- 1Division of Cardiology, Department of Internal Medicine, Seoul St. Mary’s Hospital, The Catholic University of Korea, Seoul, Republic of Korea
- 2Division of Cardiology, Department of Internal Medicine, Incheon St. Mary’s Hospital, The Catholic University of Korea, Incheon, Republic of Korea
- 3Catholic Research Institute for Intractable Cardiovascular Disease, College of Medicine, The Catholic University of Korea, Seoul, Republic of Korea
- 4Division of Cardiology, Department of Internal Medicine, Uijeongbu St. Mary’s Hospital, The Catholic University of Korea, Uijeongbu, Republic of Korea
- 5Health Promotion Center, Seoul St. Mary’s Hospital, The Catholic University of Korea, Seoul, Republic of Korea
- 6Division of Cardiology, Yonsei Cardiovascular Center, Yonsei University Health System, Seoul, Republic of Korea
Background: Despite the essential role of ectopic osteogenic calcium-phosphate metabolism in the development of calcific aortic valve disease (CAVD), the implications of high serum phosphate levels in CAVD development are not fully understood.
Methods: Asymptomatic individuals who underwent health screening using serial cardiac computed tomography (CT) and echocardiography were selected from a multicenter registry. CAVD was identified and quantified on CT images using the aortic valve calcification (AVC) score. The associations between initial serum phosphate levels and the presence of baseline CAVD, development of new CAVD, and the AVC score progression rate were investigated using multivariable regression models.
Results: A total of 736 individuals were selected for analysis, and the median interscan duration was 36.4 months. On initial CT, 83 (13.7%) participants had baseline CAVD, while 52 (7.0%) individuals developed new CAVD during follow-up. Serum phosphate levels were not associated with a higher probability of baseline CAVD but were predictive of newly developed CAVD (odds ratio per 1 mg/dl, 1.05; 95% confidence interval, 1.01–1.10; p = 0.02). Higher phosphate levels were also associated with a faster AVC score progression in those with baseline CAVD (regression coefficient per 1 mg/dl, 15.55 Agatston units/year; 95% confidence interval, 6.02–25.07; p < 0.01), an association which remained significant when the analysis was extended to include newly developed CAVD.
Conclusion: Even slight elevations in serum phosphate are associated with accelerated CAVD progression from an early stage. Further studies are needed to investigate whether the regulation of phosphate metabolism can slow the progression of CAVD to aortic stenosis.
1 Introduction
Calcific aortic valve disease (CAVD) is characterized by fibrotic thickening and calcification of the aortic valve (1). CAVD can progress to the point where the limitation of aortic valve opening becomes hemodynamically significant, which is referred to as aortic stenosis (AS)—a major cause of cardiovascular morbidity worldwide (2, 3). The detection of aortic valve calcification (AVC) on computed tomography (CT) is a sensitive and accurate method to quantify the calcific burden of CAVD and monitor disease progression (4, 5).
In the pathogenesis of CAVD, multiple pathways related to atherosclerosis and ectopic osteogenic calcium-phosphate metabolism are involved (6, 7). Although atherosclerotic risk factors are responsible for the initiation of CAVD, they are not associated with its progression (8, 9), and lipid lowering using statins did not succeed in preventing significant AS (10). Therefore, pathways related to osteogenesis and calcium-phosphate metabolism may be the key to developing pharmacologic interventions for CAVD (6, 11, 12). However, although elevated serum phosphate has been associated with the presence of CAVD, the implications of serum phosphate levels in CAVD progression are not fully understood (13, 14). Furthermore, the role of calcium-phosphate metabolism in CAVD may be of particular interest in the East Asian population, which has a lower burden of atherosclerosis compared with other ethnicities (3, 15, 16). Therefore, we sought to identify, among other factors, the relationship of serum phosphate levels with the progression of CAVD in ethnic Korean participants who were examined with cardiac CT scans as part of a general health examination.
2 Methods
2.1 Study design and population
The data used in this study were collected as part of the KOrea Initiatives on Coronary Artery calcification (KOICA) registry, which is a multicenter registry of individuals examined using cardiac CT scans during self-referred health examinations in six high-volume healthcare centers affiliated with tertiary hospitals in Korea. A total of 93,914 individuals were registered, and further details can be found in previous reports (16, 17). For this study, we selected the participants who were examined at Seoul St. Mary's Hospital, where same-day echocardiography was also part of the health examination. We limited our analysis to those with at least two CT scans during the study period (April 2009–July 2016) to identify factors associated with the progression of CAVD. Participants with bicuspid aortic valves were excluded, as they were thought to represent a heterogeneous population. Demographic factors, medical history, and current symptoms were self-reported using a detailed questionnaire. Medical records were retrospectively reviewed to ensure that the participants were free of cardiovascular symptoms at the initial or repeat CT scans which may have prompted the examination. Laboratory samples were drawn after a 12-hour fasting period and measured using an automatic analyzer (7,600–210; Hitachi Medical Corp., Tokyo, Japan). Lipid profiles were measured using a direct enzymatic method. The estimated glomerular filtration rate (eGFR) was calculated using the Chronic Kidney Disease Epidemiology Collaboration equation. The institutional review board of Seoul St. Mary's Hospital approved the study protocol (IRB KC23RISI0357) and waived the need for written informed consent because of the study's retrospective nature. The study protocol conforms to the ethical guidelines of the 1975 Declaration of Helsinki.
2.2 Data acquisition
Cardiac CT images were acquired using a 64-slice, dual-source CT scanner (SOMATOM Definition; Siemens, Forchheim, Germany). A non-contrast scan was first obtained using prospective triggering at 70% of the RR interval. The parameters used were tube voltage 120 kVp, gantry rotation time of 330 ms, and maximum tube current of 400 mA·s. Next, enhanced CT angiography scans were obtained using a retrospective electrocardiogram-gated protocol after the administration of 80–110 ml of iodinated contrast. CT images were transferred and reconstructed immediately after scanning using a computerized workstation (Advantage Windows Workstation 4.3; GE Healthcare, Milwaukee, WI, USA) with a slice thickness of 3 mm. Further details regarding the protocol for cardiac CT at our institution can be found in previous reports (18, 19).
CAVD was primarily assessed on CT images using a commercially available CT processing program (3mensio Structural Heart 10.0; Pie Medical Imaging, Maastricht, the Netherlands). As recommended in those undergoing evaluation for AS, the severity of CAVD was quantified on non-contrast axial images using the AVC score according to the Agatston method (4). Each calcified lesion from the base to the tip of the aortic valve leaflets was carefully selected, excluding calcifications found in the coronary arteries, aortic root, and left ventricular outflow tract. If necessary, the corresponding enhanced CT images were additionally referred to for the verification of anatomic structures. The AVC score was defined as the sum of the values for each calcified lesion and was assessed by two cardiologists (K.A.K. and S.-Y.L.) with 3 years of experience in cardiac imaging. A third cardiologist (H.-O.J.) with more than 20 years of cardiac imaging experience was consulted in cases of uncertainty.
The hemodynamic effects of CAVD were assessed using transthoracic echocardiography, which was performed by trained sonographers following standard guidelines (20). Images were obtained using commercially available ultrasound machines with 2.5- to 3.5-MHz transducers (GE Vivid E7 and Vivid E9, GE Healthcare, Chicago, IL, USA; Philips iE33, Amsterdam, the Netherlands). Because the majority of participants did not have findings suggestive of AS, continuous-wave Doppler measurements across the aortic valve were not routinely performed in these individuals.
2.3 Statistical analyses
The participants were classified into three groups according to the presence of CAVD on initial and subsequent CT scans: (1) no CAVD, defined as no AVC on any scan; (2) newly developed CAVD, defined as no AVC on the initial CT but with AVC score >0 on subsequent CT; (3) baseline CAVD. In the baseline characteristics, categorical data are presented as numbers and frequencies and compared using the χ2 test, and continuous variables are expressed as mean ± standard deviation and compared using one-way analysis of variance.
The relationship between serum phosphate and CAVD development was analyzed in a number of ways. First, the association between phosphate levels and both baseline and newly developed CAVD was analyzed using multivariable logistic regression models. Restricted cubic splines were also constructed to visualize the relationship between serum phosphate levels and other significant predictors with the probability of newly developed CAVD. Second, the association between phosphate levels and CAVD progression was analyzed using multivariable linear regression on the annualized AVC score progression rate in those with baseline CAVD. Finally, the association between CAVD progression and serum phosphate levels was analyzed in the entire group including both baseline CAVD and newly developed CAVD using a multivariable linear mixed-effects model with individual intercepts and the repeated effect estimated for time. Subgroup analysis was also performed using the multivariable linear mixed-effects model to confirm the effect of phosphate levels on CAVD progression in different populations. The relationship between serum phosphate levels and echocardiographic parameters was additionally explored using multivariable linear and logistic regression models.
Statistical analyses were performed using R version 4.3.2 (R Foundation for Statistical Computing, Vienna, Austria), and a two-sided p value <0.05 was considered statistically significant. Further details of the statistical methods used in this study can be found in the Supplementary Material.
3 Results
3.1 Baseline characteristics
From the KOICA registry, we selected 6,641 individuals who underwent examination at Seoul St. Mary's Hospital. After excluding two individuals with missing data and two with bicuspid aortic valves, 736 participants with at least two CT scans during the study period (April 2009–July 2016) were included in the final study (Figure 1). The median duration between the initial and final CT was 36.4 [interquartile range (IQR), 23.5–50.3] months, and a total of 1,695 CT scans were considered for analysis. Baseline CAVD was present in 83 individuals (11.2%) on the initial CT scan, and new-onset CAVD developed in 52 participants (7.1%) on subsequent scans. In participants with baseline CAVD, the median AVC score was 30.4 (IQR, 10.6–57.2) Agatston units (AU) on the initial CT (Figure 2A) and increased to 49.4 (IQR, 24.9–92.1) AU on the final CT, with an annualized progression rate of 6.0 (IQR, 2.1–16.8) AU/year (Figure 2B).
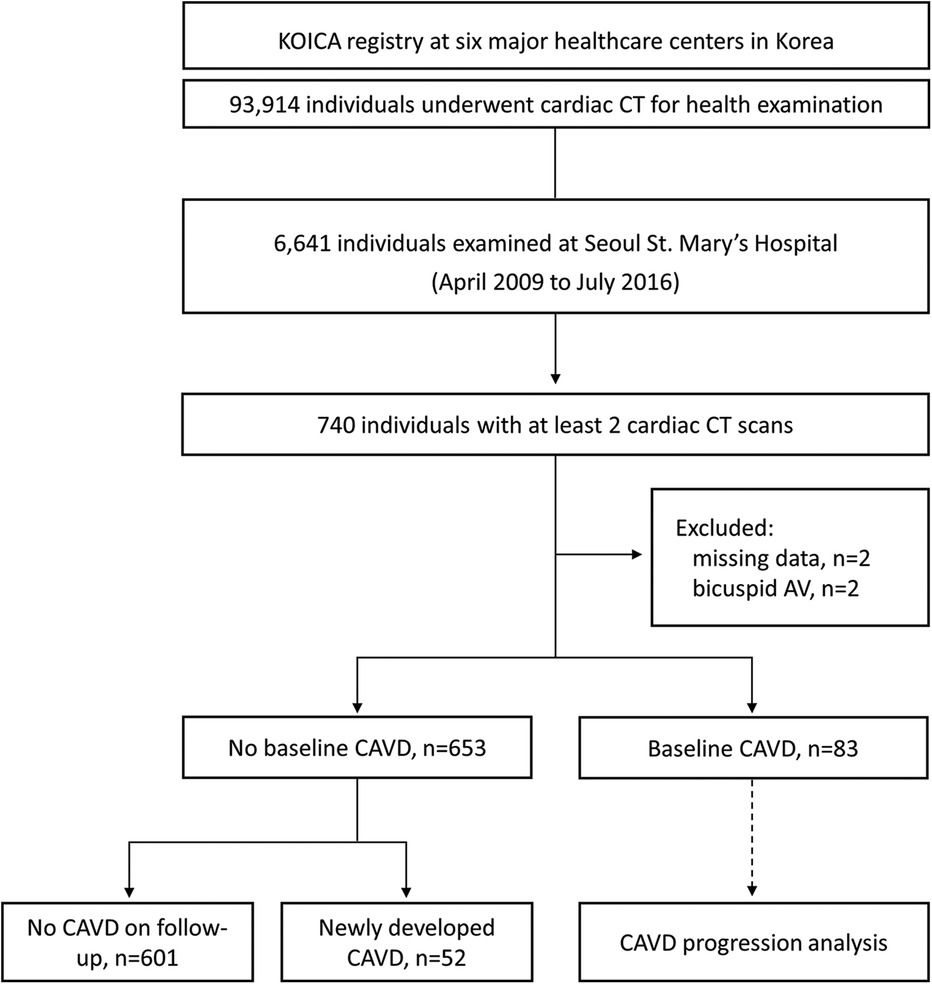
Figure 1. Selection process of the study population. KOICA, Korea initiatives on coronary artery calcification; CT, computed tomography; AV, aortic valve; CAVD, calcified aortic valve disease.
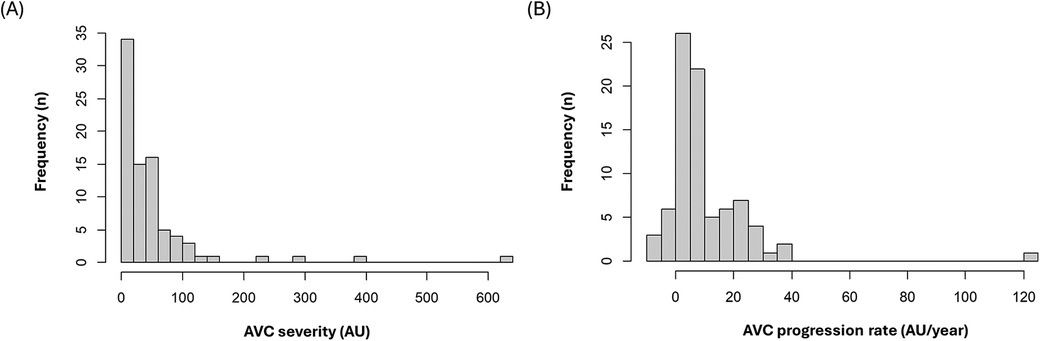
Figure 2. Distribution of (A) baseline aortic valve calcification scores (B) annualized progression rate of aortic valve calcification scores. AVC, aortic valve calcification; AU, Agatston units.
The baseline characteristics of the study population are presented in Table 1. The median age of the participants was 57 years, and 80.6% were male, with a low proportion of comorbidities overall. Compared with individuals without CAVD, those with baseline or newly developed CAVD were older, were more likely to have hypertension and higher systolic blood pressure (SBP), and had lower eGFR and higher Framingham Risk Scores (21). On echocardiography, the participants with baseline or newly developed CAVD had a higher proportion of aortic regurgitation, higher left ventricular outflow tract velocities, higher left ventricular mass index (LVMI), and worse diastolic function profiles. Two individuals in the baseline AVC group had mild AS.
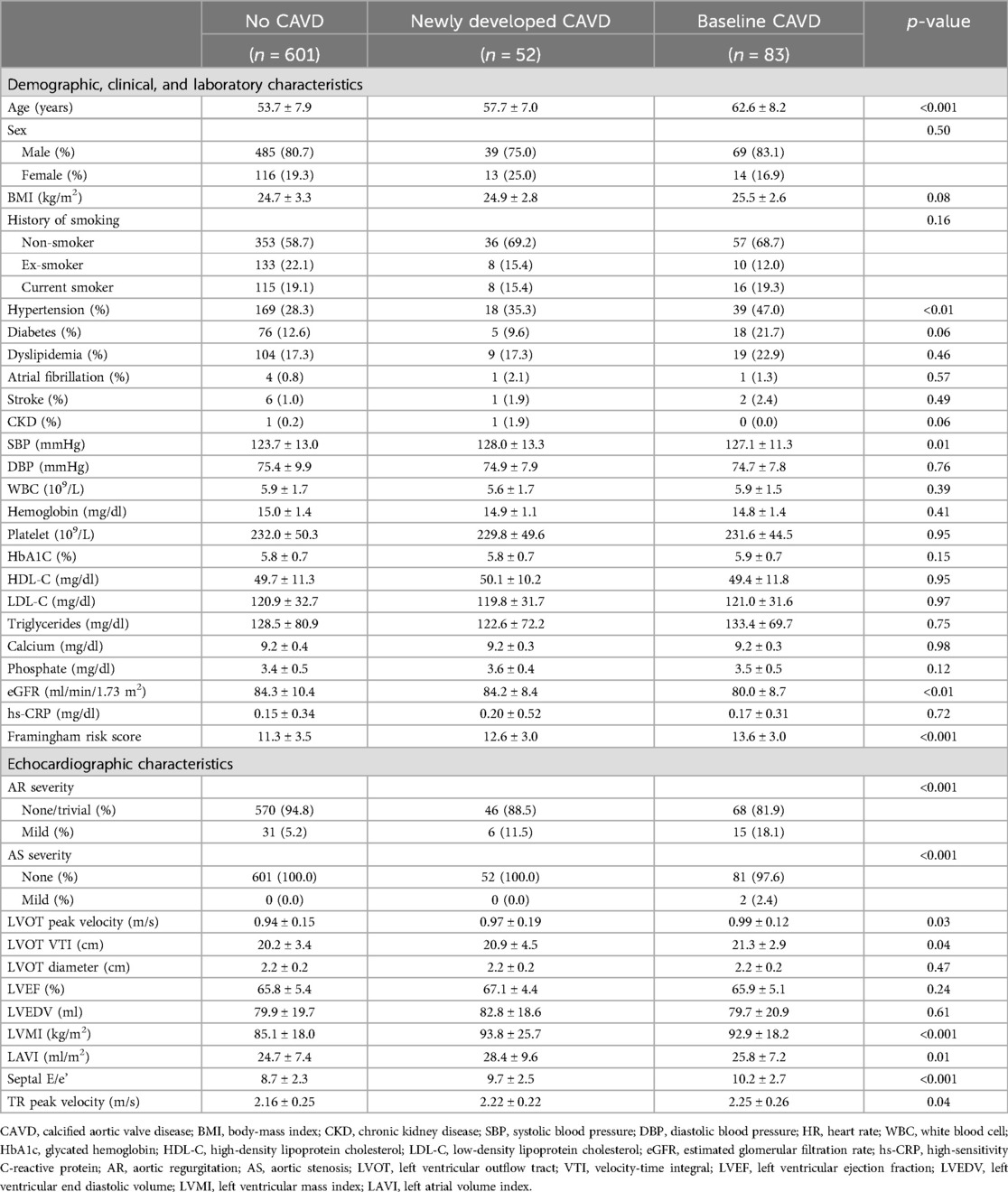
Table 1. Baseline characteristics of the study population stratified according to the presence of calcified aortic valve disease at baseline and follow-up computed tomography.
3.2 Association of serum phosphate with the probability of calcified aortic valve disease
On univariable logistic regression, there was no clear association between serum phosphate and baseline CAVD, but higher serum phosphate levels were associated with a significantly higher probability of newly developed CAVD (odds ratio [OR] per 1 mg/dl increase, 1.06; 95% confidence interval [CI], 1.01–1.10; p = 0.02) (Table 2). This association remained after adjustment for age, sex, hypertension, diabetes, dyslipidemia, and eGFR (model 1a: OR, 1.06; 95% CI, 1.02–1.11; p = 0.01); for age, sex, SBP, glycated hemoglobin, low-density lipoprotein cholesterol (LDL-C), and eGFR (model 1b: OR, 1.05; 95% CI, 1.01–1.10; p = 0.02); or for the Framingham risk score (21), glycated hemoglobin, and eGFR (model 2: OR, 1.05; 95% CI, 1.01–1.10; p = 0.02).
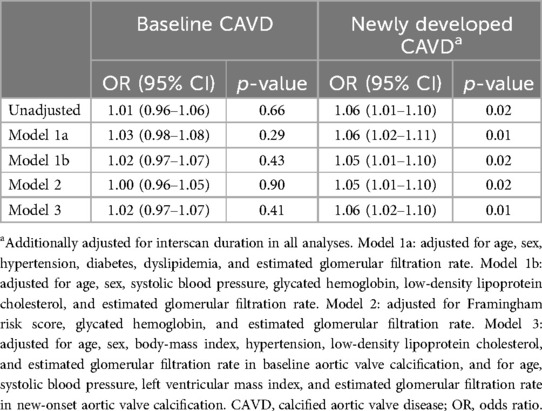
Table 2. Association of serum phosphate with the probability of baseline and newly developed calcified aortic valve disease.
Predictors of baseline and newly developed CAVD other than the traditional atherosclerosis risk factors were identified and adjusted for using a stepwise model (model 3 in Tables 2, 3). Older age (OR per 10 years, 1.15; 95% CI, 1.12–1.19; p < 0.001), higher body mass index (BMI) (OR per 5 kg/m2, 1.05; 95% CI, 1.02–1.10; p < 0.01), history of hypertension (OR, 1.05; 95% CI, 1.00–1.11; p = 0.04), and higher LDL-C levels (OR per 10 mg/dl, 1.01; 95% CI, 1.00–1.02; p = 0.02) were associated with baseline CAVD; however, serum phosphate levels were not (OR per 1 mg/dl, 1.02; 95% CI, 0.93–1.07; p = 0.41). In contrast, higher serum phosphate (OR per 1 mg/dl, 1.06; 95% CI, 1.02–1.10; p = 0.01) was a significant predictor for the development of new CAVD, as were older age (OR per 10 years, 1.05; 95% CI, 1.02–1.08; p < 0.01), higher SBP (OR per 10 mmHg, 1.04; 95% CI, 1.01–1.07; p < 0.01), and higher LVMI (OR per 10 g/m2, 1.02; 95% CI, 1.00–1.03; p = 0.03). Analysis using restricted cubic splines also showed a positive correlation between age, SBP, serum phosphate, and LVMI with the probability of newly developed CAVD (Figure 3). The increase in the probability of newly developed CAVD with increasing serum phosphate was most prominent between 3.0–4.0 mg/dl and plateaued past this range. The optimal cutoff points for predicting newly developed CAVD were age >51 years, SBP >128 mmHg, serum phosphate >3.6 mg/dl, and LVMI >99 g/m2 (Supplementary Material Figure S1), and the C-index for the regression models was 0.79 for baseline CAVD and 0.81 for newly developed CAVD (Supplementary Material Figure S2).
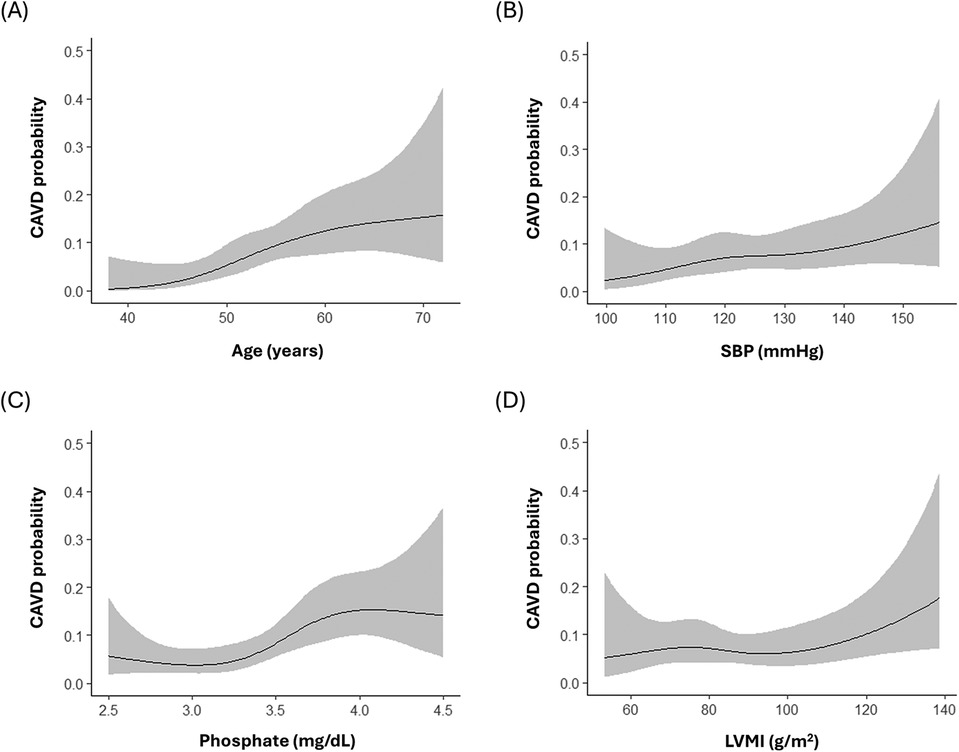
Figure 3. Relationship between the probability of newly developed calcified aortic valve disease and (A) age (B) systolic blood pressure (C) serum phosphate (D) left ventricular mass index. SBP, systolic blood pressure; LVMI, left ventricular mass index.
3.3 Association of serum phosphate with the progression rate of calcified aortic valve disease
In individuals with baseline CAVD, the initial AVC score, serum phosphate, left ventricular end-diastolic volume, and LVMI were associated with a higher annualized AVC score progression rate on univariable linear regression (Table 4). After multivariable adjustment, higher serum phosphate levels (regression coefficient per 1 mg/dl, 15.80; 95% CI, 5.65–25.96; p < 0.01), as well as the initial AVC score (regression coefficient per 1 AU, 0.29; 95% CI, 0.26–0.32; p < 0.001), male sex (regression coefficient, 15.95; 95% CI, 4.22–27.67, p = 0.01), and LVMI (regression coefficient per 10 g/m2, 2.31; 95% CI, 0.16–4.45; p = 0.04) emerged as factors significantly associated with a higher annualized AVC score progression rate.
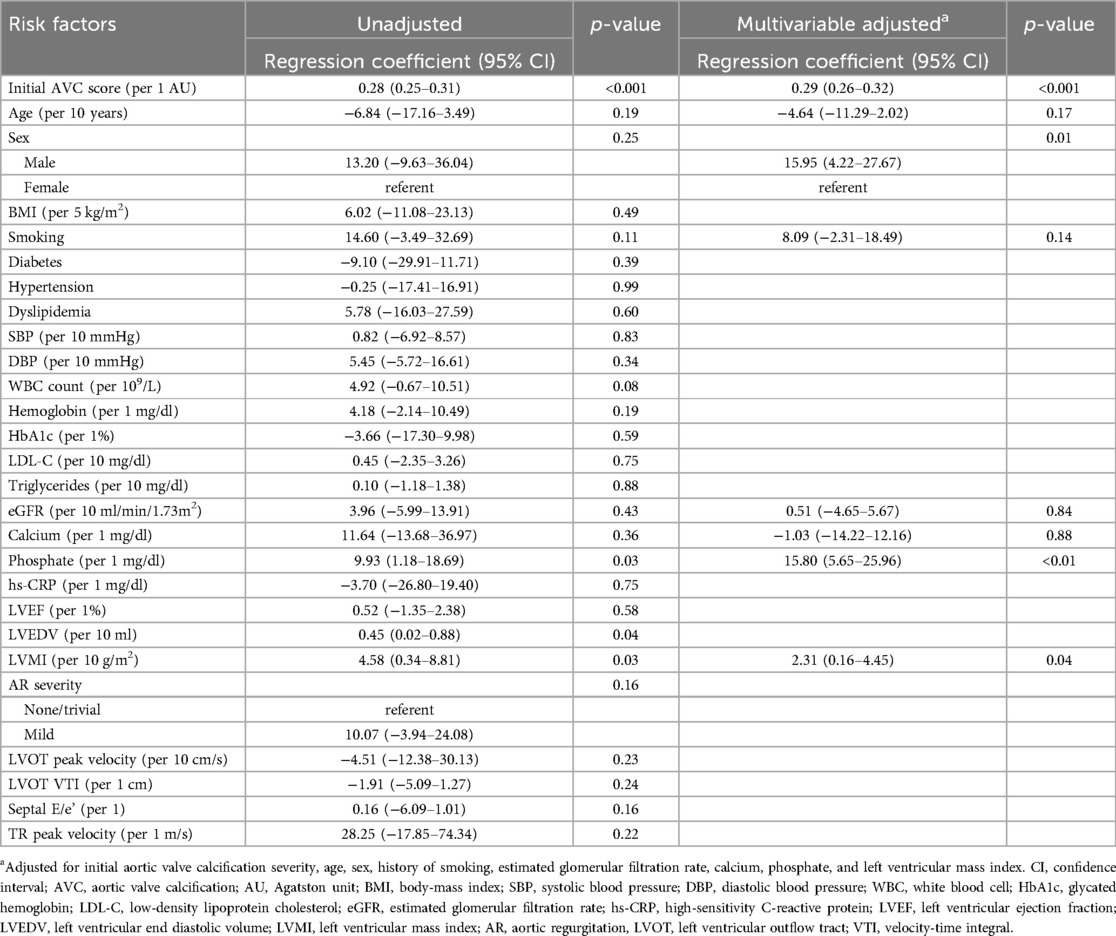
Table 4. Linear regression for the annualized progression rate of the aortic valve calcification score in the individuals with baseline calcified aortic valve disease.
When the association between CAVD progression and serum phosphate levels was analyzed in the entire group including both baseline and newly developed CAVD using a linear mixed-effects model, higher serum phosphate was again found to be independently associated with a higher annualized AVC score progression rate (regression coefficient per 1 mg/dl, 1.03; 95% CI, 0.25–1.83; p = 0.01) (Table 5). The only other risk factor associated with CAVD progression in this analysis was the initial AVC score (regression coefficient per 1 AU, 0.26; 95% CI, 0.25–0.27; p < 0.001). In subgroup analysis, higher phosphate was associated with more rapid progression in both baseline and newly developed CAVD, with a larger effect in those with baseline CAVD (regression coefficient per 1 mg/dl, 10.46; 95% CI, 0.16–22.13; p = 0.05) compared with those with newly developed CAVD (regression coefficient per 1 mg/dl, 0.54; 95% CI, 0.21–0.83; p < 0.01) (Figure 4). However, the effect of phosphate on CAVD progression was consistent across other subgroups. Finally, as a sensitivity analysis, multivariable linear mixed-effects regression was repeated using log-transformed AVC scores, and serum phosphate remained significantly associated with a higher rate of CAVD progression (p < 0.001) (Supplementary Table S1).
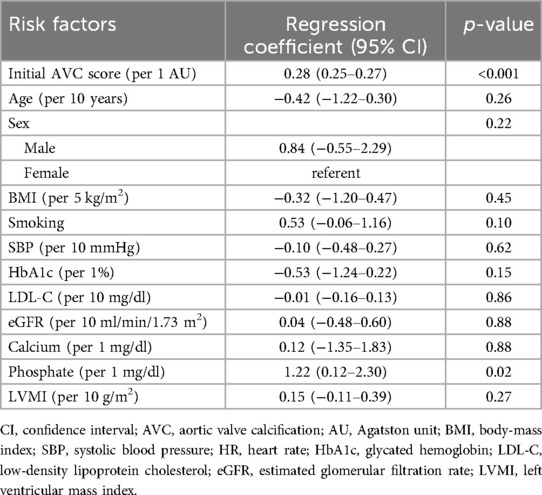
Table 5. Multivariable linear mixed-effects model for identification of risk factors with the annualized progression rate of the aortic valve calcification score in the entire population.
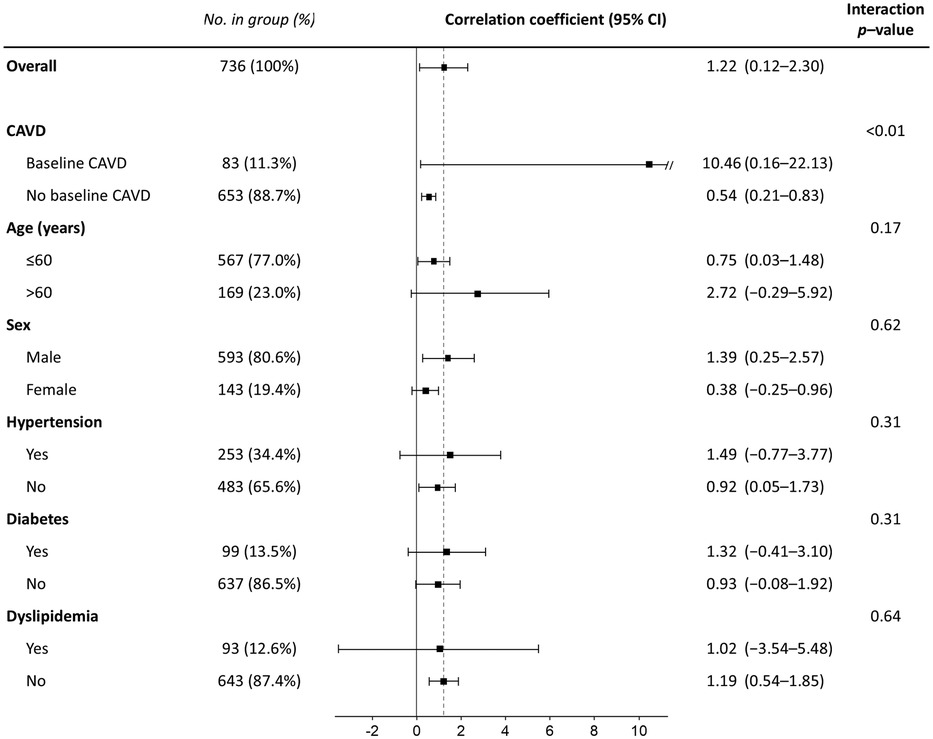
Figure 4. Subgroup analysis for the effect of serum phosphate on the annualized progression rate of the aortic valve calcification score. CI, confidence interval; CAVD, calcified aortic valve disease.
3.4 Association of serum phosphate levels with echocardiographic parameters
The association between serum phosphate levels and hemodynamic parameters measured using echocardiography are shown in Supplementary Table S2. Depending on the regression model used for adjustment, elevated phosphate had a borderline association with higher E/e’. However, serum phosphate levels did not show a clear relationship with measurements of left ventricular size and function, left ventricular outflow tract velocities, or the probability of aortic regurgitation.
4 Discussion
In this retrospective cohort study, we assessed CAVD development using cardiac CT and echocardiography in asymptomatic individuals undergoing self-referred health examination. Higher serum phosphate was associated with the development of new CAVD and a higher annualized AVC score progression rate. We also found differences in factors related to baseline CAVD and the AVC progression rate; the former was most strongly associated with age, BMI, history of hypertension, and LDL-C levels, whereas the latter was associated with the initial AVC score, male sex, serum phosphate levels, and LVMI. Meanwhile, the predictors of new-onset CAVD were intermediate between the two—age, SBP, phosphate, and LVMI.
Previous large cohort studies found that traditional atherosclerotic risk factors, such as older age, higher BMI, smoking, hypertension, and dyslipidemia, are associated with the development of CAVD (8, 22). However, despite the notable overlap, atherosclerosis alone does not fully explain the pathophysiologic process of CAVD. In the Multi-Ethnic Study of Atherosclerosis (MESA), the prime predictor of CAVD progression was the baseline AVC score per se, supporting the concept of a self-perpetuating cycle in which calcium begets calcium (8, 9). Our findings are in accord with previous studies in identifying the association of atherosclerotic risk factors with baseline CAVD, and in identifying the baseline AVC score as the most important factor in CAVD progression. The incidence rate of newly developed CAVD (2.3%/year) in the present study was comparable to that reported in MESA (1.7%/year) (8). The identification of male sex and LVMI as a factor in accelerated AVC score progression is also in accord with previous reports (8, 23–25). In addition, we found that higher serum phosphate levels within the normal range were associated with the development of new CAVD and a higher annualized AVC score progression rate, which to the best of our knowledge has not been reported previously.
Since calcification depends on the deposition of calcium-phosphate crystals in vivo, phosphate plays an essential role in both physiologic and ectopic vascular calcification (26). The role of elevated phosphate in promoting cardiovascular calcification and as a risk factor for cardiovascular events is well established in patients with chronic kidney disease (27). In addition, a number of previous studies have investigated the relationship between phosphate and CAVD in patients without renal dysfunction. In the Cardiovascular Health Study, higher phosphate levels within the normal range were associated with the presence of CAVD detected on echocardiography (13). Analysis of the Multi-Ethnic Study of Atherosclerosis (MESA) cohort also found that higher serum phosphate was associated with prevalent AVC on CT (14). Although serum phosphate levels are tightly controlled in a relatively narrow range, they are also subject to variation, notably with age (28), and thus the association between the onset of AVC and phosphate may be weakened by time. We speculate that the smaller number of individuals in our study may have resulted in the causative association between AVC and phosphate being lost at the time of baseline examination, while it may have been retained in the MESA study. In the same cohort however, phosphate levels were not associated with the progression of AVC severity (29), and similar results were found in other recent studies (30, 31). Our results are consistent with previous reports in identifying higher phosphate levels as a risk factor in CAVD development, but is also in contrast with these studies in the association of phosphate with accelerated CAVD progression.
One explanation for this apparent discrepancy may be found in the lower baseline AVC scores in our study population (median AVC score 30.4 [IQR 10.6–57.2] vs. 56 [IQR 19–137] in the MESA cohort (8). In the pathogenesis of CAVD, after initial endothelial injury and lipid infiltration, differentiation of aortic valvular interstitial cells into a osteoblast-like phenotype next takes place, a process which elevated phosphate is known to promote (7, 26, 32–36). In contrast, signaling via transforming growth factor β1 and the Wnt/β-catenin pathway in response to mechanical stress is known to be the dominant factor driving calcification in the later stages after osteogenic differentiation has occurred (6, 37–39), which is supported by the correlation between hemodynamic severity and disease progression found in established AS (40–42). There is very limited data on the AVC scores for the prediction of mild AS; however, the presence of mild calcific AS has been found even at AVC scores as low as 100 (43, 44). Thus we may hypothesize that a non-negligible proportion of the MESA cohort may have had more advanced CAVD and elevated transaortic valve pressures, which could have weakened the influence of phosphate on disease progression. However, no significant relationship between CAVD progression and hemodynamic parameters measured on echocardiography was found in our analysis. Thus, we suggest that our population may be representative of CAVD in the earlier stages of development where osteoblast differentiation is the main pathophysiologic mechanism, and which may possibly be affected by serum phosphate levels. Alternatively, there is a possibility that our results are specific to the East Asian population. Previous studies have found that there are ethnic differences in bone-mineral metabolism (45, 46), which may be an explanation for the different results found in our analysis.
It is not clear whether the association between phosphate levels and CAVD progression found in our study is directly causative in nature, or whether it reflects another underlying process in mineral metabolism. However, serum phosphate is widely measured in clinical practice, and elevated phosphate levels in patients with CAVD may indicate a higher risk of accelerated CAVD progression, which may potentially benefit from therapeutic interventions targeting various pathways in mineral metabolism (12). Further studies are needed to investigate whether the regulation of phosphate metabolism can slow the progression of early CAVD to AS.
Despite the findings of our study, it had several limitations. First, as previously mentioned, the severity of CAVD in our study population was generally low, and due to the small number of participants with higher AVC scores, we could not perform subgroup analysis to investigate the effect of phosphate levels according to the severity of CAVD or determine cut-off points. Second, although various hemodynamic parameters were included in our analysis, the effect of peak transaortic velocities on CAVD could not be investigated because our study population did not include significant AS and only a small proportion of the population had continuous-wave Doppler transaortic velocity measurements. However, we analyzed the effect of left ventricular outflow tract velocities and diastolic profiles and found no association between these parameters and CAVD progression. Although these factors have been linked with accelerated AS progression in previous studies (41, 42), our negative results suggest that hemodynamic effects may not be significant at least in the early stages of CAVD. Third, we only adjusted for the effect of laboratory and echocardiographic variables measured at baseline, which may have changed during the follow-up period. Lastly, owing to the retrospective nature of the study, there was heterogeneity in patient characteristics and duration to follow-up examination. Although we used multivariable regression to adjust for confounders, we cannot exclude the possibility of remaining bias.
5 Conclusion
In a cohort of asymptomatic individuals undergoing health examination, atherosclerotic risk factors were strongly associated with baseline CAVD. Upper normal phosphate levels were associated with the development of new CAVD and the accelerated progression of existing CAVD. The effect of phosphate on CAVD progression may be due to the lower baseline AVC scores in our study population, as the dominant pathophysiologic mechanism driving calcification may be different across the stages of CAVD development. Further studies are needed to confirm these findings, and to investigate whether therapeutic interventions targeting phosphate metabolism can slow the progression of early CAVD to AS.
Data availability statement
The datasets presented in this article are not readily available due to the conditions of the IRB approval, as the dataset contains personal data. Requests to access the datasets should be directed to the corresponding author.
Ethics statement
The studies involving humans were approved by Seoul St. Mary’s Hospital Clinical Research Coordinating Center. The studies were conducted in accordance with the local legislation and institutional requirements. The ethics committee/institutional review board waived the requirement of written informed consent for participation from the participants or the participants’ legal guardians/next of kin because of the retrospective nature of the study.
Author contributions
KAK: Conceptualization, Data curation, Formal Analysis, Investigation, Methodology, Visualization, Writing – original draft. H-OJ: Conceptualization, Writing – review & editing, Methodology, Project administration, Resources, Supervision. M-JK: Conceptualization, Writing – review & editing. S-YL: Data curation, Investigation, Validation, Visualization, Writing – review & editing. YA: Resources, Validation, Writing – review & editing. M-HJ: Resources, Writing – review & editing. W-BC: Resources, Writing – review & editing. D-HL: Resources, Writing – review & editing. H-JY: Resources, Writing – review & editing. H-JC: Project administration, Resources, Writing – review & editing.
Funding
The author(s) declare that no financial support was received for the research, authorship, and/or publication of this article.
Acknowledgment
The authors thank Jaesub Park (PhD, Wellcome-MRC Cambridge Stem Cell Institute, University of Cambridge) for statistical support.
Conflict of interest
The authors declare that the research was conducted in the absence of any commercial or financial relationships that could be construed as a potential conflict of interest.
Publisher's note
All claims expressed in this article are solely those of the authors and do not necessarily represent those of their affiliated organizations, or those of the publisher, the editors and the reviewers. Any product that may be evaluated in this article, or claim that may be made by its manufacturer, is not guaranteed or endorsed by the publisher.
Supplementary material
The Supplementary Material for this article can be found online at: https://www.frontiersin.org/articles/10.3389/fcvm.2024.1450757/full#supplementary-material
References
1. Moncla LM, Briend M, Bosse Y, Mathieu P. Calcific aortic valve disease: mechanisms, prevention and treatment. Nat Rev Cardiol. (2023) 20:546–59. doi: 10.1038/s41569-023-00845-7
2. Yadgir S, Johnson CO, Aboyans V, Adebayo OM, Adedoyin RA, Afarideh M, et al. Global, regional, and national burden of calcific aortic valve and degenerative mitral valve diseases, 1990–2017. Circulation. (2020) 141:1670–80. doi: 10.1161/CIRCULATIONAHA.119.043391
3. Whelton SP, Jha K, Dardari Z, Razavi AC, Boakye E, Dzaye O, et al. Prevalence of aortic valve calcium and the long-term risk of incident severe aortic stenosis. JACC Cardiovasc Imaging. (2024) 17:31–42. doi: 10.1016/j.jcmg.2023.02.018
4. Pawade T, Sheth T, Guzzetti E, Dweck MR, Clavel MA. Why and how to measure aortic valve calcification in patients with aortic stenosis. JACC Cardiovasc Imaging. (2019) 12:1835–48. doi: 10.1016/j.jcmg.2019.01.045
5. Doris MK, Jenkins W, Robson P, Pawade T, Andrews JP, Bing R, et al. Computed tomography aortic valve calcium scoring for the assessment of aortic stenosis progression. Heart. (2020) 106:1906–13. doi: 10.1136/heartjnl-2020-317125
6. Pawade TA, Newby DE, Dweck MR. Calcification in aortic stenosis: the Skeleton key. J Am Coll Cardiol. (2015) 66:561–77. doi: 10.1016/j.jacc.2015.05.066
7. Rajamannan NM, Subramaniam M, Rickard D, Stock SR, Donovan J, Springett M, et al. Human aortic valve calcification is associated with an osteoblast phenotype. Circulation. (2003) 107:2181–4. doi: 10.1161/01.CIR.0000070591.21548.69
8. Owens DS, Katz R, Takasu J, Kronmal R, Budoff MJ, O'Brien KD. Incidence and progression of aortic valve calcium in the multi-ethnic study of atherosclerosis (MESA). Am J Cardiol. (2010) 105:701–8. doi: 10.1016/j.amjcard.2009.10.071
9. Messika-Zeitoun D, Bielak LF, Peyser PA, Sheedy PF, Turner ST, Nkomo VT, et al. Aortic valve calcification: determinants and progression in the population. Arterioscler Thromb Vasc Biol. (2007) 27:642–8. doi: 10.1161/01.ATV.0000255952.47980.c2
10. Chan KL, Teo K, Dumesnil JG, Ni A, Tam J, Investigators A. Effect of lipid lowering with rosuvastatin on progression of aortic stenosis: results of the aortic stenosis progression observation: measuring effects of rosuvastatin (ASTRONOMER) trial. Circulation. (2010) 121:306–14. doi: 10.1161/CIRCULATIONAHA.109.900027
11. Pawade TA, Doris MK, Bing R, White AC, Forsyth L, Evans E, et al. Effect of denosumab or alendronic acid on the progression of aortic stenosis: a double-blind randomized controlled trial. Circulation. (2021) 143:2418–27. doi: 10.1161/CIRCULATIONAHA.121.053708
12. Marquis-Gravel G, Redfors B, Leon MB, Genereux P. Medical treatment of aortic stenosis. Circulation. (2016) 134:1766–84. doi: 10.1161/CIRCULATIONAHA.116.023997
13. Linefsky JP, O'Brien KD, Katz R, de Boer IH, Barasch E, Jenny NS, et al. Association of serum phosphate levels with aortic valve sclerosis and annular calcification: the cardiovascular health study. J Am Coll Cardiol. (2011) 58:291–7. doi: 10.1016/j.jacc.2010.11.073
14. Linefsky JP, O'Brien KD, Sachs M, Katz R, Eng J, Michos ED, et al. Serum phosphate is associated with aortic valve calcification in the multi-ethnic study of atherosclerosis (MESA). Atherosclerosis. (2014) 233:331–7. doi: 10.1016/j.atherosclerosis.2013.12.051
15. Kanjanauthai S, Nasir K, Katz R, Rivera JJ, Takasu J, Blumenthal RS, et al. Relationships of mitral annular calcification to cardiovascular risk factors: the multi-ethnic study of atherosclerosis (MESA). Atherosclerosis. (2010) 213:558–62. doi: 10.1016/j.atherosclerosis.2010.08.072
16. Lee JH, Hartaigh BO, Han D, Park HE, Choi SY, Sung J, et al. Reassessing the usefulness of coronary artery calcium score among varying racial and ethnic groups by geographic locations: relevance of the Korea initiatives on coronary artery calcification registry. J Cardiovasc Ultrasound. (2015) 23:195–203. doi: 10.4250/jcu.2015.23.4.195
17. Lee W, Yoon YE, Kwon O, Lee H, Park HE, Chun EJ, et al. Evaluation of coronary artery calcium progression in asymptomatic individuals with an initial score of zero. Korean Circ J. (2019) 49:448–57. doi: 10.4070/kcj.2018.0318
18. Choi IJ, Lim S, Choo EH, Kim JJ, Hwang BH, Kim TH, et al. Differential impact of chronic kidney disease on coronary calcification and atherosclerosis in asymptomatic individuals with or without diabetes: analysis from a coronary computed tomographic angiography registry. Cardiorenal Med. (2018) 8:228–36. doi: 10.1159/000489097
19. Lee DH, Youn HJ, Jung HO, Chang K, Choi YS, Jung JI. The cardiovascular risk factors associated with the plaque pattern on coronary computed tomographic angiography in subjects for health check-up. Clin Hypertens. (2017) 23:6. doi: 10.1186/s40885-017-0062-4
20. Mitchell C, Rahko PS, Blauwet LA, Canaday B, Finstuen JA, Foster MC, et al. Guidelines for performing a comprehensive transthoracic echocardiographic examination in adults: recommendations from the American society of echocardiography. J Am Soc Echocardiogr. (2019) 32:1–64. doi: 10.1016/j.echo.2018.06.004
21. D'Agostino RB Sr., Vasan RS, Pencina MJ, Wolf PA, Cobain M, Massaro JM, et al. General cardiovascular risk profile for use in primary care: the framingham heart study. Circulation. (2008) 117:743–53. doi: 10.1161/CIRCULATIONAHA.107.699579
22. Thanassoulis G, Massaro JM, Cury R, Manders E, Benjamin EJ, Vasan RS, et al. Associations of long-term and early adult atherosclerosis risk factors with aortic and mitral valve calcium. J Am Coll Cardiol. (2010) 55:2491–8. doi: 10.1016/j.jacc.2010.03.019
23. Aggarwal SR, Clavel MA, Messika-Zeitoun D, Cueff C, Malouf J, Araoz PA, et al. Sex differences in aortic valve calcification measured by multidetector computed tomography in aortic stenosis. Circ Cardiovasc Imaging. (2013) 6:40–7. doi: 10.1161/CIRCIMAGING.112.980052
24. Clavel MA, Messika-Zeitoun D, Pibarot P, Aggarwal SR, Malouf J, Araoz PA, et al. The complex nature of discordant severe calcified aortic valve disease grading: new insights from combined Doppler echocardiographic and computed tomographic study. J Am Coll Cardiol. (2013) 62:2329–38. doi: 10.1016/j.jacc.2013.08.1621
25. Elmariah S, Delaney JA, Bluemke DA, Budoff MJ, O'Brien KD, Fuster V, et al. Associations of LV hypertrophy with prevalent and incident valve calcification: multi-ethnic study of atherosclerosis. JACC Cardiovasc Imaging. (2012) 5:781–8. doi: 10.1016/j.jcmg.2011.12.025
26. Shanahan CM, Crouthamel MH, Kapustin A, Giachelli CM. Arterial calcification in chronic kidney disease: key roles for calcium and phosphate. Circ Res. (2011) 109:697–711. doi: 10.1161/CIRCRESAHA.110.234914
27. Hruska KA, Mathew S, Lund R, Qiu P, Pratt R. Hyperphosphatemia of chronic kidney disease. Kidney Int. (2008) 74:148–57. doi: 10.1038/ki.2008.130
28. Yoo KD, Kang S, Choi Y, Yang SH, Heo NJ, Chin HJ, et al. Sex, age, and the association of serum phosphorus with all-cause mortality in adults with normal kidney function. Am J Kidney Dis. (2016) 67:79–88. doi: 10.1053/j.ajkd.2015.06.027
29. Bortnick AE, Xu S, Kim RS, Kestenbaum B, Ix JH, Jenny NS, et al. Biomarkers of mineral metabolism and progression of aortic valve and mitral annular calcification: the multi-ethnic study of atherosclerosis. Atherosclerosis. (2019) 285:79–86. doi: 10.1016/j.atherosclerosis.2019.04.215
30. Diederichsen A, Lindholt JS, Moller JE, Gerke O, Rasmussen LM, Dahl JS. Sex differences in factors associated with progression of aortic valve calcification in the general population. Circ Cardiovasc Imaging. (2022) 15:e013165. doi: 10.1161/CIRCIMAGING.121.013165
31. Hisamatsu T, Miura K, Fujiyoshi A, Kadota A, Miyagawa N, Satoh A, et al. Serum magnesium, phosphorus, and calcium levels and subclinical calcific aortic valve disease: a population-based study. Atherosclerosis. (2018) 273:145–52. doi: 10.1016/j.atherosclerosis.2018.03.035
32. Aikawa E, Nahrendorf M, Figueiredo JL, Swirski FK, Shtatland T, Kohler RH, et al. Osteogenesis associates with inflammation in early-stage atherosclerosis evaluated by molecular imaging in vivo. Circulation. (2007) 116:2841–50. doi: 10.1161/CIRCULATIONAHA.107.732867
33. Liu AC, Joag VR, Gotlieb AI. The emerging role of valve interstitial cell phenotypes in regulating heart valve pathobiology. Am J Pathol. (2007) 171:1407–18. doi: 10.2353/ajpath.2007.070251
34. Li X, Yang HY, Giachelli CM. Role of the sodium-dependent phosphate cotransporter, pit-1, in vascular smooth muscle cell calcification. Circ Res. (2006) 98:905–12. doi: 10.1161/01.RES.0000216409.20863.e7
35. Speer MY, Li X, Hiremath PG, Giachelli CM. Runx2/Cbfa1, but not loss of myocardin, is required for smooth muscle cell lineage reprogramming toward osteochondrogenesis. J Cell Biochem. (2010) 110:935–47. doi: 10.1002/jcb.22607
36. Sage AP, Lu J, Tintut Y, Demer LL. Hyperphosphatemia-induced nanocrystals upregulate the expression of bone morphogenetic protein-2 and osteopontin genes in mouse smooth muscle cells in vitro. Kidney Int. (2011) 79:414–22. doi: 10.1038/ki.2010.390
37. Chen JH, Chen WL, Sider KL, Yip CY, Simmons CA. Beta-catenin mediates mechanically regulated, transforming growth factor-beta1-induced myofibroblast differentiation of aortic valve interstitial cells. Arterioscler Thromb Vasc Biol. (2011) 31:590–7. doi: 10.1161/ATVBAHA.110.220061
38. Rajamannan NM. Oxidative-mechanical stress signals stem cell niche mediated Lrp5 osteogenesis in eNOS(-/-) null mice. J Cell Biochem. (2012) 113:1623–34. doi: 10.1002/jcb.24031
39. Leibowitz D, Yoshida Y, Jin Z, Nakanishi K, Mannina C, Elkind MSV, et al. Factors associated with the progression of aortic valve calcification in older adults. Int J Cardiol. (2023) 381:76–80. doi: 10.1016/j.ijcard.2023.03.059
40. Nguyen V, Cimadevilla C, Estellat C, Codogno I, Huart V, Benessiano J, et al. Haemodynamic and anatomic progression of aortic stenosis. Heart. (2015) 101:943–7. doi: 10.1136/heartjnl-2014-307154
41. Ryu DR, Park SJ, Han H, Lee HJ, Chang SA, Choi JO, et al. Progression rate of aortic valve stenosis in Korean patients. J Cardiovasc Ultrasound. (2010) 18:127–33. doi: 10.4250/jcu.2010.18.4.127
42. Palta S, Pai AM, Gill KS, Pai RG. New insights into the progression of aortic stenosis: implications for secondary prevention. Circulation. (2000) 101:2497–502. doi: 10.1161/01.cir.101.21.2497
43. Koos R, Mahnken AH, Sinha AM, Wildberger JE, Hoffmann R, Kuhl HP. Aortic valve calcification as a marker for aortic stenosis severity: assessment on 16-MDCT. AJR Am J Roentgenol. (2004) 183:1813–8. doi: 10.2214/ajr.183.6.01831813
44. Messika-Zeitoun D, Aubry MC, Detaint D, Bielak LF, Peyser PA, Sheedy PF, et al. Evaluation and clinical implications of aortic valve calcification measured by electron-beam computed tomography. Circulation. (2004) 110:356–62. doi: 10.1161/01.CIR.0000135469.82545.D0
45. Yan L, Schoenmakers I, Zhou B, Jarjou LM, Smith E, Nigdikar S, et al. Ethnic differences in parathyroid hormone secretion and mineral metabolism in response to oral phosphate administration. Bone. (2009) 45:238–45. doi: 10.1016/j.bone.2009.04.237
Keywords: calcified aortic valve disease, atherosclerosis, cardiac computed tomography, echocardiography, calcium-phosphate metabolism
Citation: Kim KA, Jung H-O, Kim M-J, Lee S-Y, Ahn Y, Jung M-H, Chung W-B, Lee D-H, Youn H-J and Chang H-J (2024) Higher serum phosphate within the normal range is associated with the development of calcified aortic valve disease. Front. Cardiovasc. Med. 11:1450757. doi: 10.3389/fcvm.2024.1450757
Received: 18 June 2024; Accepted: 12 September 2024;
Published: 26 September 2024.
Edited by:
Ramdas G. Pai, University of California, Riverside, United StatesReviewed by:
Francesca Bartoli-Leonard, University of Bristol, United KingdomAlexander Fletcher, University of Glasgow, United Kingdom
Copyright: © 2024 Kim, Jung, Kim, Lee, Ahn, Jung, Chung, Lee, Youn and Chang. This is an open-access article distributed under the terms of the Creative Commons Attribution License (CC BY). The use, distribution or reproduction in other forums is permitted, provided the original author(s) and the copyright owner(s) are credited and that the original publication in this journal is cited, in accordance with accepted academic practice. No use, distribution or reproduction is permitted which does not comply with these terms.
*Correspondence: Hae-Ok Jung, aG9qaGVhcnRAZ21haWwuY29t