- 1Department of Cardiology, The Second Hospital of Hebei Medical University, Shijiazhuang, Hebei, China
- 2Department of Cardiology, Affiliated Hospital of Hebei University, Baoding, Hebei, China
Background: Observational studies have found that vascular endothelial growth factor (VEGF) levels are associated with the risk of cardiovascular disease. However, it remains unclear whether VEGF levels have a causal effect on the risk of atrial fibrillation.
Methods: A two-sample Mendelian randomization (MR) study was conducted to explore the causal relationship between VEGF levels and the risk of atrial fibrillation. Genetic variants associated with VEGF [VEGF-A, VEGF-C, VEGF-D, VEGF receptor−2 (VEGFR-2), VEGFR-3] and atrial fibrillation (atrial fibrillation, atrial fibrillation and flutter) were used as instrumental variables. Data on genetic variants were obtained from published genome-wide association studies (GWAS) or the IEU Open GWAS project. Inverse-variance weighted (IVW) analysis was used as the primary basis for the results, and sensitivity analyses were used to reduce bias. Causal relationships were expressed as odds ratio (OR) with 95% confidence interval (CI), and a P-value of <0.1 corrected for False Discovery Rate (FDR) (PFDR < 0.1) was considered to have a significant causal relationship.
Results: Genetically predicted high levels of VEGF-A [OR = 1.025 (95%CI: 1.004–1.047), PFDR = 0.060] and VEGF-D [OR = 1.080 (95%CI: 1.039–1.123), PFDR = 0.001]] were associated with an increased risk of atrial fibrillation, while no causal relationship was observed between VEGF-C (PFDR = 0.419), VEGFR-2 (PFDR = 0.784), and VEGFR-3 (PFDR = 0.899) and atrial fibrillation risk. Moreover, only genetically predicted high levels of VEGF-D [OR = 1.071 (95%CI: 1.014–1.132), PFDR = 0.087] increased the risk of atrial fibrillation and flutter. Sensitivity analysis demonstrated that the relationship between VEGF-D levels and the risk of atrial fibrillation was robust.
Conclusion: This study supports a causal association between high VEGF-D levels and increased risk of atrial fibrillation.
Introduction
Atrial fibrillation is the most common arrhythmia and is associated with an increased risk of death, stroke, and peripheral embolism (1). Atrial fibrillation causes a higher disease burden and the prevalence and incidence of atrial fibrillation increases with age (2, 3). Common risk factors for atrial fibrillation are age, female, smoking, alcohol consumption, body mass index, hypertension, atrial fibrosis, left ventricular hypertrophy, heart failure, and myocardial infarction (1, 4). Endothelial dysfunction, inflammation, and oxidative stress play an important role in the pathophysiological process of atrial fibrillation (5–7).
Vascular endothelial growth factor (VEGF) is a class of neurotrophic and angiogenic factors secreted by endothelial cells (8). A cohort study showed that the risk of atrial fibrillation increased with elevated VEGF-D levels (9). Another cohort study found a U-shaped association between VEGF and the risk of cardiovascular events (10). In addition, several studies have shown higher VEGF levels in patients with atrial fibrillation compared to control populations without atrial fibrillation (5, 11). The potential association of VEGF with atrial fibrillation may be related to the role of VEGF as an inflammatory and pro-fibrotic mediator and its involvement in myocardial remodeling (12, 13). However, these observational studies of the association between VEGF and atrial fibrillation cannot infer causality due to confounding factors. Mendelian randomization (MR) uses genetic variation related to exposure to assess causal effects on outcomes (14, 15). Compared to traditional observational studies, MR is less susceptible to measurement error, confounders, and reverse causation, and reflects the long-term effects of exposure on outcomes. Recent MR studies have found a positive association between VEGF levels and the risk of venous thromboembolism (16) and cardiovascular death (17), but no association between VEGF levels and ischemic heart disease (18). However, the causal association between VEGF levels and the risk of atrial fibrillation remains unclear. Thus, the purpose of this study was to assess whether VEGF levels have a causal effect on the risk of atrial fibrillation through a two-sample MR study.
Methods
Study design
The causal association between VEGF levels and atrial fibrillation was examined through a two-sample MR analysis. The study methods were compliant with the STROBE-MR checklist (19). The genetic variants [single nucleotide polymorphisms (SNPs)] significantly associated with VEGF and atrial fibrillation were selected as instrumental variables, respectively. SNPs used for MR analysis should fulfill three basic assumptions: (1) SNPs are strongly associated with exposure; (2) SNPs are not associated with confounders; and (3) SNPs affect the outcomes only through exposure (Figure 1). This study was a secondary analysis of publicly available data. Ethical approval and informed consent were obtained for each of the original studies.
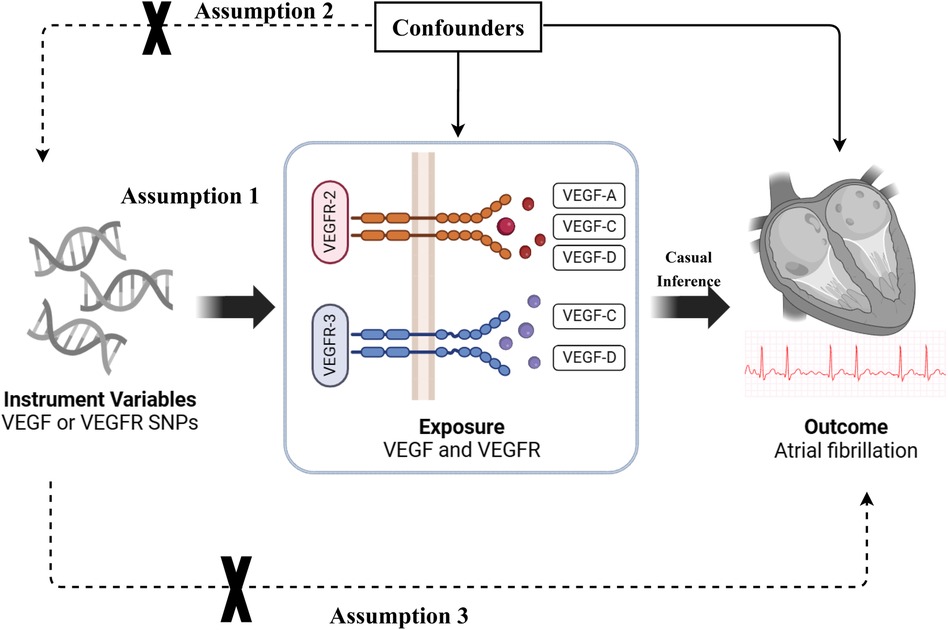
Figure 1. The basic assumptions of Mendelian randomization (MR) study. VEGF, vascular endothelial growth factor; VEGFR, vascular endothelial growth factor receptor; SNP, single nucleotide polymorphism.
Selection of instrumental variables
SNPs related to exposure and outcome in this study were obtained from published genome-wide association studies (GWAS) or the IEU Open GWAS project. The IEU Open GWAS project (https://gwas.mrcieu.ac.uk/) is a database of genetic associations from GWAS summary datasets for query or download (20). Table 1 shows the sources of SNPs related to exposure and outcome.
VEGF was the exposure variable in this study, including VEGF-A, VEGF-C, VEGF-D, VEGF receptor-2 (VEGFR-2), VEGFR-3, and VEGF (uncategorized). SNPs associated with VEGF-A, VEGF-C, VEGFR-2, and VEGFR-3 were obtained from the same GWAS that examined levels of VEGF-A, VEGF-C, VEGFR-2, and VEGFR-3 in 3,301 individuals of European descent in the INTERVAL study (21). The mean age (SD) was 43.6 (14.3) years for INTERVAL subcohort1 and 44.1 (14.2) years for INTERVAL subcohort2. There were 51.6% male in INTERVAL subcohort1 and 49.5% male in INTERVAL subcohort2. According to the GWAS (21), the procedure for testing plasma VEGF-A, VEGF-C, VEGFR-2, and VEGFR-3 is as follows: Blood samples were collected in 6-ml EDTA tubes using standard venepuncture protocols. The tubes were inverted three times and transferred to UK Biocentre (Stockport, UK) at ambient temperature for processing. Plasma was extracted by centrifugation into two 0.8 ml aliquots, which were then stored at −80°C prior to use. The relative concentrations of 3,622 plasma proteins or protein complexes were determined using a multiplexed, aptamer-based approach (SOMAscan assay) utilizing 4,034 modified aptamers.
SNPs related to VEGF-D and VEGF (uncategorized) were derived from a GWAS that examined VEGF-D and VEGF (uncategorized) levels in 21,758 individuals of European descent (22). Information about the population and the VEGF-D and VEGF (uncategorized) measurement process were not presented in the original GWAS.
Atrial fibrillation was the outcome variable in this study, including atrial fibrillation and “atrial fibrillation and flutter”. SNPs associated with atrial fibrillation were obtained from a GWAS on atrial fibrillation that analyzed 65,446 cases and 588,190 referents of 91.4% European ancestry from the Atrial Fibrillation Genetics (AFGen) consortium, the Broad AF Study (Broad AF), and the UK Biobank (UKBB) and the Biobank Japan (BBJ) (23). SNPs related to atrial fibrillation and flutter were derived from the IEU Open GWAS project that analyzed 22,068 cases and 196,724 controls of European ancestry from the FinnGen consortium (https://r7.finngen.fi/). The International Classification of Diseases, 10th Revision (ICD-10) code (I18) was used to identify atrial fibrillation and flutter for this trait, and patients with cardiovascular diseases were was excluded from the control group.
SNPs used for MR analysis are subject to rigorous screening. SNPs associated with exposure were first screened according to the following criteria: (1) SNPs were strongly related to VEGF at the genome-wide significance level threshold (P < 5 × 10−6); and (2) SNPs with linkage disequilibrium (LD) (r2 < 0.01 and clumping distance = 10,00 kb) or SNPs being palindromic with intermediate allele frequencies were excluded. After the screening for exposure-related SNPs was completed, the exposure-related SNPs and the outcome-related SNPs were harmonized so that both had the same allele.
Statistical analysis
The causal relationship between VEGF levels and the risk of atrial fibrillation was analyzed by a variety of MR methods, including inverse-variance weighted (IVW), weighted-median, MR-Egger, weighted mode, and MR-PRESSO. In this study, IVW analysis (fixed effect) was used as the primary basis for the results, supplemented by other MR analysis methods. IVW can obtain unconfounded estimates of genetically predicted exposure on outcome based on the Wald ratio method, which is the primary analysis for generating estimates of causal effects in MR analyses (24). Weighted-median provides a robust and consistent estimate of the effect even if nearly 50% of genetic variants were invalid instruments (25). MR-Egger provides valid tests and consistent estimates of causal effects even when all instrumental variables are invalid, but MR-Egger may exhibit low precision and be affected by abnormal genetic variation (26). Weighted mode requires that the most common causal effect estimate is a consistent estimate of the true causal effect even if the majority of instruments are invalid (27). MR-PRESSO detects and attempts to reduce horizontal pleiotropy by removing significant outliers, but the MR-PRESSO outlier test requires at least 50% of the genetic variants to be valid instruments and relies on Instrument Strength Independent of Direct Effect (InSIDE) assumptions (28).
For the detection of SNPs for MR analysis, strength, horizontal pleiotropy, and heterogeneity tests were performed. The strength of SNPs was examined using the F-statistic and variance explained (R2), and SNPs with an F-statistic less than 10 were considered weak instrument variables (29). The R2-value (30) and F-statistics (29) are calculated as follows:
In the formula, MAF is the minor allele frequency, β is the effect size of SNP on exposure, SD is the standard deviation, k is the number of instrumental variables, and n is the sample size of exposure.
The existence of horizontal pleiotropy, in which a genetic variant is associated with multiple risk factors on different causal pathways, violates the assumptions underlying MR analysis. The MR-Egger intercept test was used to examine horizontal pleiotropy, and a P-value of less than 0.05 for the intercept was considered to have horizontal pleiotropy (26). Heterogeneity was assessed by Cochran's Q statistic for MR-Egger and IVW analyses, with a P-value for the Q statistic of less than 0.05 considered to be present (31). For SNPs with heterogeneity, the analysis results were based on the random effects model of IVW. The leave-one-out analysis was utilized to assess whether the causal relationship was driven by an individual SNP. In addition, the bidirectional MR analysis was performed to evaluate whether there was a reverse causal association between VEGF levels and atrial fibrillation. All MR analyses were performed using the “TwoSampleMR” package in R software (version 4.2.3). A P-value <0.05 was considered statistically significant and the False Discovery Rate (FDR) was used to correct the P-value and an FDR-corrected P-value <0.1 (PFDR <0.1) was considered to have a significant causal relationship (32, 33).
Results
Characteristics of SNPs for analysis
Table 2 shows the test results of the SNPs used for MR analysis. For the analysis of atrial fibrillation, the number of SNPs used for MR analysis was 19, 13, 27, 19, 15, and 20 for VEGF-A, VEGF-C, VEGF-D, VEGF (uncategorized), VEGFR-2, and VEGFR-3, respectively. The strength test showed that the F-statistic of these included SNPs ranged from 37 to 108, representing that there were no weak instrument variables in these SNPs. The results of the horizontal pleiotropy test showed that the P-values of the intercepts of these SNPs were all greater than 0.05, indicating that there was no horizontal pleiotropy for these SNPs. The heterogeneity test demonstrated that there was no heterogeneity in the analysis between VEGF and atrial fibrillation and flutter (P > 0.05), but there was heterogeneity in the analysis between VEGF-C and atrial fibrillation as well as between VEGF (uncategorized) and atrial fibrillation (P < 0.05).
Causal effect of VEGF on the risk of atrial fibrillation
Table 3 presents the IVW results for the causal relationship between VEGF levels and the risk of atrial fibrillation. In the analysis of atrial fibrillation, genetically predicted high levels of VEGF-A [IVW: odds ratio (OR) = 1.025 (95%CI: 1.004–1.047), P = 0.020, PFDR = 0.060] and VEGF-D [IVW: OR = 1.080 (95%CI: 1.039–1.123), P = 8.66E-05, PFDR = 0.001] were associated with an increased risk of atrial fibrillation, while no causal relationship was observed between VEGF-C (PFDR = 0.419), VEGF (uncategorized) (PFDR = 0.383), VEGFR-2 (PFDR = 0.784), and VEGFR-3 (PFDR = 0.899) and atrial fibrillation risk. In the analysis of atrial fibrillation and flutter, only genetically predicted high levels of VEGF-D [IVW: OR = 1.071 (95%CI: 1.014–1.132), P = 0.014, PFDR = 0.087] increased the risk of atrial fibrillation and flutter. The supplement results of weighted-median, MR-Egger, weighted mode, and MR-PRESSO analyses of the association between VEGF and atrial fibrillation risk were shown in Supplementary Table S1. The direction of the association between VEGF-A and atrial fibrillation, between VEGF-D and atrial fibrillation, and between VEGF-D and atrial fibrillation and flutter results in weighted-median, MR-Egger, weighted mode, and MR-PRESSO analyses remained consistent with IVW. The effect of VEGF on atrial fibrillation in these supplementary analyses was consistent with the results of the IVW analysis. The scatter plots of the association between VEGF-D and the risk of atrial fibrillation and atrial fibrillation and flutter were presented in Figure 2. The leave-one-out analysis demonstrated that the causal association between VEGF-D and the risk of atrial fibrillation and atrial fibrillation and flutter was robust (Figure 3). In addition, the bidirectional MR analysis showed no reverse causal association between VEGF levels and atrial fibrillation (Supplementary Table S2).
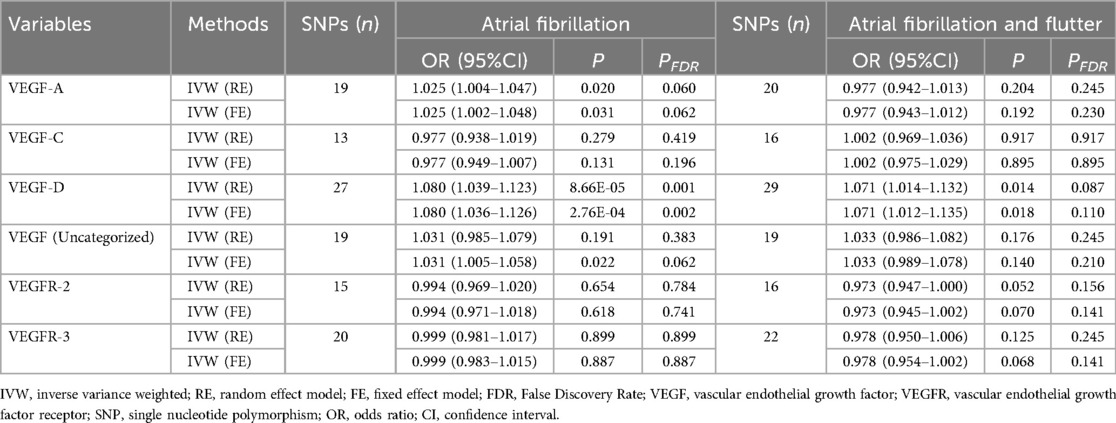
Table 3. The IVW results for the causal relationship between VEGF levels and the risk of atrial fibrillation.
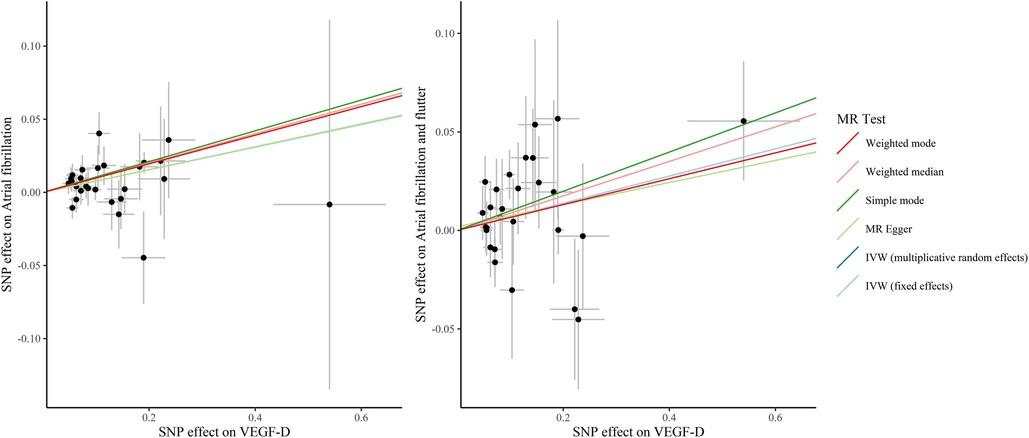
Figure 2. The scatter plots of the association between VEGF-D and the risk of atrial fibrillation and atrial fibrillation and flutter. VEGF, vascular endothelial growth factor; SNP, single nucleotide polymorphism; IVW, inverse variance weighted; MR, Mendelian randomization.
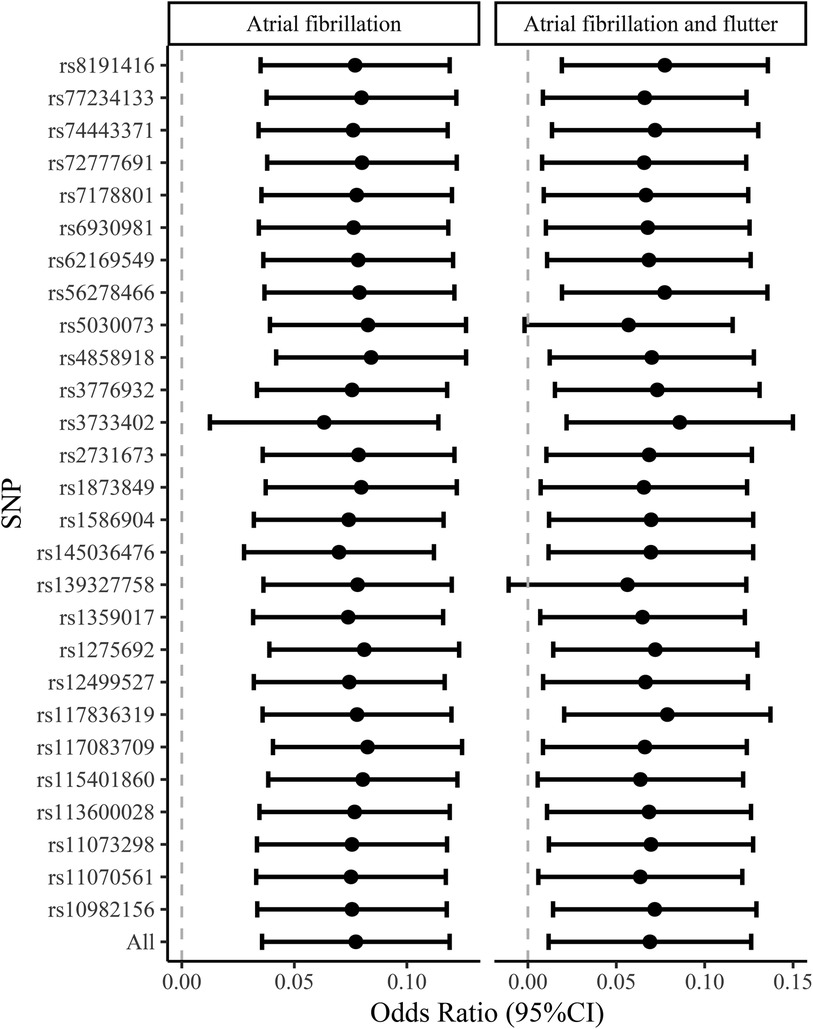
Figure 3. The leave-one-out analysis of the causal association between VEGF-D and the risk of atrial fibrillation and atrial fibrillation and flutter. VEGF, vascular endothelial growth factor.
Discussion
This study assessed the causal relationship between VEGF levels and the risk of atrial fibrillation using MR analysis. The results demonstrated that genetically predicted high levels of VEGF-D increased the risk of atrial fibrillation and atrial fibrillation and flutter. Sensitivity analysis showed that the causal relationship between VEGF-D levels and the risk of atrial fibrillation was robust.
Stretch fibrosis, epicardial adipose tissue, inflammation, autonomic nervous system imbalance, and genetic mutations have been associated with the development of atrial fibrillation (34). The VEGF family are regulators of blood and lymph vessel formation (8). Several studies have found an association between VEGF levels and cardiovascular disease risk (9, 10, 18). An observational study found that elevated VEGF-D levels were associated with an increased risk of atrial fibrillation and ischemic stroke (9). However, observational studies are susceptible to a variety of confounding factors that prevent inferences of causality. Thus, our study investigated whether VEGF levels have a causal effect on the risk of atrial fibrillation. Among the VEGF types analyzed in our study, VEGF-A mainly regulates vascular growth, and VEGF-C and VEGF-D mainly regulate lymphangiogenesis. For VEGF receptors (VEGFR), VEGFR-2 mainly promotes angiogenesis expressed in vascular endothelial cells, and VEGFR-3 mainly promotes lymphogenesis expressed in lymphatic endothelial cells, in which VEGF-A binds to VEGFR-2, while VEGF-C and VEGF-D bind to VEGFR-2 and VEGFR-3 (8). Our results found that genetically predicted high levels of VEGF-D increased the risk of atrial fibrillation. Our findings were consistent with previous observational studies in which elevated AEGF-D levels increased the risk of atrial fibrillation. However, we did not find a causal association between VEGFR-2 and VEGFR-3 levels and the risk of atrial fibrillation. VEGF is involved in a variety of functions in the body such as the promotion of angiogenesis and lymphogenesis, regulation of inflammation, resistance to oxidative stress, fibrosis, and regulation of lipid metabolism (35). The regulation of these functions may be involved in the development of atrial fibrillation.
VEGF-D has been reported to regulate lymphangiogenesis, angiogenesis, and endothelial proliferation via VEGFR-2 or VEGFR-3 (13, 36). The role of VEGF-D and its receptor VEGFR-3 in central pathways for lymphatic vessel development, growth, and maintenance may affect the regulation of interstitial fluid balance and inflammation (37). In addition, VEGF-D may promote cardiac fibrosis by stimulating myofibroblast growth, migration, and collagen synthesis, thereby regulating cardiac repair and remodeling (13). Atrial fibrosis is an important pathophysiological factor in atrial fibrillation and is associated with atrial fibrillation recurrence and complications (38). Elevated VEGF-D levels increase the risk of atrial fibrillation, and higher VEGF-D levels are also observed in patients with atrial fibrillation than in the control population (10). The pulmonary veins are an important source of ectopic beats that trigger atrial tachycardia and atrial fibrillation and maintain atrial fibrillation (39, 40). Atrial fibrillation leads to irregular blood flow, which may cause pulsatile vascular stretching and impaired blood rheology, thereby triggering VEGF secretion from cardiomyocytes and pulmonary vein smooth muscle, and consequently higher VEGF levels are observed in patients with atrial fibrillation (11, 41). However, the biological mechanisms by which elevated VEGF-D levels contribute to the increased risk of atrial fibrillation may need to be further investigated.
The current study explored the causal relationship between VEGF levels and VEGFR levels and the risk of atrial fibrillation using MR analysis. Although the MR study design is less susceptible to potential confounders and inverse causality, several limitations of this study should be considered. First, the GWAS summary data used in this study were obtained from the European population, and the findings may not be generalizable to other ethnic populations. Second, the SNP data related to atrial fibrillation included a small number of individuals from non-European ancestry (nearly 9%), which could have biased the atrial fibrillation-related results. However, we further confirmed the reliability of the association between atrial fibrillation and VEGF levels using atrial fibrillation and flutter data from the FinnGen database. Third, causal associations between VEGF levels and different types of atrial fibrillation (paroxysmal, persistent) could not be analyzed due to a lack of data. Fourth, the lack of individual raw data prevents further exploration of the non-linear association between VEGF levels and the risk of atrial fibrillation. Fifth, the small sample size of the population associated with VEGF in the original GWAS makes the instrumental variables for some of the exposures less well explained. Sixth, there was a 0.32% sample overlap when analyzing the association of VEGF-D and VEGF (Uncategorized) with atrial fibrillation (Supplementary Table S3), which may have affected the relevant results.
Conclusions
For the different types of VEGF (VEGF-A, VEGF-C, VEGF-D) and VEGFR (VEGFR-2, VEGFR-3), only genetically predicted high levels of VEGF-D increased the risk of atrial fibrillation. The causal association between VEGF levels and the risk of different types of atrial fibrillation and the biological mechanism of the effect of VEGF-D levels on the risk of atrial fibrillation may require further investigation.
Data availability statement
The datasets presented in this study can be found in online repositories. The names of the repository/repositories and accession number(s) can be found in the article/Supplementary Material.
Ethics statement
Ethical approval was not required for the study involving humans in accordance with the local legislation and institutional requirements. Written informed consent to participate in this study was not required from the participants or the participants’ legal guardians/next of kin in accordance with the national legislation and the institutional requirements.
Author contributions
SH: Writing – review & editing, Writing – original draft, Visualization, Validation, Supervision, Software, Resources, Project administration, Methodology, Investigation, Funding acquisition, Formal Analysis, Data curation, Conceptualization. LX: Writing – original draft, Writing – review & editing, Visualization, Validation, Supervision, Software, Resources, Project administration, Methodology, Investigation, Funding acquisition, Formal Analysis, Data curation, Conceptualization. CC: Writing – review & editing, Supervision, Resources, Project administration, Methodology, Investigation, Formal Analysis. JX: Writing – review & editing, Project administration, Methodology, Investigation, Formal Analysis, Data curation. FK: Writing – review & editing, Software, Resources, Investigation, Funding acquisition, Data curation, Conceptualization. FZ: Writing – review & editing, Methodology, Investigation, Data curation, Conceptualization.
Funding
The author(s) declare that no financial support was received for the research, authorship, and/or publication of this article.
Conflict of interest
The authors declare that the research was conducted in the absence of any commercial or financial relationships that could be construed as a potential conflict of interest.
Publisher's note
All claims expressed in this article are solely those of the authors and do not necessarily represent those of their affiliated organizations, or those of the publisher, the editors and the reviewers. Any product that may be evaluated in this article, or claim that may be made by its manufacturer, is not guaranteed or endorsed by the publisher.
Supplementary material
The Supplementary Material for this article can be found online at: https://www.frontiersin.org/articles/10.3389/fcvm.2024.1416412/full#supplementary-material
Abbreviations
VEGF, vascular endothelial growth factor; MR, Mendelian randomization; SNPs, single nucleotide polymorphisms; GWAS, genome-wide association studies; VEGFR, vascular endothelial growth factor receptor; LD, linkage disequilibrium; IVW, inverse-variance weighted; FDR, false discovery rate; OR, odds ratio; CI, confidence interval.
References
1. Brundel B, Ai X, Hills MT, Kuipers MF, Lip GYH, de Groot NMS. Atrial fibrillation. Nat Rev Dis Primers. (2022) 8(1):21. doi: 10.1038/s41572-022-00347-9
2. Schnabel RB, Yin X, Gona P, Larson MG, Beiser AS, McManus DD, et al. 50 Year trends in atrial fibrillation prevalence, incidence, risk factors, and mortality in the framingham heart study: a cohort study. Lancet. (2015) 386(9989):154–62. doi: 10.1016/s0140-6736(14)61774-8
3. Lippi G, Sanchis-Gomar F, Cervellin G. Global epidemiology of atrial fibrillation: an increasing epidemic and public health challenge. Int J Stroke. (2021) 16(2):217–21. doi: 10.1177/1747493019897870
4. Kornej J, Börschel CS, Benjamin EJ, Schnabel RB. Epidemiology of atrial fibrillation in the 21st century: novel methods and new insights. Circ Res. (2020) 127(1):4–20. doi: 10.1161/circresaha.120.316340
5. Li J, Solus J, Chen Q, Rho YH, Milne G, Stein CM, et al. Role of inflammation and oxidative stress in atrial fibrillation. Heart Rhythm. (2010) 7(4):438–44. doi: 10.1016/j.hrthm.2009.12.009
6. Karam BS, Chavez-Moreno A, Koh W, Akar JG, Akar FG. Oxidative stress and inflammation as central mediators of atrial fibrillation in obesity and diabetes. Cardiovasc Diabetol. (2017) 16(1):120. doi: 10.1186/s12933-017-0604-9
7. Corban MT, Toya T, Ahmad A, Lerman LO, Lee HC, Lerman A. Atrial fibrillation and endothelial dysfunction: a potential link? Mayo Clin Proc. (2021) 96(6):1609–21. doi: 10.1016/j.mayocp.2020.11.005
8. Apte RS, Chen DS, Ferrara N. Vegf in signaling and disease: beyond discovery and development. Cell. (2019) 176(6):1248–64. doi: 10.1016/j.cell.2019.01.021
9. Berntsson J, Smith JG, Johnson LSB, Söderholm M, Borné Y, Melander O, et al. Increased vascular endothelial growth factor D is associated with atrial fibrillation and ischaemic stroke. Heart. (2019) 105(7):553–8. doi: 10.1136/heartjnl-2018-313684
10. Kaess BM, Preis SR, Beiser A, Sawyer DB, Chen TC, Seshadri S, et al. Circulating vascular endothelial growth factor and the risk of cardiovascular events. Heart. (2016) 102(23):1898–901. doi: 10.1136/heartjnl-2015-309155
11. Scridon A, Morel E, Nonin-Babary E, Girerd N, Fernandez C, Chevalier P. Increased intracardiac vascular endothelial growth factor levels in patients with paroxysmal, but not persistent atrial fibrillation. Europace. (2012) 14(7):948–53. doi: 10.1093/europace/eur418
12. Zhao T, Zhao W, Chen Y, Liu L, Ahokas RA, Sun Y. Differential expression of vascular endothelial growth factor isoforms and receptor subtypes in the infarcted heart. Int J Cardiol. (2013) 167(6):2638–45. doi: 10.1016/j.ijcard.2012.06.127
13. Zhao T, Zhao W, Meng W, Liu C, Chen Y, Bhattacharya SK, et al. Vascular endothelial growth factor-D mediates fibrogenic response in myofibroblasts. Mol Cell Biochem. (2016) 413(1-2):127–35. doi: 10.1007/s11010-015-2646-1
14. Davies NM, Holmes MV, Davey Smith G. Reading Mendelian randomisation studies: a guide, glossary, and checklist for clinicians. Br Med J. (2018) 362:k601. doi: 10.1136/bmj.k601
15. Davey Smith G, Hemani G. Mendelian randomization: genetic anchors for causal inference in epidemiological studies. Hum Mol Genet. (2014) 23(R1):R89–98. doi: 10.1093/hmg/ddu328
16. Zhang Q, Zhang X, Zhang J, Wang B, Tian Q, Meng X, et al. Vascular endothelial growth factor and the risk of venous thromboembolism: a genetic correlation and two-sample Mendelian randomization study. Thromb J. (2022) 20(1):67. doi: 10.1186/s12959-022-00427-6
17. Davidsson P, Eketjäll S, Eriksson N, Walentinsson A, Becker RC, Cavallin A, et al. Vascular endothelial growth factor-D plasma levels and vegfd genetic variants are independently associated with outcomes in patients with cardiovascular disease. Cardiovasc Res. (2023) 119(7):1596–605. doi: 10.1093/cvr/cvad039
18. Au Yeung SL, Lam H, Schooling CM. Vascular endothelial growth factor and ischemic heart disease risk: a Mendelian randomization study. J Am Heart Assoc. (2017) 6(8):e005619. doi: 10.1161/jaha.117.005619
19. Skrivankova VW, Richmond RC, Woolf BAR, Yarmolinsky J, Davies NM, Swanson SA, et al. Strengthening the reporting of observational studies in epidemiology using Mendelian randomization: the strobe-mr statement. JAMA. (2021) 326(16):1614–21. doi: 10.1001/jama.2021.18236
20. Elsworth B, Lyon M, Alexander T, Liu Y, Matthews P, Hallett J, et al. The mrc ieu opengwas data infrastructure. bioRxiv. (2020) 2020:244293. doi: 10.1101/2020.08.10.244293
21. Sun BB, Maranville JC, Peters JE, Stacey D, Staley JR, Blackshaw J, et al. Genomic atlas of the human plasma proteome. Nature. (2018) 558(7708):73–9. doi: 10.1038/s41586-018-0175-2
22. Folkersen L, Gustafsson S, Wang Q, Hansen DH, Hedman Å K, Schork A, et al. Genomic and drug target evaluation of 90 cardiovascular proteins in 30,931 individuals. Nat Metab. (2020) 2(10):1135–48. doi: 10.1038/s42255-020-00287-2
23. Roselli C, Chaffin MD, Weng LC, Aeschbacher S, Ahlberg G, Albert CM, et al. Multi-ethnic genome-wide association study for atrial fibrillation. Nat Genet. (2018) 50(9):1225–33. doi: 10.1038/s41588-018-0133-9
24. Burgess S, Butterworth A, Thompson SG. Mendelian randomization analysis with multiple genetic variants using summarized data. Genet Epidemiol. (2013) 37(7):658–65. doi: 10.1002/gepi.21758
25. Bowden J, Davey Smith G, Haycock PC, Burgess S. Consistent estimation in Mendelian randomization with some invalid instruments using a weighted median estimator. Genet Epidemiol. (2016) 40(4):304–14. doi: 10.1002/gepi.21965
26. Bowden J, Davey Smith G, Burgess S. Mendelian randomization with invalid instruments: effect estimation and bias detection through egger regression. Int J Epidemiol. (2015) 44(2):512–25. doi: 10.1093/ije/dyv080
27. Hartwig FP, Davey Smith G, Bowden J. Robust inference in summary data Mendelian randomization via the zero modal pleiotropy assumption. Int J Epidemiol. (2017) 46(6):1985–98. doi: 10.1093/ije/dyx102
28. Verbanck M, Chen CY, Neale B, Do R. Detection of widespread horizontal pleiotropy in causal relationships inferred from Mendelian randomization between complex traits and diseases. Nat Genet. (2018) 50(5):693–8. doi: 10.1038/s41588-018-0099-7
29. Burgess S, Thompson SG. Bias in causal estimates from Mendelian randomization studies with weak instruments. Stat Med. (2011) 30(11):1312–23. doi: 10.1002/sim.4197
30. Wang Y, Gao L, Lang W, Li H, Cui P, Zhang N, et al. Serum calcium levels and Parkinson’s disease: a Mendelian randomization study. Front Genet. (2020) 11:824. doi: 10.3389/fgene.2020.00824
31. Bowden J, Del Greco MF, Minelli C, Zhao Q, Lawlor DA, Sheehan NA, et al. Improving the accuracy of two-sample summary-data Mendelian randomization: moving beyond the nome assumption. Int J Epidemiol. (2019) 48(3):728–42. doi: 10.1093/ije/dyy258
32. Chen H, Ye B, Su W, Song Y, Sun PL, Zhou X, et al. The causal role of gut Microbiota in susceptibility and severity of COVID-19: a bidirectional Mendelian randomization study. J Med Virol. (2023) 95(7):e28880. doi: 10.1002/jmv.28880
33. Liu Q, Zhou X, Liu K, Wang Y, Liu C, Gao C, et al. Exploring risk factors for autoimmune diseases complicated by non-hodgkin lymphoma through regulatory T cell immune-related traits: a Mendelian randomization study. Front Immunol. (2024) 15:1374938. doi: 10.3389/fimmu.2024.1374938
34. Hu Z, Ding L, Yao Y. Atrial fibrillation: mechanism and clinical management. Chin Med J. (2023) 136(22):2668–76. doi: 10.1097/cm9.0000000000002906
35. Zhou Y, Zhu X, Cui H, Shi J, Yuan G, Shi S, et al. The role of the VEGF family in coronary heart disease. Front Cardiovasc Med. (2021) 8:738325. doi: 10.3389/fcvm.2021.738325
36. Rissanen TT, Markkanen JE, Gruchala M, Heikura T, Puranen A, Kettunen MI, et al. VEGF-D is the strongest angiogenic and lymphangiogenic effector among vegfs delivered into skeletal muscle via adenoviruses. Circ Res. (2003) 92(10):1098–106. doi: 10.1161/01.Res.0000073584.46059.E3
37. Lammoglia GM, Van Zandt CE, Galvan DX, Orozco JL, Dellinger MT, Hyperplasia RJ, et al. And lymphatic regression in mice with tissue-specific, inducible overexpression of murine vegf-D. Am J Physiol Heart Circ Physiol. (2016) 311(2):H384–94. doi: 10.1152/ajpheart.00208.2016
38. Morin DP, Bernard ML, Madias C, Rogers PA, Thihalolipavan S, Estes NA 3rd. The state of the art: atrial fibrillation epidemiology, prevention, and treatment. Mayo Clin Proc. (2016) 91(12):1778–810. doi: 10.1016/j.mayocp.2016.08.022
39. Hsieh MH, Tai CT, Tsai CF, Yu WC, Lee SH, Lin YK, et al. Pulmonary vein electrogram characteristics in patients with focal sources of paroxysmal atrial fibrillation. J Cardiovasc Electrophysiol. (2000) 11(9):953–9. doi: 10.1111/j.1540-8167.2000.tb00166.x
40. Pan NH, Tsao HM, Chang NC, Chen YJ, Chen SA. Aging dilates atrium and pulmonary veins: implications for the genesis of atrial fibrillation. Chest. (2008) 133(1):190–6. doi: 10.1378/chest.07-1769
Keywords: atrial fibrillation, causal association, Mendelian randomization, vascular endothelial growth factor, VEGF-D
Citation: Han S, Xue L, Chen C, Xie J, Kong F and Zhang F (2024) Causal effect of vascular endothelial growth factor on the risk of atrial fibrillation: a two-sample Mendelian randomization study. Front. Cardiovasc. Med. 11:1416412. doi: 10.3389/fcvm.2024.1416412
Received: 5 June 2024; Accepted: 27 September 2024;
Published: 18 October 2024.
Edited by:
Rui Providencia, University College London, United KingdomReviewed by:
Amand Floriaan Schmidt, University College London, United KingdomMarcos Edgar Herkenhoff, University of São Paulo, Brazil
Georgia Damoraki, National and Kapodistrian University of Athens, Greece
Copyright: © 2024 Han, Xue, Chen, Xie, Kong and Zhang. This is an open-access article distributed under the terms of the Creative Commons Attribution License (CC BY). The use, distribution or reproduction in other forums is permitted, provided the original author(s) and the copyright owner(s) are credited and that the original publication in this journal is cited, in accordance with accepted academic practice. No use, distribution or reproduction is permitted which does not comply with these terms.
*Correspondence: Ling Xue, Mjc1MDAzMThAaGVibXUuZWR1LmNu