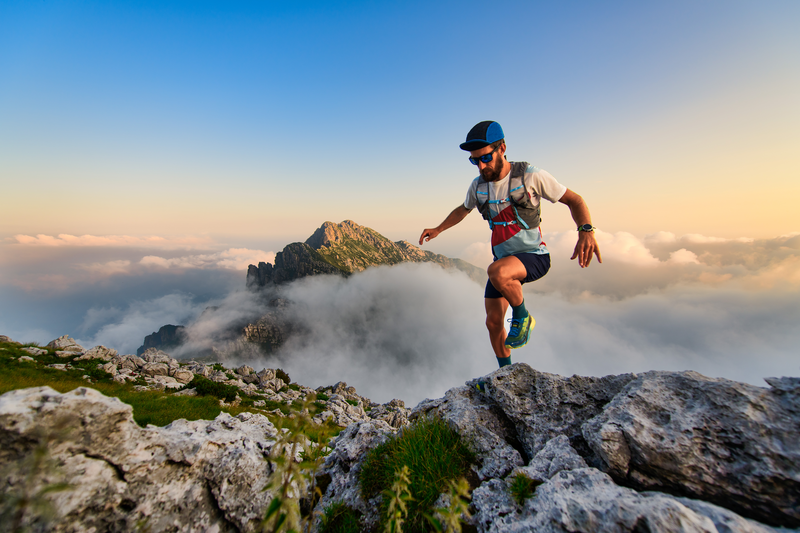
95% of researchers rate our articles as excellent or good
Learn more about the work of our research integrity team to safeguard the quality of each article we publish.
Find out more
ORIGINAL RESEARCH article
Front. Cardiovasc. Med. , 14 January 2025
Sec. Coronary Artery Disease
Volume 11 - 2024 | https://doi.org/10.3389/fcvm.2024.1466961
Background: The pharmacoinvasive (PhI) strategy is the standard-of-care for ST-elevation myocardial infarction (STEMI) patients when primary percutaneous coronary intervention (pPCI) is unfeasible. Optimal timing for post-fibrinolytic PCI (lysis-PCI) remains elusive. Therefore, this study aimed to assess the clinical and economic impacts of early vs. delayed lysis-PCI in patients with STEMI.
Methods: This retrospective cohort study included 1,043 STEMI patients classified by lysis-PCI timing. The primary outcome was in-hospital major adverse cardiovascular events (4p-MACE), with secondary outcomes such as 3p-MACE, in-hospital mortality, and costs. Multivariable logistic regression models were used to assess the association between lysis-PCI timing and outcomes. Cost analyses were conducted from the perspective of Brazilian public healthcare system, with values converted to international dollars (Int$) for broader applicability.
Results: Every 4-h delay in lysis-PCI was associated with a 44% reduction in in-hospital mortality [OR = 0.560 (95% CI: 0.381–0.775); p = 0.001] and a 13% decrease in 4p-MACE [OR = 0.877 (95% CI: 0.811–0.948); p = 0.001]. Additionally, 4-h delay in lysis-PCI was also associated with a significant reduction in in-hospital costs (Int$916.20 ± 99) and disease-induced years of productivity lost (β = −41.79 ± 151 years; p = 0.001). These significant trends remained consistent even after adjusting for confounders and applying propensity score matching. Older adults (aged ≥80) experienced an increase in 3p-MACE with earlier lysis-PCI.
Conclusion: Delaying lysis-PCI was found to be associated with reduced in-hospital cardiovascular adverse events and lower costs, particularly among older adults. Further research should develop evidence-based lysis-PCI protocols that optimize both clinical outcomes and cost-effectiveness.
Cardiovascular disease remains the preeminent global health threat, ending 39 lives every second as of 2022 (1). Within this domain, ischemic heart disease (IHD) ranks as the most lethal, accounting for nearly half of all cardiovascular deaths (1). Although advancements have been made in reducing the incidence of ST-segment elevation myocardial infarction (STEMI), the US still contends a stubbornly high mortality rate of 7.5% (2, 3), in stark contrast to the declining trends observed in non-STEMI deaths (4).
STEMI is the most time-sensitive acute coronary syndrome (5, 6), demanding rapid reperfusion interventions to save lives and mitigate disabilities (3). Evidence supports that prompt intervention significantly diminishes the severity of STEMI (7, 8), reduces mortality (8–10), and healthcare expenditures (10), highlighting the critical need for rapid restoration of coronary blood flow. Primary percutaneous coronary intervention (pPCI) within 90–120 minutes from the initial medical contact remains the gold standard treatment (11, 12). Despite improvements in pPCI access across the US (13), a substantial segment of the American population remains underserved (11). More than a quarter of STEMI cases are identified at hospitals that lack pPCI capabilities (11), and a majority of adult intensive care units are not equipped for post-procedure care (12). Alarmingly, two-thirds of US hospitals fail to meet the guideline-recommended time metrics, with 35% of transferred patients not achieving the vital 120-minute door-to-balloon benchmark (9).
In regions where pPCI is unfeasible, the pharmaco-invasive (PhI) strategy – characterized by post-fibrinolysis PCI within the first 24 hours – has been adopted as the standard of care for STEMI (6). This strategy is demonstrably superior to delayed elective post-fibrinolysis PCI, yielding reductions in cardiac mortality, reinfarction rates, and the need for further revascularization (11, 14).
Guidelines from leading cardiology associations recommend PCI timing post-fibrinolysis within windows that vary slightly: 2–24 hours according to the European Society of Cardiology, and 3–24 hours as per the American Heart Association (4, 6). Meta-analysis indicated that a 4-hour window post-fibrinolysis PCI is safe and optimal, though earlier interventions in aged adults increased the risk of bleeding, complications, and mortality (15). The most robust available evidence points to a limitation: the included clinical trials do not reflect the broader patient population, excluding those over 75, those with severe renal dysfunction, or those receiving PCI after 18 hours post-fibrinolysis. Thus, a definitive consensus on the safest and most effective timing for the PhI strategy remains elusive within the recommended 22-hours window.
The challenge of providing timely pPCI is compounded by the requirement for specialized facilities and highly skilled medical teams (16), which are not universally available, particularly in low- and middle-income countries (LMICs) (15). These regions, where over 75% of global cardiovascular deaths disproportionately occur (17, 18), place mounting pressure on healthcare systems. Although the PhI strategy has emerged as the primary treatment modality in LMICs, significant gaps remain in our understanding of the optimal timing for post-fibrinolysis PCI and its clinical and economic impacts (16). This study, therefore, aims to address this critical evidence gap by assessing the clinical and economic outcomes associated with varying intervals between fibrinolytic therapy administration and PCI initiation – commonly known as “lysis-PCI time” – among STEMI patients in a real-world PhI strategy setting.
This multicenter retrospective cohort study encompassed all patients diagnosed with STEMI who underwent a PhI strategy at two high-complexity public hospitals, namely Hospital de Base do Distrito Federal and Instituto de Cardiologia do Distrito Federal, in Brasília, Brazil, between January 2009 and December 2019. These facilities collectively managed 99.8% of public system catheterizations for acute coronary syndromes in the Brazilian capital.
Participants were included if they: (i) were 18 years or older; (ii) had a confirmed electrocardiogram diagnosis of STEMI defined as ST-segment elevation of at least 1 mm (frontal plane) or 2 mm (horizontal plane) in 2 contiguous leads, or a newly presumed left bundle branch block or right bundle branch block; (iii) showed laboratory evidence of myocardial necrosis, indicated by an increase in at least one value above the 99th percentile reference limit of CK-MB (25 U/L) and troponin I (0.04 ng/mL), followed by a subsequent decline in both; (iv) received fibrinolytic therapy within 24 hours of symptom onset; (v) underwent PCI within 24 hours after fibrinolytic therapy. Patients who only received fibrinolytic therapy followed by rescue PCI were excluded. The patient selection process is outlined in Supplementary Figure S1.
Successful fibrinolysis was defined by clinical and electrocardiographic resolution, including significant chest pain relief, ≥50% ST-segment reduction in the lead with the highest elevation within 90 minutes post-fibrinolysis, and the absence of hemodynamic instability or persistent ischemic symptoms (19). Incorporating strict criteria for successful fibrinolysis ensures a homogeneous cohort, which is critical for isolating the effects of fibrinolysis-to-PCI timing on outcomes.
The study protocol was approved by the local Institutional Review Board (IRB) (number CAAE 28530919.0.1001.8153), and a waiver of informed consent was granted, adhering to the ethical principles outlined in the Declaration of Helsinki.
Demographic, clinical, biochemical, and prescribed medications/therapies data were collected from electronic medical records by trained investigators using a standardized protocol. “Needle time”, the moment when fibrinolytic drugs were administered, was designated as the index time. Baseline characteristics were compiled up to one year prior to the index time.
Anatomical severity and the extent of coronary atherosclerotic disease, angiographic treatments, and left ventricular function were assessed through written reports. Severity of stenosis was classified as arterial lumen reduction >70% for epicardial vessels and >50% for the left main coronary artery. Multivessel disease was defined by the presence of >70% stenosis in three or more major epicardial vessels or >50% in the left main coronary artery. Left ventricular function was considered preserved if contractility was normal and classified as dysfunctional in the presence of hypokinesia or akinesia.
The primary endpoint was a composite of in-hospital major adverse cardiovascular events (4p-MACE), which included death, incident myocardial infarction, stroke, and heart failure symptoms arising over 24 hours post-STEMI. Secondary outcomes were: (i) 3p-MACE, comprising in-hospital death, recurrent myocardial infarction, ischemic stroke; (ii) in-hospital death alone; and (iii) cost outcomes including total, direct, and indirect in-hospital costs.
Death identification was conducted by consulting the Brazilian National Death Registry (SIM/SUS), which recorded causes of death through mandatory coding on death certificates.
A comprehensive analysis of direct in-hospital costs, including expenses for procedures [e.g., PCI, coronary artery bypass graft surgery (CABG), intensive care unit (ICU) stays, and hemodialysis] was performed. Costs were evaluated from the perspective of the Brazilian Unified Health System (SUS) payer, with reimbursement based on standardized national rates from the SUS price list (details in Supplementary Table 1). Costs, denominated in Brazilian currency (R$), were converted to international dollars (Int$) using a purchasing power parity (PPP) conversion factor of 2.36. The methodology for extracting data from the SUS database has been previously described (18).
The indirect impact of STEMI on this population was evaluated using disease-induced years of productivity lost (DIYPL), a metric quantifying cumulative years of workforce participation lost due to health conditions, assuming retirement at 65 years (15). DIYPL provides insights into the societal impact of STEMI, capturing both disease-related productivity losses and premature mortality.
Study participants were categorized into three lysis-PCI time subgroups based on the tertiles of lysis-PCI time: ≤575 minutes (≤9.6 hours), 576–1,079 minutes (9.7–17.9 hours), and ≥1,080 minutes (≥18 hours).
Multiple imputation techniques were employed to handle missing values. Predictive mean matching model was used for numeric variables, logistic regression (logreg) for binary variables with two levels, and Bayesian polytomous regression (polyreg) for factor variables with two or more levels. The imputed values, residual distribution, and convergence coefficients were thoroughly checked. Missing values for the outcomes were not imputed.
Data distribution was evaluated using histograms, scatterplots, and normality tests (Kolmogorov-Smirnov or Shapiro-Wilk). Comparative statistical analysis varied based on the variable nature. Categorical variables were analyzed using chi-squared tests, while continuous variables were assessed using one-way analysis of variance (ANOVA) for normally distributed data or the Kruskal-Wallis test for non-normally distributed data. Normally distributed baseline data were expressed as means ± standard deviations, while non-normally distributed data were presented as medians [IQR] (interquartile ranges).
Logistic regression models assessed the association of lysis-PCI time tertiles with primary and secondary outcomes. Univariable regression models provided initial trends [odds ratios (OR), 95% confidence intervals (95%CI)]. Variables showing significant differences between subgroups at baseline were selected as covariates for multivariable adjustment. Additionally, a stepwise logistic multivariable regression model was used to identify key predictors of outcomes. Given the observational study design, propensity score matching (PSM) using a genetic matching algorithm (20–22) balanced baseline characteristics and weighted covariates between treatment (first tertile: ≤9.6 hours lysis-PCI) and control (second and third tertiles: > 9.6 hours lysis-PCI) groups. The Mahalanobis distance score and logistic regression machine learning identified matched pairs at a 1:1 ratio.
Age-specific analysis examined potential associations between lysis-PCI time and 30-day 4p-/3p-MACE odds using generalized linear models. An interaction term between age groups (<65, 65–80, and >80 years) and lysis-PCI time was included to assess whether the relationship between lysis-PCI time and MACE differed across age subgroups. Cubic splines were used to visualize the associations, allowing for a flexible, non-linear exploration of these interactions within each age group.
Statistical significance was defined as a p-value <0.05. Analyses were conducted using RStudio for Mac (version 1.1.463) with RGui language (version 4.0.1).
A cohort of 1,043 patients diagnosed with STEMI undergoing a PhI strategy was established based on eligibility criteria. Patients were stratified into tertiles of lysis-PCI time: ≤9.6 hours (n = 344), 9.7–17.9 hours (n = 340), and ≥18 hours (n = 359). Overall, 56% of the cohort was male, and 64.7% were under the age of 65. Comprehensive baseline clinical and biochemical characteristics are presented in Table 1.
Comparative statistical analysis revealed that patients in the ≤9.6 hours subgroup had significantly faster interventions, including shorter door-lysis and lysis-PCI durations, as well as quicker tertiary care transfers. Moreover, they more frequently received glycoprotein IIb/IIIa inhibitors and used drug-eluting stents less often. Conversely, the ≥18 hours subgroup had significantly lower peak levels of troponin T, HbA1c, TSH, and LDL-C. No other significant baseline differences were observed between the study subgroups (Table 1).
Further analysis identified differences in clinical and cost outcomes between the subgroups (Table 2). The ≤9.6 hours lysis-PCI window subgroup had significantly higher rates of in-hospital death, heart failure symptoms, left ventricular failure shock, cardiac arrest beyond the first 24 hours, major bleeding events, and 4p-MACE. This subgroup also incurred higher in-hospital costs and disease-related lost productivity years (Table 2).
To address the limitations of the observational study design concerning heterogeneity of baseline characteristics in the subgroups, we utilized PS-matching through a genetic algorithm. This method balanced baseline characteristics and weighted covariate between the treatment (first tertile: ≤9.6 hours lysis-PCI) and control (second and third tertiles: >9.6 hours lysis-PCI) subgroups. As shown in Supplementary Figure S2, PS-matching significantly improved the balance of both continuous and categorical covariates (Supplementary Table 2).
The PS-matching model included age, hypertension, type 2 diabetes, family history, angina, cocaine abuse, bundle branch blocks, door-to-needle time, and left anterior descending identification. The variables measured were drug-eluting stent count, presence of no-reflow, left ventricle ejection fraction (LVEF), admission heart rate, use of glycoprotein IIb/IIIa inhibitors, blood glucose, HbA1c, troponin, thyroid-stimulating hormone, and GRACE in-hospital mortality score.
In pairwise analysis, the treatment group showed an increased prevalence of prior stroke, while the control group exhibited more hypothyroidism, previous PCI, and elevated triglycerides. The control group also had longer lysis-to-hospital and lysis-to-PCI intervals, likely due these variables not being included in the PS model.
As expected, the control group experienced significantly longer door-lysis time and pain-to-lysis delays, slower transfers to tertiary hospitals post-lysis, and longer lysis-to-PCI procedures compared to the treatment group.
Three logistic regression models assessed the association between lysis-PCI time and 4p-MACE. The models included: (i) univariable (entire cohort); (ii) univariable (PS-matched cohort); and (iii) multivariable stepwise regression. Detailed results are shown in Table 3.
Table 3. Logistic regression models for in-hospital death (18 events), 3p-MACE (40 events), and 4p-MACE (357 events) as dependent variables.
Univariable analysis revealed a 12.3% reduction in 4p-MACE risk per 4-hour delay in lysis-PCI [OR: 0.877 (95% CI: 0.811–0.948); p = 0.001]. This relationship persisted in the multivariable stepwise regression and PS-matched analyses, with an 11% and 7.1% risk decrease per 4-hour delay, respectively (Table 3).
Every 4-hour lysis-PCI delay reduced the risk of 3p-MACE by 14.4% [OR: 0.856 (0.705–0.973); p = 0.012]. This remained significant across analyses, with an 11.7% decrease in 3p-MACE risk per 4-hour lysis-PCI delay in the PS-matched cohort (Table 3). Additionally, a 4-hour delay was associated with a 44.0% reduction in in-hospital death risk [OR: 0.560 (0.381–0.775); p = 0.001]. This association persisted across analyses, with a 27.9% decrease in in-hospital death risk per 4-hour delay in the PS-matched cohort (Table 3).
The ≥18-hour lysis-PCI subgroup incurred significantly lower in-hospital costs [Int$ 29,400 (2,600); p = 0.013] and fewer disease-induced productivity loss years [293.8 ± 277.8 years; p < 0.001] compared to other subgroups (Table 2).
Every 4-hour lysis-PCI delay was associated with reduced productivity loss years (β −41.79 ± 150.55 years; p = 0.001) and in-hospital costs [Int$ −916.20 ± 98.51; p < 0.001]. PS-matched analysis remained consistent, with 4-hour lysis-PCI delays reducing productivity loss [β −31.35 ± 137.36 years; p = 0.008] and in-hospital costs [Int$ −847.5 ± 99.79; p = 0.007]. Both models showed an independent, inverse association between lysis-PCI time and disease burden and costs (Table 3; Supplementary Table 3).
Having demonstrated a robust and consistent association between lysis-PCI time, outcomes, and costs, we examined variations in 30-day 3p-/4p-MACE probabilities across age groups using generalized linear models. Notably, STEMI patients over 80 years old exhibited a statistically significant higher 3p-MACE risk with early lysis-PCI (p = 0.010, Figure 1), with probabilities converging only beyond 500 min of lysis-PCI time, as evidenced by overlapping confidence intervals (Figure 1). Furthermore, early lysis-PCI in patients over 80 years showed an inverse trend with the 30-day 4p-MACE probability (Supplementary Figure S3), although this result did not reach statistical significance.
Figure 1. Generalized linear models of 30-day 3-point major adverse cardiovascular events (3p-MACE) probability distribution by lysis-PCI time across different age groups.
To the best of our knowledge, this study is a pioneering effort in exploring real-world clinical and economic impacts of the fibrinolytic therapy-PCI timing gap for STEMI patients. Our research unveils robust, consistent evidence that ≤9.6-hour lysis-PCI is associated with increased risks of in-hospital mortality, 3p/4p-MACE, and elevated costs, regardless of the statistical approach employed. Specifically, every 4-hour lysis-PCI delay is associated with a 12.3% and 14.4% lower 4p- and 3p-MACE risk, respectively, and a substantial 44.0% reduction in in-hospital death risk.
Analysis of patients aged 80 years and older suggests that early lysis-PCI is associated with a higher 30-day probability of experiencing 3p-MACE, underscoring the need for personalized treatment strategies to mitigate risk in older STEMI populations. Our data also demonstrate that a ≤9.6-hour lysis-PCI is associated with greater direct and indirect costs. Importantly, these findings are consistent across age groups, with the relationship between lysis-PCI delays and reduced cardiovascular risk persisting even after excluding patients aged 80 years and older, known to be at higher risk for MACE (data not shown) (17, 23).
The heightened severity of symptoms observed in the ≤9.6-hour subgroup, including higher rates of in-hospital death, heart failure, cardiogenic shock, cardiac arrest, and major bleeding, supports the hypothesis that a shorter lysis-PCI interval may lead to more adverse outcomes and complications. This implies that the risks associated with rapid intervention might outweigh the potential benefits. These findings emphasize the importance of carefully considering the timing of PCI following fibrinolysis to optimize outcomes.
Although post-PCI TIMI 3 flow was slightly higher in the ≤9.6 hours group compared to the 9.7–17.9 hours and ≥18 hours groups, this observation was not accompanied by a proportional improvement in clinical outcomes. The myocardial blush grade (MBG), a key indicator of microvascular reperfusion and distal thrombotic burden (24), was worse in the ≤9.6 hours group, with a higher frequency of MBG 0 and 1. This discrepancy highlights the phenomenon of no-reflow, where the artery achieves patency (TIMI 3 flow) but microvascular obstruction limits effective perfusion (25). Furthermore, reduced contrast washout velocity, likely due to distal thrombi or microvascular damage, reinforces the disconnect between epicardial flow and myocardial reperfusion (26). These findings emphasize that achieving TIMI 3 flow does not guarantee improved prognosis, and that microvascular dysfunction plays a critical role in patient outcomes (27). While these trends were not statistically significant, they underscore the complexity of managing patients undergoing fibrinolysis followed by PCI and highlight the need for additional studies focusing on microcirculatory and inflammatory factors.
For STEMI management, prompt PCI is preferred due to its advantages over fibrinolytic therapy, such as superior infarct-artery patency, TIMI flow, and lower rates of complication like bleeding, ischemia, reinfarction, repeat revascularization, intracranial hemorrhage, and mortality (28). However, the accessibility of early PCI, which relies on specialized facilities and skilled teams, is limited, particularly in LMICs. For example, only 12.5% of Brazilian STEMI cases receive pPCI (29).
In contexts with limited resources, fibrinolytic therapy remains the first-line therapy for reducing STEMI mortality when administered within 8–12 hours of symptom onset (29). Combining fibrinolysis with routine early PCI improves outcomes where pPCI capabilities are lacking, although bleeding risks persist (30). Despite the growing adoption of pharmaco-invasive strategy, the optimal lysis-PCI interval for the best clinical and economic outcomes remains uncertain.
Our findings contest the prevailing view that minimizing myocardial ischemia through earlier reperfusion invariably improves outcomes (31). The mechanisms by which early lysis-PCI leads to increased MACE, mortality, and cost may involve heightened risks of major bleeding and no-reflow events. Our diverse cohort, which included about 5% of patients over 80 years and 5% with chronic kidney disease, highlights conditions known to increase post-fibrinolytic bleeding risk (23).
While our findings highlight the potential risks of early lysis-PCI, particularly in patients over 80 years old, it is important to emphasize that this study is observational and serves to generate hypotheses rather than provide definitive evidence to alter current guidelines or clinical practice. The small size of the 80+ subgroup, coupled with inherent survivorship bias due to their higher mortality rates, underscores the need for further investigation in larger cohorts. A larger sample would enable secondary analyses to explore additional clinical variables, such as renal dysfunction, that may influence outcomes and guide treatment personalization.
From a practical standpoint, our results do not suggest avoiding PCI within 4 hours following fibrinolysis but rather advocate for careful individual assessment of older and high-risk patients. In settings where primary PCI is unavailable, timely transfer to the catheterization lab remains essential, and further studies are needed to determine the optimal timing that balances clinical benefit and risk.
Advanced age significantly enhances the risk of no-reflow (30), characterized by microvascular thrombosis, distal embolization, and vasospasm, which are key predictors of short-term prognosis (32). Early combination therapy trials (31, 33–35) often exclude older patients due to their susceptibility to comorbidity-related event, limiting insights into adverse outcomes in this group. Consequently, the substantial representation of elderly patients in our cohort likely contributed to the observed increase in adverse outcomes associated with early lysis-PCI. Prompt post-fibrinolysis PCI may also have inadvertently worsened conditions in this vulnerable group.
While older patients face higher bleeding risks (17, 23), the increased bleeding in the ≤9.6-hour subgroup was not attributed to an overrepresentation of older adults, as the age distribution was not significantly different between subgroups. Furthermore, the relationship between lysis-PCI delays and reduced cardiovascular risk was consistent in a sensitivity analysis excluding patients aged 80 years and older (data not shown), reinforcing the reliability of our findings.
A randomized trial involving facilitated low-dose tenecteplase PCI, which specifically excluded participants aged ≥75 years (36), showed improved coronary artery patency and TIMI-3 flow rates (36). Consequently, the higher proportion of ≥80-year-olds in our study may have affected the increased early no-reflow observations.
Interestingly, a prior study indicated that matrix metalloproteinase 1 (MMP-1) peaks within 12 hours post-fibrinolysis among STEMI patients, coinciding with maximal tissue inhibitor of metalloproteinase-1 (TIMP-1) and MMP-1/TIMP-1 complex formation (37). This period of heightened MMP-1 activity without its natural inhibitor post-fibrinolysis (TIMP-1) may help explain the adverse outcome trends observed. MMP-1 inflammatory signaling responsiveness, combined with the heightened inflammatory milieu in older STEMI patients (37), suggests that plaque-disrupting PCI, an inflammatory intervention itself (38), might coincide with amplified harmful effects of MMP-1.
From a molecular standpoint, the data validates our hypothesis regarding the critical roles of matrix metalloproteinases (MMPs) in vascular remodeling and the instability of atherosclerotic plaques, mediated through inflammatory pathways (39). TIMP-1, the natural inhibitor, directly binds to MMP-1, affecting cardiac outcomes. Elevated MMP-1 levels in myocardial infarction patients, without corresponding increases in TIMP-1, correlate with poorer ventricular function (40). Furthermore, acute myocardial infarction reperfusion significantly alters MMP-1 levels over time (41).
A prior study demonstrated that after fibrinolysis in STEMI patients, MMP-1 levels rise, peaking with TIMP-1 between 9 and 12 hours post-fibrinolysis (37). This leads to the formation of the MMP-1/TIMP-1 complex. During the initial 12 hours post-fibrinolysis, increased MMP-1 activity due to inadequate TIMP-1 inhibition may contribute to adverse outcomes, including more frequent heart failure symptoms observed in patients with a lysis-PCI interval of ≤9.6 hours. Additionally, the heightened inflammatory response in older STEMI patients, coupled with the inflammatory nature of plaque-disrupting fibrinolysis, may intensify the harmful effects of MMP-1 (42). This could help explain why a shorter lysis-PCI interval, especially among older patients, could be particularly detrimental before the 12-hours peak of TIMP-1.
It is important to acknowledge certain limitation of our study. The data were derived from electronic medical records, which may have omitted key variables, leading to unmeasured residual confounding. Additionally, the study's retrospective and observational design introduces potential temporal bias and does not establish causality. However, the study was limited to two public hospitals that perform PCI procedures in the Brazilian capital, with minimal crossover between the public and private sectors. Although not a large multicenter trial, the uniqueness and consistency of our data and findings seem broadly relevant and plausible. However, limitations must be acknowledged, particularly the absence of documented PCI access routes. As femoral access increases bleeding risks compared to radial access (43), analyzing access distribution could have been informative. Unfortunately, this data was not available, making it impossible to determine whether increased bleeding in the ≤9.6-hours subgroup partly resulted from different access types – a limitation that precludes definitive conclusions regarding mechanisms. Ultimately, however, bleeding may relate at least in part to the shorter interval between fibrinolysis and PCI.
While our findings suggest potential risks of early lysis-PCI, particularly in patients over 80 years of age, we recognize that these results are hypothesis-generating and require confirmation in a robust, high-evidence study. Designing a randomized controlled trial (RCT) to test this hypothesis presents ethical and practical challenges, especially given the observational nature of our findings and the vulnerability of older patients. However, carefully constructed trials, with appropriate safeguards and patient selection criteria, are essential to determine the safest and most effective lysis-PCI timing, particularly in high-risk subgroups such as the elderly. Future studies are warranted to validate our results and inform clinical practice.
In conclusion, the lysis-PCI interval significantly impacts STEMI care. Our real-world analysis indicates that early PCI (≤9.6 hours) is associated with greater risks of mortality, MACE, and costs – particularly among the older adult. Further research should define the ideal fibrinolysis-PCI timing, balancing outcomes against costs to inform evidence-based guidelines.
The datasets presented in this article are not readily available because the data contains sensitive medical information from a real-world clinical setting. The Institutional Review Board (IRB) that approved this study did not authorize the public disclosure of individual patient data to protect participant privacy. Requests to access these datasets should be directed tobHVpenNlcmdpb2ZjQGdtYWlsLmNvbQ==;bHVpei5jYXJ2YWxob0BwLnVjYi5icg==
The studies involving humans were approved by Institute of Strategic Health Management of the Federal District (IGES-DF). The studies were conducted in accordance with the local legislation and institutional requirements. Written informed consent for participation was not required from the participants or the participants' legal guardians/next of kin in accordance with the national legislation and institutional requirements.
ADS: Formal Analysis, Methodology, Supervision, Writing – review & editing, Investigation, Writing – original draft. APS: Writing – original draft, Data curation. ACMR: Writing – original draft, Data curation. ACAR: Writing – original draft, Data curation. AM: Writing – original draft, Data curation. MO: Writing – original draft, Data curation. PM: Writing – original draft, Data curation. AS: Validation, Writing – review & editing, Data curation. AN: Validation, Data curation, Writing – original draft, Supervision. AG: Validation, Writing – original draft. GA: Validation, Writing – original draft. AC-S: Validation, Writing – original draft, Conceptualization, Formal Analysis, Investigation, Methodology, Supervision, Visualization, Writing – review & editing. LC: Conceptualization, Formal Analysis, Methodology, Supervision, Validation, Writing – review & editing, Data curation, Funding acquisition.
The author(s) declare financial support was received for the research, authorship, and/or publication of this article. This work was supported by grant 2019/09068-3 from São Paulo Research Foundation (FAPESP), grants 371/2021 and 585/2022 from Federal District Research Foundation (FAPDF), grants 310718/2021-0 from the Brazilian National Research Council (CNPq), grant 002/2023 from SOCESP and grant 29083-111 from Sebrae/FUNDEP.
The authors declare that the research was conducted in the absence of any commercial or financial relationships that could be construed as a potential conflict of interest.
All claims expressed in this article are solely those of the authors and do not necessarily represent those of their affiliated organizations, or those of the publisher, the editors and the reviewers. Any product that may be evaluated in this article, or claim that may be made by its manufacturer, is not guaranteed or endorsed by the publisher.
The Supplementary Material for this article can be found online at: https://www.frontiersin.org/articles/10.3389/fcvm.2024.1466961/full#supplementary-material
1. Vaduganathan M, Mensah GA, Turco JV, Fuster V, Roth GA. The global burden of cardiovascular diseases and risk: a compass for future health. J Am Coll Cardiol. (2022) 80:2361–71. doi: 10.1016/j.jacc.2022.11.005
2. Khan MA, Hashim MJ, Mustafa H, Baniyas MY, Al Suwaidi SKBM, AlKatheeri R, et al. Global epidemiology of ischemic heart disease: results from the global burden of disease study. Cureus. (2020) 12:e9349. doi: 10.7759/cureus.9349
3. Gandhi S, Garratt KN, Li S, Wang TY, Bhatt DL, Davis LL, et al. Ten-year trends in patient characteristics, treatments, and outcomes in myocardial infarction from national cardiovascular data registry chest pain-MI registry. Circ Cardiovasc Qual Outcomes. (2022) 15:e008112. doi: 10.1161/CIRCOUTCOMES.121.008112
4. Lawton JS, Tamis-Holland JE, Bangalore S, Bates ER, Beckie TM, Bischoff JM, et al. 2021 ACC/AHA/SCAI guideline for coronary artery revascularization: a report of the American College of Cardiology/American Heart Association joint committee on clinical practice guidelines. J Am Coll Cardiol. (2022) 79:e21–e129. doi: 10.1016/j.jacc.2021.09.006
5. Uddin M, Mir T, Khalil A, Mehar A, Gomez-Pineiro E, Babu MA, et al. ST-elevation myocardial infarction outcomes: a United States nationwide emergency departments cohort study. J Emerg Med. (2022) 62:306–15. doi: 10.1016/j.jemermed.2021.10.028
6. Byrne RA, Rossello X, Coughlan JJ, Barbato E, Berry C, Chieffo A, et al. 2023 ESC guidelines for the management of acute coronary syndromes. Eur Heart J. (2023) 44:3720–826. doi: 10.1093/eurheartj/ehad191
7. Hahn J-Y, Song YB, Gwon H-C, Choe YH, Kim JH, Sung J, et al. Relation of left ventricular infarct transmurality and infarct size after primary percutaneous coronary angioplasty to time from symptom onset to balloon inflation. Am J Cardiol. (2008) 102:1163–9. doi: 10.1016/j.amjcard.2008.06.042
8. Lønborg J, Schoos MM, Kelbæk H, Holmvang L, Steinmetz J, Vejlstrup N, et al. Impact of system delay on infarct size, myocardial salvage index, and left ventricular function in patients with ST-segment elevation myocardial infarction. Am Heart J. (2012) 164:538–46. doi: 10.1016/j.ahj.2012.07.021
9. Koul S, Andell P, Martinsson A, Gustav Smith J, van der Pals J, Scherstén F, et al. Delay from first medical contact to primary PCI and all-cause mortality: a nationwide study of patients with ST-elevation myocardial infarction. J Am Heart Assoc. (2014) 3:e000486. doi: 10.1161/JAHA.113.000486
10. Gioppatto S, Prado PS, Elias MAL, de Carvalho VH, Paiva CRC, Alexim GA, et al. The clinical and economic impact of delayed reperfusion therapy: real-world evidence. Arq Bras Cardiol. (2024) 121:e20230650. doi: 10.36660/abc.20230650
11. Keeley EC, Boura JA, Grines CL. Primary angioplasty versus intravenous thrombolytic therapy for acute myocardial infarction: a quantitative review of 23 randomised trials. Lancet. (2003) 361:13–20. doi: 10.1016/S0140-6736(03)12113-7
12. Widimský P, Budesínský T, Vorác D, Groch L, Zelízko M, Aschermann M, et al. Long distance transport for primary angioplasty vs immediate thrombolysis in acute myocardial infarction. Final results of the randomized national multicentre trial–PRAGUE-2. Eur Heart J. (2003) 24:94–104. doi: 10.1016/s0195-668x(02)00468-2
13. Andersen HR, Nielsen TT, Rasmussen K, Thuesen L, Kelbaek H, Thayssen P, et al. A comparison of coronary angioplasty with fibrinolytic therapy in acute myocardial infarction. N Engl J Med. (2003) 349:733–42. doi: 10.1056/NEJMoa025142
14. Zijlstra F, Hoorntje JC, de Boer MJ, Reiffers S, Miedema K, Ottervanger JP, et al. Long-term benefit of primary angioplasty as compared with thrombolytic therapy for acute myocardial infarction. N Engl J Med. (1999) 341:1413–9. doi: 10.1056/NEJM199911043411901
15. Madan M, Halvorsen S, Di Mario C, Tan M, Westerhout CM, Cantor WJ, et al. Relationship between time to invasive assessment and clinical outcomes of patients undergoing an early invasive strategy after fibrinolysis for ST-segment elevation myocardial infarction: a patient-level analysis of the randomized early routine invasive clinical trials. JACC Cardiovasc Interv. (2015) 8:166–74. doi: 10.1016/j.jcin.2014.09.005
16. Chandrashekhar Y, Alexander T, Mullasari A, Kumbhani DJ, Alam S, Alexanderson E, et al. Resource and infrastructure-appropriate management of ST-segment elevation myocardial infarction in low- and middle-income countries. Circulation. (2020) 141:2004–25. doi: 10.1161/CIRCULATIONAHA.119.041297
17. Lahtela HM, Bah A, Kiviniemi T, Nammas W, Schlitt A, Rubboli A, et al. Outcome of octogenarians with atrial fibrillation undergoing percutaneous coronary intervention: insights from the AFCAS registry. Clin Cardiol. (2017) 40:1264–70. doi: 10.1002/clc.22821
18. Alexim GA, Rocha LF, Dobri GP, Rosa Júnior AS, Reis RTB, Nogueira ACC, et al. Clinical and economic impact of coronary artery bypass graft and percutaneous coronary intervention in young individuals with acute coronary syndromes and multivessel disease: a real-world comparison in a middle-income country. Front Cardiovasc Med. (2022) 9:1000260. doi: 10.3389/fcvm.2022.1000260
19. O’Gara PT, Kushner FG, Ascheim DD, Casey DE, Chung MK, de Lemos JA, et al. 2013 ACCF/AHA guideline for the management of ST-elevation myocardial infarction: a report of the American College of Cardiology foundation/American Heart Association task force on practice guidelines. Circulation. (2013) 127:e362–425. doi: 10.1161/CIR.0b013e3182742cf6
20. Diamond A, Sekhon JS. Genetic matching for estimating causal effects: a general multivariate matching method for achieving balance in observational studies. Rev Econ Stat. (2013) 95:932–45. doi: 10.1162/REST_a_00318
21. Sekhon JS. Multivariate and propensity score matching software with automated balance optimization: the matching package for R. J Stat Softw. (2011) 42:1–52. doi: 10.18637/jss.v042.i07
22. Sekhon JS, Grieve RD. A matching method for improving covariate balance in cost-effectiveness analyses. Health Econ. (2012) 21:695–714. doi: 10.1002/hec.1748
23. Urban P, Mehran R, Colleran R, Angiolillo DJ, Byrne RA, Capodanno D, et al. Defining high bleeding risk in patients undergoing percutaneous coronary intervention: a consensus document from the academic research consortium for high bleeding risk. Eur Heart J. (2019) 40:2632–53. doi: 10.1093/eurheartj/ehz372
24. Caiazzo G, Musci RL, Frediani L, Umińska J, Wanha W, Filipiak KJ, et al. State of the art: no-reflow phenomenon. Cardiol Clin. (2020) 38:563–73. doi: 10.1016/j.ccl.2020.07.001
25. Rezkalla SH, Stankowski RV, Hanna J, Kloner RA. Management of no-reflow phenomenon in the catheterization laboratory. JACC Cardiovasc Interv. (2017) 10:215–23. doi: 10.1016/j.jcin.2016.11.059
26. Jaffe R, Charron T, Puley G, Dick A, Strauss BH. Microvascular obstruction and the no-reflow phenomenon after percutaneous coronary intervention. Circulation. (2008) 117:3152–6. doi: 10.1161/CIRCULATIONAHA.107.742312
27. Doherty DJ, Sykes R, Mangion K, Berry C. Predictors of microvascular reperfusion after myocardial infarction. Curr Cardiol Rep. (2021) 23:21. doi: 10.1007/s11886-021-01442-1
28. Fernandez-Avilés F, Alonso JJ, Castro-Beiras A, Vázquez N, Blanco J, Alonso-Briales J, et al. Routine invasive strategy within 24 h of thrombolysis versus ischaemia-guided conservative approach for acute myocardial infarction with ST-segment elevation (GRACIA-1): a randomised controlled trial. Lancet. (2004) 364:1045–53. doi: 10.1016/S0140-6736(04)17059-1
29. Pantea-Roșan LR, Pantea VA, Bungau S, Tit DM, Behl T, Vesa CM, et al. No-reflow after PPCI-A predictor of short-term outcomes in STEMI patients. J Clin Med. (2020) 9:2956. doi: 10.3390/jcm9092956
30. Sabin P, Koshy AG, Gupta PN, Sanjai PV, Sivaprasad K, Velappan P, et al. Predictors of no- reflow during primary angioplasty for acute myocardial infarction, from Medical College Hospital, Trivandrum. Indian Heart J. (2017) 69(Suppl 1):S34–45. doi: 10.1016/j.ihj.2016.12.012
31. Ellis SG, Armstrong P, Betriu A, Brodie B, Herrmann H, Montalescot G, et al. Facilitated intervention with enhanced reperfusion speed to stop events investigators. Facilitated percutaneous coronary intervention versus primary percutaneous coronary intervention: design and rationale of the facilitated intervention with enhanced reperfusion speed to stop events (FINESSE) trial. Am Heart J. (2004) 147:E16. doi: 10.1016/j.ahj.2003.07.025
32. Refaat H, Tantawy A, Gamal AS, Radwan H. Novel predictors and adverse long-term outcomes of no-reflow phenomenon in patients with acute ST elevation myocardial infarction undergoing primary percutaneous coronary intervention. Indian Heart J. (2021) 73:35–43. doi: 10.1016/j.ihj.2020.12.008
33. Bøhmer E, Hoffmann P, Abdelnoor M, Arnesen H, Halvorsen S. Efficacy and safety of immediate angioplasty versus ischemia-guided management after thrombolysis in acute myocardial infarction in areas with very long transfer distances results of the NORDISTEMI (Norwegian study on DIstrict treatment of ST-elevation myocardial infarction). J Am Coll Cardiol. (2010) 55:102–10. doi: 10.1016/j.jacc.2009.08.007
34. Singh M, Mathew V, Garratt KN, Berger PB, Grill DE, Bell MR, et al. Effect of age on the outcome of angioplasty for acute myocardial infarction among patients treated at the mayo clinic. Am J Med. (2000) 108:187–92. doi: 10.1016/s0002-9343(99)00429-5
35. Assessment of the Safety and Efficacy of a New Treatment Strategy with Percutaneous Coronary Intervention (ASSENT-4 PCI) investigators. Primary versus tenecteplase-facilitated percutaneous coronary intervention in patients with ST-segment elevation acute myocardial infarction (ASSENT-4 PCI): randomised trial. Lancet. (2006) 367:569–78. doi: 10.1016/S0140-6736(06)68147-6
36. Kanakakis J, Nanas JN, Tsagalou EP, Maroulidis GD, Drakos SG, Ntalianis AS, et al. Multicenter randomized trial of facilitated percutaneous coronary intervention with low-dose tenecteplase in patients with acute myocardial infarction: the Athens PCI trial. Catheter Cardiovasc Interv. (2009) 74:398–405. doi: 10.1002/ccd.22009
37. Zerva K, Sanidas E, Tzanis G, Chantziara V, Papadopoulos D, Barbetseas J. Effect of thrombolytic therapy in plaque inflammation and structural remodeling in acute myocardial infarction patients. Hellenic J Cardiol. (2017) 58:446–9. doi: 10.1016/j.hjc.2017.06.002
38. Tucker B, Vaidya K, Cochran BJ, Patel S. Inflammation during percutaneous coronary intervention-prognostic value, mechanisms and therapeutic targets. Cells. (2021) 10:1391. doi: 10.3390/cells10061391
39. Liu P, Sun M, Sader S. Matrix metalloproteinases in cardiovascular disease. Can J Cardiol. (2006) 22(Suppl B):25B–30B. doi: 10.1016/s0828-282x(06)70983-7
40. Papadopoulos DP, Moyssakis I, Makris TK, Poulakou M, Stavroulakis G, Perrea D, et al. Clinical significance of matrix metalloproteinases activity in acute myocardial infarction. Eur Cytokine Netw. (2005) 16:152–60.15941687
41. Hirohata S, Kusachi S, Murakami M, Murakami T, Sano I, Watanabe T, et al. Time dependent alterations of serum matrix metalloproteinase-1 and metalloproteinase-1 tissue inhibitor after successful reperfusion of acute myocardial infarction. Heart. (1997) 78:278–84. doi: 10.1136/hrt.78.3.278
42. Spinale FG, Villarreal F. Targeting matrix metalloproteinases in heart disease: lessons from endogenous inhibitors. Biochem Pharmacol. (2014) 90:7–15. doi: 10.1016/j.bcp.2014.04.011
Keywords: ST-segment elevation myocardial infarction, fibrinolysis, percutaneous coronary intervention, pharmacoinvasive strategy, reperfusion strategy
Citation: Stephanus AD, Santos AP, Rodrigues da Cunha ACM, Rocha ACA, Meireles AdA, de Oliveira MGS, Matsunaga PAC, Soares AAdSM, Nogueira ACC, Guimarães AdJBdA, Alexim GdA, Campos-Staffico AM and de Carvalho LSF (2025) Optimal timing of pharmacoinvasive strategy and its impact on clinical and economic outcomes in patients with ST-elevation myocardial infarction: a real-world perspective. Front. Cardiovasc. Med. 11:1466961. doi: 10.3389/fcvm.2024.1466961
Received: 18 July 2024; Accepted: 16 December 2024;
Published: 14 January 2025.
Edited by:
Gordana Krljanac, University of Belgrade, SerbiaReviewed by:
Aleksandra Djokovic, University Hospital Medical Center Bezanijska Kosa, SerbiaCopyright: © 2025 Stephanus, Santos, Rodrigues da Cunha, Rocha, Meireles, de Oliveira, Matsunaga, Soares, Nogueira, Guimarães, Alexim, Campos-Staffico and de Carvalho. This is an open-access article distributed under the terms of the Creative Commons Attribution License (CC BY). The use, distribution or reproduction in other forums is permitted, provided the original author(s) and the copyright owner(s) are credited and that the original publication in this journal is cited, in accordance with accepted academic practice. No use, distribution or reproduction is permitted which does not comply with these terms.
*Correspondence: Luiz Sergio Fernandes de Carvalho, bHVpei5jYXJ2YWxob0BwLnVjYi5icg==
Disclaimer: All claims expressed in this article are solely those of the authors and do not necessarily represent those of their affiliated organizations, or those of the publisher, the editors and the reviewers. Any product that may be evaluated in this article or claim that may be made by its manufacturer is not guaranteed or endorsed by the publisher.
Research integrity at Frontiers
Learn more about the work of our research integrity team to safeguard the quality of each article we publish.