- 1Division of Cardiology, Department of Medicine, Indiana University School of Medicine, Indianapolis, IN, United States
- 2Department of Medicine, Simon Cancer Center, Indiana University School of Medicine, Indianapolis, IN, United States
- 3Regenstrief Data Services, Regenstrief Institute, Indianapolis, IN, United States
- 4School of Public Health, Indiana University Bloomington, Bloomington, IN, United States
- 5Department of Pathology, Emory School of Medicine, Atlanta, GA, United States
- 6Heart and Vascular Services, Ohio Health, Columbus, OH, United States
- 7Center for Biomedical Informatics, Regenstrief Institute, Indianapolis, IN, United States
- 8Division of Internal Medicine and Geriatrics, Department of Medicine, Indiana University School of Medicine, Indianapolis, IN, United States
Introduction: Cancer patients may have increased risk for adverse cardiac events, but our understanding of cardiovascular risk in thymic cancer patients is not clear. We sought to characterize baseline cardiometabolic risk factors before thymic cancer diagnosis and the potential association between cancer treatment and subsequent cardiac events.
Methods: This was a retrospective cohort study evaluating patients with thymic cancer from 2003 to 2020 compared to age- and sex-matched controls without cancer. Baseline cardiovascular risk factors, cancer characteristics, and incidence of cardiac events were collected from the health information exchange. Multivariable regression was used to examine the impact of cardiovascular risk factors and cancer therapies.
Results: We compared 296 patients with pathology-confirmed thymic cancer to 2,960 noncancer controls. Prior to cancer diagnosis, thymic cancer patients (TCPs) had lower prevalence of hypertension, dyslipidemia, and diabetes mellitus and similar rates of obesity, tobacco use, and pre-existing cardiovascular disease (CVD) compared to controls. After diagnosis, high-risk TCPs (>2 cardiovascular risk factors or pre-existing CVD) had higher risk for cardiac events (HR 3.73, 95% CI 2.88–4.83, p < 0.001). In the first 3 years after diagnosis, TCPs had higher incidence of cardiac events (HR 1.38, 95% CI 1.01–1.87, p = 0.042). High-risk TCPs who received radiotherapy or chemotherapy had higher risk of cardiac events (HR 4.99, 95% CI 2.30–10.81, p < 0.001; HR 6.24, 95% CI 2.84–13.72, p < 0.001).
Discussion/conclusion: Compared to noncancer controls, TCPs experienced more cardiac events when adjusted for risk factors. Patients with multiple cardiovascular risk factors receiving radiotherapy or chemotherapy had higher incidence of cardiac events.
Introduction
Although survival from thymic cancer is relatively high, not much is known about cardiovascular (CV) outcomes. Thymic cancers are rare, with 1.5 cases occurring for every million people per year (1). Five-year survival for thymoma, thymic carcinoma, and thymic neuroendocrine tumors nears 90%, 55%, and 28%–75%, respectively (2–4). A third of patients have concomitant paraneoplastic syndromes such as myasthenia gravis, but there are limited data on adverse cardiovascular events in this subpopulation (5–7). Multimodal therapy for thymic tumors includes surgical resection potentially involving cardiac structures, cytotoxic chemotherapy (e.g., anthracyclines, platinum therapies), immunotherapies, and radiotherapy (with the heart possibly in the treatment field) (8). Side effects from cancer therapies can lead to significant cardiovascular morbidity and mortality (9) through direct cardiovascular toxicity—aggravating CV risk factors or exacerbating underlying cardiovascular disease (CVD) (10–14). To date, case reports of patients with thymic cancer have observed pericardial effusion, myocarditis, and constriction (15–21). Development of CV complications after therapy is poorly understood in thymic cancer patients. This risk may be increased in patients with underlying cardiometabolic risk factors and tobacco use (22).
The goal of this study was to assess whether patients with thymic cancer had an increased incidence of CVD compared to age- and sex-matched noncancer controls. Our comparison focused on the following questions: (1) Was the cancer's presence associated with higher CV risk factors before diagnosis and higher incidence of adverse cardiac events after treatment? (2) If there was a higher incidence of CVD, did the risk of cardiac events increase with increasing CV risk factors, and was the increased risk of cardiac events associated with clinical characteristics or cancer therapies? (3) Finally, did having a simultaneous paraneoplastic syndrome lead to an increased incidence of CVD?
Methods
We conducted a retrospective analysis comparing cardiovascular outcomes in patients with a history of thymic cancer to noncancer patients from 2003 to 2020. Our referral center partnered with the Regenstrief Institute to evaluate data from the Indiana Network for Patient Care (INPC), a statewide health information exchange (23). This study received the proper ethical oversight and was approved by the Indiana University Institutional Review Board (# 2010183517). The reporting in this manuscript adheres with the STROBE guidelines (see Supplementary Material for checklist).
Data sources
Medical and pharmacy data, notes, laboratory results, and cardiovascular imaging were obtained from the Indiana University (IU) Health Data Warehouse and INPC. The INPC, managed by the Indiana Health Information Exchange, is one of the largest health information exchanges in the country (24). Over 123 separate clinical entities contribute data representing 19 million patients, billions of clinical observations, and over 300 million clinical text reports. The INPC contains data for two-thirds of Indiana's population—a proportion that rises to 80%–100% in central Indiana where the major health systems are located (25, 26). INPC data were complemented with data from the IU Health Data Warehouse, which contains structured data from IU Health's Cerner electronic health records (EHR). As of late 2022, this database captures data on 5.6 million patients, representing 16 hospitals and numerous outpatient clinics.
Data collection
Eligible patients over the age of 35 at the time of thymic cancer diagnosis were identified by ICD-9 (164.0, 212.6) and ICD-10 (C37, D15.0) codes for thymoma, thymic carcinoma, and thymic neuroendocrine tumor (Figure 1; see Supplementary Material for the complete ICD codes list). Patients with fewer than 2 ICD diagnoses of thymic tumor in the EHR were excluded, and data were collected for two years prior to the index date. We identified 1,212 patients with thymic tumors from January 1, 2003, to December 31, 2020. Selecting patients residing in Indiana or a neighboring state allowed for closer follow-up and ensured adequate data collection but reduced the number to 680. Cancer characteristics were extracted from EHR data, including WHO staging, Masaoka staging, size of tumor, cancer therapies, and presence of a paraneoplastic syndrome. The tumor size was based on a CT scan at the time of diagnosis or final pathologic evaluation. Cardiovascular risk factors evaluated included diabetes mellitus, hypertension, dyslipidemia, obesity classified as BMI >30, and a history of tobacco use. Patients were noted to have pre-existing coronary disease if they had a diagnosis of coronary atherosclerosis/calcification from a CT scan or coronary angiogram within 1 year of thymic cancer diagnosis. Cardiac events included heart failure (including preserved and reduced ejection fraction), myocardial infarction or ischemic heart disease, stroke or transient ischemic attack, and atrial fibrillation. Patient mortality was ascertained from hospital discharge summaries and the Indiana State Department of Health's death certificate files.
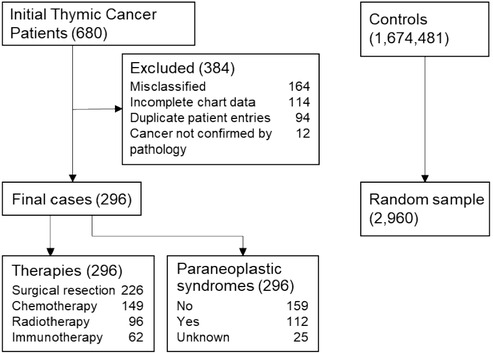
Figure 1. Consort diagram of thymic cancer patients and controls. There were initially 680 patient charts reviewed from which 296 patients were ultimately included for the final analysis. They were age-, sex- matched in a 1:10 fashion to 2,960 controls for prevalence of cardiac risk factors and cardiac outcomes. Cancer patients were analyzed for cardiac events based on their cancer therapies and presence or absence of a paraneoplastic syndrome.
All 680 patient records were individually reviewed by the first author (AK). Records were ascertained for the accuracy of diagnoses and cancer characteristics based on pathology data, imaging, and oncology notes. The list was cross-referenced with a separate internal pathologic database of patients from 2010 to 2020 (n = 155, 52.36%) to confirm thymic neoplasm. To verify the accuracy of the chart review, a blinded reviewer (SCC) was given records of 20/680 patients, half of whom had tissue biopsy confirming diagnosis and half did not, and accurately verified 19/20 (95.0%) of the diagnoses. Unclear diagnoses (n = 22) were adjudicated by two authors (PJL, SB) based on pathologic data. Cardiovascular risk factors and outcomes were captured from various settings including inpatient and outpatient clinical encounters, and at different Indiana institutions through the INPC. Although there were cancer patients from neighboring states, they continued to receive subspecialty care at the Simon Cancer Center years after their cancer diagnosis, providing reliable estimates of long-term outcomes.
A control cohort consisted of patients meeting the following criteria: no prior cancer diagnoses and at least 2 years of clinical data in the system prior to an encounter occurring the same year as a thymic cancer patient (TCP) index date. The study index date of a cancer patient was defined as the date of the first documented diagnosis of thymic neoplasm. The index date of a control patient was the first visit date in the same calendar year as the index date of the matched patient. Controls were randomly selected and were age and sex-matched to TCPs at a 10:1 ratio. Data extraction was repeated for the control cohort.
Statistical analysis
Demographic and clinical characteristics of both cohorts are presented using the mean, standard deviation, or count unless otherwise specified, and between-group comparisons used the unpaired t-test or chi-squared test, respectively. Incident events (cardiac or death) were observed from the index date and censored at the last recorded encounter at IU Health. These events could be predicted by the multiple CV risks at baseline, which were then grouped into three risk levels (low, medium, high) to streamline subsequent analyses (see Supplementary Material Table S5). Time-to-event comparisons between groups were graphed using Kaplan-Meier curves and analyzed using causal-specific Cox regression (results presented as hazard ratio [HR] with 95% confidence interval [CI]), adjusted for age, sex, race, and the 3 CV risk levels. The cumulative incidence plots (Figure 3) showed potential change-points of the proportional hazards at around three years and 7 years. With the data censored at 7 years due to insufficient number of later events, the model with time-dependent hazards (with change-point at three years) showed significantly better fit than the constant hazards model (p < 0.025).
Results
Cancer characteristics
Of the 1,212 patients identified with a thymic cancer diagnosis, 680 met the inclusion criteria. After excluding patients misclassified as having thymic cancer, duplicate entries from different health systems, patients with insufficient follow-up data, and patients without pathology-confirmed thymic cancer, the final cohorts consisted of 296 TCPs and 2,960 noncancer controls (Figure 1). Most TCPs had thymoma (74.0%), followed by thymic carcinoma (22.3%) and thymic neuroendocrine tumor (3.7%) (Table 1). Of 271 TCPs with Masaoka staging, 17.9%, 20.3%, 12.8%, and 40.5% were in stages 1–4, respectively. Paraneoplastic syndromes were present in 112 (37.8%) TCPs and 72 (24.3%) had myasthenia gravis. Treatments included surgical resection (76.4%), chemotherapy (50.3%) with 98 (33.1%) receiving anthracyclines, chest radiotherapy (32.4%), and immunotherapy (21.0%) (Figure 2).
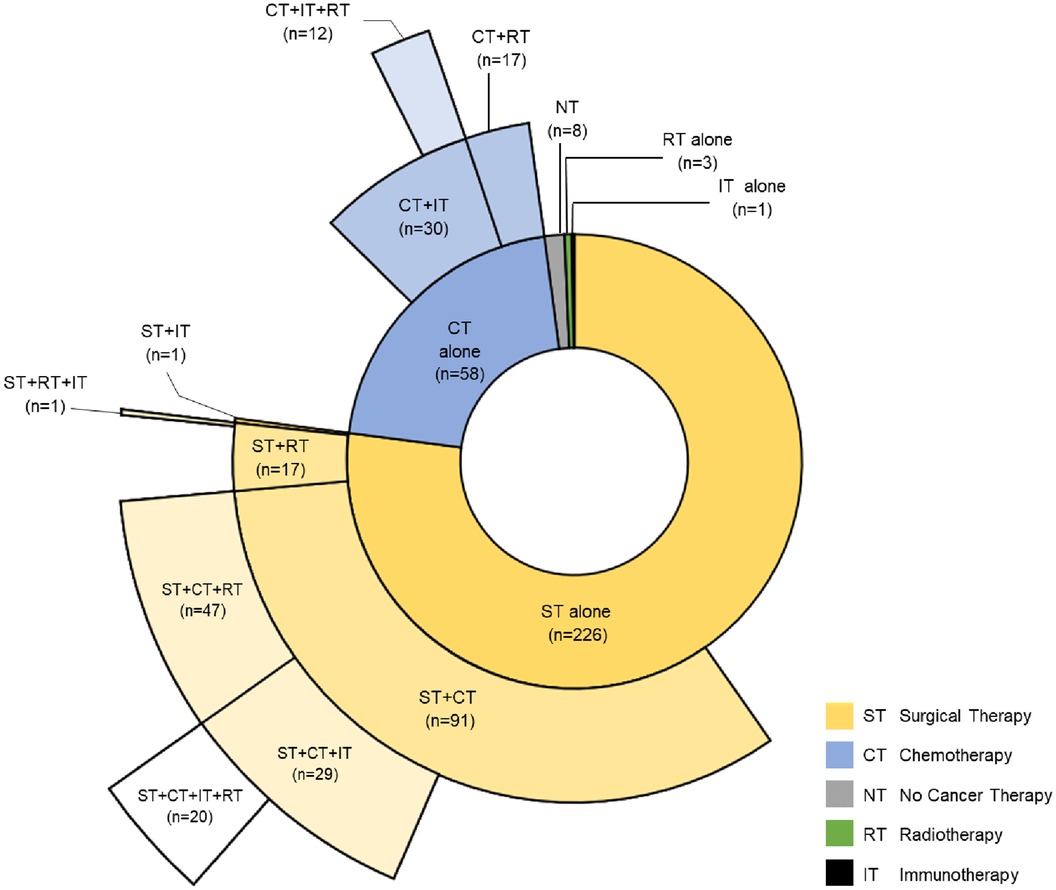
Figure 2. Distribution of cancer therapies for cohort of patients with cancer. Sunburst diagram depicting the type of cancer therapies and their combinations thymic cancer patients received.
Baseline characteristics of thymic cancer patients and controls
Age and sex distributions were similar for TCPs and controls (due to matching), and both groups were about 82% White (Table 2). Of the 296 patients, 198 (67%) were in Indiana and 98 (33%) were from neighboring states.
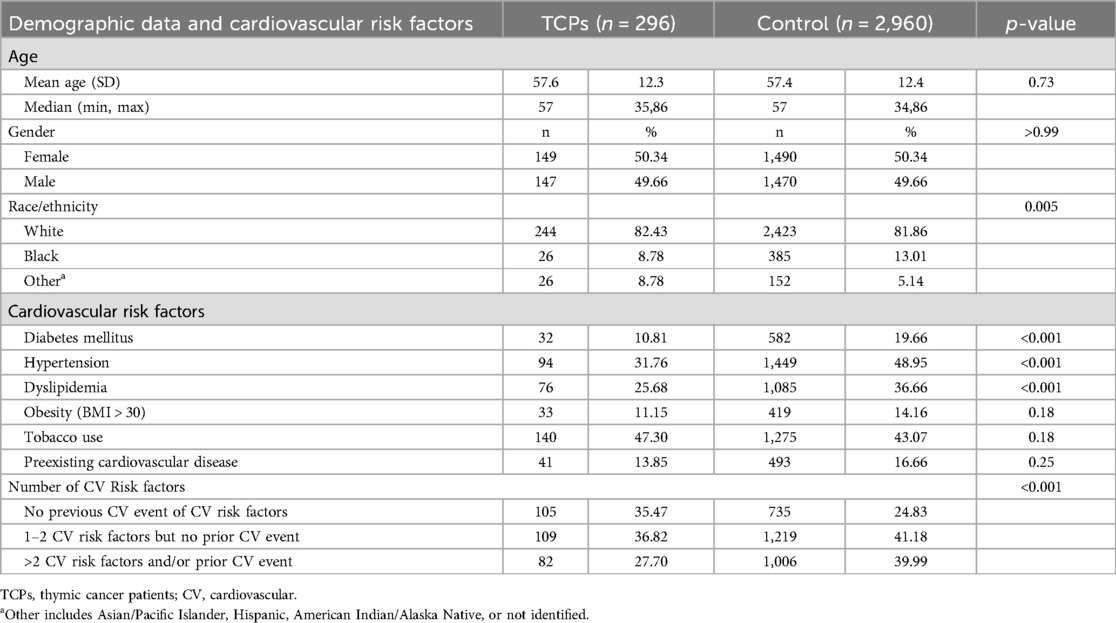
Table 2. Demographic data and cardiovascular risk factors of thymic cancer patients and patients without cancer (control group).
Cardiovascular risk factors and diseases before diagnosis
Compared to controls, TCPs had similar rates of baseline obesity, tobacco use, and pre-existing CVD but significantly lower rates of hypertension, dyslipidemia, and diabetes mellitus (Table 2) and lower total number of CV risk factors per person. Preliminary analyses of TCPs showed these risk factors were predictive of subsequent cardiac events, and TCPs could be grouped into three levels of overall CV risk: 1) low-risk (no risk factors or pre-existing CVD); 2) medium risk (1–2 risk factors and no pre-existing CVD); and 3) high risk (>2 risk factors and/or pre-existing CVD).
Cardiac events after cancer diagnosis
Over a mean follow-up period of 4.7 years (median = 4.4, range 0.5–16 years), the following events occurred: 31 TCPs and 346 controls developed heart failure (10.5% vs. 11.7%); 33 TCPs and 286 controls developed atrial fibrillation/flutter (11.2% vs. 9.7%); 14 TCPs and 195 controls had a stroke (4.7% vs. 6.6%); 15 TCPs and 259 controls had an ischemic event/myocardial infarction (5.1% vs. 8.8%); 63 TCPs and 686 controls had any cardiac event (21.3% vs. 23.2%); and 19 TCPs and 91 controls died (6.4% vs. 3.1%) (see Supplementary Material Table S5).
Prediction of incident cardiac events in thymic cancer patients and controls
The Kaplan-Meier curves (Figure 3) show that within TCPs and within noncancer controls, the incidence of CV events increases with the levels of baseline CV risk (low, medium, high) and within each risk level, the incidence of CV events was higher in TCPs than in controls. Results of the final time-dependent proportional hazards model showed that higher-incident cardiac events could be predicted by increasing age (HR = 1.05 per year) and male sex (HR = 1.37 relative to female) (Table 3). The 3 CV risk levels at baseline were also strongly predictive of the incidence of cardiac events, with HR of 1.45 (95% CI 1.11–1.90, p = 0.007) for medium risk and 3.73 (95% CI 2.88–4.83, p < 0.001) for high relative to low risk. The hazard ratios of TCPs relative to controls were 1.38 (95% CI 1.01–1.87, p = 0.042) in years 0–3 and 0.77 (95% CI 0.47–1.25, p = 0.29) in years 4–7.
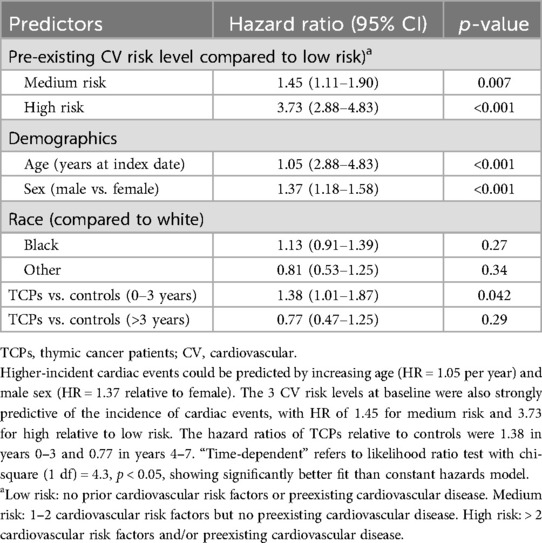
Table 3. Cox regression comparing thymic cancer patients (TCPs) to controls with time-dependent hazard ratios of any cardiac event as the outcome.
CVD outcomes as related to treatments in thymic cancer patients
To assess the validity of our data source and approach, we evaluated the known association of heart failure with anthracycline therapy in our dataset. In our cancer cohort (12 heart failures among 98 anthracycline-treated patients and 19 heart failures among 198 non-treated patients), anthracycline-treated patients had significantly higher overall heart failure events (HR = 2.77, 95% CI 1.26–6.06, p = 0.011) after controlling for demographics and baseline CV risk. Among classes of cancer therapies, immunotherapy showed no significant association with cardiac events among the cancer patients with time-dependent analysis. The effects of radiotherapy and chemotherapy were time-dependent. There was a significantly higher incidence of any cardiac event with radiotherapy and chemotherapy treatments compared to untreated cancer patients, but this difference did not manifest until after the first three years (Table 4). The data were too limited to investigate the effects of treatment combinations.
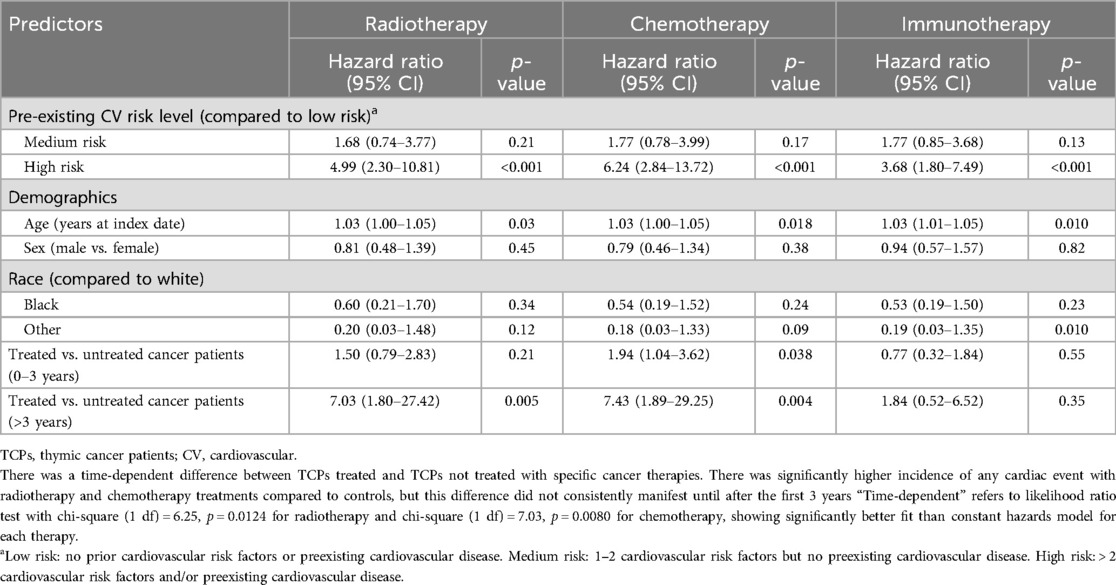
Table 4. Cox regression with time-dependent hazard ratios for any cardiac event, halted at 7 years, comparing cancer patients that received the specific cancer therapy to cancer patients who did not receive the therapy.
Secondary analyses comparing TCPs with and without a paraneoplastic process after cancer diagnosis showed no significant difference in adverse CVD or death (HR 0.71, 95% CI 0.41–1.23, p = 0.22; HR 1.24, 95% CI 0.5–3.09, p = 0.64).
Discussion
The results of this single-center, retrospective cohort study indicate that patients with thymic neoplasms may have had a lower prevalence of certain cardiovascular risk factors compared to non-cancer controls before their cancer diagnosis. This unexpected finding, along with the increased risk of CVD within the first three years after thymic cancer diagnosis, suggests there may be underlying mechanisms in thymic cancer that accelerate cardiac disease, warranting further investigation. This potential discrepancy could be attributed to incomplete data collection before the diagnosis of thymic cancer, possibly influenced by referral bias. We hope to compare these findings of lower baseline cardiovascular risk factors in patients with thymic cancer to a larger multicenter database to assess their validity. It is important to note that TCPs had a similar incidence of cardiac events compared to controls during follow-up after their cancer therapies. When adjusted for age, sex, race, and number of CV risk factors, the risk for any cardiac event was significantly higher in the cancer cohort. After grouping TCPs into three risk strata based on CV risk factors and pre-existing disease, we found an additive risk for cancer patients between the number of risk factors and incident cardiac events. In the three years after diagnosis, TCPs had a higher incidence of cardiac events than controls, regardless of baseline risk factors or cancer therapy. After three years, TCPs who received radiotherapy or chemotherapy had a higher incidence of cardiac events compared to untreated TCPs.
We did not match cohorts based on CV risk factors due to the exploratory nature of the study. By not matching, in this limited dataset, we observed that TCPs had a lower total number of baseline risk factors than matched controls in this cohort. In high-risk patients with >2 underlying risk factors or pre-existing CVD, the risk of heart failure with anthracyclines was significantly higher. Similarly, in patients receiving radiotherapy or any chemotherapy, the risk of any cardiac event was significantly higher in high-risk cancer patients. The finding of a higher risk of cardiac events in TCPs in the first three years compared to controls is interesting. TCPs may have had pre-existing heart disease that went unnoticed before their cancer diagnosis, or they may have undergone surgery impacting cardiac structures, resulting in subsequent injury or arrhythmias. A less probable scenario to consider is that some TCPs with a paraneoplastic syndrome might have experienced myocarditis, although a prior review found this to be uncommon (27). In our study, there was one TCP with myocarditis. This occurrence may be attributed to the involvement of inflammatory cytokines implicated in cardiac events in cancer patients which may have contributed (28). While there is an apparent indication of increased CVD in the initial three years for TCPs, this might be attributed to intensified monitoring because cardiac events resulting from radiotherapy and chemotherapy typically require time to manifest. As patients undergo cancer therapy, they may experience multiple CV stressors in combination with lifestyle factors and metabolic dyscrasias, potentially contributing to the development of CVD and premature mortality (29).
Our study adds important information to the current paradigms regarding cardiac events in patients with thymic neoplasms. The increased prevalence of cardiac events is observed not only in thymic cancer patients but also in those with other types of cancer as demonstrated by several studies. Armenian et al. (14) found that patients with multiple myeloma, non-Hodgkin lymphoma, kidney cancer, and breast cancer have significantly higher incidence of CVD when compared to noncancer controls. Other studies have similarly reported an elevated risk of CVD in patients with cancers sharing characteristics with thymic cancer, namely a thoracic location and similar therapeutic regimens with chemotherapy (e.g., anthracyclines) and radiation with the heart in the treatment field. These malignancies include lung cancer (12), breast cancer (13), and mediastinal lymphomas (30, 31). CVD and lung cancer share common risk factors, including tobacco use and inflammation, and pre-existing CVD before cancer therapy portends a worse prognosis (12). Complications from cancer therapies for thoracic tumors include hypertension, coronary disease, myocarditis, and arrhythmias. Certain chemotherapies such as cyclophosphamide may not immediately lead to cardiotoxicity but may activate endothelial dysfunction and trigger long term cardiac complications (32, 33). Furthermore, for these other thoracic cancers, the risk increases based on several baseline risk factors, total heart dose of radiotherapy, and additive effects from multiple therapies such as anthracyclines and other cardiotoxic agents (34–36). However, ours is the first, relatively large, comprehensive study evaluating CV risk factors and CVD in patients with pathology-confirmed thymic cancers. In a study by Okereke et al., 11% of patients with recent diagnoses of thymomas had a known history of cardiac disease—similar to our own (13.9%), although in their study, stroke was not included (37). In a smaller study by Liao et al., postoperative radiotherapy improved survival from thymomas but increased the risk of cardiac events in long-term survivors (38).
The mechanism for why TCPs had an increased incidence of cardiac events is not entirely clear. We speculate that potential cardiotoxic treatments lead to or exacerbate underlying CVD, especially in the presence of multiple CV risk factors. Almost half of the patients had a history of tobacco use, and a substantial number had hypertension, diabetes, and dyslipidemia. Chemotherapy and radiotherapy may lead to changes in blood pressure, metabolic factors, and increased inflammation (39–42). Patients receiving left-sided radiotherapy are at increased risk for developing coronary disease, valvulopathies, and cardiac death (43). Immunotherapy-related adverse events have been observed, with myocarditis being the most notable although other reported toxicities include cardiomyopathy, conduction defects, and pericarditis. Long-term complications and the value of screening and surveillance approaches for immunotherapy patients have not been delineated (44). There are several clinical implications from our data in the care of thymic patients: TCPs may benefit from more aggressive control of baseline CV risk factors prior to and during cancer treatments. There may be value in providing cardiac evaluation prior to treatment and ongoing cardiac monitoring, especially in high-risk patients receiving chemotherapy or radiotherapy. In high-risk patients, oncologists may avoid adjuvant therapy if there is minimal impact on cancer survival. These changes may lead to improved morbidity and mortality in TCPs.
Our study's strengths include having the largest cohort of pathology-confirmed TCPs for evaluation of CVD to date and using a statewide health information exchange to obtain comprehensive data. Our study is the first to evaluate baseline CV risk factors and appraise subsequent cardiac events. Previous studies have had smaller patient populations with shorter follow-ups and did not account for cardiometabolic risk factors. Given the paucity of comprehensive data in the field and that ours is a single-center, observational study, we advocate for a larger multicenter to validate our findings, including the timing of cardiac events. A prospective study evaluating the benefit of cardiac evaluation and preemptive interventions for cardiovascular risk factors prior to therapy may help determine benefits for CVD and survival.
Limitations
Our study had several limitations. It featured a single quaternary referral center's experience and included many patients with advanced-stage tumors. This may have skewed the number of cardiac events. Less complete historical data from referral patients may have biased our results. There may have been bias based on the geographical distribution of referrals as rural areas tend to provide less data to the INPC. We may have limited data prior to the index date and missed pre-existing conditions. Selection bias may have resulted from evaluating only patients over 35 and limiting the cohort to patients from Indiana or a neighboring state. By limiting the cohort geographically, we aimed to obtain comparatively more complete data with longitudinal follow-up due to the high penetration of our health information exchange. To ensure accuracy, we minimized potential recall and misclassification biases using both ICD coding and physician review of EHR data. We strove to have accurate diagnoses by only including patients with pathologic diagnoses of thymic neoplasm and confirmed with a subset of patients with a second pathology database. This led to smaller numbers of patients in the study, decreasing the power for multivariable analyses. Patients with thymic cancers in our institution have not traditionally had extensive cardiac workups prior to initiating treatment or undergoing surgical resection, which may have led to underestimation of baseline CVD. We could not separate exposure from treatments, such as concurrent chemotherapy and immunotherapy, to analyze cardiac events which likely led to confounding. We had similar distribution of thymic cancer subtypes and similar incidence of cardiac events compared to the published literature. Our subanalysis for heart failure in TCPs who received anthracyclines also reinforces validity. Since this was an exploratory study, we did not perform propensity score matching. We cannot make causal inferences but were able to highlight associations between baseline CV risk factors and higher incidence of adverse CVD in TCPs.
Conclusion
In our study, TCPs exhibited cardiac event rates comparable to controls. Stratification by baseline cardiovascular risk factors revealed an additive risk for cardiac events in TCPs. High-risk patients undergoing radiotherapy or chemotherapy experienced worse cardiac outcomes, underscoring the necessity for strategies to mitigate cardiovascular risk. Further studies are warranted to better understand specific associations contributing to increased cardiovascular events.
Data availability statement
The raw deidentified data supporting the conclusions of this article will be made available by the authors, without undue reservation.
Ethics statement
The studies involving humans were approved by this study received the proper ethical oversight and was approved by the Indiana University Institutional Review Board (# 2010183517). The studies were conducted in accordance with the local legislation and institutional requirements. Written informed consent for participation was not required from the participants or the participants’ legal guardians/next of kin in accordance with the national legislation and institutional requirements.
Author contributions
AK: Conceptualization, Formal Analysis, Funding acquisition, Investigation, Methodology, Project administration, Validation, Visualization, Writing – original draft, Writing – review & editing. SC: Conceptualization, Formal Analysis, Investigation, Methodology, Validation, Visualization, Writing – original draft, Writing – review & editing. PL: Conceptualization, Funding acquisition, Investigation, Methodology, Writing – review & editing. AR: Data curation, Formal Analysis, Writing – review & editing. LG-R: Data curation, Formal Analysis, Writing – review & editing. SB: Investigation, Methodology, Writing – review & editing. SR: Investigation, Methodology, Writing – review & editing. SH: Conceptualization, Formal Analysis, Investigation, Methodology, Validation, Visualization, Writing – original draft, Writing – review & editing. TS: Conceptualization, Formal Analysis, Funding acquisition, Investigation, Methodology, Project administration, Validation, Visualization, Writing – original draft, Writing – review & editing.
Funding
The author(s) declare financial support was received for the research, authorship, and/or publication of this article. This project was funded in part by the Advanced Scholars Program for Internists in Research and Education (ASPIRE). This project was made possible, in part, by support from the Indiana Clinical and Translational Sciences Institute (funded in part by Award Number UL1TR002529 from the National Institutes of Health, National Center for Advancing Translational Sciences) Clinical and Translational Sciences Award and the Lilly Endowment Inc. Physician Scientist Initiative.
Acknowledgments
Nicholas Berg (data extraction), Giorgos Bakoyannis and Huiping Xu (time-dependent hazards model), Thomas Maatman (graphic design), Lynn Whitaker (editing).
Conflict of interest
The authors declare that the research was conducted in the absence of any commercial or financial relationships that could be construed as a potential conflict of interest.
Publisher's note
All claims expressed in this article are solely those of the authors and do not necessarily represent those of their affiliated organizations, or those of the publisher, the editors and the reviewers. Any product that may be evaluated in this article, or claim that may be made by its manufacturer, is not guaranteed or endorsed by the publisher.
Supplementary material
The Supplementary Material for this article can be found online at: https://www.frontiersin.org/articles/10.3389/fcvm.2024.1393631/full#supplementary-material
References
1. The American Cancer Society Medical and Editorial Content Team. Key Statistics About Thymus Cancers. Cancer.Org. (2021). Available online at: https://www.cancer.org/cancer/thymus-cancer/about/key-statistics.html (Accessed June 8, 2022). #:∼:text=Although thymic tumors are the,each year is not known
2. Masaoka A. Staging system of thymoma. J Thorac Oncol. (2010) 5(10 Suppl 4):S304–12. doi: 10.1097/JTO.0b013e3181f20c05
3. Weksler B, Dhupar R, Parikh V, Nason KS, Pennathur A, Ferson PF. Thymic carcinoma: a multivariate analysis of factors predictive of survival in 290 patients. Ann Thorac Surg. (2013) 95(1):299–303. doi: 10.1016/j.athoracsur.2012.09.006
4. Kondo K, Monden Y. Therapy for thymic epithelial tumors: a clinical study of 1,320 patients from Japan. Ann Thorac Surg. (2003) 76(3):875–8. doi: 10.1016/s0003-4975(03)00555-1
5. Blum TG, Misch D, Kollmeier J, Thiel S, Bauer TT. Autoimmune disorders and paraneoplastic syndromes in thymoma. J Thorac Dis. (2020) 12(12):7571–90. doi: 10.21037/jtd-2019-thym-10
6. Shivamurthy P, Parker MW. Cardiac manifestations of myasthenia gravis: a systematic review. IJC Metab Endocr. (2014) 5:3–6. doi: 10.1016/j.ijcme.2014.08.003
7. Evoli A, Lancaster E. Paraneoplastic disorders in thymoma patients. J Thorac Oncol. (2014) 9(9 Suppl 2):S143–7. doi: 10.1097/JTO.0000000000000300
8. Turna A, Sarbay İ. Multimodality approach in treatment of thymic tumors. J Thorac Dis. (2020) 12(12):7626–34. doi: 10.21037/jtd-20-818
9. Anker MS, von Haehling S, Landmesser U, Coats AJS, Anker SD. Cancer and heart failure, more than meets the eye: common risk factors and co-morbidities. Eur J Heart Fail. (2018) 20(10):1382–4. doi: 10.1002/ejhf.1252
10. Koene RJ, Prizment AE, Blaes A, Konety SH. Shared risk factors in cardiovascular disease and cancer. Circulation. (2016) 133(11):1104–14. doi: 10.1161/CIRCULATIONAHA.115.020406
11. Ponikowski P, Voors AA, Anker SD, Bueno H, Cleland JGF, Coats AJS, et al. 2016 ESC guidelines for the diagnosis and treatment of acute and chronic heart failure: the task force for the diagnosis and treatment of acute and chronic heart failure of the European Society of Cardiology. Eur J Heart Fail. (2016) 18(8):891–975. doi: 10.1002/ejhf.592
12. Sase K, Fujisaka Y, Shoji M, Mukai M. Cardiovascular complications associated with contemporary lung cancer treatments. Curr Treat Options Oncol. (2021) 22(8):71. doi: 10.1007/s11864-021-00869-6
13. Mehta LS, Watson KE, Barac A, Beckie TM, Bittner V, Cruz-Flores S, et al. Cardiovascular disease and breast cancer: where these entities intersect—a scientific statement from the American Heart Association. Circulation. (2018) 137(8):e30–66. doi: 10.1161/CIR.0000000000000556
14. Armenian SH, Xu L, Ky B, Sun C, Farol LT, Pal SK, et al. Cardiovascular disease among survivors of adult-onset cancer: a community-based retrospective cohort study. J Clin Oncol. (2016) 34(10):1122–30. doi: 10.1200/JCO.2015.64.0409
15. Moady G, Daud E, Galilee R, Sabo E, Mazareb S, Atar S. Pericardial effusion in a young man: a rare presentation of thymoma. Isr Med Assoc J. (2020) 22(5):328–9.32378828
16. Finsterer J, Stöllberger C, Ho CY. Respiratory insufficiency from myasthenia gravis and polymyositis due to malignant thymoma triggering takotsubo syndrome. Int J Neurosci. (2018) 128(12):1207–10. doi: 10.1080/00207454.2018.1486830
17. Priemer DS, Davidson DD, Loehrer PJ, Badve SS. Giant cell polymyositis and myocarditis in a patient with thymoma and myasthenia gravis: a postviral autoimmune process? J Neuropathol Exp Neurol. (2018) 77(8):661–4. doi: 10.1093/jnen/nly041
18. Tsimpinos MD, Kanakis MA, Theodorou SN, Misthos PA, Lioulias AG. Thymoma in the middle mediastinum with blood supply from the left main coronary artery. Ann Thorac Surg. (2017) 103(6):e561. doi: 10.1016/j.athoracsur.2017.01.032
19. De Giacomo T, Patella M, Mazzesi G, Venuta F. Successful resection of thymoma directly invading the right atrium under cardiopulmonary bypass. Eur J Cardiothorac Surg. (2015) 48(2):332–3. doi: 10.1093/ejcts/ezu376
20. Hikosaka Y, Yano M, Otsuka S, Sasaki H, Moriyama S, Okuda K, et al. Post-irradiation constrictive pericarditis following thymoma treatment: a report of two cases. Gen Thorac Cardiovasc Surg. (2015) 63(7):413–5. doi: 10.1007/s11748-013-0302-9
21. Thomas A, Shanbhag S, Haglund K, Berman A, Jakopovic M, Szabo E, et al. Characterization and management of cardiac involvement of thymic epithelial tumors. J Thorac Oncol. (2013) 8(2):246–9. doi: 10.1097/JTO.0b013e31827bd931
22. Simon MS, Beebe-Dimmer JL, Hastert TA, Manson JE, Cespedes Feliciano EM, Neuhouser ML, et al. Cardiometabolic risk factors and survival after breast cancer in the women’s health initiative. Cancer. (2018) 124(8):1798–807. doi: 10.1002/cncr.31230
23. Overhage JM, Kansky JP. The Indiana health information exchange. In: Dixon BE, editor. Health Information Exchange: Navigating and Managing a Network of Health Information Systems. 2nd ed. Academic Press (2023). p. 471–87. doi: 10.1016/B978-0-323-90802-3.00022-8
24. Dixon BE, Whipple EC, Lajiness JM, Murray MD. Utilizing an integrated infrastructure for outcomes research: a systematic review. Health Info Libr J. (2016) 33(1):7–32. doi: 10.1111/hir.12127
25. Ruppert LP, He J, Martin J, Eckert G, Ouyang F, Church A, et al. Linkage of Indiana state cancer registry and Indiana network for patient care data. J Registry Manag. (2016) 43(4):174–8.29595920
26. McDonald CJ, Overhage JM, Barnes M, Schadow G, Blevins L, Dexter PR, et al. The Indiana network for patient care: a working local health information infrastructure. An example of a working infrastructure collaboration that links data from five health systems and hundreds of millions of entries. Health Aff (Millwood). (2005) 24(5):1214–20. doi: 10.1377/hlthaff.24.5.1214
27. Wakafuji S, Okada R. Twenty year autopsy statistics of myocarditis incidence in Japan. Jpn Circ J. (1986) 50(12):1288–93. doi: 10.1253/jcj.50.1288
28. Amin MN, Siddiqui SA, Ibrahim M, Hakim ML, Ahammed MS, Kabir A, et al. Inflammatory cytokines in the pathogenesis of cardiovascular disease and cancer. SAGE Open Med. (2020) 8:2050312120965752. doi: 10.1177/2050312120965752
29. Jones LW, Haykowsky MJ, Swartz JJ, Douglas PS, Mackey JR. Early breast cancer therapy and cardiovascular injury. J Am Coll Cardiol. (2007) 50(15):1435–41. doi: 10.1016/j.jacc.2007.06.037
30. van Nimwegen FA, Schaapveld M, Janus CP, Krol AD, Petersen EJ, Raemaekers JM, et al. Cardiovascular disease after hodgkin lymphoma treatment: 40-year disease risk. JAMA Intern Med. (2015) 175(6):1007–17. doi: 10.1001/jamainternmed.2015.1180
31. Stone CR, Mickle AT, Boyne DJ, Mohamed A, Rabi DM, Brenner DR, et al. Treatment for lymphoma and late cardiovascular disease risk: a systematic review and meta-analysis. Health Sci Rep. (2019) 2(10):e135. Published 2019 August 13. doi: 10.1002/hsr2.135
32. Herrmann J, Yang EH, Iliescu CA, Cilingiroglu M, Charitakis K, Hakeem A, et al. Vascular toxicities of cancer therapies: the old and the new–an evolving avenue. Circulation. (2016) 133(13):1272–89. doi: 10.1161/CIRCULATIONAHA.115.018347
33. Lenneman CG, Sawyer DB. Cardio-oncology: an update on cardiotoxicity of cancer-related treatment. Circ Res. (2016) 118(6):1008–20. doi: 10.1161/CIRCRESAHA.115.303633
34. Rahman F, Ko D, Benjamin EJ. Association of atrial fibrillation and cancer. JAMA Cardiol. (2016) 1(4):384–6. doi: 10.1001/jamacardio.2016.0582
35. Conen D, Wong JA, Sandhu RK, Cook NR, Lee IM, Buring JE, et al. Risk of malignant cancer among women with new-onset atrial fibrillation. JAMA Cardiol. (2016) 1(4):389–96. doi: 10.1001/jamacardio.2016.0280
36. Kattelus H, Kesäniemi YA, Huikuri H, Ukkola O. Cancer increases the risk of atrial fibrillation during long-term follow-up (OPERA study). PLoS One. (2018) 13(10):e0205454. doi: 10.1371/journal.pone.0205454
37. Okereke IC, Kesler KA, Morad MH, Mi D, Rieger KM, Birdas TJ, et al. Prognostic indicators after surgery for thymoma. Ann Thorac Surg. (2010) 89(4):1071–9. doi: 10.1016/j.athoracsur.2010.01.026
38. Liao J, Liu T, Zhang H, Cai F, Chen J, Dang J. The role of postoperative radiation therapy for completely resected stage III thymoma and effect of higher heart radiation dose on risk of cardiovascular disease: a retrospective cohort study. Int J Surg. (2018) 53(2018):345–9. doi: 10.1016/j.ijsu.2018.04.018
39. Chang HM, Okwuosa TM, Scarabelli T, Moudgil R, Yeh ETH. Cardiovascular complications of cancer therapy: best practices in diagnosis, prevention, and management, part 2. J Am Coll Cardiol. (2017) 70(20):2552–65. doi: 10.1016/j.jacc.2017.09.1095
40. Chang HM, Moudgil R, Scarabelli T, Okwuosa TM, Yeh ETH. Cardiovascular complications of cancer therapy: best practices in diagnosis, prevention, and management, part 1. J Am Coll Cardiol. (2017) 70(20):2536–51. doi: 10.1016/j.jacc.2017.09.1096
41. Taunk NK, Haffty BG, Kostis JB, Goyal S. Radiation-induced heart disease: pathologic abnormalities and putative mechanisms. Front Oncol. (2015) 5:39. doi: 10.3389/fonc.2015.00039
42. Belzile-Dugas E, Eisenberg MJ. Radiation-induced cardiovascular disease: review of an underrecognized pathology. J Am Heart Assoc. (2021) 10(18):e021686. doi: 10.1161/JAHA.121.021686
43. Cheng YJ, Nie XY, Ji CC, Lin XX, Liu LJ, Chen XM, et al. Long-Term cardiovascular risk after radiotherapy in women with breast cancer. J Am Heart Assoc. (2017) 6(5):e005633. Published 2017 May 21. doi: 10.1161/JAHA.117.005633
Keywords: thymic cancer, cancer survivorship, cardiovascular disease, electronic health records, thoracic oncology, cardiovascular risk factors
Citation: Khemka A, Clasen SC, Loehrer PJ, Roberts AR, Golzarri-Arroyo L, Badve SS, Raman SV, Hui SL and Schleyer TKL (2024) Cardiovascular disease in thymic cancer patients. Front. Cardiovasc. Med. 11:1393631. doi: 10.3389/fcvm.2024.1393631
Received: 29 February 2024; Accepted: 26 August 2024;
Published: 10 September 2024.
Edited by:
John David Horowitz, University of Adelaide, AustraliaReviewed by:
Philipp Stroebel, University Medical Center Göttingen, GermanyEwa Krystyna Szczepanska-Sadowska, Medical University of Warsaw, Poland
Copyright: © 2024 Khemka, Clasen, Loehrer, Roberts, Golzarri-Arroyo, Badve, Raman, Hui and Schleyer. This is an open-access article distributed under the terms of the Creative Commons Attribution License (CC BY). The use, distribution or reproduction in other forums is permitted, provided the original author(s) and the copyright owner(s) are credited and that the original publication in this journal is cited, in accordance with accepted academic practice. No use, distribution or reproduction is permitted which does not comply with these terms.
*Correspondence: Abhishek Khemka, YWtoZW1rYUBpdS5lZHU=
†These authors have contributed equally to this work