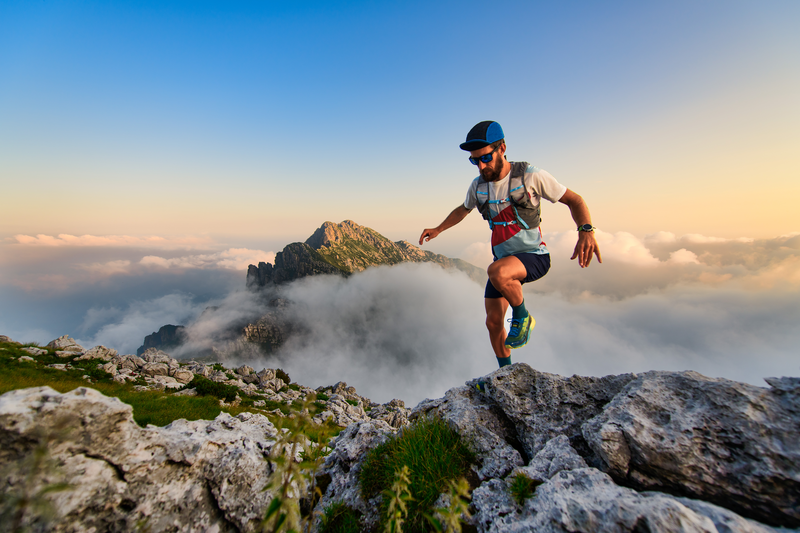
95% of researchers rate our articles as excellent or good
Learn more about the work of our research integrity team to safeguard the quality of each article we publish.
Find out more
REVIEW article
Front. Cardiovasc. Med. , 06 September 2024
Sec. General Cardiovascular Medicine
Volume 11 - 2024 | https://doi.org/10.3389/fcvm.2024.1439402
This article is part of the Research Topic The Right Heart: A Key Target for Cardiovascular Medicine View all 5 articles
Chronic Thromboembolic Pulmonary Hypertension (CTEPH) presents a significant diagnostic challenge due to its complex and often nonspecific clinical manifestations. This review outlines a comprehensive approach to the diagnostic assessment of CTEPH, emphasizing the importance of a high index of suspicion in patients with unexplained dyspnea or persistent symptoms post-acute pulmonary embolism. We discuss the pivotal role of multimodal imaging, including echocardiography, ventilation/perfusion scans, CT pulmonary angiography, and magnetic resonance imaging, in the identification and confirmation of CTEPH. Furthermore, the review highlights the essential function of right heart catheterization in validating the hemodynamic parameters indicative of CTEPH, establishing its definitive diagnosis. Advances in diagnostic technologies and the integration of a multidisciplinary approach are critical for the timely and accurate diagnosis of CTEPH, facilitating early therapeutic intervention and improving patient outcomes. This manuscript aims to equip clinicians with the knowledge and tools necessary for the efficient diagnostic workflow of CTEPH, promoting awareness and understanding of this potentially treatable cause of pulmonary hypertension.
Pulmonary hypertension (PH) is defined by an elevated mean pulmonary artery pressure (mPAP) measured via right-heart catheterization (RHC) and can arise from various diseases affecting pulmonary circulation (1). It is often associated with cardiovascular and respiratory disorders, driven by cellular proliferation and fibrosis in small pulmonary arteries, leading to increased pulmonary vascular resistance (PVR) and, ultimately, right heart failure, which is the main cause of morbidity and mortality in PAH (2). Managing PH requires a multidisciplinary approach, emphasizing collaboration between patients and healthcare providers (3).
Mild elevations in pulmonary arterial pressure are significant predictors of mortality, underscoring the need for early detection and management of PH (4).
Chronic thromboembolic pulmonary hypertension (CTEPH) is a severe form of PH, characterized by intraluminal thrombosis, fibrous webs, and obliteration of pulmonary arteries (5). The exact molecular triggers for the extensive remodeling and fibrosis in CTEPH are unknown. It is thought to result from unresolved thromboembolic material in the pulmonary arteries, where low oxygen tension promotes gene transcription involved in coagulation, angiogenesis, inflammation, and fibrosis. This condition is marked by a proliferative and inflammatory cellular environment, leading to fibrosis, scar formation, and occlusive lesions (6). Risk stratification is crucial in managing these patients (7).
This review will discuss the basic mechanisms of pulmonary hypertensive diseases, delve into the current understanding of CTEPH pathobiology, and outline diagnostic tools and management strategies to achieve optimal patient outcomes.
In 2009, Kovacs et al. analyzed RHC data from healthy individuals to establish normal values for mean pulmonary artery pressure (mPAP) at rest and during exercise. They reviewed data from 1,187 normal subjects across 47 studies and found the resting mPAP to be 14.0 ± 3.3 mmHg, a figure consistent across sex and ethnicity, with minimal influence from age and posture. A resting mPAP greater than 20 mmHg—derived from adding two standard deviations—was identified as exceeding the normal upper limit (above the 97.5th percentile) and correlated with an elevated risk of all-cause mortality. Exercise-induced mPAP often surpasses 30 mmHg, especially in older adults, complicating the establishment of standard exercise mPAP values (3, 4, 8, 9). The ERS Task Force preliminarily defines exercise PH as mPAP exceeding 30 mmHg with total pulmonary resistance (TPR) over 3 Wood units (WU) at peak exercise, alternatively suggesting an mPAP/cardiac output (CO) slope above 3 WU as a threshold. The slopes of mPAP/CO and pulmonary artery wedge pressure (PAWP)/CO are critical for evaluating pulmonary circulation during exercise, showing strong age dependency and consistency across different workloads. A PAWP/CO slope exceeding 2 WU is linked to adverse cardiovascular outcomes and helps differentiate pre- from post-capillary causes of exercise PH (10). Elevated mPAP in certain physiological states does not alone indicate pulmonary vascular disease. PVR is crucial for prognosis and clinical decision-making in pulmonary arterial hypertension (PAH), with data indicating the upper normal limit and lowest prognostically relevant PVR threshold as approximately 2 WU (11–13).
The 2022 ESC/ERS Guidelines for diagnosing and treating pulmonary hypertension classify PH into three primary types based on mPAP, PAWP, and PVR values:
• Pre-capillary pulmonary hypertension: mPAP >20 mmHg, PAWP <15 mmHg, PVR >2 WU.
• Post-capillary pulmonary hypertension (IpcPH): mPAP >20 mmHg, PAWP >15 mmHg, PVR <2 WU.
• Combined pulmonary hypertension (CpcPH): mPAP >20 mmHg, PAWP >15 mmHg, PVR >2 WU.
Additionally, exercise PH and unclassified PH are acknowledged, with the latter encompassing patients with mPAP >20 mmHg, PVR <2 WU, and PAWP <15 mmHg, often associated with increased pulmonary blood flow and conditions like congenital heart disease, liver disease, or lung disease, necessitating further etiological study and clinical follow-up (3).
PH is categorized into five groups based on pathophysiological, clinical, and therapeutic aspects:
• Group 1: Pulmonary arterial hypertension (PAH)
• Group 2: PH due to left-sided heart disease
• Group 3: PH due to lung diseases or hypoxia
• Group 4: PH associated with pulmonary artery obstruction (could be due to CTEPH, pulmonary tumor thrombotic microangiopathy or other causes of pulmonary artery obstructions, for instance sarcomas [high or intermediate grade or angiosarcoma], other malignant tumours [e.g., renal carcinoma, uterine carcinoma, germ-cell tumours of the testis], non-malignant tumours (e.g., uterine leiomyoma), arteritis without connective tissue disease, congenital pulmonary arterial stenoses, and hydatidosis) (14)
• Group 5: PH with unclear or multifactorial mechanisms
Regardless of the underlying cause, the development of PH is linked to deteriorating symptoms and heightened mortality risk (14).
Group 1 pulmonary arterial hypertension (PAH) includes:
• Idiopathic PAH
• Heritable PAH
• Drug- and toxin-induced PAH
• PAH associated with:
○ Connective tissue diseases
○ HIV infection
○ Portal hypertension
○ Congenital heart diseases
○ Schistosomiasis
○ Chronic hemolytic anemia
This classification follows the ESC/ERS guidelines, ensuring comprehensive coverage of the various causes of PAH (Supplementary Table 1).
Group 2 encompasses postcapillary PH, either isolated or combined with a precapillary component, commonly seen in heart failure with either preserved or reduced ejection fraction, affecting over 50% of such patients (15, 16). Both patient groups face a doubled mortality risk associated with right ventricular dysfunction. Likewise, 50%–70% of severe aortic stenosis patients develop PH, doubling mortality risk (17, 18). Similarly, PH is observed in 60%–70% of patients with severe, symptomatic mitral valve disease, with prevalence increasing alongside the severity of left-sided valvular diseases (19).
Patients with heart failure and preserved ejection fraction (HFpEF), primarily presenting exertional dyspnea, may exhibit normal filling pressures at rest but significantly elevated pressures during light exercise (20). This population, often elderly, shows a decrease in cardiac output during exercise due to reduced ventricular compliance and relaxation with age. Exercise stress testing can reveal early HFpEF signs, even with normal resting pulmonary capillary wedge pressure (PCWP) values (<15 mm Hg). Notably, 30% of healthy individuals over 60 years old show PCWP values above 25 mmHg during exercise tests, suggesting the potential benefit of age-adjusting PCWP diagnostic thresholds for improved diagnostic accuracy (21).
For patients with isolated post-capillary PH due to vascular congestion from left heart disease, early interventions like stabilizing intravascular volume or addressing the primary defect can often reverse PH before significant vascular remodeling and increased PVR occur (22). This underlines the critical need for strategies emphasizing early detection and diagnosis of PH to prevent irreversible vascular changes that drive elevated mPAP.
In Group 3, PH is typically mild but significantly impacts patients by exacerbating symptoms, diminishing exercise capacity, increasing hospital admissions, and elevating mortality compared to those without PH (14).
Group 4 focuses on CTEPH, a condition characterized by symptoms arising from the obstruction of pulmonary arteries by chronic fibrotic clots. The persistence of these thrombi and subsequent microvascular arteriopathy increase pulmonary vascular resistance, leading to PH and right heart failure. Uniquely, CTEPH is potentially curable through pulmonary endarterectomy (PEA). Diagnosis requires right heart catheterization to confirm:
1. mPAP >20 mmHg, PAWP ≤15 mmHg at rest, and PVR >2 WU;
2. Positive lung imaging findings, such as mismatches on ventilation/perfusion (V/Q) scintigraphy, significant perfusion defects on single-photon emission computed tomography (SPECT), pulmonary vascular abnormalities on CT/MRI, or pulmonary angiography indicating chronic occlusions;
3. Confirmation of these conditions after a minimum of 3 months on effective anticoagulation to distinguish from subacute PE (3, 23–26).
CTEPH and Chronic Thromboembolic Disease (CTED) are terms distinguishing symptomatic individuals with chronic pulmonary artery occlusions based on the presence or absence of PH at rest (24). CTED describes patients with mPAP <25 mmHg, persistent vascular obstructions, exercise intolerance, and significant symptom and quality of life impact, lacking PH at rest. Despite similar symptoms to CTEPH, CTED patients can receive similar surgical or interventional treatments. Their exercise limitation stems from exercise-induced PH and dead space ventilation (27). The 2022 guidelines’ new mPAP and PVR criteria have led to reclassification of some CTED patients as CTEPH, prompting the term “chronic thromboembolic pulmonary disease” (CTEPD) to encompass symptomatic patients with perfusion defects and chronic clot signs, regardless of rest PH (24). The transition of CTEPD patients to CTEPH, and factors influencing their prognosis, remain areas of investigation (25, 28).
Group 5 includes diseases frequently associated with PH due to diverse and complex mechanisms, encompassing conditions like chronic renal failure, sarcoidosis, and more, characterized by their varied pathophysiological origins (14).
For exercise-induced PAH, the mPAP/CO and PAWP/CO slopes are crucial, offering insight regardless of workload but demonstrating strong age dependency. A mPAP/CO slope >3 mmHg/L/min between rest and exercise is essential for diagnosis, with higher slopes indicating worse survival across cardiopulmonary conditions. A PAWP/CO slope >2 WU is linked to cardiovascular risks and helps distinguish between pre- and post-capillary exercise PH causes (10) (Figure 1).
Figure 1. Prevalence e therapeutic strategies of the five the groups of PH according to clinical classification (3).
The prevalence of PAH, categorized as Group 1 PH, is approximately 25 cases per 1 million population, with an annual incidence of around five cases per 1 million population (29, 30). The median age at diagnosis in clinical trials and registries is about 53 years (31).
For Group 4, studies show that 1.0%–8.8% of patients who survive an acute pulmonary embolism (PE) may develop CTEPH, with an incidence of about 3% according to Chausheva et al. (5). Annual incidence rates reported by registries in Spain and the UK are 0.9 and 1.75 per million, respectively (32, 33). German data indicated an incidence of 4.0 per million adults per year (14), increasing to 5.7 in 2016, while France reports 5–6 cases per million adults (34). Registry data vary, indicating a CTEPH incidence and prevalence of 2–6 and 26–38 cases per million adults, respectively (35–37). The rising number of diagnosed CTEPH cases is attributed to better disease understanding and active screening, especially in patients remaining symptomatic post-PE or at risk for CTEPH (24, 34, 38). The global prevalence and incidence of CTEPH remain uncertain, necessitating further research for precise definitions (Figure 1) (28).
In Europe and the USA, the average incidence of CTEPH is around 4%, contrasting with up to 14% in Japan (15, 39), where the rate of PE is significantly lower. In Europe, the disease affects both genders equally, while in Japan, it shows a female preponderance (5). The European CTEPH registry indicates women have a lower prevalence of certain cardiovascular risk factors but a higher incidence of obesity, cancer, and thyroid diseases (26). Notably, 50%–75% of CTEPH patients have a documented history of acute PE, a figure that drops to 15% in Japanese patients, suggesting a distinct CTEPH phenotype (24).
All meta-analyses report a CTEPH incidence of 2.7%–3%, with approximately 30% of patients developing CTED, which is symptomatic residual vasculopathy without PH (23, 34, 35). The FOCUS trial specifically reported that post-PE impairment incidence is at 16%, with CTEPH occurring in 2.3% of patients (40). It was also reported that up to 20% of patients post-PE may experience some form of impairment, underscoring the need for vigilant monitoring and active screening in these patients to manage and mitigate long-term complications effectively (40).
Risk factors for CTEPH include a history of venous thromboembolism (VTE), particularly recurrent VTE, elevated pulmonary artery pressure post-PE, history of malignancy, splenectomy, staphylococcal infection, non-O blood groups, anti-phospholipid antibodies, lupus anticoagulant, and permanent intravascular devices. Additional risk factors include inflammatory bowel disease, polycythemia vera, essential thrombocythemia, hypothyroidism, elevated levels of factor VIII and von Willebrand factor, fibrinogen polymorphism and, possibly, factor V Leiden although the role of the latter is still controversial (3, 24, 40–42). Demographic characteristics and the presence of chronic inflammatory/autoimmune diseases or hematological disorders also play a significant role in the development of the disease (43, 44) (Table 1).
CTEPH is marked by a prothrombotic state and abnormal fibrinogen molecules resistant to physiological thrombolysis, indicating an impaired resolution of the initial embolic event. Splenectomy is particularly associated with distal CTEPH types, complicating PEA procedures (44). The history of infected ventriculoatrial shunts and splenectomy is linked not only to CTEPH but also to significant inflammation and an increased risk of VTE (41). Additionally, CTEPH may be complicated by a postcapillary disease component, underscoring the importance of recognizing clinical risk factors for PH associated with left heart disease (47).
CTEPH is marked by vascular remodeling in the lungs, affecting both occluded and non-occluded small vessels, leading to high morbidity and mortality rates, particularly due to the progression to right heart failure if untreated (24, 48, 49). CTEPH is a rare, late complication of APE unresolved after more than 3 months of anticoagulation therapy, typically stemming from venous thromboembolism (50). Approximately 4% of APE cases evolve into CTEPH within 2 years (51), with 75% of CTEPH patients having a history of APE (52).
The reason why some individuals develop CTEPH post-APE remains unclear, especially given that most clots resolve with anticoagulation. CTEPH thrombi are fibrous, featuring collagenous and inflammatory components, sometimes leading to calcification (45).
According to Lorenz et al., the most significant determinant for the development of CTEPH is the presence of complete occlusion of the central pulmonary arteries, lobar pulmonary arteries, or both on initial imaging. Increased PA diameter and the presence of mosaic attenuation may also be additional predictors (46).
Contributing factors include coagulation and fibrinolysis anomalies, impaired platelet function, vascular remodeling, inflammation, blood groups, and cancer (45). Unlike classic genetic thrombogenic risk factors, Lupus Anti-Coagulant (LAC) and Anti-Phospholipid (APL) antibodies are more commonly associated with CTEPH (6). Fibrinogen polymorphism and factor V Leiden mutations contribute to thrombi's resistance to plasmin activity (53), while platelet disorders manifest as increased turnover and impaired aggregation (54, 55).
Inflammation plays a significant role, with elevated levels of C-reactive protein (CRP) and other inflammatory markers significantly higher in CTEPH patients (56). Risk factors also include malignancy, thyroid replacement therapy, non-O blood groups, ventriculo-atrial shunts, infected pacemakers, splenectomy, and previous recurrent venous thromboembolism (VTE) (57). While our understanding of CTEPH pathophysiology has advanced, many aspects, including the roles of BMPR2 mutations and microRNAs, remain to be fully elucidated (58).
CTEPH involves significant right ventricular and pulmonary circulation alterations (58). The concept of ventriculo-arterial coupling is pivotal, reflecting optimal adaptation of right ventricular function to pulmonary vascular load for efficient energy transfer (58, 59). Well-adapted right ventricles maintain efficient ventriculo-arterial coupling, while maladapted ones exhibit disrupted coupling, affecting energy transfer efficiency (58, 59). Despite reduced right ventricular ejection fraction (RVEF) in CTEPH, ventricular elastance often increases, indicating an adaptive response to increased PVR (59).
Both ventricles are affected due to their anatomical connection, with ventricular interdependency highlighted by abnormal septum motion during early diastole of the left ventricle (58). This motion results from dyssynchronous relaxation between the ventricles, indicating right ventricular overload in CTEPH (58). The leftward septum motion during early diastole, due to late right ventricular ejection, contributes to left ventricular underfilling and atrophy (58, 59).
In severe CTEPH, increased right ventricular diastolic stiffness, sometimes accompanied by fibrosis and biological changes like decreased titin phosphorylation, is observed (58). The intricate relationship between the right ventricle, arterial load, and interventricular interaction is crucial in understanding CTEPH pathophysiology, highlighting the adaptive and maladaptive changes within the right ventricle and its coupling with the pulmonary circulation (Figures 2, 3).
CTEPH is a multifaceted disease with a complex molecular backdrop. Polymorphisms such as those in the ENG and MAPK10 genes, mutations in BMPR2 and SMAD9, and dysregulated gene expressions, like those involving the PPAR signaling pathway, have been implicated in its pathophysiology. The alteration in ENG leads to resistance to fibrinolysis, while MAPK10 changes affect the MAPK signaling pathway, crucial for cell communication and proliferation (60). Mutations in BMPR2 disturb TGF-β signaling and induce pulmonary arterial smooth muscle cell (PASMC) proliferation, suggesting a pivotal role in vascular remodeling, a hallmark of CTEPH (61, 62).
A deeper dive into gene expression reveals a dysregulated orchestra of chemokine signaling, metabolic pathways, and arachidonic acid metabolism, signifying inflammation and metabolic shifts at the disease's core. The identified microRNAs and DNA methylation patterns in patients with CTEPH provide further insight into the complex regulatory mechanisms at play, affecting processes from fibrinogen degradation to TGF-β signaling and PASMC migration. Particularly, the downregulation of miR-759 and alterations in the let-7d-5p-miR-204-5p axis suggest novel areas for therapeutic targeting and prognostic evaluation (61).
Recent studies have highlighted the significant role of the NLRP3 inflammasome in PH, suggesting it as a potential therapeutic target for CTEPH. Activation of the NLRP3 inflammasome leads to proinflammatory programmed cell death known as pyroptosis, through the autoproteolytic activation of caspase-1, which subsequently triggers the cleavage of proinflammatory cytokines IL-1β and IL-18 (63, 64). This inflammatory cascade can contribute to the pulmonary vascular remodeling characteristic of CTEPH, marking inflammasome activation as a critical area for therapeutic intervention (65). Interestingly, natural products and formulations, including curcumin, resveratrol, triptolide, and allicin, have demonstrated protective effects against hypertensive organ damage by inhibiting the NLRP3 inflammasome (66).
The integration of these molecular insights is pivotal in designing preclinical studies and could potentially lead to novel therapeutic strategies aimed at these specific pathways and mechanisms. While this review provides a comprehensive overview of the molecular landscape in CTEPH, continuous research in this rapidly evolving field is crucial for validating these targets and understanding their roles in disease progression and management (60).
Clinical advancements have been observed in patients with CTEPH who are either inoperable or continue to experience PH post-PEA through the administration of Riociguat. This medication, a soluble guanylate cyclase (sGC) stimulator, underscores the critical role of the nitric oxide (NO)-sGC-cGMP pathway in managing the disease (49). Those play pivotal roles as vasodilators while also inhibiting leukocyte adherence, platelet aggregation, and the proliferation and migration of vascular smooth muscle cells. Coherently, Macitentan, an endothelin-1 (ET-1) receptor antagonist, has demonstrated promising results in clinical trials for patients with inoperable CTEPH, further highlighting the potential of targeting the NO, ET-1, and prostacyclin (PGI2) pathways in treatment strategies similar to those employed for PAH (49, 67) (Figure 4).
The clinical presentation of CTEPH is often nonspecific, posing challenges for early diagnosis as symptoms can be subtle and easily mistaken for other conditions (68). Exertional dyspnea, or shortness of breath during physical activity, is the most frequently reported symptom by patients with CTEPH, significantly impacting their exercise capacity and ability to perform previously enjoyable activities (53).
Aside from exertional dyspnea, other symptoms may emerge, albeit less commonly. Patients may experience chest pain, ranging from mild discomfort to severe, sharp pain (49, 69). Palpitations are reported by some, while others might have a cough, typically nonproductive (49, 53). Hemoptysis is considered relatively rare (53).
As CTEPH advances, symptoms indicative of right ventricular (RV) dysfunction become apparent. Lower extremity edema, manifesting as swelling in legs and feet, signals fluid retention and impaired RV function (53, 58). Exertional lightheadedness, dizziness, and even syncope (fainting) can occur, reflecting reduced cardiac output and inadequate brain perfusion, signifying a more severe disease phase (53).
Physical examination findings can also hint at CTEPH. A loud pulmonic component of the second heart sound (P2) suggests elevated pulmonary artery pressure and pulmonary vascular resistance, which can be indicative of a pulmonary flow murmur due to turbulent flow through partially occluded or re-cannulized chronic thrombi (53). However, this sign is not unique to CTEPH and may be observed in other pulmonary artery diseases (53, 70). The detection of a tricuspid regurgitation murmur points to backward blood flow through the tricuspid valve, often due to RV enlargement or dysfunction (24, 53). In some advanced cases, a palpable RV heave, where the right ventricle presses against the chest wall, might be felt (53).
Further progression towards RV failure is marked by additional signs. Increased jugular venous pulsation, visible as neck vein bulging, and hepatojugular reflux, where jugular venous pressure rises upon liver compression, are notable (24, 70). Peripheral edema, or swelling in lower limbs and other dependent areas, may intensify, collectively signaling advancing RV dysfunction and the onset of right heart failure (49, 53).
In essence, CTEPH's clinical features can vary significantly across different disease stages and are often nonspecific, underscoring the importance of a thorough and careful diagnostic process to identify this potentially severe condition.
Throughout the years, the approach to diagnosing PH has seen various iterations and improvements in diagnostic algorithms. One such innovative tool is the SCAR acronym—standing for Suspect, Confirm, and Assess Risk. This mnemonic aids in the systematic approach to CTEPH diagnosis, emphasizing the necessity for heightened suspicion to accurately identify the condition (71). The utility of multimodal imaging plays a pivotal role in the diagnosis of CTEPH, reflecting the complex nature of this disease and the need for comprehensive evaluation.
The initial step in the SCAR algorithm underscores the importance of maintaining a high index of suspicion for CTEPH, especially in patients presenting with unexplained dyspnea, a history of pulmonary embolism, or signs of right heart failure. This stage involves recognizing the potential presence of CTEPH based on clinical presentation and risk factors.
Upon suspicion, confirmation of CTEPH involves an array of diagnostic tests, with imaging modalities at the forefront. Multimodal imaging, including echocardiography, ventilation-perfusion (V/Q) scan, computed tomography pulmonary angiography (CTPA), magnetic resonance imaging (MRI), and pulmonary angiography, is crucial. Each of these imaging tools offers unique insights:
- Echocardiography serves as a non-invasive method to assess right ventricular function and estimate pulmonary artery pressures.
- The V/Q scan is highly sensitive for detecting CTEPH and is considered the gold standard screening test, capable of revealing mismatches indicative of pulmonary emboli.
- CTPA provides detailed visualization of the pulmonary vasculature, allowing for the identification of thromboembolic obstructions and vascular abnormalities.
- MRI offers additional functional and structural information about the heart and pulmonary circulation without radiation exposure.
- Pulmonary angiography remains the definitive method for visualizing the pulmonary arteries, offering precise details on the location and extent of obstructions.
This evaluation is essential to quantify the likelihood of developing CTEPH in subjects with a suggestive clinical pattern. After confirming the diagnosis, risk assessment involves evaluating the severity of PH, right ventricular function, and the general clinical status of the patient. This assessment guides the treatment strategy, including the decision for surgical intervention, such as PEA, or medical management with targeted therapies (72).
The SCAR algorithm, combined with multimodality imaging, emphasizes the personalized and stepwise approach to the diagnosis of CTEPH. The integration of advanced imaging techniques into the diagnostic process not only aids in the confirmation of CTEPH, but also provides critical information for individual risk stratification and subsequent treatment planning, demonstrating the significant role of imaging in the comprehensive care of patients with CTEPH (Figure 5).
Figure 5. CTEPH diagnosis algorithm (71).
At the time of diagnosing CTEPH, a comprehensive panel of laboratory tests is essential for assessing the patient's overall health status, identifying potential complications, and informing treatment decisions. The recommended laboratory evaluations encompass a wide range of tests:
- Total Blood Count: Essential for evaluating hemoglobin levels, white blood cells, and platelets, which can indicate anemia, infection, or other hematologic conditions.
- Serum Electrolytes: Including sodium, potassium, magnesium, chlorine, calcium, and phosphorus, to assess electrolyte balance and detect potential disturbances that could affect cardiac and muscular function.
- Renal Function Tests: Creatinine levels and the estimated glomerular filtration rate (eGFR) are critical for assessing kidney function, with urea levels providing additional insights into renal health and metabolic status.
- Liver Function Tests: Alanine aminotransferase (ALT), aspartate aminotransferase (AST), alkaline phosphatase, γ-glutamyl transpeptidase (GGT), and bilirubin levels help evaluate liver health and detect hepatic dysfunction, which can be relevant for medication metabolism and overall health.
- Uric Acid: Elevated levels may indicate increased risk for gout and can be associated with cardiovascular diseases.
- Iron Status: Measurements of serum iron, transferrin saturation, and ferritin are important for identifying iron deficiency or overload, which can have implications for anemia and overall health.
- BNP or NT-proBNP: These biomarkers of heart failure are crucial for assessing cardiac strain and guiding the management of CTEPH.
- Serological Studies: Testing for hepatitis and HIV viruses is important for identifying underlying conditions that could impact treatment options and prognosis.
- Immunology Laboratory Exam: Screening for antinuclear antibodies, anti-centromere antibodies, and anti-Ro can help identify autoimmune conditions that may have implications for CTEPH.
- Antiphospholipid Syndrome Biomarkers: Screening is recommended due to the association of antiphospholipid syndrome with thrombotic events, although broader thrombophilia screening is not advised (73).
In clinical settings, the 12-lead electrocardiogram (ECG) is a readily available diagnostic tool. ECG manifestations of right heart strain include P-pulmonale (characterized by a P wave amplitude exceeding 2.5 mm), right bundle branch block, T-wave abnormalities in chest leads, and right-axis deviation (3, 74). Signs of right ventricular hypertrophy (RVH), indicative of pulmonary arterial systolic pressure (PASP) within normal limits (<7%), are infrequently observed. ECG patterns with a high positive predictive value (>80%) for pulmonary hypertension include R in lead I ≤ 2 mm, S in lead V1 ≤ 2 mm, R/S ratio in lead V1 ≥ 1, R/S ratio in lead V6 ≤ 1, QRS axis ≥ 110°, and a qR pattern in V1. Notably, an S wave ≤2 mm in V1 predicts the presence of pulmonary hypertension with 100% certainty. The detection of any of these ECG patterns should prompt further evaluation with transthoracic echocardiography (TTE) for a more comprehensive assessment of cardiac function and PASP measurement. However, these ECG patterns demonstrate low negative predictive values, indicating that their absence does not conclusively rule out pulmonary hypertension (75). This summary outlines the specific ECG criteria for RVH or right atrial enlargement associated with pulmonary hypertension, as depicted in Figure 6.
Figure 6. ECG abnormalities in CTEPH (75).
The European Society of Cardiology (ESC) Guideline on pulmonary embolism (PE) advises echocardiography as an initial evaluation in patients with persistent dyspnea, functional limitations, or risk factors for CTEPH (3). TTE is pivotal for estimating pulmonary arterial pressure, offering crucial insights into the pathophysiology and etiology of CTEPH and the impact of chronic PAH on the heart. It also yields vital data on coexisting cardiac pathology, surgical outcomes, and potential postoperative complications like tamponade.
For patients with confirmed or highly suspected PH, a thorough assessment is essential to rule out left-sided heart disease or intracardiac shunts. Markers such as right ventricular dilatation and dysfunction are considered negative prognostic indicators. Additionally, pericardial effusion in the context of PH signals advanced disease with a poor prognosis (76).
Echocardiographic evaluation of PH includes measuring the peak velocity of tricuspid valve regurgitation, calculating atrioventricular pressure gradients, and identifying indirect signs of PH like right atrial and ventricular dilatation, diminished right ventricular contractility, and Doppler flow anomalies in the right ventricular outflow tract (3). However, these indicators might not be present in early disease stages, with echocardiography potentially missing PH in 10%–31% of cases (77).
The initial step in determining echocardiographic probability of PH involves measuring the peak of tricuspid regurgitation velocity (TRV). A flow chart for establishing CTEPH probability recommends assessing likelihood across all clinical PH groups, incorporating additional echocardiographic parameters divided into three categories indicative of PH (78):
- Ventricles: RV/LV basal diameter/area ratio >1.0; flattening of the interventricular septum; TAPSE/sPAP ratio <0.55 mm/mmHg;
- Pulmonary artery: RVOT acceleration time <105 ms and/or mid-systolic notching; early diastolic pulmonary regurgitation velocity >2.2 m/s; pulmonary artery diameter exceeding aortic root diameter or >25 mm;
- Inferior vena cava and right atrium: IVC diameter >21 mm with reduced inspiratory collapse; right atrial area >18 cm2.
A TRV >3.4 m/s suggests a high echocardiographic probability of PAH, while a TRV ≤3.4 m/s requires additional parameters from at least two categories to assess the likelihood of PAH (Figure 7).
Figure 7. Flow chart to assess the echocardiographic probability of CTEPH (79).
Additional echocardiographic markers not critical for PH likelihood determination but useful for assessing severity and providing prognostic information include right ventricular size, fractional area change, tricuspid annular plane systolic excursion (TAPSE), peak systolic RV pulsed tissue Doppler velocity, and right ventricular myocardial performance index (RIMP) (79).
Given the RV's complex geometry, advancements in echocardiography techniques like tissue Doppler, 3D volume imaging, and RV strain analysis continue to shed light on the disease's pathophysiology.
Lung Function Tests (LFTs) for evaluating CTEPH should encompass measurements of total lung capacity and the diffusing capacity of the lung for carbon monoxide (DLCO). In many patients with PAH, a mild restrictive disorder is observed, while a decreased DLCO is commonly noted in those with CTEPH (3). Although LFTs might yield normal results in CTEPH cases, some patients may exhibit mild restriction, reduced diffusion capacity, or both.
Arterial Blood Gas (ABG) analysis, or arterialized capillary blood sampling, is crucial for differentiating among the various groups of PH, evaluating comorbid conditions, and determining the necessity for supplemental oxygen or Non-Invasive Ventilation (NIV), in addition to staging disease severity. Typically, patients with PAH present with normal or slightly reduced PaO2 levels. A significant drop in PaO2 may indicate the presence of a patent foramen ovale, liver disease, other congenital heart defects with right-to-left shunt, or conditions linked to low DLCO. The partial pressure of arterial carbon dioxide (PaCO2) is often lower than normal due to alveolar hyperventilation in PAH patients and is usually associated with a poor prognosis.
Chest radiography, while often not revealing in the early stages of CTEPH, can demonstrate features such as enlargement of the central pulmonary arteries and right cardiac chambers, or asymmetry of the pulmonary vasculature as the disease progresses (80). Additionally, a chest x-ray can provide valuable insights for diagnosing other pulmonary parenchyma or cardiac pathologies, including hypertrophy that might underlie CTEPH. Key findings identifiable on chest radiographs are compiled in Table 2. However, it's important to note that a normal chest x-ray does not rule out the presence of PH (81).
Ventilation/perfusion (V/Q) scintigraphy remains pivotal in assessing patients suspected of having CTEPH, serving as a critical noninvasive diagnostic tool for detecting pulmonary vascular disease. The hallmark of CTEPH on a V/Q scan is the identification of persistent mismatched perfusion defects, which are readily recognizable. Normal V/Q scan results effectively exclude CTEPH, boasting a high sensitivity of 96%–97% and specificity of 90%–95% (82).
In CTED, typically one or more segmental or larger mismatched perfusion defects are observed. However, it's crucial to note that scan findings might not fully capture the extent of pulmonary artery obstruction, especially in cases with recanalized occlusions where distal perfusion has been re-established.
The use of single photon emission computed tomography (SPECT) V′/Q′ scanning has seen a rise in preference over planar imaging for APE diagnosis, attributed to its enhanced sensitivity and specificity, especially when comorbidities are present (83). However, the application and validation of this technique specifically for CTEPH remain less established (81).
CT Pulmonary Angiography (CTPA) is a valuable diagnostic tool for excluding CTEPH.
CTPA can provide valuable supplemental insights to those gained from V/Q scans, offering detailed anatomic localization and assessing the feasibility of surgical intervention (84). Except for pulmonary veno-occlusive disease—which can present with segmented defects—conditions affecting the distal pulmonary vascular bed generally result in normal perfusion scans or show a “patchy” pattern characterized by nonsegmental defects (85).
In cases with clinical indications of acute pulmonary embolism, CTPA can uncover previously undetected signs of CTEPH (86). It is particularly effective for identifying subsegmental or segmental artery thrombosis, critical for determining surgical eligibility for PEA (87). Additionally, high-resolution chest tomography (HRCT) offers important insights into lung parenchymal conditions, such as interstitial diseases, pulmonary emphysema, or bronchial anomalies, alongside infarcts, and vascular and thoracic wall malformations.
Despite its utility, CTPA alone is not definitive for ruling out CTEPH (88). It can reveal typical CTEPH alterations, comorbidities, and complications, including pulmonary artery dilation, large bronchial arterial collaterals, and the hypoperfusion areas indicative of the “mosaic” pattern, although this pattern can also appear in 12% of Idiopathic Pulmonary Arterial Hypertension (IPAH) patients (89). CT findings suggestive of PH include an increased pulmonary artery (PA) diameter, straightening or leftward bowing of the interventricular septum, right ventricular (RV) dilatation, and hypertrophy.
CTPA depicts vascular abnormalities specific to CTEPH, such as central pulmonary artery enlargement with reduced peripheral vessel diameters, eccentric wall-adherent thrombi (potentially calcified), differing significantly from acute PE's hallmark central filling defect. Bronchial artery dilatation, though not unique to CTEPH, is commonly observed and associated with better outcomes post-PEA (90).
Pulmonary Digital Subtraction Angiography (PDSA) is traditionally viewed as the gold standard for delineating CTEPH vascular morphology. It can identify occlusions (pouch defects), webs or bands, irregular vessel walls, and abrupt vessel narrowing or disappearance. While PDSA remains crucial, especially when CTPA results are inconclusive, advancements in noninvasive imaging techniques are changing the diagnostic landscape. Selective segmental angiography, cone-beam CT, and area-detector CT provide enhanced visualization of subsegmental vasculature, aiding in BPA procedural planning (3).
Emerging imaging modalities, including magnetic resonance pulmonary angiography, dual-energy CT, and cone-beam CT, are promising for detailed pulmonary vasculature examination (91–93), offering comprehensive diagnostic capabilities in the evaluation of CTEPH.
Cardiopulmonary Exercise Testing (CPET) serves as an advanced diagnostic tool that helps differentiate among various causes of dyspnea by examining the pathophysiology behind exercise intolerance. In individuals with PH, CPET reveals distinctive changes, including a reduced end-tidal partial pressure of carbon dioxide (PETCO2), an elevated ventilatory equivalent for carbon dioxide (VE/VCO2), diminished oxygen pulse (VO2/HR), and decreased peak oxygen uptake (VO2) (94). Similarly, those suffering from pulmonary vascular disease display a specific CPET pattern characterized by reduced exercise capacity, limited stroke volume, increased dead space ventilation, and ventilatory inefficiency (95). The utilization of CPET, alongside exercise hemodynamics, is anticipated to grow in importance for the evaluation and monitoring of CTEPD without PH, marking a significant advance in the diagnosis and follow-up of CTEPH.
CPET can detect subtle abnormalities in patients with normal resting echocardiography, such as an elevated VE/VCO2 slope and a decreased PETCO2 at the anaerobic threshold, which are indicative of pulmonary vascular disease (96, 97). Additionally, CPET parameters like peak exercise VD/VT >45% have shown high sensitivity (92%) and specificity (83%) for predicting CTEPH (98).
Invasive cardiopulmonary exercise testing (iCPET) further enhances the diagnostic capability of CPET by directly measuring central hemodynamics and gas exchange during exercise. iCPET involves the insertion of catheters into the right side of the heart and pulmonary artery, allowing for precise measurement of pulmonary artery pressure (PAP), PCWP, and cardiac output during exercise. This invasive approach can uncover hidden abnormalities in PVR and right ventricular function that are not detectable with non-invasive CPET alone (98, 99). For instance, iCPET can differentiate between pre-capillary and post-capillary causes of PH, providing critical insights for targeted treatment (97, 99).
Studies have shown that iCPET is particularly valuable in diagnosing CTEPH, as it can reveal exercise-induced increases in PAP and PVR that confirm the presence of significant pulmonary vascular obstruction. This detailed hemodynamic assessment is crucial for distinguishing CTEPH from other forms of PH and guiding appropriate therapeutic interventions (97, 99).
By integrating CPET and iCPET with other diagnostic tools, such as echocardiography and right heart catheterization, clinicians can achieve a more comprehensive assessment of patients, especially those with persistent symptoms post-PE (97–99).
Right Heart Catheterization (RHC) is indispensable for verifying that pulmonary circulation hemodynamics align with the diagnostic criteria for PH: mean pulmonary artery (PA) pressure exceeding 20 mmHg and pulmonary artery wedge pressure (PAWP) of 15 mmHg or less (100). Execution of RHC demands proficiency and adherence to standardized protocols to accurately measure pressures within the right atrium (RA), right ventricle (RV), and pulmonary artery, as well as to determine cardiac output (either via Fick's direct method or thermodilution, averaging at least three measurements) and mixed venous blood saturation. Additionally, the procedure calculates pulmonary vascular resistance, a crucial prognostic indicator for both preoperative and postoperative evaluations (101).
There are absolute contraindications to performing RHC, including the presence of thrombus or tumor within the RA or RV, recently implanted pacemakers (less than 1 month old), mechanical right heart valves, TriClip devices, and acute infections/endocarditis. The risk/benefit ratio of conducting RHC should be carefully evaluated and discussed with the patient prior to the procedure. While complications from RHC do exist, their incidence is considerably low, generally less than 1% (102). One of the most severe complications associated with RHC is the perforation of the pulmonary artery (PA), highlighting the importance of thorough patient preparation. Optimal control of pre-existing medical conditions, particularly blood pressure and volume, is essential at the time of examination.
The zero reference level for catheterization pressure measurements, including PAWP, is recommended to be the mid-thoracic level, corresponding to the left atrium's position in most patients (103). These measurements should be taken at the end of expiration, avoiding breath-hold maneuvers. However, in patients with significant intrathoracic pressure variations (e.g., those with COPD, obesity, or during exercise), averages over three to four respiratory cycles are calculated for accuracy. Accurate PAWP readings require the catheter to be placed in the wedge position for saturation measurements (104).
Measuring pulmonary arterial occlusion pressure (PAOP) is vital to exclude post-capillary PH due to comorbidities. In cases of CTEPH, where intravascular obstructions may obscure PAOP estimation, left ventricular end-diastolic pressure should be assessed via left ventricular catheterization (96). Notably, in central CTEPH, blood pressure may vary locally due to central organizing thrombi, necessitating measurements in both left and right main pulmonary arteries and PA trunks. Moreover, measuring PA wedge pressure can be complicated by the adhesion of organic thrombi, requiring careful waveform analysis prior to measurement (105). An evaluation of the main diagnostic methods can be seen in Table 3.
For an accurate and timely diagnosis of CTEPH, clinicians must maintain a high level of suspicion when encountering patients with unexplained dyspnea or symptoms indicative of right-sided heart failure. A comprehensive evaluation, employing a range of multimodal imaging techniques such as echocardiography, CT scans, and ventilation/perfusion (V/Q) scans, is essential to increase the diagnostic accuracy for CTEPH. In cases where CTEPH is suspected, Right Heart Catheterization (RHC), CTPA, and Pulmonary Digital Subtraction Angiography (PDSA)—especially when CTPA results are inconclusive—are imperative to confirm the diagnosis and guide therapeutic decision-making (Figure 8).
Screening is a crucial approach for identifying unrecognized conditions or risk markers, applicable to both individuals and populations, regardless of symptom presence. This method aims to detect potential diseases early, allowing for timely intervention to reduce morbidity and mortality. Despite advancements in therapies slowing CTEPH and PH progression, diagnosis often occurs more than 2 years after symptom onset, highlighting CTEPH as a commonly underdiagnosed PE complication. Routine PH screening post-PE isn't universally recommended, yet should be considered for those with persistent symptoms (106).
The 2019 ESC Guidelines detail a screening algorithm for post-PE syndrome, suggesting an initial patient assessment 3–6 months post-PE. This timing aligns with anticoagulation therapy review and early identification of ongoing or new dyspnea and/or functional limitations (57). Additionally, risk factors like permanent intravascular devices and specific health conditions should be considered (24, 57). Screening for antiphospholipid syndrome at CTEPH diagnosis is advised, with long-term anticoagulation therapy decisions made on a case-by-case basis (73).
For patients exhibiting symptoms or risk factors for CTEPH, echocardiography is the preferred diagnostic method due to its safety and informative value (107). TTE, the initial screening and follow-up tool for CTEPH, estimates systolic PA pressure and assesses the right ventricle/atrium, valvular structures, intracardiac shunts, and other PH causes (3, 108). With low PH probability, alternative dyspnea causes should be explored. For intermediate probability, further testing, including BNP or NT-proBNP levels and CPET, may be indicated. Normal NT-proBNP levels and typical ECG findings of RV load significantly predict CTEPH absence (109, 110).
The Leiden CTEPH rule-out criteria and the InShape II algorithm are particularly important tools for CTEPH diagnosis. The Leiden CTEPH rule-out criteria use a combination of ECG and NT-proBNP levels to effectively exclude CTEPH in patients with a history of acute PE and suspected CTEPH. This tool has been shown to have high sensitivity and specificity, making it a reliable method for ruling out the disease in a non-invasive manner (111). The InShape II algorithm also incorporates clinical parameters, ECG, and NT-proBNP levels, streamlining the diagnostic process by providing clear criteria to rule out CTEPH, thus minimizing the need for more invasive and costly procedures (112).
For high disease probability, lung V/Q scan or newer modalities like iodine subtraction mapping, DECT, and MRI perfusion are considered to detect mismatched perfusion defects post-anticoagulation therapy. Despite the theoretical advantages of these newer techniques, they are technically demanding, costlier, and lack widespread validation (3). V/Q scintigraphy remains the top noninvasive screening tool for CTEPH due to its high sensitivity and specificity (3). Recent studies have explored the sensitivity and specificity of novel CT techniques compared to traditional V/Q scans, finding them comparably effective but more complex and less accessible (113, 114).
CTEPH, often developing from APE in about 20% of cases, transitions into a chronic condition characterized by vasoconstriction, vascular remodeling, and proliferation of smooth muscle and endothelial cells, leading to increased pulmonary artery and right heart chamber pressures and subsequent right heart failure (38, 96). This review aims to provide clinicians with a comprehensive guide on the epidemiology, pathophysiology, risk factors and diagnostics for patients suspected of having CTEPH.
Due to its rarity, lethality, and disabling nature, CTEPH demands prompt diagnosis and treatment. For early detection, clinicians must maintain a high index of suspicion and perform thorough evaluations for patients presenting with unexplained dyspnea or signs of right-sided heart failure. Utilizing a combination of imaging tools, such as echocardiography, CT, and V/Q scans, is essential for improving diagnostic accuracy. Right Heart Catheterization (RHC) and Digital Subtraction Angiography (DSA) are crucial for confirming CTEPH diagnosis and guiding treatment decisions.
BS: Writing – original draft, Writing – review & editing, Conceptualization, Data curation, Formal Analysis, Funding acquisition, Investigation, Methodology, Project administration, Resources, Software, Supervision, Validation, Visualization. EM: Writing – original draft, Writing – review & editing, Conceptualization, Data curation, Formal Analysis, Funding acquisition, Investigation, Methodology, Project administration, Resources, Software, Supervision, Validation, Visualization. LS: Writing – original draft, Writing – review & editing, Conceptualization, Data curation, Formal Analysis, Funding acquisition, Investigation, Methodology, Project administration, Resources, Software, Supervision, Validation, Visualization. ER: Conceptualization, Data curation, Formal Analysis, Funding acquisition, Investigation, Methodology, Project administration, Resources, Software, Supervision, Validation, Visualization, Writing – review & editing. GS: Conceptualization, Data curation, Formal Analysis, Funding acquisition, Investigation, Methodology, Project administration, Resources, Software, Supervision, Validation, Visualization, Writing – review & editing. LS: Conceptualization, Data curation, Formal Analysis, Funding acquisition, Investigation, Methodology, Project administration, Resources, Software, Supervision, Validation, Visualization, Writing – review & editing. MB: Conceptualization, Data curation, Formal Analysis, Funding acquisition, Investigation, Methodology, Project administration, Resources, Software, Supervision, Validation, Visualization, Writing – review & editing. LA: Conceptualization, Data curation, Formal Analysis, Funding acquisition, Investigation, Methodology, Project administration, Resources, Software, Supervision, Validation, Visualization, Writing – review & editing. MF: Conceptualization, Data curation, Formal Analysis, Funding acquisition, Investigation, Methodology, Project administration, Resources, Software, Supervision, Validation, Visualization, Writing – review & editing. DV: Conceptualization, Data curation, Formal Analysis, Funding acquisition, Investigation, Methodology, Project administration, Resources, Software, Supervision, Validation, Visualization, Writing – review & editing. VV: Conceptualization, Data curation, Formal Analysis, Funding acquisition, Investigation, Methodology, Project administration, Resources, Software, Supervision, Validation, Visualization, Writing – review & editing. SS: Conceptualization, Data curation, Formal Analysis, Funding acquisition, Investigation, Methodology, Project administration, Resources, Software, Supervision, Validation, Visualization, Writing – review & editing. CV: Conceptualization, Data curation, Formal Analysis, Funding acquisition, Investigation, Methodology, Project administration, Resources, Software, Supervision, Validation, Visualization, Writing – review & editing.
The author(s) declare that no financial support was received for the research, authorship, and/or publication of this article.
The authors declare that the research was conducted in the absence of any commercial or financial relationships that could be construed as a potential conflict of interest.
All claims expressed in this article are solely those of the authors and do not necessarily represent those of their affiliated organizations, or those of the publisher, the editors and the reviewers. Any product that may be evaluated in this article, or claim that may be made by its manufacturer, is not guaranteed or endorsed by the publisher.
The Supplementary Material for this article can be found online at: https://www.frontiersin.org/articles/10.3389/fcvm.2024.1439402/full#supplementary-material
1. Beshay S, Sahay S, Humbert M. Evaluation and management of pulmonary arterial hypertension. Respir Med. (2020) 171:106099. doi: 10.1016/j.rmed.2020.106099
2. Lau EMT, Giannoulatou E, Celermajer DS, Humbert M. Epidemiology and treatment of pulmonary arterial hypertension. Nat Rev Cardiol. (2017) 14(10):603–14. doi: 10.1038/nrcardio.2017.84
3. Humbert M, Kovacs G, Hoeper MM, Badagliacca R, Berger RMF, Brida M, et al. 2022 ESC/ERS guidelines for the diagnosis and treatment of pulmonary hypertension. Eur Respir J. (2023) 61(1):2200879. doi: 10.1183/13993003.00879-2022
4. Douschan P, Kovacs G, Avian A, Foris V, Gruber F, Olschewski A, et al. Mild elevation of pulmonary arterial pressure as a predictor of mortality. Am J Respir Crit Care Med. (2018) 197(4):509–16. doi: 10.1164/rccm.201706-1215OC
5. Chausheva S, Naito A, Ogawa A, Seidl V, Winter MP, Sharma S, et al. Chronic thromboembolic pulmonary hypertension in Austria and Japan. J Thorac Cardiovasc Surg. (2019) 158(2):604–14.e2. doi: 10.1016/j.jtcvs.2019.01.019
6. Bochenek ML, Rosinus NS, Lankeit M, Hobohm L, Bremmer F, Schütz E, et al. From thrombosis to fibrosis in chronic thromboembolic pulmonary hypertension. Thromb Haemost. (2017) 117(4):769–83. doi: 10.1160/TH16-10-0790
7. Hoeper MM, Pausch C, Olsson KM, Huscher D, Pittrow D, Grünig E, et al. COMPERA 2.0: a refined four-stratum risk assessment model for pulmonary arterial hypertension. Eur Respir J. (2022) 60(1):2102311. doi: 10.1183/13993003.02311-2021
8. Kovacs G, Berghold A, Scheidl S, Olschewski H. Pulmonary arterial pressure during rest and exercise in healthy subjects: a systematic review. Eur Respir J. (2009) 34(4):888–94. doi: 10.1183/09031936.00145608
9. Kolte D, Lakshmanan S, Jankowich MD, Brittain EL, Maron BA, Choudhary G. Mild pulmonary hypertension is associated with increased mortality: a systematic review and meta-analysis. J Am Heart Assoc. (2018) 7(18):e009729. doi: 10.1161/JAHA.118.009729
10. Zeder K, Banfi C, Steinrisser-Allex G, Maron BA, Humbert M, Lewis GD, et al. Diagnostic, prognostic and differential-diagnostic relevance of pulmonary haemodynamic parameters during exercise: a systematic review. Eur Respir J. (2022) 60(4):2103181. doi: 10.1183/13993003.03181-2021
11. Maron BA, Brittain EL, Hess E, Waldo SW, Barón AE, Huang S, et al. Pulmonary vascular resistance and clinical outcomes in patients with pulmonary hypertension: a retrospective cohort study. Lancet Respir Med. (2020) 8(9):873–84. doi: 10.1016/S2213-2600(20)30317-9
12. Kovacs G, Olschewski A, Berghold A, Olschewski H. Pulmonary vascular resistances during exercise in normal subjects: a systematic review. Eur Respir J. (2012) 39(2):319–28. doi: 10.1183/09031936.00008611
13. Xanthouli P, Jordan S, Milde N, Marra A, Blank N, Egenlauf B, et al. Haemodynamic phenotypes and survival in patients with systemic sclerosis: the impact of the new definition of pulmonary arterial hypertension. Ann Rheum Dis. (2020) 79(3):370–8. doi: 10.1136/annrheumdis-2019-216476
14. Hoeper MM, Humbert M, Souza R, Idrees M, Kawut SM, Sliwa-Hahnle K, et al. A global view of pulmonary hypertension. Lancet Respir Med. (2016) 4(4):306–22. doi: 10.1016/S2213-2600(15)00543-3
15. Rosenkranz S, Gibbs JSR, Wachter R, De Marco T, Vonk-Noordegraaf A, Vachiéry JL. Affiliations expand left ventricular heart failure and pulmonary hypertension. Eur Heart J. (2016) 37(12):942–54. doi: 10.1093/eurheartj/ehv512
16. Lam CS, Roger VL, Rodeheffer RJ, Borlaug BA, Enders FT, Redfield MM. Pulmonary hypertension in heart failure with preserved ejection fraction: a community-based study. J Am Coll Cardiol. (2009) 53(13):1119–26. doi: 10.1016/j.jacc.2008.11.051
17. O’Sullivan CJ, Wenaweser P, Ceylan O, Stortecky S, Spitzer E, Zanchin T, et al. Effect of pulmonary hypertension hemodynamic presentation on clinical outcomes in patients with severe symptomatic aortic valve stenosis undergoing transcatheter aortic valve implantation: insights from the new proposed pulmonary hypertension classification. Circ Cardiovasc Interv. (2015) 8(9):e003064. doi: 10.1161/CIRCINTERVENTIONS.115.003064
18. Weber L, Rickli H, Haager PK, Joerg L, Weilenmann D, Brenner R, et al. Haemodynamic mechanisms and long-term prognostic impact of pulmonary hypertension in patients with severe aortic stenosis undergoing valve replacement. Eur J Heart Fail. (2019) 21(2):172–81. doi: 10.1002/ejhf.1322
19. Tichelbäcker T, Dumitrescu D, Gerhardt F, Stern D, Wissmüller M, Adam M, et al. Pulmonary hypertension and valvular heart disease. Herz. (2019) 44(6):491–501. doi: 10.1007/s00059-019-4823-6
20. Andersen MJ, Olson TP, Melenovsky V, Kane GC, Borlaug BA. Differential hemodynamic effects of exercise and volume expansion in people with and without heart failure. Circ Heart Fail. (2015) 8(1):41–8. doi: 10.1161/CIRCHEARTFAILURE.114.001731
21. Wolsk E, Bakkestrøm R, Thomsen JH, Balling L, Andersen MJ, Dahl JS, et al. The influence of age on hemodynamic parameters during rest and exercise in healthy individuals. JACC Heart Fail. (2017) 5(5):337–46. doi: 10.1016/j.jchf.2016.10.012
22. Rich S, Rabinovitch M. Diagnosis and treatment of secondary (non-category 1) pulmonary hypertension. Circulation. (2008) 118(21):2190–9. doi: 10.1161/CIRCULATIONAHA.107.723007
23. Wilkens H, Konstantinides S, Lang IM, Bunck AC, Gerges M, Gerhardt F, et al. Chronic thromboembolic pulmonary hypertension (CTEPH): updated recommendations from the Cologne consensus conference 2018. Int J Cardiol. (2018) 272(Suppl):69–78. doi: 10.1016/j.ijcard.2018.08.079
24. Delcroix M, Torbicki A, Gopalan D, Sitbon O, Klok FA, Lang I, et al. ERS statement on chronic thromboembolic pulmonary hypertension. Eur Respir J. (2021) 57(6):2002828. doi: 10.1183/13993003.02828-2020
25. Simonneau G, Fadel E, Vonk Noordegraaf A, Toshner M, Lang IM, Klok FA, et al. Highlights from the international chronic thromboembolic pulmonary hypertension congress 2021. Eur Respir Rev. (2023) 32(167):220132. doi: 10.1183/16000617.0132-2022
26. Barco S, Klok FA, Konstantinides SV, Dartevelle P, Fadel E, Jenkins D, et al. Sex-specific differences in chronic thromboembolic pulmonary hypertension. Results from the European CTEPH registry. J Thromb Haemost. (2020) 18(1):151–61. doi: 10.1111/jth.14629
27. Claeys M, Claessen G, La Gerche A, Petit T, Belge C, Meyns B, et al. Impaired cardiac reserve and abnormal vascular load limit exercise capacity in chronic thromboembolic disease. JACC Cardiovasc Imaging. (2019) 12(8 Pt 1):1444–56. doi: 10.1016/j.jcmg.2018.07.021
28. Swietlik EM, Ruggiero A, Fletcher AJ, Taboada D, Knightbridge E, Harlow L, et al. Limitations of resting haemodynamics in chronic thromboembolic disease without pulmonary hypertension. Eur Respir J. (2019) 53(1):1801787. doi: 10.1183/13993003.01787-2018
29. Maron BA, Abman SH, Elliott CG, Frantz RP, Hopper RK, Horn EM, et al. Pulmonary arterial hypertension: diagnosis, treatment, and novel advances. Am J Respir Crit Care Med. (2021) 203(12):1472–87. doi: 10.1164/rccm.202012-4317SO
30. Maron BA, Galiè N. Diagnosis, treatment, and clinical management of pulmonary arterial hypertension in the contemporary era: a review. JAMA Cardiol. (2016) 1(9):1056–65. doi: 10.1001/jamacardio.2016.4471
31. McGoon MD, Miller DP. REVEAL: a contemporary US pulmonary arterial hypertension registry. Eur Respir Rev. (2012) 21(123):8–18. doi: 10.1183/09059180.00008211
32. Condliffe R, Kiely DG, Gibbs JSR, Corris PA, Peacock AJ, Jenkins DP, et al. Improved outcomes in medically and surgically treated chronic thromboembolic pulmonary hypertension. Am J Respir Crit Care Med. (2008) 177(10):1122–7. doi: 10.1164/rccm.200712-1841OC
33. Escribano-Subias P, Blanco I, López-Meseguer M, Jimenez Lopez-Guarch C, Roman A, Morales P, et al. Survival in pulmonary hypertension in Spain: insights from the Spanish registry. Eur Respir J. (2012) 40(3):596–603. doi: 10.1183/09031936.00101211
34. Kramm T, Wilkens H, Fuge J, Schäfers HJ, Guth S, Wiedenroth CB, et al. Incidence and characteristics of chronic thromboembolic pulmonary hypertension in Germany. Clin Res Cardiol. (2018) 107(7):548–53. doi: 10.1007/s00392-018-1215-5
35. Poli D, Grifoni E, Antonucci E, Arcangeli C, Prisco D, Abbate R, et al. Incidence of recurrent venous thromboembolism and of chronic thromboembolic pulmonary hypertension in patients after a first episode of pulmonary embolism. J Thromb Thrombolysis. (2010) 30(3):294–9. doi: 10.1007/s11239-010-0452-x
36. Lang IM, Madani M. Update on chronic thromboembolic pulmonary hypertension. Circulation. (2014) 130(6):508–18. doi: 10.1161/CIRCULATIONAHA.114.009309
37. Klok FA, Dzikowska-Diduch O, Kostrubiec M, Vliegen HW, Pruszczyk P, Hasenfuß G, et al. Derivation of a clinical prediction score for chronic thromboembolic pulmonary hypertension after acute pulmonary embolism. J Thromb Haemost. (2016) 14(1):121–8. doi: 10.1111/jth.13175
38. Leber L, Beaudet A, Muller A. Epidemiology of pulmonary arterial hypertension and chronic thromboembolic pulmonary hypertension: identification of the most accurate estimates from a systematic literature review. Pulm Circ. (2021) 11(1):2045894020977300. doi: 10.1177/2045894020977300
39. Opitz I, Ulrich S. Chronic thromboembolic pulmonary hypertension. Swiss Med Wkly. (2018) 148:w14702. doi: 10.4414/smw.2018.14702
40. Valerio L, Mavromanoli AC, Barco S, Abele C, Becker D, Bruch L, et al. Chronic thromboembolic pulmonary hypertension and impairment after pulmonary embolism: the FOCUS study. Eur Heart J. (2022) 43(36):3387–98. doi: 10.1093/eurheartj/ehac206
41. Papamatheakis DG, Poch DS, Fernandes TM, Kerr KM, Kim NH, Fedullo PF. Chronic thromboembolic pulmonary hypertension: JACC focus seminar. J Am Coll Cardiol. (2020) 76(18):2155–69. doi: 10.1016/j.jacc.2020.08.074
42. de Perrot M, Gopalan D, Jenkins D, Lang IM, Fadel E, Delcroix M, et al. Evaluation and management of patients with chronic thromboembolic pulmonary hypertension—consensus statement from the ISHLT. J Heart Lung Transplant. (2021) 40(11):1301–26. doi: 10.1016/j.healun.2021.07.020
43. Papa S, Miotti C, Manzi G, Scoccia G, Luongo F, Toto F, et al. Peripheral arterial stiffness in acute pulmonary embolism and pulmonary hypertension at short-term follow-up. J Clin Med. (2021) 10(14):3008. doi: 10.3390/jcm10143008
44. Bonderman D, Jakowitsch J, Adlbrecht C, Schemper M, Kyrle PA, Schönauer V, et al. Medical conditions increasing the risk of chronic thromboembolic pulmonary hypertension. Thromb Haemost. (2005) 93(3):512–6. doi: 10.1160/TH04-10-0657
45. Wagenvoort CA. Pathology of pulmonary thromboembolism. Chest. (1995) 107(1 Suppl):10S–7. doi: 10.1378/chest.107.1_supplement.10s
46. Lorenz G, Saeedan MB, Bullen J, Klok FA, Kroft LJM, Meijboom LJ, et al. CT-based biomarkers for prediction of chronic thromboembolic pulmonary hypertension after an acute pulmonary embolic event. AJR Am J Roentgenol. (2020) 215(4):800–6. doi: 10.2214/AJR.19.22541
47. Gerges C, Pistritto AM, Gerges M, Friewald R, Hartig V, Hofbauer TM, et al. Left ventricular filling pressure in chronic thromboembolic pulmonary hypertension. J Am Coll Cardiol. (2023) 81(7):653–64. doi: 10.1016/j.jacc.2022.11.049
48. Mayer E, Jenkins D, Lindner J, D’Armini A, Kloek J, Meyns B, et al. Surgical management and outcome of patients with chronic thromboembolic pulmonary hypertension: results from an international prospective registry. J Thorac Cardiovasc Surg. (2011) 141(3):702–10. doi: 10.1016/j.jtcvs.2010.11.024
49. Humbert M, Kovacs G, Hoeper MM, Badagliacca R, Berger RMF, Brida M, et al. 2022 ESC/ERS guidelines for the diagnosis and treatment of pulmonary hypertension. Eur Heart J. (2022) 43(38):3618–731. doi: 10.1093/eurheartj/ehac237
50. Simonneau G, Torbicki A, Dorfmüller P, Kim N. The pathophysiology of chronic thromboembolic pulmonary hypertension. Eur Respir Rev. (2017) 26(143):160112. doi: 10.1183/16000617.0112-2016
51. Pengo V, Lensing AWA, Prins MH, Marchiori A, Davidson BL, Tiozzo F, et al. Incidence of chronic thromboembolic pulmonary hypertension after pulmonary embolism. N Engl J Med. (2004) 350(22):2257–64. doi: 10.1056/NEJMoa032274
52. Pepke-Zaba J, Delcroix M, Lang I, Mayer E, Jansa P, Ambroz D, et al. Chronic thromboembolic pulmonary hypertension (CTEPH): results from an international prospective registry. Circulation. (2011) 124(18):1973–81. doi: 10.1161/CIRCULATIONAHA.110.015008
53. Mullin CJ, Klinger JR. Chronic thromboembolic pulmonary hypertension. Heart Fail Clin. (2018) 14(3):339–51. doi: 10.1016/j.hfc.2018.02.009
54. Suntharalingam J, Goldsmith K, van Marion V, Long L, Treacy CM, Dudbridge F, et al. Fibrinogen Aalpha Thr312Ala polymorphism is associated with chronic thromboembolic pulmonary hypertension. Eur Respir J. (2008) 31(4):736–41. doi: 10.1183/09031936.00055107
55. Remkova A, Simkova I, Valkovicova T. Platelet abnormalities in chronic thromboembolic pulmonary hypertension. Int J Clin Exp Med. (2015) 8(6):9700–7. PMID: 26309645. PMCID: PMC4538090.26309645
56. Quarck R, Wynants M, Verbeken E, Meyns B, Delcroix M. Contribution of inflammation and impaired angiogenesis to the pathobiology of chronic thromboembolic pulmonary hypertension. Eur Respir J. (2015) 46(2):431–43. doi: 10.1183/09031936.00009914
57. Bonderman D, Wilkens H, Wakounig S, Schäfers HJ, Jansa P, Lindner J, et al. Risk factors for chronic thromboembolic pulmonary hypertension. Eur Respir J. (2009) 33(2):325–31. doi: 10.1183/09031936.00087608
58. Vonk Noordegraaf A, Chin KM, Haddad F, Hassoun PM, Hemnes AR, Hopkins SR, et al. Pathophysiology of the right ventricle and of the pulmonary circulation in pulmonary hypertension: an update. Eur Respir J. (2019) 53(1):1801900. doi: 10.1183/13993003.01900-2018
59. Maron BA, Kovacs G, Vaidya A, Bhatt DL, Nishimura RA, Mak S, et al. Cardiopulmonary hemodynamics in pulmonary hypertension and heart failure: JACC review topic of the week. J Am Coll Cardiol. (2020) 76(22):2671–81. doi: 10.1016/j.jacc.2020.10.007
60. Halliday SJ, Matthews DT, Talati MH, Austin ED, Su YR, Absi TS, et al. A multifaceted investigation into molecular associations of chronic thromboembolic pulmonary hypertension pathogenesis. JRSM Cardiovasc Dis. (2020) 9:2048004020906994. doi: 10.1177/2048004020906994
61. Xu W, Deng M, Meng X, Sun X, Tao X, Wang D, et al. The alterations in molecular markers and signaling pathways in chronic thromboembolic pulmonary hypertension, a study with transcriptome sequencing and bioinformatic analysis. Front Cardiovasc Med. (2022) 9:961305. doi: 10.3389/fcvm.2022.961305
62. Opitz I, Kirschner MB. Molecular research in chronic thromboembolic pulmonary hypertension. Int J Mol Sci. (2019) 20(3):784. doi: 10.3390/ijms20030784
63. Paik S, Kim JK, Silwal P, Sasakawa C, Jo EK. An update on the regulatory mechanisms of NLRP3 inflammasome activation. Cell Mol Immunol. (2021) 18(5):1141–60. doi: 10.1038/s41423-021-00670-3
64. Al-Qazazi R, Lima PDA, Prisco SZ, Potus F, Dasgupta A, Chen KH, et al. Macrophage-NLRP3 activation promotes right ventricle failure in pulmonary arterial hypertension. Am J Respir Crit Care Med. (2022) 206(5):608–24. doi: 10.1164/rccm.202110-2274OC
65. Foley A, Steinberg BE, Goldenberg NM. Inflammasome activation in pulmonary arterial hypertension. Front Med (Lausanne). (2022) 8:826557. doi: 10.3389/fmed.2021.826557
66. Liao X, Han Y, Shen C, Liu J, Wang Y. Targeting the NLRP3 inflammasome for the treatment of hypertensive target organ damage: role of natural products and formulations. Phytother Res. (2023) 37(12):5622–38. doi: 10.1002/ptr.8009
67. Simonneau G, Dorfmüller P, Guignabert C, Mercier O, Humbert M. Chronic thromboembolic pulmonary hypertension: the magic of pathophysiology. Ann Cardiothorac Surg. (2022) 11(2):106–19. doi: 10.21037/acs-2021-pte-10
68. Currie B, Davies E, Beaudet A, Stassek L, Kleinman L. Symptoms, impacts, and suitability of the pulmonary arterial hypertension- symptoms and impact (PAH-SYMPACT) questionnaire in patients with chronic thromboembolic pulmonary hypertension (CTEPH): a qualitative interview study. J Patient Rep Outcomes. (2021) 5(1):51. doi: 10.1186/s41687-021-00327-9
69. Wolf M, Boyer-Neumann C, Parent F, Eschwege V, Jaillet H, Meyer D, et al. Thrombotic risk factors in pulmonary hypertension. Eur Respir J. (2000) 15(2):395–9. doi: 10.1034/j.1399-3003.2000.15b28.x
70. Mandras SA, Mehta HS, Vaidya A. Pulmonary hypertension: a brief guide for clinicians. Mayo Clin Proc. (2020) 95(9):1978–88. doi: 10.1016/j.mayocp.2020.04.039
71. Kim NH, Delcroix M, Jenkins DP, Channick R, Dartevelle P, Jansa P, et al. Chronic thromboembolic pulmonary hypertension. J Am Coll Cardiol. (2013) 62(25 Suppl):D92–9. doi: 10.1016/j.jacc.2013.10.024
72. Simeone B, Maggio E, Schirone L, Rocco E, Sarto G, Spadafora L, et al. Chronic thromboembolic pulmonary hypertension: the therapeutic assessment. Front Cardiovasc Med. (2024) 11:1439411. doi: 10.3389/fcvm.2024.1439411
73. Connors JM. Thrombophilia testing and venous thrombosis. N Engl J Med. (2017) 377(12):1177–87. doi: 10.1056/NEJMra1700365
74. Bonderman D, Wexberg P, Martischnig AM, Heinzl H, Lang MB, Sadushi R, et al. A noninvasive algorithm to exclude pre-capillary pulmonary hypertension. Eur Respir J. (2011) 37(5):1096–103. doi: 10.1183/09031936.00089610
75. Al-Naamani K, Hijal T, Nguyen V, Andrew S, Nguyen T, Huynh T. Predictive values of the electrocardiogram in diagnosing pulmonary hypertension. Int J Cardiol. (2008) 127(2):214–8. doi: 10.1016/j.ijcard.2007.06.005
76. Grapsa J, Carmo Pereira Nunes M, Tan TC, Zimbarra Cabrita I, Coulter T, Smith BCF, et al. Echocardiographic and hemodynamic predictors of survival in precapillary pulmonary hypertension: seven-year follow-up. Circ Cardiovasc Imaging. (2015) 8(6):e002107. doi: 10.1161/CIRCIMAGING.114.002107
77. Held M, Linke M, Jany B. Echocardiography and right heart catheterization in pulmonal hypertension. Dtsch Med Wochenschr. (2014) 139(30):1511–7. doi: 10.1055/s-0034-1370161
78. Konstantinides SV, Meyer G, Becattini C, Bueno H, Geersing GJ, Harjola VP, et al. 2019 ESC guidelines for the diagnosis and management of acute pulmonary embolism developed in collaboration with the European respiratory society (ERS). Eur Heart J. (2020) 41(4):543–603. doi: 10.1093/eurheartj/ehz405
79. Augustine DX, Coates-Bradshaw LD, Willis J, Harkness A, Ring L, Grapsa J, et al. Echocardiographic assessment of pulmonary hypertension: a guideline protocol from the British society of echocardiography. Echo Res Pract. (2018) 5(3):G11–24. doi: 10.1530/ERP-17-0071
80. Woodruff WW 3rd, Hoeck BE, Chitwood WR Jr, Lyerly HK, Sabiston DC Jr, Chen JT. Radiographic findings in pulmonary hypertension from unresolved embolism. AJR Am J Roentgenol. (1985) 144(4):681–6. doi: 10.2214/ajr.144.4.681
81. Remy-Jardin M, Ryerson CJ, Schiebler ML, Leung ANC, Wild JM, Hoeper MM, et al. Imaging of pulmonary hypertension in adults: a position paper from the Fleischner society. Eur Respir J. (2021) 57(1):2004455. doi: 10.1183/13993003.04455-2020
82. Tunariu N, Gibbs SJR, Win Z, Gin-Sing W, Graham A, Gishen P, et al. Ventilation-perfusion scintigraphy is more sensitive than multidetector CTPA in detecting chronic thromboembolic pulmonary disease as a treatable cause of pulmonary hypertension. J Nucl Med. (2007) 48(5):680–4. doi: 10.2967/jnumed.106.039438
83. Quirce R, Ibáñez-Bravo S, Jiménez-Bonilla J, Martínez-Rodríguez I, Martínez-Amador N, Ortega-Nava F, et al. Contribution of V/Q SPECT to planar scintigraphy in the diagnosis of pulmonary embolism. Rev Esp Med Nucl Imagen Mol. (2014) 33(3):153–8. doi: 10.1016/j.remn.2013.12.001
84. Ryan KL, Fedullo PF, Davis GB, Vasquez TE, Moser KM. Perfusion scan findings understate the severity of angiographic and hemodynamic compromise in chronic thromboembolic pulmonary hypertension. Chest. (1988) 93(6):1180–5. doi: 10.1378/chest.93.6.1180
85. Bailey CL, Channick RN, Auger WR, Fedullo PF, Kerr KM, Yung GL, et al. “High probability” perfusion lung scans in pulmonary venoocclusive disease. Am J Respir Crit Care Med. (2000) 162(5):1974–8. doi: 10.1164/ajrccm.162.5.2003045
86. Guérin L, Couturaud F, Parent F, Revel MP, Gillaizeau F, Planquette B, et al. Prevalence of chronic thromboembolic pulmonary hypertension after acute pulmonary embolism. Prevalence of CTEPH after pulmonary embolism. Thromb Haemost. (2014) 112(3):598–605. doi: 10.1160/TH13-07-0538
87. Dong C, Zhou M, Liu D, Long X, Guo T, Kong X. Diagnostic accuracy of computed tomography for chronic thromboembolic pulmonary hypertension: a systematic review and meta-analysis. PLoS One. (2015) 10(4):e0126985. doi: 10.1371/journal.pone.0126985
88. Sherrick AD, Swensen SJ, Hartman TE. Mosaic pattern of lung attenuation on CT scans: frequency among patients with pulmonary artery hypertension of different causes. AJR Am J Roentgenol. (1997) 169(1):79–82. doi: 10.2214/ajr.169.1.9207504
89. Kawakami T, Ogawa A, Miyaji K, Mizoguchi H, Shimokawahara H, Naito T, et al. Novel angiographic classification of each vascular lesion in chronic thromboembolic pulmonary hypertension based on selective angiogram and results of balloon pulmonary angioplasty. Circ Cardiovasc Interv. (2016) 9(10):e003318. doi: 10.1161/CIRCINTERVENTIONS.115.003318
90. Heinrich M, Uder M, Tscholl D, Grgic A, Kramann B, Schäfers HJ. CT scan findings in chronic thromboembolic pulmonary hypertension: predictors of hemodynamic improvement after pulmonary thromboendarterectomy. Chest. (2005) 127(5):1606–13. doi: 10.1378/chest.127.5.1606
91. Rajaram S, Swift AJ, Telfer A, Hurdman J, Marshall H, Lorenz E, et al. 3D contrast-enhanced lung perfusion MRI is an effective screening tool for chronic thromboembolic pulmonary hypertension: results from the ASPIRE registry. Thorax. (2013) 68(7):677–8. doi: 10.1136/thoraxjnl-2012-203020
92. Fukuda T, Ogo T, Nakanishi N, Ueda J, Sanda Y, Morita Y, et al. Evaluation of organized thrombus in distal pulmonary arteries in patients with chronic thromboembolic pulmonary hypertension using cone-beam computed tomography. Jpn J Radiol. (2016) 34(6):423–31. doi: 10.1007/s11604-016-0538-2
93. Hoey ET, Mirsadraee S, Pepke-Zaba J, Jenkins DP, Gopalan D, Screaton NJ. Dual-energy CT angiography for assessment of regional pulmonary perfusion in patients with chronic thromboembolic pulmonary hypertension: initial experience. AJR Am J Roentgenol. (2011) 196(3):524–32. doi: 10.2214/AJR.10.4842
94. Sun XG, Hansen JE, Oudiz RJ, Wasserman K. Exercise pathophysiology in patients with primary pulmonary hypertension. Circulation. (2001) 104(4):429–35. doi: 10.1161/hc2901.093198
95. Weatherald J, Farina S, Bruno N, Laveneziana P. Cardiopulmonary exercise testing in pulmonary hypertension. Ann Am Thorac Soc. (2017) 14(Supplement_1):S84–92. doi: 10.1513/AnnalsATS.201610-788FR
96. Gopalan D, Delcroix M, Held M. Diagnosis of chronic thromboembolic pulmonary hypertension. Eur Respir Rev. (2017) 26(143):160108. doi: 10.1183/16000617.0108-2016
97. Zhu H, Sun X, Cao Y, Pudasaini B, Yang W, Liu J, et al. Cardiopulmonary exercise testing and pulmonary function testing for predicting the severity of CTEPH. BMC Pulm Med. (2021) 21(1):324. doi: 10.1186/s12890-021-01668-3
98. Pezzuto B, Agostoni P. The current role of cardiopulmonary exercise test in the diagnosis and management of pulmonary hypertension. J Clin Med. (2023) 12(17):5465. doi: 10.3390/jcm12175465
99. Held M, Grün M, Holl R, Hübner G, Kaiser R, Karl S, et al. Cardiopulmonary exercise testing to detect chronic thromboembolic pulmonary hypertension in patients with normal echocardiography. Respiration. (2014) 87(5):379–87. doi: 10.1159/000358565
100. Galiè N, Humbert M, Vachiery JL, Gibbs S, Lang I, Torbicki A, et al. 2015 ESC/ERS guidelines for the diagnosis and treatment of pulmonary hypertension: the joint task force for the diagnosis and treatment of pulmonary hypertension of the European Society of Cardiology (ESC) and the European respiratory society (ERS): endorsed by: association for European Paediatric and Congenital Cardiology (AEPC), International Society for Heart and Lung Transplantation (ISHLT). Eur Heart J. (2016) 37(1):67–119. doi: 10.1093/eurheartj/ehv317
101. Klok FA, van der Hulle T, den Exter PL, Lankeit M, Huisman MV, Konstantinides S. The post-PE syndrome: a new concept for chronic complications of pulmonary embolism. Blood Rev. (2014) 28(6):221–6. doi: 10.1016/j.blre.2014.07.003
102. Hoeper MM, Lee SH, Voswinckel R, Palazzini M, Jais X, Marinelli A, et al. Complications of right heart catheterization procedures in patients with pulmonary hypertension in experienced centers. J Am Coll Cardiol. (2006) 48(12):2546–52. doi: 10.1016/j.jacc.2006.07.061
103. Kovacs G, Avian A, Olschewski A, Olschewski H. Zero reference level for right heart catheterisation. Eur Respir J. (2013) 42(6):1586–94. doi: 10.1183/09031936.00050713
104. Viray MC, Bonno EL, Gabrielle ND, Maron BA, Atkins J, Amoroso NS, et al. Role of pulmonary artery wedge pressure saturation during right heart catheterization: a prospective study. Circ Heart Fail. (2020) 13(11):e007981. doi: 10.1161/CIRCHEARTFAILURE.120.007981
105. Simonneau G, Montani D, Celermajer DS, Denton CP, Gatzoulis MA, Krowka M, et al. Haemodynamic definitions and updated clinical classification of pulmonary hypertension. Eur Respir J. (2019) 53(1):1801913. doi: 10.1183/13993003.01913-2018
106. Held M, Hesse A, Gött F, Holl R, Hübner G, Kolb P, et al. A symptom-related monitoring program following pulmonary embolism for the early detection of CTEPH: a prospective observational registry study. BMC Pulm Med. (2014) 14:141. doi: 10.1186/1471-2466-14-141
107. Lang RM, Badano LP, Mor-Avi V, Afilalo J, Armstrong A, Ernande L, et al. Recommendations for cardiac chamber quantification by echocardiography in adults: an update from the American society of echocardiography and the European association of cardiovascular imaging. Eur Heart J Cardiovasc Imaging. (2015) 16(3):233–70. doi: 10.1093/ehjci/jev014
108. Raisinghani A, Ben-Yehuda O. Echocardiography in chronic thromboembolic pulmonary hypertension. Semin Thorac Cardiovasc Surg. (2006) 18(3):230–5. doi: 10.1053/j.semtcvs.2006.09.006
109. Klok FA, Tesche C, Rappold L, Dellas C, Hasenfuß G, Huisman MV, et al. External validation of a simple non-invasive algorithm to rule out chronic thromboembolic pulmonary hypertension after acute pulmonary embolism. Thromb Res. (2015) 135(5):796–801. doi: 10.1016/j.thromres.2014.12.009
110. Klok FA, Surie S, Kempf T, Eikenboom J, van Straalen JP, van Kralingen KW, et al. A simple non-invasive diagnostic algorithm for ruling out chronic thromboembolic pulmonary hypertension in patients after acute pulmonary embolism. Thromb Res. (2011) 128(1):21–6. doi: 10.1016/j.thromres.2011.03.004
111. Luijten D, Meijer FMM, Boon GJAM, Ende-Verhaar YM, Bavalia R, El Bouazzaoui LH, et al. Diagnostic efficacy of ECG-derived ventricular gradient for the detection of chronic thromboembolic pulmonary hypertension in patients with acute pulmonary embolism. J Electrocardiol. (2022) 74:94–100. doi: 10.1016/j.jelectrocard.2022.08.007
112. Boon GJAM, Ende-Verhaar YM, Bavalia R, El Bouazzaoui LH, Delcroix M, Dzikowska-Diduch O, et al. Non-invasive early exclusion of chronic thromboembolic pulmonary hypertension after acute pulmonary embolism: the InShape II study. Thorax. (2021) 76(10):1002–9. doi: 10.1136/thoraxjnl-2020-216324
113. Haramati A, Haramati LB. Imaging of chronic thromboembolic disease. Lung. (2020) 198(2):245–55. doi: 10.1007/s00408-020-00344-3
114. He J, Fang W, Lv B, He JG, Xiong CM, Liu ZH, et al. Diagnosis of chronic thromboembolic pulmonary hypertension: comparison of ventilation/perfusion scanning and multidetector computed tomography pulmonary angiography with pulmonary angiography. Nucl Med Commun. (2012) 33(5):459–63. doi: 10.1097/MNM.0b013e32835085d9
Keywords: pulmonary hypertension, chronic thromboembolic pulmonary hypertension (CTEPH), rare disease, diagnosis, narrative review
Citation: Simeone B, Maggio E, Schirone L, Rocco E, Sarto G, Spadafora L, Bernardi M, Ambrosio LD, Forte M, Vecchio D, Valenti V, Sciarretta S and Vizza CD (2024) Chronic thromboembolic pulmonary hypertension: the diagnostic assessment. Front. Cardiovasc. Med. 11:1439402. doi: 10.3389/fcvm.2024.1439402
Received: 27 May 2024; Accepted: 28 August 2024;
Published: 6 September 2024.
Edited by:
Simona Sperlongano, University of Campania Luigi Vanvitelli, ItalyReviewed by:
Alessandra Cuomo, University of Naples Federico II, ItalyCopyright: © 2024 Simeone, Maggio, Schirone, Rocco, Sarto, Spadafora, Bernardi, Ambrosio, Forte, Vecchio, Valenti, Sciarretta and Vizza. This is an open-access article distributed under the terms of the Creative Commons Attribution License (CC BY). The use, distribution or reproduction in other forums is permitted, provided the original author(s) and the copyright owner(s) are credited and that the original publication in this journal is cited, in accordance with accepted academic practice. No use, distribution or reproduction is permitted which does not comply with these terms.
*Correspondence: Beatrice Simeone, YmVhdHJpY2VzaW1lb25lLm1kQGdtYWlsLmNvbQ==
†These authors have contributed equally to this work and share first authorship
Disclaimer: All claims expressed in this article are solely those of the authors and do not necessarily represent those of their affiliated organizations, or those of the publisher, the editors and the reviewers. Any product that may be evaluated in this article or claim that may be made by its manufacturer is not guaranteed or endorsed by the publisher.
Research integrity at Frontiers
Learn more about the work of our research integrity team to safeguard the quality of each article we publish.