- 1Division of Pediatric Cardiology, Department of Women’s and Children’s Health, University Hospital of Padua, Padua, Italy
- 2Pediatric Department, San Bortolo Hospital, Vicenza, Italy
- 3PhD School in Developmental Medicine and Health Planning Sciences, University of Padua, Padua, Italy
- 4Division of Cardiology, Department of Medical and Surgical Science, Magna Graecia University, Catanzaro, Italy
- 5Pediatric Diabetology, Department of Women’s and Children’s Health, University Hospital of Padua, Padua, Italy
Background: Cardiac dysfunction and endothelial damage are known complications of type 1 diabetes mellitus (T1D) mainly affecting adults. However, some studies have shown that subclinical myocardial impairment already present during adolescence. Myocardial work (MW) has emerged as an afterload-independent tool that allows early identification of subclinical damage. This study aims to provide a comprehensive non-invasive cardiovascular evaluation of T1D adolescents using both conventional and advanced echocardiography.
Methods: We enrolled 31 patients, aged between 13 and 19 years, who were diagnosed with T1D for at least 10 years and were followed up by the Paediatric Diabetology Unit of our institution. We collected data relating to anthropometry, lifestyle, blood tests, glycemic control parameters, and conventional and advanced echocardiographic measurements. A comparison of MW parameters with the data from 31 age- and sex-matched healthy volunteers from a previous study in our lab was carried out.
Results: In our population, the glycemic control parameters showed suboptimal control. While diastolic parameters were in the normal range for all the patients, E′ velocities and left atrial diameter were significantly worse in patients with poorer glycemic controls. Global longitudinal strain (GLS), global work index (GWI), and global work efficiency (GWE) were significantly lower in the T1D population compared to those in the healthy population (p < 0.001), while global wasted work was significantly higher in the T1D population (p < 0.001). Patients with stage 1 hypertension or a pre-hypertensive state exhibited pathological pulse wave velocities with values exceeding 8 m/s (>99th percentile).
Conclusions: To the best of our knowledge, this was the first study to investigate MW in T1D adolescents. The descriptive parameters of GLS and MW showed subclinical cardiac damage already during this timeframe. Therefore, these tools should be integrated into the cardiovascular assessment of diabetic adolescents, and preventive strategies should be implemented to maximize glycemic and pressure control effectiveness.
1 Introduction
Cardiovascular risk assessment in diabetic adolescents has become critically important in preventive medicine. Type 1 diabetes mellitus (T1D) is one of the most common endocrine and metabolic conditions in childhood. Echocardiography and vascular function parameters in T1D adolescents with type 1 diabetes mellitus (T1D) are significantly altered. In this age group, research suggests a correlation between T1D and early cardiac health issues (1, 2). From an echocardiographic perspective, conventional parameters often reveal a higher incidence of structural and functional alterations in cardiac chambers, such as left ventricular thickening and compromised diastolic function (3–5). Furthermore, several studies have identified significant reductions in global longitudinal strain (GLS), an indicator of myocardial function, in T1D adolescents compared to their healthy peers. This suggests that diabetes may impact heart contractility even at a young age. These changes may have implications for long-term cardiovascular health. Regarding vascular function, investigations into pulse wave velocity (PWV) have detected increased arterial stiffness in young individuals with T1D (6, 7). This increase may be associated with a higher risk of cardiovascular complications throughout life.
However, all the cardiac parameters studied in this population are dependent on afterload, so it is possible that the abnormal measurements are only a reflection of increased afterload.
Myocardial work (MW), an innovative technique that integrates left ventricular pressure into strain measurement on echocardiography, was officially approved and introduced to the market in 2018. In contrast to GLS, it assesses LV performance by integrating afterload determination via blood pressure cuff, thereby offering a measure that is less dependent on afterload (8).
Therefore, this study aims to provide a comprehensive non-invasive cardiovascular evaluation of T1D adolescents using conventional echocardiography, speckle tracking analysis, and for the first time myocardial work.
2 Methods
This cross-sectional single-center study was conducted at the Paediatric Department of the University Hospital of Padua between June 2023 and October 2023. A summary outline of the study design and its main results can be found in Supplementary Figure 1.
2.1 Population
The inclusion criteria were patients diagnosed with type 1 diabetes mellitus for at least 10 years with intensive therapeutic management. The exclusion criteria were patients diagnosed with diabetes other than type 1, patients without glycemic reports, patients already diagnosed with cardiovascular or congenital heart diseases, patients with genetic cardiological diseases, and patients with a poor acoustic window potentially affecting data analysis.
Thus, we enrolled 31 patients, aged between 13 and 19 years, who were diagnosed with type 1 diabetes mellitus for at least 10 years and who were followed up by the Paediatric Diabetology Unit of our institution. The diagnosis of diabetes followed the criteria defined by the International Society for Paediatric and Adolescent Diabetes (ISPAD) (9). Our cardiological evaluation was intended as best practice in the follow-up for these patients. Informed consent was obtained directly from patients and their parents.
The GLS and myocardial work parameters were then compared to data from 31 age- and sex-matched healthy volunteers from a previous study in our lab (10). The inclusion criteria were patients aged 13–19 years, patients with no previous history of cardiovascular or lung disease and no abnormalities during physical examination (except for a physiologic heart murmur), and patients with normal ECG and normal standard echocardiography. Subjects with minor defects, such as small atrial septal defect, patent ductus arteriosus, and irregular rhythm, or those with images of poor quality were excluded.
2.2 Clinical data
We collected clinical data relating to vital parameters and puberal stage from all patients. The puberal stage was determined according to Tanner's classification.
2.3 Glycaemic control parameters
The following indices of glycaemic control were studied:
• glycated hemoglobin during the last consultation, target values <7%;
• time in range (TIR—the amount of monitored time with glycemia with values between 70 and 180 mg/dl), target values >70%. In our study, we considered three categories of time in range: TIR > 70%, TIR between 50% and 70%, and TIR inferior to 50%;
• time above range (TAR—the amount of monitored time with glycemia with values between 180 and 250 mg/dl), target values <25%;
• time very above range (TVAR— the amount of monitored time with glycemia with values > 250 mg/dl), target values <5%; and
• coefficient of variation (CV), optimal glycemic variability ≤36%.
2.4 Conventional echocardiography
All patients underwent a standard echocardiographic assessment using a Vivid E95 Ultrasound Machine (GE Vingmed Ultrasound AS, Horten, Norway) equipped with a 4Vc probe. Echocardiograms were performed by one pediatric cardiologist (MG). Images were then analyzed offline on the EchoPAC software version 204 by a single experienced reader (IC) blinded to the clinical status and laboratory data. According to the latest recommendations, the conventional parameters of systolic and diastolic function were measured, namely, left ventricular volume, cardiac mass, LV ejection fraction, left atrial anteroposterior diameter, left atrial volume, atrioventricular valve inflow, and annular velocity. The left ventricular end-diastolic volume was calculated by averaging the end-diastolic volumes measured from the four-chamber and two-chamber views. The cardiac mass was defined using the Devereux formula, starting from interventricular septal thickness, posterior wall thickness, and left ventricular end-diastolic diameter on a parasternal long-axis view. The values were then indexed by both body surface area (BSA) and height elevated to 2.7 (11). The obtained values were then interpreted according to previous studies (12). The ejection fraction was assessed using the biplane Simpson method. The left atrial anteroposterior diameter was measured at the end systole on a 2D parasternal long-axis view, while the left atrial volume was estimated from an apical four-chamber view in the same phase of the cardiac cycle. Mitral inflow velocities were measured by placing the pulsed wave Doppler cursor at the tips of the mitral leaflets on an apical four-chamber view. Peaks E and A were consequently identified. Tissue Doppler imaging (TDI) analysis of diastolic velocities was performed from the same view by placing the pulsed wave Doppler on the medial and lateral sides of the mitral annulus to define septal and lateral E′, respectively.
2.5 Speckle tracking analysis
In addition, atrial strain and left ventricular longitudinal and circumferential strain (CS) were determined by speckle tracking. To ensure optimal tracking, we obtained images with a frame rate between 50 and 80 fps and a sinus rhythm and heart rate variability ≤10% (13). According to the European Association of Cardiovascular Imaging consensus document, the left ventricular longitudinal strain (GLS) was calculated from three apical views (apical four-chamber, apical two-chamber, and apical three-chamber views) (14). The left ventricle circumferential strain was calculated from a parasternal short-axis view at the level of the papillary muscles. The left atrial strain was assessed from an apical four-chamber view (15). Once the images were collected, the endocardial border was traced. The automatized tracking given by the software was inspected before proceeding to the results: The tracking was considered good when it followed the endocardial border throughout the cardiac cycle. We reported peak systolic strain for the GLS and CS, while for atrial strain, we considered the positive deformation occurring during the reservoir phase.
2.6 Myocardial work analysis
Once the strain analysis curves from the three apical views were obtained, a dedicated function of the GE software was used to estimate myocardial work (MW). Systolic and diastolic blood pressure values, obtained non-invasively using a digital sphygmomanometer with brachial cuff, were entered into the software. The time of aortic and mitral valve opening and closing was identified by the operator based on the three-chamber recording, as required for the synchronization of strain and pressure data (10).
Therefore, the global work index (GWI, mmHg%) was calculated, together with the derived parameters:
• global constructive work (GCW, mmHg%): work performed by a segment during shortening in systole plus negative work during lengthening in isovolumetric relaxation;
• global wasted work (GWW, mmHg%): negative work performed by one segment during lengthening in systole plus work performed during shortening in isovolumic relaxation; and
• global work efficiency (GWE): constructive work divided by the sum of constructive work and wasted work (0%–100%).
2.7 Pulse wave velocity
In addition, we investigated the possible markers of vascular damage by determining the pulse wave velocity (PWV) (6). PWV was calculated as the distance/transit time (m/s) and was assessed by measuring the carotid aortic pulse wave (16).
2.8 Statistical analysis
The continuous variables and clinical and echocardiographic data are displayed as mean ± standard deviation, if normally distributed, or as median and interquartile range (IQR), if not normally distributed. The normality distribution was tested using the Shapiro–Wilk test. The binomial and ordinal qualitative variables are presented as frequencies and percentages. The correlation between the continuous variables was tested using Pearson's correlation or Spearman's test. The comparison between the two groups was assessed using the Student’s t-test or Mann–Whitney test. The comparison between multiple groups was assessed by one-way ANOVA or the Kruskall–Wallis test. A two-sided p-value of 0.05 was considered statistically significant for all tests. All statistical analyses were performed with SPSS software version 25.0 (International Business Machines Corporation, Armonk, NY, USA).
3 Results
3.1 Population characteristics
Of the 38 initially eligible patients, 7 were excluded due to refusal to participate in the study. Overall, we studied 31 patients, of whom 21 were male (67.7%) and 10 were female (32.3%). Patients’ diabetic features are shown in Table 1.
All patients presented an advanced pubertal stage (at least G3, PH3, and I3 for males and B4, PH4, and I3 for females). Average BMI fell into the normal range (around 75th percentile). Nine patients (29%) had a BMI score in the overweight range (BMI between the 85th and 95th percentile), while one patient had a BMI above the 95th percentile. There were no underweight patients.
Considering blood pressure values, four patients (12.9%) had both systolic and diastolic hypertension, while two patients had isolated diastolic hypertension (6.5%), as shown in Table 2. Six patients showed pressure values between the 90th and 95th percentile.
Thirty-one age- and sex-matched healthy volunteers enrolled in a previous study were also included. Their anthropometric features are reported in Table 1.
3.2 Glycemic control parameters
Regarding the time in range (TIR), six patients presented a value of >70% (19.4% of the population); 15 patients, between 50% and 70% (48.4% of the population); and 10 patients, <50% (32.3% of the population). Glycemic variability (CV) was <36% in 9 patients (29% of the population) and >36% in the remaining 21 patients (71% of the population). The test population had a median TAR of 24% (IQR 6) and a median TVAR of 12% (IQR 13). The HbA1c value of 12 patients at the last check-up was <7% (38.7%), and that of the remaining 19 was >7% (61.3%). If 6.5% is considered as the HbA1c cutoff value, 7 and 24 patients had a value below and above this threshold (22.5% and 77.5%, respectively).
3.3 Conventional echocardiographic parameters and glycaemic control
Overall, our population showed normal average values of diameter, volume, ejection fraction, cardiac mass, and diastolic function parameters. The average values and standard deviations or median values and interquartile ranges are reported in Table 3.
The analysis of our population revealed a statistically significant trend toward higher septal thickness in patients with lower time in range (7.6 ± 1.7 mm in patients with TIR < 50% vs. 6 ± 1.2 mm in patients with TIR > 70%, p = 0.004). In addition, the diastolic parameters showed a correlation with glycemic control. E′ lateral velocity, in fact, appeared to be lower in patients with lower time in range (18 IQR 0.6 cm/s vs. 22 IQR 0.4 cm/s, p = 0.013). The same trend was also observed for septal E′ (13.8 ± 0.2 cm/s vs. 16.5 ± 0.1 cm/s, p = 0.01). When considering E′ velocities and other parameters of glycemic controls, the same relation was observed: E′ septal was lower for control variability >36% (p = 0.03), E′ lateral was lower in the group with glycated hemoglobin >6.5% (p = 0.01), and E′ septal negatively correlates with the total time above range (TTAR) (R2 = 0.201, p = 0.022). Moreover, the left atrium diameter was also higher in patients with less glycemic control (2.9 ± 0.3 cm vs. 2.4 ± 0.5 cm, p = 0.011) and positively correlated with TTAR (R2 = 0.258, p = 0.003).
3.4 Speckle tracking echocardiography and myocardial work
Regarding speckle tracking-derived parameters, we did not find any statistically significant relation with glycemic control. However, GLS showed a trend toward more positive values in patients with lower time in range (GLS −17.1% ± 2.3 in patients with TIR > 70%, while GLS −15.8% ± 1.6 in patients with TIR <50%, p = 0.346). Circumferential strain (CS), instead, showed higher values in patients with worst glycemic control: CS −21.9% ± 3.5 in patients with CV < 36% vs. CS −22.7% ± 3.4 in patients with CV >36%; CS −21.7% ± 2.8 in patients with glycated hemoglobin <6.5% vs. CS −22.7% ± 3.5 in patients with glycate hemoglobin >6.5%; and CS −21.5% ± 3 in patients with TIR > 70% vs. CS −22.9% ± 4.1 in patients with TIR < 50%. These relations did not reach statistical significance but highlighted possible trends.
GLS showed a good negative correlation with GCW (r = −0.708, p < 0.001), GWI (r = −0.572, p = 0.013), and GWE (r = −0.484, p = 0.006). No correlation was found between GLS and GWW. Finally, GLS was found to correlate with septal thickness, showing more positive values for increased thickness (r = 0.344, p = 0.016).
Similarly, GWW appeared to correlate with cardiac mass (ρ = 0.361, p = 0.046) and septal width during diastole (ρ = 0.381, p = 0.034).
3.5 Pulse wave velocity
The median pulse wave velocity (PWV) in our population was around 5.2 m/s (2.7). As shown in Figure 1, there was a positive association between PWV with hypertension or pre-hypertensive state, both for systolic and diastolic blood pressure (p = 0.045 among different percentiles of systolic blood pressure; p = 0.009 for different percentiles of diastolic pressure). In patients with a pre-hypertensive state or first-degree hypertension, PWV reached pathological values around 8–10 m/s. However, no correlation was found with glycemic control, GLS, and the descriptive parameters of myocardial work.
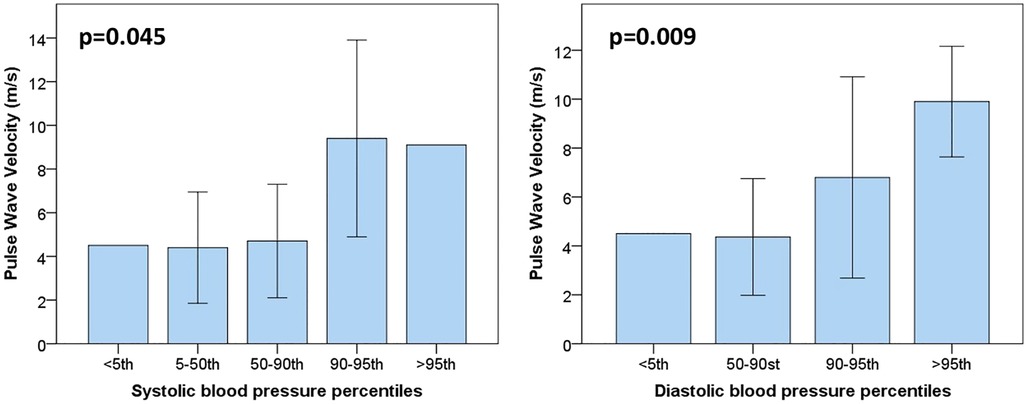
Figure 1. Pulse wave velocity values are plotted for systolic (on the left) and diastolic blood pressure percentiles (on the right). As it is possible to notice, PWV values start to be altered from pressure values above the 90th percentile (pre-hypertension state).
3.6 Comparison with healthy volunteers
GLS and myocardial work data from diabetic adolescents were also compared with the data from 31 age- and sex-matched healthy volunteers from a previous study in our lab (Table 4). Although ejection fraction did not show any impairment, GLS and myocardial work indexes appeared to be significantly impaired if compared to those of our reference population. Diabetic patients showed significantly lower values of GLS (−16.7% ± 2.2 in diabetic patients vs. −20.5% ± 1.9 in healthy volunteers, p < 0.001). As shown in Figure 2, the myocardial work parameters appeared to be significantly different in diabetic patients with lower global work index (1,466 mmHg% ± 148 in diabetic patients vs. 1,744 ± 234 mmHg% in healthy volunteers, p < 0.001), increased global wasted work (169 mmHg% IQR 86 in diabetic patients vs. 67 mmHg% IQR 39 in healthy volunteers, p < 0.001), and lower global work efficiency (91% IQR 4 in diabetic patients vs. 96.8 IQR 6 in healthy volunteers, p < 0.001). No significative difference was found among the amount of global constructive work [1,941 (491) mmHg% in diabetic adolescents vs. 2,120 (280) mmHg% in healthy volunteers, p = 0.097].
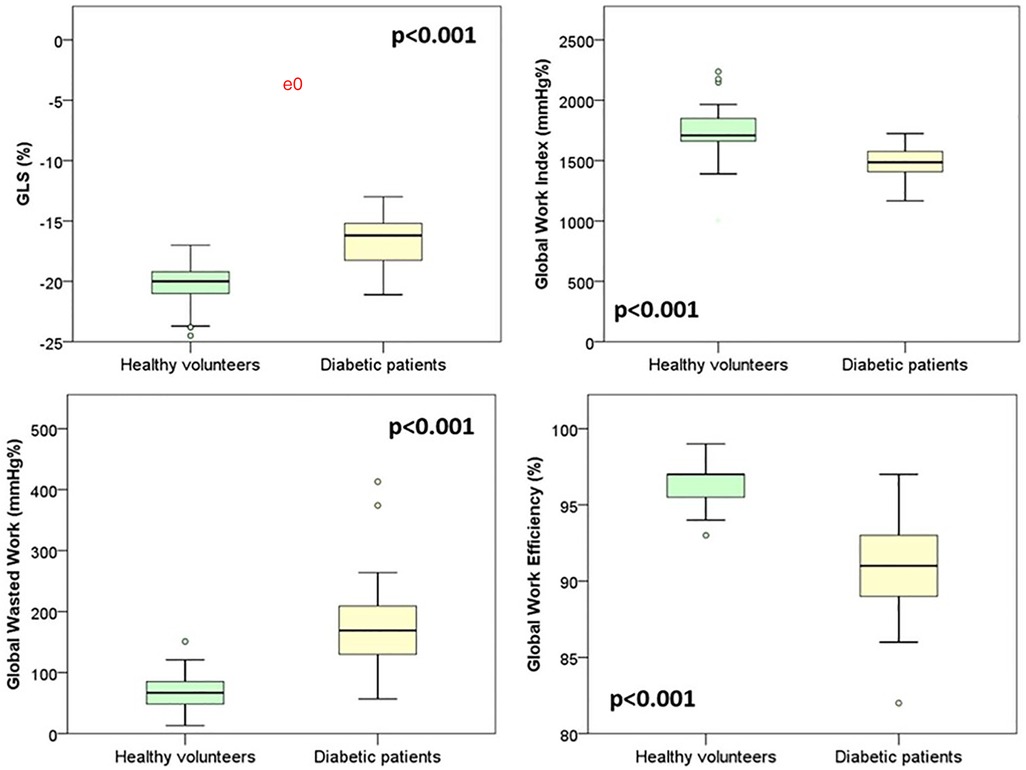
Figure 2. Comparison of speckle tracking and myocardial work data between diabetic patients and healthy volunteers.
4 Discussion
This paper presents the data from a cross-sectional study on non-invasive conventional and advanced echocardiographic assessment in adolescents diagnosed with type 1 diabetes mellitus for at least 10 years. We found that GLS, global work index, global wasted work, and global work efficiency were impaired in our population if compared to healthy volunteers. Moreover, conventional echocardiography showed a significative trend toward early impairment of diastolic parameters in patients with the worst glycemic control, and pulse wave velocity fell into the pathological range for hypertensive and pre-hypertensive patients.
To the best of our knowledge, this appears to be the first study to explore the existence of early cardiovascular complication markers using advanced echocardiography techniques (MW) in a population of adolescents with type 1 diabetes mellitus.
Regarding glycemic control assessment, our sample consistently fell outside the target values in the ISPAD guidelines indicative of good control and protection against the long-term risk of complications, for all examined parameters [HbA1c, time in range (TIR), time above range (TAR), time below range, coefficient of variation (CV)] (17, 18). Overall, the majority of subjects exhibited high glycemic variability, with a low TIR, reflected in HbA1c levels that averaged above the desirable target in specialized and technologically advanced settings (6.5%). This suggests a prolonged influence of this risk factor in most subjects.
Moreover, our sample showed also suboptimal pressure control. Comparing systolic and diastolic values with pediatric reference tables for age and height, 12 out of 31 patients (38.7%) showed significant blood pressure alterations: 6 were in the “elevated pressure” range (systolic or diastolic blood pressure >90th percentile but <95th percentile), while the other 6 had stage 1 hypertension (19). These data confirm the potential utility of afterload-independent cardiac function parameters.
Speckle tracking data from diabetic adolescents were compared with the data from 31 age- and sex-matched healthy volunteers enrolled in a previous study. Two samples were comparable in terms of age, weight, and height, whereas the diabetic adolescents seemed to have significantly higher BSA (p = 0.037) and increased diastolic pressures (p < 0.001) if compared to the healthy subjects. Nevertheless, since the literature suggests the independence of global longitudinal strain (GLS) from BSA, a comparison of the two samples was deemed feasible. The comparison revealed significantly lower (more positive) GLS in the diabetic adolescents cohort (−16.7% ± 2.2 in diabetic patients vs. −20.5% ± 1.9 in healthy volunteers, p < 0.001). Further investigation into myocardial work showed a significantly lower global work index (p < 0.001) and global work efficiency (p < 0.001) along with a higher global wasted work (p < 0.001), as if there was an increased energy loss and futile work during both contraction and isovolumetric relaxation. Surprisingly, GCW resulted comparable between diabetic patients and healthy volunteers. These data suggest that cardiac mechanical impairment is more attributable to a rise in futile work during systole and isovolumetric relaxation than to a loss of positive work in the same phases. Whether this pattern of subclinical dysfunction might be linked to the specific damages related to hyperglycemia remains to be understood.
The existing literature on myocardial work and adult diabetic patients is mostly focused on type 2 diabetes mellitus. In a recent study on type 2 diabetic adult patients, Liao et al. (20) observed decreased GLS, GWI, and GWE and increased GWW. Similar to our study, global constructive work was comparable between patients and controls. These changes observed in GLS and MW descriptive parameters are reversible by improving glycemic control (21).
These parameters significantly reflect myocardial involvement, and we therefore suggest their integration into the clinical follow-up of these patients.
Given the extensive literature documenting the predictive power of GLS for unfavorable long-term cardiovascular outcomes, our finding of reduced GLS among diabetic patients should raise particular concern since multiple risk factors may coincide during growth and adulthood in this patient category. Although, as previously mentioned, our sample did not exhibit statistically significant correlations or associations between GLS, global circumferential strain (GCS), and glycemic control parameters, a trend was observed based on our measurements: more negative GLS values in individuals with better glycemic control parameters and, conversely, more negative GCS values in individuals with worse glycemic control. This observation, consistent with the literature findings for other pathologies causing primary GLS reduction, may be attributed to an initial compensatory mechanism by GCS, which would be consistently increased in individuals with worse GLS (5). However, the validity of this explanatory hypothesis in the context of our sample remains to be demonstrated (22–27). The lack of correlation between speckle tracking data and glycemic control parameters in our opinion can be explained by the substantial homogeneity of our sample in terms of glycated hemoglobin, time in range, and coefficient of variation.
In line with the available literature on left ventricular hypertrophy with unfavorable cardiovascular outcomes and the onset of ventricular arrhythmias, GLS and GWW in our study exhibited a positive correlation with interventricular septum thickness (less negative GLS values for greater interventricular septum thickness; increased wasted work for higher septal thickness) (28, 29).
In literature, left ventricular thickness and cardiac mass are reported to show an early tendency to increase in diabetic patients. However, in our sample, cardiac mass did not differ significantly from that of healthy subjects from previous studies. This might suggest that subclinical alterations in myocardial function appear in the natural history of type 1 diabetes mellitus before actual hypertrophic adaptation occurs.
Concerning diastolic functionality parameters, no frank pathological alteration was evident in the echocardiographic parameters of individuals in our sample. However, TDI diastolic function parameters and left atrial diameters showed a deteriorating trend with worse glycemic control. Both septal and lateral E′ velocities were higher in individuals with TIR > 70% compared to those with lower TIR; lateral E′ velocity was significantly higher in individuals with onset HbA1c below 6.5%, and septal E′ velocity was significantly higher in individuals with CV ≤36%. Additionally, larger left atrial diameters were observed in individuals with HbA1c above 6.5%. A statistically significant correlation was found between left atrial diameter and TVAR and between septal E′ velocity and TVAR. These data align with the existing literature indicating that one of the earlier cardiovascular alterations in type 1 diabetes mellitus involves diastolic function parameters before ejection fraction impairment. The correlation between TVAR and these parameters is also explained by preclinical studies on the effects of hyperglycemia and its variations on tissues, demonstrating how even short-term and non-sustained glycemic alterations can be responsible for subclinical cardiac dysfunction (30–32). On the other hand, some studies in the literature show that even relatively short periods (e.g., 1 month) of improved glycemic control can lead to a significant improvement in cardiac performance (33).
Finally, this study also considered indices of vascular damage. Pulse wave velocity (PWV) is a sensitive marker of arterial stiffness and consequently cardiovascular outcomes. As in previous studies, our data showed no correlation between PWV and glycemic control parameters (34). On the other hand, the positive association between PWV and blood pressure values was confirmed (35–37). Comparing our data to the reference values in the literature, we found that PWV from our diabetic adolescents fell on average in between the 50th and 75th percentile (38). However, in patients with a pre-hypertensive state or first-degree hypertension, PWV fell into the pathological range reaching values superior to 8 m/s (>99th percentile of PWV) (38). These data suggest the necessity to raise our awareness toward hypertension and maximize our efforts to effectively screen our diabetic adolescents.
Although clinical manifestations occur in adulthood, atherosclerosis is a continuum process that begins early in childhood, especially if major risk factors, such as diabetes and hypertension, are present. Thus, it is particularly important to address cardiovascular risk factors early in life as clearly emphasized in the clinical practice guidelines. On the same note, there is a need for further research to fully understand the impact of T1D on the heart and blood vessels of adolescents. These investigations can contribute to the development of targeted preventive and therapeutic strategies to preserve cardiovascular health in this population of diabetic patients.
5 Limitations
This study has some limitations. The small number of individuals in our sample is the most relevant, as statistical analyses may not have highlighted results suitable for a comprehensive discussion due to the insufficient number of subjects. However, our patients represent a very well-selected cohort since the patients were diagnosed with diabetes for at least 10 years and showed comparable anthropometric features and glycemic control parameters. Moreover, the patients were scanned under the same conditions over a short amount of time.
6 Conclusions
Conventional echocardiography showed a significative trend toward early impairment of diastolic parameters in patients with the worst glycaemic control. Moreover, both global longitudinal strain and descriptive parameters of myocardial work were significantly altered in the sample examined compared to healthy individuals, highlighting a subclinical cardiac damage already present during adolescence. The analysis of pulse wave velocity showed pathologically increased arterial stiffness in diabetic patients with blood pressure above the 90th percentile. Therefore, PWV, GLS, and myocardial work can be useful tools in assessing cardiovascular function in diabetic patients, while preventive strategies should be implemented to maximize glycemic and pressure control effectiveness.
Additional investigations, with long-term follow-up and large-scale cohorts, will be necessary to correlate myocardial strain, myocardial work alteration, and pulse wave velocity with the clinical outcomes of this patient group.
Data availability statement
The raw data supporting the conclusions of this article will be made available by the authors, without undue reservation.
Ethics statement
The studies involving humans were approved by the Ethical Committee of the Central-Eastern Veneto Area. The studies were conducted in accordance with the local legislation and institutional requirements. Written informed consent for participation in this study was provided by the participants' legal guardians/next of kin.
Author contributions
MG: Formal Analysis, Methodology, Writing – original draft, Conceptualization, Investigation, Writing – review & editing. IC: Methodology, Writing – original draft, Writing – review & editing, Formal Analysis. JS: Conceptualization, Supervision, Writing – review & editing. AP: Writing – review & editing. JF: Writing – review & editing. MA: Conceptualization, Methodology, Writing – review & editing. JG: Writing – review & editing, Methodology, Supervision, Conceptualization. CM: Conceptualization, Methodology, Supervision, Writing – review & editing. BC: Methodology, Supervision, Writing – review & editing. GS: Conceptualization, Methodology, Supervision, Writing – review & editing.
Funding
The authors declare financial support was received for the research, authorship, and/or publication of this article. University of Padua supported the publication of this paper. MG was supported with a Pediatric residency grant from University of Padua. IC, AP and JF were supported with a PhD grant from the same University.
Conflict of interest
The authors declare that the research was conducted in the absence of any commercial or financial relationships that could be construed as a potential conflict of interest.
The authors declared that they were an editorial board member of Frontiers, at the time of submission. This had no impact on the peer review process and the final decision.
Publisher's note
All claims expressed in this article are solely those of the authors and do not necessarily represent those of their affiliated organizations, or those of the publisher, the editors and the reviewers. Any product that may be evaluated in this article, or claim that may be made by its manufacturer, is not guaranteed or endorsed by the publisher.
Supplementary material
The Supplementary Material for this article can be found online at: https://www.frontiersin.org/articles/10.3389/fcvm.2024.1476456/full#supplementary-material
Supplementary Figure 1 | A summary outline of the study design and its main results are reported. DM1, type 1 Diabetes; HbA1, glycated haemoglobin; TIR, time in range; TAR, time above range; TVAR, time very above range; GLS, global longitudinal strain; PWV pulse wave velocity; GWI, global work index; GCW, global constructive work; GWW, global wasted work; GWE, global work efficiency; E/e', inflow to relaxation velocity ratio.
References
1. de Ferranti SD, de Boer IH, Fonseca V, Fox CS, Golden SH, Lavie CJ, et al. Type 1 diabetes mellitus and cardiovascular disease: a scientific statement from the American Heart Association and American Diabetes Association. Diabetes Care. (2014) 37:2843–63. doi: 10.2337/dc14-1720
2. Luévano-Contreras C, Gómez-Ojeda A, Macías-Cervantes MH, MaE G-S. Dietary advanced glycation end products and cardiometabolic risk. Curr Diab Rep. (2017) 17:63. doi: 10.1007/s11892-017-0891-2
3. Kimball TR, Daniels SR, Khoury PR, Magnotti RA, Turner AM, Dolan LM. Cardiovascular status in young patients with insulin-dependent diabetes mellitus. Circulation. (1994) 90:357–61. doi: 10.1161/01.CIR.90.1.357
4. Suys BE, Katier N, Rooman RPA, Matthys D, Op De Beeck L, Du Caju MVL, et al. Female children and adolescents with type 1 diabetes have more pronounced early echocardiographic signs of diabetic cardiomyopathy. Diabetes Care. (2004) 27:1947–53. doi: 10.2337/diacare.27.8.1947
5. Fang ZY, Leano R, Marwick TH. Relationship between longitudinal and radial contractility in subclinical diabetic heart disease. Clin Sci. (2004) 106:53–60. doi: 10.1042/CS20030153
6. Kolasa M, Olejnik A, Rusak E, Chobot A. Atherosclerosis: risk assessment and the role of aiming for optimal glycaemic control in young patients with type 1 diabetes. Pediatr Endocrinol Diabetes Metab. (2023) 29:42–7. doi: 10.5114/pedm.2022.122546
7. Kao KT, Sabin MA. Type 2 diabetes mellitus in children and adolescents. Aust Fam Physician. (2016) 45(6):401–6.27622231
8. Marzlin N, Hays AG, Peters M, Kaminski A, Roemer S, O’Leary P, et al. Myocardial work in echocardiography. Circ Cardiovasc Imaging. (2023) 16:198–9. doi: 10.1161/CIRCIMAGING.122.014419
9. Libman I, Haynes A, Lyons S, Pradeep P, Rwagasor E, Tung JY, et al. ISPAD Clinical Practice Consensus Guidelines 2022: definition, epidemiology, and classification of diabetes in children and adolescents. Pediatr Diabetes. (2022) 23:1160–74. doi: 10.1111/pedi.13454
10. Sabatino J, Leo I, Strangio A, La Bella S, Borrelli N, Avesani M, et al. Echocardiographic normal reference ranges for non-invasive myocardial work parameters in pediatric age: results from an international multi-center study. Front Cardiovasc Med. (2022) 9:2. doi: 10.3389/fcvm.2022.792622
11. National High Blood Pressure Education Program Working Group on High Blood Pressure in Children and Adolescents. The fourth report on the diagnosis, evaluation, and treatment of high blood pressure in children and adolescents. Pediatrics. (2004) 114:555–76. doi: 10.1542/peds.114.S2.555
12. Khoury PR, Mitsnefes M, Daniels SR, Kimball TR. Age-specific reference intervals for indexed left ventricular mass in children. J Am Soc Echocardiogr. (2009) 22:709–14. doi: 10.1016/j.echo.2009.03.003
13. Sabatino J, Di Salvo G, Krupickova S, Fraisse A, Prota C, Bucciarelli V, et al. Left ventricular twist mechanics to identify left ventricular noncompaction in childhood. Circ Cardiovasc Imaging. (2019) 12:3. doi: 10.1161/CIRCIMAGING.118.007805
14. Badano LP, Kolias TJ, Muraru D, Abraham TP, Aurigemma G, Edvardsen T, et al. Standardization of left atrial, right ventricular, and right atrial deformation imaging using two-dimensional speckle tracking echocardiography: a consensus document of the EACVI/ASE/industry task force to standardize deformation imaging. Eur Heart J Cardiovasc Imaging. (2018) 19:591–600. doi: 10.1093/ehjci/jey042
15. Voigt J-U, Mălăescu G-G, Haugaa K, Badano L. How to do LA strain. Eur Heart J Cardiovasc Imaging. (2020) 21:715–7. doi: 10.1093/ehjci/jeaa091
16. Williams B, Mancia G, Spiering W, Agabiti Rosei E, Azizi M, Burnier M, et al. ESC/ESH guidelines for the management of arterial hypertension. Eur Heart J. (2018) 2018(39):3021–104. doi: 10.1093/eurheartj/ehy339
17. Bjornstad P, Dart A, Donaghue KC, Dost A, Feldman EL, Tan GS, et al. ISPAD Clinical Practice Consensus Guidelines 2022: microvascular and macrovascular complications in children and adolescents with diabetes. Pediatr Diabetes. (2022) 23:1432–50. doi: 10.1111/pedi.13444
18. de Bock M, Codner E, Craig ME, Huynh T, Maahs DM, Mahmud FH, et al. ISPAD Clinical Practice Consensus Guidelines 2022: glycemic targets and glucose monitoring for children, adolescents, and young people with diabetes. Pediatr Diabetes. (2022) 23:1270–6. doi: 10.1111/pedi.13455
19. Flynn JT, Kaelber DC, Baker-Smith CM, Blowey D, Carroll AE, Daniels SR, et al. Clinical practice guideline for screening and management of high blood pressure in children and adolescents. Pediatrics. (2017) 140:1271. doi: 10.1542/peds.2017-1904
20. Liao L, Shi B, Ding Z, Chen L, Dong F, Li J, et al. Echocardiographic study of myocardial work in patients with type 2 diabetes mellitus. BMC Cardiovasc Disord. (2022) 22:59. doi: 10.1186/s12872-022-02482-3
21. Russo V, Malvezzi Caracciolo D’Aquino M, Caturano A, Scognamiglio G, Pezzullo E, Fabiani D, et al. Improvement of global longitudinal strain and myocardial work in type 2 diabetes patients on sodium–glucose cotransporter 2 inhibitors therapy. J Cardiovasc Pharmacol. (2023) 82:196–200. doi: 10.1097/FJC.0000000000001450
22. Motoki H, Borowski AG, Shrestha K, Troughton RW, Tang WHW, Thomas JD, et al. Incremental prognostic value of assessing left ventricular myocardial mechanics in patients with chronic systolic heart failure. J Am Coll Cardiol. (2012) 60:2074–81. doi: 10.1016/j.jacc.2012.07.047
23. Munk K, Andersen NH, Terkelsen CJ, Bibby BM, Johnsen SP, Bøtker HE, et al. Global left ventricular longitudinal systolic strain for early risk assessment in patients with acute myocardial infarction treated with primary percutaneous intervention. J Am Soc Echocardiogr. (2012) 25:644–51. doi: 10.1016/j.echo.2012.02.003
24. Dahl JS, Videbæk L, Poulsen MK, Rudbæk TR, Pellikka PA, Møller JE. Global strain in severe aortic valve stenosis. Circ Cardiovasc Imaging. (2012) 5:613–20. doi: 10.1161/CIRCIMAGING.112.973834
25. Buss SJ, Emami M, Mereles D, Korosoglou G, Kristen A V, Voss A, et al. Longitudinal left ventricular function for prediction of survival in systemic light-chain amyloidosis. J Am Coll Cardiol. (2012) 60:1067–76. doi: 10.1016/j.jacc.2012.04.043
26. DeAnda A, Komeda M, Moon MR, Green GR, Bolger AF, Nikolic SD, et al. Estimation of regional left ventricular wall stresses in intact canine hearts. Am J Physiol. (1998) 275:H1879–85. doi: 10.1152/ajpheart.1998.275.5.H1879
27. Heng MK, Janz RF, Jobin J. Estimation of regional stress in the left ventricular septum and free wall: an echocardiographic study suggesting a mechanism for asymmetric septal hypertrophy. Am Heart J. (1985) 110:84–90. doi: 10.1016/0002-8703(85)90519-8
28. Verdecchia P, Carini G, Circo A, Dovellini E, Giovannini E, Lombardo M, et al. Left ventricular mass and cardiovascular morbidity in essential hypertension: the MAVI study. J Am Coll Cardiol. (2001) 38:1829–35. doi: 10.1016/S0735-1097(01)01663-1
29. Draper TS, Silver JS, Gaasch WH. Adverse structural remodeling of the left ventricle and ventricular arrhythmias in patients with depressed ejection fraction. J Card Fail. (2015) 21:97–102. doi: 10.1016/j.cardfail.2014.10.018
30. Tao L, Huang X, Xu M, Yang L, Hua F. Mir-144 protects the heart from hyperglycemia-induced injury by regulating mitochondrial biogenesis and cardiomyocyte apoptosis. FASEB J. (2020) 34:2173–97. doi: 10.1096/fj.201901838R
31. Wu S, Lu Q, Ding Y, Wu Y, Qiu Y, Wang P, et al. Hyperglycemia-driven inhibition of AMP-activated protein kinase α2 induces diabetic cardiomyopathy by promoting mitochondria-associated endoplasmic reticulum membranes in vivo. Circulation. (2019) 139:1913–36. doi: 10.1161/CIRCULATIONAHA.118.033552
32. Aboalgasm H, Ballo R, Mkatazo T, Gwanyanya A. Hyperglycaemia-induced contractile dysfunction and apoptosis in cardiomyocyte-like pulsatile cells derived from mouse embryonic stem cells. Cardiovasc Toxicol. (2021) 21:695–709. doi: 10.1007/s12012-021-09660-3
33. Hensel KO, Grimmer F, Jenke AC, Wirth S, Heusch A. The influence of real-time blood glucose levels on left ventricular myocardial strain and strain rate in pediatric patients with type 1 diabetes mellitus—a speckle tracking echocardiography study. BMC Cardiovasc Disord. (2015) 15:175. doi: 10.1186/s12872-015-0171-5
34. Reusz GS, Cseprekal O, Temmar M, Kis E, Cherif AB, Thaleb A, et al. Reference values of pulse wave velocity in healthy children and teenagers. Hypertension. (2010) 56:217–24. doi: 10.1161/HYPERTENSIONAHA.110.152686
35. Bechlioulis A, Vakalis K, Naka KK, Bourantas C V, Papamichael ND, Kotsia A, et al. Increased aortic pulse wave velocity is associated with the presence of angiographic coronary artery disease in overweight and obese patients. Am J Hypertens. (2013) 26:265–70. doi: 10.1093/ajh/hps039
36. Vakalis K, Bechlioulis A, Naka KK, Pappas K, Katsouras CS, Michalis LK. Clinical utility of digital volume pulse analysis in prediction of cardiovascular risk and the presence of angiographic coronary artery disease. Artery Res. (2014) 9:33. doi: 10.1016/j.artres.2014.10.001
37. Vlachopoulos C, Aznaouridis K, Stefanadis C. Clinical appraisal of arterial stiffness: the argonauts in front of the golden fleece. Heart. (2006) 92:1544–50. doi: 10.1136/hrt.2005.067025
Keywords: GLS, myocardial work, type 1 diabetes mellitus, diabetic adolescents, advanced echocardiography
Citation: Ghirardo M, Cattapan I, Sabatino J, Pozza A, Fumanelli J, Avesani M, Gutierrez De Rubalcava Doblas J, Moretti C, Castaldi B and Di Salvo G (2025) Cardiovascular function shows early impairment in asymptomatic adolescents diagnosed with type 1 diabetes mellitus: an ultrasound-derived myocardial work study. Front. Cardiovasc. Med. 11:1476456. doi: 10.3389/fcvm.2024.1476456
Received: 5 August 2024; Accepted: 26 September 2024;
Published: 5 February 2025.
Edited by:
Ciro Santoro, Federico II University Hospital, ItalyReviewed by:
Lucia La Mura, Federico II University Hospital, ItalyMaria Concetta Pastore, University of Siena, Italy
Copyright: © 2025 Ghirardo, Cattapan, Sabatino, Pozza, Fumanelli, Avesani, Gutierrez De Rubalcava Doblas, Moretti, Castaldi and Di Salvo. This is an open-access article distributed under the terms of the Creative Commons Attribution License (CC BY). The use, distribution or reproduction in other forums is permitted, provided the original author(s) and the copyright owner(s) are credited and that the original publication in this journal is cited, in accordance with accepted academic practice. No use, distribution or reproduction is permitted which does not comply with these terms.
*Correspondence: Irene Cattapan, aXJlbmUuY2F0dGFwYW5Ac3R1ZGVudGkudW5pcGQuaXQ=
†These authors share first authorship