- 1Guizhou Medical University, Guiyang, Guizhou, China
- 2Department of Ultrasound, Affiliated Hospital of Guizhou Medical University, Guiyang, Guizhou, China
Background: Heart failure is a common cause of adverse cardiovascular outcomes in patients with chronic kidney disease (CKD). Left atrial (LA) characteristics are thought to be involved in the development of heart failure. However, LA assessment is complex. Though a variety of parameters have been defined, there is no single parameter that best defines LA function. Pilot data indicate that left atrial volumetric/mechanical coupling index (LACI) may be useful, but data with CKD are lacking.
Aim: The objective of this study was to define LACI in a cohort of patients with CKD and to assess its value in evaluating LA function and predicting heart failure.
Methods: A cohort of patients with CKD was enrolled at our hospital between 2021 and 2023. Follow-up was performed for heart failure. LACI is a volumetric to mechanical coupling index, calculated as the ratio of the LA volume index to the tissue-Doppler myocardial velocity at atrial contraction. Spearman’s rank correlation or Pearson’s correlation was used to calculate the correlation between LACI and echocardiographic/hemodynamic variables. Receiver operating characteristic curve (ROC) analysis was utilised to derive the area under the curve (AUC) for LACI, LVGLS, LASr, LASct and LASI for the detection of heart failure. Kaplan-Meier survival curves were employed to compare clinical outcomes based on LACI thresholds. A multivariable logistic regression analysis was employed to assess the relationship between risk factors and elevated LACI. Cox proportional hazards regression was used to identify risk factors for heart failure.
Results: LACI showed a positive correlation with NT-proBNP, CK-MB, LAVI, E/e’ and LASI (r = 0.504, 0.536, 0.856, 0.541 and 0.509, p < 0.001); and a negative correlation with LASr (r = −0.509, p < 0.001). On the ROC analysis for the determination of heart failure, the AUC of LACI was comparable to those of LVGLS (0.588 vs. 509, p = 0.464), LASr (0.588 vs. 0.448, p = 0.132), LASct (0.588 vs. 0.566, p = 0.971) and LASI (0.588 vs. 0.570, p = 0.874). The cardiovascular risk factors increased by LACI were age, BMI, diabetes, triglycerides, LA size, LASr, LASI, E/A, E/e’ and EF (p < 0.05). During a median follow-up of 16 months (range, 6–28 months), the event-free survival curves demonstrated a higher risk of heart failure in the group with LACI > 5.0 (log-rank test: P < 0.001). LACI > 5.0 was an independent predictor of heart failure [OR: 0.121, 95% CI (0.020–0.740), p = 0.022].
Conclusion: LACI may prove to be a valuable tool for assessing LA function in patients with CKD, and could be integrated into the routine assessment of LA for the purpose of prognostic assessment and clinical decision-making in patients with CKD.
1 Introduction
Chronic kidney disease (CKD) is a significant global health issue. Individuals with CKD have up to a 30-fold increased risk of cardiovascular mortality compared to the general population and are more likely to die from cardiovascular disease than to progress to dialysis stages (1, 2). Heart failure is one of the most common complications and the major cause of death in patients with CKD (3–5). Studies have demonstrated that echocardiographic findings, including left ventricular hypertrophy (LVH), reduced ejection fraction (EF), and diastolic dysfunction, are predictive of future risk of adverse cardiovascular events and all-cause mortality (6). LA is often considered a passive bystander of these pathophysiologic alterations (7). Yet, heart failure is not wholly caused by impairment of LV structure and function (8). More recently, there is evidence that LA dysfunction in patients with chronic kidney disease (CKD) is independently modulated by renal function, which may be indicative of a CKD-associated atrial myopathy (9). Furthermore, in conditions associated with systemic inflammation, such as CKD, alterations in LA function independent of diastolic dysfunction have been described (10).
Recent studies have highlighted new methods for LA assessment, suggesting that LA characteristics might influence heart failure outcomes (11, 12). Conventionally, LA function is assessed by volumetric measures. Diastolic tissue-Doppler myocardial velocity at atrial contraction (TDI-a’) is an established measure of LA mechanics, highly correlated to complex measures of LA performance (13, 14). Therefore, when assessing LA function, it is important to couple LA volume index (LAVI) to LA mechanical activity (TDI-a’), known as the left atrial volume/mechanical coupling index (LACI), which has shown promising results in other clinical contexts including atrial fibrillation, heart failure, stroke and acute ischaemic heart disease (15–19). However, it remains unclear whether LACI provides incremental information in CKD. We gathered such a cohort and measured LACI for the first time in the context of CKD to evaluate its determinants, and potential value.
2 Methods
2.1 Study participants
A total of 213 patients with chronic kidney disease (CKD) were enrolled in a prospective study conducted at our hospital between 2021 and 2023. Therefore, the study was a retrospective observational analysis of a prospective cohort database. CKD was classified based on the underlying cause, the glomerular filtration rate (GFR) category (G1–G5), and the stage of the disease (20). The following exclusion criteria were applied: significant mitral valve diseases, atrial fibrillation (AF) or flutter, congenital heart disease, or a history of cardiac surgery or permanent pacemaker implantation, cardiomyopathy, pericardial disease, patients with previous heart failure, inadequate echocardiographic acoustic windows, pregnancy, and age under 18 years old. Significant mitral valve disease was defined as more than moderate mitral valve stenosis or regurgitation on transthoracic echocardiography.
2.2 Clinical and echocardiographic data
The patient history and clinical characteristics were extracted from the electronic medical records without any modification. The glomerular filtration rate (GFR) was estimated using the Cockcroft-Gault formula. Furthermore, all patients underwent echocardiography within 48 h of admission.
All echocardiographic measurements were guided by the American Society of Echocardiography recommendations. The LA anteroposterior diameter (LAd) was evaluated from the parasternal long-axis view. The LA volume index (LAVI) corrected for body surface area was derived using the heart model, with the patient’s height and weight entered as inputs. In patients with sinus rhythm, diastolic filling was assessed using early (E) and late (A) inflow velocities, the E/A ratio, e’ (mean of septal and lateral wall derivation) and a’ (mean of septal and lateral wall derivation) derived from tissue Doppler, and the E/e’ ratio. LACI is a volumetric to mechanical coupling index, calculated as the ratio left atrial volume index/tissue-Doppler myocardial velocity at atrial contraction.
Speckle echocardiography was used with in vivo quantitative analysis software to automatically track myocardial motion and generate the region of interest (ROI), measured the LV and LA speckle tracking parameters. LA phasic strains included reservoir phase (LASr), conduit phase (LAScd) and contractility (LASct). The LA stiffness index (LASI = E/e’/LASr) was also calculated. The region of interest was subsequently adjusted in order to obtain the global longitudinal strain of the left ventricle (LVGLS). Digital echocardiographic data containing at least 3 consecutive beats were acquired, transferred to a server for storage and interpreted by 2 independent cardiologists blinded to each other's reading.
2.3 Follow- up data
Participants were followed for a median of 16 months (range: 6–28 months) with the primary outcome being rehospitalization for heart failure, defined by the Framingham criteria (21). All patients were followed up either in outpatient clinics or by telephone conversation.
2.4 Statistical analysis
Data were analyzed using SPSS 27.0. The results are presented as mean ± SD for the normally distributed variables and as median (IQR) for skewed variables. Correlations between LACI and echocardiographic/hemodynamic variables were calculated using Spearman's rank or Pearson correlation. Receiver operating characteristic (ROC) curve analysis was used to obtain the area under the curve (AUC) for LACI, LVGLS, LASr, LASct, and LASI for heart failure detection, with comparisons made using the Delong method. Survival rates (±SE) were estimated using the Kaplan–Meier method and compared using the log-rank test. Determinants of increased LACI were assessed by logistic regression and selected based on pathophysiologic links to LA function. Cox proportional hazards models identified risk factors for heart failure. Variables with p < 0.05 in univariate analysis were included in multifactorial analysis, with significance set at p < 0.05.
3 Results
3.1 Baseline characteristics
Among the 213 CKD patients, 129 (60.6%) were male and 84 (39.4%) were female, with an average age of 57.4 ± 14.3 years. Median follow up was 16 months (interquartile range 13–21 months). Overall 23 patients (10.8%) reached the end point. Baseline clinical and laboratory characteristics of study subjects are shown in Table 1, and echocardiographic measures are shown in Table 2.
3.2 Correlations among LACI, NT-proBNP, CK-MB and echocardiographic parameters
Figure 1 demonstrated that LACI was moderately correlated with NT-proBNP, CK-MB, E/e’, LASI, and LASr (r = 0.504, r = 0.536, r = 0.541, r = 0.603, r = −0.509, P < 0.0001) and highly correlated with LAVI (r = 0.856, P < 0.0001).
3.3 Prognostic performance of LACI
Figure 2 showed the prognostic performance of LACI, LASr, LASct, LASI, and LVGLS for predicting heart failure during follow-up. The AUC of LACI for the entire study population was comparable to that of LVGLS (0.588 vs. 509, P = 0.464), LASr (0.588 vs. 0.448, P = 0.132), LASct (0.588 vs. 566, P = 0.971) and LASI (0.588 vs. 0.570, P = 0.874). A LACI of 5.0 was the best cut-off value to identify heart failure.
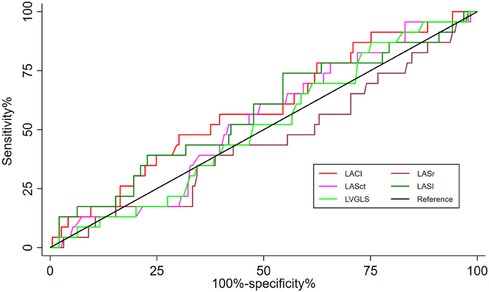
Figure 2 Differences in the prognostic impact of LACI, LVGLS, LASr, LASct, and LASI on hospitalisation for heart failure were compared between groups using the delong test at the 2-year time point, all p > 0.05.
3.4 Determinants of increased LACI
Table 3 showed the increased cardiovascular risk factors for LACI. At multivariable analysis, LACI > 5 was significantly related to age [OR, 1.016 (95% CI, 1.021–1.102), P < 0.05], BMI[OR, 1.029 (95% CI, 1.004–1.055), P < 0.05], diabetes [OR, 1.319 (95% CI, 1.319–13.917), P < 0.05], triglycerides [OR, 0.425 (95% CI, 0.425–0.994), P < 0.05], LASr [OR, 0.877 (95% CI, 0.877–0.976), P < 0.05], LAd [OR, 1.16(95% CI, 1.16–1.479), P < 0.001], LASI [OR, 0.158 (95% CI, 0.158–0.939), P < 0.05], E/A [OR, 1.903 (95% CI, 1.903–25.808), P < 0.05], E/e’ [OR, 1.019 (95% CI, 1.019–1.24), P < 0.05].
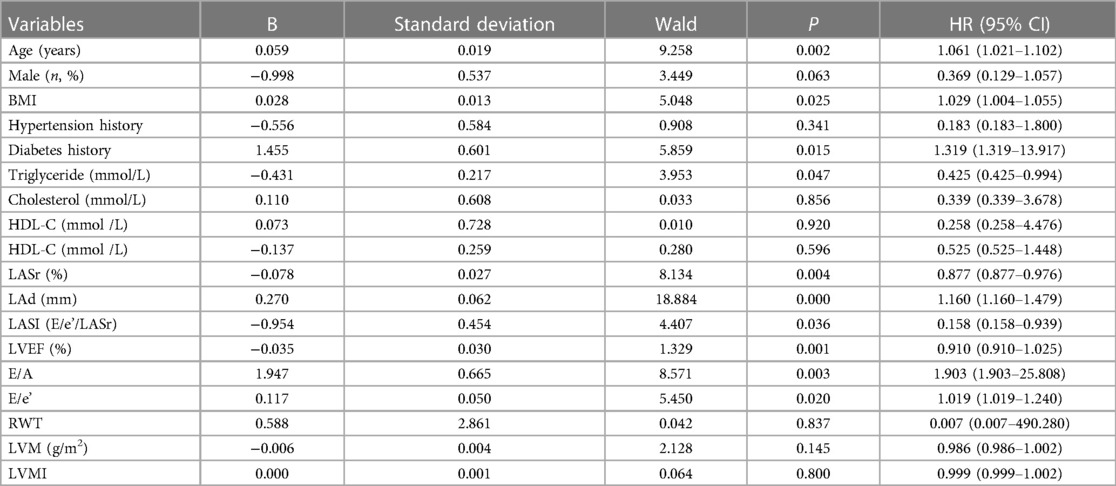
Table 3 Multivariate logistic regression analysis was performed to analyze the determinants of LACI > 5.
3.5 LACI > 5 as a predictor of clinical outcomes
During a median follow-up of 16 months (range, 6 to 28 months), the group with LACI > 5.0 had a higher incidence of heart failure than the group with LACI ≤ 5.0 (9.3% vs. 14.3%, P < 0.05). Event-free survival curves also showed a higher risk of heart failure in the group of LACI > 5.0 (log-rank test; P < 0.001, Figure 3). In Cox proportional hazards multivariate analysis, LACI > 5 was an independent predictor of heart failure (Table 4).
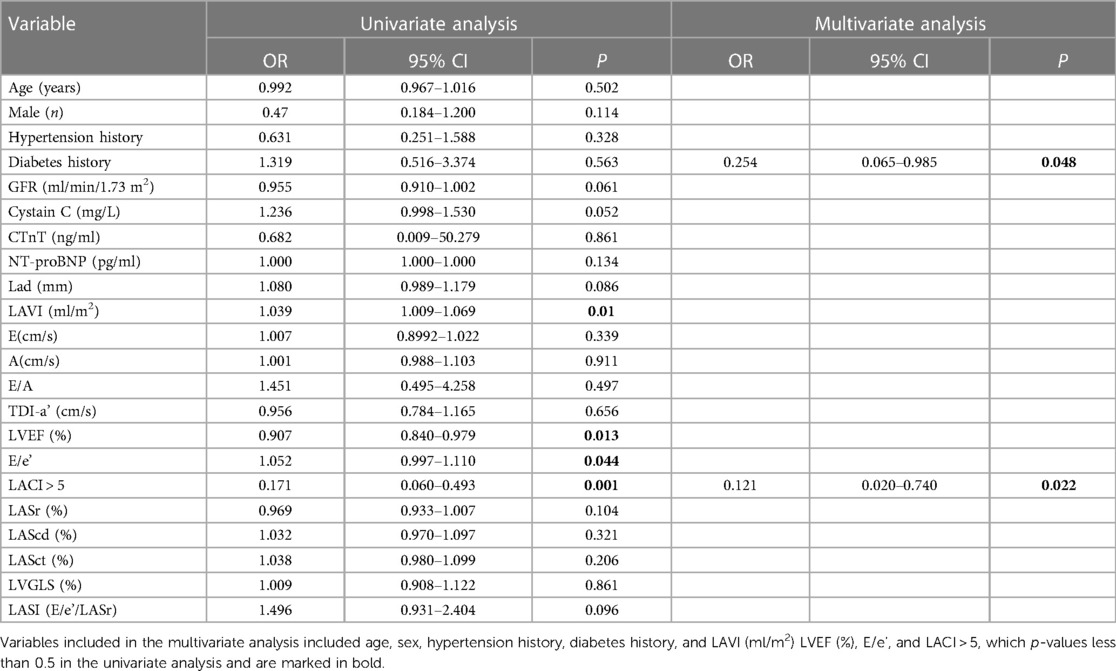
Table 4 Multivariate COX proportional hazards regression models predict heart failure during follow up.
4 Discussion
This study was the first to measure LACI in the context of CKD and to assess the determinants of LACI and the potential incremental value of LACI. The main findings were as follows: (1) LACI correlated well with NT-proBNP, CK-MB, and echocardiographic diastolic parameters; (2) Higher LACI corresponded to weaker atrial mechanical activity for larger volume index, with higher E/e’ and with several markers of increased volume/pressure overload. (3) LACI demonstrated comparable predictive ability to LVGLS, LASr, LASct and LASI in patients with CKD. (4) Risk factors for increased LACI included age, BMI, diabetes, triglycerides, left atrial size, LASr, LASI, EF, E/A, and E/e’. (5) LACI > 5 was an independent predictor of heart failure. Therefore, the atrial coupling index measured by LACI should be part of routine LA assessment in clinical practice, prognostic assessment, and clinical decision-making in patients diagnosed with CKD.
The LA has an important role in modulating LV performance, contributing up to a third of cardiac output (22). LA functions in a three-phase cycle, consisting of a reservoir, conduit, and booster pump, which dynamically interplay with the LV (23). In the early preclinical stages of heart failure, there is a compensatory increase in active LA contribution to LV filling, known as LA booster pump function (8, 24). This contributes to the maintenance of cardiovascular hemodynamics, cardiac output, and neurohumoral balance (25, 26). In patients with CKD, the LA is more sensitive to fluid overload and increased LV filling pressure, which can lead to a decrease in LA contribution to diastolic performance and an increase in LA volume due to elevated LV filling pressure (25, 27). Chronic exposure to elevated LV filling pressure can induce fibrotic changes in the LA, resulting in reduced compliance (28, 29). Previous researchers have found a significant correlation between impaired LV compliance and the degree of LA wall fibrosis as assessed by delayed enhancement magnetic resonance imaging (MRI) (30). Consistent with previous studies (31), our findings also revealed that patients with CKD have impaired LA reserve strain and increased LA stiffness index. The results of a study conducted by Daniel A. Morris et al. (32) demonstrated that the incorporation of LA strain into LAVI significantly enhanced the ability to identify patients at risk of LV diastolic dysfunction with preserved LVEF. This may also account for the similar predictive efficacy observed between LACI and both the LASI and LASct in this study. This is likely due to the impairment of the LA pump function and the concomitant decrease in compliance and increase in stiffness.
The volumetric analysis of the LA using LAVI is an adequate method to estimate the cumulative effect of increased LV filling pressures over time (33). Previous studies found that higher severity of CKD stages was associated with higher values of LA volume (34). The LAVI as an indicator of LA remodeling in chronic kidney disease (35). In addition, diastolic tissue-Doppler myocardial velocity at atrial contraction (TDI-a’) is an established measure of LA mechanics (36). A’ value measurement is a non-invasive and useful method for risk stratification in HFpEF as confirmed by Fumi Oike et al (37). Diastolic late mitral annular velocity (a’) measured by transthoracic echocardiography is reported to represent LA pump function and the severity of LA remodelling (38). It is highly correlated with complex measures of LA performance and can be measured in routine practice (39). Thus, when assessing LA function, it is crucial to combine a measurement of atrial stretch (LAVI) with a measurement of LA mechanical activity (TDI-a’, which is less affected by LV diastolic function than A wave). These complex interactions were consistent with our findings. Cardiovascular risk factors (age, BMI, diabetes mellitus, hyperlipidaemia) contribute to increased LACI, and increased LACI is also associated with reduced LA compliance (reduced LA strain and increased stiffness) and LV diastolic dysfunction. In addition, short-term follow-up results suggested a relationship between LACI and poor prognosis in heart failure.
It should be noted that the study is subject to several limitations. Firstly, the study was conducted at a single centre with a relatively small sample size, which may have implications for the validation of the methodology and the validity of the data. Secondly, due to our short follow-up period, many patients had not yet experienced an endpoint event. We hope that future prolonged follow-up may yield more significant differences and prognostic assessments. Furthermore, the low reproducibility of left atrial volume measurements reported in some of the literature should not be overlooked (40). Finally, patients with advanced chronic kidney disease are more likely to have severe hypertension. Consequently, the efficacy of treatment for hypertension may have influenced the results of our study.
5 Conclusions
LACI is a valuable tool for assessing left atrial function in CKD patients, offering incremental prognostic value beyond traditional measures. It should be included in routine evaluations, with further research needed to standardize clinical thresholds.
Data availability statement
The original contributions presented in the study are included in the article/Supplementary Material, further inquiries can be directed to the corresponding author.
Ethics statement
The requirement of ethical approval was waived by the Ethics Committee of the Affiliated Hospital of Guizhou Medical University for the studies involving humans because The Ethics Committee of the Affiliated Hospital of Guizhou Medical University. The studies were conducted in accordance with the local legislation and institutional requirements. The participants provided their written informed consent to participate in this study.
Author contributions
LJ: Conceptualization, Data curation, Formal Analysis, Investigation, Methodology, Writing – original draft, Writing – review & editing. XG: Conceptualization, Data curation, Investigation, Writing – original draft. WX: Data curation, Investigation, Writing – original draft. SY: Resources, Supervision, Writing – review & editing.
Funding
The author(s) declare financial support was received for the research, authorship, and/or publication of this article.
Supported by Guizhou Provincial Science and Technology Projects (QKHJC-ZK [2023] General 373; QKHCG-LC [2024] 092). Doctoral Research Initiation Fund Project of Affiliated Hospital of Guizhou Medical University, Project Contract: No. gyfybsky-2021-19; Finance Code: J-2021-19.
Conflict of interest
The authors declare that the research was conducted in the absence of any commercial or financial relationships that could be construed as a potential conflict of interest.
Publisher's note
All claims expressed in this article are solely those of the authors and do not necessarily represent those of their affiliated organizations, or those of the publisher, the editors and the reviewers. Any product that may be evaluated in this article, or claim that may be made by its manufacturer, is not guaranteed or endorsed by the publisher.
References
1. Szlagor M, Dybiec J, Mlynarska E, Rysz J, Franczyk B. Chronic kidney disease as a comorbidity in heart failure. Int J Mol Sci. (2023) 24(3):2988. doi: 10.3390/ijms24032988
2. Bikbov B, Purcell CA, Levey AS, Smith M, Abdoli A, Abebe M, et al. Global, regional, and national burden of chronic kidney disease, 1990–2017: a systematic analysis for the global burden of disease study 2017. Lancet. (2020) 395(10225):709–33. doi: 10.1016/S0140-6736(20)30045-3
3. Köttgen A, Gall EC-L, Halbritter J, Kiryluk K, Mallett AJ, Parekh RS, et al. Genetics in chronic kidney disease: conclusions from a kidney disease: improving global outcomes (KDIGO) controversies conference. Kidney Int. (2022) 101(6):1126–41. doi: 10.1016/j.kint.2022.03.019
4. Zoccali C, Mark PB, Sarafidis P, Agarwal R, Adamczak M, Bueno DOR, et al. Diagnosis of cardiovascular disease in patients with chronic kidney disease. Nat Rev Nephrol. (2023) 19(11):733–46. doi: 10.1038/s41581-023-00747-4
5. Chen J, Li Y, Liu P, Wu H, Su G. A nomogram to predict the in-hospital mortality of patients with congestive heart failure and chronic kidney disease. ESC Heart Fail. (2022) 9(5):3167–76. doi: 10.1002/ehf2.14042
6. Panoulas VF, Sulemane S, Konstantinou K, Bratsas A, Elliott SJ, Dawson D, et al. Early detection of subclinical left ventricular myocardial dysfunction in patients with chronic kidney disease. Eur Heart J Cardiovasc Imaging. (2015) 16(5):539–48. doi: 10.1093/ehjci/jeu229
7. Hauser R, Nielsen AB, Skaarup KG, Lassen M, Duus LS, Johansen ND, et al. Left atrial strain predicts incident atrial fibrillation in the general population: the Copenhagen city heart study. Eur Heart J Cardiovasc Imaging. (2021) 23(1):52–60. doi: 10.1093/ehjci/jeab202
8. Bisbal F, Baranchuk A, Braunwald E, Bayes DLA, Bayes-Genis A. Atrial failure as a clinical entity: JACC review topic of the week. J Am Coll Cardiol. (2020) 75(2):222–32. doi: 10.1016/j.jacc.2019.11.013
9. Unger ED, Dubin RF, Deo R, Daruwalla V, Friedman JL, Medina C, et al. Association of chronic kidney disease with abnormal cardiac mechanics and adverse outcomes in patients with heart failure and preserved ejection fraction. Eur J Heart Fail. (2016) 18(1):103–12. doi: 10.1002/ejhf.445
10. Mondillo S, Cameli M, Caputo ML, Lisi M, Palmerini E, Padeletti M, et al. Early detection of left atrial strain abnormalities by speckle-tracking in hypertensive and diabetic patients with normal left atrial size. J Am Soc Echocardiogr. (2011) 24(8):898–908. doi: 10.1016/j.echo.2011.04.014
11. Cameli M, Pastore MC, Mandoli GE. Left atrial strain: a key element for the evaluation of patients with HFpEF. Int J Cardiol. (2021) 323:197–8. doi: 10.1016/j.ijcard.2020.09.078
12. Tanasa A, Tapoi L, Ureche C, Sascau R, Statescu C, Covic A. Left atrial strain: a novel “biomarker” for chronic kidney disease patients? Echocardiography. (2021) 38(12):2077–82. doi: 10.1111/echo.15259
13. Poh KK, Chan MY, Yang H, Yong QW, Chan YH, Ling LH. Prognostication of valvular aortic stenosis using tissue Doppler echocardiography: underappreciated importance of late diastolic mitral annular velocity. J Am Soc Echocardiogr. (2008) 21(5):475–81. doi: 10.1016/j.echo.2007.08.031
14. Nagueh SF, Sun H, Kopelen HA, Middleton KJ, Khoury DS. Hemodynamic determinants of the mitral annulus diastolic velocities by tissue doppler. J Am Coll Cardiol. (2001) 37(1):278–85. doi: 10.1016/S0735-1097(00)01056-1
15. Benfari G, Skaarup KG, Johansen ND, Jensen G, Schnohr P, Mogelvang R, et al. Left atrial volumetric/mechanical coupling index in the general population: distribution and prediction of incident atrial fibrillation: from the Copenhagen city heart study. J Am Soc Echocardiogr. (2023) 36(3):339–41. doi: 10.1016/j.echo.2022.11.013
16. Essayagh B, Benfari G, Antoine C, Maalouf J, Pislaru S, Thapa P, et al. Incremental prognosis by left atrial functional assessment: the left atrial coupling index in patients with floppy mitral valves. J AM HEART ASSOC. (2022) 11(9):e24814. doi: 10.1161/JAHA.121.024814
17. Benfari G, Essayagh B, Nistri S, Maalouf J, Rossi A, Thapa P, et al. Left atrial volumetric/mechanical coupling index: a novel predictor of outcome in heart failure with reduced ejection fraction. Circ Cardiovasc Imaging. (2021) 14(1):e11608. doi: 10.1161/CIRCIMAGING.120.011608
18. Matsuura H, Yamada A, Sugimoto K, Sugimoto K, Iwase M, Ishikawa T, et al. Clinical implication of LAVI over a’ ratio in patients with acute coronary syndrome. Heart Asia. (2018) 10(2):e11038. doi: 10.1136/heartasia-2018-011038
19. Park HJ, Jung HO, Min J, Park MW, Park CS, Shin DI, et al. Left atrial volume index over late diastolic mitral annulus velocity (LAVi/a’) is a useful echo index to identify advanced diastolic dysfunction and predict clinical outcomes. Clin Cardiol. (2011) 34(2):124–30. doi: 10.1002/clc.20850
20. KDIGO 2018 clinical practice guideline for the prevention, diagnosis, evaluation, and treatment of hepatitis C in chronic kidney disease. Kidney Int Suppl (2011). (2018) 8(3):91–165. doi: 10.1016/j.kisu.2018.06.001
21. McKee PA, Castelli WP, McNamara PM, Kannel WB. The natural history of congestive heart failure: the framingham study. N Engl J Med. (1971) 285(26):1441–6. doi: 10.1056/NEJM197112232852601
22. Matsuda Y, Toma Y, Ogawa H, Matsuzaki M, Katayama K, Fujii T, et al. Importance of left atrial function in patients with myocardial infarction. Circulation. (1983) 67(3):566–71. doi: 10.1161/01.CIR.67.3.566
23. Cameli M, Lisi M, Focardi M, Reccia R, Natali BM, Sparla S, et al. Left atrial deformation analysis by speckle tracking echocardiography for prediction of cardiovascular outcomes. Am J Cardiol. (2012) 110(2):264–9. doi: 10.1016/j.amjcard.2012.03.022
24. Tanasa A, Burlacu A, Popa C, Kanbay M, Brinza C, Macovei L, et al. A systematic review on the correlations between left atrial strain and cardiovascular outcomes in chronic kidney disease patients. Diagnostics (Basel). (2021) 11(4):671. doi: 10.3390/diagnostics11040671
25. Guo L, Fu B, Liu Y, Hao N, Ji Y, Yang H. Diuretic resistance in patients with kidney disease: challenges and opportunities. Biomed Pharmacother. (2023) 157:114058. doi: 10.1016/j.biopha.2022.114058
26. Mark PB, Mangion K, Rankin AJ, Rutherford E, Lang NN, Petrie MC, et al. Left ventricular dysfunction with preserved ejection fraction: the most common left ventricular disorder in chronic kidney disease patients. Clin Kidney J. (2022) 15(12):2186–99. doi: 10.1093/ckj/sfac146
27. Song J, Navarro-Garcia JA, Wu J, Saljic A, Abu-Taha I, Li L, et al. Chronic kidney disease promotes atrial fibrillation via inflammasome pathway activation. J Clin Invest. (2023) 133(19):e167517. doi: 10.1172/JCI167517
28. Kadappu KK, Kuncoro AS, Hee L, Aravindan A, Spicer ST, Suryanarayanan G, et al. Chronic kidney disease is independently associated with alterations in left atrial function. Echocardiography. (2014) 31(8):956–64. doi: 10.1111/echo.12503
29. Della RD, Magnocavallo M, Van Niekerk CJ, Gilhofer T, Ha G, D'Ambrosio G, et al. Prognostic value of chronic kidney disease in patients undergoing left atrial appendage occlusion. Europace. (2023) 25(11):euad315. doi: 10.1093/europace/euad315
30. Kuppahally SS, Akoum N, Burgon NS, Badger TJ, Kholmovski EG, Vijayakumar S, et al. Left atrial strain and strain rate in patients with paroxysmal and persistent atrial fibrillation: relationship to left atrial structural remodeling detected by delayed-enhancement MRI. Circ Cardiovasc Imaging. (2010) 3(3):231–9. doi: 10.1161/CIRCIMAGING.109.865683
31. Sonaglioni A, Cara MD, Nicolosi GL, Eusebio A, Bordonali M, Santalucia P, et al. Rapid risk stratification of acute ischemic stroke patients in the emergency department: the incremental prognostic role of left atrial reservoir strain. J Stroke Cerebrovasc Dis. (2021) 30(11):106100. doi: 10.1016/j.jstrokecerebrovasdis.2021.106100
32. Morris DA, Belyavskiy E, Aravind-Kumar R, Kropf M, Frydas A, Braunauer K, et al. Potential usefulness and clinical relevance of adding left atrial strain to left atrial volume index in the detection of left ventricular diastolic dysfunction. JACC Cardiovasc Imaging. (2018) 11(10):1405–15. doi: 10.1016/j.jcmg.2017.07.029
33. Nagueh SF, on behalf of the ASE and EAE Diastology Writing Group Letter by Nagueh, et al. Regarding article, “tissue doppler imaging in the estimation of intracardiac filling pressure in decompensated patients with advanced systolic heart failure”. Circulation. (2009) 120(7):e44. doi: 10.1161/CIRCULATIONAHA.109.856187
34. Nguyen H, Do CV, Dang D, Do LD, Doan LH, Dang H. Progressive alterations of left atrial and ventricular volume and strain across chronic kidney disease stages: a speckle tracking echocardiography study. Front Cardiovasc Med. (2023) 10:1197427. doi: 10.3389/fcvm.2023.1197427
35. Rudenko T, Kamyshova E, Bobkova I. The left atrial volume index as an indicator of left atrial remodeling in chronic kidney disease. Saudi J Kidney Dis Transpl. (2021) 32(1):273–5. doi: 10.4103/1319-2442.318540
36. Khankirawatana B, Khankirawatana S, Peterson B, Mahrous H, Porter TR. Peak atrial systolic mitral annular velocity by Doppler tissue reliably predicts left atrial systolic function. J Am Soc Echocardiogr. (2004) 17(4):353–60. doi: 10.1016/j.echo.2003.12.023
37. Oike F, Yamamoto E, Sueta D, Tokitsu T, Usuku H, Nishihara T, et al. Clinical significance of diastolic late mitral annular velocity in heart failure with preserved ejection fraction. Int J Cardiol. (2020) 316:145–51. doi: 10.1016/j.ijcard.2020.03.077
38. Masuda M, Iwakura K, Inoue K, Okamura A, Koyama Y, Toyoshima Y, et al. Estimation of left atrial blood stasis using diastolic late mitral annular velocity. Eur Heart J Cardiovasc Imaging. (2013) 14(8):752–7. doi: 10.1093/ehjci/jes241
39. de Simone G, Mancusi C. Diastolic function in chronic kidney disease. Clin Kidney J. (2023) 16(11):1925–35. doi: 10.1093/ckj/sfad177
Keywords: left atrial volumetric/mechanical coupling index, left atrium, left atrial function, chronic kidney disease, heart failure
Citation: Ji L, Gao X, Xiao W and Yu S (2024) Assessment of left atrial function provides incremental value: the left atrial volumetric/mechanical coupling index in patients with chronic kidney disease. Front. Cardiovasc. Med. 11:1407531. doi: 10.3389/fcvm.2024.1407531
Received: 26 March 2024; Accepted: 14 June 2024;
Published: 9 July 2024.
Edited by:
David C. Rotzinger, Centre Hospitalier Universitaire Vaudois (CHUV), SwitzerlandReviewed by:
Stefano Nistri, CMSR Veneto Medica, ItalyAndrea Sonaglioni, IRCCS MultiMedica, Italy
Tetsuji Kitano, University of Occupational and Environmental Health, Japan
© 2024 Ji, Gao, Xiao and Yu. This is an open-access article distributed under the terms of the Creative Commons Attribution License (CC BY). The use, distribution or reproduction in other forums is permitted, provided the original author(s) and the copyright owner(s) are credited and that the original publication in this journal is cited, in accordance with accepted academic practice. No use, distribution or reproduction is permitted which does not comply with these terms.
*Correspondence: Shaomei Yu, MTg4ODUxMTc3OTdAcXEuY29t