- 1Cardiac Surgery, Fujian Medical University, Fuzhou, Fujian, China
- 2Department of Cardiovascular Surgery, Union Hospital, Fujian Medical University, Fuzhou, Fujian, China
- 3Key Laboratory of Cardio-Thoracic Surgery, (Fujian Medical University), Fujian Province University, Fuzhou, Fujian, China
- 4Thoracic and Cardiovascular Surgery, Fuzhou Changle District People’s Hospital, Fuzhou, Fujian, China
Objective: This study strives to the variation and significance of microRNA-21 (miR-21) in children with congenital heart disease (CHD)-related pulmonary artery hypertension (PAH).
Methods: Children with CHD (n = 179) were selected as subjects, including 101 children without PAH and 78 children with PAH. All children underwent general data collection, laboratory examination, echocardiography and cardiac catheterization. After detection of serum miR-21 expression, the predictive value and the impacts of serum miR-21 for PAH and postoperative critical illness were analyzed.
Results: Serum creatine kinase isoenzyme (CK-MB), B-type natriuretic peptide (BNP) and miR-21 were elevated, but ejection fraction (EF) and cardiac index (CI) were decreased in the CHD-PAH group. Serum miR-21 assisted in predicting PAH in CHD children, with the area under curve (AUC) of 0.801 (95% CI of 0.735∼0.857), a cut-off value of 2.56, sensitivity of 73.08, and specificity of 72.28%. Serum miR-21 in children with CHD-PAH was correlated with clinicopathological indicators such as systolic pulmonary artery pressure, mean pulmonary arterial pressure, BNP and CI. Serum miR-21 helped predict the development of postoperative critical illness in children with CHD-PAH, with an AUC of 0.859 (95% CI: 0.762–0.927, cut-off value: 4.55, sensitivity: 69.57%, specificity: 92.73%). Increased serum miR-21 was an independent risk factor of postoperative critical illness in children with CHD-PAH.
Conclusion: Serum miR-21 was upregulated in children with CHD-PAH, which may serve as a predictive biomarker for the onset of PAH and postoperative critical illness in CHD children.
Introduction
Congenital heart disease (CHD) is a disease of abnormal fetal cardiac and cardiovascular development that affects approximately 1% of infants born each year (1). Patients with CHD are at a high risk of developing pulmonary artery hypertension (PAH) (2). Chronic right ventricular pressure overload makes children with PAH associated with CHD (CHD-PAH) prone to right ventricular hypertrophy, volume overload and failure, eventually evolving into right ventricular dysfunction (3). Noticeably, the development of PAH may substantially increase the mortality in patients with CHD (4). Unfortunately, children with CHD are more susceptible to developing PAH instead of adults with CHD (5).
Cardiac catheterization, a gold standard for definitive diagnosis of PAH so far, enables direct evaluation of pulmonary hemodynamics and implementation of vasoreactivity test (6). However, this approach is likely to put patients at risk of complications, such as arrhythmia, hypertensive crisis, pulmonary embolism and even death (7, 8). In contrast, echocardiography (echo) is a non-invasive method that can be extensively used in patients with CHD-PAH (3), but the accuracy of echo is affected by various factors, such as the experience of operators, equipment quality and patient compliance with examinations, to name a few. At present, there is a lack of specific, economic and non-invasive methods for CHD-PAH screening.
MicroRNAs (miRNAs), small non-coding RNA, have garnered significant research attention in recent years as they participate in the pathophysiological process of various cells through their regulation of gene expression (9). Dysregulation of miRNAs appears to implicate in various heart diseases including CHD (10–13). miR-21 is an encoding gene in the fragile site FRA17B within the human 17q23.2 chromosomal region, and it has been identified by previous studies to be associated with cardiac hypertrophy, heart failure, and myocardial infarction (14, 15). Reportedly, increasingly expressed miR-21 was found in myocardial fibroblasts and associated with vascular endothelial disorder and pulmonary vascular remodeling (16, 17). Accordingly, it is assumed that abnormal miR-21 expression may be related to the development of PAH and postoperative crisis in children with CHD-PAH. More importantly, a recent report disclosed that upregulation of miR-21 in the early stage of PAH results in right ventricular hypertrophy, whereas in the late stage of PAH, right ventricular dysfunction is caused by its downregulation, which triggers a biphasic regulation of cardiac remodeling and cardiomyocyte apoptosis (18). In a pressure overload-induced PAH model in sheep, miR-21 was identified as a critical factor for the occurrence of right ventricular hypertrophy and dysfunction (19). All this information indicates that miR-21 may show great importance in children with CHD-PAH, but the number of studies focusing on the change of miR-21 and its clinical significance in children with CHD-PAH is limited. This study is designed to discover the change of serum miR-21 and its significance in children with CHD-PAH, providing a novel insight for clinical diagnosis and treatment of CHD-PAH.
Materials and methods
Ethics statement
This study was reviewed and granted by the Ethics Committee of Union Hospital, Fujian Medical University and complied with the Declaration of Helsinki. Written informed consent was obtained from the guardian of each enrolled participant.
Participants
A total of 256 children with CHD admitted to the hospital from February 2020 to May 2023 were enrolled, and 179 children with CHD were selected as the study subjects according to the inclusion and exclusion criteria. Based on the occurrence of PAH, the children with CHD were stratified into CHD (without CHD-related PAH, n = 101) and CHD-PAH groups (with PAH associated with CHD, n = 78). General information, laboratory findings, echo results, and cardiac catheterization results were collected from all children with CHD. Another 65 healthy participants who had normal physical examination results were chosen as the control group.
Inclusion and exclusion criteria
Diagnostic criteria for CHD: examined by echo and confirmed by vascular CT or cardiovascular angiography.
Diagnostic criteria for CHD-related PAH: diagnosed by cardiac catheterization with mean pulmonary arterial pressure (mPAP) ≥ 20 mmHg by right heart catheterization when the child was resting (20).
Inclusion criteria: 1. age < 12 years old; 2. all children underwent cardiac catheterization before intervention; 3. treated with minimally invasive cardiac surgery for the first time; 4. complete clinical data.
Exclusion criteria: 1. combination of severe infection, tumor, severe cardiopulmonary insufficiency, diabetes mellitus, systemic hypertension, cardiomyopathy, hepatic insufficiency or renal failure and bleeding tendency; 2. Presence of hematological system diseases, connective tissue diseases, and other respiratory system diseases.
Case information collection
The medical records of children with CHD were consulted to collect age, sex, heart rate (HR), arterial systolic arterial pressure (SBP), arterial diastolic arterial pressure (DBP), ejection fraction (EF), cardiac index (CI), left ventricular end-diastolic diameter (LVEDd), systolic pulmonary artery pressure (sPAP), diastolic pulmonary artery pressure (dPAP), mPAP, serum creatine kinase isoenzyme (CK-MB), B-type natriuretic peptide (BNP), pulmonary vascular resistance (PVR), and other information.
RNA isolation and reverse transcription polymerase chain reaction (Rt-PCR)
All children with CHD underwent minimally invasive cardiac surgery and were subjected to elbow venous blood sampling (5 ml) using a non-anticoagulant vacuum tube. After centrifugation for 15 min (radius 8 cm, 4,000 r/min), the serum was preserved in a refrigerator at −80°C for testing. An miRNA extraction kit (Beijing Tiangen Biotechnology Co., Ltd., Beijing, China) was used to extract miRNAs which were reverse transcribed to cDNA with a reverse transcription kit (TaKaRa Bio, Tokyo, Japan). RT-PCR method and MX3000PPCR instrument from USA Agilent were adopted to amplify miR-21 target segments. With U6 snRNA as the housekeeping gene, the RT-PCR experiment was performed with a reaction system of 20 μl under the conditions of pre-denaturation at 95°C for 10 min, 95°C for 30s and 60°C for 30 s, for a total of 40 cycles. The ratio of the target gene level to the U6 level was calculated and the relative level of miR-21 was expressed by 2−ΔΔCt. See Table 1 for specific primer sequences.
Postoperative critical illness score
Pediatric critical illness score (PCIS) was adopted to evaluate 10 items: HR, blood pressure, serum creatinine, urobilin, partial arterial oxygen pressure, serum potassium level, serum sodium level, hemoglobin level, respiratory system status and gastrointestinal system status (21). The maximum abnormal value of each index was recorded within 24 h after the children were admitted to the monitoring room, and the corresponding scores were summed up to get the total score out of 100 points. The lower the score, the more critical the condition. A PCIS score of > 80 points represents a non-critical case, a PCIS score of 71–80 points indicates a critical case, and a PCIS score of ≤ 70 points represents a very critical case.
Statistic analysis
Data were statistically analyzed and graphed using SPSS 21.0 statistical software (SPSS, Inc, Chicago, IL, USA) and GraphPad Prism 6.0 software (GraphPad Software Inc., San Diego, CA, USA). The Kolmogorov-Smirnov test was used to test for normal distribution, and measurements conformed to normal distribution were expressed as mean ± standard deviation. Comparisons between two groups were performed using the independent sample t-test, whereas multiple groups were analyzed by one-way analysis of variance (ANOVA), and post-hoc tests were performed using Tukey's multiple comparisons test. Non-normally distributed measurements were expressed as quartiles, i.e., median (minimum, maximum), and comparisons between groups were made using the Mann-Whitney U-test; count data were expressed as case numbers and percentages, and comparisons between groups were made using the chi-square test. A multi-factor logistic regression model was established to analyze the impacts of changes in serum miR-21 level on the occurrence of PAH and postoperative critical illness in children with CHD, and the Enter method was selected to screen the independent variables. ROC curve was used to analyze the predictive value of serum miR-21 level on the occurrence of PAH and postoperative critical illness in children with CHD, and the cut-off value and the area under the ROC curve (AUC) of serum miR-21 were obtained. P was a two-sided test, and the difference was considered statistically significant at P < 0.05.
Results
Comparison of baseline data between the three groups
This study selected 179 children with CHD admitted to the hospital from February 2020 to May 2023 as the study subjects and stratified them into CHD (without CHD-related PAH, n = 101) and CHD-PAH groups (with CHD-related PAH, n = 78). Another 65 healthy subjects were included as the control group. No significant difference was found in sex, age, body mass index (BMI), HR, SBP, DPB, LVEDd and other indexes among the three groups (all P > 0.05, Table 2), and the CHD group had no significant difference in disease type and mPAP compared with the CHD-PAH group (all P > 0.05, Table 2). However, the EF, BNP and CK-MB levels were substantially different among the three groups (all P < 0.05, Table 2), and the children in the CHD-PAH group had higher BNP and CK-MB levels and lower EF. Moreover, sPAP, mPAP, dPAP, and PVR were higher in the CHD-PAH group than those in the CHD group, but the CI in the CHD-PAH group was decreased compared with the CHD group (all P < 0.05, Table 2).
Children with CHD-PAH had higher serum miR-21 levels, which assisted in predicting the occurrence of PAH in children with CHD
Based on the comparisons of serum miR-21 levels among control, CHD, and CHD-PAH groups, the relative expression of serum miR-21 of the control group was (1.08 ± 0.44), and that in the children in the CHD group and CHD-PAH group was (2.10 ± 1.05) and (3.64 ± 1.46) respectively; the serum miR-21 levels of the children in the CHD group were significantly higher than those of the control group, and those of the CHD-PAH group were higher than those of the CHD group (P < 0.001, Figure 1A). The predictive value of serum miR-21 level for the occurrence of PAH in children with CHD was further evaluated. According to the plotted ROC curve, the serum miR-21 was shown to assist in predicting the occurrence of PAH in children with CHD with an AUC of 0.801, a 95% CI of 0.735∼0.857, a cut-off value of 2.56, a sensitivity of 73.08%, and a specificity of 72.28% (Figure 1B).
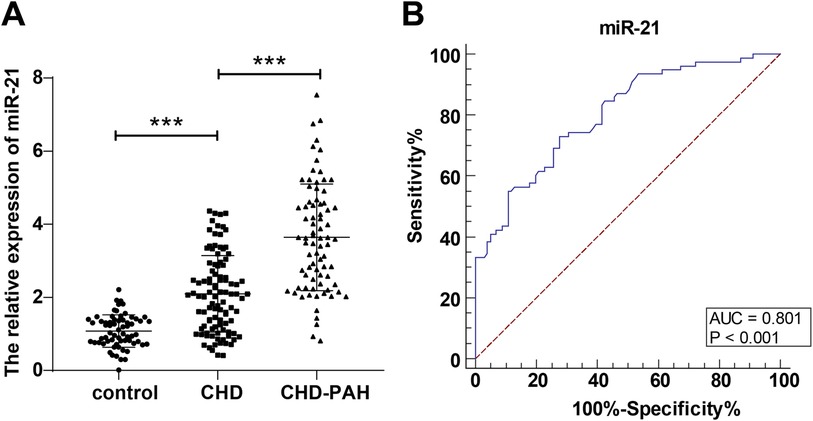
Figure 1. Change in serum miR-21 level in the three groups and predictive value of serum miR-21 for the occurrence of PAH in children with CHD. (A): comparison of relative expression of serum miR-21 in the three groups; (B): predictive value of miR-21 for the occurrence of PAH in children with CHD based on plotted ROC curve. Serum miR-21 level was detected through RT-qPCR and expressed as average ± standard deviation. One-way analysis of variance was used for comparisons among multiple groups and Tukey's multiple comparisons test for post-hoc test. ***P < 0.001. CHD, congenital heart disease; PAH, pulmonary artery hypertension.
Relationship between serum miR-21 level in children with CHD-PAH and their clinical pathological indexes
Children with CHD-PAH were categorized into miR-21 high group and miR-21 low group according to the ROC cut-off value of miR-21, and the relationship between serum miR-21 levels in children with CHD-PAH and their clinical pathological indexes were analyzed. According to the results, there was no significant difference in the serum miR-21 levels in children with CHD-PAH who had different ages, sex, BMI, disease type, EF, HR, SBP, DBP, LVEDd, dPAP, mRAP, PVR, and CK-MB (all P > 0.05), but the sPAP, mPAP, and BNP levels of the children in the miR-21 high group were significantly higher than those of the children in the miR-21 low group, and the CI in the miR-21 high group was lower than those in the miR-21 low group (all P < 0.05, Table 3).
Increased serum miR-21 level was an independent risk factor for PAH in children with CHD
For accurate evaluation of the impacts of serum miR-21 level on the occurrence and development of PAH, EF, CI, BNP CK-MB, and serum miR-21 level were included in multi-factor logistics regression analysis. Accordingly, increased levels of BNP and miR-21 were considered independent risk factors for the occurrence of PAH in children with CHD (Table 4).
Serum miR-21 level in children with CHD-PAH assisted in predicting postoperative critical illness
The children were further divided into the non-critical group (>80 points), critical and very critical group (≤80 points) and the ROC curve was plotted to analyze the predictive value of serum miR-21 level for postoperative critical illness in children with CHD-PAH. As shown in Figure 2, the ACU of serum miR-21 assisting in predicting postoperative critical illness in children with CHD-PAH was 0.859, the 95% CI was 0.762∼0.927, the cutoff value was 4.55, the sensitivity was 69.57% and the specificity was 92.73%.
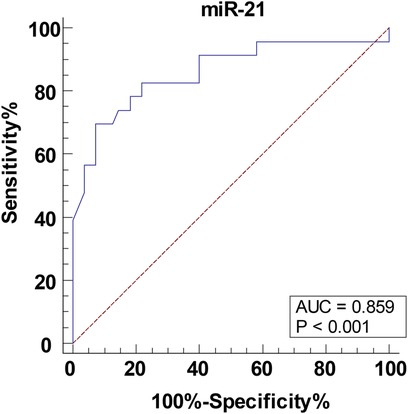
Figure 2. The predictive value of serum miR-21 level for postoperative critical illness in children with CHD-PAH. The predictive value of serum miR-21 for the occurrence of postoperative critical illness in children with CHD-PAH analyzed through the ROC curve. CHD, congenital heart disease; PAH, pulmonary artery hypertension.
Increased serum miR-21 level was an independent risk factor for postoperative critical illness in children with CHD-PAH
Sex, age, BMI, HR, SBP, DBP, sPAP, mPAP, dPAP, EF, CI, PVR, BNP, CK-MB, and serum miR-21 level were included in single-factor logistics regression analysis, and the age, BMI, sPAP, mPAP, EF, CI, PVR, BNP, CK-MB and miR-21 level of children with CHD-PAH were considered as independent risk factors for postoperative critical illness. Then, indexes with P < 0.05 in the single-factor logistics regression analysis were used as independent variables for multi-factor logistics regression analysis, which demonstrated that higher serum miR-21 level was an independent risk factor for postoperative critical illness in children with CHD-PAH (Table 5).
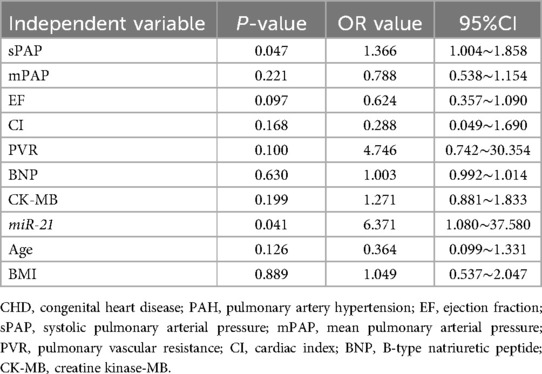
Table 5. Multi-factor logistics regression analysis for the occurrence of postoperative critical illness in children with CHD-PAH.
Discussion
CHD-PAH occurs predominantly in patients with uncorrected CHD (22). Our study found that children with CHD-PAH had elevated sPAP, mPAP, dPAP and PVR, which are common indexes used for the diagnosis of CHD (23), indicating that the occurrence of PAH may deteriorate the condition of children with CHD. This study mainly explored the variation of serum miR-21 levels and its significance in children with CHD-PAH, and demonstrated an increase in serum miR-21 levels in children with CHD-PAH. The findings of this study can assist in predicting the occurrence of PAH in children with CHD and the severity of conditions post-operation.
miR-21 has been identified as an upregulated transpulmonary exosomal miRNA in patients with CHD-PAH (17). In patients with hypertensive heart disease, miR-21 was also detected to be upregulated, and suppressing miR-21 attenuated hypertrophic stimulation-triggered cardiac remodeling in angiotensin II-treated neonatal rats (24). Our study first observed miR-21 upregulation in children with CHD-PAH compared to children with CHD. miR-21 was also identified to assist in the occurrence of PAH in children with CHD, with a sensitivity of 73.8% and a specificity of 72.28%. PAH is a fatal disease with multiple pathophysiological characteristics such as pulmonary vasoconstriction, inflammation, right ventricular hypertrophy and right ventricular systolic pressure (11). In miR-21 knockout mice, Nano-li-stimulated pulmonary inflammation, damage, and fibrosis appeared to be mitigated (25). This study also found higher sPAP, mPAP and BNP, as well as lower CI in patients with higher miR-21 expression. The results of the multi-factor logistics regression analysis is a further reminder of how important miR-21 is for the prediction of the occurrence of PAH. Through the analysis, miR-21 was identified as an independent risk factor for the occurrence of PAH in children with CHD, along with BNP, which is a biomarker secreted by cardiomyocytes in response to an increase in ventricular wall stress (26). In heritable PAH and idiopathic PAH, the critical breakthrough over the past decades is that bone morphogenetic protein (BMP) signaling reduction is caused by pathogenic mutations of BMP receptor 2 (BMPR2), and triggers proliferation and anti-apoptosis of pulmonary artery smooth muscle cells; however, CHD-PAH showed the lowest level of BMPR2 mutation but commonly suppressed BMP cascade in lungs (27). BMP-7 overexpression in diabetic mice significantly reduced miR-21 and elevated Smad7, restrained Smad3 activation, and mitigated epithelial-mesenchymal transition and extracellular matrix deposition (28). BMP-4 was reported to activate miR-21, which inhibited its downstream target Dedicator of cytokines protein family to result in pulmonary vascular smooth muscle cell contractility (29, 30). The above studies imply the implication of miR-21 in vascular contractility and fibrosis, but also hint at gene regulation functions of miR-21. miR-21 may be activated by BMP and then regulate its downstream targets to affect PAH; however, due to time and budget constraints, the specific molecular mechanisms of miR-21 in CHD-PAH remain to be investigated in the future.
In case of CHD-PAH, medication can stabilize the condition but fail to cure the PAH, and correction of the anatomical deformity is recommended as soon as possible; however, children still face the risks of severe hypoxemia, reactive PAH, etc. in the early postoperative period (31). Postoperative PCIS is a tool frequently used for clinical evaluation of postoperative crisis in children (32), by which the condition can be evaluated through the frequency of score increase and interventions can be deployed dynamically. However, the score system is an early estimation formula under no-condition state. Therefore, there is a growing realization that non-invasive and effective biomarkers must be determined for clinical practice, and it is imperative for early individual evaluation of postoperative crisis in children with PAH. This study demonstrated that serum miR-21 level can assist in predicting postoperative critical illness in children with CHD-PAH. Further multi-factor logistics regression analysis determined upregulated serum miR-21 expression as independent risk factor of the occurrence of postoperative critical illness in children with CHD-PAH.
This study is a retrospective single-center analysis with a small sample size. The results obtained should be verified with a larger sample size in the future. Moreover, miR-21 serves effectively as a biomarker for diagnosing pulmonary hypertension (PH), enabling the differentiation between World Health Organization group 1 PH, group 2 PH, and group 3 PH (33). The relationship between miR-21 and CHD-PAH has been extensively investigated in various studies (17, 34). Previous research in animal models has revealed a biphasic regulation on cardiac remodeling and cardiomyocyte apoptosis under pulmonary hypertension triggered by the up-regulation of miR-21 at the early phase (right ventricular hypertrophy) and its down-regulation at the late phase (right ventricular dysfunction) (18). Notably, in a sheep model of pressure overload-induced pulmonary hypertension, miR-21 was identified as a crucial contributor to right ventricular hypertrophy and dysfunction (19). A prior study has demonstrated that miR-21 influences pulmonary hypertension via the TGF-β1/Smad2 pathway (35). Additionally, miR-21 has been implicated in the occurrence and development of hypoxic pulmonary hypertension by modulating DDAH1 expression (36). Despite these findings, the specific biological mechanism by which miR-21 affects CHD-PAH is still of high research value. Additionally, the specific pathogenesis of miR-21 affecting CHD-PAH in the current study remains incompletely understood. Therefore, additional experiments are warranted to elucidate the potential pathogenesis in subsequent investigations. Our team will further explore the molecular mechanisms of miR-21 upregulation in CHD-PAH by developing a multi-center prospective study and enlarging the sizes of samples and matched controls to increase the sensitivity of tests and the credibility of results.
Data availability statement
The original contributions presented in the study are included in the article/Supplementary Material, further inquiries can be directed to the corresponding author.
Ethics statement
The studies involving humans were approved by Union Hospital, Fujian Medical University. The studies were conducted in accordance with the local legislation and institutional requirements. Written informed consent for participation in this study was provided by the participants’ legal guardians/next of kin. Written informed consent was obtained from the minor(s)' legal guardian/next of kin for the publication of any potentially identifiable images or data included in this article.
Author contributions
YS: Conceptualization, Methodology, Data curation, Investigation, Validation, Writing – original draft. DL: Conceptualization, Methodology, Funding acquisition, Project administration, Supervision, Writing – review & editing. WS: Investigation, Resources, Software, Visualization, Writing – review & editing. LC: Formal Analysis, Validation, Writing – review & editing.
Funding
The author(s) declare financial support was received for the research, authorship, and/or publication of this article. This work was supported by the Fujian Provincial Natural Science Foundation (No. 2022J01749).
Conflict of interest
The authors declare that the research was conducted in the absence of any commercial or financial relationships that could be construed as a potential conflict of interest.
Publisher's note
All claims expressed in this article are solely those of the authors and do not necessarily represent those of their affiliated organizations, or those of the publisher, the editors and the reviewers. Any product that may be evaluated in this article, or claim that may be made by its manufacturer, is not guaranteed or endorsed by the publisher.
References
1. Williams K, Carson J, Lo C. Genetics of congenital heart disease. Biomolecules. (2019) 9(12):879. doi: 10.3390/biom9120879
2. Mares A, Mukherjee D, Lange RA, Nickel NP. Targeted therapies in patients with pulmonary arterial hypertension due to congenital heart disease. Curr Vasc Pharmacol. (2022) 20(4):341–60. doi: 10.2174/1570161120666220811150853
3. Jone PN, Ivy DD, Hauck A, Karamlou T, Truong U, Coleman RD, et al. Pulmonary hypertension in congenital heart disease: a scientific statement from the American Heart Association. Circ Heart Fail. (2023) 16(7):e00080. doi: 10.1161/HHF.0000000000000080
4. Papamichalis M, Xanthopoulos A, Papamichalis P, Skoularigis J, Triposkiadis F. Adult congenital heart disease with pulmonary arterial hypertension: mechanisms and management. Heart Fail Rev. (2020) 25(5):773–94. doi: 10.1007/s10741-019-09847-5
5. Rosenzweig EB, Krishnan U. Congenital heart disease-associated pulmonary hypertension. Clin Chest Med. (2021) 42(1):9–18. doi: 10.1016/j.ccm.2020.11.005
6. Rosenzweig EB, Abman SH, Adatia I, Beghetti M, Bonnet D, Haworth S, et al. Paediatric pulmonary arterial hypertension: updates on definition, classification, diagnostics and management. Eur Respir J. (2019) 53(1):1801916. doi: 10.1183/13993003.01916-2018
7. Hasnie AA, Parcha V, Hawi R, Trump M, Shetty NS, Ahmed MI, et al. Complications associated with transesophageal echocardiography in transcatheter structural cardiac interventions. J Am Soc Echocardiogr. (2023) 36(4):381–90. doi: 10.1016/j.echo.2022.12.023
8. Chen Y, Shlofmitz E, Khalid N, Bernardo NL, Ben-Dor I, Weintraub WS, et al. Right heart catheterization-related complications: a review of the literature and best practices. Cardiol Rev. (2020) 28(1):36–41. doi: 10.1097/CRD.0000000000000270
9. Pang XF, Lin X, Du JJ, Zeng DY. Downregulation of microRNA-592 protects mice from hypoplastic heart and congenital heart disease by inhibition of the notch signaling pathway through upregulating KCTD10. J Cell Physiol. (2019) 234(5):6033–41. doi: 10.1002/jcp.27190
10. Zhao X, Wang Y, Sun X. The functions of microRNA-208 in the heart. Diabetes Res Clin Pract. (2020) 160:108004. doi: 10.1016/j.diabres.2020.108004
11. Zhang YZ, Li G, Yan DL, Liu YL. MicroRNA-98 can serve as a diagnostic marker for congenital heart disease-associated pulmonary artery hypertension. Chin Med J (Engl. (2020) 133(24):3007–9. doi: 10.1097/CM9.0000000000001193
12. Song R, Dasgupta C, Mulder C, Zhang L. MicroRNA-210 controls mitochondrial metabolism and protects heart function in myocardial infarction. Circulation. (2022) 145(15):1140–53. doi: 10.1161/CIRCULATIONAHA.121.056929
13. Liu W, Cheng L, Chen K, Wu J, Peng R, Tang YL, et al. Identification of novel single-nucleotide variants with potential of mediating malfunction of MicroRNA in congenital heart disease. Front Cardiovasc Med. (2021) 8:739598. doi: 10.3389/fcvm.2021.739598
14. Xu L, Tian L, Yan Z, Wang J, Xue T, Sun Q. Diagnostic and prognostic value of miR-486-5p, miR-451a, miR-21-5p and monocyte to high-density lipoprotein cholesterol ratio in patients with acute myocardial infarction. Heart Vessels. (2023) 38(3):318–31. doi: 10.1007/s00380-022-02172-2
15. Angelopoulos A, Oikonomou E, Antonopoulos A, Theofilis P, Zisimos K, Katsarou O, et al. Expression of circulating miR-21 and -29 and their association with myocardial fibrosis in hypertrophic cardiomyopathy. Curr Med Chem. (2024) 31(25):3987–96. doi: 10.2174/0109298673286017240103073130
16. Jayawardena E, Medzikovic L, Ruffenach G, Eghbali M. Role of miRNA-1 and miRNA-21 in acute myocardial ischemia-reperfusion injury and their potential as therapeutic strategy. Int J Mol Sci. (2022) 23(3):1512. doi: 10.3390/ijms23031512
17. Chang WT, Lee WC, Lin YW, Shih JY, Hong CS, Chen ZC, et al. Transpulmonary expression of exosomal microRNAs in idiopathic and congenital heart disease-related pulmonary arterial hypertension. J Am Heart Assoc. (2023) 12(23):e031435. doi: 10.1161/JAHA.123.031435
18. Chang WT, Wu CC, Lin YW, Shih JY, Chen ZC, Wu SN, et al. Dynamic changes in miR-21 regulate right ventricular dysfunction in congenital heart disease-related pulmonary arterial hypertension. Cells. (2022) 11(3):564. doi: 10.3390/cells11030564
19. Chang WT, Fisch S, Dangwal S, Mohebali J, Fiedler AG, Chen M, et al. MicroRNA-21 regulates right ventricular remodeling secondary to pulmonary arterial pressure overload. J Mol Cell Cardiol. (2021) 154:106–14. doi: 10.1016/j.yjmcc.2021.01.003
20. Habedank D, Ittermann T, Kaczmarek S, Stubbe B, Heine A, Obst A, et al. Cardiopulmonary exercise testing and the 2022 definition of pulmonary hypertension. Pulm Circ. (2024) 14(2):e12398. doi: 10.1002/pul2.12398
21. Pan T, Guo X, Yang D, Ding J, Chen C. Expression and significance of procalcitonin, leukotriene B4, serum amyloid A, and C-reactive protein in children with different types of pneumonia: an observational study. Medicine (Baltimore). (2024) 103(19):e37817. doi: 10.1097/MD.0000000000037817
22. Li X, Li T. Inadequate dosage may lead to the recurrence of postoperative pulmonary hypertension in patients with congenital heart disease. Front Pharmacol. (2021) 12:660405. doi: 10.3389/fphar.2021.660405
23. He YY, Yan Y, Chen JW, Liu S, Hua L, Jiang X, et al. Plasma metabolomics in the perioperative period of defect repair in patients with pulmonary arterial hypertension associated with congenital heart disease. Acta Pharmacol Sin. (2022) 43(7):1710–20. doi: 10.1038/s41401-021-00804-3
24. Watanabe K, Narumi T, Watanabe T, Otaki Y, Takahashi T, Aono T, et al. The association between microRNA-21 and hypertension-induced cardiac remodeling. PLoS One. (2020) 15(2):e0226053. doi: 10.1371/journal.pone.0226053
25. Mo Y, Zhang Y, Wan R, Jiang M, Xu Y, Zhang Q. miR-21 mediates nickel nanoparticle-induced pulmonary injury and fibrosis. Nanotoxicology. (2020) 14(9):1175–97. doi: 10.1080/17435390.2020.1808727
26. Said F, Haarman MG, Roofthooft MTR, Hillege HL, Ploegstra MJ, Berger RMF. Serial measurements of N-terminal pro-B-type natriuretic peptide serum level for monitoring pulmonary arterial hypertension in children. J Pediatr. (2020) 220:139–45. doi: 10.1016/j.jpeds.2020.01.001
27. Meng L, Teng X, Liu Y, Yang C, Wang S, Yuan W, et al. Vital roles of gremlin-1 in pulmonary arterial hypertension induced by systemic-to-pulmonary shunts. J Am Heart Assoc. (2020) 9(15):e016586. doi: 10.1161/JAHA.120.016586
28. Liu L, Wang Y, Yan R, Liang L, Zhou X, Liu H, et al. BMP-7 inhibits renal fibrosis in diabetic nephropathy via miR-21 downregulation. Life Sci. (2019) 238:116957. doi: 10.1016/j.lfs.2019.116957
29. Kang H, Davis-Dusenbery BN, Nguyen PH, Lal A, Lieberman J, Van Aelst L, et al. Bone morphogenetic protein 4 promotes vascular smooth muscle contractility by activating microRNA-21 (miR-21), which down-regulates expression of family of dedicator of cytokinesis (DOCK) proteins. J Biol Chem. (2012) 287(6):3976–86. doi: 10.1074/jbc.M111.303156
30. Jiang C, Guo Y, Yu H, Lu S, Meng L. Pleiotropic microRNA-21 in pulmonary remodeling: novel insights for molecular mechanism and present advancements. Allergy Asthma Clin Immunol. (2019) 15:33. doi: 10.1186/s13223-019-0345-2
31. Xi SB, Wang SS, Qian MY, Xie YM, Li JJ, Zhang ZW. Predictors of operability in children with severe pulmonary hypertension associated with congenital heart disease. Chin Med J (Engl). (2019) 132(7):811–8. doi: 10.1097/CM9.0000000000000145
32. Shi Q, Zhao Z, Lin J, Zhang Y, Dai J. A prediction model for the efficacy of continuous positive airway pressure on bronchiolitis. Front Pediatr. (2022) 10:1033992. doi: 10.3389/fped.2022.1033992
33. Dimitry MO, Soliman YMA, ElKorashy RI, Raslan HM, Kamel SA, Hassan EM, et al. Role of micro-RNAs 21, 124 and other novel biomarkers in distinguishing between group 1 WHO pulmonary hypertension and group 2, 3 WHO pulmonary hypertension. Egypt Heart J. (2023) 75(1):76. doi: 10.1186/s43044-023-00395-w
34. Duzgun Z, Kayikcioglu LM, Aktan C, Bara B, Eroglu FZ, Yagmur B, et al. Decreased circulating microRNA-21 and microRNA-143 are associated to pulmonary hypertension. Turk J Med Sci. (2023) 53(1):130–41. doi: 10.55730/1300-0144.5566
35. Si J, Yang B, Yu J, Li Y, Gao P. Effect of MiR-21 on pulmonary arterial hypertension via the TGF-beta1/Smad2 signal pathway. Minerva Med. (2020) 111(2):181–3. doi: 10.23736/S0026-4806.19.06014-2
Keywords: congenital heart disease, pulmonary artery hypertension, miR-21, pediatric critical illness score, clinical significance
Citation: Shen Y, Liao D, Shangguan W and Chen L (2024) Variation and significance of serum microRNA-21 level in pediatric pulmonary artery hypertension associated with congenital heart disease. Front. Cardiovasc. Med. 11:1424679. doi: 10.3389/fcvm.2024.1424679
Received: 28 April 2024; Accepted: 16 August 2024;
Published: 5 September 2024.
Edited by:
Xupei Huang, Florida Atlantic University, United StatesReviewed by:
Ricardo Adrian Nugraha, Airlangga University, IndonesiaYingcai Wang, Florida Atlantic University, United States
Copyright: © 2024 Shen, Liao, Shangguan and Chen. This is an open-access article distributed under the terms of the Creative Commons Attribution License (CC BY). The use, distribution or reproduction in other forums is permitted, provided the original author(s) and the copyright owner(s) are credited and that the original publication in this journal is cited, in accordance with accepted academic practice. No use, distribution or reproduction is permitted which does not comply with these terms.
*Correspondence: Dongshan Liao, bGlhb2RzaGFuMjlmekAxNjMuY29t