- 1Unitat D'Arritmies, Servei de Cardiologia, Hospital Universitari Vall D'Hebron, Vall d’Hebron Institut de Recerca (VHIR), Vall d’Hebron Barcelona Hospital Campus, Barcelona, Spain
- 2Departament de Medicina, Universitat Autònoma de Barcelona, Bellaterra, Spain
- 3CIBER de Enfermedades Cardiovasculares (CIBER-CV), Instituto de Salud Carlos III, Madrid, Spain
The prevalence of congenital heart disease (CHD) in adult patients has risen with advances in diagnostic and surgical techniques. Surgical modifications and hemodynamic changes increase the susceptibility to arrhythmias, impacting morbidity and mortality rates, with arrhythmias being the leading cause of hospitalizations and sudden deaths. Patients with CHD commonly experience both supraventricular and ventricular arrhythmias, with each CHD type associated with different arrhythmia patterns. Macroreentrant atrial tachycardias, particularly cavotricuspid isthmus-dependent flutter, are frequently reported. Ventricular arrhythmias, including monomorphic ventricular tachycardia, are prevalent, especially in patients with surgical scars. Pharmacological therapy involves antiarrhythmic and anticoagulant drugs, though data are limited with potential adverse effects. Catheter ablation is preferred, demanding meticulous procedural planning due to anatomical complexity and vascular access challenges. Combining imaging techniques with electroanatomic navigation enhances outcomes. However, risk stratification for sudden death remains challenging due to anatomical variability. This article practically reviews the most common tachyarrhythmias, treatment options, and clinical management strategies for these patients.
Introduction
The number of adult patients with congenital heart disease (CHD) has significantly increased in recent years, primarily due to technological advances in diagnostic and surgical techniques (1). A considerable proportion of these patients are prone to developing arrhythmias because of surgical modifications, such as suture lines and prosthetic materials like patches, as well as hemodynamic alterations arising from long-term pressure or volume overload, atrial and ventricular remodeling, and, in some cases, cyanosis. Up to 50% of patients with CHD may experience supraventricular arrhythmias (SVAs), and in certain CHDs, such as Tetralogy of Fallot (ToF), ventricular arrhythmias may occur in up to 14%, carrying a non-negligible risk of SD (2).
Arrhythmias in patients with CHD have been associated with increased morbidity and mortality, exerting a negative impact on quality of life (3). Additionally, according to the Dutch Concor registry, arrhythmias constitute the most frequent cause of hospital admission in patients with CHD, accounting for up to 31% of cases (4). For instance, in a Canadian study involving over 38,000 patients with CHD, the presence of atrial arrhythmias was associated with a 50% increased risk of mortality and a 50% increase in morbidity in the form of stroke or heart failure (5). This worse prognosis may be more pronounced when considering populations with more complex cardiac conditions. For example, in palliative transposition of the great arteries (TGA) with atrial switch surgery, atrial arrhythmias increase the risk of mortality fivefold. Similarly, in patients undergoing Fontan surgeries, the risk increases sixfold (6). In all CHDs, the most common cause of sudden death (SD) is arrhythmic (7). Arrhythmias represent an increase of up to more than three times the risk of SD in the general population with CHD (8), with two potential mechanisms: complete atrioventricular block and ventricular arrhythmias.
In this article, the most common tachyarrhythmias, treatment options, and clinical management strategies for these patients are discussed. The information provided is mostly based on a narrative review of the literature, incorporating the authors' own experience in the subject as well.
Arrhythmia substrates in congenital heart disease
Patients with CHD can be affected by various types of arrhythmias, including intranodal reentry tachycardias, accessory pathway tachycardias, focal atrial tachycardia, macroreentrant atrial tachycardias (MRAT), atrial fibrillation (AF), ventricular tachycardia (VT), and ventricular fibrillation. MRAT are the most common arrhythmias in patients with CHD. Cavotricuspid isthmus-dependent flutter (CTI), similar to the general population, remains the most frequently reported, accounting for up to 40%–60% of these arrhythmias (2, 9).
The occurrence of each type of arrhythmia is determined by the timing and type of repair, the scars generated, and the subsequent remodeling. Therefore, each form of CHD is associated with different types of arrhythmia (1, 10) (Table 1). The Fontan procedure and physiological correction of TGA (Senning and Mustard), which involve creating suture lines and altering hemodynamics, render these patients the most predisposed to developing SVAs. This risk can reach up to 50% for patients with the classic Fontan procedure at 10 years post-surgery (11). Furthermore, these patients often have poorer hemodynamic tolerance due to limited distensibility of neo-atria, dysfunction of the single ventricle or systemic ventricle, and volume or pressure overload resulting from valve abnormalities or residual shunts.
In other CHD, pre-existing arrhythmias may be observed prior to surgical intervention. This is evident in cases such as atrioventricular connections in patients with Ebstein's anomaly and congenitally corrected transposition of the great arteries (ccTGA).
Management of arrhythmias in congenital heart disease
Arrhythmias diagnosis
The diagnosis of symptomatic arrhythmias in patients with CHD is conducted similarly to the general population. Diagnostic tools such as cumulative Holter monitoring, 2-week continuous monitors, single-lead ECG devices integrated into smartwatches and smartphones, and implantable loop recorders (ILRs) are available (12–20).
In patients presenting with syncope, the ILR and electrophysiological study play an important role. In patients with a high clinical risk profile, especially those with myocardial scar or conduction disturbances, EPS not only yields a substantial number of diagnoses but also identifies patients at lower risk of arrhythmic syncope (21–29). However, its negative predictive value has been considered to be suboptimal (around 70%) (24, 30), and in such cases, further work up might be needed. The implantation of an ILR enables prolonged cardiac monitoring, offering the possibility to correlate arrhythmic events with symptoms (17, 21, 22, 31–33). This allows for additional diagnostic yield and is considered safe (17, 21, 22, 31–33).
Screening asymptomatic patients with periodic assessments beyond 12-lead ECGs or periodic Holter monitoring is of uncertain value, as a high prevalence of asymptomatic findings has been reported that seldom impact management (12, 34). Further studies are necessary to determine whether early event detection and intervention can reduce morbidity and mortality in this patient cohort.
Hemodynamic assessment and treatment
Similarly to the general population, the initial step in the treatment of arrhythmias depends on their hemodynamic tolerance. SVAs may be poorly tolerated and can manifest as heart failure, shock, syncope or even electromechanical dissociation in extreme cases (2, 5, 21, 35–36). In the case of MRAT, the presence of patches and surgical scars leads to a slowing of conduction velocity, resulting in slower atrial cycles during tachycardia. This favors faster atrioventricular conduction, leading to hemodynamic compromise and even myocardial stunning. Patients with highly complex CHDs, such as TGA, those with dysfunction of the systemic ventricle, or severe dilation of the venous atrium, are at a higher risk of experiencing severe clinical manifestations during tachycardia episodes (37). If the patient exhibits hemodynamic compromise, urgent cardioversion should be considered. Anteroposterior pad placement is usually suitable for most patients and provides the highest rate of success (2). It should be adapted to the cardiac position (e.g., in patients with dextrocardia). In the case of SVAs, some patients, such as those with TGA, may experience sinus arrest or severe bradycardia after cardioversion, often requiring the administration of atropine/isoproterenol or external cardiac stimulation.
If patients with ASDs demonstrate good tolerance and a duration >48 h, the presence of cardiac thrombus should be ruled out through transesophageal echocardiography or appropriate anticoagulation (for more than 3 weeks), and medications for heart rate control (beta-blocker or calcium antagonist, depending on the characteristics of the heart disease) should be considered before cardioversion (1). In the case of ventricular tachycardias with good hemodynamic tolerance, the administration of intravenous drugs (amiodarone or procainamide) may be considered before considering electrical cardioversion (ECV).
In addition, reversible causes such as hyperthyroidism, an inflammatory process, or anemia need to be ruled out. In patients with CHD, the presence of hemodynamic alterations should be assessed, as they may influence the onset of arrhythmias and warrant consideration of targeted treatment. For example, in patients having undergone classic Fontan surgery, associated with a high prevalence of MRAT; in some cases, a conversion to Fontan surgeries with intra or extracardiac tunnel may be considered. Other examples include possible intracardiac shunts, progressive valvular abnormalities, etc.
For a comprehensive assessment of all these factors, it is essential to promptly refer the patient to a specialized center with a multidisciplinary team experienced in the treatment of arrhythmias in patients with CHD (Figure 1).
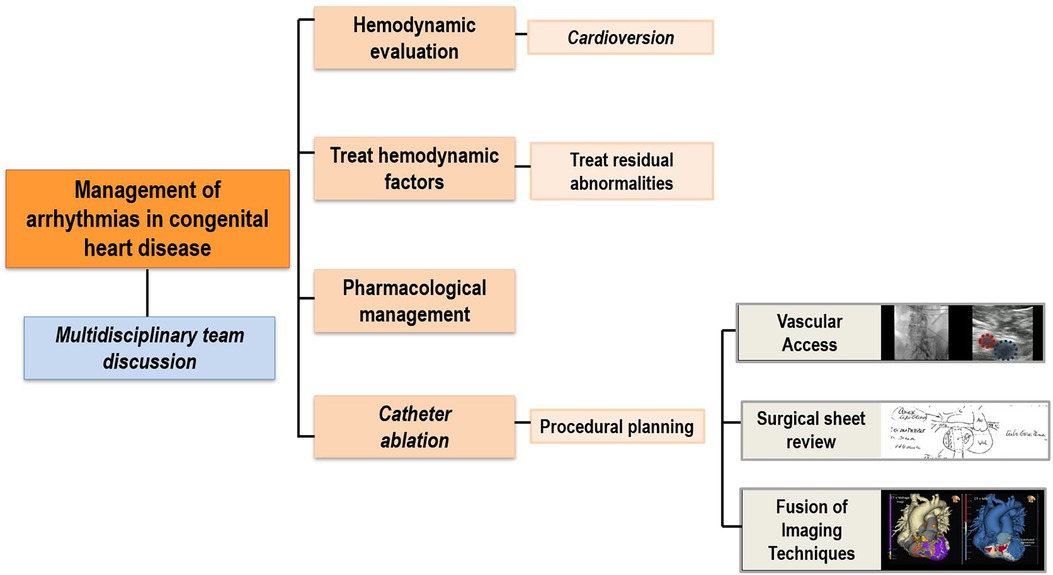
Figure 1. General arrhythmia workflow. Diagram outlining the description of the main steps in the evaluation and treatment of arrhythmias in patients with congenital heart diseases.
Pharmacological management
Anticoagulant therapy
Regarding anticoagulant drugs, there is no clear consensus on indications, and specific risk stratification is needed. Thromboembolic cerebrovascular complications are a fundamental cause of morbidity in the CHD population. According to the TACTIC study, thromboembolic events are more associated with the complexity of the heart disease than with risk scales used in the general population. However, the CHA2DS2-VASc score may be applicable in simple CHD (38). Anatomical groups with a higher known embolic risk include uncorrected cyanotic heart diseases, Eisenmenger physiology, uncorrected atrial septal defects, and Fontan circulation (2).
Antiarrhythmic therapy
There is a lack of data due to the heterogeneity and limited number of patients with CHD included in the studies. Rhythm control is the preferred approach in this population, as the loss of sinus rhythm is frequently poorly tolerated, especially in patients with complex cardiac conditions. Similarly to the general population, antiarrhythmic drugs have low efficacy and significant adverse effects, both extracardiac and cardiac, due to negative inotropic and/or dromotropic effects.
In the acute treatment of arrhythmias, adenosine is the drug of choice for tachycardias involving the AV node and serves as a differential diagnostic method. Special care must be taken in patients with a low ejection fraction whose cardiac output depends on heart rate. It is noteworthy that in patients with Fontan physiology, adenosine may be ineffective due to its rapid metabolism through enzymatic degradation in blood and peripheral tissues, without manifesting its cardiac effects (2).
In terms of chronic treatment, class I antiarrhythmic drugs are recommended for patients without significant ventricular dysfunction, hypertrophy, or atrial scars. However, they are not recommended for treating MRAT, as their effect on slowing intra-atrial conduction may facilitate faster ventricular responses.
Class III antiarrhythmic drugs, specifically amiodarone, are the most commonly used in these patients, particularly in those with moderate and severe CHD. Amiodarone is highly effective for maintaining sinus rhythm and treating chronic ventricular arrhythmias; however, it is associated with many long-term side effects. Up to 56% of significant side effects were reported in a cohort of patients with moderate and complex CHD during a median follow-up of 2.7 years, with 30% experiencing amiodarone-induced thyrotoxicosis, more frequently observed in Fontan physiology patients. These adverse effects led to discontinuation of the drug in 42% of cases, despite its effectiveness in those who tolerated it, with an overall or partial efficacy rate of 98% (39). Caution is advised in cyanotic patients or those with pre-existing thyroid, pulmonary, or hepatic dysfunction.
Sotalol is preferred in young patients due to its fewer side effects. As has been reported in a case series with moderate and complex CHD, sotalol has proven to be reasonably well-tolerated (only 18% discontinued treatment primarily due to fatigue or dyspnea) and safe (no arrhythmias or sudden deaths were documented, and only 13% of patients experienced significant bradycardia, mainly those with Fontan surgery). Sotalol was completely or partially effective in 94% of patients (40).
There is limited experience with dronedarone in this population, but it may be another therapeutic option that has the advantage of avoiding the thyroid effects associated with amiodarone. Liver function should also be monitored, and its use is contraindicated in heart failure (41).
Finally, beta-blockers are recommended for both heart rate control in SVAs and non-sustained ventricular arrhythmias. In acute management, they should be administered carefully to patients with ventricular dysfunction due to their negative inotropic effect. Calcium channel blockers and digoxin may also be useful for rate control.
Catheter ablation
Due to the limitations of antiarrhythmic drugs, catheter ablation (CA) has become the first-line treatment for arrhythmias in CHD (2, 5, 42–46). This procedure represents a challenge, given the anatomical complexity and the frequent difficulty in vascular access. Meticulous procedure planning is crucial, considering the patient's anatomical characteristics and surgical techniques (2, 24, 45). Correct identification of the specific conduction system in relation to individual anatomy is essential in these patients. This is particularly important in the ablation of some arrhythmias, such as intranodal reentrant tachycardias, or in VT in patients with rToF with surgical closure of VSD, in whom the ablation site may be more challenging to locate due to variability in reference structures, increasing the risk of complete atrioventricular (AV) block.
For all these reasons, patients should be referred to centers with experience in arrhythmia ablation in CHD, especially in the case of complex CHD (47). Careful pre-procedure planning is always important.
Vascular access
In the planning of ablation procedures for patients with CHD, it is necessary to anticipate potential challenges with conventional femoral accesses. It is not uncommon for these patients to experience femoral vein occlusion, inferior vena cava atresia, or surgical obstacles such as prostheses or patches (Figure 2). To overcome these challenges, alternative vascular access, including internal jugular vein, subclavian vein, transapical, or transhepatic access may be necessary (48). In certain cases, a transesophageal catheter is employed, particularly in pediatric cases with difficulties in vascular access, to obtain intracardiac electrograms (49). Performing complex accesses, such as transeptal or transpatch procedures, requires significant electrophysiology expertise, especially in patients with corrected TGA using Senning or Mustard surgery or those with Fontan physiology involving an intracardiac tube. Utilizing intraprocedural imaging techniques like intravascular ultrasound or the fusion of previous imaging tests and electroanatomic mapping (EAM) enhances procedural guidance (50). Pre-procedural imaging tests, such as computed tomography (CT), play a crucial role in assessing vascular accesses beforehand and serving as an intraprocedural reference.
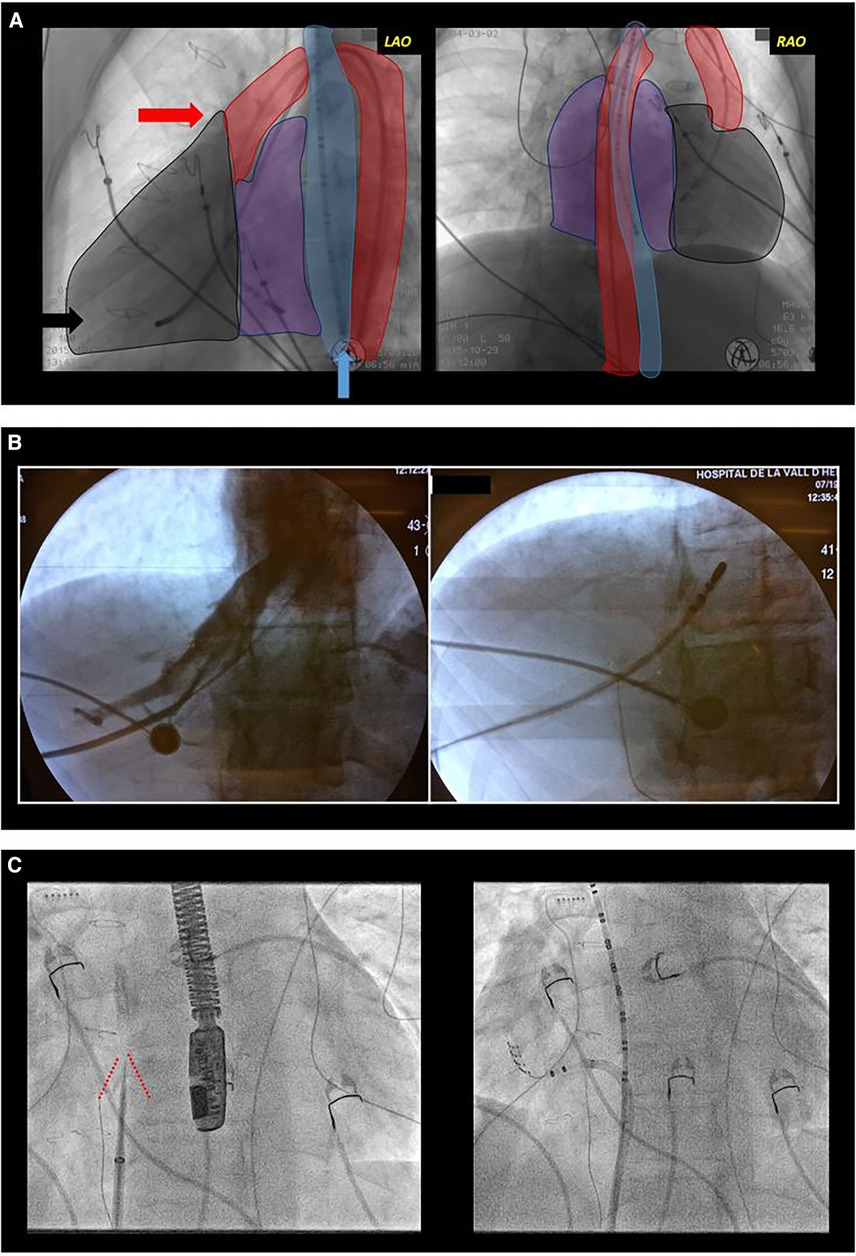
Figure 2. Difficult access. (A) Complex congenital heart disease with a single ventricle type, mesocardia, and dextroapex, initially repaired with classic Fontan surgery which was later converted to Fontan with intracardiac tube. Electrophysiological study was performed due to syncopal supraventricular tachycardia. Conventional radiological projections are shown: left anterior oblique (LAO, left image) and right anterior oblique (RAO, right image). The outlines of the esophagus (blue), aorta (red), ventricle (black), and atria (purple) are shaded. Due to the absence of femoral venous access, a transesophageal diagnostic catheter (blue arrow) was introduced for atrial sensing, and through the femoral artery, a tetrapolar catheter to the ventricle (black arrow) for rescue ventricular stimulation and a mapping-ablation catheter for treatment (red arrow) were progressed. (B) Transhepatic access guided by fluoroscopy. In the first fluoroscopy image, contrast injection into the hepatic venous system can be observed. In the second image, the catheter is progressed through this venous system. (C) Transeptal access in a patient with transposition of the great arteries corrected with atrial switch surgery (Senning). Both fluoroscopy images show an left anterior oblique projection. In the left image, a transeptal puncture needle oriented anteriorly and towards the right shoulder. Tenting of the interatrial baffle is observed (red lines). In the right image, a mapping-ablation catheter has been advanced through a long sheath to the neo-pulmonary venous atria.
Anatomical knowledge
Accurate knowledge of the patient's anatomy is crucial, requiring a comprehensive examination of surgical records for those who have undergone cardiac repair. Evaluation of ventricular function and identification of residual hemodynamic anomalies, such as leaks or valve issues, is essential. These findings have implications for arrhythmias and may influence the planning of ablation procedures. The presence of significant hemodynamic abnormalities can significantly influence the occurrence of arrhythmias, prompting consideration for repair if necessary. Some of these alterations may impact ablation procedures, either by facilitating access to cardiac chambers (for example, in cases of residual communications between neo-atria in patients having undergone Senning or Mustard surgeries) or by acting as an anatomical barrier to accessing specific regions (for example, in cases of percutaneous pulmonary valve prostheses in ToF or mechanical prostheses in any location) (Figure 3).
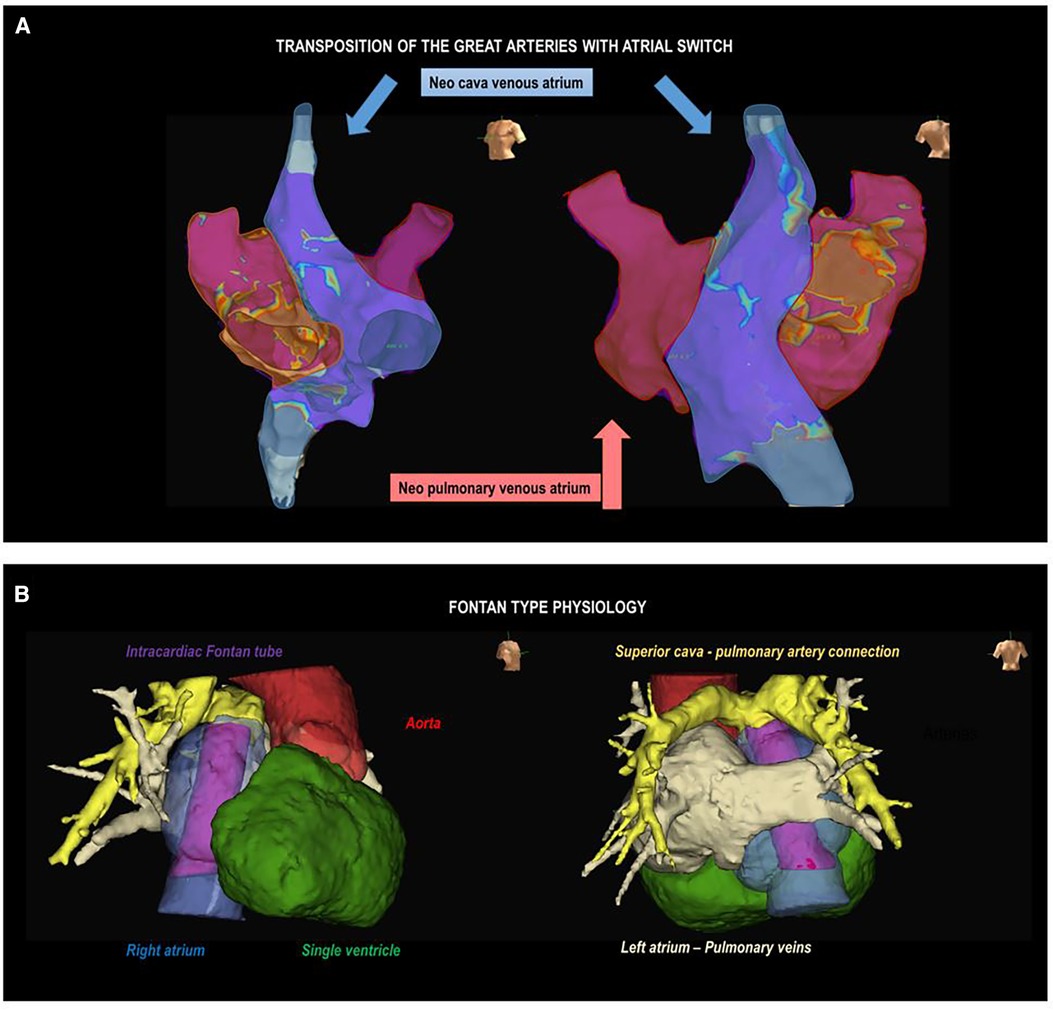
Figure 3. Complex anatomies. (A) Electroanatomic voltage map of the atrium in a patient with a transposition of the great arteries corrected with atrial switch surgery (Senning). Left oblique (left) and right posterolateral (right) projections are observed. The shaded areas represent the neopulmonary vein atrium (red) and the neocaval vein atrium (blue). (B) Anatomical reconstruction using image post-processing software in a patient with Fontan surgery, mesocardia, and dextroapex. A right lateral projection (on the left) shows the intracardiac tube (purple), right atrium (blue), aorta (red), and single ventricle (green); and a posteroanterior projection shows the superior vena cava-pulmonary artery connection (yellow) and left atrium-pulmonary veins (beige).
Conventional imaging methods such as echocardiograms may be insufficient, requiring right and left heart catheterization and cardiac magnetic resonance imaging (MRI) for detailed assessments, especially in complex cases like univentricular hearts or tetralogy of Fallot. The increasing use of three-dimensional models in CHD patients enhances understanding and aids in planning interventions and educating medical professionals, patients, and families (51).
Fusion of imaging techniques
Multimodality imaging techniques, particularly cardiac MRI and CT, are increasingly crucial for diagnosing and monitoring CHD patients. These techniques not only aid in pre-ablation planning but also serve as intraprocedural guidance by fusing with EAM. Fusion has proven beneficial in complex heart conditions, such as VT and left-sided flutter ablation, enhancing outcomes (52–54). In patients with ToF, the correlation of cardiac MRI findings with EAM has also been demonstrated (55). Substrate characterization often involves image post-processing software (e.g., ADAS Galgo Medical), enhancing integration for comprehensive planning and guidance during procedures.
Ablation techniques
Because of the anatomical difficulties and the high prevalence of complex arrhythmias in CHD patients, systematic planning of these procedures using electroanatomic navigation systems is recommended (2, 42, 44, 56). Continuous technological advancements in navigation systems allow for better anatomical and substrate characterization, such as identifying surgical scars and areas of fibrosis. This aids in the identification of reentries and the planning of ablation lines (Figure 4). High-density mapping catheters provide precise mapping, even in challenging accesses like transhepatic or trans-baffle, contributing to the prediction of ablation success (57, 58).
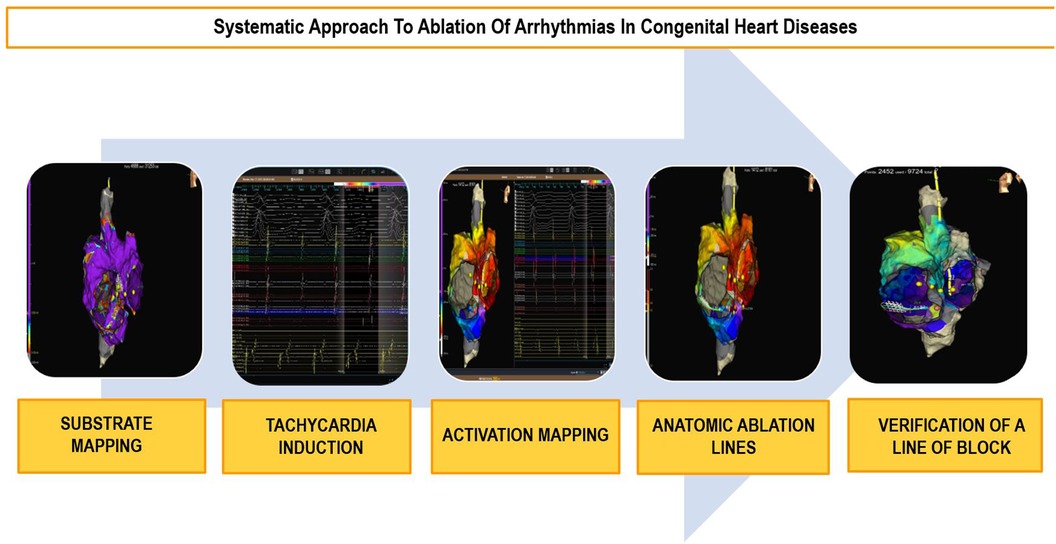
Figure 4. Ablation workflow in congenital heart diseases. Diagram outlining the systematic approach to ablation in congenital heart diseases in five steps.
Electroanatomic navigation systems offer the advantage of reducing radiation exposure. Fusion systems, combining fluoroscopy with EAM (Carto, Univu, Biosense Webster), intravascular echocardiography with EAM (Cartosound, Biosense Webster), and transesophageal echocardiography with fluoroscopy, reduce radiation exposure and enhance mapping efficiency (59). These advances are also very useful in those patients that require a transseptal or trans-baffle approach. Image techniques are often necessary to ensure the correct localization of the needle tip before puncture and to avoid potential complications, especially in those patients with more distorted anatomies. In addition to puncture, trans-baffle access can be challenging due to tissue rigidity, which may sometimes require the use of steerable sheaths or ones with a smoother profile, or even dilation of the same.
Beyond access difficulties, the anomalous location of the specific conduction system poses a challenge in CHD patients. Cryoablation is frequently used in the ablation of arrhythmias near the AV node, offering reversible lesions and greater stability due to catheter adhesion to the endocardial surface. Its safety and moderate effectiveness were demonstrated in a series of complex CHD cases (60). For ablations in other locations, irrigated-tip catheters with steerable sheaths that facilitate the introduction of catheters into areas with complex access are recommended. Intracardiac echocardiography is acquiring an increasing role in this type of ablations. Among other benefits, it allows live visualization of the real anatomy, planning of ablation lines, and also ensures optimal tissue contact.
Remote magnetic navigation is a technological solution adaptable to CHD patients, enabling access to unconventional areas and reducing the risk of perforation. Its main limitations are its cost and availability. Its utility has been demonstrated, for example, for retroaortic access to the atrium of the pulmonary veins in cases of TGA with Senning or Mustard surgery. Remote magnetic navigation has proven to be effective and safe in this context (61).
Specific arrhythmia types
Atrial tachycardia
Atrial tachycardia (AT), predominantly MRAT, is the most frequently documented arrhythmia in patients with CHD. For example, in recent studies (2, 42, 62), incidences of 30% have been described in patients with Ebstein's anomaly and TGA treated with atrial switch, 20% in patients with atrial septal defects and in patients with repaired TOF, or even up to half of patients undergoing Fontan surgeries.
The natural history of AT varies significantly depending on the type of CHD. In patients with complex heart diseases, AT tends to occur at an earlier age and with a higher arrhythmic burden (62). Thus, in patients with univentricular physiology and in TGA, the onset of arrhythmias usually occurs around the second decade of life, whereas in patients with atrial septal defects, it is delayed until the fifth decade (62).
The presence of surgical scars, prosthetic material, anatomic obstacles, and other areas of fibrosis demarcates tissue with slowed conduction, a crucial factor for the onset of MRAT. In these areas of anomalous tissue, there is the possibility of unidirectional block, serving as the necessary substrate for the occurrence of macroreentrant arrhythmias. Due to slowed intra-atrial conduction, MRAT involving the CTI is much more frequent than in the general population and, in fact, constitutes the most frequently encountered macroreentrant circuit in this population (2, 63, 64). However, other circuits that do not involve the CTI are also common, representing, according to some case series, more than 50% or even 70% of cases in certain substrates (65). Likewise, it is not uncommon for the same patient to have both types of tachycardia (CTI-dependent and non-CTI-dependent). In a study of 94 patients, 51% presented CTI-dependent MRAT, 21% CTI-independent MRAT, and 28% both mechanisms (63). The complexity of CHD was a strong predictor of CTI-independent MRAT. The electrocardiogram (ECG) and cardiac monitoring registers in patients with complex CHD can be challenging to interpret (2, 14, 66). On the one hand, about a third of ECGs with patterns not suggestive of CTI-dependent flutter may, in fact, be CTI-dependent (63). On the other, some patients may have very low-amplitude atrial waves due to extensive atrial fibrosis, which can even make the arrhythmia go unnoticed (67, 68) (Figure 5).
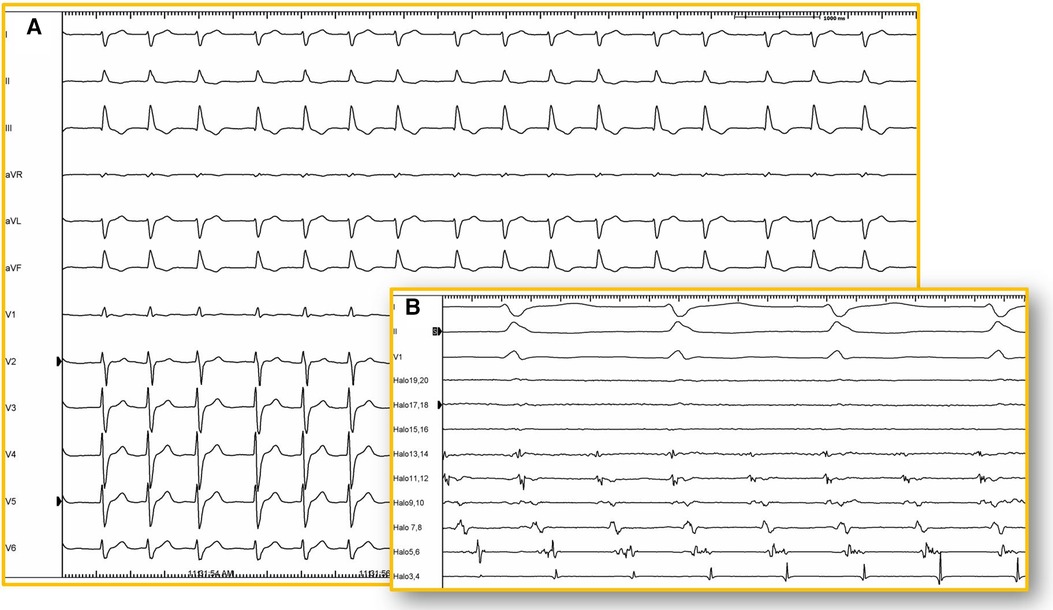
Figure 5. 12-lead ECG. (A) Twelve-lead electrocardiogram (25 mm/s) of a patient with corrected d-TGA using the Senning technique. Atrial activity is very difficult to visualize due to its low amplitude and may be interpreted as atrial fibrillation. However, in the intracavitary recording (B) of the same patient, atrial activity is shown recorded by a duodecapolar catheter positioned between the superior and inferior vena cava (100 mm/s), revealing organized and regular activity. Electroanatomical mapping confirmed the presence of typical atrial flutter with a circuit around the tricuspid annulus (isthmus-dependent).
Less frequently, ATs may also manifest a focal pattern (5%–10% of cases) (2, 68, 69). Electrocardiographically, they are often indistinguishable from MRAT and the diagnosis is typically established during mapping in the electrophysiology study (EPS).
Antiarrhythmic therapy
There are currently no randomized clinical trials available, nor are any expected, to assess the efficacy of antiarrhythmic treatments in this population. Therefore, the evidence on pharmacological management is based on a few case series and expert opinions. Both antiarrhythmic drugs and rate-controlling agents may play a role; however, rhythm control is the preferred approach.
Amiodarone is likely the most commonly used antiarrhythmic drug, both acutely to restore sinus rhythm and chronically in this context. Ibutilide, dofetilide and sotalol may also be useful for restoring sinus rhythm. To achieve rate control, beta-blockers, calcium channel blockers, and digoxin may be useful with some considerations. Digoxin is often less effective for heart rate control during exercise in young patients, especially in cases of MRAT.
Catheter ablation
Catheter ablation is the first-line treatment for MRAT. While acute efficacy is high at around 80%, short-term recurrence is significant (30%–50%), due to the substantial atrial myopathy present in these patients (2, 46, 64). In a series of 130 patients with CHD treated at our center, with a high percentage of complex heart disease, during a mean follow-up of 4 years, 23% experienced a recurrence of the same arrhythmia, 14% developed another left MRAT, and 8% developed AF. The efficacy of a second procedure was high, with 78% maintaining long-term sinus rhythm (46). The presence of non-ICT-dependent MRAT and the induction or prior history of AF was associated with a higher risk of recurrences.
Meticulous procedure planning is essential, considering patient anatomical characteristics and surgical techniques. During the ablation, special attention must be paid to the location of the phrenic nerve and the conduction tissue, which may be anatomically displaced. It is highly useful to have a multipolar catheter around the AV ring in the venous atrium (e.g., a duodecapolar catheter) and in the coronary sinus. Not only does this allow for the evaluation of activation patterns, but it also aids in diagnosing changes in MRAT (Figure 6).
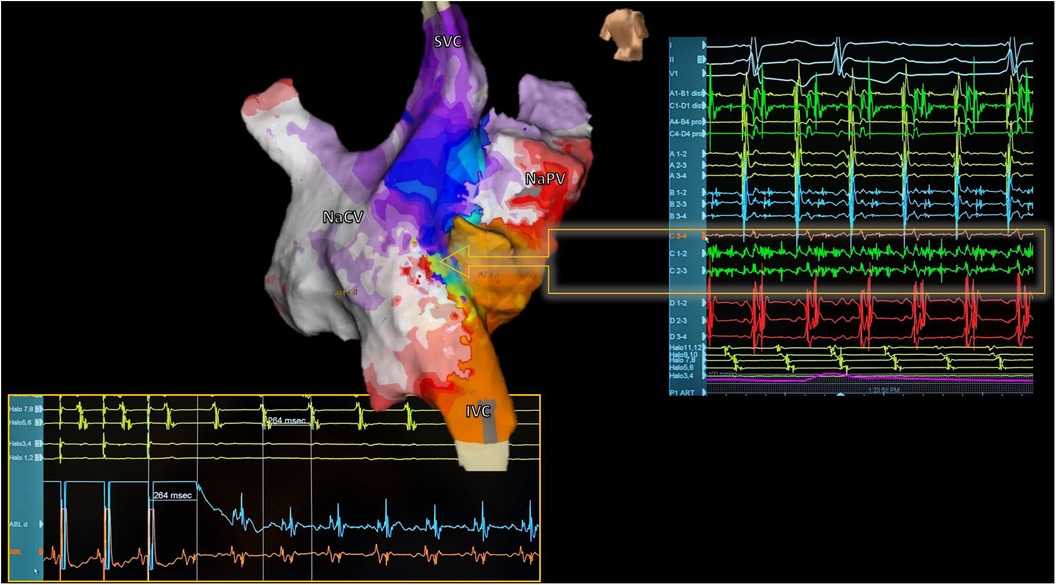
Figure 6. Reentrant atrial tachycardia electroanatomical mapping. Electroanatomic activation map of both neoatria during atrial tachycardia in a patient with corrected d-TGA using the Senning technique (Posterior view). Septal activation shows reentry-compatible activation (Arrow): Careful analysis of electrograms during mapping with a multipolar catheter identified a zone with fragmented and pandiastolic electrograms highly suggestive of the protected isthmus of the tachycardia (Right). At the bottom of the image, the catheter ablation maneuver positioned in this zone is shown, confirming the diagnosis. The tachycardia resolved during the first radiofrequency application. SVC, superior vena cava; IVC, inferior vena cava; NaVC, neocaval atrium; NaPV, neopulmonary vein atrium.
Electroanatomical navigation systems are very useful when dealing with scar-related flutter, figure-eight patterns, and multiple circuits, which are more common in patients with CHD. These advanced systems enable precise and efficient characterization of chamber anatomy, identifying blocking zones and fibrosis areas essential for planning the ablation line. Despite the increasing accuracy of automatic annotation systems, traditional electrophysiology remains valuable. Operators analyzing electrograms ensure proper annotation, particularly in areas with double potentials, delayed potentials, or absence of capture, contributing to precise anatomical substrate characterization. Accurate identification of blocking zones and dense scar, and also additional diagnostic maneuvers, such as entrainment, are useful when planning the ablation line. For instance, in patients with atriotomy scars (such as those performed in the majority of repaired ToF cases), a possible figure-eight pattern may be identified after EAM, with a counterclockwise circuit in the CTI and a clockwise circuit in the lateral wall, peri-scar (63). While only a small proportion genuinely exhibit a figure-eight flutter, ablation in the CTI is often sufficient to eliminate the tachycardia. Entrainments are also important for diagnosis. A long return cycle from the posterolateral aspect of the right atrium (posterior to the atriotomy scar) will suggest a passive rotation (Figure 7).
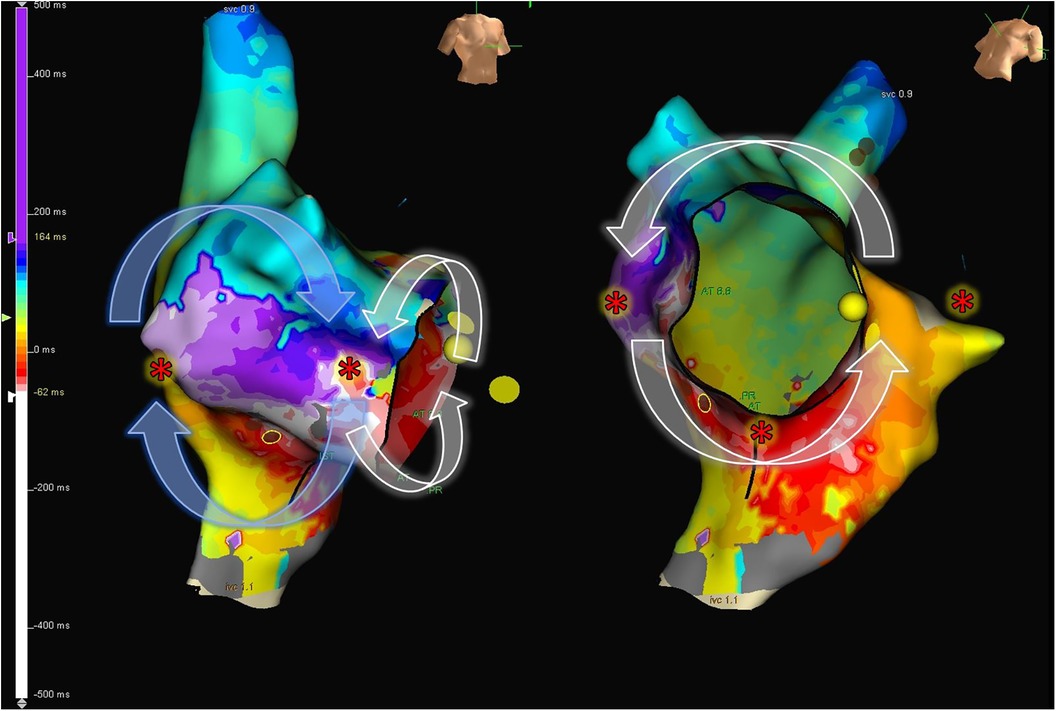
Figure 7. 8-shape figure atrial flutter. Electroanatomic map of the right atrium, showing activation during tachycardia in a patient with repaired Tetralogy of Fallot. An 8-shaped figure with counterclockwise rotation around the cavotricuspid isthmus and clockwise rotation in the lateral wall is observed. The “*” indicates sites frequently used to perform entrainment maneuvers to determine the active circuit of the tachycardia.
The main objective of ablation is the termination and non-reinduction of clinical arrhythmia (2, 45, 66, 70–71). However, it is not uncommon for the patient to present in sinus rhythm, or for more than one arrhythmia to be induced. In the first scenario, it is important to conduct an atrial induction protocol (since in most cases, at least one AT can be induced) and map it. In situations where mapping and determining the critical isthmus of the tachycardia cannot be achieved, either due to a lack of inducibility or the presence of unstable arrhythmias, empirical ablation of the CTI or its equivalent would be appropriate, given that it is the most frequently involved anatomical substrate. If other arrhythmias that can be mapped are induced after the ablation of the clinical arrhythmia, these can also be ablated. Recent research indicates a lower recurrence of arrhythmias in patients who underwent ablation for all induced ATs compared to those where only the clinical arrhythmia was addressed (45). There remains some debate surrounding the need to empirically address possible anatomical isthmuses that have not been confirmed to be involved in the genesis of a clinically or induced AT.
An ablation line must be created between two anatomical obstacles or scars to interrupt the circuit, preferably addressing the protected isthmus. It is essential to emphasize the importance of verifying bidirectional block of the ablation line to prevent future recurrences. Although it may be more challenging in complex anatomies, maneuvers such as differential pacing, preferably associated with new EAM, generally allow for confirmation of the blockade of the line or for the identification of possible gaps in the event of persistent conduction.
Atrial fibrillation
Atrial fibrillation was previously considered much less frequent than MRAT. Nevertheless, its prevalence has significantly increased in current cohorts due to improved survival of patients with complex cardiopathies (2, 62). AF often appears in patients with a history of prior MRAT, indicating a more advanced stage of atrial cardiomyopathy. A rhythm control strategy is usually preferred, as the loss of atrial contraction can be poorly tolerated. However, treatment effectiveness is suboptimal, and despite therapeutic efforts, patients may experience recurrences and a tendency toward chronicity. The results of AF ablation in patients with complex CHD have been disappointing thus far, likely because the automatic activity of the pulmonary veins (typically addressed in conventional AF ablation) represents only a small part of its pathophysiology (44). AF appears in more advanced stages of the disease, with highly fibrotic and diseased atria. Therefore, treating MRAT, which often precedes AF, in its early stages is crucial to attempting to prevent the progression of atrial cardiomyopathy secondary to the tachyarrhythmia. In this context, it is also important to assess and treat potential reversible causes, such as residual communications or obstructions, in order to reduce recurrence. Another important aspect to consider is the embolic risk and the need for anticoagulation. Commonly used scales (such as CHA2DS2-VASc) have not been validated in this population, so the decision for long-term anticoagulation should be made on an individualized basis. In patients with complex CHD with persistent or recurrent AF, long-term anticoagulation is likely indicated regardless of other thrombotic risk factors (2).
Other supraventricular arrhythmias
Supraventricular arrhythmias, such as atrioventricular nodal reentrant tachycardia and accessory pathway-mediated tachycardia, can also occur in patients with CHD. Accessory pathways may be present in up to 20% of patients with Ebstein's anomaly. “Atypical” pathways, such as atriofascicular (Mahaim) pathways or the presence of multiple pathways, are also more prevalent in these patients (2). A nearly specific type of SVA in cases of right isomerism syndrome and congenitally corrected TGA is twin atrioventricular nodal reentrant tachycardia. This rare type of reentry occurs in patients who have two AV nodes, resulting in an atrioventricular reentry circuit between them.
Similar to the general population, CA is usually the treatment of choice, although the procedure is often more complex due to anatomical peculiarities. Nevertheless, experienced centers achieve high success rates and safety in CA. Knowledge of anatomy is crucial when planning the ablation. Special attention should be given to the location of the conduction system, as some substrates such as TGA, heterotaxy syndromes, or patients with septal defects may be displaced, increasing the risk of AV block during the procedure.
Ventricular arrhythmias
Ventricular arrhythmias in this population include monomorphic VT, polymorphic VT, and ventricular fibrillation. Monomorphic VT is more prevalent in patients with incisions or patches in the ventricular myocardium. In patients with CHD, monomorphic VTs can be very fast and hemodynamically poorly tolerated, potentially leading to SD, even in patients with preserved ventricular function. In fact, in patients with repaired ToF and TGA who have implantable cardioverter-defibrillators (ICD), most appropriate therapies correspond to episodes of high-frequency monomorphic VT (72, 73).
Risk stratification
The identification of patients at a higher risk of ventricular arrhythmias would allow for the implementation of primary prevention measures and the minimization of complications from unnecessary therapies. However, the stratification of arrhythmic risk in the CHD population is complex due to the significant anatomical variability and variations in surgical repairs, the low SD rate, and the need for prolonged follow-up, making randomized clinical trials challenging. Although moderate-to-severe ventricular dysfunction, especially of the systemic ventricle, is a risk factor associated with SD, patients with preserved or only slightly impaired ventricular function may experience fatal arrhythmic events. Repaired ToF (rToF) is the best-studied cardiac condition, with multiple risk factors associated with ventricular arrhythmias and/or SD having been described (Table 2) (74–77).
In patients with rToF, the occurrence of non-sustained ventricular tachycardia identifies a specific subset of patients who require careful monitoring. Some accessible non-invasive markers, such as filtered QRS duration, low amplitude signal duration in the terminal portion of the filtered QRS complex and the ratio of the maximum short-axis diameters of the right and left ventricles have been associated with potentially malignant ventricular arrhythmias (78, 79).
However, when considered individually, the predictive value of these risk factors is limited. Therefore, the indication for implantation of an ICD in primary prevention in patients with repaired ToF, aside from severe dysfunction of the systemic ventricle (left ventricular ejection fraction <35%) in patients in NYHA functional class II-III (class IIa), is based on the combination of several risk factors (class IIa), including systolic or diastolic dysfunction of the left ventricle, documentation of non-sustained VT, QRS duration ≥180 ms, inducibility of sustained VT in EPS, and the presence of extensive fibrosis in the right ventricle analyzed by cardiac magnetic resonance imaging (1, 55, 80).
In recent years, new tools for stratifying the risk of SCD in patients with CHD have emerged, such as the PREVENTION-ACHD risk score model (81). This model incorporates common clinical variables like coronary artery disease, NYHA class II/III, supraventricular tachycardia, systemic ejection fraction <40%, subpulmonary ejection fraction <40%, and QRS duration. Its accuracy exceeds current guideline indications, potentially aiding in the selection of CHD patients who may benefit from preventive implantable ICD implantation (81).
Mechanism and anatomic substrate of ventricular tachycardia
Patients with CHD exhibit a substrate that predisposes them to ventricular arrhythmias, due to a combination of surgical scars, anatomical barriers, and myocardial tissue injury. Repaired ToF is the most emblematic example of CHD repair with this substrate. Studies have demonstrated that the most common ventricular arrhythmia in patients with repaired ToF is monomorphic ventricular tachycardia (82), and the underlying mechanism is macro-reentry (83). These macro-reentrant circuits are typically situated in the right ventricular outflow tract (RVOT) and are associated with the repair surgery (83, 84). Previously, ToF repair surgery involved a ventricular approach, aiming for extensive release of the RVOT, working from the assumption that residual pulmonary insufficiency had no long-term repercussions. Consequently, in addition to the patch for closing the VSD, most patients have a ventriculotomy scar, and in many cases, a transannular patch for RVOT enlargement (Figure 8). The substrate formed by surgical repair and myocardial changes due to hemodynamic overload from residual lesions, with residual pulmonary insufficiency being the most common, is responsible for the development of ventricular arrhythmias in adulthood among these patients.
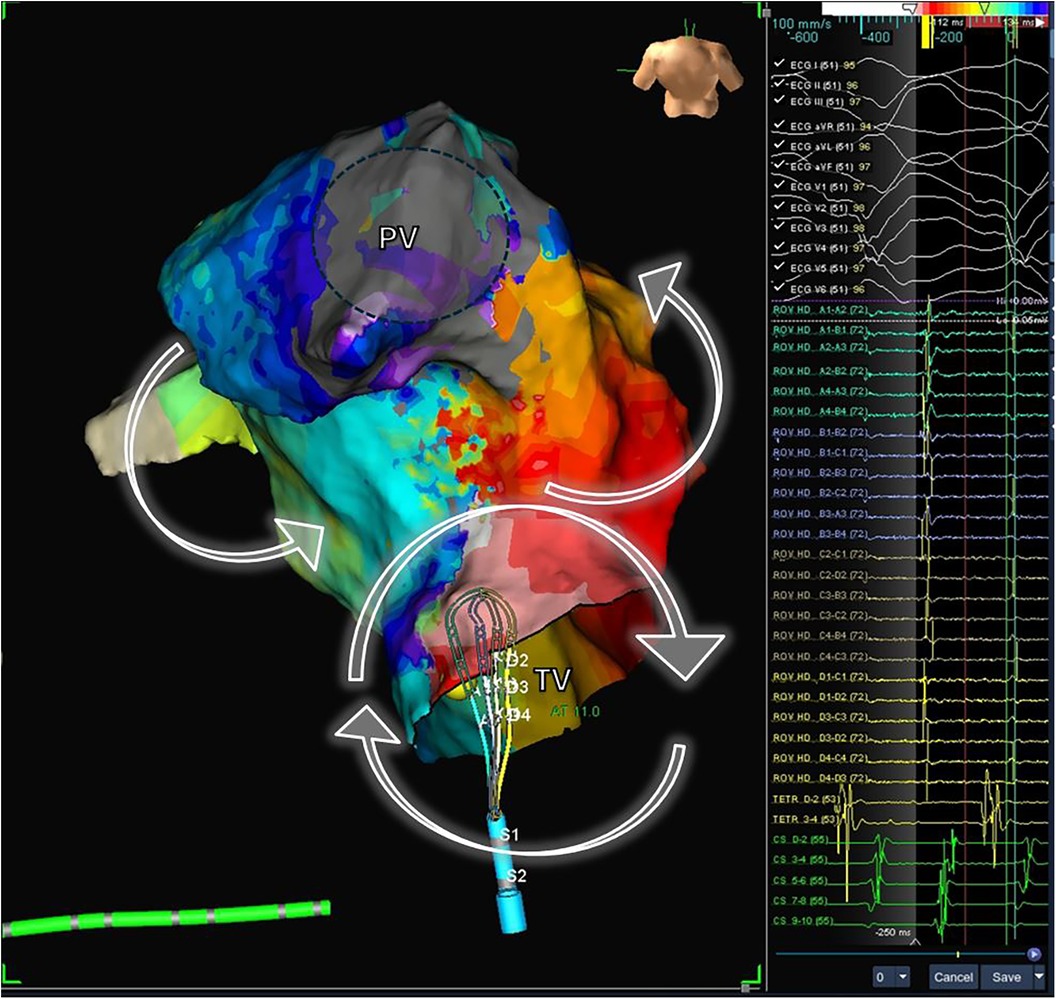
Figure 8. Electrophysiological study and electroanatomic mapping of ventricular tachycardia. The electroanatomic activation map of a ventricular tachycardia in a patient with repaired Tetralogy of Fallot is shown. The mechanism of VT is a macro-reentrant circuit with clockwise activation around the tricuspid valve (VP) and counterclock activation around the pulmonary valve (PV). The mapping catheter is located in the critical isthmus, showing mid-diastolic potentials (right panel). PV, pulmonary valve; TV, tricuspid valve.
Electroanatomic mapping
Electroanatomic mapping techniques, combined with electrophysiological entrainment maneuvers, enable precise identification of the macro-reentrant electrical circuit in these arrhythmias and establish the critical isthmus maintaining the arrhythmia (Figure 8). In repaired Tetralogy of Fallot (rToF), the critical anatomical isthmuses sustaining these macro-reentrant circuits are delineated by the non-excitable tissue of the valvular rings, prosthetic patches, and surgical scars. Electro-anatomic mapping of right ventricular voltage in sinus rhythm (considering electrograms >1.5 mV as high voltage and electrically non-excitable tissue as electrograms with voltage <0.5 mV and without ventricular capture with 10 mA stimulation and 2 ms) has identified four anatomical isthmuses that may be present in these patients (85). These four isthmuses are defined between the following structures: isthmus 1 between the transannular patch/ventriculotomy and the tricuspid ring, isthmus 2 between ventriculotomy and the pulmonary ring, isthmus 3 between VSD patch and the pulmonary ring, and isthmus 4 between the VSD patch and the tricuspid ring (Figure 9) (85). It is noteworthy that although the majority of rToF patients have at least one of these anatomical isthmuses, not all can propagate sustained monomorphic VT. In this regard, it has been observed that arrhythmogenic isthmuses are longer, narrower, and have a slower conduction velocity, which can help define the anatomical location of the ablation (86).
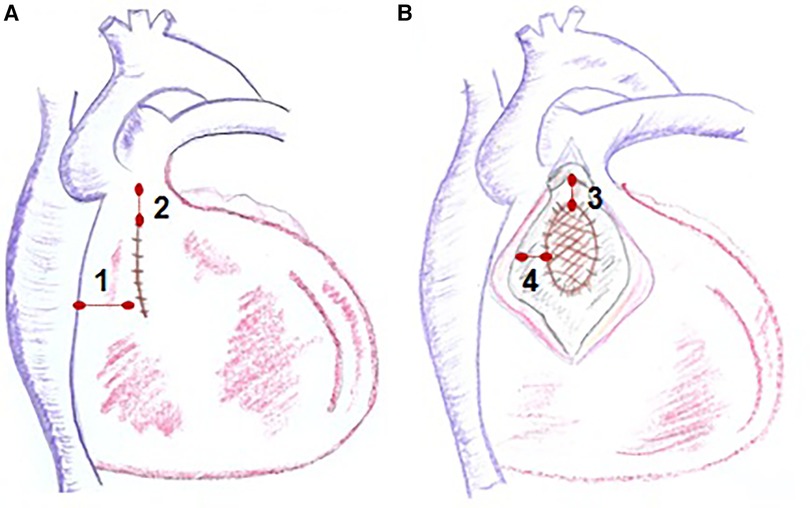
Figure 9. Anatomical isthmuses in repaired tetralogy of fallot. Diagram of the anatomical isthmuses responsible for ventricular tachycardia (VT) in repaired ToF, as defined by Zeppenfeld et al. (A) Between ventriculotomy or transannular patch and tricuspid annulus (isthmus 1), between ventriculotomy and pulmonary annulus (isthmus 2). (B) Between VSD patch and pulmonary annulus (isthmus 3) and between VSD and tricuspid annulus (isthmus 4). VSD, ventricular septal defect.
In other anatomical substrates, scientific evidence regarding the mechanism of VT and the arrhythmic substrate is limited, and additional anatomical isthmuses have been described in patients with TGA, VSD, or repaired pulmonary stenosis (87).
Ablation
Radiofrequency ablation of VT in patients with repaired CHD is feasible, with reported acute efficacy ranging from 50% to 100% and variable recurrence rates (85, 88). Comparing published studies is complex due to small sample sizes, the use of diverse mapping and ablation technologies, and different efficacy criteria. Kapel et al. reported an acute efficacy of 74% with no recurrences in a mean follow-up of 46 months, utilizing EAM systems and irrigated ablation catheters. The procedure was considered effective when achieving conduction block at the critical anatomical isthmus level and demonstrating absence of inducibility. Therefore, considering the macro-reentrant mechanism of these arrhythmias, it seems reasonable to aim for bidirectional block of the critical isthmus and absence of inducibility in ablation procedures.
In those patients in whom sustained monomorphic VT is induced with good hemodynamic tolerance, the identification of arrhythmogenic isthmuses for ablation can be based on electro-anatomic mapping during tachycardia and entrainment maneuvers. However, complete mapping during VT is often not possible due to lack of inducibility or poor hemodynamic tolerance (72, 73). In the case of patients with rToF, considering the association between the substrate identified in sinus rhythm and the induced VTs, substrate ablation can be considered with the goal of blocking conduction through the arrhythmogenic isthmuses considered (86).
The efficacy of ablation may be limited due to dilation of the cavities, hypertrophy of the myocardial tissue, or the presence of prosthetic material [e.g., after pulmonary valve replacement (PVR)]. In patients with rToF where blockade of septal isthmuses cannot be achieved, either due to hypertrophy or the presence of a pulmonary valve prosthesis, it may be useful to complement the ablation line contralaterally using a left-sided approach.
The role of empirical ablation at the time of PVR in patients with rToF and severe residual pulmonary insufficiency is a subject of controversy. Since residual hemodynamic defects are associated with a higher risk of VT and SD, addressing their treatment is important. However, there is doubt as to whether PVR reduces the long-term arrhythmic risk and whether it should be associated with ablation of anatomical isthmuses (89, 90). Sometimes, the arrhythmogenic substrate does not completely revert after PVR, even with overall remodeling of the right ventricle (reduction in volumes and improvement in systolic function). Additionally, access to isthmus 3 (between the pulmonary ring and VSD) may be limited after PVR due to the interposition of the prosthesis in the pulmonary position. Therefore, it seems reasonable to consider associating ablation of arrhythmogenic isthmuses before or during PVR with the aim of preventing monomorphic VTs during follow-up. Although cryoablation during PVR surgery has not been reported to be proarrhythmic and could have a protective role in the long term (59), long-term studies are needed to clarify this point.
Assessment of arrhythmic substrate using imaging techniques
Late gadolinium enhancement study in cardiac MRI allows the extent and distribution of fibrotic tissue to be determined. In fact, the 3D reconstruction of images obtained with late gadolinium enhancement MRI has proven to be useful for characterizing the arrhythmic substrate of the left ventricle in patients with ischemic heart disease. A good correlation has been documented in the identification of fibrosis and conduction channels between EAM and late gadolinium enhancement MRI, with the latter providing appropriate guidance for planning and guiding the ablation procedure (52, 91). However, the analysis of the right ventricle, which is often involved in VTs in patients with CHD, is more complex due to its thinner wall and the proximity of epicardial fat. There appears to be an association between the extent of fibrosis in late gadolinium enhancement MRI and the history of arrhythmias in patients with repaired Tetralogy of Fallot (92). Additionally, the 3D reconstruction of late gadolinium enhancement MRI of the right ventricle has demonstrated correlation with the substrate identified by voltage mapping, both in the extent and location of fibrosis (55, 93), as well as in the identification of anatomical isthmuses (55), making it potentially useful for guiding the ablation procedure.
Future perspectives
The shift in the surgical approach during corrective procedures, avoiding ventriculotomy through transatrial and transpulmonary methods, and the minimization of residual hemodynamic lesions, will modify the arrhythmic substrate of the ventricular myocardium in patients with repaired CHD. This change has the potential to influence the risk of arrhythmias and the treatment approach through ablation. The characterization of the arrhythmogenic substrate and anatomical isthmuses, achieved via 3D reconstruction of late gadolinium-enhanced MRI, EPS and EAM in sinus rhythm, could emerge as a valuable tool for risk stratification concerning VT and SD in individuals with repaired CHD (23, 55, 94, 95). Further studies are necessary to elucidate the role of empirical ablation during pulmonary valve replacement in patients with repaired Tetralogy of Fallot (96, 97).
Author contributions
JF-P: Conceptualization, Data curation, Formal Analysis, Investigation, Methodology, Resources, Supervision, Validation, Visualization, Writing – original draft, Writing – review & editing. NM: Writing – original draft. AS-O: Writing – review & editing. NR-G: Conceptualization, Project administration, Writing – review & editing.
Funding
The author(s) declare financial support was received for the research, authorship, and/or publication of this article.
This work was partially funded by Instituto de Salud Carlos III - CIBER de Enfermedades Cardiovasculares (CIBER-CV).
Acknowledgments
The authors would like to thank Ms Josefina Gándara Rosales for her help with the illustrations.
Conflict of interest
The arrhythmia unit at H Vall d'Hebron receives research and teaching grants from Abbott, Boston Scientific, Medtronic, Biotronik and Microport. JF-P and receives consulting fees and honoraria for teaching from Abbott. NR-G received consulting fees and honoraria for teaching from Abbott, Medtronic and Johnson & Johnson.
The remaining authors declare that the research was conducted in the absence of any commercial or financial relationships that could be construed as a potential conflict of interest.
Publisher's note
All claims expressed in this article are solely those of the authors and do not necessarily represent those of their affiliated organizations, or those of the publisher, the editors and the reviewers. Any product that may be evaluated in this article, or claim that may be made by its manufacturer, is not guaranteed or endorsed by the publisher.
References
1. Baumgartner H, de Backer J, Babu-Narayan S V, Budts W, Chessa M, Diller GP, et al. 2020 ESC guidelines for the management of adult congenital heart disease. Eur Heart J. (2021) 42:563–645. doi: 10.1093/EURHEARTJ/EHAA554
2. Hernández-Madrid A, Paul T, Abrams D, Aziz PF, Blom NA, Chen J, et al. Arrhythmias in congenital heart disease: a position paper of the European Heart Rhythm Association (EHRA), association for European Paediatric and Congenital Cardiology (AEPC), and the European Society of Cardiology (ESC) working group on grown-up congenital heart disease, endorsed by HRS, PACES, APHRS, and SOLAECE. Europace. (2018) 20:1719–20. doi: 10.1093/EUROPACE/EUX380
3. Casteigt B, Samuel M, Laplante L, Shohoudi A, Apers S, Kovacs AH, et al. Atrial arrhythmias and patient-reported outcomes in adults with congenital heart disease: an international study. Heart Rhythm. (2021) 18:793–800. doi: 10.1016/j.hrthm.2020.09.012
4. Verheugt CL, Uiterwaal CSPM, Van Der Velde ET, Meijboom FJ, Pieper PG, Sieswerda GT, et al. The emerging burden of hospital admissions of adults with congenital heart disease. Heart. (2010) 96:872–8. doi: 10.1136/hrt.2009.185595
5. Bouchardy J, Therrien J, Pilote L, Ionescu-Ittu R, Martucci G, Bottega N, et al. Atrial arrhythmias in adults with congenital heart disease. Circulation. (2009) 120(17):1679–86. doi: 10.1161/CIRCULATIONAHA.109.866319
6. Dos L, Teruel L, Ferreira IJ, Rodriguez-Larrea J, Miro L, Girona J, et al. Late outcome of senning and mustard procedures for correction of transposition of the great arteries. Heart. (2005) 91:652–6. doi: 10.1136/hrt.2003.029769
7. Silka MJ, Hardy BG, Menashe VD, Morris CD. A population-based prospective evaluation of risk of sudden cardiac death after operation for common congenital heart defects. J Am Coll Cardiol. (1998) 32:245–51. doi: 10.1016/S0735-1097(98)00187-9
8. Koyak Z, Harris L, De Groot JR, Silversides CK, Oechslin EN, Bouma BJ, et al. Sudden cardiac death in adult congenital heart disease. Circulation. (2012) 126:1944–54. doi: 10.1161/CIRCULATIONAHA.112.104786
9. Roca-Luque I, Rivas-Gándara N, Subirà LD, Francisco-Pascual J, Pijuan-Domenech A, Pérez-Rodon J, et al. Mechanisms of intra-atrial re-entrant tachycardias in congenital heart disease: types and predictors. Am J Cardiol. (2018) 122(4):672–82. doi: 10.1016/j.amjcard.2018.04.041
10. Ntiloudi D, Rammos S, Karakosta M, Kalesi A, Kasinos N, Giannakoulas G. Arrhythmias in patients with congenital heart disease: an ongoing morbidity. J Clin Med. (2023) 12:7020. doi: 10.3390/jcm12227020
11. Walsh EP, Cecchin F. Arrhythmias in adult patients with congenital heart disease. Circulation. (2007) 115(4):534–45. doi: 10.1161/CIRCULATIONAHA.105.592410
12. Koole MA, de Jong S, Mulder BJ, Bouma BJ, Schuuring MJ. Value of extended arrhythmia screening in adult congenital heart disease patients. Arrhythm Electrophysiol Rev. (2024) 13:e07. doi: 10.15420/AER.2023.28
13. Francisco-Pascual J, Olivella San Emeterio A, Rivas-Gándara N, Pérez-Rodón J, Benito B, Santos-Ortega A, et al. High incidence of subclinical atrial fibrillation in patients with syncope monitored with implantable cardiac monitor. Int J Cardiol. (2020) 316:110–6. doi: 10.1016/j.ijcard.2020.05.078
14. Francisco-Pascual J, Cantalapiedra-Romero J, Pérez-Rodon J, Benito B, Santos-Ortega A, Maldonado J, et al. Cardiac monitoring for patients with palpitations. World J Cardiol. (2021) 13:608–27. doi: 10.4330/WJC.V13.I11.608
15. Pagola J, Juega J, Francisco-Pascual J, Rodriguez M, Dorado L, Martinez R, et al. Intensive 90-day textile wearable holter monitoring: an alternative to detect paroxysmal atrial fibrillation in selected patients with cryptogenic stroke. Heart Vessels. (2023) 38(1):114–21. doi: 10.1007/s00380-022-02141-9
16. Pagola J, Juega J, Francisco-Pascual J, Moya A, Sanchis M, Bustamante A, et al. Yield of atrial fibrillation detection with textile wearable Holter from the acute phase of stroke: pilot study of crypto-AF registry. Int J Cardiol. (2018) 251:45–50. doi: 10.1016/j.ijcard.2017.10.063
17. Soulaidopoulos S, Arsenos P, Doundoulakis I, Tsiachris D, Antoniou CK, Dilaveris P, et al. Syncope associated with supraventricular tachycardia: diagnostic role of implantable loop recorders. Ann Noninvasive Electrocardiol. (2021) 26:e12850. doi: 10.1111/ANEC.12850
18. Steinberg JS, Varma N, Cygankiewicz I, Aziz P, Balsam P, Baranchuk A, et al. 2017 ISHNE-HRS expert consensus statement on ambulatory ECG and external cardiac monitoring/telemetry. Heart Rhythm. (2017) 14(7):e55–96. doi: 10.1016/j.hrthm.2017.03.038
19. Sana F, Isselbacher EM, Singh JP, Heist EK, Pathik B, Armoundas AA. Wearable devices for ambulatory cardiac monitoring: JACC state-of-the-art review. J Am Coll Cardiol. (2020) 75:1582–92. doi: 10.1016/j.jacc.2020.01.046
20. PérezRodon J, FranciscoPascual J, RivasGándara N, RocaLuque I, Bellera N, MoyaMitjans À. Cryptogenic stroke and role of loop recorder. J Atr Fibrillation. (2014) 7(4):1178. doi: 10.4022/jafib.1178
21. Francisco-Pascual J, Rodenas-Alesina E, Rivas-Gándara N, Belahnech Y, Olivella San Emeterio A, Pérez-Rodón J, et al. Etiology and prognosis of patients with unexplained syncope and mid-range left ventricular dysfunction. Heart Rhythm. (2021) 18(4):597–604. doi: 10.1016/j.hrthm.2020.12.009
22. Roca-Luque I, Francisco-Pascual J, Oristrell G, Rodríguez-García J, Santos-Ortega A, Martin-Sanchez G, et al. Syncope, conduction disturbance, and negative electrophysiological test: predictive factors and risk score to predict pacemaker implantation during follow-up. Heart Rhythm. (2019) 16(6):905–12. doi: 10.1016/j.hrthm.2018.12.015
23. Rivas-Gándara N, Francisco-Pascual J, Pijuan-Domenech A, Ribera-Solé A, Dos-Subirá L, Benito B, et al. Risk stratification of ventricular arrhythmias in repaired tetralogy of Fallot. Rev Española Cardiol (Engl Ed). (2021) e-pub ahead of print.
24. Francisco-Pascual J, Rivas-Gándara N, Maymi-Ballesteros M, Badia-Molins C, Bach-Oller M, Benito B, et al. Arrhythmic risk in single or recurrent episodes of unexplained syncope with complete bundle branch block. Rev Esp Cardiol (Engl Ed). (2023) 76(8):609–17. (English, Spanish). doi: 10.1016/j.rec.2022.11.009
25. Menozzi C, Brignole M, Garcia-Civera R, Moya A, Botto G, Tercedor L, et al. Mechanism of syncope in patients with heart disease and negative electrophysiologic test. Circulation. (2002) 105:2741–5. doi: 10.1161/01.CIR.0000018125.31973.87
26. Roca-Luque I, Oristrell G, Francisco-Pasqual J, Rodríguez-García J, Santos-Ortega A, Martin-Sanchez G, et al. Predictors of positive electrophysiological study in patients with syncope and bundle branch block: PR interval and type of conduction disturbance. Clin Cardiol. (2018) 41(12):1537–42. doi: 10.1002/clc.23079
27. Roca-Luque I, Francisco-Pasqual J, Oristrell G, Rodríguez-García J, Santos-Ortega A, Martin-Sanchez G, et al. Flecainide versus procainamide in electrophysiological study in patients with syncope and wide QRS duration. JACC Clin Electrophysiol. (2019) 5(2):212–9. doi: 10.1016/j.jacep.2018.09.015
28. Francisco-Pascual J, Lal-Trehan Estrada N. Syncope. Med Clin (Barc). (2024) S0025–7753(24):00031–9. (English, Spanish). doi: 10.1016/j.medcli.2023.12.014
29. Doundoulakis I, Soulaidopoulos S, Arsenos P, Dilaveris P, Tsiachris D, Antoniou C-K, et al. Unexplained syncope: the importance of the electrophysiology study. Hearts. (2021) 2:495–504. doi: 10.3390/HEARTS2040038
30. Sheldon RS, Lei LY, Solbiati M, Chew DS, Raj SR, Costantino G, et al. Electrophysiology studies for predicting atrioventricular block in patients with syncope: a systematic review and meta-analysis. Heart Rhythm. (2021) 18:1310–7. doi: 10.1016/j.hrthm.2021.04.010
31. Brignole M, Moya A, De Lange FJ, Deharo JC, Elliott PM, Fanciulli A, et al. 2018 ESC guidelines for the diagnosis and management of syncope. Eur Heart J. (2018) 39(21):1883–948. doi: 10.1093/eurheartj/ehy037
32. Garcia-Civera R, Ruiz-Granell R, Morell-Cabedo S, Sanjuan-Mañez R, Perez-Alcala F, Plancha E, et al. Selective use of diagnostic tests in patients with syncope of unknown cause. J Am Coll Cardiol. (2003) 41:787–90. doi: 10.1016/S0735-1097(02)02929-7
33. Francisco-Pascual J, Rivas-Gándara N, Bach-Oller M, Badia-Molins C, Maymi-Ballesteros M, Benito B, et al. Sex-related differences in patients with unexplained syncope and bundle branch block: lower risk of AV block and lesser need for cardiac pacing in women. Front Cardiovasc Med. (2022) 9:838473. doi: 10.3389/FCVM.2022.838473
34. Francisco-Pascual J, Rivas-Gándara N, Santos-Ortega A, Pérez-Rodón J, Benito B, Belahnech Y, et al. Cardiac biometric variables and arrhythmic events during COVID-19 pandemic lockdown in patients with an implantable cardiac monitor for syncope work-up. Med Clin (Engl Ed). (2021) 156(10):496–9. doi: 10.1016/j.medcle.2020.12.017
35. Francisco Pascual J, Jordan Marchite P, Rodríguez Silva J, Rivas Gándara N. Arrhythmic syncope: from diagnosis to management. World J Cardiol. (2023) 15:119–41. doi: 10.4330/wjc.v15.i4.119
36. Francisco-Pascual J, Rodenas E, Belahnech Y, Rivas-Gándara N, Pérez-Rodon J, Santos-Ortega A, et al. Syncope in patients with severe aortic stenosis: more than just an obstruction issue. Can J Cardiol. (2021) 37(2):284–91. doi: 10.1016/j.cjca.2020.04.047
37. Roca-Luque I, Rivas Gándara N, Dos Subirà L, Francisco Pascual J, Pérez-Rodon J, Pijuan Domenech A, et al. Intra-atrial re-entrant tachycardia in patients with congenital heart disease: factors associated with disease severity. Europace. (2018) 20(8):1343–51. doi: 10.1093/europace/eux180
38. Khairy P, Aboulhosn J, Broberg CS, Cohen S, Cook S, Dore A, et al. Thromboprophylaxis for atrial arrhythmias in congenital heart disease: a multicenter study. Int J Cardiol. (2016) 223:729–35. doi: 10.1016/j.ijcard.2016.08.223
39. Moore BM, Cordina RL, McGuire MA, Celermajer DS. Adverse effects of amiodarone therapy in adults with congenital heart disease. Congenit Heart Dis. (2018) 13:944–51. doi: 10.1111/chd.12657
40. Moore BM, Cordina RL, McGuire MA, Celermajer DS. Efficacy and adverse effects of sotalol in adults with congenital heart disease. Int J Cardiol. (2019) 274:74–9. doi: 10.1016/j.ijcard.2018.06.066
41. Hindricks G, Potpara T, Kirchhof P, Kühne M, Ahlsson A, Balsam P, et al. 2020 ESC guidelines for the diagnosis and management of atrial fibrillation developed in collaboration with the European Association for Cardio-Thoracic Surgery (EACTS). Eur Heart J. (2021) 42:373–498. doi: 10.1093/eurheartj/ehaa612
42. Wasmer K, Eckardt L. Management of supraventricular arrhythmias in adults with congenital heart disease. Heart. (2016) 102:1614–9. doi: 10.1136/HEARTJNL-2015-309068
43. Guarguagli S, Kempny A, Cazzoli I, Barracano R, Gatzoulis MA, Dimopoulos K, et al. Efficacy of catheter ablation for atrial fibrillation in patients with congenital heart disease. EP Europace. (2019) 21:1334–44. doi: 10.1093/EUROPACE/EUZ157
44. Soulaidopoulos S, Brili S, Drakopoulou M, Sotiropoulos I, Archontakis S, Dilaveris P, et al. Catheter ablation in grown-up congenital heart disease patients: a single-center experience. Int J Cardiol Congenit Heart Dis. (2022) 7:100326. doi: 10.1016/j.ijcchd.2022.100326
45. Waldmann V, Amet D, Zhao A, Ladouceur M, Otmani A, Karsenty C, et al. Catheter ablation of intra-atrial reentrant/focal atrial tachycardia in adult congenital heart disease: value of final programmed atrial stimulation. Heart Rhythm. (2020) 17:1953–9. doi: 10.1016/J.HRTHM.2020.05.039
46. Roca-Luque I, Rivas-Gándara N, Dos Subirà L, Francisco Pascual J, Pijuan-Domenech A, Pérez-Rodon J, et al. Long-term follow-up after ablation of intra-atrial re-entrant tachycardia in patients with congenital heart disease: types and predictors of recurrence. JACC Clin Electrophysiol. (2018) 4(6):771–80. doi: 10.1016/j.jacep.2018.04.011
47. Fischer AJ, Enders D, Wasmer K, Marschall U, Baumgartner H, Diller GP. Impact of specialized electrophysiological care on the outcome of catheter ablation for supraventricular tachycardias in adults with congenital heart disease: independent risk factors and gender aspects. Heart Rhythm. (2021) 18:1852–9. doi: 10.1016/j.hrthm.2021.07.009
48. Singh SM, Neuzil P, Skoka J, Kriz R, Popelova J, Love BA, et al. Percutaneous transhepatic venous access for catheter ablation procedures in patients with interruption of the inferior vena cava. Circ Arrhythm Electrophysiol. (2011) 4:235–41. doi: 10.1161/CIRCEP.110.960856
49. Erdǒan I, Özer S, KaragÖz T, Çeliker A. Transesophageal electrophysiologic study to determine the inducibility after successful ablation of supraventricular tachycardia in children. Pacing Clin Electrophysiol. (2009) 32:1402–6. doi: 10.1111/j.1540-8159.2009.02475.x
50. Laredo M, Waldmann V, Soulat G, Amet D, Marijon E, Iserin L, et al. Transbaffle/transconduit puncture using a simple CARTO-guided approach without echocardiography in patients with congenital heart disease. J Cardiovasc Electrophysiol. (2020) 31:2049–60. doi: 10.1111/jce.14590
51. Illmann CF, Ghadiry-Tavi R, Hosking M, Harris KC. Utility of 3D printed cardiac models in congenital heart disease: a scoping review. Heart. (2020) 106:1631–7. doi: 10.1136/heartjnl-2020-316943
52. Andreu D, Penela D, Acosta J, Fernández-Armenta J, Perea RJ, Soto-Iglesias D, et al. Cardiac magnetic resonance–aided scar dechanneling: influence on acute and long-term outcomes. Heart Rhythm. (2017) 14:1121–8. doi: 10.1016/j.hrthm.2017.05.018
53. Roney CH, Sillett C, Whitaker J, Lemus JAS, Sim I, Kotadia I, et al. Applications of multimodality imaging for left atrial catheter ablation. Eur Heart J Cardiovasc Imaging. (2021) 23(1):31–41. doi: 10.1093/ehjci/jeab205
54. Roca-Luque I, Rivas-Gándara N, Francisco-Pascual J, Rodriguez-Sanchez J, Cuellar-Calabria H, Rodriguez-Palomares J, et al. Preprocedural imaging to guide transcoronary ethanol ablation for refractory septal ventricular tachycardia. J Cardiovasc Electrophysiol. (2019) 30:448–56. doi: 10.1111/jce.13816
55. Rivas-Gándara N, Dos-Subirá L, Francisco-Pascual J, Rodríguez-García J, Pijuan-Domenech A, Benito B, et al. Substrate characterization of the right ventricle in repaired tetralogy of Fallot using late enhancement cardiac magnetic resonance. Heart Rhythm. (2021) 18:1868–75. doi: 10.1016/j.hrthm.2021.05.032
56. Roca-Luque I, Rivas-Gándara N, Dos-Subirà L, Francisco-Pascual J, Pijuan-Domenech A, Pérez-Rodon J, et al. Predictors of acute failure ablation of intra-atrial re-entrant tachycardia in patients with congenital heart disease: cardiac disease, atypical flutter, and previous atrial fibrillation. J Am Heart Assoc. (2018) 7:e008063. doi: 10.1161/JAHA.117.008063
57. Martin CA, Yue A, Martin R, Claridge S, Sawhney V, Maury P, et al. Ultra–high-density activation mapping to aid isthmus identification of atrial tachycardias in congenital heart disease. JACC Clin Electrophysiol. (2019) 5:1459–72. doi: 10.1016/j.jacep.2019.08.001
58. Mantziari L, Butcher C, Shi R, Kontogeorgis A, Opel A, Chen Z, et al. Characterization of the mechanism and substrate of atrial tachycardia using ultra-high-density mapping in adults with congenital heart disease: impact on clinical outcomes. J Am Heart Assoc. (2019) 8:e010535. doi: 10.1161/JAHA.118.010535
59. Jone PN, Haak A, Ross M, Wiktor DM, Gill E, Quaife RA, et al. Congenital and structural heart disease interventions using echocardiography-fluoroscopy fusion imaging. J Am Soc Echocardiogr. (2019) 32:1495–504. doi: 10.1016/j.echo.2019.07.023
60. Ávila P, Bessière F, Mondésert B, Abadir S, Dore A, Mongeon FP, et al. Cryoablation for perinodal arrhythmia substrates in patients with congenital heart disease and displaced atrioventricular conduction systems. JACC Clin Electrophysiol. (2018) 4:1328–37. doi: 10.1016/j.jacep.2018.05.026
61. Roy K, Gomez-Pulido F, Ernst S. Remote magnetic navigation for catheter ablation in patients with congenital heart disease: a review. J Cardiovasc Electrophysiol. (2016) 27:45–56. doi: 10.1111/jce.12903
62. Ávila P, Oliver JM, Gallego P, González-García A, Rodríguez-Puras MJ, Cambronero E, et al. Natural history and clinical predictors of atrial tachycardia in adults with congenital heart disease. Circ Arrhythm Electrophysiol. (2017) 10(9):e005396. doi: 10.1161/CIRCEP.117.005396
63. Roca-Luque I, Rivas-Gándara N, Dos Subirà L, Francisco-Pascual J, Pijuan-Domenech A, Pérez-Rodon J, et al. Mechanisms of intra-atrial re-entrant tachycardias in congenital heart disease: types and predictors. Am J Cardiol. (2018) 122:672–82. doi: 10.1016/J.AMJCARD.2018.04.041
64. Brouwer C, Hazekamp MG, Zeppenfeld K. Anatomical substrates and ablation of reentrant atrial and ventricular tachycardias in repaired congenital heart disease. Arrhythm Electrophysiol Rev. (2016) 5:150–60. doi: 10.15420/AER.2016.19.2
65. Roca-Luque I, Rivas Gándara N, Dos Subirà L, Pascual JF, Domenech AP, Pérez-Rodon J, et al. Intra-atrial re-entrant tachycardia in congenital heart disease: types and relation of isthmus to atrial voltage. Europace. (2018) 20(2):353–61. doi: 10.1093/europace/eux250
66. Francisco-Pascual J, Santos-Ortega A, Roca-Luque I, Rivas-Gándara N, Pérez-Rodón J, Milà-Pascual L, et al. Diagnostic yield and economic assessment of a diagnostic protocol with systematic use of an external loop recorder for patients with palpitations. Rev Esp Cardiol (Engl Ed). (2019) 72:473–8. doi: 10.1016/j.rec.2018.04.007
67. Hernández-Romero D, Vílchez JA, Lahoz Á, Romero-Aniorte AI, Jover E, García-Alberola A, et al. Galectin-3 as a marker of interstitial atrial remodelling involved in atrial fibrillation. Sci Rep. (2017) 7:40378. doi: 10.1038/srep40378
68. Brouwer C, Hebe J, Lukac P, Nürnberg JH, Cosedis Nielsen J, De Riva Silva M, et al. Contemporary patients with congenital heart disease: uniform atrial tachycardia substrates allow for clear ablation endpoints with improved long-term outcome. Circ Arrhythm Electrophysiol. (2021) 14:829–40. doi: 10.1161/CIRCEP.120.009695
69. Roca-Luque I, Rivas N, Francisco J, Perez-Rodon J, Dos L, Moya A, et al. Para-Hisian atrial tachycardia ablation in a patient with congenitally corrected transposition of great vessels. HeartRhythm Case Rep. (2017) 3:340–3. doi: 10.1016/j.hrcr.2017.04.010
70. Pérez-Rodon J, Rodriguez-García J, Sarrias-Merce A, Rivas-Gandara N, Roca-Luque I, Francisco-Pascual J, et al. Predictors of acute inefficacy and the radiofrequency energy time required for cavotricuspid isthmus-dependent atrial flutter ablation. J Interv Card Electrophysiol. (2017) 49:83–91. doi: 10.1007/s10840-017-0232-z
71. Roca-Luque I, Gandara NR, Dos Subira L, Pascual JF, Pérez-Rodon J, Domenech AP, et al. Intra-atrial re-entrant tachycardia in patients with congenital heart disease: factors associated with disease severity. Europace. (2018) 20:1343–51. doi: 10.1093/EUROPACE/EUX180
72. Khairy P, Harris L, Landzberg MJ, Fernandes SM, Barlow A, Mercier LA, et al. Sudden death and defibrillators in transposition of the great arteries with intra-atrial baffles: a multicenter study. Circ Arrhythm Electrophysiol. (2008) 1(4):250–7. doi: 10.1161/CIRCEP.108.776120
73. Khairy P, Harris L, Landzberg MJ, Viswanathan S, Barlow A, Gatzoulis MA, et al. Implantable cardioverter-defibrillators in tetralogy of Fallot. Circulation. (2008) 117:363–70. doi: 10.1161/CIRCULATIONAHA.107.726372
74. Rivas-Gándara N, Francisco-Pascual J, Pijuan-Domenech A, Ribera-Solé A, Dos-Subirá L, Benito B, et al. Risk stratification of ventricular arrhythmias in repaired tetralogy of Fallot. Rev Española Cardiol (Engl Ed). (2021) 74:935–42. doi: 10.1016/j.rec.2020.12.003
75. Valente AM, Gauvreau K, Assenza GE, Babu-Narayan SV, Schreier J, Gatzoulis MA, et al. Contemporary predictors of death and sustained ventricular tachycardia in patients with repaired tetralogy of fallot enrolled in the INDICATOR cohort. Heart. (2014) 100(3):247–53. doi: 10.1136/heartjnl-2013-304958
76. Gatzoulis MA, Balaji S, Webber SA, Siu SC, Hokanson JS, Poile C, et al. Risk factors for arrhythmia and sudden cardiac death late after repair of tetralogy of Fallot: a multicentre study. Lancet. (2000) 356:975–81. doi: 10.1016/S0140-6736(00)02714-8
77. Drakopoulou M, Apostolos A, Tsioufis K, Gatzoulis K. Fragmented QRS: an electrocardiographic prognostic marker in tetralogy of Fallot. Int J Cardiol Congenit Heart Dis. (2022) 10:100422. doi: 10.1016/J.IJCCHD.2022.100422
78. Gatzoulis KA, Arsenos P, Trachanas K, Dilaveris P, Antoniou C, Tsiachris D, et al. Signal-averaged electrocardiography: past, present, and future. J Arrhythm. (2018) 34:222–9. doi: 10.1002/JOA3.12062
79. Brili S, Aggeli C, Gatzoulis K, Tzonou A, Hatzos C, Pitsavos C, et al. Echocardiographic and signal averaged ECG indices associated with non-sustained ventricular tachycardia after repair of tetralogy of Fallot. Heart. (2001) 85:57–60. doi: 10.1136/HEART.85.1.57
80. Rodríguez-García J, Pijuan-Domenech A, Francisco-Pascual J, Dos-Subirà L, Miranda-Barrio B, Santos-Ortega A, et al. Right ventricular resynchronization in a patient with repaired tetralogy of Fallot and severe ventricular dysfunction. Pacing Clin Electrophysiol. (2021) 44:2119–23. doi: 10.1111/PACE.14340
81. Vehmeijer JT, Koyak Z, Leerink JM, Zwinderman AH, Harris L, Peinado R, et al. Identification of patients at risk of sudden cardiac death in congenital heart disease: the PRospEctiVE study on implaNTable cardIOverter defibrillator therapy and suddeN cardiac death in adults with congenital heart disease (PREVENTION-ACHD). Heart Rhythm. (2021) 18:785–92. doi: 10.1016/j.hrthm.2021.01.009
82. Khairy P, Aboulhosn J, Gurvitz MZ, Opotowsky AR, Mongeon FP, Kay J, et al. Arrhythmia burden in adults with surgically repaired tetralogy of fallot: a multi-institutional study. Circulation. (2010) 122(9):868–75. doi: 10.1161/CIRCULATIONAHA.109.928481
83. Horowitz LN, Vetter VL, Harken AH, Josephson ME. Electrophysiologic characteristics of sustained ventricular tachycardia occurring after repair of tetralogy of fallot. Am J Cardiol. (1980) 46:446–52. doi: 10.1016/0002-9149(80)90014-4
84. Misaki T, Tsubota M, Watanabe G, Watanabe Y, Matumoto Y, Ishida K, et al. Surgical treatment of ventricular tachycardia after surgical repair of tetralogy of Fallot relation between intraoperative mapping and histological findings. Circulation. (1994) 90(1):264–71. doi: 10.1161/01.cir.90.1.264
85. Zeppenfeld K, Schalij MJ, Bartelings MM, Tedrow UB, Koplan BA, Soejima K, et al. Catheter ablation of ventricular tachycardia after repair of congenital heart disease: electroanatomic identification of the critical right ventricular isthmus. Circulation. (2007) 116(20):2241–52. doi: 10.1161/CIRCULATIONAHA.107.723551
86. Kapel GFL, Sacher F, Dekkers OM, Watanabe M, Blom NA, Thambo JB, et al. Arrhythmogenic anatomical isthmuses identified by electroanatomical mapping are the substrate for ventricular tachycardia in repaired tetralogy of Fallot. Eur Heart J. (2017) 38:268–76. doi: 10.1093/eurheartj/ehw202
87. Nair GM, Mbbs JS, Healey EG, Mbbs D, Morillo CAM. Case report catheter ablation of hemodynamically unstable ventricular tachycardia in a patient with dextro-transposition of the great arteries and mustard’s repair. Indian Pacing Electrophysiol J. (2011) 11(4):120–5.21760683
88. Morwood JG, Triedman JK, Berul CI, Khairy P, Alexander ME, Cecchin F, et al. Radiofrequency catheter ablation of ventricular tachycardia in children and young adults with congenital heart disease. Heart Rhythm. (2004) 1:301–8. doi: 10.1016/j.hrthm.2004.05.009
89. Harrild DM, Berul CI, Cecchin F, Geva T, Gauvreau K, Pigula F, et al. Pulmonary valve replacement in tetralogy of fallot: impact on survival and ventricular tachycardia. Circulation. (2009) 119(3):445–51. doi: 10.1161/CIRCULATIONAHA.108.775221
90. Therrien J, Siu SC, Harris L, Dore A, Niwa K, Janousek J, et al. Impact of pulmonary valve replacement on arrhythmia propensity late after repair of tetralogy of Fallot. Circulation. (2001) 103(20):2489–94. doi: 10.1161/01.cir.103.20.2489
91. Andreu D, Ortiz-Pérez JT, Fernández-Armenta J, Guiu E, Acosta J, Prat-González S, et al. 3D delayed-enhanced magnetic resonance sequences improve conducting channel delineation prior to ventricular tachycardia ablation. Europace. (2015) 17(6):938–45. doi: 10.1093/europace/euu310
92. Babu-Narayan SV, Kilner PJ, Li W, Moon JC, Goktekin O, Davlouros PA, et al. Ventricular fibrosis suggested by cardiovascular magnetic resonance in adults with repaired tetralogy of fallot and its relationship to adverse markers of clinical outcome. Circulation. (2006) 113(3):405–13. doi: 10.1161/CIRCULATIONAHA.105.548727
93. Ghonim S, Ernst S, Keegan J, Giannakidis A, Spadotto V, Voges I, et al. Circulation: arrhythmia and electrophysiology three-dimensional late gadolinium enhancement cardiovascular magnetic resonance predicts inducibility of ventricular tachycardia in adults with repaired tetralogy of Fallot. Circ Arrhythm Electrophysiol. (2020) 13:8321. doi: 10.1161/CIRCEP.119.008321
94. Gatzoulis K, Frogoudaki A, Brili S, Stefanadis C. Implantable defibrillators: from the adult cardiac to the grown up congenital heart disease patient. Int J Cardiol. (2004) 97:117–22. doi: 10.1016/J.IJCARD.2004.08.016
95. Rotes AS, Connolly HM, Warnes CA, Ammash NM, Phillips SD, Dearani JA, et al. Ventricular arrhythmia risk stratification in patients with tetralogy of Fallot at the time of pulmonary valve replacement. Circ Arrhythm Electrophysiol. (2015) 8:110–6. doi: 10.1161/CIRCEP.114.001975
96. Waldmann V, Bessiere F, Gardey K, Bakloul M, Belli E, Bonnet D, et al. Systematic electrophysiological study prior to pulmonary valve replacement in tetralogy of Fallot: a prospective multicenter study. Circ Arrhythm Electrophysiol. (2023) 16:E011745. doi: 10.1161/CIRCEP.122.011745
97. Moore JP, Aboulhosn JA, Zeppenfeld K, Waldmann V, Bessière F, Blom NA, et al. Rationale and design of the multicenter catheter ablation of ventricular tachycardia before transcatheter pulmonary valve replacement in repaired tetralogy of Fallot study. Am J Cardiol. (2023) 204:14–21. doi: 10.1016/J.AMJCARD.2023.07.087
Keywords: congenital heart disease, arrhythmias, rhythm disorders, tachycardia, sudden cardiac death
Citation: Francisco-Pascual J, Mallofré Vila N, Santos-Ortega A and Rivas-Gándara N (2024) Tachyarrhythmias in congenital heart disease. Front. Cardiovasc. Med. 11:1395210. doi: 10.3389/fcvm.2024.1395210
Received: 3 March 2024; Accepted: 20 May 2024;
Published: 3 June 2024.
Edited by:
Konstantinos Athanasios Gatzoulis, National and Kapodistrian University of Athens, GreeceReviewed by:
Vincenzo Mirco La Fazia, Texas Cardiac Arrhythmia Institute, United StatesMartin Manninger, Medical University of Graz, Austria
Despoina Ntiloudi, Tzaneio Hospital, Greece
© 2024 Francisco-Pascual, Mallofré Vila, Santos-Ortega and Rivas-Gándara. This is an open-access article distributed under the terms of the Creative Commons Attribution License (CC BY). The use, distribution or reproduction in other forums is permitted, provided the original author(s) and the copyright owner(s) are credited and that the original publication in this journal is cited, in accordance with accepted academic practice. No use, distribution or reproduction is permitted which does not comply with these terms.
*Correspondence: Jaume Francisco-Pascual, jaume.francisco@vallhebron.cat
†ORCID:
Jaume Francisco-Pascual
orcid.org/0000-0002-8841-2581