Corrigendum: Genetically predicted lipids mediate the association between intrahepatic cholestasis of pregnancy and cardiovascular disease
- 1Department of Obstetrics and Gynecology, The Second Affiliated Hospital of Chongqing Medical University, Chongqing, China
- 2Department of Hepatobiliary Surgery, The Second Affiliated Hospital of Chongqing Medical University, Chongqing, China
Introduction: Intrahepatic cholestasis of pregnancy (ICP), the most prevalent liver disorder specific to pregnancy, affects approximately 1.5%-4% of pregnancies. However, the influence of ICP on cardiovascular disease (CVD), including hypertension (HTN) and coronary artery disease (CAD), has not been thoroughly investigated.
Methods: This study explores the causal relationship between ICP and CVD (HTN, CAD) using Mendelian Randomization (MR). Utilizing summary-level data from Genome-Wide Association Studies (GWAS), we applied the inverse-variance weighted (IVW) method, supplemented by sensitivity and reverse MR analyses, to ascertain robustness.
Results: Our findings reveal significant causal links, indicating ICP notably increases the risk of CVD (P = 0.001), hypertension (HTN, P = 0.024), and coronary artery disease (CAD, P = 0.039). A two-step MR analysis highlighted the mediation role of lipid profiles, with LDL, TC, and Apo-B contributing to increased CVD risk by 25.5%, 12.2%, and 21.3%, respectively. Additionally, HTN was identified as a mediator in the ICP-CAD association, accounting for a 14.5% mediation effect.
Discussion: The results underscore the genetic predisposition of ICP to elevate CVD risk and the critical mediating role of lipid levels, emphasizing the need for vigilant lipid monitoring and early intervention in individuals with ICP.
1 Introduction
Intrahepatic cholestasis of pregnancy (ICP), the most prevalent liver disorder specific to pregnancy, affects approximately 1.5%–4% of pregnancies (1, 2). The incidence of ICP varies according to geographic location and ethnicity, with a higher incidence in South American populations and a lower incidence in European populations (3). Its clinical manifestations include pruritus and elevated levels of serum bile acids and transaminases (4). Typical symptoms of ICP begin to appear around the third trimester of pregnancy and the condition worsens as the pregnancy progresses. Typically, ICP symptoms subside within 48 h postpartum, and biochemical irregularities normalize within 2–8 weeks (5).
Notably, ICP is linked to several adverse perinatal outcomes, including preterm labor, unexplained stillbirth, and postpartum hemorrhage (6, 7). The pathogenesis of sudden intrauterine death related to ICP remains unclear, but it is hypothesized to involve disruptions in fetal circulation due to abnormal bile acid concentrations. Recent studies of ICP fetal outcomes have shown that the risk of adverse fetal outcomes increases with increasing maternal serum bile acid levels (8). The most effective pharmacologic treatment to improve clinical symptoms and biochemical abnormalities in patients with ICP is Ursodeoxycholic acid (UDCA), and this has also been shown to reduce placental abnormalities and improve placental bile acid transport in in vitro studies (9, 10).
The pathogenesis of ICP is multifactorial, involving environmental factors, hormonal changes, and genetic mutations (11). ICP is more likely to occur in winter and in populations in areas with lower dietary selenium intake, suggesting that environmental factors play a role in the development of ICP (12). Reproductive hormones also play a role in ICP, and women with higher levels of estrogen and progesterone are more likely to experience ICP symptoms (13). In terms of genetic factors, the more widely studied gene is ABCB4, and mutations at loci such as ABCB11 and ABCC2 have also been reported in ICP (14).
Associations between ICP and various conditions, such as hepatitis C, non-alcoholic fatty liver disease, cholecystitis, pancreatitis, and autoimmune diseases, have been documented (15). Several studies have shown that ICP may coexist with other pregnancy-related conditions such as pre-eclampsia, acute fatty liver of pregnancy and gestational diabetes (16). Previous studies have shown that ICP patients have a threefold increased risk of gestational diabetes and pre-eclampsia compared to normal pregnant women (17). Both gestational diabetes and pre-eclampsia are recognized as risk factors for cardiovascular disease (18). However, the impact of ICP on cardiovascular disease (CVD) remains underexplored, with most research focusing on fetal cardiac implications (19). Observational studies have indicated an elevated risk of preeclampsia in women with a history of ICP (20). Yet, the influence of ICP on adult cardiovascular event risk, including hypertension (HTN) and coronary artery disease (CAD), requires further investigation. Currently, only a few observational studies have reported on the relationship between ICP and cardiovascular disease, and they have not obtained uniform results. Traditional observational studies in this context are often subject to limitations like residual confounding and reverse causality bias.
Mendelian randomization (MR) refers to an analytical method for assessing causal relationships between observed modifiable exposures or risk factors and clinically relevant outcomes. It provides a valuable tool, especially when randomized controlled trials examining causality are not feasible and when observational studies provide biased associations due to confounding or reverse causality. These issues are addressed by using genetic variants as instrumental variables (IVs) for testing exposure. Because alleles of exposure-related genetic variants are randomly assigned, the results obtained by MR are not affected by confounders and reverse causation (21). Large-scale genome-wide association studies (GWAS) conducted over the past decade have identified many genetic variants associated with cardiometabolic traits and risk factors. These findings have enabled the design of MR, which has been increasingly applied to predict cardiovascular risk factors in recent years. In this study, we used two-sample and two-step MR to explore the relationship between ICP and cardiovascular disease and to elucidate potential mediators in the pathway linking ICP with cardiovascular disease.
2 Methods
2.1 Study design
In this study, we used two-sample MR analysis (TSMR) and two-step MR to investigate the causal associations between ICP and cardiovascular disease, using summary statistics from genome-wide association studies (GWAS). This study adhered to the key principles outlined in the Strengthening the Reporting of Observational Studies in Epidemiology Mendelian randomization (STROBE-MR) guidelines (22). To ensure the accuracy and reliability of the results, this MR study strictly followed the three basic assumptions of Mendelian randomization. First, IVs must be strongly correlated with exposure factors. Second, IVs cannot be directly correlated with the outcome. Finally, IVs must be excluded from being associated with any confounding factors. Figure 1 illustrates the basic assumptions of MR and our study design.
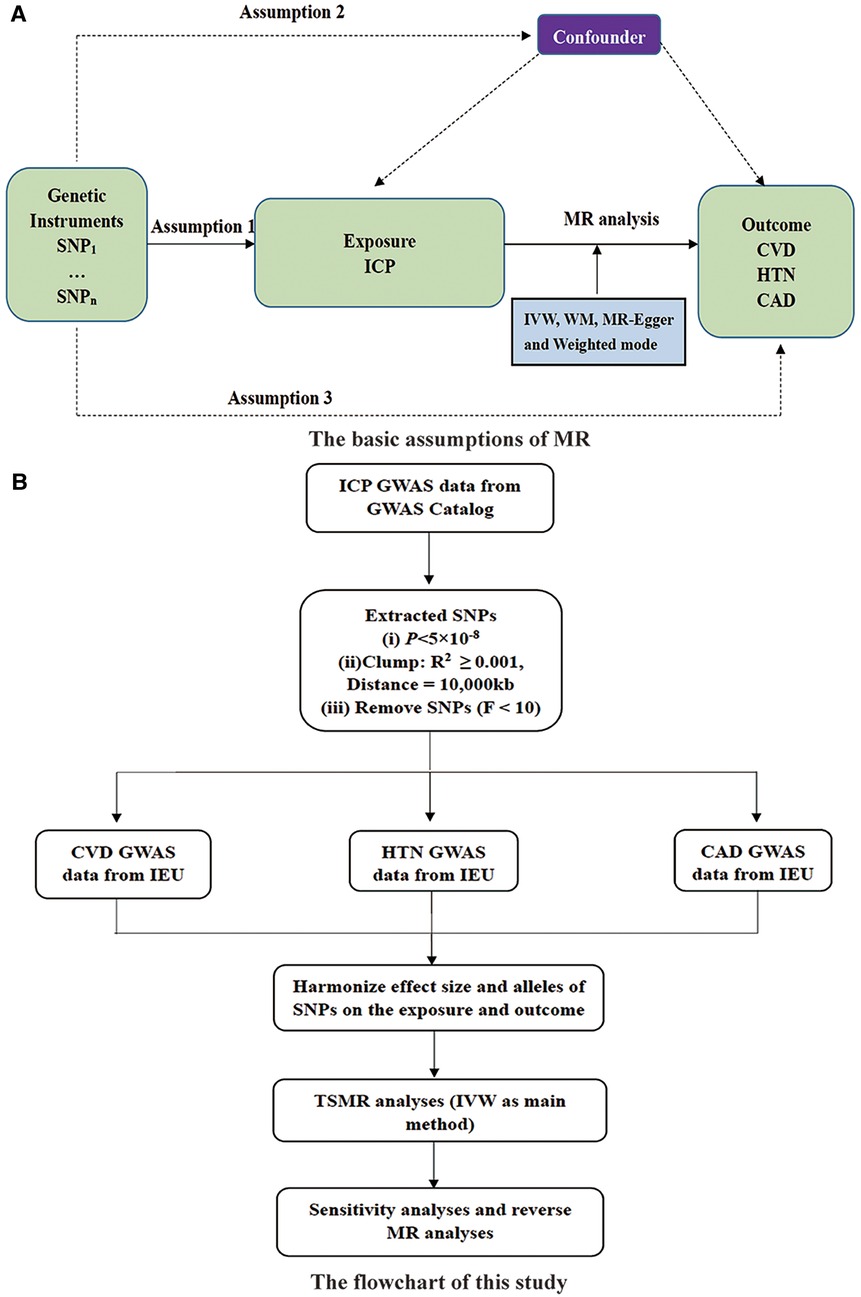
Figure 1 (A) The basic assumptions of MR and (B) study design. SNP, single nucleotide polymorphisms; ICP, intrahepatic cholestasis of pregnancy; IVW, inverse variance weighted; WM, weighted median; CVD, cardiovascular disease; HTN, hypertension; CAD, coronary artery disease; GWAS, genome-wide association studies; IEU, integrative epidemiology unit open GWAS project.
2.2 Data sources
The exposure in this MR study was ICP, and the outcome factors were CVD, HTN, and CAD. This study categorizes the potential mediator factors into two major groups, as follows: (1) Blood lipids: low-density lipoprotein [LDL], high-density lipoprotein [HDL], total cholesterol [TC], triglycerides [TG], apolipoprotein A [Apo-A], and apolipoprotein B [Apo-B]; (2) Liver function markers: alanine aminotransferase [ALT], aspartate aminotransferase [AST], alkaline phosphatase [ALP], gamma-glutamyl transferase [γ-GGT], and bile acid. The GWAS summary-level data for ICP were derived from a study by Dixon PH et al. through GWAS Catalog (https://www.ebi.ac.uk/gwas/), which conducted GWAS and a meta-analysis across three studies on ICP, encompassing a total of 1,138 cases and 153,642 controls (23). The GWAS summary-level data about CVD, HTN, CAD, blood lipids and liver function markers used in this study was issued by the Integrative Epidemiology Unit (IEU) open GWAS project (https://gwas.mrcieu.ac.uk/). This project, supported by the Medical Research Council Integrative Epidemiology Unit (MRC IEU) at the University of Bristol, collated and analyzed GWAS data from UK Biobank (24), published articles, and FinnGen biobank (25). There is no significant sample overlap between the GWAS data. More information about the GWAS summary-level data used in this study is presented in Table 1.
2.3 The selection of IVs
In MR analyses, single nucleotide polymorphisms (SNPs) were used as instrumental variables to represent exposures and outcomes to explore causal relationships between them. We screened for SNPs that were strongly associated with exposure at a genome-wide significance level (P < 5 × 10−8). A total of 11 SNPs were associated with ICP at the genome-wide significant threshold. All of them were not in linkage disequilibrium (LD, R2 ≥ 0.001 and within 10 mb) and not overlapped with the known risk of CVD. Furthermore, to assess the strength of the screened IVs, this study introduced the F statistic to reflect the ability of the IVs to represent the phenotype. The F statistic was calculated from the sample size, the number of IVs, the minor allele frequency (MAF), and the β-value (26). IVs with F-statistics <10 were regarded as weak genetic instruments. In this study, SNPs with F statistic less than 10 should be removed (27). The details of instrumental SNPs in this study are shown in Table 2. All the F-statistics in this study are greater than 10, indicating that the IVs used satisfy the requirement of a strong association with exposures.
2.4 Statistical analysis
In this study, we utilized four MR methods—Inverse Variance Weighted (IVW), Weighted Median (WM), MR-Egger, and Weighted Mode—to assess the causal impact of ICP on CVD. Each method is based on distinct assumptions regarding horizontal pleiotropy. Primarily, the IVW approach synthesizes Wald ratios (the ratio of the SNP-associated outcome effect to the SNP-associated exposure effect) through meta-analysis to deduce the aggregate causal relationship between the exposure and the outcome (28). To mitigate reverse causation, we conducted a reverse MR analysis, which swaps the roles of exposure and outcome. The IVW model's heterogeneity was evaluated using Cochran's Q test, with a p-value less than 0.05 signifying heterogeneity (29). Furthermore, the Mendelian Randomization Pleiotropy Residual Sum and Outlier (MR-PRESSO) test was applied to ascertain the degree of horizontal pleiotropy among the IVs (30). A leave-one-out analysis was also conducted to determine the influence of individual SNPs on the overall results, leading to the immediate exclusion of any identified outliers. Subsequent analyses were carried out post-outlier removal.
A two-step MR analysis was employed to investigate potential mediation effects by lipid profiles (LDL, HDL, TC, TG, Apo-A and Apo-B) and liver function markers (ALT, AST, ALP, γ-GGT, and bile acid) in the relationship between ICP and VCD. The principle of two-step MR analysis was shown in Figure 2. In the two-step MR analysis, βa is the effect of ICP on the mediator and βb is the effect of the mediator on CVD. βc denotes the total effect (TE) of ICP on CVD and βc’ denotes the direct effect (DE) of ICP on CVD (βc’ = βc—βa*βb). We estimated the proportion of individual mediation by dividing the individual mediated effect (IE) (βa*βb) by the total effect (βc) (31). The indirect effects of ICP on VCD through mediators were quantified using the product of coefficients and the multivariate delta methods. All analyses were performed in R software (version 4.2.0) using the Two Sample MR and MVMR package. In this study, P < 0.05 was considered to have a causal relationship. MR estimates were shown as odds ratios (OR) with 95% confidence intervals (CI) or β estimates with 95% CI.
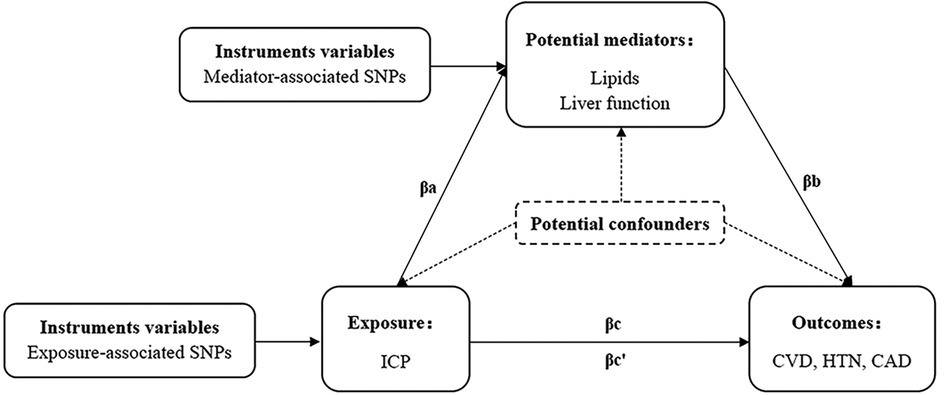
Figure 2 The principle of two-step MR analysis. SNP, single nucleotide polymorphisms; ICP, intrahepatic cholestasis of pregnancy; CVD, cardiovascular disease; HTN, hypertension; CAD, coronary artery disease.
3 Results
3.1 Causal effect between ICP and CVD (CAD, HTN) via TSMR
In this investigation, three causal associations were established using the IVW method (P < 0.05, Figure 3). It was determined that ICP elevates the risk of CVD, as evidenced by an Odds Ratio (OR) of 1.004 and a 95% CI ranging from 1.002 to 1.007 (P = 0.001). Comparable causal relationships were observed in the WM analysis, yielding an OR of 1.005 with a 95% CI from 1.003–1.008 (P = 7.760 × 10−6). Furthermore, a significant correlation between ICP and an augmented risk of HTN was identified through the IVW method, with an OR of 1.002 and a 95% CI between 1.000 and 1.005 (P = 0.024). Consistent risk estimates were also derived from MR-Egger, WM, and Weighted Mode analyses. Additionally, a notable link between ICP and CAD was detected via IVW analysis, indicated by an OR of 1.039 and a 95% CI from 1.002 to 1.079 (P = 0.039). Reverse MR analysis revealed that CVD, CAD, and HTN do not influence the likelihood of developing ICP. Further details of MR analyses and reverse MR analyses are presented in Supplementary Material Table S1 and S2.
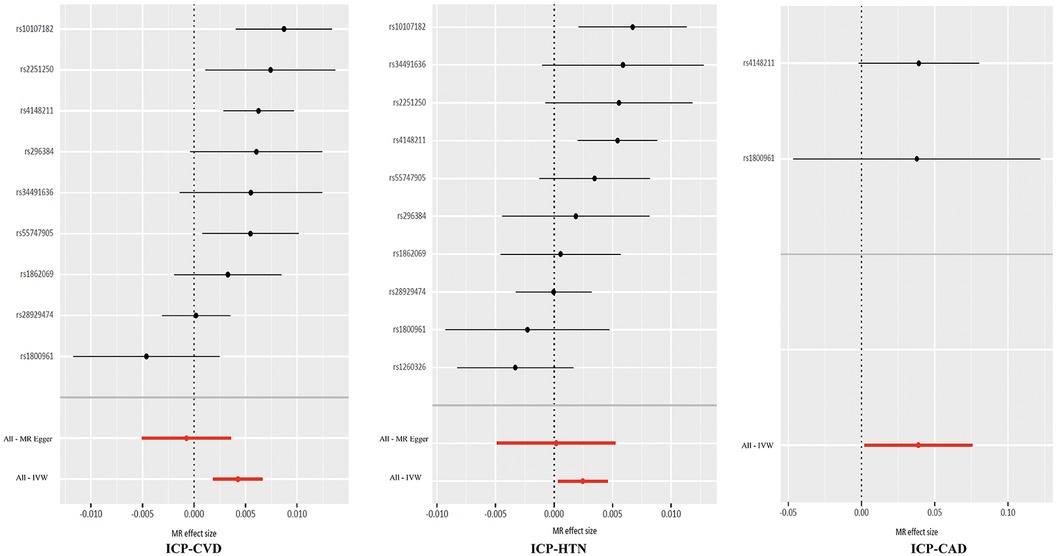
Figure 3 Three causal associations between ICP and CVD, HTN and CAD. ICP, intrahepatic cholestasis of pregnancy; CVD, cardiovascular disease; HTN, hypertension; CAD, coronary artery disease.
After removing outlier SNP, the causal relationship between ICP and CVD still remained. The robustness of the results after removing outlier SNP was assessed through Cochran's Q test, the MR-Egger intercept test and MR-PRESSO, as detailed in Supplementary Material Table S3. The MR-Egger intercept tests yielded P-values greater than 0.05, indicating an absence of horizontal pleiotropy. Moreover, the lack of detected pleiotropy by the Egger intercept implies that the MR estimates remained unbiased by pleiotropy, notwithstanding observed heterogeneity. The results of the leave-one-out analyses are depicted in Figure 4.
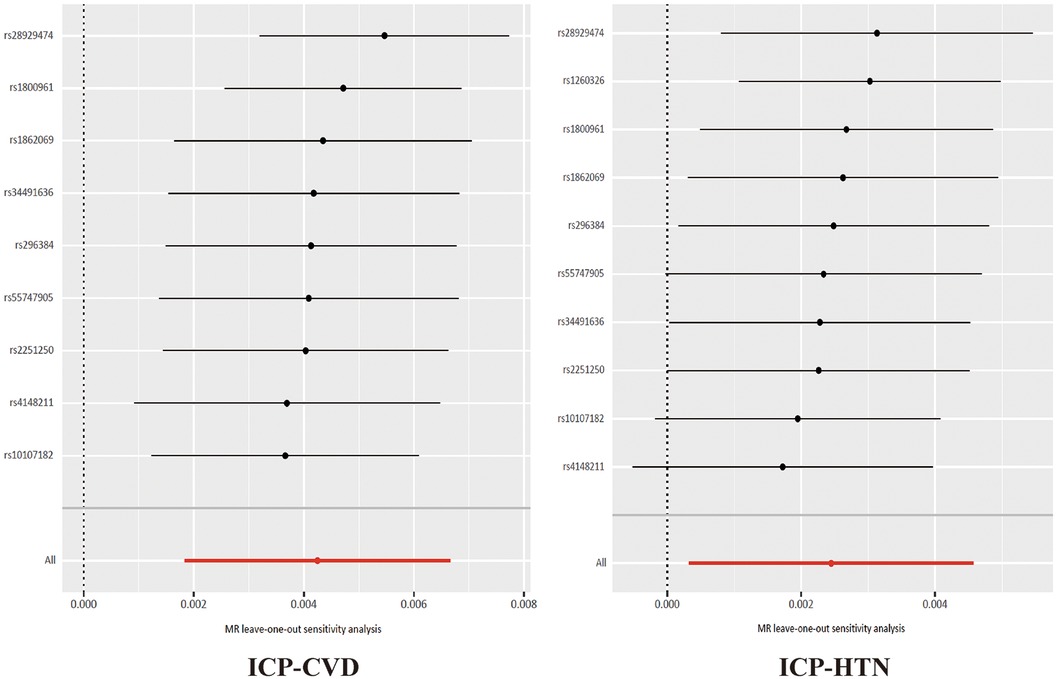
Figure 4 The results of the leave-one-out analyses about ICP, CVD and HTN. ICP, intrahepatic cholestasis of pregnancy; CVD, cardiovascular disease; HTN, hypertension.
3.2 Causal effects of ICP, lipid traits, liver function, and CVD (CAD, HTN) via two-step MR mediation analysis
To investigate the potential mediation of six lipid profiles (LDL, HDL, TC, TG, Apo-A, Apo-B) and five liver function indices (ALT, AST, ALP, γ-GGT, Bile acid) in the relationship between ICP and CVD, CAD, and HTN, a two-step MR analysis was employed (Figure 2). This analysis identified lipids, notably LDL, TC, and Apo-B, as significant mediators in the impact of ICP on CVD, CAD, and HTN (P < 0.05). Detailed results of the screening of mediators are shown in Supplementary Material Table S4. However, the liver function indices did not exhibit a substantial mediating effect on the association between ICP and CVD (P > 0.05). Specifically, the analysis demonstrated that ICP indirectly increased the risk of CVD by elevating levels of LDL, TC, and Apo-B, with their respective mediation percentages (IE/TE) being 25.5%, 12.2%, and 21.3%. Further exploration into the mediation effect between ICP and CAD indicated that LDL alone contributed a 23.7% mediation effect, while TC and Apo-B showed no significant mediation. Moreover, LDL and Apo-B were found to mediate the relationship between ICP and HTN, with mediation effects of 11.0% and 9.3%, respectively, but no mediating role for TC was observed. Additionally, the analysis extended to the interplay between ICP, CAD, and HTN, revealing that HTN could act as a mediator in the increased risk of CAD attributable to ICP, with a mediation effect of 14.5%. Details of the two-step MR analysis were provided in Table 3.
In conclusion, our findings delineate two primary mediating pathways linking ICP with CVD: Firstly, ICP exerts an indirect causal influence on the risk of CVD, including CAD and HTN, by modulating the concentrations of LDL, TC, and Apo-B. Secondly, ICP indirectly impacts the risk of CAD through its effect on the likelihood of developing HTN.
4 Discussion
While the causal link between ICP and CVD remains ambiguous, our MR analysis supports a causal association of ICP with an enhanced risk of CVD, corroborating the observational findings of Shemer et al. (32) Their study reported a marginally increased risk of CVD in later life stages among women with ICP [Hazard Ratio (HR) 1.12, 95% CI 1.06–1.19]. Conversely, the research conducted by Suvi-Tuulia Hämäläinen et al. indicated a lower incidence of CVD-related mortality in women with ICP compared to a control group, a variance potentially attributed to factors such as age at enrollment and follow-up duration (33). Additionally, this analysis extended to explore the causal connections of ICP with CAD and HTN, suggesting that ICP elevates the risks of both conditions. While studies focusing on the ICP-CAD nexus are scant, limited cohort research has hinted at a divergent risk profile for CAD in women with a history of ICP compared to those without. Furthermore, it is widely acknowledged that ICP predisposes to hypertensive complications during pregnancy, and our findings affirm that this risk persists into later life. This study is the first research to find a causal relationship between ICP and CVD at the genetic level using MR methods, which is important for deepening the understanding of ICP complications and guiding follow-up protocols.
Factors such as lipid dysregulation, insulin resistance, endothelial damage, and an enhanced systemic inflammatory response may contribute to the increased CVD risk associated with ICP (34). This study focused on the role of dyslipidemia in abnormal pathophysiologic processes. Research by Chen Y et al. demonstrated a higher prevalence of hyperlipidemia in individuals with ICP compared to those without (5.96% vs. 3.80%), identifying ICP as an independent risk factor for dyslipidemia (35). Building on this, our study employed mediator MR analysis to substantiate the significant indirect effect of ICP on CVD via lipid levels. This mediational MR analysis revealed that LDL, TC, and Apo-B predominantly mediate the indirect impact of ICP on CVD, aligning with the meta-analysis findings of Zhan Y et al. Zhang's research further indicated that severe maternal dyslipidemia was more common in the severe ICP cohort, hinting at a potential link between the severity of ICP and dyslipidemia (36). LDL plays a key role in the development of atherosclerosis (37). LDL is converted from very low-density lipoprotein (VLDL). LDL particles contain about 50% cholesterol and are the most cholesterol-rich lipoproteins in the blood, so they are called cholesterol-rich lipoproteins (38). 95% or more of the Apo in LDL is Apo-B (39). LDL carries cholesterol to peripheral tissues, and most of it is metabolized through the catabolism of the LDL receptor (LDLR) in the hepatocytes and extrahepatic tissues. The pathways through which ICP disrupts lipid metabolism remain intricate, with some evidence pointing to anomalies in farnesoid X receptor (FXR) activity (40). Activating FXR leads to a suppression of endogenous bile acid synthesis while concurrently decreasing levels of triglycerides, total cholesterol, and glucose in the plasma (41). Increased levels of the 3β-sulfated progesterone metabolite epiallopregnanolone sulfate in ICP pregnancies antagonized the FXR (42, 43). Hence, the diminished activity of FXR could play a role in pregnancies with ICP, potentially affecting maternal metabolic processes (44). Although the mechanism is not yet clear, even the detection and intervention of dyslipidemia has important clinical value for the treatment of the disease itself and related complications in patients with ICP. In addition, it has been shown that gut microbiota may also play a role in lipid metabolism during ICP pregnancies (45). Although high bile acid levels have been implicated in cardiovascular toxicity, our MR analysis did not find substantial evidence to support the mediating role of liver function markers, including bile acids, in the association between ICP and CVD.
Interestingly, this analysis revealed a mediating role of HTN in the relationship between ICP and CAD. The connection between HTN and CAD is well-established and unequivocal. Chronic hypertension induces hemodynamic alterations that activate blood platelets, thereby accelerating the formation of atherosclerotic plaques (46). These plaques can lead to myocardial ischemia, hypoxia, or necrosis, culminating in CAD. Research by P. Oikonomou highlighted that patients with preeclampsia exhibit a pathophysiological profile akin to those observed in cardiovascular conditions, predisposing them to an increased risk of CAD (47). This evidence lends theoretical support to our study's findings.
This study explored the association between ICP and CVD at the genetic level. Because of the strong gene-phenotype association, the results of this study are limited to a causal relationship between a single disease (ICP) and CVD and cannot be extrapolated to other types of cholestasis. However, a review of the relevant literature shows that multiple causes of chronic cholestasis (biliary obstruction, PBC, and other diseases) seem to be associated with an increased risk of CVD and that abnormal lipid levels are involved in an important role (48–50). This suggests that a variety of cholestasis, including ICP, may modify the risk of CVD, but the causal relationship between them requires further subsequent validation.
This study boasts several notable strengths. Primarily, it is the inaugural MR analysis investigating the causal link between ICP and CVD, marking a significant advancement in this research domain. Additionally, we conducted a thorough examination of potential mediators within the causal pathway from ICP to CVD, enhancing the depth and breadth of our analysis. Nonetheless, the study is not without its limitations. A primary constraint is the exclusive manifestation of ICP in one sex, coupled with the absence of large-scale, sex-specific GWAS, necessitating the use of sex-combined population data. However, the selected SNPs for MR analysis exhibited no significant sex-based genetic effect differences, thereby minimally influencing the outcomes. Another concern is the potential for sample overlap between the lipid profiles and liver function markers employed in the mediation analysis. Furthermore, the reliance on data predominantly from European populations constrains the generalizability of our conclusions across diverse ethnic groups. Finally, despite the rigorous quality control adopted in this study to minimize the influence of confounding factors on the results, it is possible that the results may still be affected to some extent due to the extremely strong correlation between lipids and CVD itself.
In conclusion, it is important for individuals diagnosed with ICP to be made aware of their elevated risk for future CVD and the importance of monitoring lipid profiles for early intervention. Furthermore, there is a pressing need for additional research to elucidate the precise mechanisms through which ICP influences CVD risk.
5 Conclusion
In summary, this study is the first comprehensive MR analysis to explore the causal link between ICP and CVD using genome-wide data. Bidirectional MR analyses revealed that genetically predicted ICP is causally linked to an increased risk of CVD, with no evidence supporting a causal effect of genetically predicted CVD on ICP risk. Furthermore, MR-mediated analyses have substantiated both a direct causal impact of ICP on CVD risk and a notable indirect effect mediated through lipid profiles, specifically LDL, TC, and Apo-B. These results underscore the critical mediating role of lipids in the causal pathway from ICP to CVD.
Data availability statement
The original contributions presented in the study are included in the article/Supplementary Material, further inquiries can be directed to the corresponding authors.
Ethics statement
Ethical approval was not required for the study involving humans in accordance with the local legislation and institutional requirements. Written informed consent to participate in this study was not required from the participants or the participants’ legal guardians/next of kin in accordance with the national legislation and the institutional requirements.
Author contributions
JC: Conceptualization, Data curation, Formal Analysis, Investigation, Methodology, Resources, Software, Validation, Visualization, Writing – original draft. QZ: Data curation, Formal Analysis, Resources, Software, Visualization, Writing – original draft. MC: Conceptualization, Supervision, Writing – review & editing. ZY: Funding acquisition, Project administration, Supervision, Writing – review & editing.
Funding
The author(s) declare that no financial support was received for the research, authorship, and/or publication of this article.
Acknowledgments
We want to acknowledge the participants and investigators of the MRC IEU at the University of Bristol for sharing the genetic data.
Conflict of interest
The authors declare that the research was conducted in the absence of any commercial or financial relationships that could be construed as a potential conflict of interest.
Publisher's note
All claims expressed in this article are solely those of the authors and do not necessarily represent those of their affiliated organizations, or those of the publisher, the editors and the reviewers. Any product that may be evaluated in this article, or claim that may be made by its manufacturer, is not guaranteed or endorsed by the publisher.
Supplementary material
The Supplementary Material for this article can be found online at: https://www.frontiersin.org/articles/10.3389/fcvm.2024.1401010/full#supplementary-material
References
1. Palmer KR, Xiaohua L, Mol BW. Management of intrahepatic cholestasis in pregnancy. Lancet. (2019) 393:853–4. doi: 10.1016/S0140-6736(18)32323-7
2. Saad AF, Pacheco LD, Chappell L, Saade GR. Intrahepatic cholestasis of pregnancy: toward improving perinatal outcome. Reprod Sci Thousand Oaks Calif. (2022) 29:3100–5. doi: 10.1007/s43032-021-00740-x
3. Hobson S, Gandhi S, Sobel M. Intrahepatic cholestasis of pregnancy. CMAJ Can Med Assoc J J Assoc Medicale Can. (2022) 194:E1650. doi: 10.1503/cmaj.220334
4. Williamson C, Geenes V. Intrahepatic cholestasis of pregnancy. Obstet Gynecol. (2014) 124:120–33. doi: 10.1097/AOG.0000000000000346
5. Smith DD, Rood KM. Intrahepatic cholestasis of pregnancy. Clin Obstet Gynecol. (2020) 63:134–51. doi: 10.1097/GRF.0000000000000495
6. Piechota J, Jelski W. Intrahepatic cholestasis in pregnancy: review of the literature. J Clin Med. (2020) 9:1361. doi: 10.3390/jcm9051361
7. Ovadia C, Seed PT, Sklavounos A, Geenes V, Di Ilio C, Chambers J, et al. Association of adverse perinatal outcomes of intrahepatic cholestasis of pregnancy with biochemical markers: results of aggregate and individual patient data meta-analyses. Lancet Lond Engl. (2019) 393:899–909. doi: 10.1016/S0140-6736(18)31877-4
8. Glantz A, Marschall H-U, Mattsson L-A. Intrahepatic cholestasis of pregnancy: relationships between bile acid levels and fetal complication rates. Hepatol Baltim Md. (2004) 40:467–74. doi: 10.1002/hep.20336
9. Ovadia C, Sajous J, Seed PT, Patel K, Williamson NJ, Attilakos G, et al. Ursodeoxycholic acid in intrahepatic cholestasis of pregnancy: a systematic review and individual participant data meta-analysis. Lancet Gastroenterol Hepatol. (2021) 6:547–58. doi: 10.1016/S2468-1253(21)00074-1
10. Gurung V, Middleton P, Milan SJ, Hague W, Thornton JG. Interventions for treating cholestasis in pregnancy. Cochrane Database Syst Rev. (2013) 2013(6):CD000493. doi: 10.1002/14651858.CD000493.pub22
11. Dixon PH, Williamson C. The pathophysiology of intrahepatic cholestasis of pregnancy. Clin Res Hepatol Gastroenterol. (2016) 40:141–53. doi: 10.1016/j.clinre.2015.12.008
12. Reyes H, Báez ME, González MC, Hernández I, Palma J, Ribalta J, et al. Selenium, zinc and copper plasma levels in intrahepatic cholestasis of pregnancy, in normal pregnancies and in healthy individuals, in Chile. J Hepatol. (2000) 32:542–9. doi: 10.1016/s0168-8278(00)80214-7
13. Williamson C, Hems LM, Goulis DG, Walker I, Chambers J, Donaldson O, et al. Clinical outcome in a series of cases of obstetric cholestasis identified via a patient support group. BJOG Int J Obstet Gynaecol. (2004) 111:676–81. doi: 10.1111/j.1471-0528.2004.00167.x
14. Xiao J, Li Z, Song Y, Sun Y, Shi H, Chen D, et al. Molecular pathogenesis of intrahepatic cholestasis of pregnancy. Can J Gastroenterol Hepatol. (2021) 2021:6679322. doi: 10.1155/2021/6679322
15. Goins EC, Wein LE, Watkins VY, Campbell AIK, Heine RP, Hughes BL, et al. Maternal and neonatal outcomes in patients with hepatitis C and intrahepatic cholestasis of pregnancy: the sum of the parts. PLoS One. (2023) 18:e0293030. doi: 10.1371/journal.pone.0293030
16. Terrault NA, Williamson C. Pregnancy-associated liver diseases. Gastroenterology. (2022) 163:97–117.e1. doi: 10.1053/j.gastro.2022.01.060
17. Liu C, Gao J, Liu J, Wang X, He J, Sun J, et al. Intrahepatic cholestasis of pregnancy is associated with an increased risk of gestational diabetes and preeclampsia. Ann Transl Med. (2020) 8:1574. doi: 10.21037/atm-20-4879
18. Wu P, Green M, Myers JE. Hypertensive disorders of pregnancy. Br Med J. (2023) 381:e071653. doi: 10.1136/bmj-2022-071653
19. Fan X, Zhou Q, Zeng S, Zhou J, Peng Q, Zhang M, et al. Impaired fetal myocardial deformation in intrahepatic cholestasis of pregnancy. J Ultrasound Med Off J Am Inst Ultrasound Med. (2014) 33:1171–7. doi: 10.7863/ultra.33.7.1171
20. Mor M, Shmueli A, Krispin E, Bardin R, Sneh-Arbib O, Braun M, et al. Intrahepatic cholestasis of pregnancy as a risk factor for preeclampsia. Arch Gynecol Obstet. (2020) 301:655–64. doi: 10.1007/s00404-020-05456-y
21. Larsson SC, Butterworth AS, Burgess S. Mendelian randomization for cardiovascular diseases: principles and applications. Eur Heart J. (2023) 44:4913–24. doi: 10.1093/eurheartj/ehad736
22. Skrivankova VW, Richmond RC, Woolf BAR, Yarmolinsky J, Davies NM, Swanson SA, et al. Strengthening the reporting of observational studies in epidemiology using Mendelian randomization: the STROBE-MR statement. JAMA. (2021) 326:1614–21. doi: 10.1001/jama.2021.18236
23. Dixon PH, Levine AP, Cebola I, Chan MMY, Amin AS, Aich A, et al. GWAS meta-analysis of intrahepatic cholestasis of pregnancy implicates multiple hepatic genes and regulatory elements. Nat Commun. (2022) 13:4840. doi: 10.1038/s41467-022-29931-z
25. Kurki MI, Karjalainen J, Palta P, Sipilä TP, Kristiansson K, Donner KM, et al. Finngen provides genetic insights from a well-phenotyped isolated population. Nature. (2023) 613:508–18. doi: 10.1038/s41586-022-05473-8
26. Kintu C, Soremekun O, Kamiza AB, Kalungi A, Mayanja R, Kalyesubula R, et al. The causal effects of lipid traits on kidney function in Africans: bidirectional and multivariable Mendelian-randomization study. EBioMedicine. (2023) 90:104537. doi: 10.1016/j.ebiom.2023.104537
27. Burgess S, Thompson SG. CRP CHD genetics collaboration. Avoiding bias from weak instruments in Mendelian randomization studies. Int J Epidemiol. (2011) 40:755–64. doi: 10.1093/ije/dyr036
28. Hartwig FP, Davey Smith G, Bowden J. Robust inference in summary data Mendelian randomization via the zero modal pleiotropy assumption. Int J Epidemiol. (2017) 46:1985–98. doi: 10.1093/ije/dyx102
29. Chen X, Kong J, Pan J, Huang K, Zhou W, Diao X, et al. Kidney damage causally affects the brain cortical structure: a Mendelian randomization study. EBioMedicine. (2021) 72:103592. doi: 10.1016/j.ebiom.2021.103592
30. Ong J-S, MacGregor S. Implementing MR-PRESSO and GCTA-GSMR for pleiotropy assessment in Mendelian randomization studies from a practitioner’s perspective. Genet Epidemiol. (2019) 43:609–16. doi: 10.1002/gepi.22207
31. Sanderson E. Multivariable Mendelian randomization and mediation. Cold Spring Harb Perspect Med. (2021) 11:a038984. doi: 10.1101/cshperspect.a038984
32. Wikström Shemer EA, Stephansson O, Thuresson M, Thorsell M, Ludvigsson JF, Marschall H-U. Intrahepatic cholestasis of pregnancy and cancer, immune-mediated and cardiovascular diseases: a population-based cohort study. J Hepatol. (2015) 63:456–61. doi: 10.1016/j.jhep.2015.03.010
33. Hämäläinen S-T, Turunen K, Mattila KJ, Sumanen M. Intrahepatic cholestasis of pregnancy and associated causes of death: a cohort study with follow-up of 27–46 years. BMC Womens Health. (2018) 18:1–5. doi: 10.1186/s12905-018-0606-0
34. Erlinger S. Intrahepatic cholestasis of pregnancy: a risk factor for cancer, autoimmune and cardiovascular diseases? Clin Res Hepatol Gastroenterol. (2016) 40:139–40. doi: 10.1016/j.clinre.2015.09.003
35. Chen Y, Zhang H, Ning W, Chen Y, Wen C. The impact of intrahepatic cholestasis on pregnancy outcomes: a retrospective cohort study. BMC Gastroenterol. (2023) 23:16. doi: 10.1186/s12876-023-02652-3
36. Zhang Y, Lan X, Cai C, Li R, Gao Y, Yang L, et al. Associations between maternal lipid profiles and pregnancy complications: a prospective population-based study. Am J Perinatol. (2021) 38:834–40. doi: 10.1055/s-0039-3402724
37. Hartley A, Haskard D, Khamis R. Oxidized LDL and anti-oxidized LDL antibodies in atherosclerosis—novel insights and future directions in diagnosis and therapy. Trends Cardiovasc Med. (2019) 29:22–6. doi: 10.1016/j.tcm.2018.05.010
38. Winklhofer-Roob BM, Faustmann G, Roob JM. Low-density lipoprotein oxidation biomarkers in human health and disease and effects of bioactive compounds. Free Radic Biol Med. (2017) 111:38–86. doi: 10.1016/j.freeradbiomed.2017.04.345
39. Packard CJ, Demant T, Stewart JP, Bedford D, Caslake MJ, Schwertfeger G, et al. Apolipoprotein B metabolism and the distribution of VLDL and LDL subfractions. J Lipid Res. (2000) 41:305–18. doi: 10.1016/S0022-2275(20)32065-4
40. Ma K, Saha PK, Chan L, Moore DD. Farnesoid X receptor is essential for normal glucose homeostasis. J Clin Invest. (2006) 116:1102–9. doi: 10.1172/JCI25604
41. Chiang JYL, Ferrell JM. Bile acid receptors FXR and TGR5 signaling in fatty liver diseases and therapy. Am J Physiol Gastrointest Liver Physiol. (2020) 318:G554–73. doi: 10.1152/ajpgi.00223.2019
42. Keitel V, Dröge C, Häussinger D. Targeting FXR in cholestasis. Handb Exp Pharmacol. (2019) 256:299–324. doi: 10.1007/164_2019_231
43. Abu-Hayyeh S, Papacleovoulou G, Lövgren-Sandblom A, Tahir M, Oduwole O, Jamaludin NA, et al. Intrahepatic cholestasis of pregnancy levels of sulfated progesterone metabolites inhibit farnesoid X receptor resulting in a cholestatic phenotype. Hepatol Baltim Md. (2013) 57:716–26. doi: 10.1002/hep.26055
44. Menżyk T, Bator M, Derra A, Kierach R, Kukla M. The role of metabolic disorders in the pathogenesis of intrahepatic cholestasis of pregnancy. Clin Exp Hepatol. (2018) 4:217–23. doi: 10.5114/ceh.2018.80122
45. Schoeler M, Caesar R. Dietary lipids, gut microbiota and lipid metabolism. Rev Endocr Metab Disord. (2019) 20:461–72. doi: 10.1007/s11154-019-09512-0
46. Metoki H, Iwama N, Hamada H, Satoh M, Murakami T, Ishikuro M, et al. Hypertensive disorders of pregnancy: definition, management, and out-of-office blood pressure measurement. Hypertens Res Off J Jpn Soc Hypertens. (2022) 45:1298–309. doi: 10.1038/s41440-022-00965-6
47. Oikonomou P, Tsonis O, Paxinos A, Gkrozou F, Korantzopoulos P, Paschopoulos M. Preeclampsia and long-term coronary artery disease: how to minimize the odds? Eur J Obstet Gynecol Reprod Biol. (2020) 255:253–8. doi: 10.1016/j.ejogrb.2020.10.061
48. Black DD. Chronic cholestasis and dyslipidemia: what is the cardiovascular risk? J Pediatr. (2005) 146:306–7. doi: 10.1016/j.jpeds.2004.11.027
49. Longo M, Crosignani A, Battezzati PM, Giussani CS, Invernizzi P, Zuin M, et al. Hyperlipidaemic state and cardiovascular risk in primary biliary cirrhosis. Gut. (2002) 51:265–9. doi: 10.1136/gut.51.2.265
Keywords: intrahepatic cholestasis of pregnancy, lipid, cardiovascular disease, Mendelian randomization, GWAS
Citation: Cui J, Zhai Q, Chen M and Yang Z (2024) Genetically predicted lipids mediate the association between intrahepatic cholestasis of pregnancy and cardiovascular disease. Front. Cardiovasc. Med. 11: 1401010. doi: 10.3389/fcvm.2024.1401010
Received: 20 March 2024; Accepted: 18 April 2024;
Published: 30 April 2024.
Edited by:
Chieko Mineo, University of Texas Southwestern Medical Center, United StatesReviewed by:
Shuang Zhang, Hefei University of Technology, ChinaNa Wu, China Medical University, China
© 2024 Cui, Zhai, Chen and Yang. This is an open-access article distributed under the terms of the Creative Commons Attribution License (CC BY). The use, distribution or reproduction in other forums is permitted, provided the original author(s) and the copyright owner(s) are credited and that the original publication in this journal is cited, in accordance with accepted academic practice. No use, distribution or reproduction is permitted which does not comply with these terms.
*Correspondence: Mengjie Chen, chenmengjie@hospital.cqmu.edu.cn; Zhu Yang, yangzhu@hospital.cqmu.edu.cn
†These authors have contributed equally to this work and share first authorship
‡ORCID:
Mengjie Chen
orcid.org/0009-0008-0215-4501
Zhu Yang
orcid.org/0000-0002-0610-499X