- 1Interdepartmental Research Center for Hypertension and Related Conditions, University of Naples, Naples, Italy
- 2Department of Public Health, Federico II University, Naples, Italy
- 3Department of Clinical Medicine and Surgery, Federico II University, Naples, Italy
Anderson–Fabry disease (AFD) is a lysosomal storage disorder, depending on defects in alpha galactosidase A activity, due to a mutation in the galactosidase alpha gene. Cardiovascular involvement represents the leading cause of death in AFD. Cardiac imaging plays a key role in the evaluation and management of AFD patients. Echocardiography is the first-line imaging modality for the identification of the typical features of AFD cardiomyopathy. Advanced echocardiography that allows assessment of myocardial deformation has provided insights into the cardiac functional status of AFD patients. The present review highlights the value and the perspectives of advanced ultrasound imaging in AFD.
Introduction
Anderson–Fabry disease (AFD) is a lipidosis caused by deficient αGLA (α-galactosidase A) enzyme activity due to a mutation in the galactosidase alpha gene leading to progressive lysosomal accumulation of complex sphingolipids in vascular endothelial and smooth-muscle cells throughout the body and in the cells of kidney, nervous system, eyes, and heart (1, 2). Cardiac involvement represents the main cause of impaired quality of life and of reduced life expectancy (3). In the heart, accumulation of globotriaosylceramide (Gb3) affects all cell types, including myocytes, endocardium, valvular fibroblasts, and specific myocardium cardiomyocytes. Imaging represents a key tool in the diagnostic and therapeutic approaches to AFD cardiac manifestations (4, 5). Two-dimensional (2D) transthoracic echocardiographic assessment is the first step in to detail morphologic and functional aspects of heart in AFD, namely: left ventricular (LV) concentric hypertrophy, preserved ejection fraction, disproportionate hypertrophy of papillary muscles and, often, right ventricular (RV) hypertrophy. Advancement in cardiac ultrasound imaging allows to quantify myocardial deformation in the different spatial directions offering an innovative evaluation of LV function. Tissue Doppler strain rate curves and speckle tracking echocardiography (STE) unrevealed an impairment of myocardial function in AFD patients with preserved ejection fraction. Given its angle dependency, tissue Doppler is limited in assessing LV apex. Owing the ability to assess myocardial deformation in all segments of LV walls, STE allows to overcome such limitations. Myocardial deformation measurements by 2D STE have been validated against both sonomicrometry and 2D-tagged cardiac magnetic resonance imaging (6). Further technological advancement of real-time three-dimensional (3D) echocardiography has developed software that tracks the motion of speckles irrespective of their direction and allows to obtain a homogeneous spatial distribution of all three components of the myocardial displacement vector. Myocardial strain can be analyzed from full-volume acquisitions, potentially overcoming the out-of-plane loss of speckles associated with 2D STE analyses. Thus, a series of studies revealing the impairment of LV function caused by AFD flourished during the last two decades. New insights have been provided in subclinical detection of AFD-related abnormalities as well as in disease staging and in prognostication. Given that AFD is a rare disease, most studies offered insights into small cohorts of patients. This paper aims to provide a comprehensive review of current knowledge and of ongoing research into the evaluation of AFD cardiomyopathy with use of advanced echocardiography.
Left ventricular systolic function
The heart in AFD patients presents a phenocopy of hypertrophic cardiomyopathy with preserved LV ejection until the late stages of disease. Strain imaging revealed that the AFD patients may have an impairment of LV systolic function, despite an ejection fraction within the normal range. Studies using tissue Doppler echocardiography demonstrated subclinical LV dysfunction even in early stages of disease (7, 8). Weidemann et al. found out that both peak systolic strain rate and systolic strain were significantly reduced in either the radial or longitudinal direction in 16 AFD patients compared with controls (9). In 2007, the same group described a double peak sign in tissue Doppler strain rate curves in myocardial segments with late gadolinium enhancement by magnetic resonance imaging (10) and demonstrated that a pattern-based analysis was more sensitive and more specific for detecting fibrosis than peak strain (11). Studies by STE showed a decrease in LV longitudinal strain (12–14) involving mainly basal segments (15–17) although apical segments were not completely spared, unlike amyloidosis related cardiomyopathy (15, 18). Moreover, AFD patients with LV hypertrophy were found to have a worse longitudinal function than patients with non-obstructive hypertrophic cardiomyopathy (14). By using the quantitative measurement of myocardial fibrosis with magnetic resonance imaging, Kramer et al, demonstrated an association between the impairment of longitudinal strain and the amount of myocardial replacement fibrosis (19). Interestingly, measuring time-to-peak longitudinal strain unveiled a high prevalence of intraventricular dyssynchrony in AFD patients with LV hypertrophy (20). Cardiac sympathetic denervation has been described in AFD related cardiomyopathy (21–24). It has been found that the presence of denervated areas affects segmental longitudinal strain yielding reduction of global LV function (25). Several studies have highlighted the reduction of LV global longitudinal function before the occurrence of LV hypertrophy, suggesting that myocardial functional impairment is an intrinsic feature of disease and not a consequence of increased LV mass (13, 17, 26, 27). In AFD patients, cardiomyocyte glycosphingolipid storage causes myofibrillolysis and myofilament derangement resulting in a detrimental functional effect (28). A study including a quite large cohort of patients with late- onset cardiac variant showed that AFD patients without LV hypertrophy still had a reduced global longitudinal strain when compared to healthy subjects, despite similar LV mass and morphology (29). It has been suggested that basal longitudinal strain should be considered when screening for cardiac involvement in AFD, particularly in female AFD patients with normal LV wall thickness (17). Reduction in longitudinal strain was found associated with low native T1 in AFD patients without LV hypertrophy (30, 31). Furthermore, in females carrying α-Gal A mutation and without LV hypertrophy, LV global longitudinal strain was impaired in presence of focal myocardial inflammation, identified as focal 18F-luorodeoxyglucose uptake by cardiac positron emission tomography (32).
There are limited data on the impairment of LV circumferential strain in AFD (13, 14, 19, 33). Circumferential strain refers to mid-wall fibers, the same myocardial portion where fibrosis finds its most typical distribution in AFD. Shanks et al. did not find any difference in circumferential function between AFD patients and age- and gender matched healthy subjects (13), while other studies by echocardiography (14, 21, 33) or cardiac magnetic resonance (34) demonstrated that, alongside with impairment in longitudinal function, AFD patients experienced the decrease of global circumferential strain and the loss of base to apex gradient irrespectively of LV geometry (14). Conversely, patients with nonobstructive hypertrophic cardiomyopathy compensated the decrease in longitudinal function with an increase in global circumferential strain and preservation of the base-to-apex gradient (14). Thus, the loss of base to apex gradient seems to be specific to AFD cardiomyopathy and could be caused by the greater impairment of subepicardial fibers, which are mainly responsible for circumferential strain (35).
The data on LV radial strain are even more scarce. Color Doppler myocardial imaging demonstrated an impairment in radial strain rate of posterior wall in AFD patients with LV hypertrophy as well as in female patients with normal LV mass and evidence of late gadolinium enhancement by cardiac magnetic resonance (9, 36). Studies by 2D STE reported conflicting findings (13, 37). While an early study showed normal values of radial strain (13), another study including a larger population demonstrated an early deterioration in LV radial strain, affecting even patients without clear-cut wall hypertrophy (37). Interestingly, global longitudinal strain was significantly associated to LV mass whereas, radial strain was not. However, a recent study by 3D echocardiography has shown an inverse correlation between LV mass and radial strain in 75 AFD patients (51% with LV hypertrophy or concentric remodeling). The use in 3D analysis of a different method for radial strain assessment that was based on volume conservation might account for the different results (38). However, among the various myocardial deformation components, global longitudinal strain has shown the best ability in detecting subclinical LV systolic dysfunction. Figure 1 shows representative examples of longitudinal strain in AFD patients (panel 1). Nevertheless, longitudinal strain is influenced by loading conditions. Myocardial work derived by pressure-strain analysis is a novel non-invasive method to characterize myocardial deformation in relation to afterload conditions (39). Early findings indicate that myocardial work may have an additive value in the functional assessment of AFD cardiomyopathy (40, 41). In the Figure 1, representative examples of myocardial work from AFD patients are shown (panel 2).
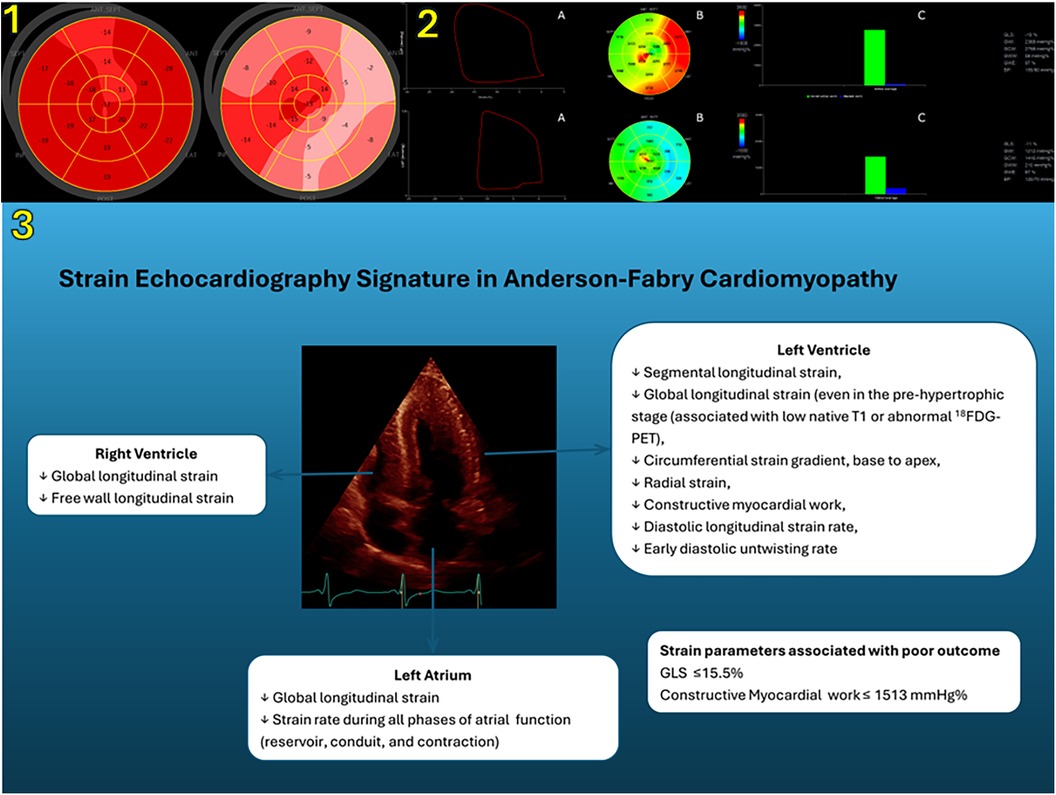
Figure 1. Panel 1. Left ventricular longitudinal strain measurements by means of two-dimensional speckle tracking echocardiography. Bull’s-eye rendering of LV longitudinal strain from a 42-year-old female AFD patient without LV hypertrophy (left) and a 53-year-old male AFD patient with LV hypertrophy (right). Panel 2. (A) Representative example of pressure–strain loops by estimated LV pressure and LV longitudinal strain; (B) Bull’s eye plot showing segmental LV myocardial work index in a 17-segment model; (C) constructive work (green column) and wasted work (blue column) values from the same patients as in the panel 1 (upper, female patient without LV hypertrophy; lower, male patient with LV hypertrophy). Panel 3 shows the role of strain echocardiography in the characterization of cardiac involvement in AFD. LV, left ventricular; AFD, Anderson–Fabry disease; SEPT, septal; LAT, lateral; ANT, anterior; INF, inferior; POST, posterior; LVP, left ventricular pressure; GLS, global longitudinal strain; GWI, global work index; GCW, global constructive work; GWW, global wasted work; GWE, global work efficiency; BP, blood pressure; 18F-FDG PET, 18F-luorodeoxyglucose uptake by cardiac positron emission tomography.
Left ventricular diastolic function
Progressive LV hypertrophy with preserved ejection fraction and diastolic dysfunction have been described as the major echocardiographic features of AFD cardiomyopathy (42). There is a growing awareness of diastolic dysfunction being an early sign of cardiac involvement in AFD. It has been suggested that the tissue Doppler derived diastolic index, namely early diastolic mitral annulus velocities (i.e., e′) could provide satisfactory preclinical evidence for diastolic dysfunction in patients with AFD (7). Yet, this was not found in subsequent studies (8, 29). The diastolic strain rate measured by 2D STE emerged as a sensitive tool in detecting diastolic dysfunction, better than conventional diastolic indices (43). Shanks et al. showed that longitudinal strain rate parameters, particularly those measured during early diastole, identify AFD patients from healthy controls, independent of LV hypertrophy and in a more specific manner than tissue Doppler measurements (13). A recent study confirmed the impairment in diastolic longitudinal strain rate of AFD patients without clear-cut LV wall hypertrophy (44). LV diastolic longitudinal strain rate is attenuated by myocardial fibrosis, a typical feature of AFD cardiomyopathy (45). Similarly, LV diastolic rotational mechanics may be also impaired in AFD patients. Indeed, reduced early diastole untwisting rate has been demonstrated associated to myocardial sympathetic denervation (21).
Left atrial function
Histopathological findings demonstrated the accumulation of Gb3 in the left atrium (LA) of AFD patients supporting atrial myopathy (42, 46, 47). However, few studies have investigated the effects of AFD on LA size and mechanical function. LA acts as a blood reservoir during ventricular systole, as a passive conduit during the passage of blood from the pulmonary veins to the left ventricle during early diastole and as a contractile chamber to increase ventricular filling during atrial systole. Strain and strain rate imaging allows to assess atrial function via the analysis of the cardiac cycle. Boyd et al. used tissue Doppler imaging with a four-point segmental approach to assess LA strain and strain rate and demonstrated that LA systolic strain and early diastolic strain rate were selectively reduced in AFD patients with LV hypertrophy. Interestingly, LA enlargement and reduced atrial compliance were found in the subgroup without LV hypertrophy, despite a normal diastolic function with e′ values like those in controls (48). Almost all studies explored LA function using 2D STE, as the technique allows a complete assessment of endocardial strain. Morris et al. could detect LA myocardial dysfunction in AFD patients, even when LA volume was normal. However, in their study data on conduit function were not reported (12). Notwithstanding, a retrospective study comparing 50 AFD patients with 50 healthy control subjects demonstrated that LA reservoir, conduit, and contractile functions were all affected in AFD patients (49). Saccheri et al. analyzed LA function in AFD patients with LV hypertrophy in comparison with patients with hypertrophic cardiomyopathy and found out that both disorders exhibited a severe functional impairment, although LA volume was lower in AFD (50). Conversely, a lower LA volume and a lower impairment of all three phases of LA mechanics have been detected in AFD patients than in patients with cardiac amyloidosis (51, 52). Several data suggest that differential echocardiographic diagnostic work-up of unclear LV hypertrophy can be improved by integrating LA strain analysis. Frumkin et al. analyzed patients with AFD cardiomyopathy and patients with LV hypertrophy due to other causes and found that LA conduit strain showed the highest diagnostic accuracy to discriminate AFD, superior to the posterolateral strain impairment and papillary muscle hypertrophy pattern (53). Likewise, in a recent study by cardiac magnetic resonance imaging the impairment in LA reservoir strain performed better than the established approach using LV mass index and low native T1 in identifying early disease (54). Although none of the above parameters has so far been validated as independent predictor in large enough cohorts, deformation analysis by means of advanced echocardiography or other cardiac imaging modality has the potential to provide valuable insights into LA functional status of AFD patients. Bradyarrhythmia are common manifestations of cardiac involvement in AFD, often requiring pacemaker implantation. It has been demonstrated that LA reservoir dysfunction can be a useful marker associated to bradyarrhythmia (55). Atrial fibrillation is a possible complication of AFD occurring in about 13% of patients. The risk factors for atrial fibrillation hitherto identified are limited to age, LV hypertrophy and atrial dilatation. Few data suggest an association between the impairment of LA strain parameters with the occurrence of atrial fibrillation and stroke in FD patients (49). However, the role of LA dysfunction as a risk factor for atrial fibrillation needs to be addressed in large studies. Quite common features of central nervous system involvement in AFD are non-specific periventricular and deep white matter lesions along with silent lacunar infarctions of the brain. In a small cohort of AFD patients, Esposito et al. found that LA function expressed as peak atrial longitudinal strain was inversely associated with the presence of non-specific white matter lesions (56).
Right ventricular function
Anatomopathological findings demonstrated that structural changes such as the accumulation of Gb3 take place also in the right ventricle (RV). RV hypertrophy, defined as wall thickness >5 mm, is more frequent with increasing age, and the extent is correlated with the degree of coexisting LV hypertrophy (57–61). When assessed by conventional echocardiography, indices of RV systolic function may be found within the normal range, even when severe RV hypertrophy is present (12, 60). Indeed, only in the late disease stages RV involvement progresses to severe systolic and diastolic RV dysfunction (57). Morris et al. (12) evaluated longitudinal systolic strain peak from the free and septal wall (i.e., RV strain) and just from the free wall of the RV (i.e., RV FW strain) and unveiled systolic dysfunction in 20% of patients (61, 62). Lillo found out that the physiologic difference between the RV-FW strain and the global RV strain was preserved regardless of the presence of overt cardiomyopathy (62). Compared to patients with hypertrophic cardiomyopathy, AFD patients showed worse RV FW longitudinal strain, despite comparable conventional parameters (52, 61). Conversely, a minimal involvement of RV function has been documented in AFD compared to cardiac amyloidosis (51). According to 2D echocardiography, RV involvement seems to be a late phenomenon of the disease as RV strain is preserved in the pre-hypertrophic phase (62). Nevertheless, a pilot study by 3D STE showed an early subclinical functional damage (63). Nevertheless, the putative 3D imaging advantages that derive from the independency from the through-plane phenomenon and the ability to provide real information on volume and wall deformation with no need for geometric assumptions (64), still need to be confirmed in larger patient cohorts.
Advanced echocardiography and prognosis
Identifying patients who are at risk of adverse cardiac outcome may facilitate more evidence-based treatment guidance (65–67). The assessment of LV function by longitudinal strain has become widely adopted, but its prognostic value in AFD remains unclear. Early findings indicated a link between alterations in LV global longitudinal function and symptomatic status and prognosis (12). Interestingly, also basal longitudinal strain reduction was found associated with major adverse cardiovascular events (17). In a cohort of 96 AFD patients, global longitudinal strain showed an incremental prognostic value over clinical factors, LV mass index and diastolic function, during a median follow-up of 5.2 years (40). The prognostic value of LV global longitudinal strain has been confirmed by other studies including one by 3D echocardiography (38, 68, 69). Mechanical dispersion STE has been proposed as an additional risk marker (70). The only study utilizing strain derived myocardial work for the assessment of LV function in AFD suggested a higher accuracy of myocardial work in comparison with global longitudinal strain (GLS) in predicting event free survival has been observed, with constructive myocardial work being the best performing index (40).
Advanced echocardiography and the effects of therapy
While there is a growing acceptance of the role of strain imaging in early detecting of cardiac involvement of AFD and thus, in determining the candidacy to disease- specific therapy, there is a lack of findings regarding its usefulness in assessing the effects of therapy Already in 2003, Weidemann et al. demonstrated a significant improvement in longitudinal and radial strain values by tissue Doppler after 1 year of enzyme replacement therapy (9), whereas the presence of myocardial fibrosis did not benefit from therapy over a period of 3 years (71). A significant decrease of longitudinal systolic strain rate at basal-mid level of LV lateral wall was observed in AFD patients treated prospectively with enzyme replacement therapy for 6 years (72). There are findings suggesting that enzyme replacement therapy may delay the onset of cardiac involvement, thus, supporting the initiation of therapy at an earlier stage of the disease (73). Recently, a significant improvement in apical circumferential strain was observed during enzyme replacement therapy (44) The effects of therapy on LA function have been scarcely investigated. Pichette et al. reported an improvement in LA reservoir strain and in some cases in conduit and contractile strains after 12 months of treatment (49). However, therapy was able to improve LA strain, but not to reduce LA volume (62). A recent study demonstrated no improvement, rather a stabilized LA strain in patients treated with enzyme replacement therapy as well as in those receiving chaperone therapy (74). Finally, therapy seems to have no direct impact on RV morphology and function (59).
Advanced echocardiography: benefits and pitfalls
The diagnosis AFD is based on signs and symptoms suggestive of a systemic disease, family history, an absent or reduced (<5% of normal) leukocyte α-GalA activity level (in men) and is confirmed by genotype testing. Standard and advanced echocardiography are not enough to confirm diagnosis of AFD, but provide essential insights in the functional evaluation AFD, unrevealing myocardial dysfunction in patients with LV hypertrophy and preserved ejection fraction. Once a diagnosis of AFD disease has been established, the presence of abnormal global or segmental strain in an otherwise normal heart may be suggestive of early involvement and should trigger closer clinical follow-up. In the Figure 1, the role of strain echocardiography in identifying the features of heart involvement in AFD is shown (panel 3). When comparing standard and advanced echocardiography to other morphological analysis such as cardiac magnetic resonance, it has to be kept in mind that the information is often additive, more than alternative. Indeed, cardiac magnetic resonance can provide a precise evaluation of heart morphology and tissue characteristics. Native T1 mapping allows early detection of cardiac involvement in a pre-hypertrophic stage and can discriminate between control subjects and AFD patients without LV hypertrophy. Moreover, low myocardial T1 values in pre- hypertrophic stage correlate with reduced global longitudinal strain (30). However, its wide adoption is hampered by the lack of standardized cut-off values for T1 mapping as the analysis is influenced by imaging equipment and protocols. In this perspective, echocardiography has the advantage of being widely spread, less expensive and easily repeatable. Nevertheless, cardiac imaging findings, either by advanced echocardiography or by cardiac magnetic resonance, are not specific nor pathognomonic of AFD. Some feature can help diagnosis: in the setting of LV hypertrophy the presence of a typical pattern of midmyocardial late gadolinium enhancement in the basal to mid inferolateral wall may aid in differential diagnosis (10). Longitudinal strain has proven to be less useful in distinguishing AFD patients from other conditions associated with LV hypertrophy (14, 18, 50–52, 61, 75–80). Loss of base to apex gradient of LV circumferential strain, irrespectively of the increase in LV wall thickness, seems to be specific for AFD (12, 33, 34). Table 1 summarizes myocardial strain characteristics of AFD and of other forms of cardiac hypertrophy such as nonobstructive hypertrophic cardiomyopathy and cardiac amyloidosis.
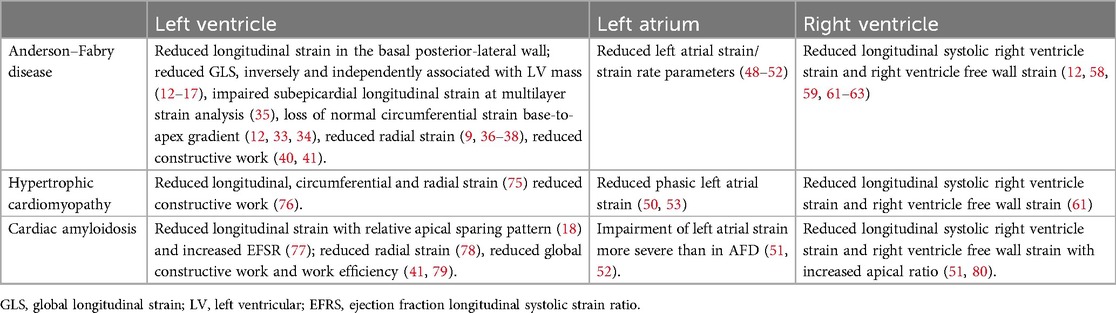
Table 1. Strain echocardiography features of Anderson–Fabry disease and other conditions of left ventricular hypertrophy in adults.
Conclusions
Advanced cardiac imaging has played a crucial role in defining features of the unique cardiac involvement due to AFD. Strain imaging by cardiac ultrasound is involved in many aspects: the initial diagnostic suspicion of AFD in case of evidence of unexplained heart damage, the differential diagnosis with other cardiomyopathies, the early detection of heart involvement in patients with already diagnosed AFD, the decisions regarding the initiation of chaperone or enzyme replacement therapy. Further large studies are warranted to ascertain the prognosticator value of LV longitudinal strain in defining patient risk profile and monitoring evolution of AFD cardiomyopathy. Research should be prompted to verify whether and at what extent advanced echocardiography may provide insights into the impact of disease-specific therapy on the heart of AFD patients.
Author contributions
LS: Conceptualization, Writing – original draft, Writing – review & editing. AB: Writing – original draft. ER: Writing – original draft. AP: Writing – original draft. GI: Conceptualization, Writing – original draft, Writing – review & editing.
Funding
The author(s) declare financial support was received for the research, authorship, and/or publication of this article. This article is based upon work from Italian Ministry of University and Research Enlarged Partnership 8 “A novel publice-private socioeconomic, biomedical and technological solutions for an inclusive Italian ageing society” (Proect number: PE0000015), supported by the Italian National Recovery and Resilience Plan, financed by Next Generation Europe program.
Conflict of interest
The authors declare that the research was conducted in the absence of any commercial or financial relationships that could be construed as a potential conflict of interest.
The author(s) declared that they were an editorial board member of Frontiers, at the time of submission. This had no impact on the peer review process and the final decision.
Publisher's note
All claims expressed in this article are solely those of the authors and do not necessarily represent those of their affiliated organizations, or those of the publisher, the editors and the reviewers. Any product that may be evaluated in this article, or claim that may be made by its manufacturer, is not guaranteed or endorsed by the publisher.
References
1. Zarate YA, Hopkin RJ. Fabry’s disease. Lancet. (2008) 372(9647):1427–35. doi: 10.1016/S0140-6736(08)61589-5
2. Aerts JM, Groener JE, Kuiper S, Donker-Koopman WE, Strijland A, Ottenhoff R, et al. Elevated globotriaosylsphingosine is a hallmark of Fabry disease. Proc Natl Acad Sci U S A. (2008) 105(8):2812–7. doi: 10.1073/pnas.0712309105
3. Patel MR, Cecchi F, Cizmarik M, Kantola I, Linhart A, Nicholls K, et al. Cardiovascular events in patients with fabry disease natural history data from the fabry registry. J Am Coll Cardiol. (2011) 57(9):1093–9. doi: 10.1016/j.jacc.2010.11.018
4. Perry R, Shah R, Saiedi M, Patil S, Ganesan A, Linhart A, et al. The role of cardiac imaging in the diagnosis and management of Anderson–Fabry disease. JACC Cardiovasc Imaging. (2019) 12(7 Pt 1):1230–42; Erratum in: JACC Cardiovasc Imaging. (2019) 12(9):1903. doi: 10.1016/j.jcmg.2018.11.039
5. Augusto JB, Nordin S, Vijapurapu R, Baig S, Bulluck H, Castelletti S, et al. Myocardial edema, myocyte injury, and disease severity in Fabry disease. Circ Cardiovasc Imaging. (2020) 13(3):e010171. doi: 10.1161/CIRCIMAGING.119.010171
6. Amzulescu MS, Langet H, Saloux E, Manrique A, Boileau L, Slimani A, et al. Head-to-head comparison of global and regional two-dimensional speckle tracking strain versus cardiac magnetic resonance tagging in a multicenter validation study. Circ Cardiovasc Imaging. (2017) 10:e006530. doi: 10.1161/CIRCIMAGING.117.006530
7. Pieroni M, Chimenti C, Ricci R, Sale P, Russo MA, Frustaci A. Early detection of Fabry cardiomyopathy by tissue Doppler imaging. Circulation. (2003) 107:1978–84. doi: 10.1161/01.CIR.0000061952.27445.A0
8. Toro R, Perez-Isla L, Doxastaquis G, Barba MA, Gallego AR, Pintos G, et al. Clinical usefulness of tissue Doppler imaging in predicting preclinical Fabry cardiomyopathy. Int J Cardiol. (2009) 132(1):38–44. doi: 10.1016/j.ijcard.2008.04.075
9. Weidemann F, Breunig F, Beer M, Sandstede J, Turschner O, Voelker W, et al. Improvement of cardiac function during enzyme replacement therapy in patients with Fabry disease: a prospective strain rate imaging study. Circulation. (2003) 108(11):1299–301. doi: 10.1161/01.CIR.0000091253.71282.04
10. Moon JC, Sachdev B, Elkington AG, McKenna WJ, Mehta A, Pennell DJ, et al. Gadolinium enhanced cardiovascular magnetic resonance in Anderson–Fabry disease. Evidence for a disease specific abnormality of the myocardial interstitium. Eur Heart J. (2003) 24(23):2151–5. doi: 10.1016/j.ehj.2003.09.017
11. Weidemann F, Niemann M, Herrmann S, Kung M, Störk S, Waller C, et al. A new echocardiographic approach for the detection of non-ischaemic fibrosis in hypertrophic myocardium. Eur Heart J. (2007) 28(24):3020–6. doi: 10.1093/eurheartj/ehm454
12. Morris DA, Blaschke D, Canaan-Kühl S, Krebs A, Knobloch G, Walter TC, et al. Global cardiac alterations detected by speckle-tracking echocardiography in Fabry disease: left ventricular, right ventricular, and left atrial dysfunction are common and linked to worse symptomatic status. Int J Cardiovasc Imaging. (2015) 28:301–13. doi: 10.1007/s10554-014-0551-4
13. Shanks M, Thompson RB, Paterson ID, Putko B, Khan A, Chan A, et al. Systolic and diastolic function assessment in fabry disease patients using speckle-tracking imaging and comparison with conventional echocardiographic measurements. J Am Soc Echocardiogr. (2013) 26(12):1407–14. doi: 10.1016/j.echo.2013.09.005
14. Gruner C, Verocai F, Carasso S, Vannan MA, Jamorski M, Clarke JT, et al. Systolic myocardial mechanics in patients with Anderson–Fabry disease with and without left ventricular hypertrophy and in comparison to nonobstructive hypertrophic cardiomyopathy. Echocardiography. (2012) 29(7):810–7. doi: 10.1111/j.1540-8175.2012.01704.x.
15. Esposito R, Galderisi M, Santoro C, Imbriaco M, Riccio E, Maria Pellegrino A, et al. Prominent longitudinal strain reduction of left ventricular basal segments in treatment-naïve Anderson–Fabry disease patients. Eur Heart J Cardiovasc Imaging. (2019) 20(4):438–45. doi: 10.1093/ehjci/jey108
16. Suwalski P, Klingel K, Landmesser U, Heidecker B. Apical sparing on speckle tracking in Morbus Fabry. Eur Heart J. (2020) 41(36):3486. doi: 10.1093/eurheartj/ehaa517
17. Zada M, Lo Q, Boyd AC, Bradley S, Devine K, Denaro CP, et al. Basal segmental longitudinal strain: a marker of subclinical myocardial involvement in Anderson–Fabry disease. J Am Soc Echocardiogr. (2021) 34(4):405–413.e2. doi: 10.1016/j.echo.2020.11.009
18. Liu D, Hu K, Niemann M, Herrmann S, Cikes M, Störk S, et al. Effect of combined systolic and diastolic functional parameter assessment for differentiation of cardiac amyloidosis from other causes of concentric left ventricular hypertrophy. Circ Cardiovasc Imaging. (2013) 6(6):1066–72. doi: 10.1161/CIRCIMAGING.113.000683
19. Krämer J, Niemann M, Liu D, Hu K, Machann W, Beer M, et al. Two-dimensional speckle tracking as a non-invasive tool for identification of myocardial fibrosis in Fabry disease. Eur Heart J. (2013) 34(21):1587–96. doi: 10.1093/eurheartj/eht098
20. Cianciulli TF, Saccheri MC, Rísolo MA, Lax JA, Méndez RJ, Morita LA, et al. Mechanical dispersion in Fabry disease assessed with speckle tracking echocardiography. Echocardiography. (2020) 37(2):293–301. doi: 10.1111/echo.14592
21. Spinelli L, Pellegrino T, Pisani A, Giudice CA, Riccio E, Imbriaco M, et al. Relationship between left ventricular diastolic function and myocardial sympathetic denervation measured by (123)I-meta-iodobenzylguanidine imaging in Anderson–Fabry disease. Eur J Nucl Med Mol Imaging. (2016) 43(4):729–39. doi: 10.1007/s00259-015-3273-5
22. Yamamoto S, Suzuki H, Sugimura K, Tatebe S, Aoki T, Miura M, et al. Focal reduction in cardiac 123I-metaiodobenzylguanidine uptake in patients with Anderson–Fabry disease. Circ J. (2016) 80(12):2550–1. doi: 10.1253/circj.CJ-16-0690
23. Imbriaco M, Pellegrino T, Piscopo V, Petretta M, Ponsiglione A, Nappi C, et al. Cardiac sympathetic neuronal damage precedes myocardial fibrosis in patients with Anderson–Fabry disease. Eur J Nucl Med Mol Imaging. (2017) 44(13):2266–73. doi: 10.1007/s00259-017-3778-1
24. Gatterer C, Wollenweber T, Pichler V, Vraka C, Sunder-Plassmann G, Lenz M, et al. Detection of sympathetic denervation defects in Fabry disease by hybrid [11C]meta-hydroxyephedrine positron emission tomography and cardiac magnetic resonance. J Nucl Cardiol. (2023) 30(5):1810–21. doi: 10.1007/s12350-023-03205-7
25. Spinelli L, Imbriaco M, Giugliano G, Nappi C, Gaudieri V, Riccio E, et al. Focal reduction in left ventricular 123I-metaiodobenzylguanidine uptake and impairment in systolic function in patients with Anderson–Fabry disease. J Nucl Cardiol. (2021) 28(2):641–9. doi: 10.1007/s12350-019-01734-8
26. Saccheri MC, Cianciulli TF, Lax JA, Gagliardi JA, Cáceres GL, Quarin AE, et al. Two-dimensional speckle tracking echocardiography for early detection of myocardial damage in young patients with Fabry disease. Echocardiography. (2013) 30(9):1069–77. doi: 10.1111/echo.12216
27. Costanzo L, Buccheri S, Capranzano P, Di Pino L, Curatolo G, Rodolico M, et al. Early cardiovascular remodelling in Fabry disease. J Inherit Metab Dis. (2014) 37(1):109–16. doi: 10.1007/s10545-013-9607-1
28. Chimenti C, Hamdani N, Boontje NM, DeCobelli F, Esposito A, Bronzwaer JG, et al. Myofilament degradation and dysfunction of human cardiomyocytes in Fabry disease. Am J Pathol. (2008) 172(6):1482–90. doi: 10.2353/ajpath.2008.070576
29. Lu DY, Huang WM, Wang WT, Hung SC, Sung SH, Chen CH, et al. Reduced global longitudinal strain as a marker for early detection of Fabry cardiomyopathy. Eur Heart J Cardiovasc Imaging. (2022) 23(4):487–95. doi: 10.1093/ehjci/jeab214
30. Vijapurapu R, Nordin S, Baig S, Liu B, Rosmini S, Augusto J, et al. Global longitudinal strain, myocardial storage and hypertrophy in Fabry disease. Heart. (2019) 105(6):470–6. doi: 10.1136/heartjnl-2018-313699
31. Augusto JB, Johner N, Shah D, Nordin S, Knott KD, Rosmini S, et al. The myocardial phenotype of Fabry disease pre-hypertrophy and pre-detectable storage. Eur Heart J Cardiovasc Imaging. (2021) 22(7):790–9. doi: 10.1093/ehjci/jeaa101
32. Spinelli L, Imbriaco M, Nappi C, Nicolai E, Giugliano G, Ponsiglione A, et al. Early cardiac involvement affects left ventricular longitudinal function in females carrying α-galactosidase a mutation: role of hybrid positron emission tomography and magnetic resonance imaging and speckle-tracking echocardiography. Circ Cardiovasc Imaging. (2018) 11(4):e007019. doi: 10.1161/CIRCIMAGING.117.007019
33. Labombarda F, Saloux E, Milesi G, Bienvenu B. Loss of base-to-apex circumferential strain gradient: a specific pattern of Fabry cardiomyopathy? Echocardiography. (2017) 34(4):504–10. doi: 10.1111/echo.13496
34. Mathur S, Dreisbach JG, Karur GR, Iwanochko RM, Morel CF, Wasim S, et al. Loss of base-to-apex circumferential strain gradient assessed by cardiovascular magnetic resonance in Fabry disease: relationship to T1 mapping, late gadolinium enhancement and hypertrophy. J Cardiovasc Magn Reson. (2019) 21(1):45. doi: 10.1186/s12968-019-0557-0
35. Esposito R, Santoro C, Sorrentino R, Riccio E, Citro R, Buonauro A, et al. Layer-specific longitudinal strain in Anderson–Fabry disease at diagnosis: a speckle tracking echocardiography analysis. Echocardiography. (2019) 36(7):1273–81. doi: 10.1111/echo.14399
36. Niemann M, Herrmann S, Hu K, Breunig F, Strotmann J, Beer M, et al. Differences in Fabry cardiomyopathy between female and male patients: consequences for diagnostic assessment. JACC Cardiovasc Imaging. (2011) 4(6):592–601. doi: 10.1016/j.jcmg.2011.01.020
37. Spinelli L, Giugliano G, Imbriaco M, Esposito G, Nappi C, Riccio E, et al. Left ventricular radial strain impairment precedes hypertrophy in Anderson–Fabry disease. Int J Cardiovasc Imaging. (2020) 36:1465–76. doi: 10.1007/s10554-020-01847-z
38. Marek J, Chocholová B, Rob D, Paleček T, Mašek M, Dostálová G, et al. Three-dimensional echocardiographic left ventricular strain analysis in Fabry disease: correlation with heart failure severity, myocardial scar, and impact on long-term prognosis. Eur Heart J Cardiovasc Imaging. (2023) 24(12):1629–37. doi: 10.1093/ehjci/jead121
39. Russell K, Eriksen M, Aaberge L, Wilhelmsen N, Skulstad H, Remme EW, et al. A novel clinical method for quantification of regional left ventricular pressure-strain loop area: a non-invasive index of myocardial work. Eur Heart J. (2012) 33(6):724–33. doi: 10.1093/eurheartj/ehs016
40. Spinelli L, Giugliano G, Pisani A, Imbriaco M, Riccio E, Russo C, et al. Does left ventricular function predict cardiac outcome in Anderson–Fabry disease? Int J Cardiovasc Imaging. (2021) 37(4):1225–36. doi: 10.1007/s10554-020-02105-y
41. Fan J, Ma C, Wang H, Zhou B. The value of myocardial work in patients with left ventricular hypertrophy. Int J Cardiovasc Imaging. (2023) 39(6):1105–13. doi: 10.1007/s10554-023-02818-w
42. Linhart A, Kampmann C, Zamorano JL, Sunder-Plassmann G, Beck M, Mehta A, et al. Cardiac manifestations of Anderson–Fabry disease: results from the international Fabry outcome survey. Eur Heart J. (2007) 28(10):1228–35. doi: 10.1093/eurheartj/ehm153.
43. Wang J, Khoury DS, Thohan V, Torre-Amione G, Nagueh SF. Global diastolic strain rate for the assessment of left ventricular relaxation and filling pressures. Circulation. (2007) 115(11):1376–83. doi: 10.1161/CIRCULATIONAHA.106.662882
44. Huang S, Wang J, Zhang W, Gao F, Chen Y, Shui W, et al. Clinical study of left ventricular structure and function in patients with Anderson–Fabry disease before and after enzyme replacement therapy. J Clin Ultrasound. (2024) 52(1):20–9. doi: 10.1002/jcu.23592
45. Liu D, Oder D, Salinger T, Hu K, Müntze J, Weidemann F, et al. Association and diagnostic utility of diastolic dysfunction and myocardial fibrosis in patients with Fabry disease. Open Heart. (2018) 5(2):e000803. doi: 10.1136/openhrt-2018-000803
46. Sheppard MN, Cane P, Florio R, Kavantzas N, Close L, Shah J, et al. A detailed pathologic examination of heart tissue from three older patients with Anderson–Fabry disease on enzyme replacement therapy. Cardiovasc Pathol. (2010) 19(5):293–301. doi: 10.1016/j.carpath.2009.05.003
47. Chimenti C, Russo MA, Frustaci A. Atrial biopsy evidence of Fabry disease causing lone atrial fibrillation. Heart. (2010) 96(21):1782–3. doi: 10.1136/hrt.2010.196162
48. Boyd AC, Lo Q, Devine K, Tchan MC, Sillence DO, Sadick N, et al. Left atrial enlargement and reduced atrial compliance occurs early in Fabry cardiomyopathy. J Am Soc Echocardiogr. (2013) 26(12):1415–23. doi: 10.1016/j.echo.2013.08.024
49. Pichette M, Serri K, Pagé M, Di LZ, Bichet DG, Poulin F. Impaired left atrial function in Fabry disease: a longitudinal speckle-tracking echocardiography study. J Am Soc Echocardiogr. (2017) 30(2):170–179.e2. doi: 10.1016/j.echo.2016.10.014
50. Saccheri MC, Cianciulli TF, Challapa Licidio W, Lax JA, Beck MA, Morita LA, et al. Comparison of left atrial size and function in hypertrophic cardiomyopathy and in Fabry disease with left ventricular hypertrophy. Echocardiography. (2018) 35(5):643–50. doi: 10.1111/echo.13829
51. Mattig I, Steudel T, Klingel K, Barzen G, Frumkin D, Spethmann S, et al. Right heart and left atrial strain to differentiate cardiac amyloidosis and Fabry disease. Sci Rep. (2024) 14(1):2445. doi: 10.1038/s41598-024-52890-y
52. Meucci MC, Lillo R, Mango F, Marsilia M, Iannaccone G, Tusa F, et al. Left atrial structural and functional remodelling in Fabry disease and cardiac amyloidosis: a comparative analysis. Int J Cardiol. (2024) 19:131891. doi: 10.1016/j.ijcard.2024.131891
53. Frumkin D, Mattig I, Laule N, Al Daas M, Canaan-Kuhl S, Knebel F, et al. Comparative analysis of phasic left atrial strain and left ventricular posterolateral strain pattern to discriminate Fabry cardiomyopathy from other forms of left ventricular hypertrophy. Echocardiography. (2021) 38(11):1870–8. doi: 10.1111/echo.15224
54. Halfmann MC, Altmann S, Schoepf UJ, Reichardt C, Hennermann JB, Kreitner KF, et al. Left atrial strain correlates with severity of cardiac involvement in Anderson–Fabry disease. Eur Radiol. (2023) 33(3):2039–51. doi: 10.1007/s00330-022-09183-7
55. Di LZ, Pichette M, Nadeau R, Bichet DG, Poulin F. Severe bradyarrhythmia linked to left atrial dysfunction in Fabry disease-A cross-sectional study. Clin Cardiol. (2018) 41(9):1207–13. doi: 10.1002/clc.23019
56. Esposito R, Russo C, Santoro C, Cocozza S, Riccio E, Sorrentino R, et al. Association between left atrial deformation and brain involvement in patients with Anderson–Fabry disease at diagnosis. J Clin Med. (2020) 9(9):2741. doi: 10.3390/jcm9092741
57. Kampmann C, Baehner FA, Whybra C, Bajbouj M, Baron K, Knuf M, et al. The right ventricle in Fabry disease. Acta Paediatr Suppl. (2005) 94(447):15–8; discussion 9–10. doi: 10.1111/j.1651-2227.2005.tb02104.x
58. Palecek T, Dostalova G, Kuchynka P, Karetova D, Bultas J, Elleder M, et al. Right ventricular involvement in Fabry disease. J Am Soc Echocardiogr. (2008) 21(11):1265–8. doi: 10.1016/j.echo.2008.09.002
59. Niemann M, Breunig F, Beer M, Herrmann S, Strotmann J, Hu K, et al. The right ventricle in Fabry disease: natural history and impact of enzyme replacement therapy. Heart. (2010) 96(23):1915–9. doi: 10.1136/hrt.2010.204586
60. Graziani F, Laurito M, Pieroni M, Pennestrì F, Lanza GA, Coluccia V, et al. Right ventricular hypertrophy, systolic function, and disease severity in Anderson–Fabry disease: an echocardiographic study. J Am Soc Echocardiogr. (2017) 30(3):282–91. doi: 10.1016/j.echo.2016.11.014
61. Militaru S, Jurcuț R, Adam R, Roşca M, Ginghina C, Popescu BA. Echocardiographic features of Fabry cardiomyopathy. Comparison with hypertrophy-matched sarcomeric hypertrophic cardiomyopathy. Echocardiography. (2019) 36(11):2041–9. doi: 10.1111/echo.14508
62. Lillo R, Graziani F, Panaioli E, Mencarelli E, Pieroni M, Camporeale A, et al. Right ventricular strain in Anderson–Fabry disease. Int J Cardiol. (2021) 330:84–90. doi: 10.1016/j.ijcard.2021.02.038.
63. Pucci M, Iadevaia V, Gammaldi V, Iervolino A, Capece LM, Sciascia D, et al. Right ventricular myocardial involvement in Anderson–Fabry disease at diagnosis: evaluation with three-dimensional strain imaging. Life. (2023) 13(7):1571. doi: 10.3390/life13071571
64. Smith BC, Dobson G, Dawson D, Charalampopoulos A, Grapsa J, Nihoyannopoulos P. Three-dimensional speckle tracking of the right ventricle: toward optimal quantification of right ventricular dysfunction in pulmonary hypertension. J Am Coll Cardiol. (2014) 64(1):41–51. doi: 10.1016/j.jacc.2014.01.084
65. Patel V, O'Mahony C, Hughes D, Rahman MS, Coats C, Murphy E, et al. Clinical and genetic predictors of major cardiac events in patients with Anderson–Fabry disease. Heart. (2015) 101(12):961–6. doi: 10.1136/heartjnl-2014-306782
66. Krämer J, Bijnens B, Störk S, Ritter CO, Liu D, Ertl G, et al. Left ventricular geometry and blood pressure as predictors of adverse progression of fabry cardiomyopathy. PLoS One. (2015) 10(11):e0140627. doi: 10.1371/journal.pone.0140627
67. Meucci MC, Lillo R, Del Franco A, Monda E, Iannaccone G, Baldassarre R, et al. Prognostic implications of the extent of cardiac damage in patients with Fabry disease. J Am Coll Cardiol. (2023) 82(15):1524–34. doi: 10.1016/j.jacc.2023.07.026
68. Rob D, Marek J, Dostalova G, Linhart A. Heart failure in Fabry disease revisited: application of current heart failure guidelines and recommendations. ESC Heart Fail. (2022) 9(6):4043–52. doi: 10.1002/ehf2.14091
69. Chang HC, Kuo L, Sung SH, Niu DM, Yu WC. Prognostic implications of left ventricular hypertrophy and mechanical function in Fabry disease: a longitudinal cohort study. J Am Soc Echocardiogr. (2024) 2:S0894-7317(24)00200-1. doi: 10.1016/j.echo.2024.04.010
70. Faro DC, Losi V, Rodolico MS, Licciardi S, Monte IP. Speckle tracking echocardiography-derived parameters as new prognostic markers in hypertrophic cardiomyopathies. Eur Heart J Open. (2023) 3(2):oead014. doi: 10.1093/ehjopen/oead014
71. Weidemann F, Niemann M, Breunig F, Herrmann S, Beer M, Störk S, et al. Long-term effects of enzyme replacement therapy on fabry cardiomyopathy: evidence for a better outcome with early treatment. Circulation. (2009) 119(4):524–9. doi: 10.1161/CIRCULATIONAHA.108.794529
72. Weidemann F, Niemann M, Störk S, Breunig F, Beer M, Sommer C, et al. Long-term outcome of enzyme-replacement therapy in advanced Fabry disease: evidence for disease progression towards serious complications. J Intern Med. (2013) 274(4):331–41. doi: 10.1111/joim.12077
73. Zamorano J, Serra V, Pérez de Isla L, Feltes G, Calli A, Barbado FJ, et al. Usefulness of tissue Doppler on early detection of cardiac disease in Fabry patients and potential role of enzyme replacement therapy (ERT) for avoiding progression of disease. Eur J Echocardiogr. (2011) 12(9):671–7. doi: 10.1093/ejechocard/jer109
74. Pogoda C, Brand SM, Duning T, Schmidt-Pogoda A, Sindermann J, Lenders M, et al. Impact of enzyme replacement therapy and migalastat on left atrial strain and cardiomyopathy in patients with Fabry disease. Front Cardiovasc Med. (2023) 10:1223635. doi: 10.3389/fcvm.2023.1223635
75. Serri K, Reant P, Lafitte M, Berhouet M, Le Bouffos V, Roudaut R, et al. Global and regional myocardial function quantification by two-dimensional strain: application in hypertrophic cardiomyopathy. J Am Coll Cardiol. (2006) 47(6):1175–81. doi: 10.1016/j.jacc.2005.10.061
76. Galli E, Vitel E, Schnell F, Le Rolle V, Hubert A, Lederlin M, et al. Myocardial constructive work is impaired in hypertrophic cardiomyopathy and predicts left ventricular fibrosis. Echocardiography. (2019) 36(1):74–82. doi: 10.1111/echo.14210
77. Pagourelias ED, Mirea O, Duchenne J, Van Cleemput J, Delforge M, Bogaert J, et al. Echo parameters for differential diagnosis in cardiac amyloidosis: a head-to-head comparison of deformation and non-deformation parameters. Circ Cardiovasc Imaging. (2017) 10(3):e005588. doi: 10.1161/CIRCIMAGING.116.005588
78. Lo Q, Haluska B, Chia EM, Lin MW, Richards D, Marwick T, et al. Alterations in regional myocardial deformation assessed by strain imaging in cardiac amyloidosis. Echocardiography. (2016) 33(12):1844–53. doi: 10.1111/echo.13355
79. Clemmensen TS, Eiskjær H, Mikkelsen F, Granstam SO, Flachskampf FA, Sørensen J, et al. Left ventricular pressure-strain-derived myocardial work at rest and during exercise in patients with cardiac amyloidosis. J Am Soc Echocardiogr. (2020) 33(5):573–82. doi: 10.1016/j.echo.2019.11.018
80. Moñivas Palomero V, Durante-Lopez A, Sanabria MT, Cubero JS, González-Mirelis J, Lopez-Ibor JV, et al. Role of right ventricular strain measured by two-dimensional echocardiography in the diagnosis of cardiac amyloidosis. J Am Soc Echocardiogr. (2019) 32(7):845–853.e1. doi: 10.1016/j.echo.2019.03.005
Keywords: Anderson–Fabry disease, cardiac function, myocardial strain, speckle-tracking echocardiography, tissue doppler imaging
Citation: Spinelli L, Bianco A, Riccio E, Pisani A and Iaccarino G (2024) Cardiac involvement in Anderson–Fabry disease. The role of advanced echocardiography. Front. Cardiovasc. Med. 11:1440636. doi: 10.3389/fcvm.2024.1440636
Received: 29 May 2024; Accepted: 19 August 2024;
Published: 2 September 2024.
Edited by:
Maria Concetta Pastore, University of Siena, ItalyReviewed by:
Hiroaki Kitaoika, Kōchi University, JapanCopyright: © 2024 Spinelli, Bianco, Riccio, Pisani and Iaccarino. This is an open-access article distributed under the terms of the Creative Commons Attribution License (CC BY). The use, distribution or reproduction in other forums is permitted, provided the original author(s) and the copyright owner(s) are credited and that the original publication in this journal is cited, in accordance with accepted academic practice. No use, distribution or reproduction is permitted which does not comply with these terms.
*Correspondence: Letizia Spinelli, letspine@unina.it