- 1Faculty of Medicine, Tabriz University of Medical Sciences, Tabriz, Iran
- 2Shahid Rajaie Cardiovascular Medical and Research Center, Iran University of Medical Sciences, Tehran, Iran
- 3Department of Stem Cell and Developmental Biology, Cell Science Research Center, Royan Institute for Stem Cell Biology and Technology, ACECR, Tehran, Iran
Objectives: The COVID-19 pandemic has challenged global health systems since December 2019, with the novel virus SARS-CoV-2 causing multi-systemic disease, including heart complications. While acute cardiac effects are well-known, long-term implications are understudied. This review hopes to fill a gap in the literature and provide valuable insights into the long-term cardiac consequences of the virus, which can inform future public health policies and clinical practices.
Methods: This systematic review was prepared using PRISMA reporting guidelines. The databases searched were PubMed, Scopus, Web of Science, and Cochrane. Risk of Bias was assessed using ROBINS-I. The GRADE approach was employed to evaluate the level of certainty in the evidence for each outcome. A meta-analysis was conducted using the Comprehensive Meta-Analysis (CMA) software. In order to identify the underlying cause of high heterogeneity, a subgroup analysis was conducted. Sensitivity analysis was checked.
Results: Sixty-six studies were included in this review. Thirty-two of them enrolled in meta-analysis and the rest in qualitative synthesis. Most outcomes showed a moderate certainty of evidence according to the GRADE framework. Post-COVID individuals with no prior heart diseases showed significant changes in left ventricular (LV) and right ventricular (RV) echocardiographic indices compared to controls. These significant findings were seen in both post-acute and long-COVID survivors regardless of the severity of initial infection.
Conclusion: This review implies that individuals recovering from post-acute and long-term effects of COVID-19 may experience changes in myocardial function as a result of the novel coronavirus. These changes, along with cardiac symptoms, have been observed in patients without prior heart diseases or comorbidities.
Systematic Review Registration: PROSPERO, identifier (CRD42024481337).
1 Introduction
Since the beginning of December 2019, the COVID-19 pandemic, caused by a novel virus known as SARS-CoV-2 that originated in Wuhan, China, has presented a major challenge to global health and healthcare systems. Although COVID-19 is predominantly associated with lung-related symptoms and distinct functional and morphological changes, it has become evident that the infection can also lead to a multi-systemic disease affecting various organs including heart (1–3).
There is growing evidence of COVID-19's harmful effects on the heart, including acute events like heart attacks and long-term consequences even after recovery. The precise mechanisms underlying the cardiac damage caused by COVID-19 remain incompletely understood. COVID-19 has been associated with various patterns of cardiovascular dysfunction, including myocarditis, ischemic heart disease (e.g., myocardial infarction), hypovolemia, RV dysfunction resulting from pulmonary embolism, and, in some cases, cardiovascular dysfunction due to superimposed bacterial or fungal sepsis (4). The pathological findings suggest that SARS-CoV-2 can trigger hyper myocardial inflammation by infecting cardiomyocytes, leading to myocyte necrosis. This, in turn, may contribute to an increased risk of acute myocardial infarction, heart failure, arrhythmia, cardiac arrest, and acute coronary syndrome. Furthermore, apart from the potential harm caused by the illness itself, certain medications used in the treatment of COVID-19 and drug interactions may also have specific side effects on the heart (5).
Despite the advancements in treatments for COVID-19, it is anticipated that long-term consequences of the disease, particularly those affecting the heart, will persist in survivors. Therefore, the investigation of myocardial dysfunction following recovery from COVID-19 plays a vital role in the development of post-discharge monitoring programs and the formulation of public health, economic, and social policies (6). Cardiac imaging studies can serve as valuable predictive tools and aid in the comprehension of the underlying mechanisms of cardiac involvement. Echocardiography has been recognized as an available, non-invasive, and informative diagnostic tool, to identify cardiac manifestation (7). Echocardiographic findings in individuals with COVID-19 may exhibit variability, ranging from specific regional wall motion abnormalities of the LV or RV to varying degrees of global cardiac dysfunction associated with myocarditis or a systemic dysregulated inflammatory response to viral infection. Echocardiography is therefore essential in distinguishing these patterns, guiding treatment strategies, and monitoring the clinical progression over time (8).
Existing research has extensively focused on the impact of acute COVID-19 on cardiac function and complications, with numerous reviews and studies providing valuable insights into this area (9). However, there is a lack of information in the research on the lasting impacts of COVID-19, known as long covid, on heart function. Previous reviews have tried to examine this connection, but they have been restricted in their coverage and have not carried out thorough meta-analyses. Furthermore, these reviews have not taken into consideration possible influencing factors like the severity of the initial COVID-19 infection, the time elapsed since the infection, and the presence of other medical conditions. Hence, it is essential to conduct more in-depth and thorough research that specifically looks into the lasting effects of COVID-19 on the heart, considering important factors. To tackle this issue, we carried out a systematic review and meta-analysis that focused on echocardiographic imaging to study the long-term impact of SARS-CoV-2 infection on heart function and the risk of future cardiac complications.
2 Materials and methods
This systematic review and meta-analysis follows the Preferred Reporting Items for Systematic Reviews and Meta-Analyses (PRISMA) guidelines (10). The PRISMA checklist is provided as supplement (Supplementary S1 document). The protocol for this work was registered in the International Prospective Register of Systematic Reviews (PROSPERO) (identifier: CRD42024481337). This review also followed the published protocol for evaluating risk factors and prognostic implications of imaging left ventricular diastolic dysfunction in individuals diagnosed with COVID-19, adhering to a systematic approach (8).
2.1 Eligibility criteria
To be considered for inclusion, published studies had to meet the following criteria: (1) studies employed valid research designs with clearly defined methodology, (2) studies assessed cardiac function using Echocardiography in COVID-19 patient after recovery, (3) studies identified COVID-19 infection according to the World Health Organization interim guidance, (4) studies reported at least one echocardiographic parameter measuring myocardial function and/or structure, (5) studies excluded cases with pre-existing cardiac disease including ischemic heart disease, valvular disease, arrhythmias-conduction disorders, heart failure, cardiomyopathies, pericarditis, pericardial effusion, pulmonary hypertension, pulmonary embolism, sarcoidosis, amyloidosis, active cancer, recent pregnancy, postpartum. The overall exclusion criteria were as follows: (1) studies involved cases during acute stage of COVID-19, (2) studies evaluated cardiac function using any other imaging technique other than echocardiography, (3) studies reported as abstracts, case reports, case series, reviews, or practice guidelines.
2.2 Information sources
A thorough search was conducted in the PubMed, Scopus, Web of Science, and Cochrane databases to locate relevant studies published until March 2024. Additionally, a manual search of the reference lists of the identified articles was carried out.
2.3 Search strategy
The search strategy of Scopus was conducted as follows: (TITLE-ABS-KEY (((“left ventric*” OR “right ventric*” OR “left cardiac*” OR “right heart” OR “right cardiac” OR “left heart” OR atri* OR myocardi* OR diastol* OR systol*) PRE/1 (dysfunction OR function OR remodeling OR impair* OR hypertroph* OR active* OR volume OR mass* OR dimension* OR diameter OR thickness OR index* OR “ejection time” OR “ejection fraction”)) OR echo OR echocardiograph*)) AND [TITLE-ABS-KEY (“covid-19” OR “sars cov 2”)]. The search strategy employed for PubMed, Web of Science, and the Cochrane Library was similar to that used for Scopus and its table is provided as supplement (Supplementary S2 document). Furthermore, three reviewers independently reviewed the reference lists of systematic reviews and selected studies to ensure that all pertinent articles were included in the analysis.
2.4 Study selection
Three reviewers independently assessed each title and abstract, and if the articles fulfilled the inclusion criteria, the full text was reviewed. The eligibility of the selected articles was then assessed by the same three reviewers through an evaluation of their full texts. Any discrepancies were resolved through discussion with a fourth reviewer. The study selection process was summarized using the PRISMA flow diagram.
2.5 Data extraction and data items
Following the extraction of data, the information was gathered through Microsoft Excel spreadsheets. The subsequent dataset comprises: studies' basic characteristics (study design, year of publication, country, and first author), participant characteristics (age, body mass index, number of cases and control groups), echocardiographic indices and major findings of each study. Potential confounding factors were carefully considered to ensure the robustness of the study findings. These factors included severity of COVID-19 infection, persistent post-COVID symptoms, duration from COVID acute phase to echocardiography examination in recovery phase, presence of comorbid disease. Data related to these factors were extracted from the studies to address their potential influence on the findings.
2.6 Risk of bias assessment
ROBINS-I was employed to evaluate the methodological quality and risk of bias for non-randomized control trials. This tool encompasses the assessment of seven potential sources of bias, including confounding bias, bias in participant selection, bias in intervention classification, bias due to deviations from intended interventions, bias resulting from missing data, bias in outcome measurement, and bias in the selection of reported results (11). Importantly, no studies were excluded based on the assessment of bias risk.
2.7 Outcome quality assessment
The certainty of overall evidence was assessed using the Grading of Recommendations Assessment, Development and Evaluation (GRADE) method (12). The assessment of evidence certainty for individual outcomes relied on five distinct criteria: (1) limitations of the study design; (2) consistency of results; (3) directness; (4) precision and (5) potential for publication bias. A decrement of one level in certainty was implemented for each unfulfilled criterion.
2.8 Synthesis methods
The mean differences (MD) pooled the data, with 95% confidence intervals (CIs). The I2 statistic was used to analyze the interstudy statistical heterogeneity. To calculate the pooled effect, either fixed-effects or random-effects model was used according to the heterogeneity, study design and sample size. I2 values of 25%, 50%, and 75% were considered to represent low, moderate, and high levels of heterogeneity, respectively. Subgroup meta-analyses were conducted to uncover the underlying heterogeneity. A univariate meta-regression model was used to explore the impact of age and BMI as potential moderators. A sensitivity analysis was carried out in cases where the decision-making values had arbitrary or unclear ranges. Publication bias was assessed by visually inspecting funnel plots of MD vs. standard error. When at least 10 studies were available for analysis, Begg's tests and Egger's tests were employed to evaluate the potential publication bias. If there was an obvious publication bias, a trim-and-fill analysis was used to determine the underlying origin of the publication bias. All analyses were conducted using Comprehensive Meta-Analysis Version 3. P-value < 0.05 was considered significant in all tests.
3 Results
3.1 Study selection
The study flowchart is shown in Figure 1; our search strategy revealed 2,602 studies in PubMed, 6,502 in Scopus, 2,994 in WOS and 42 in Cochrane. After removing duplications, 5,942 studies underwent title assessment. Of these, 2,321 studies were eligible for abstract review. After surveying abstracts, 107 studies were perused for full text. Finally, 66 studies were qualified to be included in this systematic review and meta-analysis, and the rest did not meet the inclusion criteria; the reasons for their exclusions are provided in the supporting information section (Supplementary S3 document).
3.2 Characteristics of studies
Table 1 presents the key features of the sixty-six studies (13–76) included in this research. The search process resulted in the identification of 66 studies, out of which 41 were designed as cohort studies, 16 were cross-sectional studies, 8 were case-control studies, and one study (20) was a combined cross-sectional and longitudinal cohort. The majority of these studies utilized real-time PCR (rt-PCR) as the diagnostic method for COVID-19, while a few employed the IgG antibody titer for diagnosis (32, 37). Most of the studies focused on adult patients who had recovered from COVID-19, whereas 6 studies specifically examined athletes who had overcome the disease (13, 17, 19, 29, 52, 75). A total of 32 studies were conducted comparing post-COVID patients to a control group of individuals who tested negative for COVID-19. All 32 studies were included in a meta-analysis, with the exception of one study (39) where patients and controls were not matched, and two studies (42, 43) where matching status was unknown. Four studies (16, 27, 28, 40) categorized COVID-19 cases into groups based on the severity of the infection. To maintain consistency with the other included studies, we treated these studies as separate entities, each having a common healthy control group. Furthermore, two studies (30, 43) assessed COVID-19 cases based on the presence of dyspnea symptoms in patients. These studies were also divided into two distinct studies. Three studies (16, 24, 37) divided COVID-19 cases based on the time duration between diagnosis and echocardiography. Similarly, one study (17) divided COVID-19 cases into male and female athletes. Each of these studies was separated into two distinct studies as well.
The severity of COVID-19 infection was not addressed in 12 studies (19, 29, 30, 32, 37, 45, 46, 48, 60, 66, 72, 77). Regarding the COVID-19 vaccination, only six studies (24, 29, 30, 46, 52, 64) provided information on the vaccination status of patients. It is noteworthy that data collection in most of the studies was conducted before the availability of any vaccines. Thirteen studies lacked information on post-COVID symptoms at the time of study enrollment (16, 20, 39, 40, 50, 51, 55, 62, 65, 66, 74, 77, 78). In most studies, the time interval between the acute phase of COVID-19 and echocardiography during the recovery phase was over 1 month, except for 3 studies (13, 16, 29) that were conducted within at least 10 days. Thirty studies reported the exclusion of patients with comorbid disease. On the other hand, three studies did not provide any information regarding the comorbid diseases (49, 66, 78). The primary focus of the studies pertained to the evaluation of LV function, with a secondary emphasis on RV function. A subset of studies also conducted concurrent assessments of both LV and RV function. Majority of the studies found significant changes in echocardiographic parameters, indicating subclinical alterations in the function of the LV and/or RV in post-COVID patients. However, 17 studies (17, 18, 27, 29, 30, 37, 41, 44, 46, 47, 49, 64, 68, 69, 73, 74, 78) did not report any significant findings.
3.3 Studies' risk of bias
Figure 2 depicts a summary of the RoB-1 assessment. The overall risk of bias was found to be low to moderate. A low percentage (<15%) of serious risk of bias was identified in various domains, including confounding, selection of participants, classification of interventions, deviations from intended interventions, and missing data bias. Moderate risk of bias (25%–50%) was noted in confounding and deviations from intended interventions. There was no significant bias detected in the selection of reported results. Among 32 studies enrolled in the meta-analysis, five were found to have a serious risk of confounding bias (19, 30, 32, 37, 45). These studies did not provide information on the severity of COVID-19 infection in the patients. Additionally, 12 studies were rated as having a moderate risk of bias due to the presence of comorbid diseases that could impact heart function (15, 16, 20–22, 24, 27, 28, 35, 38, 40, 41). Three studies found a serious risk of bias in participant selection due to an unmatched case-control group (39, 42, 43), while five studies indicated a moderate risk due to the inclusion of specific populations such as athletes, women, and young adults that may not accurately represent the general population (13, 17, 19, 31, 36). Concerning bias due to classification of interventions, one study (21) found a serious risk of bias in comparing echo findings between two groups with reduced and normal-LVGLS, while two (24, 43) were deemed to have moderate risk due to incorrectly classifying post-COVID patients and comparing echo measures between them instead of with controls. Three studies demonstrated a moderate risk of bias due to deviations from intended interventions (30, 34, 39). Their main focus was on evaluating cardiopulmonary function rather than cardiac alone. Seven studies were found to have a moderate risk of bias due to missing data, and they reported small amounts of echocardiographic indices (15, 25, 30, 34, 37, 39, 43). Regarding bias in outcome measurement, 10 studies (14, 19, 22, 24, 26, 27, 33, 36, 42, 45) found a moderate risk of bias in reporting certain echo indices that deviated from the ranges reported in other studies (detailed in Table 2). Thirty-four studies only had post-COVID cases. Regarding the confounding factors, one study had a serious risk of bias as it did not provide information about the comorbid diseases of the patients (66). Twenty studies had moderate risks due to the presence of comorbid diseases (29, 51, 57, 59, 61–63, 67–69, 71, 72, 74, 76, 77) and lack of information on the severity of COVID-19 infection (29, 46, 48, 49, 72, 77). Considering the risk of bias in participant selection, 6 studies had moderate risks for reasons of inclusion of specific populations such as athletes (29, 52, 75) and having no classification and comparison among patients (49, 55, 68). Regarding bias due to deviations from intended interventions, one study had a serious risk of bias as its main focus was on hepatic abnormalities rather than cardiac alone (60). Twenty-one studies revealed moderate risk due to laboratory and biomarker evaluations, electrocardiogram evaluations, various surveys and lifestyle changes, return to play evaluation of athletes, chest computer tomography, post-COVID-19 functional status scale, cardio-ankle vascular index, ankle-brachial index, myocardial work analysis, walk test, pulmonary function tests, and cardiopulmonary exercise tests (48, 49, 51–59, 65, 67–71, 74–77). Bias due to missing data was serious in 3 studies as they reported small amounts of echocardiographic indices (48, 62, 74). Figure 3 represents the traffic light plot of risk of bias assessment for each included study.
3.4 Outcome quality assessment
The certainty of evidence for outcomes, as assessed by GRADE framework, is delineated in Table 2. The meta-analysis indicates a moderate level of certainty in the majority of outcomes, primarily attributable to the inherent susceptibility to bias in observational studies. Outcomes with low certainty are typically caused by a small number of studies, significant heterogeneity, and the existence of potential biases.
3.5 Result of synthesis
3.5.1 Overall outcomes
Among the echocardiographic measures of LV systolic function, LV-GLS and LVEF were found to be significantly different between the two groups being compared. The analysis of 26 studies showed a notable decrease in LV-GLS (less negative) in post-COVID patients (n = 1,810) compared to controls (n = 1,254), with a mean difference of 1.21 [95%CI (0.681, 1.75), p = 0.000, I2 = 91%]. Post-COVID patients (n = 2,173) exhibited a lower LVEF compared to controls (n = 1,770), with a MD of −0.829 [95%CI (−1.397, −0.262), p = 0.004, I2 = 73%]. Additionally, the meta-analysis of 12 studies revealed that LAD was significantly increased in post-COVID patients (n = 833) comparing to controls (n = 892) with a MD of 1.603 [95%CI (0.696, 2.511), p = 0.001, I2 = 80.7%]. However, LAVI was not significantly different comparing two groups with a MD of 0.895 [95% CI (−0.509, 2.29), p = 0.211, I2 = 82.7%]. In terms of RV evaluation, post-COVID patients showed significantly lower RV-GLS (less negative) and higher RV-MPI values compared to controls, with mean differences of 2.179 [95%CI (1.099, 3.260), p = 0.000, I2 = 85.4%] and 0.060 [95% CI (0.030, 0.089), p = 0.009, I2 = 99%], respectively. No significant differences were found in the diastolic and geometric indices of the left ventricle between the two groups being compared (Table 3). Forest plots are provided in supporting information (Supplementary S4 document).
3.5.2 Subgroup analysis
3.5.2.1 Grouped by duration from acute COVID to echo examination in recovery phase
The subgroup meta-analysis found that among LV geometric indices, LVESD was notably higher in post-COVID patients (n = 505) compared to controls (n = 698) for a duration of ≥3 months, showing a MD of 0.928 [95% CI (0.566, 1.289), p = 0.000, I2 = 0%]. Moreover, post-COVID patients exhibited a significant decrease in IVSD and LVM compared to controls for a duration of ≥3 months, with a MD of −0.132 [95% CI (−0.258, −0.007), p = 0.039, I2 = 0%] and −19.37 [95%CI (−29.8, −8.92), p = 0.000, I2 = 0%], respectively.
In terms of systolic function lasting ≥3 months, LVEF was found to be significantly lower in post-COVID patients (n = 836) compared to controls (n = 1,172), with a MD of −1.16 [95% CI (−1.94, −0.375), p = 0.004, I2 = 60.1%]. Furthermore, post-COVID patients exhibited a significantly decreased LV-GLS (less negative) compared to controls for durations of both 1–3 months and ≥3 months, with MDs of 1.22 [95% CI (0.181, 2.27), p = 0.021, I2 = 57.5%] and 1.37 [95% CI (0.637, 2.11), p = 0.000, I2 = 92.9%], respectively.
There were significant differences in the E/A ratio and mitral A wave among diastolic function indices. Within a period of ≥3 months, post-COVID patients exhibited a significant reduction in the E/A ratio and an increase in the mitral A wave compared to the control group. The MDs were −0.084 [95%CI (−0.129, −0.039), p = 0.000, I2 = 32%] for the E/A ratio and 0.039 [95% CI (0.017, 0.060), p = 0.000, I2 = 30.6%] for the mitral A wave. Additionally, post-COVID patients (n = 410) exhibited a significant elevation in LAD in comparison to the control subjects (n = 604), over a duration of ≥3 months, with a MD of 1.863 [95% CI (0.694, 3.032), p = 0.002, I2 = 86.2%]. However, a meta-analysis of 2 studies showed a significant increase in LAVI in post-COVID patients (n = 236) compared to controls (n = 174) within a timeframe of 1–3 months, with a MD of 1.95 [95% CI (0.728, 3.17), p = 0.002, I2 = 8.4%].
In subgroup meta-analysis of RV function, RV-MPI, RVD and sPAP were significantly higher in post-COVID patients compared to control group for a duration of ≥3 months, with MDs of 0.035 [95% CI (0.008, 0.062), p = 0.012, I2 = 98.9], 0.900 [95% CI (0.510, 1.290), p = 0.000, I2 = 0%] and 5.172 [95%CI (2.668, 7.676), p = 0.000, I2 = 95.2%], respectively. Moreover, a significant decrease in TAPSE and RV-GLS (less negative) were observed in post-COVID patients compared to controls with a MD of −1.160 [95% CI (−1.885, −0.466), p = 0.001, I2 = 80.3%] and 1.842 [95%CI (0.853, 2.831), p = 0.000, I2 = 74.3%], respectively. Detailed information is provided in Table 3.
3.5.2.2 Grouped by severity of COVID-19 infection
3.5.2.2.1 Mild COVID-19 infection
In terms of mild COVID-19 infection and LV geometric indices, significant increase was observed in LVEDV and LVESD in post-COVID patients compared to controls with MDs of 8.39 [95% CI (3.57, 13.20), p = 0.001, I2 = 0%], and 0.908 [95% CI (0.488, 1.32), p = 0.000, I2 = 32.1%], respectively. LVM was significantly lower in post-COVID patients (n = 191) compared to controls (n = 139), with a MD of −13.71 [95 CI% (−25.30, −2.11), p = 0.020, I2 = 0%]. Moreover, significant changes in systolic function were observed in mild infection cases. Post-COVID patients reveled to have a decrease in LVEF and LV-GLS (less negative) compared to control groups, with MDs of −0.886 [95% CI (−1.64, −0.128), p = 0.022, I2 = 78%] and 0.815 [95% CI (0.047, 1.58), 0.038, I2 = 88.1%], respectively. Among LV diastolic indices, E/A ratio was significantly lower and LAVI was significantly increased in mild infection compared to controls, with MDs of −0.042 [95% CI (−0.076, −0.007), p = 0.017, I2 = 45%] and 0.922 [95% CI (0.139, 1.845), p = 0.023, I2 = 46%], respectively. RVD and sPAP were significantly higher in post-COVID patients compared to controls with MDs of 0.865 [95% CI (0.412, 1.317), p = 0.000, I2 = 0%] and 3.749 [95% CI (0.817, 6.682), p = 0.012, I2 = 0.012, I2 = 92.3%], respectively. Detailed information is provided in Table 3.
3.5.2.2.2 Moderate and/or severe COVID-19 infection
Post-COVID patients exhibited higher values of LVEDV, PWD, and LVM compared to the control group. The MDs for LVEDV, PWD, and LVM were 10.09 [95% CI (2.29, 17.89), p = 0.011, I2 = 0%], 0.614 [95% CI (0.259, 0.969), p = 0.001, I2 = 52.7%], and 9.018 [95% CI (0.458, 17.57), p = 0.039, I2 = 0%], respectively. Concerning systolic function, there was no significant difference in LVEF between the two groups, as indicated by a MD of −0.900 [95% CI (−1.96, 0.169), p = 0.098, I2 = 69%]. Conversely, LV-GLS exhibited significantly lower (less negative) values in post-COVID patients in comparison to the control group, with a MD of 1.84 [95% CI (0.751, 2.94), I2 = 90.1%]. Among LV diastolic indices, E/A ratio and mitral E wave values were significantly decreased and mitral A wave was significantly increased in post-COVID patients compared to controls. The MDs for E/A, E wave and A wave were −0.134 [95% CI (−0.258, −0.010), p = 0.034, I2 = 82%], −0.052 [95% CI (−0.082, −0.022), p = 0.001, I2 = 0%] and 0.086 [95%CI (0.022, 0.150), p = 0.008, I2 = 0%], respectively.
Additionally, significantly higher values were found in both LAD and LAVI in post-COVID patients compared to controls. The MD for LAD was 2.305 [95% CI (1.058, 3.74), p = 0.000, I2 = 63.7%], and for LAVI it was 1.475 [95% CI (0.374, 2.575), p = 0.009, I2 = 32.1%].
Regarding RV indices, post-COVID patients showed significantly increased value in sPAP with MDs of 4.306 [95% CI (2.398, 6.214), p = 0.000, I2 = 83.7%]. Moreover, TAPSE and RV-GLS values were significantly lower in post-COVID patients compared to controls with MDs of −1.234 [95% CI (−2.197, −0.270), p = 0.012, I2 = 57%] and 6.686 [95% CI (3.109, 9.662), p = 0.000, I2 = 95.2%], respectively. Detailed information is provided in Table 3.
3.5.2.3 Grouped by presence of comorbid diseases
Post-COVID patients with comorbidities showed higher values of LVEDV and LVESV compared to comorbid-matched control group with MDs of 10.35 [95% CI (4.93, 15.76), p = 0.000, I2 = 62.6%] and 5.55 [95% CI (1.15, 9.96), p = 0.013, I2 = 0%], respectively. There was a significant decrease in LVESD in post-COVID patients with comorbidities and an increase in cases without comorbidities compared to their comorbid-matched controls with MDs of −1.292 95% CI [−2.089, −0.495], p = 0.001, I2 = 43.2%) and 0.905 [95% CI (0.567, 1.24), p = 0.000, I2 = 0%], respectively. LVEF exhibited a significant decrease in post-COVID patients with comorbidities and those without comorbidities when compared to their comorbid-matched controls. The MDs were −0.852 [95% CI (−1.66, −0.038), p = 0.040, I2 = 56.5%] and −0.833 [95%CI (−1.64, −0.005), p = 0.049, I2 = 79.6%], respectively. Furthermore, LV-GLS was significantly decreased (less negative) in both groups of post-COVID patients, with MDs of 0.919 [95% CI (0.07, 1.76), p = 0.033, I2 = 88.8%] and 1.43 [95% CI (0.703, 2.16), p = 0.000, I2 = 93.7%] compared to their respective controls. E/A ratio was significantly lower in post-COVID patients with comorbidities compare to its comorbid-matched controls, with a MD of −0.137 [95% CI (−0.424, −0.032), p = 0.010, I2 = 57.9%]. Significantly higher values of both LAD and LAVI were observed in post-COVID patients with comorbidities compared to their matched controls. The MDs were 2.287 [95% CI (0.910, 3.664), p = 0.001, I2 = 77.4%] and 1.135 [95% CI (0.290, 1.980), p = 0.008, I2 = 0%], respectively.
Regarding RV function, RV-GLS was notably decreased (less negative) in both post-COVID patients with and without comorbidities compared to their controls, with MDs of 2.228 [95% CI (0.377, 4.079), p = 0.018, I2 = 90%] and 2.152 [95% CI (0.807, 3.498), p = 0.002, I2 = 79%]. Additionally, post-COVID patients without comorbidities presented higher values of RV-MPI with a MD of 0.060 [95% CI (0.030, 0.089), p = 0.009, I2 = 99%], compared to matched-controls. In post-COVID patients without comorbidities, TAPSE values were significantly lower, whereas no significant difference was found in cases with comorbidities when compared to their matched controls. The MDs were −1.440 [95%CI (−2.296, −0.585), p = 0.001, I2 = 87.4%] and −0.337 [95% CI (−1.213, 0.540), p = 0.452, I2 = 76%], respectively. Moreover, sPAP presented higher values in post-COVID patients with comorbidities and no significant result in cases without comorbidities compared to their matched controls with MDs of 6.777 [95% CI (4.463, 9.091), p = 0.000, I2 = 91.2%] and 2.039 [95%CI (−0.181, 4.258), p = 0.072, I2 = 91%], respectively. Detailed information is provided in Table 3. Forest plots are provided in supporting information (Supplementary S4 document).
Table 4 represent the summary of quantitative synthesis.
3.6 Sensitivity analysis
3.6.1 LVEDV
The study by Wood et al. (42), showed a high risk of bias for LVEDV in overall result of synthesis. Excluding this study revealed a significant difference between two groups of comparison with a MD of 4.732 [95% CI (1.367, 8.096), p = 0.006, I2 = 46.3%]. However, no significant difference was observed between two groups when grouped by duration ≥3 months and absence of comorbidities with MDs of 5.727 [95% CI (−0.209, 11.66), p = 0.059, I2 = 59.7%] and 1.964 [95% CI (−3.076, 7.00), 0.445, I2 = 57%], respectively.
3.6.2 IVSD
The studies by Ardahanli et al. (23) and Akbulut et al. (27) were found to have a high risk of bias for IVSD for in overall result of synthesis and duration of ≥3 months. Excluding these studies did not change the direction, or statistical significance of the summary estimate with MDs 0.011 [95% CI (−0.147, 0.170), p = 0.891, I2 = 77%] and 0.135 [95%CI (−0.124, 0.394), p = 0.307, I2 = 88.5%], respectively. However, excluding these studies revealed significant difference between two groups of comparison in moderate-severe COVID-19 infection, presence and absence of comorbid disease with MDs of 0.539 [95%CI (0.281, 0.798), p = 0.000, I2 = 77%] and 0.320 [95%CI (0.019, 0.620), p = 0.037, I2 = 88.5%], −0.083 [95%CI (−0.143, −0.023), p = 0.007, I2 = 36%], respectively.
3.6.3 LVM
Two studies (17, 19) were at high risk of bias for LVM due to involving athletes as their cases. A sensitivity analysis by excluding them did not change the direction, or statistical significance of the summary estimate with effect size of −5.78 [95%CI (−27.2, 15.3), p = 0.597, I2 = 83%].
3.6.4 LVMI
The study by Turpin et al. (17) was deemed to have a high risk of bias for LVMI due to the inclusion of athletes as study participants. However, excluding this study did not change the direction, or statistical significance of the summary estimate with an effect size of −0.722 [95%CI (−6.575, 5.123), p = 0.809, I2 = 86.9%]. Furthermore, subgroup analyses focusing on mild COVID-19 infection and the absence of comorbid diseases also showed no change in the significance of the results when excluding this study. The effect sizes for mild COVID-19 infection and absence of comorbid diseases were 2.07 [95%CI (−7.21, 11.36), p = 0.622, I2 = 80.2%] and −1.06 [95%CI (−10.82, 8.70), p = 0.831, I 2 = 89%], respectively.
3.6.5 LVEF
Three studies, conducted by Turpin et al. (17), Tudoran et al. (36) and Akbulut et al. (27), were deemed to have a high risk of bias in relation to LVEF. In a sensitivity analysis focusing on overall, mild COVID-19 and cases without comorbidities, the exclusion of these studies resulted in a change in the significance of the summary estimate. The effect size was found to be −0.499 [95% CI (−0.935, 0.037), p = 0.070, I2 = 63%] for overall cases, −0.229 [95% CI (−0.842, 0.383), p = 0.463, I2 = 62%] for mild cases, and −0.036 [95% CI (−0.686, 0.613), p = 0.913, I2 = 56.5%] for cases with absent comorbidities. However, excluding these studies did not change the direction, or statistical significance of the summary estimate for meta-analysis of duration ≥3 months with a MD of −0.693 [95% CI (−1.298, −0.087), p = 0.025, I2 = 47%].
3.6.6 LV-GLS
Akkabulut et al. (27) was found to have a high risk of bias in the meta-analysis of LV-GLS for both overall results and durations of ≥3 months. Conducting a sensitivity analysis by excluding this study did not affect the significance of the results, with effect sizes of 1.43 [95%CI (0.900, 1.961), p = 0.000, I2 = 91%] and 1.78 [95%CI (1.049, 2.516), p = 0.000, I2 = 92%], respectively. Furthermore, excluding this study did not alter the significant findings in the subgroup analysis of severity of COVID-19 infection. The effect sizes remained significant at 1.021 [95%CI (0.265, 1.776), p = 0.008, I2 = 87%] for mild infection and 2.289 [95%CI (1.201, 2.314), p = 0.000, I2 = 89%] for moderate-severe infection.
3.6.7 E/A ratio
Hamdy et al. (45) showed a high risk of bias in relation to this specific outcome. A sensitivity analysis was conducted by removing this study, changed the significancy of summary estimate for overall outcome and a duration of ≥3 months. The effect sizes were −0.079 [95%CI (−0.127, −0.032), p = 0.001, I2 = 64.6%] and −0.079 [95%CI (−0.141, −0.018), p = 0.011, I2 = 71.8%], respectively. However, excluding this study did not change the direction, or statistical significance of the summary estimate for the absence of comorbid diseases, with an effect size of −0.053 [95%CI (−0.109, 0.002), p = 0.061, I2 = 63.2%].
3.6.8 E/e' ratio
Hamdy et al. (45) and Wood et al. (42) were found to have a high risk of bias regarding this outcome. Excluding these studies did not change the direction, or statistical significance of the summary estimate for the overall outcome and duration of ≥3 months. The effect sizes remained at 0.092 [95%CI (−0.229, 0.412), p = 0.575, I2 = 76.3%] and 0.333 [95%CI (−0.094, 0.759), p = 0.126, I2 = 72.4%] for each respective outcome.
3.6.9 LAVI
Hamdy et al. (45) was found to have a high risk of bias for the outcome. Excluding this study did not change the direction, or statistical significance of the summary estimate for the overall outcome and duration of ≥3 months, with effect sizes of 0.578 [95%CI (−0.361, 1.517), p = 0.227, I2 = 51.4%] and 0.674 [95%CI (−0.077, 1.424), p = 0.079, I2 = 42.2%], respectively. The exclusion of this study also did not alter the lack of significance for the absence of comorbid disease, with an effect size of 0.214 [95%CI (−1.261, 1.688), p = 0.776, I2 = 73.4%].
3.6.10 RV-MPI
The study by Günay et al. (14) had a high risk of bias for this particular outcome. A sensitivity analysis was conducted by removing this study did not change the significancy of summary estimate for the overall outcome, showing an effect size of 0.035 [95% CI (0.008, 0.062), p = 0.012, I2 = 98.9%].
3.6.11 RVD
The study by Günay et al. (14) was found to have a high risk of bias for this particular outcome. Excluding this study changed the statistical significancy of summery estimates for the overall outcome, duration of 1–3 months, and the absence of comorbid disease, with effect sizes of 0.654 [95%CI (0.321, 0.987), p = 0.000, I2 = 17%], −0.277 [95%CI (−1.046, 0.493), p = 0.481, I2 = 21.3%] and 0.607 [95%CI (0.115, 1.099), p = 0.016, I2 = 0%], respectively. However, the sensitivity analysis for moderate-severe COVID-19 infection did not alter the direction or statistical significance of the summary estimate of the results. The effect sizes for these outcomes and 0.444 [95%CI (−0.099, 0.987), p = 0.109, I2 = 61.8%], respectively.
3.6.12 sPAP
Küçük et al. (33) had a high risk of bias for moderate to severe COVID-19 infection. A sensitivity analysis that excluded this study showed that the result remained significant with an effect size of 8.016 [95%CI (6.800, 9.232), p = 0.000, I2 = 26.5%]. De et al. (24) was also at high risk of bias for the presence of comorbid disease. However, excluding this study in a sensitivity analysis did not change the direction or statistical significance of the summary estimate, with an effect size of 8.097 [95%CI (7.08, 9.113), p = 0.000, I2 = 0%].
Forest plots of sensitivity analysis are provided in supporting information (Supplementary S5 document).
3.7 Meta-regression
The results of the univariate meta-regression showed a significant positive correlation between MDs of RV-GLS and age. The effect size was 0.150 [95% CI (0.027, 0.272), p = 0.016, R2 = 0.32]. Moreover, MDs of TAPSE was negatively correlated with post-COVID patients' age with an effect size of −0.077 [95%CI (−0.152, −0.003), p = 0.04, R2 = 0.09]. There were no other significant correlations observed between echocardiographic variables and age or BMI. Detailed information is presented in Table 5. Scatter plots are provided in supporting information (Supplementary S6 document).
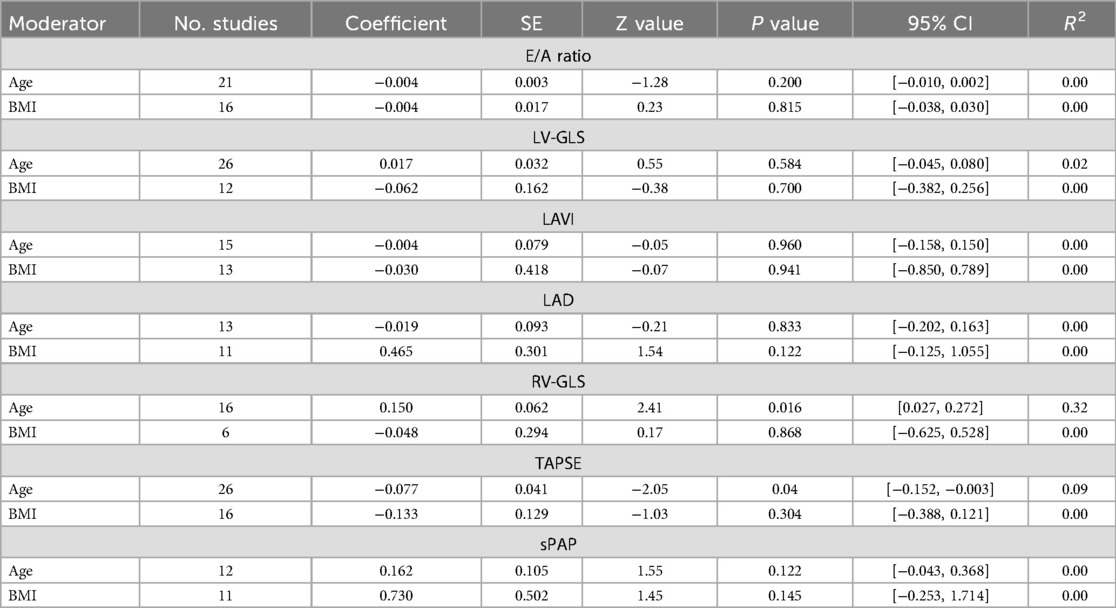
Table 5. Meta-regression results between echocardiographic indices and baseline characteristics of patients.
3.8 Publication bias
A clear publication bias was observed when examining LVEF, LAVI, LAD and sPAP. After applying Duval and Tweedie's trim and fill method, it was determined that 9 studies needed to be added on the right side of the scatter plot for LVEF analysis. Following this adjustment, the effect size was calculated to be −0.120, with a 95%CI of (−0.711, 0.471). In the case of LAVI analysis, 5 studies needed to be imputed on the right side of the scatter plot, resulting in a summary effect size of 1.92, with a 95%CI of (0.689, 3.168). For LAD, 4 studies needed to be added on the left side of the scatter plot. The adjusted effect size was calculated 0.800 with a 95%CI of (−0.115, 1.716). Lastly, for sPAP correction analysis, 5 studies required imputation on the left side of the scatter plot, leading to a summary effect size of 1.29, with a 95%CI of (0.882, 1.717). Funnel plots and findings of Egger's and Begg's tests for all indices are provided in supporting information (Supplementary S7 document).
4 Discussion
In the present systematic review and meta-analysis, we performed a pooled analysis of 66 studies to evaluate the effect of SARS-CoV-2 infection on cardiac function in post-COVID-19 survivors without a prior history of cardiac issues or abnormalities. Following strict inclusion and exclusion criteria, we identified 32 studies that met the eligibility criteria for meta-analysis. This meta-analysis revealed significant myocardial alterations in individuals who have recovered from COVID-19 when compared to control groups. Furthermore, differences were observed in the function of the right and left ventricles in post-COVID patients compared to controls, especially in subgroup analyses based on the time since the onset of acute COVID-19 and echocardiogram evaluation during recovery, the severity of the initial infection, and the presence of comorbidities.
4.1 Definition
“Long COVID” or “post-COVID syndrome” is the term used to describe the ongoing presence of symptoms after a SARS-CoV-2 infection, lasting for weeks or months, regardless of whether the virus is still present in the body. These symptoms can persist or come back intermittently and may consist of either lingering symptoms from the initial COVID infection or new symptoms (79). The National Institute for Health and Care Excellence (NICE), the Scottish Intercollegiate Guidelines Network, and the Royal College of General Practitioners have collaborated to develop guidelines for individuals who have recuperated from COVID-19 but are still facing symptoms. They have coined the terms “post-acute COVID-19” for symptoms persisting 4–12 weeks after the initial infection and “long-COVID” for symptoms lasting beyond 12 weeks (80).
4.2 Echocardiographic evaluation of long COVID
The current systematic review and meta-analysis found that chronic COVID-19 patients exhibit impaired cardiac function in both the right and left sides of the heart. Unlike in previous reviews and meta-analyses, these patients did not have any history of cardiac disease and/or comorbidities that could affect their cardiac function.
4.2.1 Left ventricular function
LV systolic dysfunction has been observed as a consequence of acute COVID-19 infection. Multiple studies have shown significant reductions in LVEF after 3 months of recovery from COVID-19, across a spectrum of symptoms and severity levels (24, 36, 39, 40). Additionally, there have been reports of reduced LVEF in chronic COVID-19 survivors, although these studies lacked a control group (70, 71, 75, 81).
LV-GLS provides valuable insight into LV function and is considered a more precise measure compared to LVEF (82). Long COVID patients, with and without a control group, were found to have reduced (less negative) LV-GLS (24, 25, 28, 31, 33, 34, 38, 66, 72, 77). However, there were reports of studies with no significant findings of LVEF and LV-GLS in long-COVID cases (26, 27, 37, 41, 74, 76, 78). The present meta-analysis revealed that individuals with long-COVID had significantly lower LV-GLS and LVEF compared to the control group. Unlike LVEF, decreased LV-GLS was also observed in COVID-19 patients with both mild and moderate-severe infections. Furthermore, reduced LVEF and LV-GLS were observed in COVID-19 patients with and without comorbidities compared to their matched groups.
Several studies have reported LV diastolic dysfunction in addition to LV systolic dysfunction. Long-COVID patients were found to have lower E/A and E/e' ratios compared to the control group (24, 28, 32, 36, 38, 40). In a study conducted by Sharma and colleagues (71), it was found that individuals with moderate to severe cases of COVID-19 had a greater likelihood of experiencing left ventricular diastolic dysfunction compared to those with mild cases when assessed through echocardiography six months post-infection (71). However, the present meta-analysis did not find any significant differences in E/e', E/A, mitral A wave, and mitral E wave between long-COVID patients and the control group.
Diastolic dysfunction is characterized by an irregular filling pattern in the left ventricle, often resulting in significant elevations in end-diastolic pressure during the filling of the ventricle (83). Left atrium enlargement is a key indicator of the structural remodeling process that occurs in reaction to chronically elevated LV end-diastolic pressure, typically resulting from diastolic dysfunction (83). In the current meta-analysis, it was found that LAD was significantly higher in long-COVID patients compared to the control group. However, there were no significant differences observed in LAVI between the two groups. In subgroup analysis, LAD and LAVI were increased in patients with history of moderate-severe COVID-19 infection and comorbid disease compared to their matched controls.
Additionally, abnormal LV shape can be a sign of both systolic and diastolic dysfunction. Several studies have shown that patients with long-lasting COVID-19 symptoms have significant alterations in LV geometric measurements (27, 32, 36, 38, 73, 75). In the current meta-analysis, it was observed that long-COVID patients exhibited lower LVM and IVSD compared to the control group, which could potentially suggest systolic dysfunction. Nevertheless, it is crucial to understand that lower LVM and IVSD levels may not necessarily signal systolic dysfunction. Instead, a decrease in LVM and IVSD may simply suggest a reduction in the size and thickness of the LV muscle. This decline could be attributed to factors like weight loss or reduced physical activity, which were not specifically examined in the present study (84).
Although long-COVID patients showed a decrease in LVM, further analysis by subgroup indicated an increase in LVM for those with moderate to severe COVID-19 infection and a decrease in LVM for those with mild infection. The pathophysiology of LV remodeling in the context of a SARS-CoV-2 infection is not fully understood, but it is likely related to the systemic inflammatory response triggered by the virus. It is suggested that COVID-19 can lead to a cytokine storm, where the immune system releases large amounts of pro-inflammatory cytokines in response to the infection. This excessive inflammation can damage the heart muscle and lead to LV hypertrophy over time (85). Moreover, these late pathological findings may be linked to the severity of the initial COVID-19 illness, the duration since the acute phase, and the presence of lingering symptoms (86).
4.2.2 Right ventricular function
Research suggests that individuals may be at increased risk for right ventricular dysfunction after experiencing a severe case of COVID-19. This vulnerability is thought to be caused by the damage to the lungs and the rise in pulmonary vascular resistance resulting from the virus (87, 88). Several studies have demonstrated evidence of impaired RV function in individuals who have recovered from acute COVID-19, ranging from mild to severe infection, despite having no pre-existing cardiac conditions, for a duration exceeding 3 months comparing to control group (26, 28, 32, 34, 35, 40). However, studies conducted without a control group found that RV function was preserved in long-COVID cases (66, 69, 71, 73, 75). In the study conducted by Chamtouri et al., patients with severe pulmonary lesions detected on CT scans had a higher probability of experiencing subclinical myocardial injury during the mid-term monitoring period (77). In the current meta-analysis, long-COVID patients showed significantly impaired RV echocardiographic indices including increased RV diameter, sPAP, RV-MPI and reduced RV-GLS (less negative) and TAPSE compared to the control group. Pulmonary remodeling can lead to increased sPAP and RV-MPI. This can have a negative impact on the function of the right ventricle, leading to reductions in TAPSE and RV-GLS (89). In the subgroup analysis grouped by COVID-19 severity of infection, reduced RV-GLS and TAPSE were only observed in moderate-severe post-COVID patients compared to the control group. Earlier research indicates that even mild cases of COVID-19 may result in lasting cardiovascular complications (90). There is a possible relationship between the severity of inflammation during acute infection and long-term RV-GLS measurements (26). In addition, previous studies have demonstrated that assessing RV-GLS can be beneficial in predicting outcomes for patients with ARDS (91). It is believed that inflammation contributes to an increased workload and damage to the RV, ultimately leading to RV failure, which can be evaluated through RV-GLS (92).
In patients with COVID-19, it is crucial to recognize subclinical RV dysfunction, as reduced RV strain has been associated with increased mortality (93). This type of dysfunction appears to be common during post-recovery monitoring, even in individuals without preexisting cardiovascular or respiratory conditions or signs of heart failure, indicating potential unrecognized heart damage and compromised circulation following COVID-19 (94, 95).
4.3 Echocardiographic evaluation of post-acute COVID-19
4.3.1 Left ventricular function
Among parameters indicating the LV systolic function, subgroup analysis showed impaired LV-GLS in post-COVID patients. Impaired LV-GLS was present in both mild infection and moderate-severe infections of COVID-19. Regarding the comorbidities, both patients with and without comorbid diseases had significant impairment in LV-GLS. These findings underscore the impact of COVID-19 on LVGLS, irrespective of disease severity or the presence of comorbidities. Samiei et al. (50) compared COVID-19 patients according to the severity of their infection. Reported LVGLS were in the normal range in all COVID-19 groups in their study (mild: −22.2 ± 2; moderate: −20.6 ± 2; severe: −19.3 ± 1). However, their investigation revealed that in the early recovery phase (1.5 months post-infection), LV-GLS was significantly lower in individuals who had suffered from a severe form of COVID-19 compared to others who experienced a milder clinical course. Özer et al. (51, 96) reported LVGLS below the normal range in the presence of COVID-19-caused myocardial injury (37) (−17.7 ± 2.6). They reported that myocardial LV-GLS values were impaired in one out of every three patients 1-month post-COVID-19 recovery. Tudoran et al. (58) conducted an assessment of the cardiac morphology and function in patients 1–3 months post-COVID-19 infection, analyzing and comparing the results based on the presence of cardiac abnormalities. They reported lower LV-GLS in 8.66% of patients with cardiac abnormalities. Since impaired LV-GLS shows subclinical myocardial deformation and is suggestive of LV-impaired systolic performance, post-acute COVID-19 patients are prone to the LV systolic dysfunction.
Moreover, the current meta-analysis on LV diastolic parameters found that the mitral A wave was significantly elevated and the E/A ratio was notably reduced when compared to the control group. This difference was both present in mild and moderate-severe COVID-19 infections. Furthermore, the difference was significant among patients with comorbid diseases. Sollazzo et al. (52) evaluated the cardiac function of athletes after mild or moderate COVID-19 infection. According to their findings, significant difference was observed in the E/A ratio, one month after the COVID-19 infection. Tudoran et al. (58) also reported an E/A ratio over 2, thus a type III diastolic dysfunction; and an E/A ratio of under 0.8, thus a type I diastolic dysfunction in a subset of their patients. According to these results, it can be concluded that COVID-19 causes impaired relaxation and consequently LV diastolic dysfunction in the early recovery phase.
Additionally, a notable disparity in LAVI was seen between post-acute COVID-19 patients and the control groups. The difference was seen in both mild infection and moderate-severe infection and the presence of comorbid diseases. Tudoran et al. (58) also reported increased LAVI in patients with cardiac abnormality 1–3 months after COVID-19 infection. COVID-19 infection might cause left atrial remodeling in the initial diastolic dysfunction phase by increased participation of left atrial active contraction to surpass the relaxation difficulty and thus, leading to A wave increase as well.
4.3.2 Right ventricular function
In the present meta-analysis, only RV diameter showed a significant decrease in COVID-19 patients compared to the control groups. Erdem et al. (61) compared COVID-19 patients according to their hospitalization status and pulmonary involvement. Unlike the results of our meta-analysis, their findings showed that 2–3 months after recovering from COVID-19, RVD is increased in patients without a history of risk factors. Furthermore, the increases correlate with the severity of COVID-19 and the extent of pulmonary involvement. Tudoran et al. (55) compared patients with and without pulmonary hypertension following COVID-19 infection. As expected, RV diameter was significantly higher in patients with pulmonary hypertension. This controversy in the results might be due to the low number of studies included in our meta-analysis (5 studies). Although the occurrence of diastolic dysfunction prior to the systolic dysfunction might contribute to the decreased LV diameter.
4.4 A review of previous systematic reviews
Our search identified three systematic reviews (97–99) and two meta-analyses (100, 101) that investigated cardiac function in COVID-19 survivors.
In the meta-analysis conducted by Rahmati et al., a total of 21 studies were included (100). The inclusion criteria were studies that examined individuals who had recovered from COVID-19, in comparison to a control group, and presented findings of cardiac indices measured through Cardiac Magnetic Resonance (CMR), or echocardiography. They reported significant decrease in LVEDV, LVSV and LVEF in COVID-19 survivors compared to controls [(standardized mean difference (SMD) = −0.39, 95% CI = −0.56 to −0.22, p = 0.00001), (MD = −4.33, 95% CI = −5.72 to −2.94, p = 0.0000), and (SMD = −0.18, 95% CI = −0.34 to −0.01, p = 0.04), respectively]. No significant results were observed in LVEF across different post-COVID-19 follow-up periods in subgroup analysis. However, a decrease in LVEF was only evident where patients had a prior history of ICU admission grouped by the severity of COVID-19. LVM was significantly increased in COVID-19 cases in comparison to controls [(SMD = 0.23, 95%CI = 0.05–0.40, p = 0.01)]. Subgroup analysis showed that LVM started to increase significantly 3 months after recovery from COVID-19 infection. A meta-analysis of 5 studies showed a reduction of LV-GLS in recovered COVID-19 patients compared to controls (MD = −1.52, 95% CI = −1.64 to −0.97, p = 0.00001). Subgroup analysis revealed a decrease in LV-GLS would exist 2 months to 1 year after recovery. In terms of right heart indices, there was a significant reduction in RVEF, RVEDV, RVESV, RVSV and TAPSE of COVID-19 survivors compared to controls [(SMD = −0.29, 95% CI = −0.50 to −0.09, p = 0.005), (SMD = −0.42, 95% CI = −0.55 to −0.29, p = 0.00001), (SMD = −0.16, 95% CI = −0.29 to −0.03, p = 0.02), (MD = −0.50, 95% CI = −0.75 to −0.205, p = 0.0001) and (SMD = −0.91, 95% CI = −1.30 to −0.51, p = 0.00001), respectively] (100). Subgroup analysis revealed that TAPSE was reduced between 2 months and 1year post-COVID-19 recovery, while RVEF was reduced between 2 and 6 months after recovery. Subgroup analysis based on the severity of the acute COVID-19 phase and subsequent chronic outcome demonstrated a decrease in RVEF and RVESV only in patients who had been admitted to the ICU.
In the meta-analysis by Herold et al., they reviewed 32 CMR studies involving patients with COVID-19 that utilized myocardial longitudinal magnetization relaxation time constant (T1), transverse magnetization relaxation time constant (T2) mapping, extracellular volume, and late gadolinium enhancement (101). The authors suggested that T1 and T2 provided dynamic measures of cardiac involvement in COVID-19 survivors, indicating the improvement of cardiomyocyte injury and myocardial inflammation during recovery. In contrast, late gadolinium enhancement and, to a lesser extent, extracellular volume are seen as more static markers influenced by preexisting risk factors linked to adverse changes in myocardial tissue (101).
Ramadan et al. performed a comprehensive review to evaluate heart complications following recovery from COVID-19 (99). Of the studies analyzed, 12 employed CMR while 9 utilized echocardiography for cardiac function assessment. The median time for CMR evaluation was 63 days. The results indicated higher T1 intensity in 19% of cases, late gadolinium enhancement in 12% of cases, increased T2 intensity in 7% of cases, reduced GLS in 5% of cases, and decreased LVEF in 1.5%. In the echocardiography evaluation, the median time was 41 days. Reported outcomes included reduced LVEF, global hypokinesis, LV hypertrophy, diastolic dysfunction, pulmonary hypertension, and reduced GLS. Moreover, in the 3 to 6-month follow-up period, results showed a 30% decrease in LV-GLS, late gadolinium enhancement in 10% of cases on CMR, and diastolic dysfunction in 40% of cases on echocardiography (99).
Elhiny et al. conducted a review focusing on post-COVID-19 complications, including cardiovascular complications, in adults (97). Out of the studies analyzed, only three studies provided information on cardiac function assessment via imaging techniques. Two of these studies highlighted abnormal findings in CMR imaging. One study reported myocardial edema in 54% of COVID-19 survivors and positive late gadolinium enhancement in 31% of patients. Another study documented elevated myocardial native T1 and T2 values, myocardial, late gadolinium enhancement, pericardial enhancement, and reduced LVEF (less than 50%) in a subset of patients. Additionally, one study utilized trans-thoracic echocardiography to assess cardiac function, revealing a high prevalence of diastolic dysfunction, pulmonary hypertension, and pericardial effusion. Overall, this systematic review primarily focused on post-COVID-19 symptoms and complications across various organs, and the evaluation of cardiac function was found to be limited in scope.
Hassani et al. conducted a systematic review summarizing the CMR findings of COVID-19 adult survivors as reported in all available case series and cohort studies (98). Median follow-up time to MRI was at least 2 weeks after hospital discharge or diagnosis. The authors pooled data from 12 cohorts and 10 case series. Regarding the cardiac function, in 4 out of 8 cohort studies, RVEF was significantly lower than that in the control group. Mean/median LVEF fell in the normal range in all studies. However, six studies also reported the number of cases with LVEF <50%. One study found that RV and LV strains significantly decreased in COVID-19 with late gadolinium enhancement compared to those without late gadolinium enhancement and normal controls.
In contrast to earlier systematic reviews, we implemented measures to eliminate the possible interference of pre-existing heart conditions on the cardiac function of individuals who have recovered from COVID-19. Instead of combining echocardiography and CMR in the present meta-analysis like previous assessments, we focused solely on echocardiography to reduce variability and improve the accuracy of our results. Additionally, we conducted subgroup analysis to provide a more thorough understanding of the data. Moreover, we have incorporated a greater quantity of recent studies to validate the strength of our conclusions.
4.5 Limitations
It is important to acknowledge certain limitations when comparing the provided reports. To eliminate the heterogeneity of studies and the reported echocardiographic measurements, a subgroup meta-analysis was conducted. However, it is important to note that due to intrinsic limitations of the studies included, some degree of heterogeneity was inevitable. The parameters evaluated in echocardiography exhibited a wide range of variability, making it impossible to assess all the reported parameters. Additionally, there are several factors that may impact the function of the LV and RV, such as the vaccination status of patients and the treatment approach for COVID-19 infection during the acute phase. Unfortunately, the absence of data on these two variables in the research it was not feasible to compare the data.
4.6 Implications for research, practice and policy
Further research should focus on identifying risk factors for developing cardiac complications following COVID-19, as well as potential preventive measures and investigate potential treatments and interventions to prevent or manage cardiac dysfunction in COVID-19 survivors. Healthcare providers should be aware of the increased risk of cardiac dysfunction in individuals who have recovered from COVID-19, even if they did not experience severe symptoms during their initial illness. Regular cardiac monitoring and follow-up assessments should be considered for COVID-19 survivors, especially those with pre-existing cardiovascular conditions or other risk factors for heart disease. Policy makers should consider implementing guidelines for cardiac screening and follow-up care for COVID-19 survivors, to ensure early detection and appropriate management of cardiac dysfunction.
5 Conclusion
This systematic review discusses emerging research on the possible development of cardiac dysfunction following the resolution of acute COVID-19 infection. Findings revealed subclinical changes in both left and right ventricular systolic and diastolic function among post-acute and long COVID patients without a prior history of heart disease, including individuals lacking cardiovascular risk factors such as diabetes and hypertension, irrespective of the severity of their initial illness. While these changes remained within normal limits, they were markedly different from those in non-COVID control subjects, indicating potential underlying issues that warrant further exploration.
Data availability statement
The original data are presented in original full-texts of studies included in this review. Data-set are presented in forest plots/Supplementary Material, further inquiries can be directed to the corresponding author.
Author contributions
MD: Conceptualization, Data curation, Formal Analysis, Funding acquisition, Investigation, Methodology, Project administration, Resources, Software, Supervision, Validation, Visualization, Writing – original draft, Writing – review & editing. ST-M: Conceptualization, Data curation, Formal Analysis, Funding acquisition, Investigation, Methodology, Project administration, Resources, Software, Supervision, Validation, Visualization, Writing – original draft, Writing – review & editing. RK: Data curation, Methodology, Project administration, Supervision, Validation, Visualization, Writing – review & editing. SY: Methodology, Project administration, Supervision, Validation, Visualization, Writing – review & editing. YP: Data curation, Methodology, Visualization, Writing – review & editing.
Funding
The author(s) declare that no financial support was received for the research, authorship, and/or publication of this article.
Acknowledgments
We extend our sincere gratitude to ChatGPT, an advanced language model developed by OpenAI, for its invaluable assistance in the refinement of our manuscript. ChatGPT played a crucial role in paraphrasing complex sentences and rectifying grammatical intricacies, thereby enhancing the clarity and coherence of our writing.
Conflicts of interest
The authors declare that the research was conducted in the absence of any commercial or financial relationships that could be construed as a potential conflict of interest.
Publisher's note
All claims expressed in this article are solely those of the authors and do not necessarily represent those of their affiliated organizations, or those of the publisher, the editors and the reviewers. Any product that may be evaluated in this article, or claim that may be made by its manufacturer, is not guaranteed or endorsed by the publisher.
Supplementary material
The Supplementary Material for this article can be found online at: https://www.frontiersin.org/articles/10.3389/fcvm.2024.1458389/full#supplementary-material
Supplementary S1 Document | PRISMA 2020 Checklist for systematic reviews and meta-analyses.
Supplementary S2 Document | Table of search strategy conducted in online databases.
Supplementary S3 Document | Table of excluded studies during final screening.
Supplementary S4 Document | Forest plots of meta-analysis.
Supplementary S5 Document | Forest plots of sensitivity analysis.
Supplementary S6 Document | Scatter plots of meta-regression.
Supplementary S7 Document | Funnel plots of meta-analysis.
Abbreviations
LVEDD, left ventricular end-diastolic diameter; LVESD, left ventricular end-systolic diameter; LVEDV, left ventricular end-diastolic volume; LVESV, left ventricular end-systolic volume; LVEF, left ventricular ejection fraction; PWD, posterior wall diameter; IVSD, interventricular septum diameter; LVM, left ventricular mass; LVMI, left ventricular mass index; LV-GLS, left ventricular global longitudinal strain; LAD, left atrium diameter; LAVI, left atrium volume index; LV-MPI, left ventricular myocardial performance index; E/A, the ratio of peak velocity blood flow from left ventricular relaxation in early diastole (the E wave) to peak velocity flow in late diastole caused by atrial contraction (the A wave); E/e', the ratio of E wave to early diastolic mitral annular velocity (E'); RVD, right ventricular diameter; RAD, right atrium diameter; RV-GLS, right ventricular global longitudinal strain; TAPSE, tricuspid annular plane systolic excursion; sPAP, systolic pulmonary artery pressure; RV-MPI, right ventricular myocardial performance index.
References
1. Elhiny R, Al-Jumaili AA, Yawuz MJ. What might COVID-19 patients experience after recovery? A comprehensive review. Int J Pharm Pract. (2022) 30(5):404–13. doi: 10.1093/ijpp/riac026
2. Arcari L, Luciani M, Cacciotti L, Musumeci MB, Spuntarelli V, Pistella E, et al. Incidence and determinants of high-sensitivity troponin and natriuretic peptides elevation at admission in hospitalized COVID-19 pneumonia patients. Intern Emerg Med. (2020) 15(8):1467–76. doi: 10.1007/s11739-020-02498-7
3. Arcari L, Luciani M, Cacciotti L, Pucci M, Musumeci MB, Pietropaolo L, et al. Coronavirus disease 2019 in patients with cardiovascular disease: clinical features and implications on cardiac biomarkers assessment. J Cardiovasc Med (Hagerstown). (2021) 22(11):832–9. doi: 10.2459/JCM.0000000000001252
4. Xiong Q, Xu M, Li J, Liu Y, Zhang J, Xu Y, et al. Clinical sequelae of COVID-19 survivors in Wuhan, China: a single-centre longitudinal study. Clin Microbiol Infect. (2021) 27(1):89–95. doi: 10.1016/j.cmi.2020.09.023
5. Parhizgar P, Yazdankhah N, Rzepka AM, Chung KYC, Ali I, Fur RLF, et al. Beyond acute COVID-19: a review of long-term cardiovascular outcomes. Can J Cardiol. (2023) 39(6):726–40. doi: 10.1016/j.cjca.2023.01.031
6. Becker RC. Anticipating the long-term cardiovascular effects of COVID-19. J Thromb Thromb. (2020) 50(3):512–24. doi: 10.1007/s11239-020-02266-6
7. Kumar K, Seetharam K, Poonam F, Gulati A, Sadiq A, Shetty V. The role of cardiac imaging in the evaluation of cardiac involvement in systemic diseases. Cureus. (2021) 13(12):e20708. doi: 10.7759/cureus.20708
8. Tian L, Duan F, Li X, Zhou C. Incidence, risk factors and prognostic effect of imaging left ventricular diastolic dysfunction in patients with COVID-19: protocol for a systematic review. BMJ Open. (2022) 12(4):e059281. doi: 10.1136/bmjopen-2021-059281
9. Sewanan LR, Clerkin KJ, Tucker NR, Tsai EJ. How does COVID-19 affect the heart? Curr Cardiol Rep. (2023) 25(3):171–84. doi: 10.1007/s11886-023-01841-6
10. Moher D, Liberati A, Tetzlaff J, Altman DG. Preferred reporting items for systematic reviews and meta-analyses: the PRISMA statement. PLoS Med. (2009) 6(7):e1000097. doi: 10.1371/journal.pmed.1000097
11. Sterne JA, Hernán MA, Reeves BC, Savović J, Berkman ND, Viswanathan M, et al. ROBINS-I: a tool for assessing risk of bias in non-randomised studies of interventions. Br Med J. (2016) 355:i4919. doi: 10.1136/bmj.i4919
12. Guyatt G, Oxman AD, Akl EA, Kunz R, Vist G, Brozek J, et al. GRADE guidelines: 1. Introduction—GRADE evidence profiles and summary of findings tables. J Clin Epidemiol. (2011) 64(4):383–94. doi: 10.1016/j.jclinepi.2010.04.026
13. Lakatos BK, Tokodi M, Fábián A, Ladányi Z, Vágó H, Szabó L, et al. Frequent constriction-like echocardiographic findings in elite athletes following mild COVID-19: a propensity score-matched analysis. Front Cardiovasc Med. (2022) 8:760651. doi: 10.3389/fcvm.2021.760651
14. Günay N, Demiröz Ö, Kahyaoğlu M, Başlılar Ş, Aydın M, Özer M, et al. The effect of moderate and severe COVID-19 pneumonia on short-term right ventricular functions: a prospective observational single pandemic center analysis. Int J Cardiovasc Imaging. (2021) 37(6):1883–90. doi: 10.1007/s10554-021-02171-w
15. Gul M, Ozyilmaz S, Bastug Gul Z, Kacmaz C, Satilmisoglu MH. Evaluation of cardiac injury with biomarkers and echocardiography after COVID-19 infection. J Physiol Pharmacol. (2022) 73(1):89–95. doi: 10.26402/jpp.2022.1.09
16. Tryfou ES, Kostakou PM, Chasikidis CG, Kostopoulos VS, Serafetinidis II, Ferdianaki EK, et al. Biventricular myocardial function in COVID-19 recovered patients assessed by speckle tracking echocardiography: a prospective cohort echocardiography study. Int J Cardiovasc Imaging. (2022) 38(5):995–1003. doi: 10.1007/s10554-021-02498-4
17. Turpin VG, Parr SK, Hammond ST, White ZJ, Tickner PJ, Chisam CE, et al. Cardiac changes in collegiate athletes following SARS-CoV-2 infection and quarantine: a prospective case-control study. Ann Med. (2023) 55(2):2269586. doi: 10.1080/07853890.2023.2269586
18. Kurtoğlu E, Afsin A, Aktaş İ, Aktürk E, Kutlusoy E, Çağaşar Ö. Altered cardiac autonomic function after recovery from COVID-19. Ann Noninvasive Electrocardiol. (2022) 27(1):e12916. doi: 10.1111/anec.12916
19. Schellenberg J, Ahathaller M, Matits L, Kirsten J, Kersten J, Steinacker JM. Left ventricular global longitudinal strain as a parameter of mild myocardial dysfunction in athletes after COVID-19. J Cardiovasc Dev Dis. (2023) 10(5):189. doi: 10.3390/jcdd10050189
20. Honchar O, Ashcheulova T, Chumachenko T, Chumachenko D, Bobeiko A, Blazhko V, et al. A prognostic model and pre-discharge predictors of post-COVID-19 syndrome after hospitalization for SARS-CoV-2 infection. Front Public Health. (2023) 11:1276211. doi: 10.3389/fpubh.2023.1276211
21. Mahajan S, Kunal S, Shah B, Garg S, Palleda GM, Bansal A, et al. Left ventricular global longitudinal strain in COVID-19 recovered patients. Echocardiography. (2021) 38(10):1722–30. doi: 10.1111/echo.15199
22. Turan T, Özderya A, Şahin S, Konuş AH, Kul S, Akyüz AR, et al. Left ventricular global longitudinal strain in low cardiac risk outpatients who recently recovered from coronavirus disease 2019. Int J Cardiovasc Imaging. (2021) 37(10):2979–89. doi: 10.1007/s10554-021-02376-z
23. Ardahanli I, Akhan O, Sahin E, Akgun O, Gurbanov R. Myocardial performance index increases at long-term follow-up in patients with mild to moderate COVID-19. Echocardiography. (2022) 39(4):620–5. doi: 10.1111/echo.15340
24. De A, Bansal M. Clinical profile and the extent of residual myocardial dysfunction among patients with previous coronavirus disease 2019. Int J Cardiovasc Imaging. (2023) 39(5):887–94. doi: 10.1007/s10554-022-02787-6
25. Baltodano-Arellano R, Cupe-Chacalcaje K, Rojas P, Meneses G, Urdanivia-Ruiz D, Rafael-Horna E, et al. Comparative analysis of myocardial deformation in patients recovered from mild SARS-CoV-2 infection. Arch Peru Cardiol Cir Cardiovasc. (2021) 2(4):227–32. doi: 10.47487/apcyccv.v2i4.175
26. Akkaya F, Yenerçağ FNT, Kaya A, Şener YZ, Bağcı A. Long term effects of mild severity COVID-19 on right ventricular functions. Int J Cardiovasc Imaging. (2021) 37(12):3451–7. doi: 10.1007/s10554-021-02340-x
27. Akbulut M, Tan S, Gerede Uludağ DM, Kozluca V, Dinçer İ. Evaluation of cardiac function in uncomplicated COVID-19 survivors by 2-dimensional speckle tracking imaging. Anatol J Cardiol. (2022) 26(11):841–8. doi: 10.5152/AnatolJCardiol.2022.1360
28. Baykiz D, Govdeli EA, Ozer PK, Karaayvaz EB, Catma Y, Medetalibeyoglu A, et al. Evaluation the relationship of left ventricular global longitudinal strain and laboratory parameters in discharged patients with COVID-19: a follow-up study. Int J Cardiovasc Imaging. (2021) 37(8):2451–64. doi: 10.1007/s10554-021-02228-w
29. Rasmusen HK, Aarøe M, Madsen CV, Gudmundsdottir HL, Mertz KH, Mikkelsen AD, et al. The COVID-19 in athletes (COVA) study: a national study on cardio-pulmonary involvement of SARS-CoV-2 infection among elite athletes. Eur Clin Respir J. (2023) 10(1):2149919. doi: 10.1080/20018525.2022.2149919
30. Beaudry RI, Brotto AR, Varughese RA, de Waal S, Fuhr DP, Damant RW, et al. Persistent dyspnea after COVID-19 is not related to cardiopulmonary impairment; a cross-sectional study of persistently dyspneic COVID-19, non-dyspneic COVID-19 and controls. Front Physiol. (2022) 13:917886. doi: 10.3389/fphys.2022.917886
31. Gherbesi E, Bergamaschi L, Cusmano I, Tien TT, Paolisso P, Foà A, et al. The usefulness of speckle tracking echocardiography in identifying subclinical myocardial dysfunction in young adults recovered from mild COVID-19. Echocardiography. (2022) 39(9):1190–7. doi: 10.1111/echo.15431
32. Gumanova NG, Gorshkov AU, Bogdanova NL, Korolev AI. Effects of COVID-19 infection in healthy subjects on cardiac function and biomarkers of oxygen transport, blood coagulation and inflammation. Viruses. (2023) 15(8):1623. doi: 10.3390/v15081623
33. Küçük U, Gazi E, Duygu A, Akşit E. Evaluation of aortic elasticity parameters in survivors of COVID-19 using echocardiography imaging. Med Princ Pract. (2022) 31(3):276–83. doi: 10.1159/000522626
34. Lambadiari V, Mitrakou A, Kountouri A, Thymis J, Katogiannis K, Korakas E, et al. Association of COVID-19 with impaired endothelial glycocalyx, vascular function and myocardial deformation 4 months after infection. Eur J Heart Fail. (2021) 23(11):1916–26. doi: 10.1002/ejhf.2326
35. Barros LSA, Castillo JM, Lacerda HR. Abnormal right ventricular echocardiographic findings in recovered patients associated with severe acute respiratory syndrome in COVID-19. Echocardiography. (2023) 40(3):227–34. doi: 10.1111/echo.15538
36. Tudoran C, Bende F, Bende R, Giurgi-Oncu C, Dumache R, Tudoran M. Correspondence between aortic and arterial stiffness, and diastolic dysfunction in apparently healthy female patients with post-acute COVID-19 syndrome. Biomedicines. (2023) 11(2):492. doi: 10.3390/biomedicines11020492
37. Yang J, Liu P, Zhong M, Luo T, Lei G, Liao C. Effect evaluation of echocardiography on right ventricular function in patients after the recovering from coronavirus disease 2019. Comput Math Methods Med. (2022) 2022:6161015. doi: 10.1155/2022/6161015
38. Rácz G, Takács H, Kormányos Á, Polestyuk B, Borbás J, Gyenes N, et al. Screening for myocardial injury after mild SARS-CoV-2 infection with advanced transthoracic echocardiography modalities. Diagnostics (Basel). (2022) 12(8):1941. doi: 10.3390/diagnostics12081941
39. Rajotiya S, Mishra S, Singh AK, Singh P, Bareth H, Singh M, et al. Post-COVID-19 cardio-pulmonary manifestations after 1-year of SARS-CoV-2 infection among Indian population: a single centre, case-control study (OneCoV2 study). J Infect Public Health. (2024) 17(1):145–51. doi: 10.1016/j.jiph.2023.11.013
40. Ozer PK, Govdeli EA, Baykiz D, Karaayvaz EB, Medetalibeyoglu A, Catma Y, et al. Impairment of right ventricular longitudinal strain associated with severity of pneumonia in patients recovered from COVID-19. Int J Cardiovasc Imaging. (2021) 37(8):2387–97. doi: 10.1007/s10554-021-02214-2
41. Uziębło-Życzkowska B, Krzesiński P, Domino B, Chciałowski A, Maciorowska M, Gielerak G. Echocardiographic assessment of cardiac function after mild coronavirus disease 2019: a preliminary report. J Clin Ultrasound. (2022) 50(1):17–24. doi: 10.1002/jcu.23094
42. Wood G, Kirkevang TS, Agergaard J, Leth S, Hansen ESS, Laustsen C, et al. Cardiac performance and cardiopulmonary fitness after infection with SARS-CoV-2. Front Cardiovasc Med. (2022) 9:871603. doi: 10.3389/fcvm.2022.871603
43. Cotella JI, Hasbani J, Hasbani E, Prado A. Abnormal longitudinal strain reduction of basal left ventricular segments in patients recovered of COVID-19. J Cardiovasc Echogr. (2022) 32(2):107–11. doi: 10.4103/jcecho.jcecho_138_20
44. Taş S, Taş Ü. Effects of COVID-19 on the autonomic cardiovascular system: heart rate variability and turbulence in recovered patients. Tex Heart Inst J. (2023) 50(4):227952. doi: 10.14503/THIJ-22-7952
45. Hamdy RM, Abdelaziz OH, Shamsseldain HE, Eltrawy HH. Functional outcomes in post COVID-19 patients with persistent dyspnea: multidisciplinary approach. Int J Cardiovasc Imaging. (2023) 39(6):1115–22. doi: 10.1007/s10554-023-02819-9
46. ZeinElabdeen SG, Sherif A, Kandil NT, Altabib AMO, Abdelrashid MA. Left atrial longitudinal strain analysis in long COVID-19 syndrome. Int J Cardiovasc Imaging. (2023) 39(5):939–44. doi: 10.1007/s10554-023-02801-5
47. Zein ESG, El-Dosouky II, ELShabrawy AM, El Maghawry LM. Atrial electromechanical delay in post-COVID-19 postural orthostatic tachycardia: innocent bystander or pathologic factor. Indian Heart J. (2023) 75(4):292–7. doi: 10.1016/j.ihj.2023.06.001
48. Sarıçam E, Dursun AD, Türkmen Sarıyıldız G, Can N, Bozkurt E, Gönüllü U, et al. Laboratory and imaging evaluation of cardiac involvement in patients with post-acute COVID-19. Int J Gen Med. (2021) 14:4977–85. doi: 10.2147/IJGM.S321156
49. Tabacof L, Wood J, Breyman E, Tosto-Mancuso J, Kelly A, Wilkey K, et al. Dysautonomia, but not cardiac dysfunction, is common in a cohort of individuals with long COVID. J Pers Med. (2023) 13(11):1606. doi: 10.3390/jpm13111606
50. Samiei N, Rahnamoun Z, Kamali M, Asadian S, Rezaei Y, Ghadrdoost B, et al. Paradoxical increase in left atrial strains early after COVID-19 infection, a result of comprehensive recovery phase four-chamber strains study. Int J Cardiovasc Imaging. (2023) 39(8):1437–47. doi: 10.1007/s10554-023-02865-3
51. Özer S, Candan L, Özyıldız AG, Turan OE. Evaluation of left ventricular global functions with speckle tracking echocardiography in patients recovered from COVID-19. Int J Cardiovasc Imaging. (2021) 37(7):2227–33. doi: 10.1007/s10554-021-02211-5
52. Sollazzo F, Pengue L, Monti R, DI Murro E, Cea G, Modica G, et al. Italian return to play protocol after COVID-19 in young competitive and professional athletes: diagnostic yield and cost-benefit analysis. Minerva Cardiol Angiol. (2023) 71(3):233–41. doi: 10.23736/S2724-5683.22.06191-9
53. Tudoran C, Tudoran M, Pop GN, Giurgi-Oncu C, Cut TG, Lazureanu VE, et al. Associations between the severity of the post-acute COVID-19 syndrome and echocardiographic abnormalities in previously healthy outpatients following infection with SARS-CoV-2. Biology (Basel). (2021) 10(6):469. doi: 10.3390/biology10060469
54. Tudoran M, Tudoran C, Lazureanu VE, Marinescu AR, Pop GN, Pescariu AS, et al. Alterations of left ventricular function persisting during post-acute COVID-19 in subjects without previously diagnosed cardiovascular pathology. J Pers Med. (2021) 11(3):225. doi: 10.3390/jpm11030225
55. Tudoran C, Tudoran M, Lazureanu VE, Marinescu AR, Pop GN, Pescariu AS, et al. Evidence of pulmonary hypertension after SARS-CoV-2 infection in subjects without previous significant cardiovascular pathology. J Clin Med. (2021) 10(2):199. doi: 10.3390/jcm10020199
56. Tudoran C, Tudoran M, Lazureanu VE, Marinescu AR, Cut TG, Oancea C, et al. Factors influencing the evolution of pulmonary hypertension in previously healthy subjects recovering from a SARS-CoV-2 infection. J Clin Med. (2021) 10(22):5272. doi: 10.3390/jcm10225272
57. Tudoran C, Tudoran M, Cut TG, Lazureanu VE, Bende F, Fofiu R, et al. The impact of metabolic syndrome and obesity on the evolution of diastolic dysfunction in apparently healthy patients suffering from post-COVID-19 syndrome. Biomedicines. (2022) 10(7):1519. doi: 10.3390/biomedicines10071519
58. Tudoran C, Tudoran M, Cut TG, Lazureanu VE, Oancea C, Marinescu AR, et al. Evolution of echocardiographic abnormalities identified in previously healthy individuals recovering from COVID-19. J Pers Med. (2022) 12(1):46. doi: 10.3390/jpm12010046
59. Tudoran C, Bende R, Bende F, Giurgi-Oncu C, Enache A, Dumache R, et al. Connections between diabetes mellitus and metabolic syndrome and the outcome of cardiac dysfunctions diagnosed during the recovery from COVID-19 in patients without a previous history of cardiovascular diseases. Biology (Basel). (2023) 12(3):370. doi: 10.3390/biology12030370
60. Bende F, Tudoran C, Sporea I, Fofiu R, Bâldea V, Cotrău R, et al. A Multidisciplinary approach to evaluate the presence of hepatic and cardiac abnormalities in patients with post-acute COVID-19 syndrome—a pilot study. J Clin Med. (2021) 10(11):2507. doi: 10.3390/jcm10112507
61. Erdem K, Duman A. Pulmonary artery pressures and right ventricular dimensions of post-COVID-19 patients without previous significant cardiovascular pathology. Heart Lung. (2023) 57:75–9. doi: 10.1016/j.hrtlng.2022.08.023
62. Kujur PP, Jhala M, Bhondve A, Lanjewar C, Matta R, Deshmukh H. Left ventricular global longitudinal strain imaging in identifying subclinical myocardial dysfunction among COVID-19 survivors. Indian Heart J. (2022) 74(1):51–5. doi: 10.1016/j.ihj.2021.12.007
63. Vera-Pineda R, Carrizales-Sepúlveda EF, Morales-Rendón EJ, Ordaz-Farías A, Solís JG, Benavides-González MA, et al. Echocardiographic manifestations during the first 3 months after an episode of COVID-19 and their relationship with disease severity and persistence of symptoms. Am J Med Sci. (2023) 366(1):32–7. doi: 10.1016/j.amjms.2023.04.002
64. Osada SS, Szeghy RE, Stute NL, Province VM, Augenreich MA, Putnam A, et al. Monthly transthoracic echocardiography in young adults for 6 months following SARS-CoV-2 infection. Physiol Rep. (2023) 11(1):e15560. doi: 10.14814/phy2.15560
65. Can Y, Kocayigit I, Kocayiğit H, Sarıbıyık Çakmak B, Şahinöz M, Akdemir R. Ongoing effects of SARS-CoV-2 infection on arterial stiffness in healthy adults. Angiology. (2024) 75(2):116–21. doi: 10.1177/00033197231183227
66. Yaroslavskaya EI, Gorbatenko EA, Krinochkin DV, Shirokov NE, Osokina NA, Migacheva AV, et al. Predictors of reduced left ventricle global longitudinal strain one year after COVID-19 pneumonia. Kardiologiia. (2023) 63(12):39–45. doi: 10.18087/cardio.2023.12.n2415
67. Luchian ML, Motoc A, Lochy S, Magne J, Belsack D, De Mey J, et al. Subclinical myocardial dysfunction in patients with persistent dyspnea one year after COVID-19. Diagnostics (Basel). (2021) 12(1):57. doi: 10.3390/diagnostics12010057
68. Matejova G, Radvan M, Bartecku E, Kamenik M, Koc L, Horinkova J, et al. Cardiac sequelae after COVID-19: results of a 1-year follow-up study with echocardiography and biomarkers. Front Cardiovasc Med. (2022) 9:1067943. doi: 10.3389/fcvm.2022.1067943
69. Wu X, Deng KQ, Li C, Yang Z, Hu H, Cai H, et al. Cardiac involvement in recovered patients from COVID-19: a preliminary 6-month follow-up study. Front Cardiovasc Med. (2021) 8:654405. doi: 10.3389/fcvm.2021.654405
70. Stavileci B, Özdemir E, Özdemir B, Ereren E, Cengiz M. De-novo development of fragmented QRS during a six-month follow-up period in patients with COVID-19 disease and its cardiac effects. J Electrocardiol. (2022) 72:44–8. doi: 10.1016/j.jelectrocard.2022.02.012
71. Sharma D, Rohila A, Deora S, Garg MK, Misra S. Cardiac assessment of patients during post COVID-19 recovery phase: a prospective observational study. Glob Cardiol Sci Pract. (2022) 2022(3):e202218. doi: 10.21542/gcsp.2022.18
72. Ródenas-Alesina E, Rodríguez-Palomares J, Bach-Oller M, Jordán P, Badia C, Herrador L, et al. Echocardiographic assessment of COVID19 sequelae in survivors with elevated cardiac biomarkers. Int J Cardiol. (2022) 360:104–10. doi: 10.1016/j.ijcard.2022.04.070
73. Chudzik M, Lewek J, Kapusta J, Banach M, Jankowski P, Bielecka-Dabrowa A. Predictors of long COVID in patients without comorbidities: data from the polish long-COVID cardiovascular (PoLoCOV-CVD) study. J Clin Med. (2022) 11(17):4980. doi: 10.3390/jcm11174980
74. Kattamuri LP, Sharma V, Sarda R, Sharma K, Ajayababu A, Gupta G, et al. Cardiopulmonary outcomes in COVID-19 patients discharged from a tertiary care center: a prospective study. Natl Acad Sci Lett. (2023) 46(6):571–8. doi: 10.1007/s40009-023-01236-9
75. Hamburger RF, Taha Y, Ruzieh M, Clugston JR, Handberg EM, Reifsteck F, et al. Longitudinal cardiac remodeling in collegiate American football players as assessed by echocardiography during their collegiate career. Clin Cardiol. (2023) 46(9):1090–6. doi: 10.1002/clc.24121
76. D'Ávila LBO, Milani M, Le Bihan DCS, de Lima A, Milani J, Cipriano GFB, et al. Longitudinal strain and myocardial work in symptomatic patients having recovered from COVID-19 and possible associations with the severity of the disease. Int J Cardiovasc Imaging. (2024) 40(4):745–56. doi: 10.1007/s10554-023-03042-2
77. Chamtouri I, Kaddoussi R, Abroug H, Abdelaaly M, Lassoued T, Fahem N, et al. Mid-term subclinical myocardial injury detection in patients who recovered from COVID-19 according to pulmonary lesion severity. Front Cardiovasc Med. (2022) 9:950334. doi: 10.3389/fcvm.2022.950334
78. Flores R, Pires O, Alves J, Pereira VH. An echocardiographic insight into post-COVID-19 symptoms. Cureus. (2023) 15(4):e38039. doi: 10.7759/cureus.38039
79. Raveendran AV, Jayadevan R, Sashidharan S. Long COVID: an overview. Diabetes Metab Syndr. (2021) 15(3):869–75. doi: 10.1016/j.dsx.2021.04.007
80. Venkatesan P. NICE guideline on long COVID. Lancet Respir Med. (2021) 9(2):129. doi: 10.1016/S2213-2600(21)00031-X
81. Zhang JJ, Kang J, Song XL, Yang S, Yang Y, Qiao J, et al. Effects of hysteroscopic septum incision versus expectant management on IVF outcomes in women with complete septate uterus: a retrospective study. BMC Womens Health. (2024) 24(1):54. doi: 10.1186/s12905-024-03022-1
82. Karlsen S, Dahlslett T, Grenne B, Sjøli B, Smiseth O, Edvardsen T, et al. Global longitudinal strain is a more reproducible measure of left ventricular function than ejection fraction regardless of echocardiographic training. Cardiovasc Ultrasound. (2019) 17(1):18. doi: 10.1186/s12947-019-0168-9
83. Jeong EM, Dudley SC Jr. Diastolic dysfunction. Circ J. (2015) 79(3):470–7. doi: 10.1253/circj.CJ-15-0064
84. Jakicic JM, Rogers RJ, Lang W, Gibbs BB, Yuan N, Fridman Y, et al. Impact of weight loss with diet or diet plus physical activity on cardiac magnetic resonance imaging and cardiovascular disease risk factors: heart health study randomized trial. Obesity (Silver Spring). (2022) 30(5):1039–56. doi: 10.1002/oby.23412
85. Jiang Y, Zhao T, Zhou X, Xiang Y, Gutierrez-Castrellon P, Ma X. Inflammatory pathways in COVID-19: mechanism and therapeutic interventions. MedComm (2020). (2022) 3(3):e154. doi: 10.1002/mco2.154
86. Gyöngyösi M, Alcaide P, Asselbergs FW, Brundel B, Camici GG, Martins PDC, et al. Long COVID and the cardiovascular system—elucidating causes and cellular mechanisms in order to develop targeted diagnostic and therapeutic strategies: a joint scientific statement of the ESC working groups on cellular biology of the heart and myocardial and pericardial diseases. Cardiovasc Res. (2023) 119(2):336–56. doi: 10.1093/cvr/cvac115
87. Lassen MCH, Skaarup KG, Lind JN, Alhakak AS, Sengeløv M, Nielsen AB, et al. Recovery of cardiac function following COVID-19—eCHOVID-19: a prospective longitudinal cohort study. Eur J Heart Fail. (2021) 23(11):1903–12. doi: 10.1002/ejhf.2347
88. Karagodin I, Singulane CC, Descamps T, Woodward GM, Xie M, Tucay ES, et al. Ventricular changes in patients with acute COVID-19 infection: follow-up of the world alliance societies of echocardiography (WASE-COVID) study. J Am Soc Echocardiogr. (2022) 35(3):295–304. doi: 10.1016/j.echo.2021.10.015
89. Iovănescu ML, Florescu DR, Marcu AS, Donoiu I, Militaru S, Florescu C, et al. The dysfunctional right ventricle in dilated cardiomyopathies: looking from the right point of view. J Cardiovasc Dev Dis. (2022) 9(10):359. doi: 10.3390/jcdd9100359
90. Terzic CM, Medina-Inojosa BJ. Cardiovascular complications of coronavirus disease-2019. Phys Med Rehabil Clin N Am. (2023) 34(3):551–61. doi: 10.1016/j.pmr.2023.03.003
91. Pischke SE, Hestenes S, Johansen HT, Fure H, Bugge JF, Espinoza A, et al. Sepsis causes right ventricular myocardial inflammation independent of pulmonary hypertension in a porcine sepsis model. PLoS One. (2019) 14(6):e0218624. doi: 10.1371/journal.pone.0218624
92. Bursi F, Santangelo G, Sansalone D, Valli F, Vella AM, Toriello F, et al. Prognostic utility of quantitative offline 2D-echocardiography in hospitalized patients with COVID-19 disease. Echocardiography. (2020) 37(12):2029–39. doi: 10.1111/echo.14869
93. Li Y, Li H, Zhu S, Xie Y, Wang B, He L, et al. Prognostic value of right ventricular longitudinal strain in patients with COVID-19. JACC Cardiovasc Imaging. (2020) 13(11):2287–99. doi: 10.1016/j.jcmg.2020.04.014
94. Nuzzi V, Castrichini M, Collini V, Roman-Pognuz E, Di Bella S, Luzzati R, et al. Impaired right ventricular longitudinal strain without pulmonary hypertension in patients who have recovered from COVID-19. Circ Cardiovasc Imaging. (2021) 14(4):e012166. doi: 10.1161/CIRCIMAGING.120.012166
95. Pelà G, Goldoni M, Cavalli C, Perrino F, Tagliaferri S, Frizzelli A, et al. Long-term cardiac sequelae in patients referred into a diagnostic post-COVID-19 pathway: the different impacts on the right and left ventricles. Diagnostics (Basel. (2021) 11(11):2059. doi: 10.3390/diagnostics11112059
96. Arias Labrador E, Vilaró Casamitjana J, Blanco Díaz S, Ariza Turiel G, Paz Bermejo MA, Brugada Terradellas R. Effects of home-based strength training during COVID-19 lockdown in acute coronary syndrome. Rehabilitacion (Madr). (2022) 56(1):11–9. doi: 10.1016/j.rh.2021.04.002
97. Elhiny R, Al-Jumaili AA, Yawuz MJ. What might COVID-19 patients experience after recovery? A comprehensive review. Int J Pharm Pract. (2022) 30(5):404–13. doi: 10.1093/ijpp/riac026
98. Shafiabadi Hassani N, Talakoob H, Karim H, MozafariBazargany M, Rastad H. Cardiac magnetic resonance imaging findings in 2954 COVID-19 adult survivors: a comprehensive systematic review. J Magn Reson Imaging. (2022) 55(3):866–80. doi: 10.1002/jmri.27852
99. Ramadan MS, Bertolino L, Zampino R, Durante-Mangoni E. Cardiac sequelae after coronavirus disease 2019 recovery: a systematic review. Clin Microbiol Infect. (2021) 27(9):1250–61. doi: 10.1016/j.cmi.2021.06.015
100. Rahmati M, Koyanagi A, Banitalebi E, Yon DK, Lee SW, Il Shin J, et al. The effect of SARS-CoV-2 infection on cardiac function in post-COVID-19 survivors: A systematic review and meta-analysis. J Med Virol. (2023) 95(1):e28325. doi: 10.1002/jmv.28325
Keywords: COVID-19, long-term effects, myocardial function, GRADE approach, systematic review, meta-analysis
Citation: Dehghan M, Mirzohreh ST, Kaviani R, Yousefi S and Pourmehran Y (2024) A deeper look at long-term effects of COVID-19 on myocardial function in survivors with no prior heart diseases: a GRADE approach systematic review and meta-analysis. Front. Cardiovasc. Med. 11:1458389. doi: 10.3389/fcvm.2024.1458389
Received: 2 July 2024; Accepted: 31 October 2024;
Published: 19 November 2024.
Edited by:
Sebastian Kelle, German Heart Center Berlin, GermanyReviewed by:
Constantin Jahnke, German Heart Center Berlin, GermanyMichelangelo Luciani, Sapienza University of Rome, Italy
Copyright: © 2024 Dehghan, Mirzohreh, Kaviani, Yousefi and Pourmehran. This is an open-access article distributed under the terms of the Creative Commons Attribution License (CC BY). The use, distribution or reproduction in other forums is permitted, provided the original author(s) and the copyright owner(s) are credited and that the original publication in this journal is cited, in accordance with accepted academic practice. No use, distribution or reproduction is permitted which does not comply with these terms.
*Correspondence: Shiva Yousefi, ZHIuc2gueW91c2VmaUBnbWFpbC5jb20=
†These authors have contributed equally to this work and share first authorship
‡These authors have contributed equally to this work and share senior authorship