Zhu X, Wang F, You Y, et al. The role of macrophage-derived exosomes in reversing peritoneal fibrosis: insights from astragaloside IV. Phytomedicine. (2024) 129:155683. doi: 10.1016/j.phymed.2024.155683
100. Shi L, Deng J, He J, Zhu F, Jin Y, Zhang X, et al. Integrative transcriptomics and proteomics analysis reveal the protection of astragaloside IV against myocardial fibrosis by regulating senescence. Eur J Pharmacol. (2024) 975:176632. doi: 10.1016/j.ejphar.2024.176632
101. Zhang X, Qu H, Yang T, Liu Q, Zhou H. Astragaloside IV attenuate MI-induced myocardial fibrosis and cardiac remodeling by inhibiting ROS/caspase-1/GSDMD signaling pathway. Cell Cycle. (2022) 21(21):2309–22. doi: 10.1080/15384101.2022.2093598
102. Essawy AE, Abd Elkader HTAE, Khamiss OA, Eweda SM, Abdou HM. Therapeutic effects of astragaloside IV and Astragalus spinosus saponins against bisphenol A-induced neurotoxicity and DNA damage in rats. PeerJ. (2021) 9:e11930. doi: 10.7717/peerj.11930
103. Xiao Y, Liu T, Liu X, Zheng L, Yu D, Zhang Y, et al. Total astragalus saponins attenuates CVB3-induced viral myocarditis through inhibiting expression of tumor necrosis factor α and fas ligand. Cardiovasc Diagn Ther. (2019) 9(4):337. doi: 10.21037/cdt.2019.07.11
104. Tang Z, Huang G. Extraction, structure, and activity of polysaccharide from radix astragali. Biomed Pharmacother. (2022) 150:113015. doi: 10.1016/j.biopha.2022.113015
105. Liu T, Zhang M, Niu H, Liu J, Ruilian MA, Wang Y, et al. Astragalus polysaccharide from astragalus melittin ameliorates inflammation via suppressing the activation of TLR-4/NF-κB p65 signal pathway and protects mice from CVB3-induced virus myocarditis. Int J Biol Macromol. (2019) 126:179–86. doi: 10.1016/j.ijbiomac.2018.12.207
106. Yu L, Zhou C, Luo Z, Zeng W, Lai F, Han G, et al. The lipid-lowering effects of Danhong and Huangqi injections: a meta-analysis of clinical controlled trials. Lipids Health Dis. (2018) 17:1–13. doi: 10.1186/s12944-018-0760-2
107. Li Z, Yang W, Yang Y, Wu J, Luo P, Liu Y. The astragaloside IV derivative LS-102 ameliorates obesity-related nephropathy. Drug Des Devel Ther. (2023) 16:647–64. doi: 10.2147/dddt.s346546
108. Chen XY, Wang TT, Shen Q, Ma H, Li Z, Yu XN, et al. Preclinical investigations on anti-fibrotic potential of long-term oral therapy of sodium astragalosidate in animal models of cardiac and renal fibrosis. ACS Pharmacol Transl Sci. (2024) 7(2):421–31. doi: 10.1021/acsptsci.3c00264
109. Wu S, Da L, Xiao Q, Pan Q, Zhang J, Yang J. ASAP: a platform for gene functional analysis in Angelica sinensis. BMC genomics. (2024) 25(1):96. doi: 10.1186/s12864-024-09971-z
110. Zhi X, Ren C, Li Q, Xi H, Li D, Chen Q, et al. Therapeutic potential of Angelica sinensis in addressing organ fibrosis: a comprehensive review. Biomed Pharmacother. (2024) 173:116429. doi: 10.1016/j.biopha.2024.116429
111. Ren C, Wang L, Li X, Tang Y, Zhi X, Zhuang M, et al. Elucidating the mechanism of action of radix Angelica sinensis (oliv.) diels and radix Astragalus mongholicus bunge ultrafiltration extract on radiation-induced myocardial fibrosis based on network pharmacology and experimental research. Eur J Pharm Sci. (2024) 199:106794. doi: 10.1016/j.ejps.2024.106794
112. Zhao YF, Xu J, Tang JQ, Wang H, Shang GHJ, Guan HS. Effect of Angelica on myocardial fibrosis post myocardial infarction in rats. Chin J Pathophysiol. (2006) 22:1965–9.
113. Song X, Kong J, Song J, Pan R, Wang L. Angelica sinensis polysaccharide alleviates myocardial fibrosis and oxidative stress in the heart of hypertensive rats. Comput Math Methods Med. (2021) 2021(1):6710006. doi: 10.1155/2021/6710006
114. Pan H, Zhu L. Retracted: angelica sinensis polysaccharide protects rat cardiomyocytes H9c2 from hypoxia-induced injury by down-regulation of microRNA-22. Biomed Pharmacother. (2018) 106:225–31. doi: 10.1016/j.biopha.2018.06.120
115. Jin Y, Qu C, Tang Y, Pang H, Liu L, Zhu Z, et al. Herb pairs containing Angelicae Sinensis radix (danggui): a review of bio-active constituents and compatibility effects. J Ethnopharmacol. (2016) 181:158–71. doi: 10.1016/j.jep.2016.01.033
116. Bi SJ, Fu RJ, Li JJ, Chen YY, Tang YP. The bioactivities and potential clinical values of Angelica sinensis polysaccharides. Nat Prod Commun. (2021) 16(3):1934578X21997321. doi: 10.1177/1934578X21997321
117. Sun S, Gong D, Liu R, Wang R, Chen D, Yuan T, et al. Puerarin inhibits NLRP3-caspase-1-GSDMD-mediated pyroptosis via P2X7 receptor in cardiomyocytes and macrophages. Int J Mol Sci. (2023) 24(17):13169. doi: 10.3390/ijms241713169
118. Ming Y, Jiachen L, Tao G, Zhihui W. Exploration of the mechanism of Tripterygium Wilfordii in the treatment of myocardial fibrosis based on network pharmacology and molecular docking. Curr Comput Aided Drug Des. (2023) 19(1):68. doi: 10.2174/1573409919666221028120329
119. Zhen J, Bai J, Liu J, Men H, Yu H. Ginsenoside RG1-induced mesenchymal stem cells alleviate diabetic cardiomyopathy through secreting exosomal circNOTCH1 to promote macrophage M2 polarization. Phytother Res. (2024) 38(4):1745–60. doi: 10.1002/ptr.8018
120. Ni SY, Zhong XL, Li ZH, Huang DJ, Xu WT, Zhou Y, et al. Puerarin alleviates lipopolysaccharide-induced myocardial fibrosis by inhibiting PARP-1 to prevent HMGB1-mediated TLR4-NF-κB signaling pathway. Cardiovasc Toxicol. (2020) 20:482–91. doi: 10.1007/s12012-020-09571-9
121. Zhang Y, Lu F. Molecular mechanism of triptolide in myocardial fibrosis through the wnt/β-catenin signaling pathway. Scand Cardiovasc J. (2024) 58(1):2295785. doi: 10.1080/14017431.2023.2295785
122. Wang Y, An X, Wang F, Jiang Y. Ginsenoside RH4 inhibits ang II-induced myocardial remodeling by interfering with NFIL3. Biomed Pharmacother. (2024) 172:116253. doi: 10.1016/j.biopha.2024.116253
123. Zhu M, Chen L, Xu Z, Xi X, Shi X, Zhang Y. Clinical efficacy of qili qiangxin capsule combined with exercise rehabilitation in the treatment of chronic heart failure. Explore. (2023) 19(3):445–9. doi: 10.1016/j.explore.2022.10.002
124. Qin Y, Lv C, Zhang X, Ruan W, Xu X, Chen C, et al. Protective effect of qiliqiangxin against doxorubicin-induced cardiomyopathy by suppressing excessive autophagy and apoptosis. Cardiovasc Ther. (2022) 2022(1):9926635. doi: 10.1155/2022/9926635
125. Wu N, Chi J, Cai H, Hu J, Lai Y, Lin C, et al. Traditional Chinese medication qili qiangxin capsule protects against myocardial ischemia-reperfusion injury through suppressing autophagy via the phosphoinositide 3-kinase/protein kinase B/forkhead box O3 axis. J Ethnopharmacol. (2025) 337:118821. doi: 10.1016/j.jep.2024.118821
126. Sun M, Liu C, Gao K, Xu X, Chen K, Qiu L, et al. Qili qiangxin capsule attenuates myocardial fibrosis by modulating collagen homeostasis post-infarction in rats. PLoS One. (2024) 19(9):e0310897. doi: 10.1371/journal.pone.0310897
127. Ji X, Yang D, Cui X, Lou L, Nie B, Zhao J, et al. Mechanism of qili qiangxin capsule for heart failure based on miR133a-endoplasmic Reticulum stress. Chin J Integr Med. (2024) 30(5):398–407. doi: 10.1007/s11655-024-3654-3
128. Xing X, Guo J, Mo J, Li H, Zhang H, Shao B, et al. Qili qiangxin capsules for chronic heart failure: a GRADE-assessed clinical evidence and preclinical mechanism. Front Cardiovasc Med. (2023) 9:1090616. doi: 10.3389/fcvm.2022.1090616
129. Packer M. Qiliqiangxin: a multifaceted holistic treatment for heart failure or a pharmacological probe for the identification of cardioprotective mechanisms? Eur J Heart Fail. (2023) 25(12):2130–43. doi: 10.1002/ejhf.3068
130. Lu C, Yang Y, Lu Y, Zhu Y, Zhang A, Lyu S, et al. QiShen YiQi dripping pills for cardiovascular diseases: effects and mechanisms. Chin J Integr Med. (2023) 29(9):857–64. doi: 10.1007/s11655-022-3288-2
131. Wu L, Fan Z, Gu L, Liu J, Cui Z, Yu B, et al. QiShen YiQi dripping pill alleviates myocardial ischemia-induced ferroptosis via improving mitochondrial dynamical homeostasis and biogenesis. J Ethnopharmacol. (2023) 308:116282. doi: 10.1016/j.jep.2023.116282
132. Lv S, Zhang W, Yuan P, Lu C, Dong J, Zhang J. QiShen YiQi pill for myocardial collagen metabolism and apoptosis in rats of autoimmune cardiomyopathy. Pharm Biol. (2022) 60(1):722–8. doi: 10.1080/13880209.2022.2056206
133. Lv S, Wang Q, Wu M, Li M, Wang X, Xu L, et al. QiShen YiQi pill improves myocardial hypertrophy caused by pressure overload in rats. Evid Based Complement Alternat Med. (2021) 2021(1):5536723. doi: 10.1155/2021/5536723
134. Lu Y, Wang D, Yuan X, Wang M, Li Z, Bao X, et al. Protective effect of Qishen Yiqi dropping pills on the myocardium of rats with chronic heart failure. Exp Ther Med. (2019) 17(1):378–82. doi: 10.3892/etm.2018.6957
135. Mao J, Zhang J, Lam CSP, Zhu M, Yao C, Chen S, et al. Qishen Yiqi dripping pills for chronic ischaemic heart failure: results of the CACT-IHF randomized clinical trial. ESC Heart Fail. (2020) 7(6):3881–90. doi: 10.1002/ehf2.12980
136. Wang M, Shan Y, Wu C, Cao P, Sun W, Han J, et al. Efficacy and safety of Qishen Yiqi dripping pill for heart failure with preserved ejection fraction: a systematic review and meta-analysis. Front Pharmacol. (2020) 11:626375. Quantitative Imaging in Medicine and Surgery. All rights reserved. Quant Imaging Med Surg, 2023, 13(9): 5877–5886. doi: 10.3389/fphar.2020.626375
137. Wang H, Li L, Qing X, Zhang S, Li S. Efficacy of Qishen Yiqi drop pill for chronic heart failure: an updated meta-analysis of 85 studies. Cardiovasc Ther. (2020) 2020(1):8138764. doi: 10.1155/2020/8138764
138. Xin J, Wang T, Hou B, Lu X, Han N, He Y, et al. Tongxinluo capsule as a multi-functional traditional Chinese medicine in treating cardiovascular disease: a review of components, pharmacological mechanisms, and clinical applications. Heliyon. (2024) 10(13):e33309. doi: 10.1016/j.heliyon.2024.e33309
139. Li L, Feng P, Zhou W, Luo B, Deng L, Gan D, et al. Efficacy and safety of tongxinluo capsule for angina pectoris of coronary heart disease: an overview of systematic reviews and meta-analysis. Front Cardiovasc Med. (2024) 11:1229299. doi: 10.3389/fcvm.2024.1229299
140. Yin Y, Zhang Q, Zhao Q, Ding G, Wei C, Chang L, et al. Tongxinluo attenuates myocardiac fibrosis after acute myocardial infarction in rats via inhibition of endothelial-to-mesenchymal transition. BioMed Res Int. (2019) 2019(1):6595437. doi: 10.1155/2019/6595437
141. Wei Y, Hou Y, Yin Y, Li Z, Liu Y, Han N, et al. Tongxinluo activates PI3K/AKT signaling pathway to inhibit endothelial mesenchymal transition and attenuate myocardial fibrosis after ischemia-reperfusion in mice. Chin J Integr Med. (2024) 30(7):608–15. doi: 10.1007/s11655-024-3652-5
142. Wang X, Mu C, Mu T, Gao L, Zhao Y, Zhang Y, et al. Effects of tongxinluo on myocardial fibrosis in diabetic rats. J Chin Med Assoc. (2016) 79(3):130–6. doi: 10.1016/j.jcma.2015.06.022
143. Li L, Yunlong H. The structure-function remodeling and therapeutic effect of tongxinluo capsule in rabbit hearts of myocardial infarction. FASEB J. (2018) 32:901.2–901.2. doi: 10.1096/fasebj.2018.32.1_supplement.901.2
144. Chenhao Z, Jia W, Yiying L, Xiaohe Y, Xuesen W, Meili G, et al. Effect of tongxinluo capsule on the use of anti-ischemic drugs in patients with qi deficiency and blood stasis chronic coronary syndrome:a multicenter, prospective cohort study. Chin J Exp Pharm. (2024) 30(23):149–56. doi: 10.13422/j.cnki.syfjx.20250392
145. Wang J, Li TL, Chang PF, Gao YQ, Fan JS, Zhang CH, et al. Clinical effects and mechanisms of a Chinese patent medicine, tongxinluo capsule, as an adjuvant treatment in coronary heart disease. Heliyon. (2024) 10(6):e27460. doi: 10.1016/j.heliyon.2024.e27460
146. Liu K, Lv M, Ji X, Lou L, Nie B, Zhao J, et al. Wenxin granules regulate endoplasmic Reticulum stress unfolded protein response and improve ventricular remodeling on rats with myocardial infarction. Evid Based Complement Alternat Med. (2021) 2021(1):7375549. doi: 10.1155/2021/7375549
147. Zhang M, Guo F, Li X, Xian M, Wang T, Wu H, et al. Yi-Xin-Shu capsule ameliorates cardiac hypertrophy by regulating RB/HDAC1/GATA4 signaling pathway based on proteomic and mass spectrometry image analysis. Phytomedicine. (2022) 103:154185. doi: 10.1016/j.phymed.2022.154185
148. Wang X, Yang J, Lu C, Hu Y, Xu Z, Wan Q, et al. Qifu yixin formula improves heart failure by enhancing β-Arrestin2 mediated the SUMOylation of SERCA2a. Drug Des Devel Ther. (2024) 18:781–99. doi: 10.2147/DDDT.S446324
149. Makarov I, Voronkina D, Gurshchenkov A, Ryzhkov A, Starshinova A, Kudlay D, et al. Are endomyocardial ventricular biopsies useful for assessing myocardial fibrosis? J Clin Med. (2024) 13(11):3275. doi: 10.3390/jcm13113275
150. Zhou E, Shen Q, Hou Y. Integrating artificial intelligence into the modernization of traditional Chinese medicine industry: a review. Front Pharmacol. (2024) 15:1181183. doi: 10.3389/fphar.2024.1181183
Keywords: myocardial fibrosis, Western medicine, Chinese medicine, drugs, excessive extracellular matrix
Citation: Zhu Y, Zhang F, Li Z, Zhou Y, Shu Y, Ruan J and Chen G (2025) Chinese and western medicine treatment of myocardial fibrosis drugs. Front. Cardiovasc. Med. 11:1477601. doi: 10.3389/fcvm.2024.1477601
Received: 8 August 2024; Accepted: 16 December 2024;
Published: 15 January 2025.
Edited by:
Juncheng Wei, Temple University, United StatesReviewed by:
Hector A. Cabrera-Fuentes, Imam Abdulrahman bin Faisal University, Saudi ArabiaHuiliang Qiu, Mayo Clinic Arizona, United States
Copyright: © 2025 Zhu, Zhang, Li, Zhou, Shu, Ruan and Chen. This is an open-access article distributed under the terms of the Creative Commons Attribution License (CC BY). The use, distribution or reproduction in other forums is permitted, provided the original author(s) and the copyright owner(s) are credited and that the original publication in this journal is cited, in accordance with accepted academic practice. No use, distribution or reproduction is permitted which does not comply with these terms.
*Correspondence: Guo Chen, NDcwMjQwNTU5QHFxLmNvbQ==
†These authors have contributed equally to this work and share first authorship