- 1Department of Cardiology, Beijing Hospital, National Center of Gerontology, Institute of Geriatric Medicine, Chinese Academy of Medical Sciences, Beijing, China
- 2Graduate School of Peking Union Medical College, Chinese Academy of Medical Sciences, Beijing, China
Background: There is limited knowledge regarding the association between heart rate (HR) during different exercise phases and coronary artery disease (CAD). This study aimed to evaluate the relationship between four exercise-related HR metrics detected by cardiopulmonary exercise testing (CPET) and CAD. These metrics include HR at the anaerobic threshold (HRAT), HR at respiratory compensatory point (HRRCP), maximal HR (HRmax), and HR 60 s post-exercise (HRRec60s).
Methods: The 705 participants included 383 with CAD and 322 without CAD in Beijing Hospital, who underwent CPET between January 2021 and December 2022. The Logistic regression analysis was applied to estimate the odds ratio and the 95% confidence interval. Additionally, the multivariable Logistic regression analyses with restricted cubic splines were conducted to characterize the dose-response association and explore whether the relationship was linear or nonlinear.
Results: Our primary finding indicates that for each one-beat increase in HRAT, there is a 2.8% reduction in the adjusted risk of CAD in the general population. Similarly, a one-beat increase in HRRCP corresponds to a 2.6% reduction in the adjusted risk of CAD. Subgroup analyses revealed significant interactions between HRAT and factors such as sex, hypertension, and lung cancer, as well as between HRRCP and sex and hypertension, in relation to CAD. The dose-response analysis further confirmed that higher HRAT and HRRCP are associated with a reduced risk of CAD.
Conclusion: These results are suggestive of a good association between HRAT, HRRCP, and CAD. The lower HRAT, and HRRCP are signs of poor HR response to exercise in CAD. HRAT and HRRCP are potentially good indicators of poor HR response to exercise without considering maximal effort.
1 Introduction
Coronary artery disease (CAD) is a leading cause of morbidity and mortality, posing a growing public health burden worldwide (1). Prediction and early diagnosis of CAD facilitates appropriate intervention in its early stages, aiming to improve the prognosis, delay the progression, and reduce the burden on patients and their families. In accordance with the latest guidelines from the American College of Cardiology and the American Heart Association, exercise stress testing was recommended as an initial diagnostic test for suspected CAD patients (2).
Cardiopulmonary exercise testing (CPET) is a non-invasive method of evaluating an individual's cardiovascular, muscular, respiratory, and metabolic responses to physical stress through exercise stress testing combined with expired gas analysis (3). Unlike the current “gold standard” for diagnosing CAD, which relies on invasive coronary angiography, CPET offers a safer, less expensive, and more psychologically comfortable alternative for patients. Although CPET involves a variety of complex indicators, each parameter provides distinct diagnostic and prognostic insights, making this area increasingly important in clinical practice.
We will emphasize the two vital lactic acid (LA)-related metabolism points during exercise in our study. As the incremental exercise testing proceeds, the LA in the circulation begins to accumulate eventually leading to hypercapnia at the end of the exercise (4). Once a working skeletal muscle cell begins to produce LA, the anaerobic threshold (AT) is reached. AT marks the transition to mixed aerobic-anaerobic metabolism (5). The work intensity increases continuously and gradually to go beyond a certain point called the respiratory compensation point (RCP), where LA production can no longer be compensated by circulating bicarbonate, then hyperventilation begins. RCP represents the transition to predominant anaerobic metabolism (5).
Heart rate (HR) is thought to have a broad and complex relationship with the cardiac function of CAD. However, the significance of HR, particularly exercise-induced HR, in understanding cardiovascular pathophysiology, prognosis, and treatment is often underestimated. This may be due to the complex nature of its effects, despite HR being a familiar and easily measurable parameter (6). Previous evidence has consistently shown that elevated resting heart rate (HR) is an independent predictor of both all-cause and cardiovascular mortality in patients with CAD (7–9). Additionally, poor exercise capacity and inadequate HR response during exercise and recovery are significant indicators of higher overall mortality and an increased risk of CAD (10–12). However, most previous studies have primarily focused on resting HR and HR recovery post-exercise in CAD patients, often neglecting the importance of HR at various stages of exercise.
In this study, we examined four specific HR metrics during different phases of CPET as potential predictors of CAD: HR at the anaerobic threshold (HRAT), HR at the respiratory compensatory point (HRRCP), maximal HR (HRmax), and HR 60 s post-exercise (HRRec60s). This cross-sectional, population-based study aimed to compare these exercise-related HR measurements to determine which one is most strongly associated with CAD in the general population.
2 Method
2.1 Ethics statements
This cross-sectional study conformed to the Declaration of Helsinki and was approved by the Committee of Beijing Hospital (2023BJYYEC-116-01).
2.2 Study population
This cross-sectional study included 705 patients, aged 18–60, who underwent CPET at Beijing Hospital between January 2021 and December 2022. The testing was conducted to screen for cardiopulmonary disease or to evaluate exercise capacity and/or the severity of CAD.
The diagnosis of coronary artery disease (CAD) was confirmed by reviewing each patient's inpatient and/or outpatient medical records. Documented CAD was defined by the presence of at least one of the following criteria: (1) ≥50% stenosis in at least one coronary artery trunk or major branch as demonstrated by percutaneous coronary angiography or computed tomography; (2) typical exertional angina symptoms with a positive stress test (electrocardiogram stress test, stress echocardiography, or nuclear myocardial stress imaging); (3) previously diagnosed myocardial infarction; (4) previously diagnosed unstable angina pectoris (typical ischemic chest pain + ECG changes + increased markers of muscle damage; or the dynamic changes of ST segment during ischemic attack, or coronary angiography confirmed the existence of severe lesions leading to symptoms) (13, 14). According to the history of CAD, there were 322 participants in the non-CAD group and 383 participants in the CAD group.
2.3 Data collection
Baseline characteristics for the target population were gathered from electronic medical records. These included demographics, comorbidities, chronic medications, past medical history, and biochemical data such as total cholesterol (TC), triglyceride (TG), high-density lipoprotein cholesterol (HDL-C), low-density lipoprotein cholesterol (LDL-C), and N-terminal pro-B-type natriuretic peptide (NT-proBNP) or brain natriuretic peptide (BNP).
2.4 Cardiopulmonary exercise test
The cardiopulmonary function detector (MasterScreen CPX, Jaeger, Switzerland) was used to detect the changes of oxygen consumption (VO2) and carbon dioxide (VCO2) emission on an upright cycle ergometer (Ergoselect 100p, Ergoline, Germany) or mechanical treadmill (T2100-ST2, GE, America). Meanwhile, a 12-lead ECG recorder (CASE, GE, America) and a dynamic blood pressure monitor (TangoM2, SunTech, America) records continuously the HR and blood pressure (BP).
CPET monitored the following parameters for each participant at resting, AT, RCP, and peak states, and 1, 2 and 3 min after exercise, including work load (WL), minute ventilation (VE), VO2, oxygen consumption/kilogram (VO2/kg; which is considered as the peak VO2 at the maximal WL), VCO2, HR, respiratory rate (RR), oxygen pulse (VO2/HR), dead space (VD), tidal volume (VT), systolic blood pressure (SBP), diastolic blood pressure (DBP), breathing reserve (BR), respiratory quotient (RQ), end-tidal carbon dioxide pressure (PETCO2), end-tidal oxygen pressure (PETO2), and oxygen saturation (SpO2). The VE/VCO2 was calculated. The detailed CPET assessment protocol includes a 3-min rest and 3-min warm-up at 0 watts (W), followed by a continuous increase in the Work Rate (WR) by 10 W/min to 20 W/min until exhaustion. A respiratory exchange ratio (RER, ratio of VCO2/VO2 at peak exercise) of ≥1.05 was considered an objective indicator of peak effort during assessment (15). Borg Scale (scale 6–20) > 17 was regarded as a subjective index. Discontinue the exercise test if any of the following occurs: abnormal hemodynamic or ECG exercise response or other reasons such as dyspnea, angina, or lower extremity muscle fatigue (16, 17).
AT and RCP were located by visual inspection. AT is deemed reached when the following criteria are met: (1) the VE/VO2 curve starts to rise with the VE/VCO2 curve remaining constant, and (2) PETO2 starts to rise with PETCO2 remaining unchanged. RCP is deemed to be reached when the following criteria are met: (1) a decrease in PETCO2 after reaching a maximal level; (2) a rapid nonlinear increase in VE (second deflection); (3) the VE/VCO2 ratio reached a minimum and began to increase and (4) a nonlinear increase in VCO2 vs. VO2 (departure from linearity) (4, 18).
2.5 Heart rate
Continuous ambulatory electrocardiograms were recorded using a 12-lead ECG recorder (CASE, GE, America) during the maximum symptom-limited CPET. HR was recorded at AT and RCP, designated as HRAT and HRRCP, respectively. HRmax was defined as the highest HR achieved during the CPET. Additionally, HR 60 s after the exercise session, referred to as HRRec60s, was measured during the recovery period.
2.6 Statistical analysis
The random forest method was used to impute missing data (19). Based on their CAD history, participants were categorized into a CAD group and a non-CAD group. The distributions of variables in each group were assessed using the Kolmogorov-Smirnov test. Continuous variables that followed a normal distribution were reported as mean ± standard deviation and analyzed using Student's t-test. For variables that did not follow a normal distribution, data were presented as median and interquartile range (IQR), with the rank-sum test applied for comparison. Categorical variables were expressed as counts and percentages and compared using the Chi-square test.
Logistic regression analysis was used to estimate the odds ratios (OR) and 95% confidence intervals (CIs) for the continuous HR-related indices (HRRCP, HRAT, HRmax, and HRRec60s) in relation to the outcome of CAD. Additionally, we categorized the HR-related indices into tertiles and compared the associations between the medium and highest tertiles with the lowest tertile. Subgroup analysis was performed to determine whether the relationship between CAD and HR-related indices differed across various subgroups defined by covariates and comorbid conditions. We examined the interaction effects of CAD with HR-related indices in several participant subgroups (age grouping, sex, hypertension, diabetes mellitus, hyperlipidemia, and lung cancer status), and the Wald test determined the P for interaction. We constructed three multivariable Logistic regression models. Model 1 was unadjusted. The second and third adjustment models with robust adjustment for covariates are thought to be potential confounders of the associations of the HR-related indices with CAD. Thus, model 2 included age (Continuous), sex (male or female), and body mass index [normal (18.5–25 kg/m2), overweight (≥25 kg/m2) or low (<18.5 kg/m2)]; model 3 further adjusted for hypertension, diabetes mellitus, hyperlipidemia, chronic kidney disease, and lung cancer status (yes or no).
The multivariable Logistic regression analyses with restricted cubic splines (RCS) were used to characterize the dose-response association and explore the potential linear or nonlinear relationship of the HR-related indices with the CAD. The Akaike information criterion (AIC) was used to identify the knots for the splines to balance best fit and overfitting in the RCS (20). The medians of the HR-related indices were assigned as the reference values. The test result for nonlinearity was checked first. If the test for nonlinearity was insignificant, the overall association test result was checked, with the considerable result indicating the linear association.
For statistical analysis, R (version 4.2.2; https://www.R-project.org) was utilized. A result with a two-sided P value < 0.05 was considered statistically significant when testing the hypotheses of the study.
3 Result
3.1 Comparison of baseline characteristics between CAD group and non-CAD group
A total of 705 eligible participants met all the inclusion and none of the exclusion criteria. The average age was 59.40 ± 11.44 years, and 408 (57.90%) were men. Compared with the non-CAD group, participants with CAD tended to be male, older, and have higher BMI, VO2/HR in the period of resting, AT, RCP, and peak (all P < 0.05). The proportions of Co-morbid conditions, including hypertension, diabetes mellitus (DM), hyperlipidemia, chronic kidney disease (CKD), and stroke were higher in the CAD group. The participants in the non-CAD group had higher TC, HDL-C, LDL-C, and HR in the period of resting, AT, RCP, and peak, and VO2/kg in the period of AT, RCP, and peak, and lower percentage of lung cancer, than in the CAD group (all P < 0.05) (Table 1). The baseline characteristics of participants grouped by HR-related indices tertiles were shown in the Supplementary Tables S1–S4.
3.2 Multivariable logistic regression analysis of HR-related indices associated with CAD
Tables 2, 3 shows the results of associations between HR-related indices and risk of CAD using the multivariable Logistic regression analysis. The fully multivariable-adjusted ORs (95% CIs) per 1 unit increase of HRAT, HRRCP, HRmax, and HRRec60s for CAD were 0.972 (0.960, 0.984), 0.974 (0.963, 0.985), 0.980 (0.971, 0.990), and 0.984 (0.975, 0.993), respectively. Compared with participants with HRAT <96 bpm, the multivariable-adjusted ORs (95% CIs) were 0.618 (0.390, 0.974) and 0.368 (0.229, 0.589) for CAD in participants with HRAT ranged from 96 to 110 bpm and ≥110 bpm. Compared with participants with HRRCP < 111 beats per minute (bpm), the multivariable-adjusted ORs (odds ratio, 95% CIs) were 0.394 (0.244, 0.629) and 0.336 (0.204, 0.546) for CAD in participants with HRRCP ranged from 111 to 127 bpm and ≥127 bpm. Compared with participants with HRmax < 121 bpm, the multivariable-adjusted ORs (95% CIs) were 0.448 (0.279, 0.713) and 0.317 (0.192, 0.518) for CAD in participants with HRmax ranged from 121 to 142 bpm and ≥142 bpm. Compared with participants with HRRec60s < 105 bpm, the multivariable-adjusted ORs (95% CIs) were 0.375 (0.232, 0.597) and 0.376 (0.231, 0.608) for CAD in participants with HRRec60 s ranged from 105 to 123 bpm, and ≥123 bpm.
3.3 Subgroup analyses
Tables 4, 5 summarized the results of subgroup analysis between the HR-related indices and CAD according to different subgroups, including age, sex, hypertension, DM, hyperlipidemia, and lung cancer status, using multivariable Logistic regression analyses adjusting for age (continuous), sex (male or female), body mass index (BMI, normal [18.5–25 kg/m2], overweight [≥25 kg/m2] or low [<18.5 kg/m2], hypertension, diabetes mellitus, hyperlipidemia, chronic kidney disease, and lung cancer status (yes or no).
In subgroup analyses, statistically significant interactions were not observed between HRRec60s and any study covariates in relation to CAD (all P for interaction > 0.05). The interactions between HRAT and sex, hypertension status, and lung cancer in relation to CAD were statistically significant (P for interaction = 0.001, 0.036, and 0.036, respectively). Statistically meaningful interactions were noted between HRRCP and sex and hypertension status in relation to CAD (P for interaction = 0.001 and 0.021, respectively). The interactions between HRmax and sex and hypertension status in relation to CAD were also statistically significant (P for interaction = 0.027 and 0.037, respectively).
3.4 Dose-response analysis of the HR-related indices with CAD
Multivariable-adjusted RCS analyses revealed a linear association of HRAT and HRRCP and with CAD (all P for nonlinear > 0.05; Figures 1B, D). With increasing HR, the risk of CAD is reduced sharply. Nonlinear relationships of HRmax and HRRec60s with CAD were observed (all P for nonlinear < 0.05; Figures 1F, H).
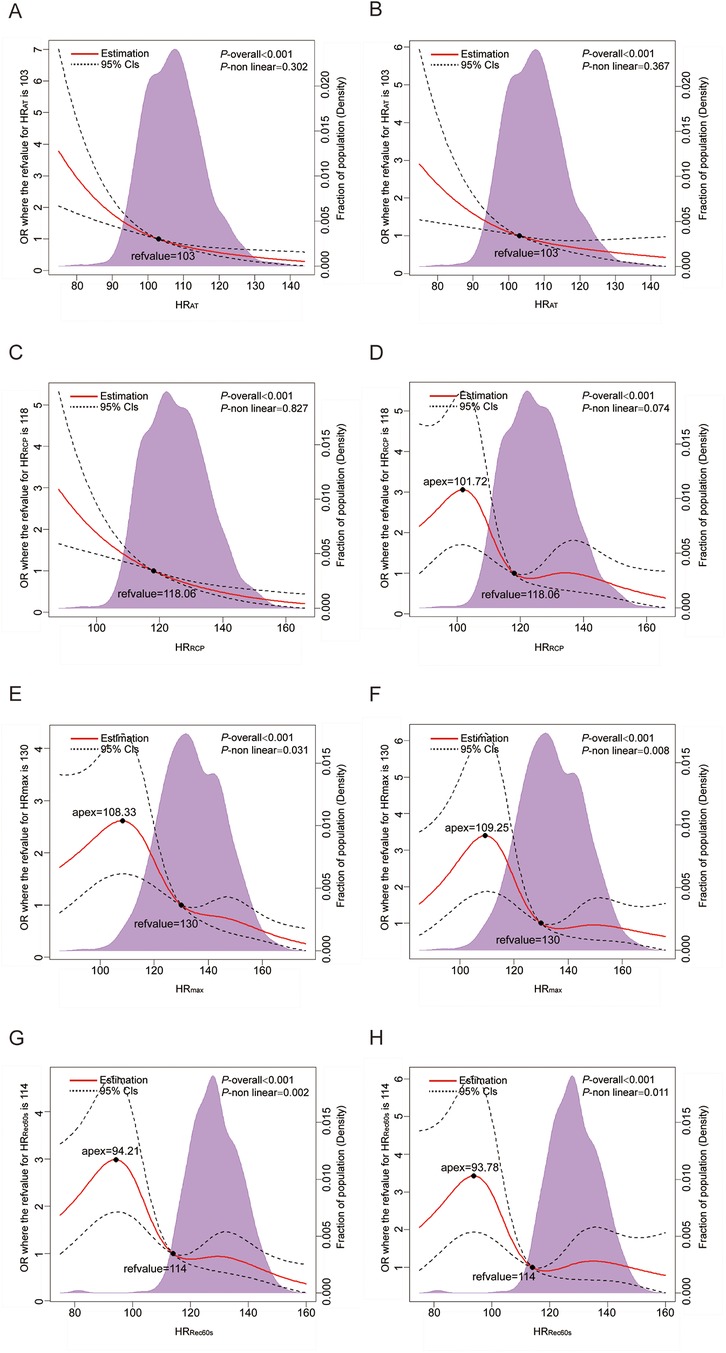
Figure 1. Dose–response analysis of the HR-related indices with CAD. (A,C,E,G) Dose–response analysis of HRAT, HRRCP, HRmax, and HRRec60s using model 1; (B,D,F,H) Dose–response analysis of HRAT, HRRCP, HRmax, and HRRec60s using model 3. Model 1: unadjusted model; Model 3: Further adjusted for hypertension, diabetes mellitus, hyperlipidemia, chronic kidney disease and lung cancer status (yes or no). HR, heart rate; RCP, respiratory compensation point; AT, anaerobic threshold; max, maximum; Rec60 s, post-exercise after 60 s.
4 Discussion
This present study aimed to investigate the association of HRAT, HRRCP, HRmax, and HRRec60s assessed by CPET with CAD. Analyzing a robust sample of 705 participants—322 without CAD and 383 with CAD—we mainly found that each additional beat per minute in HRAT was associated with a 2.8% lower adjusted risk of CAD, and each additional beat per minute in HRRCP was linked to a 2.6% lower adjusted risk of CAD in the general population. Referring to participants with HRAT < 96 bpm, the risk of CAD of the participants with HRAT ranged from 96 to 110 bpm and ≥110 bpm lower 38.2% and 63.2%, respectively. Referring to participants with HRRCP < 111 bpm, the risk of CAD of the participants with HRRCP ranged from 111 to 127 bpm and ≥127 bpm lower 60.6% and 66.4%, respectively. Further subgroup analysis showed significant interactions between HRAT and sex, hypertension and lung cancer; HRRCP and sex and hypertension in relation to CAD. The Dose-Response Analysis revealed with increasing HRAT and HRRCP, the risk of CAD is reduced sharply. These results are suggestive of a good association between HRAT and HRRCP with CAD. Thus, it is necessary to fully exploit the potential clinic diagnostic value of HRAT and HRRCP.
Around the world, the prevalence of CAD has increased dramatically due to an aging population, unhealthy lifestyles, and environmental changes following decades of rapid economic development. According to the Report on Cardiovascular Health and Diseases in China 2022 (21): An Updated Summary, the number of current CAD patients in China is estimated to be 11.39 million. Early diagnosis and recognition of CAD is essential. Thoroughly exploring the clinical diagnostic value of CPET is required, as it is a more sensitive and comprehensive method of screening for CAD than resting electrocardiogram (ECG) and ECG-only cardiac stress testing in adults with suspected CAD, especially asymptomatic people (22). The gas analysis can detect myocardial ischemia with reduced pulse volume and cardiac output during exercise before ST-segment changes or chest pain develops (23).
The evidence base for CPET screening and diagnosing CAD has grown exponentially over the past few decades. The European Association for Cardiovascular Prevention and Rehabilitation (EACPR) and American Heart Association (AHA) recommended a diagnostic stratification chart for patients with suspected myocardial ischemia, applying primary CPET variables such as O2 pulse trajectory, per cent-predicted Peak oxygen uptake (VO2peak), and ΔVO2/ΔWR trajectory (24). In this chart, the progressive variables are indicative of poorer aerobic fitness and possibly increased CAD severity. Further, existing studies suggested respiratory equivalent during anaerobic threshold (VE/VCO2) (25, 26), VO2peak (27), time to reach the anaerobic threshold (TAT) (28), VO2/HR (29), the ratio of the increase in VO2 to the increase in work rate (ΔVO2/ΔWR) (22, 23) can be abnormal for CAD patients thus them are also significant parameters supporting diagnosis of CAD. However, for most of these CPET variables, a prerequisite for their accuracy and suggestive value is that the patients reach peak exercise at or near maximum effort or have a RER greater than 1.05. In the clinical process, it was hard for CAD patients, especially those with severe symptoms or long-term no exercise, to perform the near-maximal effort in the cardiopulmonary exercise test. Approximately 4% to 22% of patients with cardiovascular disease fail to reach peak effort due to premature interruption of exercise testing for some motivational or emotional (anxiety) reason or medical reasons assessed by the supervisor (30).
During exercise with progressively increasing workload, ventilation follows three distinct domains regulated respectively by oxygen uptake, carbon dioxide production, and unbuffered acidosis. The entire progressive exercise is therefore divided into three domains in order: from beginning to AT, between AT and RCP, and from RCP to the end (31). AT is a submaximal index of exercise capacity, which signifies a metabolic transition toward increased glycolysis and raised lactate with an associated metabolic acidosis (32). The RCP is a point that marks the onset of hyperventilation during incremental exercise, which forms the boundary between the heavy and severe exercise intensity domains (33). Very few studies have reported the relationship between AT, RCP, and cardiovascular disease, especially CAD. Nakade et al. demonstrated that the duration between the RCP and AT (RCP-AT time) can predict the severity of cardiac disorders and prognosis in patients with heart failure with reduced fraction ejection (34). Alberto et al. found RCP-AT time significantly predicts CAD in patients with anginal chest pain and Left bundle branch block. Our study focused on the HR at AT and RCP in CAD patients for the first time (28).
The acute HR response to exercise, HR increase during exercise, and HR recovery after exercise provide unique insights into cardiac physiology compared to resting HR and can therefore be used to gain more information about cardiac function (35, 36). An impaired HR response to exercise (i.e., chronotropic incompetence, CI) has been shown to be predictive of all-cause mortality and risk of incident CAD, even after accounting for age, physical fitness, and standard cardiovascular risk factors (37). CI is commonly considered when (1) HRmax during exercise < 85% of the maximal age-predicted heart rate; or (2) failure to attain 80% of heart rate reserve (38). However, it is vital to consider the patient's level of effort and the reason for terminating the exercise test before diagnosing CI (39). That means the conclusion of CI requires that the patient perform near-maximal effort in CPET. For CAD patients who find it hard to reach peak effort, how do we properly find “poor HR response to exercise”? Our main finding of association between CAD and HRAT or HRRCP perhaps provide a potential clinic diagnostic value to find impaired chronotropic response upon heavy not severe intensity exercise.
The HR at any moment reflects the dynamic balance between sympathetic and parasympathetic nerves in the autonomic nervous system. Unlike resting HR, exercise HR is also largely influenced by cardiorespiratory fitness (40). The gradually increasing HR is the most significant contributor to the ability to sustain aerobic exercise. An intact HR response is essential to closely match a patient's cardiac output to the metabolic demands of exercise. Not only the inability to achieve maximal HR, submaximal HR insufficiency, or HR instability during exertion are all signs of an impaired chronotropic response (39). These complaints are relatively common in CAD, sick sinus syndrome, atrioventricular block, heart failure, and so on. Based on our analysis, the lower HRAT, HRRCP, and HRmax are signs of impaired chronotropic response in CAD. HRAT and HRRCP are potentially good indicators of impaired chronotropic response without considering maximal effort. In addition, the underlying mechanisms for CI in CAD and other cardiovascular disorders are incompletely understood. Referring to the mechanism of CI, we assumed that lower HRAT and HRRCP for CAD are related to autonomic nervous system dysfunction. These results are suggestive of a good association between HRAT, HRRCP, and CAD. Based on our analysis, the lower HRAT, HRRCP, and HRmax are signs of impaired chronotropic response in CAD. HRAT and HRRCP are potentially good indicators of impaired chronotropic response without considering maximal effort.
The study has potential limitation. First, the patients included in this study were all from Beijing Hospital and only represented a single-center study. The sample size of patients included was limited. Further confirmation clinic trials involving larger sample sizes and multiple centers are necessary. Second, this study was cross-sectional and does not allow for causal inferences; a longitudinal study is needed before forming any causal links. Third, the degree of stenosis of the coronary arteries in the included population should be graded to assess the association between HR and CAD further, but limited due to that not every participant had a result of invasive coronary angiography or coronary computer tomography angiography. Fourth, the diagnostic value of HRAT and HRRCP for suspected CAD is inappropriate for patients who cannot exercise and/or augment the HR response (advanced CAD, autonomic dysfunction, and HR-limiting medications causing CI).
5 Conclusion
These results are suggestive of a good association between HRAT, HRRCP, and CAD. The lower HRAT, HRRCP, and HRmax are signs of poor HR response to exercise in CAD. HRAT and HRRCP are potentially good indicators of poor HR response to exercise without considering maximal effort.
Data availability statement
The original contributions presented in the study are included in the article/Supplementary Material, further inquiries can be directed to the corresponding authors.
Ethics statement
The studies involving humans were approved by Ethics Committee of Beijing Hospital (No.2023BJYYEC-116-01). The studies were conducted in accordance with the local legislation and institutional requirements. The Ethics Committee/institutional review board waived the requirement of written informed consent for participation from the participants or the participants’ legal guardians/next of kin because this study is retrospective and involves analysis of existing medical records and data, so written informed consent is waived. This study poses minimal risk to participants as it does not involve any direct interaction or intervention. In addition, all personal and identifying information in this study is anonymized to protect the privacy of the patients and further reduce any potential risks.
Author contributions
YK: Conceptualization, Formal Analysis, Investigation, Methodology, Writing – original draft. RS: Conceptualization, Formal Analysis, Investigation, Methodology, Writing – original draft. TX: Data curation, Investigation, Writing – review & editing. JZ: Data curation, Investigation, Writing – review & editing. CX: Data curation, Writing – review & editing. TZ: Project administration, Writing – review & editing. FW: Funding acquisition, Project administration, Supervision, Writing – review & editing.
Funding
The author(s) declare financial support was received for the research, authorship, and/or publication of this article. This work was supported by Beijing Jiekai Cardiovascular Health Foundation.
Conflict of interest
The authors declare that the research was conducted in the absence of any commercial or financial relationships that could be construed as a potential conflict of interest.
Publisher's note
All claims expressed in this article are solely those of the authors and do not necessarily represent those of their affiliated organizations, or those of the publisher, the editors and the reviewers. Any product that may be evaluated in this article, or claim that may be made by its manufacturer, is not guaranteed or endorsed by the publisher.
Supplementary material
The Supplementary Material for this article can be found online at: https://www.frontiersin.org/articles/10.3389/fcvm.2024.1442857/full#supplementary-material
Abbreviations
AT, anaerobic threshold; CAD, coronary artery disease; CI, chronotropic incompetence; CPET, cardiopulmonary exercise test; ECG, electrocardiogram; HR, heart rate; RCP, respiratory compensatory point; RER, respiratory exchange ratio; VO2, oxygen consumption.
References
1. Martin SS, Aday AW, Almarzooq ZI, Anderson CA, Arora P, Avery CL, et al. 2024 Heart disease and stroke statistics: a report of US and global data from the American Heart Association. Circulation. (2024) 149(8):e347–913. doi: 10.1161/CIR.0000000000001209
2. Otto C, Nishimura R, Bonow R, Carabello B, Erwin J, Gentile F, et al. 2020 ACC/AHA guideline for the management of patients with valvular heart disease: executive summary: a report of the American College of Cardiology/American Heart Association Joint Committee on clinical practice guidelines. Circulation. (2021) 143(5):e35–71. doi: 10.1161/CIR.0000000000000932
3. Society. AT. ATS/ACCP statement on cardiopulmonary exercise testing. Am J Respir Crit Care Med. (2003) 167(2):211–77. doi: 10.1164/rccm.167.2.211
4. Beaver W, Wasserman K, Whipp B. A new method for detecting anaerobic threshold by gas exchange. J Appl Physiol. (1986) 60(6):2020–7. doi: 10.1152/jappl.1986.60.6.2020
5. Glaab T, Taube C. Practical guide to cardiopulmonary exercise testing in adults. Respir Res. (2022) 23(1):9. doi: 10.1186/s12931-021-01895-6
6. Fox K, Ferrari R. Heart rate: a forgotten link in coronary artery disease? Nat Rev Cardiol. (2011) 8(7):369–79. doi: 10.1038/nrcardio.2011.58
7. Diaz A, Bourassa M, Guertin M, Tardif J. Long-term prognostic value of resting heart rate in patients with suspected or proven coronary artery disease. Eur Heart J. (2005) 26(10):967–74. doi: 10.1093/eurheartj/ehi190
8. Zhang D, Wang W, Li F. Association between resting heart rate and coronary artery disease, stroke, sudden death and noncardiovascular diseases: a meta-analysis. CMAJ. (2016) 188(15):E384–E92. doi: 10.1503/cmaj.160050
9. Lang C, Gupta S, Kalra P, Keavney B, Menown I, Morley C, et al. Elevated heart rate and cardiovascular outcomes in patients with coronary artery disease: clinical evidence and pathophysiological mechanisms. Atherosclerosis. (2010) 212(1):1–8. doi: 10.1016/j.atherosclerosis.2010.01.029
10. Lauer M, Okin P, Larson M, Evans J, Levy D. Impaired heart rate response to graded exercise. Prognostic implications of chronotropic incompetence in the Framingham heart study. Circulation. (1996) 93(8):1520–6. doi: 10.1161/01.CIR.93.8.1520
11. Sandvik L, Erikssen J, Ellestad M, Erikssen G, Thaulow E, Mundal R, et al. Heart rate increase and maximal heart rate during exercise as predictors of cardiovascular mortality: a 16-year follow-up study of 1960 healthy men. Coron Artery Dis. (1995) 6(8):667–79. doi: 10.1097/00019501-199508000-00012
12. Johnson N, Goldberger J. Prognostic value of late heart rate recovery after treadmill exercise. Am J Cardiol. (2012) 110(1):45–9. doi: 10.1016/j.amjcard.2012.02.046
13. Pepine C, Handberg E, Cooper-DeHoff R, Marks R, Kowey P, Messerli F, et al. A calcium antagonist vs a non-calcium antagonist hypertension treatment strategy for patients with coronary artery disease. The international verapamil-trandolapril study (INVEST): a randomized controlled trial. JAMA. (2003) 290(21):2805–16. doi: 10.1001/jama.290.21.2805
14. Liu Y, Zhu B, Zhou W, Du Y, Qi D, Wang C, et al. Triglyceride-glucose index as a marker of adverse cardiovascular prognosis in patients with coronary heart disease and hypertension. Cardiovasc Diabetol. (2023) 22(1):133. doi: 10.1186/s12933-023-01866-9
15. Mehra M, Canter C, Hannan M, Semigran M, Uber P, Baran D, et al. The 2016 international society for heart lung transplantation listing criteria for heart transplantation: a 10-year update. J Heart Lung Transplant. (2016) 35(1):1–23. doi: 10.1016/j.healun.2015.10.023
16. Guazzi M, Arena R, Halle M, Piepoli M, Myers J, Lavie C. 2016 focused update: clinical recommendations for cardiopulmonary exercise testing data assessment in specific patient populations. Circulation. (2016) 133(24):e694–711. doi: 10.1161/CIR.0000000000000406
17. Guazzi M, Arena R, Halle M, Piepoli M, Myers J, Lavie C. 2016 focused update: clinical recommendations for cardiopulmonary exercise testing data assessment in specific patient populations. Eur Heart J. (2018) 39(14):1144–61. doi: 10.1093/eurheartj/ehw180
18. Price S, Wiecha S, Cieśliński I, Śliż D, Kasiak P, Lach J, et al. Differences between treadmill and cycle ergometer cardiopulmonary exercise testing results in triathletes and their association with body composition and body mass index. Int J Environ Res Public Health. (2022) 19(6):3557. doi: 10.3390/ijerph19063557
19. Shah A, Bartlett J, Carpenter J, Nicholas O, Hemingway H. Comparison of random forest and parametric imputation models for imputing missing data using MICE: a CALIBER study. Am J Epidemiol. (2014) 179(6):764–74. doi: 10.1093/aje/kwt312
20. Johannesen CDL, Langsted A, Mortensen MB, Nordestgaard BG. Association between low density lipoprotein and all cause and cause specific mortality in Denmark: prospective cohort study. Br Med J. (2020) 372:n422. doi: 10.1136/bmj.n422
21. The W. Report on cardiovascular health and diseases in China 2022: an updated summary. Biomed Environ Sci. (2023) 36(8):669–701. doi: 10.3967/bes2023.106
22. Belardinelli R, Lacalaprice F, Tiano L, Muçai A, Perna G. Cardiopulmonary exercise testing is more accurate than ECG-stress testing in diagnosing myocardial ischemia in subjects with chest pain. Int J Cardiol. (2014) 174(2):337–42. doi: 10.1016/j.ijcard.2014.04.102
23. Belardinelli R, Lacalaprice F, Carle F, Minnucci A, Cianci G, Perna G, et al. Exercise-induced myocardial ischaemia detected by cardiopulmonary exercise testing. Eur Heart J. (2003) 24(14):1304–13. doi: 10.1016/S0195-668X(03)00210-0
24. Guazzi M, Adams V, Conraads V, Halle M, Mezzani A, Vanhees L, et al. EACPR/AHA scientific statement. Clinical recommendations for cardiopulmonary exercise testing data assessment in specific patient populations. Circulation. (2012) 126(18):2261–74. doi: 10.1161/CIR.0b013e31826fb946
25. Mazaheri R, Shakerian F, Vasheghani-Farahani A, Halabchi F, Mirshahi M, Mansournia M. The usefulness of cardiopulmonary exercise testing in assessment of patients with suspected coronary artery disease. Postgrad Med J. (2016) 92(1088):328–32. doi: 10.1136/postgradmedj-2015-133576
26. Dominguez-Rodriguez A, Abreu-Gonzalez P, Gomez M, Garcia-Baute MC, Arroyo-Ucar E, Avanzas P, et al. Myocardial perfusion defects detected by cardiopulmonary exercise testing: role of VE/VCO2 slope in patients with chest pain suspected of coronary artery disease. Int J Cardiol. (2012) 155(3):470–1. doi: 10.1016/j.ijcard.2011.12.063
27. Letnes J, Dalen H, Vesterbekkmo E, Wisløff U, Nes B. Peak oxygen uptake and incident coronary heart disease in a healthy population: the HUNT fitness study. Eur Heart J. (2019) 40(20):1633–9. doi: 10.1093/eurheartj/ehy708
28. Dominguez-Rodriguez A, Abreu-Gonzalez P, Gomez M, Garcia-Baute MC, Arroyo-Ucar E, Avanzas P, et al. Assessing coronary artery disease in patients with anginal chest pain and left bundle branch block: an emerging role for a new parameter of cardiopulmonary exercise testing. Crit Pathw Cardiol. (2012) 11(4):214–7. doi: 10.1097/HPC.0b013e31826298d6
29. Laukkanen J, Kurl S, Salonen J, Lakka T, Rauramaa R. Peak oxygen pulse during exercise as a predictor for coronary heart disease and all cause death. Heart (British Cardiac Society). (2006) 92(9):1219–24. doi: 10.1136/hrt.2005.077487
30. Vanhees L, Stevens A, Schepers D, Defoor J, Rademakers F, Fagard R. Determinants of the effects of physical training and of the complications requiring resuscitation during exercise in patients with cardiovascular disease. Eur J Cardiovasc Prev Rehabil. (2004) 11(4):304–12. doi: 10.1097/01.hjr.0000136458.44614.a2
31. Binder R, Wonisch M, Corra U, Cohen-Solal A, Vanhees L, Saner H, et al. Methodological approach to the first and second lactate threshold in incremental cardiopulmonary exercise testing. Eur J Cardiovasc Prev Rehabil. (2008) 15(6):726–34. doi: 10.1097/HJR.0b013e328304fed4
32. Poole D, Rossiter H, Brooks G, Gladden L. The anaerobic threshold: 50+ years of controversy. J Physiol (Lond). (2021) 599(3):737–67. doi: 10.1113/JP279963
33. Wasserman K, Whipp B, Koyl S, Beaver W. Anaerobic threshold and respiratory gas exchange during exercise. J Appl Physiol. (1973) 35(2):236–43. doi: 10.1152/jappl.1973.35.2.236
34. Nakade T, Adachi H, Murata M, Naito S. Relationship between respiratory compensation point and anaerobic threshold in patients with heart failure with reduced ejection fraction. Circ J. (2019) 84(1):76–82. doi: 10.1253/circj.CJ-19-0561
35. Fletcher G, Balady G, Amsterdam E, Chaitman B, Eckel R, Fleg J, et al. Exercise standards for testing and training: a statement for healthcare professionals from the American Heart Association. Circulation. (2001) 104(14):1694–740. doi: 10.1161/hc3901.095960
36. van de Vegte Y, Tegegne B, Verweij N, Snieder H, van der Harst P. Genetics and the heart rate response to exercise. Cell Mol Life Sci. (2019) 76(12):2391–409. doi: 10.1007/s00018-019-03079-4
37. Dresing T, Blackstone E, Pashkow F, Snader C, Marwick T, Lauer M. Usefulness of impaired chronotropic response to exercise as a predictor of mortality, independent of the severity of coronary artery disease. Am J Cardiol. (2000) 86(6):602–9. doi: 10.1016/S0002-9149(00)01036-5
38. Azarbal B, Hayes S, Lewin H, Hachamovitch R, Cohen I, Berman D. The incremental prognostic value of percentage of heart rate reserve achieved over myocardial perfusion single-photon emission computed tomography in the prediction of cardiac death and all-cause mortality: superiority over 85% of maximal age-predicted heart rate. J Am Coll Cardiol. (2004) 44(2):423–30. doi: 10.1016/j.jacc.2004.02.060
39. Brubaker P, Kitzman D. Chronotropic incompetence: causes, consequences, and management. Circulation. (2011) 123(9):1010–20. doi: 10.1161/CIRCULATIONAHA.110.940577
Keywords: heart rate, coronary artery disease, anaerobic threshold, respiratory compensatory point, cardiopulmonary exercise test
Citation: Kong Y, Shen R, Xu T, Zhou J, Xia C, Zou T and Wang F (2024) The association of coronary artery disease with heart rate at anaerobic threshold and respiratory compensatory point. Front. Cardiovasc. Med. 11:1442857. doi: 10.3389/fcvm.2024.1442857
Received: 3 June 2024; Accepted: 23 September 2024;
Published: 2 October 2024.
Edited by:
Josip A. Borovac, University Hospital Split, CroatiaReviewed by:
Ziliang Ye, Erasmus Medical Center, NetherlandsYujie Zhou, Capital Medical University, China
Copyright: © 2024 Kong, Shen, Xu, Zhou, Xia, Zou and Wang. This is an open-access article distributed under the terms of the Creative Commons Attribution License (CC BY). The use, distribution or reproduction in other forums is permitted, provided the original author(s) and the copyright owner(s) are credited and that the original publication in this journal is cited, in accordance with accepted academic practice. No use, distribution or reproduction is permitted which does not comply with these terms.
*Correspondence: Fang Wang, bjh_wangfang@163.com; Tong Zou, zoutong2001@163.com
†These authors have contributed equally to this work and share first authorship
‡ORCID:
Tao Xu
orcid.org/0009-0001-4060-6635