- 1School of Medicine, Tehran University of Medical Sciences, Tehran, Iran
- 2School of Medicine, Shahid Beheshti University of Medical Sciences, Tehran, Iran
- 3School of Medicine, Babol University of Medical Sciences, Babol, Iran
- 4School of Medicine, Shiraz University of Medical Sciences, Shiraz, Iran
- 5Department of Vascular and Endovascular Surgery, Rouhani Hospital, Babol University of Medical Sciences, Babol, Iran
- 6Clinical Research Development Unit of Rouhani Hospital, Babol University of Medical Sciences, Babol, Iran
- 7Department of Cardiology, Babol University of Medical Sciences, Babol, Iran
Background: The arterial stiffness measured by pulsed wave velocity (PWV) is associated with heart failure (HF). However, the effectiveness of arterial stiffness and PWV as prognostic indicators in patients with HFpEF and HFrEF is still unclear. In this systematic review and meta-analysis, we synthesized the prognostic value of PWV and arterial stiffness in HF patients.
Methods: Four databases, including Embase, PubMed, Scopus, and Web of Science, were systematically searched for published studies assessing the relationship between PWV and HF from inception up to August 31, 2023. The Newcastle-Ottawa Scale (NOS) was used to assess the quality of the included studies. The standardized mean difference (SMD) and their corresponding 95% confidence intervals (CI) were used to compare PWV in HF (HFrEF and HFpEF) and controls. Meta-regressions based on age, year of publication, sample size, and gender (male percentage) were also conducted.
Results: The systematic search yielded 5,977 results, of which 58 met our inclusion criteria and 24 were analyzed quantitatively. Studies included 64,687 patients with a mean age of 53.7 years, and 41,803 (67.3%) were male. Meta-analysis of 19 studies showed that PWV was significantly higher in HF patients compared to the controls (SMD 1.04, 95% CI 0.43–1.66, P < 0.001, I2 = 93%). Moreover, nine studies have measured PWV among HFrEF and HFpEF patients and found no significant difference (SMD −0.51, 95% CI −1.03 to 0.02, P = 0.057, I2 = 95%). Moreover, increased PWV was linked to an increased chance of developing new-onset HF in individuals with cardiovascular risk factors.
Conclusions: Patients with HF exhibit significantly higher arterial stiffness, as indicated by PWV, compared to the normal population. However, this association was not significant between HFrEF and HFpEF patients. Future research is warranted to establish the potential prognostic role of PWV in HF.
Systematic Review Registration: https://www.crd.york.ac.uk/prospero/display_record.php?ID=CRD42023479683, PROSPERO (CRD42023479683).
1 Introduction
Heart failure (HF) is a diverse and potentially fatal syndrome impacting over 60 million people worldwide (1). HF is marked by a high rate of mortality and morbidity, a low quality of life, and a heavy financial and resource strain on healthcare systems (1). The already alarming HF epidemic is anticipated to worsen as the population ages (2). Diabetes, hypertension, and ischemic heart disease are the most prevalent etiologies of HF (3, 4), followed by cardiomyopathies and infections such as viral myocarditis and Chagas' disease (5). Two predominant phenotypes of HF based on left ventricular ejection fraction (EF) have been recognized: HF with preserved EF (HFpEF) and HF with reduced EF (HFrEF). These subtypes differ in their underlying pathophysiology, clinical characteristics, and treatment response (6, 7).
Arterial stiffness, an artery's decreased capacity to expand and contract in response to pressure changes, can predict left ventricular (LV) diastolic dysfunction and is associated with cardiovascular risk (8–11). Greater arterial stiffness is connected to LV diastolic dysfunction and HF with preserved EF (12, 13). The pulse wave velocity (PWV) has been acknowledged as the validated test for examining stiffness in large arteries in the consensus document on ventricular-arterial coupling in cardiac disease (14). PWV is determined by dividing the distance between two points by the time it takes for the pulse to travel between them (2). Increased arterial stiffness, as indicated by elevated PWV, is correlated with poor prognosis in several cardiovascular diseases, particularly in the case of HF (15, 16).
HFpEF has not shown positive responses to conventional pharmacological interventions, except nitrate; hence, there is a growing interest in identifying novel prognostic markers and therapeutic targets for HF (2). This systematic review and meta-analysis aimed to assess the effectiveness of arterial stiffness and PWV as prognostic indicators in patients with HFpEF and HFrEF. By synthesizing data from relevant studies, we seek to elucidate the relationship between arterial stiffness, PWV, and different HF phenotypes, providing insights into their potential utility in risk stratification, management, and therapeutic decision-making for HF patients.
2 Materials and methods
This study was written based on the Preferred Reporting Items for Systematic Reviews and Meta-Analyses (PRISMA) 2020 checklist (17). This systematic review and meta-analysis protocol was registered in PROSPERO (CRD42023479683).
2.1 Literature search strategy
Four databases, including Embase (Elsevier), PubMed (US National Library of Medicine), Scopus (Elsevier), and Web of Science, were systematically searched for published studies assessing the relationship between PWV and HF from inception up to August 31, 2023. The search terms we used in this study included: “Pulse Wave Analysis” OR “Ankle-Brachial Pulse Wave Velocit*” OR “Pulse Wave Velocity” AND “Heart Failure, Systolic” OR “Heart Failure” OR “Heart Failure, Diastolic” OR “Heart Failure” OR “Cardiac Failure” OR “Congestive Heart Failure” OR “Heart Failure, Reduced Ejection Fraction” OR “Heart Failure, Preserved Ejection Fraction”. Additional details about the medical subject headings, keywords, and entered terms are presented in Supplementary Table S1.
2.2 Study selection and eligibility criteria
Two reviewers (Z.E. and Z.V.) assessed each record separately using EndNote 21 software (Tomson Reuters, New York, USA). First, duplicates were removed, and records were screened based on their titles and abstracts. Afterward, the full texts of the studies were assessed; selection of studies adhered to the inclusion and exclusion criteria. The third author (A.A.) acted as the facilitator of agreement meetings to address any potential disputes among reviewers.
Studies were included in this review using the following inclusion criteria: (1) subjects were adults (aged ≥18 years), (2) clinical studies that assessed and compared PWV in HF subtypes, (3) studies that assessed PWV in HF patients and compared them with the normal population, (4) papers that reported baseline PWV in the normal population and assessed for probable incident HF, and (5) studies that reported PWV in different stages of HF. Finally, duplicate publications, studies not reporting PWV, animal studies, case reports, abstracts, and reviews were excluded.
2.3 Data extraction and quality assessment
Two reviewers (P.B. and Z.V.) independently extracted the following data from the included studies into a pre-made Excel sheet: first author name, year of publication, study location, study design, sample size, study population (normal or HF), age, gender, and EF. The extracted data were admitted by a third reviewer for probable disparities (A.H.B.).
The Newcastle-Ottawa Scale (NOS) was used to assess the quality of the included studies. The Cochrane Handbook recommends and has created this tool for evaluating the quality of observational studies (18). In cohort studies, there are three key areas to evaluate: selection, comparability, and outcome, with ratings of up to four, two, and three stars; in cross-sectional studies, three aspects were evaluated: selection, comparability, and outcome, with maximus ratings of three, two, and two stars, respectively. A rating of ≥7 is viewed as top quality on this scale. Two separate writers (Z.V. and Z.E.) evaluated the characteristics, and if there was any conflict, a third author (A.K.) settled the matter.
2.4 Statistical analysis
Statistical analyses of this study were conducted using the R program [version 4.3.0]. We used Hedges' g standardized mean difference (SMD) and their corresponding 95% confidence intervals (CI) to compare PWV in HF patients and controls and to compare PWV in different HF subtypes, including HFrEF and HFpEF patients (19). We conducted univariable meta-regression based on age, year of publication, sample size, gender (male percentage), and subgroup analysis among HF subtypes based on the locations and the devices used for PWV assessments. The heterogeneity of studies was assessed using Cochrane's Q and Higgins' I2 tests. There was high inter-study heterogenicity if I2 > 50% and P < 0.1 for the result of the Q test (20). The random-effects model was applied to accommodate the heterogeneity of the enrolled studies (20). P < 0.05 reflected statistical significance for all data analyses. Finally, Egger's statistical test and funnel plot were performed for publication bias (21).
3 Results
3.1 Study selection
The systematic search of electronic databases yielded 5,977 results, including 624 from PubMed, 3,274 from Embase, 1,086 from Web of Science, and 993 from Scopus. After duplicate removal, 2,974 studies remained. Among those, 2,488 records were excluded during the initial screening based on their title and abstract, and 386 records underwent further full-text screening. The full texts of 378 studies were retrieved and went through an eligibility assessment, from which 319 were excluded. Finally, 58 studies met our inclusion criteria and remained for our qualitative evaluation (22–78). Twenty-four studies were analyzed quantitatively. Figure 1 demonstrates the study selection process in detail.
3.2 Baseline characteristics and quality assessment
A summary of the characteristics of the included studies is demonstrated in Table 1. A total of 64,687 patients were included in our study, with sample sizes ranging from 16 to 40,064 participants. Twenty-four studies were conducted in Europe, twenty-four in Asia, nine in America, and one in Africa. The mean age of the population was 53.67 years, and 41,803 (67.27%) were male. The range of mean EF was 21.8 to 68.4%. The carotid-femoral and ankle-brachial arteries were the most frequently assessed anatomical sites for measuring PWV. Carotid-femoral PWV was measured in 25 studies (14, 24, 25, 28, 29, 32–34, 37, 40, 44, 46–49, 51, 53, 54, 57, 61, 65–67, 70, 72, 77, 78), while 13 studies reported ankle-brachial PWV measurements (38–40, 43, 55, 56, 58, 64, 71–75). Of the included studies, 47 had a high-quality score with an overall score of seven or higher based on the NOS tool, while six and five studies scored 6 and 5, respectively (Supplementary Table S2).
3.3 PWV in HF vs. Normal Population
Nineteen studies with a total population of 2,662 patients measured PWV in HF and healthy individuals and were included in the meta-analysis (14, 25, 28–30, 32, 33, 38, 41–43, 46–49, 52, 61, 65, 66). The results showed that PWV was significantly higher in HF patients compared to the controls (SMD 1.04, 95% CI 0.43 to 1.66, P < 0.001), as depicted in the forest plot in Figure 2. Subgroup analysis was conducted based on the location and the devices used for the PWV measurement. As demonstrated in Figure 3, the pooled effect estimates were significant across the carotid-femoral, ankle-brachial, and brachioradial subgroups (SMD 0.92, 95% CI 0.07–1.77; SMD 0.98, 95% CI 0.50–1.46; SMD 2.94, 95% CI 2.22–3.67, respectively). The findings of the analysis based on the devices remained significantly different among studies using SphygmoCor and non-SphygmoCor devices (SMD 0.46, 95% CI 0.17–0.74; SMD 1.79, 95% CI 0.30–3.28) (Supplementary Figure S1).
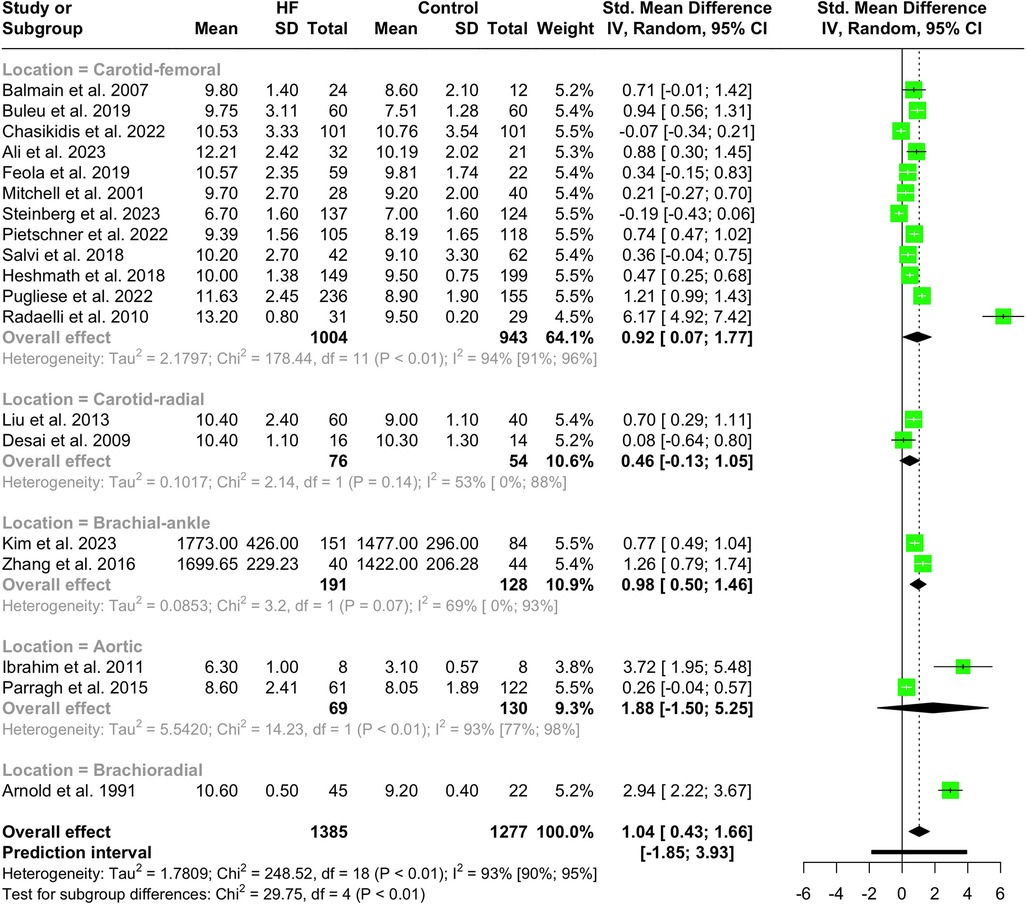
Figure 3 Subgroup analysis of PWV in HF vs. normal population based on the location of PWV measurement.
Addressing high heterogeneity in our initial analysis (I2 93%, 95% CI 90.1%–94.7%, P < 0.01), five outlier studies were omitted (29, 41, 42, 47, 66). The remaining reports, including 1,385 HF patients and 1,277 controls, showed that PWV was significantly higher among HF patients compared to controls (SMD 0.66, 95% CI 0.47–0.85, P < 0.0001, I2 75%) (Supplementary Figure S2). The findings of the sensitivity analysis are demonstrated in Supplementary Figure S3, indicating that the pooled SMD estimate was not significantly modified.
Meta-regression revealed a significant association between gender and the PWV in two groups (β −0.0166, 95% CI −0.0315 to −0.0017], P = 0.02). However, no significant associations were found between the effect size and other investigated variables, including age, year, and sample size (Supplementary Table S3 and Figures S4–S7).
Publication bias was detected when comparing PWV between HF and the normal population, according to the funnel plot asymmetry and Egger's test (P = 0.04). The funnel plot can be observed in Figure 4.
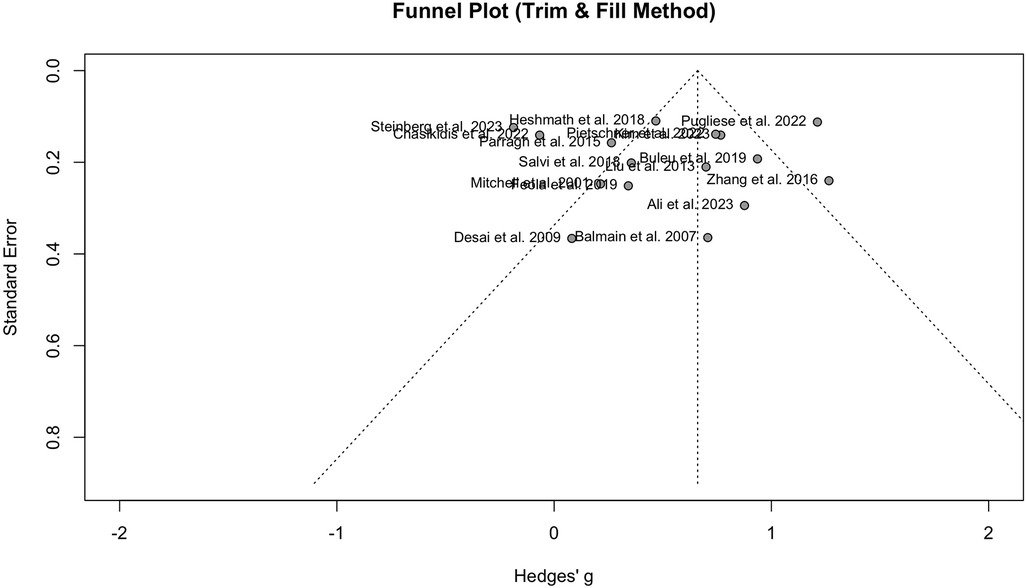
Figure 4 Funnel plot showing the presence of publication bias in the analysis of HF vs. normal population.
3.4 PWV in HFrEF vs. HFpEF
Nine studies with a population of 1,345 participants measured PWV among HFrEF and HFpEF patients. As illustrated in Figure 5, our meta-analysis found only a marginally lower PWV in HFrEF patients, which was not statistically significant (SMD −0.51, 95% CI −1.03 to 0.02, P = 0.057, I2 = 95%). Moreover, no change was observed by removing the identified outlier (65) (SMD −0.27, 95% CI −0.60 to 0.05, P = 0.1, I2 = 81%) (Supplementary Figure S8). The funnel plot shows a symmetrical pattern, and Egger's test did not disclose any publication bias (P = 0.92) (Figure 6). Subgroup analysis according to the ankle-brachial and brachial measurements of PWV found significantly higher values in HFpEF compared to HFrEF patients (SMD −0.58, 95% CI −0.91 to −0.25; SMD −0.49, 95% CI −0.78 to −0.2) (Figure 7). Findings of the subgroup analysis based on the devices used for the PWV measurement are shown in Supplementary Figure S9. The results show a significantly lower PWV in HFrEF patients compared to HFpEF in the subgroup of studies using non-SphygmoCor devices (SMD −0.41, 95% CI −0.66 to −0.16).
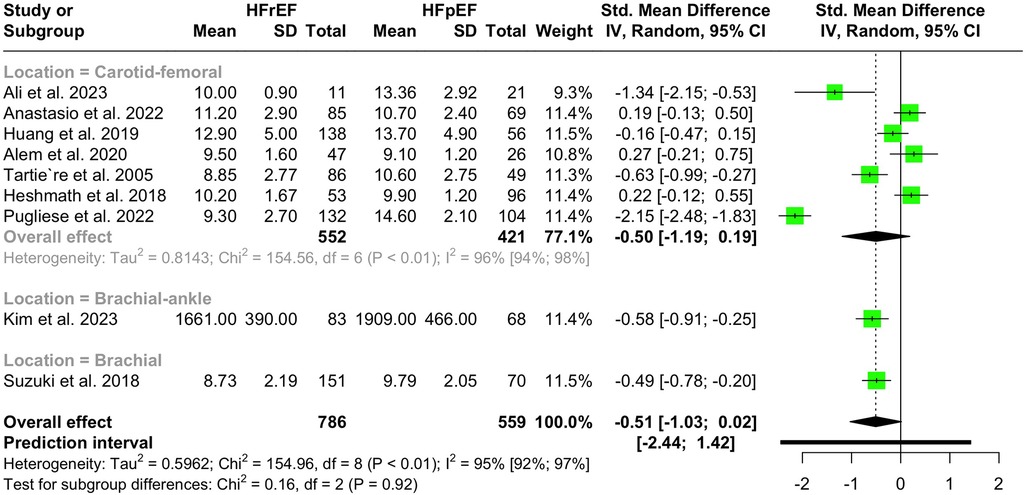
Figure 7 Subgroup analysis of PWV in hFrEF vs. HFpEF patients based on the location of PWV measurement.
Sensitivity analysis yielded significantly reduced pooled SMD by removing three studies, Alem et al. (45), Heshmath et al. (61), and Anastasio et al. (34) (SMD −0.6, 95% CI −1.16 to −0.05; SMD −0.6, 95% CI −1.16 to −0.04; SMD −0.6, 95% CI −1.16 to −0.04; respectively). (Supplementary Figure S10) Meta-regression showed no statistically significant correlation between any investigated moderators and PWV in the two groups (Supplementary Table S4 and Figures S11-S14).
3.5 PWV and adverse outcomes in HF
Thirteen studies have investigated the role of PWV measurements on adverse outcomes in HF patients.
3.5.1 Mortality
Four studies (50, 51, 55, 59) assessed the relationship between PWV and mortality in HF patients. PWV was associated with higher rates of mortality in the study by Demir et al. (50) (OR 1.2, 95% CI 1.04–1.38), Giannitsi et al. (51) (HR 1.32, 95% CI 1.15–1.53), and Regnault et al. (59) (HR 1.16, 95% CI 1.03–1.30). However, Takae et al. (55) found no significant association between mortality and PWV in the HF population.
3.5.2 Mortality and hospitalization
Four studies have explored the correlation of PWV with the composite outcome of death or hospitalization. PWV was associated with higher rates of death or hospitalization reported by Spronck et al. (26) (HR 1.58, 95% CI 1.06–2.36), Bonapace et al. (27) (HR 2.49, 95% CI 1.3–4.6), and Giannitsi et al. (51) (HR 1.12, 95% CI 1.02–1.22). However, no significant association was observed in Dohaei et al.'s investigation (22).
3.5.3 Readmission
The association of PWV with readmission was investigated in two studies (37, 58). PWV was a significant predictor of readmission in the study conducted by Fantin et al. (37) (OR 1.9, 95% CI 1.11–3.44, P = 0.02) and Meguro et al. (58) (HR 5.1, 95% CI 1.034–25.166).
3.5.4 Other outcomes
There was a positive correlation between higher levels of PWV (≥1800cm/s) in HFrEF patients and total cardiovascular events (death, hospitalization, nonfatal MI or stroke, unstable angina, and coronary revascularization) in the study by Takae et al. (55) (HR 6.64, 95% CI 1.66 to 26.4). However, Tokitsu et al.'s (56) investigation on HFpEF patients showed a significant association of total cardiovascular events with both the lowest (<1,300 cm/s) and highest (≥2,200 cm/s) quintiles of PWV (HR 2.88, 95% CI 1.12–7.38; HR 2.56, 95% CI 1.28–5.14; respectively). Two studies conducted by Sung et al. found PWV as a predictor of adverse outcomes, including mortality, rehospitalization, nonfatal MI, and stroke within six months (53) and two years (54) following discharge (HR 1.43, 95% CI 1.02–2.00; HR 1.43, 95% CI 1.12–1.82; respectively). In another study, Anastasio et al. (34) reported that PWV was an independent factor of free-event survival in HF patients with acute decompensation (HR 1.7, 95% CI 1.1–2.7).
3.6 PWV in specific populations
3.6.1 High cardiovascular risk
Aisu et al. (71) and Lee et al. (72) investigated PWV in patients with cardiovascular risk factors (hypertension, obesity, type 2 diabetes mellitus, atrial fibrillation, and ischemic heart disease). Their results show that higher PWV was associated with hospitalization for new-onset HF (brachial-ankle pulse wave velocity (baPWV) (71): HR 1.28, 95% CI 1.04–1.58; carotid-femoral pulse wave velocity (cfPWV) (72): HR 1.29, 95% CI 1.02–1.63).
3.6.2 Dyspneic patients
Two studies have explored PWV in patients with dyspnea without a diagnosis of HF compared to dyspneic HF patients. Cong et al. (73) assessed baPWV in a cohort of patients with acute dyspnea and found that HF patients presented a higher level of baPWV compared to dyspneic patients without HF diagnosis (OR 2.26, 95% CI 1.15–4.44). The same results were obtained by Weber et al. (78) (OR 1.57, 95% CI 1.28–1.93). These studies suggest using PWV as a predictor of HF in symptomatic patients.
3.7 Increased risk of incident HF with a higher PWV
There was a link between higher PWV and the risk of new-onset HF in three studies by Zheng et al. (74) (HR 2.24, 95% CI 1.49–3.38), Heffernan et al. (76) (HR 4.79, 95% CI 2.43–9.45), and Tsao et al. (77) (HR 1.29, 95% CI 1.02–1.64).
4 Discussion
4.1 Main findings
The systematic review and meta-analysis aimed to assess arterial stiffness and PWV in patients with HF and uncovered various vital discoveries. First, the PWV was notably elevated in individuals with HF compared to the control population, mainly when measured in the carotid-femoral and brachial-ankle regions. However, the disparity in PWV between HFrEF and HFpEF was not statistically significant, especially in the carotid-femoral area. Significantly, elevated PWV levels were associated with higher chances of experiencing various cardiovascular events and adverse outcomes, such as death, hospitalization, and readmission. PWV demonstrated the potential to predict new cases of HF in patients experiencing dyspnea, indicating its potential value in detecting HF in symptomatic patients. Moreover, increased PWV was linked to a heightened likelihood of developing new-onset HF in individuals with cardiovascular risk factors, underscoring its importance as a prognostic indicator for HF onset. These results highlight how PWV is crucial as a predictive marker and could be valuable in evaluating cardiovascular risk and treating patients with HF.
Arterial stiffness is a predictor of cardiovascular events and all-cause mortality (15). Since LV diastolic function is also a predictor of increased mortality from HF (79, 80), increased arterial stiffness may contribute to cardiovascular events by causing diastolic dysfunction. The pathophysiology of HFpEF is characterized by diastolic dysfunction, resulting in insufficient ventricular filling during diastole due to impaired ventricular relaxation and increased stiffness. Even though the LV cavity size is usually normal, the LV wall is thick and stiff, leading to a higher LV mass to end-diastolic volume ratio (81). Increased arterial stiffness plays a crucial role in HFpEF, contributing to higher LV pressure afterload. This compromises ventricular-arterial coordination and exacerbates diastolic dysfunction. The rigidity of large elastic arteries decreases their capacity to absorb the rhythmic flow produced by the heart, resulting in higher LV filling pressures and lower aortic pressures in the relaxation phase, intensifying the pulse pressure (82). The rise in pulse pressure heightens the demand for oxygen in the heart muscle during contraction, leading to LV thickening, further hindering blood flow to the coronary arteries during relaxation (83).
In contrast, HFrEF is defined by systolic dysfunction, where the heart muscle's weakened ability to contract leads to a lower ejection fraction and diminished cardiac output. This can result from a heart attack, inflammation of the heart muscle, or other heart conditions causing changes and expansion of the ventricles (84). In HFrEF, arterial stiffness plays a crucial role in the progression of the disease by exacerbating LV systolic dysfunction (85). Increased vascular resistance and reduced compliance, demonstrated by elevated PWV, lead to a compromised oxygen supply-demand balance in the heart, contributing to adverse changes in cardiac structure. The connection between arterial stiffness and diastolic dysfunction is not solely due to the development of LV hypertrophy, as arterial stiffness is also linked to diastolic dysfunction even when ventricular hypertrophy is present (86, 87).
Arterial tonometry is a simple, non-invasive method to assess arterial rigidity that can be conducted at the patient's bedside (88). Various techniques to measure arterial stiffness include carotid-femoral pulse wave velocity (cfPWV), brachial-ankle pulse wave velocity (baPWV), cardio-ankle vascular index (CAVI), and augmentation index (AIx) (89–91). The baPWV is obtained by dividing the distance between the arms and ankles, as determined by anthropometric data depending on a person's height, by the propagation time of the pulse wave between these two points, which is measured using occlusion cuffs (92). The cfPWV is calculated by manually measuring the distance between the carotid and femoral arteries, then dividing by the time it takes for the pulse wave to travel between the two locations (93). The varying influence of PWV measurement sites on outcomes highlights the significance of considering the specific vascular region under evaluation. The carotid-femoral location is commonly seen as the best method for evaluating central arterial stiffness, offering information on the stiffness of major elastic arteries such as the aorta, which are important for dampening pulsatile flow (94). However, baPWV consistently demonstrates a 17%–20% increase compared to cfPWV (95), showing that baPWV assesses further elements of arterial stiffness. cfPWV focuses solely on the central arterial tree, providing insight into central arterial stiffness, which predominantly affects afterload. In contrast, baPWV considers both the central and peripheral arterial trees, providing a more comprehensive understanding of the afterload effects on diastolic dysfunction by reflecting the overall resistance and compliance of the arterial system. This comprehensive measurement may explain the different values obtained by cfPWV and baPWV. While central arteries primarily determine afterload, peripheral arteries become significant, especially when peripheral arterial disease is present (9). Additional factors that could account for the significant variability in this research may stem from the increased reliance on the person administering the test for cfPWV compared to baPWV, as well as the required use of a handheld pressure transducer to measure pulse waves at the neck and groin (96). Also, the lack of significant differences in PWV between HFrEF and HFpEF, particularly in the carotid-femoral location, may suggest that systemic arterial stiffness is a shared characteristic across both HF phenotypes. A similar systematic meta-analysis was conducted to study the association between arterial stiffness assessed by arterial tonometry and echocardiographic markers of diastolic dysfunction, which is essential for diagnosing heart failure with preserved ejection fraction (HFpEF). Twenty-seven studies included 6,626 patients. baPWV showed significant correlations with the E/A ratio, e0, and E/e0 ratio. Similarly, cfPWV was significantly correlated with the E/A and the E/e0 ratios, but not e0. AIx showed a strong relationship with E/A ratio (r = −0.356, 95% CI −0.255 to −0.450), e0 (r = −0.313, 95% CI −0.195 to −0.423), and E/e0 ratio (r = 0.321, 95% CI 0.250–0.388). CAVI had a strong correlation with the E/A ratio, e0, and baPWV showed a significant correlation with diastolic dysfunction compared to other tonometry techniques (97).
5 Strengths and limitations
Adding a significant number of research studies involving 64,687 patients increased the statistical strength and reliability of the meta-analysis. A significant percentage of the studies analyzed were rated as high quality based on their Newcastle-Ottawa Scale (NOS) scores. This enhances the credibility and accuracy of the results. Subgroup analyses were conducted to investigate possible reasons for diversity, like different locations for PWV measurement and HF subtype (HFpEF vs. HFrEF), as well as the devices used for the PWV measurement, leading to a more detailed understanding of the findings. Meta-regression analysis was used to examine how gender, age, and sample size affect observed associations, improving comprehension of potential moderators.
This study carries some limitations. Although attempts were made to deal with differences through subgroup analyses and sensitivity analyses, numerous meta-analyses still showed significant heterogeneity, which may restrict the applicability of the results. Differences in research structure, patient traits, and methods, like variations in PWV measurement methods and anatomical locations, HF subtype (HFpEF vs. HFrEF), and disease severity, probably played a part in the diversity. Also, we could not analyze PWV based on the blood pressure levels, as this information was not sufficiently reported in the included studies. Future research should aim to collect and report BP data to allow for a more comprehensive analysis. Even though the majority of the studies analyzed were well-conducted, a few had methodological flaws that may have influenced the results of the meta-analysis.
6 Conclusion
In conclusion, our systematic review and meta-analysis revealed that patients with HF exhibit significantly higher arterial stiffness, as indicated by PWV, compared to the normal population. This association was consistent across various anatomical sites of PWV measurement, including carotid-femoral and ankle-brachial arteries. Furthermore, PWV was found to be a predictor of adverse outcomes in HF patients, including mortality, hospitalization, and readmission. Although there was no statistically significant difference in PWV between patients with HFrEF and HFpEF, subgroup analyses indicated potential differences based on the site of PWV measurement. Additionally, PWV was associated with adverse outcomes in high-risk cardiovascular populations and individuals with dyspnea, underscoring its potential utility as a predictive tool for HF development. Future research should focus on elucidating the underlying mechanisms linking PWV to adverse outcomes in HF patients and exploring its role in risk stratification and therapeutic interventions to improve patient outcomes.
Data availability statement
The original contributions presented in the study are included in the article/Supplementary Material, further inquiries can be directed to the corresponding author.
Author contributions
ZE: Data curation, Investigation, Methodology, Project administration, Writing – original draft, Writing – review & editing. PB: Data curation, Investigation, Methodology, Project administration, Supervision, Writing – original draft, Writing – review & editing. ZV: Data curation, Investigation, Project administration, Writing – original draft, Writing – review & editing. AA: Supervision, Validation, Writing – original draft, Writing – review & editing. AHB: Data curation, Formal Analysis, Methodology, Supervision, Writing – original draft, Writing – review & editing. AK: Data curation, Writing – original draft, Writing – review & editing. AB: Writing – original draft, Writing – review & editing. PT: Conceptualization, Writing – original draft, Writing – review & editing. NZ: Conceptualization, Writing – original draft, Writing – review & editing.
Funding
The author(s) declare that no financial support was received for the research, authorship, and/or publication of this article.
Conflict of interest
The authors declare that the research was conducted in the absence of any commercial or financial relationships that could be construed as a potential conflict of interest.
Publisher's note
All claims expressed in this article are solely those of the authors and do not necessarily represent those of their affiliated organizations, or those of the publisher, the editors and the reviewers. Any product that may be evaluated in this article, or claim that may be made by its manufacturer, is not guaranteed or endorsed by the publisher.
Supplementary material
The Supplementary Material for this article can be found online at: https://www.frontiersin.org/articles/10.3389/fcvm.2024.1435677/full#supplementary-material
References
1. Savarese G, Becher PM, Lund LH, Seferovic P, Rosano GMC, Coats AJS. Global burden of heart failure: a comprehensive and updated review of epidemiology. Cardiovasc Res. (2022) 118(17):3272–87. doi: 10.1093/cvr/cvac013
2. Marti CN, Gheorghiade M, Kalogeropoulos AP, Georgiopoulou VV, Quyyumi AA, Butler J. Endothelial dysfunction, arterial stiffness, and heart failure. J Am Coll Cardiol. (2012) 60(16):1455–69. doi: 10.1016/j.jacc.2011.11.082
3. Frâncu V, Veștemean I. Assessment of the programme of promoting a healthy lifestyle in adults. Part I. Acta Med Transil. (2013) 18(2):183–6.
4. Roger VL, Go AS, Lloyd-Jones DM, Adams RJ, Berry JD, Brown TM, et al. Heart disease and stroke statistics—2011 update: a report from the American Heart Association. Circulation. (2011) 123(4):e18–e209. doi: 10.1161/CIR.0b013e3182009701
5. Kemp CD, Conte JV. The pathophysiology of heart failure. Cardiovasc Pathol. (2012) 21(5):365–71. doi: 10.1016/j.carpath.2011.11.007
6. Ohtani T, Mohammed SF, Yamamoto K, Dunlay SM, Weston SA, Sakata Y, et al. Diastolic stiffness as assessed by diastolic wall strain is associated with adverse remodelling and poor outcomes in heart failure with preserved ejection fraction. Eur Heart J. (2012) 33(14):1742–9. doi: 10.1093/eurheartj/ehs135
7. Behnoush AH, Khalaji A, Naderi N, Ashraf H, von Haehling S. Acc/Aha/Hfsa 2022 and esc 2021 guidelines on heart failure comparison. ESC Heart Fail. (2023) 10(3):1531–44. doi: 10.1002/ehf2.14255
8. Cecelja M, Chowienczyk P. Role of arterial stiffness in cardiovascular disease. JRSM Cardiovasc Dis. (2012) 1(4):1–10. doi: 10.1258/cvd.2012.012016
9. Chow B, Rabkin SW. Brachial-ankle pulse wave velocity is the only index of arterial stiffness that correlates with a mitral valve indices of diastolic dysfunction, but no index correlates with left atrial size. Cardiol Res Pract. (2013) 2013:986847. doi: 10.1155/2013/986847
10. Chung C-M, Chang S-T, Cheng H-W, Yang T-Y, Wan P-C, Pan K-L, et al. Quantification of aortic stiffness to predict the degree of left ventricular diastolic function. Am J Med Sci. (2010) 340(6):468–73. doi: 10.1097/MAJ.0b013e3181f0142c
11. Polak JF, Pencina MJ, Pencina KM, O'Donnell CJ, Wolf PA, D'Agostino Sr RB. Carotid-Wall intima–Media thickness and cardiovascular events. N Engl J Med. (2011) 365(3):213–21. doi: 10.1056/NEJMoa1012592
12. Abhayaratna WP, Barnes ME, O’Rourke MF, Gersh BJ, Seward JB, Miyasaka Y, et al. Relation of arterial stiffness to left ventricular diastolic function and cardiovascular risk prediction in patients≥ 65 years of age. Am J Cardiol. (2006) 98(10):1387–92. doi: 10.1016/j.amjcard.2006.06.035
13. Mottram PM, Haluska BA, Leano R, Carlier S, Case C, Marwick TH. Relation of arterial stiffness to diastolic dysfunction in hypertensive heart disease. Heart. (2005) 91(12):1551–6. doi: 10.1136/hrt.2004.046805
14. Feola M, Testa M, Ferreri C, Rosso G, Rossi A, Ruocco G. The analysis of arterial stiffness in heart failure patients in comparison with healthy subjects and patients with cardiovascular risk factors. J Clin Med. (2019) 8(10):1721. doi: 10.3390/jcm8101721
15. Vlachopoulos C, Aznaouridis K, Stefanadis C. Prediction of cardiovascular events and all-cause mortality with arterial stiffness: a systematic review and meta-analysis. J Am Coll Cardiol. (2010) 55(13):1318–27. doi: 10.1016/j.jacc.2009.10.061
16. Ben-Shlomo Y, Spears M, Boustred C, May M, Anderson SG, Benjamin EJ, et al. Aortic pulse wave velocity improves cardiovascular event prediction: an individual participant meta-analysis of prospective observational data from 17,635 subjects. J Am Coll Cardiol. (2014) 63(7):636–46. doi: 10.1016/j.jacc.2013.09.063
17. Page MJ, McKenzie JE, Bossuyt PM, Boutron I, Hoffmann TC, Mulrow CD, et al. The prisma 2020 statement: an updated guideline for reporting systematic reviews. Syst Rev. (2021) 10(1):89. doi: 10.1186/s13643-021-01626-4
18. Lo CK, Mertz D, Loeb M. Newcastle-Ottawa scale: comparing Reviewers’ to Authors’ assessments. BMC Med Res Methodol. (2014) 14:45. doi: 10.1186/1471-2288-14-45
19. Lin L, Aloe AM. Evaluation of Various estimators for standardized mean difference in meta-analysis. Stat Med. (2021) 40(2):403–26. doi: 10.1002/sim.8781
20. Higgins JP, Thompson SG, Deeks JJ, Altman DG. Measuring inconsistency in meta-analyses. Br Med J. (2003) 327(7414):557–60. doi: 10.1136/bmj.327.7414.557
21. Egger M, Davey Smith G, Schneider M, Minder C. Bias in meta-analysis detected by a simple, graphical test. Br Med J. (1997) 315(7109):629–34. doi: 10.1136/bmj.315.7109.629
22. Dohaei A, Taghavi S, Amin A, Rahimi S, Naderi N. Does aortic pulse wave velocity have any prognostic significance in advanced heart failure patients? J Cardiovasc Thorac Res. (2017) 9(1):35–40. doi: 10.15171/jcvtr.2017.05
23. El Fol A, Ammar W, Sharaf Y, Youssef G. The central arterial stiffness parameters in decompensated versus compensated states of heart failure: a paired comparative cohort study. Egypt Heart J. (2022) 74(1):2. doi: 10.1186/s43044-021-00236-8
24. Shah A, Gkaliagkousi E, Ritter JM, Ferro A. Endothelial function and arterial compliance are not impaired in subjects with heart failure of non-ischemic origin. J Card Fail. (2010) 16(2):114–20. doi: 10.1016/j.cardfail.2009.10.019
25. Balmain S, Padmanabhan N, Ferrell WR, Morton JJ, McMurray JJ. Differences in arterial compliance, microvascular function and venous capacitance between patients with heart failure and either preserved or reduced left ventricular systolic function. Eur J Heart Fail. (2007) 9(9):865–71. doi: 10.1016/j.ejheart.2007.06.003
26. Spronck B, Obeid MJ, Paravathaneni M, Gadela NV, Singh G, Magro CA, et al. Predictive ability of pressure-corrected arterial stiffness indices: comparison of pulse wave velocity, cardio-ankle vascular Index (cavi), and cavio. Am J Hypertens. (2021) 35(3):272–80. doi: 10.1093/ajh/hpab168
27. Bonapace S, Rossi A, Cicoira M, Targher G, Valbusa F, Benetos A, et al. Increased aortic pulse wave velocity as measured by echocardiography is strongly associated with poor prognosis in patients with heart failure. J Am Soc Echocardiogr. (2013) 26(7):714–20. doi: 10.1016/j.echo.2013.03.022
28. Buleu FN, Luca CT, Tudor A, Badalica-Petrescu M, Caraba A, Pah A, et al. Correlations between vascular stiffness indicators, opg, and 25-oh vitamin D3 status in heart failure patients. Medicina (Kaunas). (2019) 55(6):309. doi: 10.3390/medicina55060309
29. Chasikidis C, Oikonomou E, Lazaros G, Christoforatou E, Vogiatzi G, Chrysohoou C, et al. Association of arterial stiffness with functional parameters in patients with systolic heart failure: data from the corinthia study. Hellenic J Cardiol. (2022) 63:86–8. doi: 10.1016/j.hjc.2021.04.005
30. Liu C, Zheng D, Zhao L, Li P, Li B, Murray A, et al. Elastic properties of peripheral arteries in heart failure patients in comparison with normal subjects. J Physiol Sci. (2013) 63(3):195–201. doi: 10.1007/s12576-013-0254-y
31. Coksevim M, Akcay M, Yuksel S, Yenercag M, Cerik B, Gedikli O, et al. The effect of cardiac resynchronization therapy on arterial stiffness and central hemodynamic parameters. J Arrhythm. (2020) 36(3):498–507. doi: 10.1002/joa3.12331
32. Ali D, Tran P, Ennis S, Powell R, McGuire S, McGregor G, et al. Rising arterial stiffness with accumulating comorbidities associates with heart failure with preserved ejection fraction. ESC Heart Fail. (2023) 10(4):2487–98. doi: 10.1002/ehf2.14422
33. Desai AS, Mitchell GF, Fang JC, Creager MA. Central aortic stiffness is increased in patients with heart failure and preserved ejection fraction. J Card Fail. (2009) 15(8):658–64. doi: 10.1016/j.cardfail.2009.03.006
34. Anastasio F, Testa M, Ferreri C, Rossi A, Ruocco G, Feola M. The analysis of arterial stiffness in heart failure patients: the prognostic role of pulse wave velocity, augmentation Index and stiffness Index. J Clin Med. (2022) 11(12):3507. doi: 10.3390/jcm11123507
35. Fehérvári L, Szabo I, Kocsis L, Frigy A. Evaluation of arterial stiffness in systolic heart failure. Acta Marisiensis—Seria Medica. (2021) 67:86–9. doi: 10.2478/amma-2021-0009
36. Fehérvári L, Frigy A, Kocsis L, Szabó IA, Szabo TM, Urkon M, et al. Serum osteoprotegerin and carotid intima-Media thickness are related to high arterial stiffness in heart failure with reduced ejection fraction. Diagnostics (Basel). (2021) 11(5):764. doi: 10.3390/diagnostics11050764
37. Fantin F, Giani A, Franconi A, Zoico E, Urbani S, Rossi AP, et al. Arterial stiffness, subendocardial impairment, and 30-day readmission in heart failure older patients. Front Cardiovasc Med. (2022) 9:918601. doi: 10.3389/fcvm.2022.918601
38. Kim HL, Chung J, Han S, Joh HS, Lim WH, Seo JB, et al. Arterial stiffness and its associations with left ventricular diastolic function according to heart failure types. Clin Hypertens. (2023) 29(1):8. doi: 10.1186/s40885-022-00233-2
39. Hsu PC, Lin TH, Lee CS, Lee HC, Chu CY, Su HM, et al. Mismatch between arterial stiffness increase and left ventricular diastolic dysfunction. Heart Vessels. (2010) 25(6):485–92. doi: 10.1007/s00380-010-0021-4
40. Huang WM, Sung SH, Yu WC, Cheng HM, Huang CJ, Guo CY, et al. Perturbations of pulsatile hemodynamics and clinical outcomes in patients with acute heart failure and reduced, mid-range or preserved ejection fraction. PLoS One. (2019) 14(8):e0220183. doi: 10.1371/journal.pone.0220183
41. Ibrahim el SH, Miller AB, White RD. The relationship between aortic stiffness and E/a filling ratio and myocardial strain in the context of left ventricular diastolic dysfunction in heart failure with normal ejection fraction: insights from magnetic resonance imaging. Magn Reson Imaging. (2011) 29(9):1222–34. doi: 10.1016/j.mri.2011.08.003
42. Arnold JM, Marchiori GE, Imrie JR, Burton GL, Pflugfelder PW, Kostuk WJ. Large artery function in patients with chronic heart failure. Studies of brachial artery diameter and hemodynamics. Circulation. (1991) 84(6):2418–25. doi: 10.1161/01.cir.84.6.2418
43. Zhang J, Deng M, He S, Zhou W. Relationship between brachial-ankle pulse wave velocity and early mild diastolic heart failure in elderly hypertensive Chinese patients. Int J Clin Exp Med. (2016) 9:3344–52.
44. Kim DB, Baek SH, Jang SW, Her SH, Shin DI, Park CS, et al. Improvement of arterial stiffness in the transition from acute decompensated heart failure to chronic compensated heart failure. Clin Cardiol. (2013) 36(6):358–62. doi: 10.1002/clc.22127
45. Alem MM, Alshehri AM. Inter-Relationships between left ventricular mass, geometry and arterial stiffness. J Int Med Res. (2020) 48(4):300060520903623. doi: 10.1177/0300060520903623
46. Mitchell GF, Tardif JC, Arnold JM, Marchiori G, O'Brien TX, Dunlap ME, et al. Pulsatile hemodynamics in congestive heart failure. Hypertension. (2001) 38(6):1433–9. doi: 10.1161/hy1201.098298
47. Steinberg RS, Udeshi E, Dickert N, Quyyumi A, Chirinos JA, Morris AA. Novel measures of arterial hemodynamics and wave reflections associated with clinical outcomes in patients with heart failure. J Am Heart Assoc. (2023) 12(6):e027666. doi: 10.1161/jaha.122.027666
48. Pietschner R, Bosch A, Kannenkeril D, Striepe K, Schiffer M, Achenbach S, et al. Is vascular remodelling in patients with chronic heart failure exaggerated? ESC Heart Fail. (2023) 10(1):245–54. doi: 10.1002/ehf2.14174
49. Salvi P, Grillo A, Tan I, Simon G, Salvi L, Gao L, et al. Systolic time intervals assessed from analysis of the carotid pressure waveform. Physiol Meas. (2018) 39(8):084002. doi: 10.1088/1361-6579/aad51b
50. Demir S, Akpınar O, Akkus O, Nas K, Unal I, Molnar F, et al. The prognostic value of arterial stiffness in systolic heart failure. Cardiol J. (2013) 20(6):665–71. doi: 10.5603/cj.2013.0168
51. Giannitsi S, Bougiakli M, Bechlioulis A, Kotsia A, Lakkas L, Girdis I, et al. The prognostic role of aortic stiffness in patients hospitalized for an acute heart failure syndrome. Artery Res. (2021) 27:7–13. doi: 10.2991/artres.k.200930.001
52. Parragh S, Hametner B, Bachler M, Kellermair J, Eber B, Wassertheurer S, et al. Determinants and covariates of central pressures and wave reflections in systolic heart failure. Int J Cardiol. (2015) 190:308–14. doi: 10.1016/j.ijcard.2015.04.183
53. Sung SH, Yu WC, Cheng HM, Chuang SY, Wang KL, Huang CM, et al. Pulsatile hemodynamics and clinical outcomes in acute heart failure. Am J Hypertens. (2011) 24(7):775–82. doi: 10.1038/ajh.2011.26
54. Sung SH, Yu WC, Cheng HM, Lee CW, Lin MM, Chuang SY, et al. Excessive wave reflections on admission predict post-discharge events in patients hospitalized due to acute heart failure. Eur J Heart Fail. (2012) 14(12):1348–55. doi: 10.1093/eurjhf/hfs124
55. Takae M, Yamamoto E, Tokitsu T, Oike F, Nishihara T, Fujisue K, et al. Clinical significance of brachial-ankle pulse wave velocity in patients with heart failure with reduced left ventricular ejection fraction. Am J Hypertens. (2019) 32(7):657–67. doi: 10.1093/ajh/hpz048
56. Tokitsu T, Yamamoto E, Oike F, Hirata Y, Tsujita K, Yamamuro M, et al. Clinical significance of brachial-ankle pulse-wave velocity in patients with heart failure with preserved left ventricular ejection fraction. J Hypertens. (2018) 36(3):560–8. doi: 10.1097/hjh.0000000000001589
57. Tartière JM, Logeart D, Safar ME, Cohen-Solal A. Interaction between pulse wave velocity, augmentation index, pulse pressure and left ventricular function in chronic heart failure. J Hum Hypertens. (2006) 20(3):213–9. doi: 10.1038/sj.jhh.1001965
58. Meguro T, Nagatomo Y, Nagae A, Seki C, Kondou N, Shibata M, et al. Elevated arterial stiffness evaluated by brachial-ankle pulse wave velocity is deleterious for the prognosis of patients with heart failure. Circ J. (2009) 73(4):673–80. doi: 10.1253/circj.cj-08-0350
59. Regnault V, Lagrange J, Pizard A, Safar ME, Fay R, Pitt B, et al. Opposite predictive value of pulse pressure and aortic pulse wave velocity on heart failure with reduced left ventricular ejection fraction: insights from an eplerenone post-acute myocardial infarction heart failure efficacy and survival study (ephesus) substudy. Hypertension. (2014) 63(1):105–11. doi: 10.1161/hypertensionaha.113.02046
60. Ryabov V. Arterial stiffness in patients with heart failure and preserved ejection fraction 12 months after myocardial infarction. Polski Przeglad Kardiologiczny. (2012) 14:165–72.
61. Hashmath Z, Lee J, Gaddam S, Ansari B, Oldland G, Javaid K, et al. Vitamin K Status, warfarin use, and arterial stiffness in heart failure. Hypertension. (2019) 73(2):364–70. doi: 10.1161/hypertensionaha.118.12157
62. Bonapace S, Rossi A, Cicoira M, Targher G, Marino P, Benfari G, et al. Echocardiographically derived pulse wave velocity and diastolic dysfunction are associated with an increased incidence of atrial fibrillation in patients with systolic heart failure. Echocardiography. (2016) 33(7):1024–31. doi: 10.1111/echo.13230
63. Kaiser DR, Mullen K, Bank AJ. Brachial artery elastic mechanics in patients with heart failure. Hypertension. (2001) 38(6):1440–5. doi: 10.1161/hy1201.096539
64. Sun M, Gao L, Bai H, Hu W, Zhang X, Xiao J, et al. Association between visceral fat, blood pressure and arterial stiffness in patients with hfpef: a mediation analysis. Diabetes Metab Syndr Obes. (2023) 16:653–62. doi: 10.2147/dmso.S399928
65. Pugliese NR, Balletti A, Armenia S, De Biase N, Faita F, Mengozzi A, et al. Ventricular-Arterial coupling derived from proximal aortic stiffness and aerobic capacity across the heart failure Spectrum. JACC Cardiovasc Imaging. (2022) 15(9):1545–59. doi: 10.1016/j.jcmg.2022.03.024
66. Radaelli A, Castiglioni P, Balestri G, Cesana F, De Carlini C, Soriano F, et al. Increased pulse wave velocity and not reduced ejection fraction is associated with impaired baroreflex control of heart rate in congestive heart failure. J Hypertens. (2010) 28(9):1908–12. doi: 10.1097/HJH.0b013e32833c2088
67. Radaelli A, Mancia G, Balestri G, Rovati A, Anzuini A, Di Rienzo M, et al. Cardiovascular variability is similarly altered in coronary patients with normal left ventricular function and in heart failure patients. J Hypertens. (2014) 32(11):2261–6. doi: 10.1097/hjh.0000000000000312
68. Suzuki S, Yoshihisa A, Sato Y, Watanabe S, Yokokawa T, Sato T, et al. Association between sleep-disordered breathing and arterial stiffness in heart failure patients with reduced or preserved ejection fraction. ESC Heart Fail. (2018) 5(3):284–91. doi: 10.1002/ehf2.12273
69. Tokitsu T, Yamamoto E, Hirata Y, Kusaka H, Fujisue K, Sueta D, et al. Clinical significance of pulse pressure in patients with heart failure with preserved left ventricular ejection fraction. Eur J Heart Fail. (2016) 18(11):1353–61. doi: 10.1002/ejhf.559
70. Trembach N, Zabolotskikh I. Arterial baroreflex sensitivity: relationship with peripheral chemoreflex in patients with chronic heart failure. Artery Res. (2018) 24:9–15. doi: 10.1016/j.artres.2018.10.002
71. Aisu H, Saito M, Inaba S, Morofuji T, Takahashi K, Sumimoto T, et al. Association of worsening arterial stiffness with incident heart failure in asymptomatic patients with cardiovascular risk factors. Hypertens Res. (2017) 40(2):173–80. doi: 10.1038/hr.2016.116
72. Lee CJ, Yoon M, Ha J, Oh J, Park S, Lee SH, et al. Comparison of the association between arterial stiffness indices and heart failure in patients with high cardiovascular risk: a retrospective study. Front Cardiovasc Med. (2021) 8:782849. doi: 10.3389/fcvm.2021.782849
73. Cong T, Jiang S, Wang K, Zhong L, Wu J, Su D. Predictive value of brachial-ankle artery pulse wave velocity to heart failure with preserved ejection fraction in hospitalised patients with acute dyspnoea. Pak J Med Sci. (2015) 31(3):516–21. doi: 10.12669/pjms.313.6833
74. Zheng H, Wu S, Liu X, Qiu G, Chen S, Wu Y, et al. Association between arterial stiffness and new-onset heart failure: the kailuan study. Arterioscler Thromb Vasc Biol. (2023) 43(2):e104–e11. doi: 10.1161/atvbaha.122.317715
75. Kang S, Fan HM, Li J, Fan LY, Miao AY, Bao Y, et al. Relationship of arterial stiffness and early mild diastolic heart failure in general middle and aged population. Eur Heart J. (2010) 31(22):2799–807. doi: 10.1093/eurheartj/ehq296
76. Heffernan KS, Charry D, Xu J, Tanaka H, Churilla JR. Estimated pulse wave velocity and incident heart failure and its subtypes: findings from the multi-ethnic study of atherosclerosis. Am Heart J Plus. (2023) 25:100238. doi: 10.1016/j.ahjo.2022.100238
77. Tsao CW, Lyass A, Larson MG, Levy D, Hamburg NM, Vita JA, et al. Relation of central arterial stiffness to incident heart failure in the community. J Am Heart Assoc. (2015) 4(11):e002189. doi: 10.1161/jaha.115.002189
78. Weber T, Wassertheurer S, O'Rourke MF, Haiden A, Zweiker R, Rammer M, et al. Pulsatile hemodynamics in patients with exertional dyspnea: potentially of value in the diagnostic evaluation of suspected heart failure with preserved ejection fraction. J Am Coll Cardiol. (2013) 61(18):1874–83. doi: 10.1016/j.jacc.2013.02.013
79. Kane GC, Karon BL, Mahoney DW, Redfield MM, Roger VL, Burnett JC Jr, et al. Progression of left ventricular diastolic dysfunction and risk of heart failure. JAMA. (2011) 306(8):856–63. doi: 10.1001/jama.2011.1201
80. Lam CS, Lyass A, Kraigher-Krainer E, Massaro JM, Lee DS, Ho JE, et al. Cardiac dysfunction and noncardiac dysfunction as precursors of heart failure with reduced and preserved ejection fraction in the community. Circulation. (2011) 124(1):24–30. doi: 10.1161/circulationaha.110.979203
81. Youn JC, Ahn Y, Jung HO. Pathophysiology of heart failure with preserved ejection fraction. Heart Fail Clin. (2021) 17(3):327–35. doi: 10.1016/j.hfc.2021.02.001
82. Tartière-Kesri L, Tartière JM, Logeart D, Beauvais F, Cohen Solal A. Increased proximal arterial stiffness and cardiac response with moderate exercise in patients with heart failure and preserved ejection fraction. J Am Coll Cardiol. (2012) 59(5):455–61. doi: 10.1016/j.jacc.2011.10.873
83. Rabkin SW, Chan SH. Correlation of pulse wave velocity with left ventricular mass in patients with hypertension once blood pressure has been normalized. Heart Int. (2012) 7(1):e5. doi: 10.4081/hi.2012.e5
84. Ge Z, Li A, McNamara J, Dos Remedios C, Lal S. Pathogenesis and pathophysiology of heart failure with reduced ejection fraction: translation to human studies. Heart Fail Rev. (2019) 24(5):743–58. doi: 10.1007/s10741-019-09806-0
85. Feola M. The influence of arterial stiffness in heart failure: a clinical review. J Geriatr Cardiol. (2021) 18(2):135–40. doi: 10.11909/j.issn.1671-5411.2021.02.004
86. Takami T, Saito Y. Azelnidipine plus olmesartan versus amlodipine plus olmesartan on arterial stiffness and cardiac function in hypertensive patients: a randomized trial. Drug Des Devel Ther. (2013) 7:175–83. doi: 10.2147/dddt.S42338
87. Wang CP, Hung WC, Yu TH, Hsu HL, Chen YH, Chiu CA, et al. Brachial-Ankle pulse wave velocity as an early indicator of left ventricular diastolic function among hypertensive subjects. Clin Exp Hypertens. (2009) 31(1):31–43. doi: 10.1080/10641960802409796
88. DeLoach SS, Townsend RR. Vascular stiffness: its measurement and significance for epidemiologic and outcome studies. Clin J Am Soc Nephrol. (2008) 3(1):184–92. doi: 10.2215/CJN.03340807
89. Asmar R, Topouchian J, Pannier B, Benetos A, Safar M. Pulse wave velocity as endpoint in large-scale intervention trial. The complior® study. J Hypertens. (2001) 19(4):813–8. doi: 10.1097/00004872-200104000-00019
90. Nakae I, Matsuo S, Matsumoto T, Mitsunami K, Horie M. Augmentation Index and pulse wave velocity as indicators of cardiovascular stiffness. Angiology. (2008) 59(4):421–6. doi: 10.1177/0003319707306299
91. Takaki A, Ogawa H, Wakeyama T, Iwami T, Kimura M, Hadano Y, et al. Cardio-ankle vascular index is superior to brachial-ankle pulse wave velocity as an Index of arterial stiffness. Hypertens Res. (2008) 31(7):1347–55. doi: 10.1291/hypres.31.1347
92. Kim HL, Jeon WK, Joh HS, Lim WH, Seo JB, Kim SH, et al. Brachial-Ankle pulse wave velocity as a predictor of long-term cardiovascular events in 2174 subjects with type 2 diabetes mellitus: a retrospective cohort study. Medicine (Baltimore). (2022) 101(45):e31758. doi: 10.1097/md.0000000000031758
93. Weber T, Ammer M, Rammer M, Adji A, O'Rourke MF, Wassertheurer S, et al. Noninvasive determination of carotid-femoral pulse wave velocity depends critically on assessment of travel distance: a comparison with invasive measurement. J Hypertens. (2009) 27(8):1624–30. doi: 10.1097/HJH.0b013e32832cb04e
94. Najjar SS, Scuteri A, Shetty V, Wright JG, Muller DC, Fleg JL, et al. Pulse wave velocity is an independent predictor of the longitudinal increase in systolic blood pressure and of incident hypertension in the Baltimore longitudinal study of aging. J Am Coll Cardiol. (2008) 51(14):1377–83. doi: 10.1016/j.jacc.2007.10.065
95. Liu B, Gao L, Zheng B, Yang Y, Jia J, Sun P, et al. Comparison of carotid-femoral and brachial-ankle pulse wave velocity in association with carotid plaque in a Chinese community-based population. J Clin Hypertens (Greenwich). (2022) 24(12):1568–76. doi: 10.1111/jch.14602
96. Chuang SY, Chen CH, Cheng CM, Chou P. Combined use of brachial-ankle pulse wave velocity and ankle-brachial Index for fast assessment of arteriosclerosis and atherosclerosis in a community. Int J Cardiol. (2005) 98(1):99–105. doi: 10.1016/j.ijcard.2004.01.019
Keywords: arterial stiffness, pulsed wave velocity, heart failure, systematic review, meta-analysis
Citation: Esmaeili Z, Bahiraie P, Vaziri Z, Azarboo A, Behnoush AH, Khalaji A, Bazrgar A, Tayebi P and Ziaie N (2024) The association between pulse wave velocity and heart failure: a systematic review and meta-analysis. Front. Cardiovasc. Med. 11: 1435677. doi: 10.3389/fcvm.2024.1435677
Received: 20 May 2024; Accepted: 2 July 2024;
Published: 23 July 2024.
Edited by:
Filippo Valbusa, Sacro Cuore Don Calabria Hospital (IRCCS), ItalyReviewed by:
Audrey Adji, Victor Chang Cardiac Research Institute, AustraliaDario Leone, University of Turin, Italy
© 2024 Esmaeili, Bahiraie, Vaziri, Azarboo, Behnoush, Khalaji, Bazrgar, Tayebi and Ziaie. This is an open-access article distributed under the terms of the Creative Commons Attribution License (CC BY). The use, distribution or reproduction in other forums is permitted, provided the original author(s) and the copyright owner(s) are credited and that the original publication in this journal is cited, in accordance with accepted academic practice. No use, distribution or reproduction is permitted which does not comply with these terms.
*Correspondence: Naghmeh Ziaie, emlhaWV4bkB5YWhvby5jb20=
†These authors have contributed equally to this work and share first authorship
‡ORCID:
Zahra Esmaeili
orcid.org/0000-0002-7319-7233
Pegah Bahiraie
orcid.org/0009-0005-4766-698X
Zahra Vaziri
orcid.org/0000-0002-4043-7544
Alireza Azarboo
orcid.org/0000-0003-3399-2360
Amir Hossein Behnoush
orcid.org/0000-0002-9955-4227
Amirmohammad Khalaji
orcid.org/0000-0002-6138-1914
Aida Bazrgar
orcid.org/0000-0003-0922-832X
Pouya Tayebi
orcid.org/0000-0002-6923-0221
Naghmeh Ziaie
orcid.org/0000-0003-4933-8240