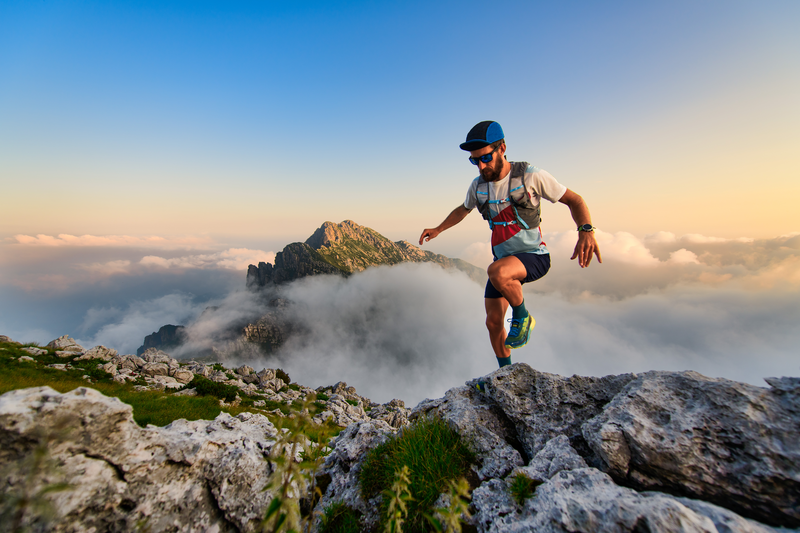
95% of researchers rate our articles as excellent or good
Learn more about the work of our research integrity team to safeguard the quality of each article we publish.
Find out more
REVIEW article
Front. Cardiovasc. Med. , 27 August 2024
Sec. Precision Cardiology
Volume 11 - 2024 | https://doi.org/10.3389/fcvm.2024.1380639
This article is part of the Research Topic Personalized Physical Activity and Exercise in Preventive Cardiology View all 5 articles
Optimizing endurance exercise intensity prescription is crucial to maximize the clinical benefits and minimize complications for individuals at risk for or with cardiovascular disease (CVD). However, standardization remains incomplete due to variations in clinical guidelines. This review provides a practical and updated guide for health professionals on how to prescribe endurance exercise intensity for cardiovascular rehabilitation (CR) populations, addressing international guidelines, practical applicability across diverse clinical settings and resource availabilities. In the context of CR, cardiopulmonary exercise test (CPET) is considered the gold standard assessment, and prescription based on ventilatory thresholds (VTs) is the preferable methodology. In settings where this approach isn't accessible, which is frequently the case in low-resource environments, approximating VTs involves combining objective assessments—ideally, exercise tests without gas exchange analyses, but at least alternative functional tests like the 6-minute walk test—with subjective methods for adjusting prescriptions, such as Borg's ratings of perceived exertion and the Talk Test. Therefore, enhancing exercise intensity prescription and offering personalized physical activity guidance to patients at risk for or with CVD rely on aligning workouts with individual physiological changes. A tailored prescription promotes a consistent and impactful exercise routine for enhancing health outcomes, considering patient preferences and motivations. Consequently, the selection and implementation of the best possible approach should consider available resources, with an ongoing emphasis on strategies to improve the delivery quality of exercise training in the context of FITT-VP prescription model (frequency, intensity, time, type, volume, and progression).
Consistently engaging in physical activity (PA) stands out as the most effective approach to preventing and managing non-communicable diseases, including cardiovascular diseases (CVD), while ensuring overall health (1). A variety of documented health benefits, such as improved quality of life and reduced cardiovascular and overall mortality, are achieved through regular PA and structured exercise in cardiovascular rehabilitation (CR) (2–4). Therefore, exercise training is strongly advised in primary and secondary prevention guidelines for CVD and is considered an essential component of healthcare plans for individuals with this profile (5–7).
The current recommendations from the World Health Organization (WHO) emphasize the importance of intensity as a significant component of exercise prescription for achieving meaningful health benefits. These recommendations state that adults should engage in a minimum of 150–300 min of moderate-intensity endurance physical activity per week, or at least 75–150 min of vigorous-intensity endurance physical activity per week, or an equivalent combination (8). Even greater volumes of PA are advised when greater health benefits are ambitioned (e.g., fat mass loss) (1, 9). Additionally, in the context of CR programs, although structured endurance exercise typically ranges from 24 to 30 min, extending these sessions to 35–45 min has been suggested to yield better outcomes across various cardiovascular populations (10–12).
For a patient at risk for (e.g., a person with obesity or diabetes) or with CVD, a tailored intensity prescription aims to optimize the benefits of the exercise intervention while minimizing associated risks (6, 13–15). Prescribing exercise training should align with the FITT-VP model, encompassing factors such as frequency, intensity, duration (time), specific type of exercise, volume, and progression (16, 17). Within this framework, establishing the ideal endurance exercise intensity is a cornerstone in enhancing intervention results (7, 18–21). However, it has become clear that clinicians and healthcare professionals still struggle with this task (7).
Therefore, the present document aims to offer a practical and critically revised update on assessing and determining the most optimal endurance exercise intensity in various settings for health professionals engaged in CR. Additionally, the document will offer a worldwide overview of the current exercise intensity domains recommended by CR guidelines, along with the application of different methods in clinical cases. Hence, it aims to bridge the gaps between best evidence and clinical practice, considering different available resources in the context of endurance exercise prescription for CR.
Evidence indicates that one metabolic equivalent of tasks (MET) increase in exercise capacity during CR is linked to a 13% reduction in mortality (22). Recently, Kokkinos et al. (23), in a study involving 750,302 adults, reported an inverse and graded association between cardiorespiratory fitness (CRF), determined by peak MET (METpeak), and mortality risk across the age spectrum, among men, women, and all races independently. In this study, individuals with the lowest CRF exhibited a 4-fold higher mortality risk than those with the highest fitness levels. Even in obese individuals, it has been shown that increments in METpeak during follow-up relate to reductions in mortality, but weight loss did not show such an association (24). Hence, in terms of prognosis “fitness” seems more important than “fatness”.
Optimizing exercise prescription is therefore becoming a growing goal, with an increasing interest in identifying factors contributing to changes in CRF throughout CR programs (25). Iannetta et al. (26) examined 2,310 CVD patients who completed a 12-week center-based CR program, and reported that the applied exercise intensity was one of the main predictors of METpeak post-CR, along with baseline METpeak, training duration, body mass index, and age. Uddin et al. (4), in a meta-regression analysis of 51 trials, including 7,553 patients with coronary artery disease (CAD) and heart failure (HF), reported that for each 10% increase in the applied exercise intensity, measured as percentual of peak oxygen uptake (%VO2peak) or percentual of peak heart rate (%HRpeak), a more substantial average improvement in peak oxygen uptake (VO2peak) by 1.0 ml/kg/min was noted across trials. This means that a large increment in exercise intensity must be exert (e.g., by 20%) to induce significantly greater improvements in VO2peak (e.g., +2 ml/kg/min). Another meta-analysis involving 13,220 patients from 128 studies found that prescribing higher exercise intensities during CR resulted in the most significant pooled effect for the change in relative VO2peak. Nevertheless, while all effects were statistically significant, they exhibited high heterogeneity, and the comparisons of intensities may lack clinical significance, with moderate, moderate-to-vigorous, and vigorous interventions showing a mean increase, expressed as ±95% CI, of 4.1 ± 2.3, 4.9 ± 0.9, and 5.5 ± 1.3 ml/kg/min, respectively (27).
In addition, exercise intensity matters for fat mass loss and weight control. A recent meta-analysis (involving 2,190 participants from 40 randomised controlled trials) demonstrated a dose-response effect of −0.15 (−0.23 to −0.07; p < 0.001) per 1,000 calories deficit per week for visceral fat mass loss in overweight/obese persons (28). Regarding lipid profile, in a systematic review with meta-analysis and meta-regression involving 2,990 sedentary adults with three or more metabolic syndrome factors, endurance exercise training significantly improved all lipid parameters (P < 0.001), with intensity associated with changes in triglycerides and volume associated with high-density lipoprotein-cholesterol (HDL-C) and low-density lipoprotein-cholesterol (LDL-C) (29). Another meta-analysis of randomized controlled trials showed that endurance exercise (at least moderate intensity, ≥12 weeks) positively affects lipid biomarkers linked to cardiovascular disease risk in sedentary adults by reducing atherogenic apolipoproteins, lipoprotein sub-fractions, and atherogenic lipid ratios while increasing antiatherogenic apolipoproteins and lipoprotein subfractions (30).
For significant blood pressure reductions, a sufficiently endurance exercise intensity (at moderate to high levels) must also be applied (31), and comparisons between different exercise types have been studied. A recent systematic review and meta-analysis found that both high-intensity interval training (HIIT) and moderate-intensity continuous training (MICT) reduce systolic blood pressure in hypertensive individuals, with HIIT showing greater reductions in diastolic blood pressure and potentially greater improvements in VO2peak compared to MICT (32). Other meta-analyses reported comparable reductions in blood pressure in adults with pre- to established hypertension for both modalities, with HIIT again showing greater improvements in VO2peak compared to MICT (33). Similar findings emerged in a separate meta-analysis of 10 studies involving 266 older adults where both HIIT and MICT demonstrated comparable reductions in blood pressure (34). The exercise-related reductions in blood pressure have not been fully elucidated and involve a combination of various mechanisms that differ among individuals, populations, and exercise training protocols (35, 36). A recent meta-analysis of 18 trials, involving 803 participants undergoing various exercise regimens (e.g., endurance, resistance, or combined) for at least 4 weeks, revealed significant reductions in systolic and diastolic blood pressure (mean difference: −6.2 mmHg and −4.5 mmHg, respectively) (37). These reductions were accompanied by mechanisms involving positive modulation of the renin–angiotensin–aldosterone system and sympathetic nervous system activity, resulting in decreased plasma angiotensin-II, aldosterone, and norepinephrine levels. Other mechanisms may include, for instance, local vascular resistance regulation influenced by endothelium-driven factors (36, 38), with an increase in nitric oxide (a potent vasodilator) (38, 39) and a reduction in endothelin-1 (a potent vasoconstrictor) (39, 40).
Significantly, HIIT protocols can vary widely, and exercise approaches exhibit inadequate reporting across diverse health conditions. Such unclear prescription and delivery methods affect the understanding of the “dose” of exercise needed to optimize health outcomes and, consequently, may limit its translation from research to practical application.
In a meta-analysis of 24 studies (involving 1,080 participants), HIIT demonstrated significantly greater improvement in VO2peak compared to MICT (+1.40 ml/min/kg for HIIT; 95% CI: 0.69–2.11; P < 0.001). This improvement persisted when examining patients with CAD and HF with reduced ejection fraction (HFrEF) separately, but was evident only with higher total energy expenditure (41). In another meta-analysis of 664 CAD and HF patients (15 studies), HIIT outperformed MICT in improving VO2peak for CAD, with no significant difference in HF. The most significant VO2peak improvement with HIIT occurred within eight weeks, and this modality also led to significantly greater increases in the first ventilatory threshold (VT1) and in left ventricular ejection fraction (LVEF) compared to MICT, with no significant differences in the ventilatory efficiency (VE/VCO2 slope). Yue et al. (42), in a meta-analysis of twenty-two studies (949 participants), concluded that HIIT is safe and more effective than MICT for improving CRF in CVD patients. Notably, HIIT performed three times weekly for over 12 weeks resulted in the greatest improvements.
Conversely, other meta-analyses, including CAD patients (12 studies, n = 609 patients) (43), and HFrEF patients (13 studies, n = 411 patients) (44), found that HIIT was not superior to MICT in increasing VO2peak when isocaloric exercise training programs were compared. Hence, it seems that HIIT could offer a more time-efficient method for improving VO2peak, considering that the total exercise duration was considerably shorter compared to MICT (45, 46). So, it is reasonable that all CR programs should consider the incorporation of HIIT as a supplementary or alternative for traditional MICT (44, 47, 48), not solely because of the described time-efficiency, but also due to its potential for superior or at least equivalent benefits compared to MICT in selected patients, as well as its positive impact on adherence for those who find it more motivating.
Moderate-intensity interval training (MIIT) has been proposed as a feasible and effective alternative, as reported, for instance, in community-based cardiac maintenance programs for patients with CVD, improving exercise performance and regulating blood glucose levels (49). Additionally, implementing MIIT before HIIT in training programs can be a valid strategy to boost body adaptations and adherence (50, 51). MIIT can also offer an advantage and reach a broader population by encouraging those averse to high-intensity exercises, such as some physically inactive and unfit individuals (50).
In a study evaluating body composition, resting blood pressure, heart rate (HR), and functional performance in elderly women, subjects underwent MIIT similar to HIIT but at moderate intensity to discern if HIIT benefits stemmed from its intermittency or intensity (52). The findings indicate that eight weeks of MIIT produced slightly better body composition results than MICT but were statistically lower than HIIT, emphasizing intensity's significance for this outcome. However, the effects of intermittency can't be dismissed, as MIIT surpassed MICT in some areas, supporting prior claims about its potential benefits (50). Although not extensively studied, intermittent and continuous exercises have shown different physiological responses despite similar levels of energy expenditure and intensity. Intermittent exercise may particularly favor mitochondrial development by possibly increasing the recruitment of fast-twitch fibers and inducing more pronounced metabolic variations (53).
Notably, interval training's complexity is underscored by controversies, impeding the ability to reach general conclusions (54). To support researchers and health professionals, experimental studies should provide detailed methodological reports, while future reviews and meta-analyses need to critically evaluate included articles' methods to prevent unwarranted generalizations (54). Additionally, more research is needed to understand MIIT's role in performance and health, and to compare its effects with HIIT and MICT in similar populations, elucidating which benefits can be attributed to changes in training parameters like intensity or stimulus type (50).
Other evidence also highlighted the importance of exercise intensity component in various other relevant health outcomes, as exemplified below. In a recent systematic review and meta-analysis on the dose-response relationship between PA levels and glycemic control in type 2 diabetes, it was found that individuals with diabetes may benefit from higher-than-recommended physical activity levels for optimal health outcomes. The optimum activity dose was 1,100 MET min per week, regardless of the baseline level of glycemic control. On average, this equals 244 min of moderate-intensity or 157 min of vigorous-intensity endurance activity per week (55). In a different systematic review and meta-analysis that assessed 25 pertinent studies, it was reported that both HIIT and MICT yield comparable effects on the quality of life and mental health of individuals with CVD. However, HIIT stands out as particularly advantageous for improving patients' self-perceived physiological functioning, taking into account their health status and social adjustment, especially among those with CAD (56).
Noteworthy, certain essential aspects of CR, including those pertaining to the intensity of endurance exercise, have not been entirely standardized yet (13), given the considerable variation in guidelines globally regarding recommendations (Table 1).
An appropriate endurance exercise intensity prescription depends on an adequate assessment of the patient (6) and may consider the available resources in different settings to optimize exercise interventions (64, 65).
The CPET is considered the gold standard assessment for exercise intensity prescription, and the threshold-based methodology should be the primary choice (5, 6, 14, 72–74) because it incorporates parameters that offer a comprehensive description of the body's response to exercise in terms of oxygen transport and utilization (13). Hence, this approach is recognized for accurately quantifying intensity and for being more tailored to individual phenotype (5, 6, 14, 72–74). This concept is grounded in physiological principles, as individual differences in transitioning from aerobic to anaerobic metabolism are associated with clinical factors such as prior interventions, medication use, and habitual physical activity levels, as well as with abnormalities in ventilation, cardiovascular function, or muscle physiology (73, 75).
The first ventilatory threshold (VT1) signifies the point at which there is a transition from predominantly aerobic metabolism to a stage where blood lactate begins to increase but ultimately reaches a balance. This balance is achieved through an equal rate of lactate production and consumption as a reactant in metabolic chemical reactions, thereby maintaining lactate levels within the range of 1.5–2 mmol/L (6). The second ventilatory threshold (VT2), also known as the respiratory compensation point, is the intensity at which blood lactate rapidly increases. Lactate accumulation thus emerges due to tissue anaerobiosis, leading to a disproportionate increase in minute ventilation (VE) relative to carbon dioxide production (VCO2), with lactate levels between 3 and 5 mmol/L (6).
In contrast to peak exercise capacity indices, both VT1 and VT2 are achievable by most CVD patients and are not dependent on maximum effort (6). So, it is recommended to prescribe the exercise intensity based on VTs, extrapolated to HR, load, or time (6, 14, 60, 62, 66, 68, 69).
While it is widely recommended to base exercise prescriptions on %VO2peak, which is directly measured during CPET, it is crucial to note that relying on fixed percentages of VO2peak may not be optimal. This approach fails to consider individual variations in fitness, body mass, age, and sex, all of which significantly influence cardiac and metabolic demands, particularly in low fitness levels (76), leading to varied and unexpected cardiac and metabolic demands (77). For example, Milani et al. (78) showed that in CVD patients the VT1 varied from 52% to 65% of VO2peak, and VT2 from 85% to 93% of VO2peak, which are considerable wide ranges.
While CPET is the optimal standard for prescribing endurance exercise, it is costly and may not always be accessible before CR. Furthermore, VTs estimation can be inaccurate, affected by inter-observer variability, and sometimes impractical for assessment, particularly in highly deconditioned individuals and those with HF (13).
According to the official position statement from the Secondary Prevention and Rehabilitation Section of the European Association of Preventive Cardiology regarding exercise intensity assessment and prescription in CR (6), performing an exercise test without gas exchange analysis is considered the minimum standard for accurately prescribing endurance exercise. This exam is commonly used in clinical practice because it is easy to perform and cost-effective, yielding measurements such as peak heart rate (HRpeak), peak workload (Wpeak), and METpeak that have been utilized for years to tailor exercise intensity. Most CR guidelines recommend that exercise intensity should then be based on peak effort indicators, such as percentages of peak workload (%Wpeak) and %HRpeak, or heart rate reserve (%HRR) (79). Therefore, it is crucial to explicitly define the correlation of these indices with the established preferable standards, the VT1 and VT2 direct measure method (13). Substantial differences in individual physiological responses at the VTs, such as HR or workload, and their alignment with widely used guideline-directed exercise intensity domains have been noted (72, 78, 80–85). This is important, as improperly setting exercise intensities may lead to under- or overtraining and can impact patient motivation and safety (6, 72, 78, 80–82, 84).
Importantly, the training status notably affects the relationship between VT1 or VT2 and exercise intensity domains. For instance, Hansen et al. (80), comparing physically unfit CVD patients (VO2peak < 15 ml/min/kg) to fit ones (VO2peak > 25 ml/min/kg) revealed significantly different exercise intensity domains for VT1 and VT2. Therefore, clinicians should exercise caution when applying guideline-recommended percentages of exercise intensity levels, considering changes in CRF during CR (80).
Furthermore, it is important to consider other cardiovascular conditions that may also influence exercise intensity prescription, including the presence of an ischemic threshold identified during exercise testing and its clinical consequences such as angina, arrhythmias, and hypotension (86). Additionally, symptoms like dyspnea or angina leading to test termination, slow HR recovery 1 min after the test, and abnormal hemodynamic and/or electrocardiographic responses provide additional evidence of poor prognosis and increased severity (87). It is also crucial to consider peripheral arterial disease, which requires evaluating the lower limb claudication threshold on a treadmill. It's worth noting that exercise testing performed on a cycle-ergometer allows for more precise cardiopulmonary evaluation in these cases (86).
Given the limitations highlighted regarding HR-based approaches and the incomplete representation of CRF by Wpeak in CVD patients (13), it is recommended to incorporate additional subjective methods guided by health professionals, such as the “talk test” (TT) and Borg Ratings of Perceived Exertion (RPE), for monitoring and adjusting exercise intensity during sessions (6).
According to the International Council of Cardiovascular Prevention and Rehabilitation Consensus (64), conducting exercise assessments, such as field-based tests like walking or step-up protocols, is essential even in settings with limited resources. Hence, it is recognized that all CR programs have the capability to conduct at least some objective physical assessment of physiological responses to exertion, which is considered the minimum requirement.
Consequently, functional tests are essential, especially when exercise tests with or without gas exchange analyses are unavailable or unfeasible. Functional tests assist in clinical assessment, provide criteria for comparing functional capacity during training, and obtain accurate individual physiological parameters during physical exertion to guide exercise prescription (14, 88–91).
The 6-minute walk test (6MWT) is the most recommended submaximal functional assessment according to current guidelines, followed by the Shuttle–Walk Test (SWT) and Step Test (ST) (Table 1).
The 6MWT is documented as a valid and reliable field test, reflecting the performance of daily life activities (90, 91). It is an uncomplicated assessment that requires no specialized equipment or advanced training. The test evaluates an individual's submaximal functional capacity according to the maximum distance covered as they walk on a flat, hard surface for 6 min. Additionally, it collects information on the physiological responses such as blood pressure, HR, pulse-oximetry, and the patient's self-assessment of fatigue and dyspnea (90).
In patients with HF the 6MWT is a safe and low-cost alternative for the prescription of endurance exercise (92), with prognostic value comparable to VO2peak (93–95). However, caution should be applied when prescribing endurance exercise based on HRpeak in the 6MWT. Some studies indicate a correlation with VT1 (89, 96), while other authors report that HRpeak in the exam closely resembles HR at VT2 (97). Importantly, the studies in the first situation used HR data from the final minute of the 6MWT, while in the second reported situation, HRpeak was identified considering the entire test.
Oliveira et al. (89) reported that exercise prescription for patients with HFrEF can be determined using the combination of the 6MWT and ST, relying on the HR values obtained during these tests. A robust correlation was observed between HR at VT1 and 6MWT HR (r = 0.81; p < 0.0001) as well as between HRpeak and ST HR (r = 0.89; p < 0.0001). Additionally, the authors proposed two types of exercise prescriptions to achieve the ideal HR target using these tests: (i) HR6MWT + 10%, or (ii) HR6MWT to HRST − 10% (both associated with Borg RPE scale). Previous research also reported the robust correlation between ST HR and HRpeak (98).
SWT is also a functional test option reported in CR guidelines (57, 66, 71), which is characterized by incremental intensity and external pacing (99). This test has proven to be reliable (100, 101), and it is employed in diverse clinical populations, encompassing individuals with CVD (102). Incorporating the SWT into the initial stages of an exercise training program is considered a valid option for tailoring the appropriate exercise intensity and functions as an outcome measure to assess the program's effectiveness (103). Nevertheless, there is also a notable absence of specific, objective recommendations on current guidelines concerning exercise intensity prescription based on these submaximal tests (Table 1).
Exercise intensity prescription can be guided by utilizing subjective assessments such as Borg scales or the TT (6, 73, 104). These tools are particularly valuable for overseeing the progression of exercise intensity in MICT programs, especially in home-based settings (6), and are strongly endorsed in current guidelines (Table 1).
In specific clinical groups, such as individuals on beta-blockers with a limited chronotropic reserve, monitoring HR becomes less advantageous (104). Moreover, training responses resulting from subjective methods closely mirror those prescribed through traditional methods (105, 106), suggesting that in these cases, subjective methods could even serve as a primary means for determining intensity (107).
A recent study by Bok et al. (104) confirmed the reliability and validity of both tools in defining ventilatory and lactate thresholds, stating that these measures can efficiently induce homeostatic disturbances associated with moderate, heavy, and severe-intensity domains during continuous exercise.
Consequently, when peak exercise data are unavailable or when HR is inaccessible or inapplicable (e.g., atrial fibrillation, pacemaker usage, or chronotropic incompetence) the RPE scale is as a valid alternative, despite its variability (108). Patient-reported RPE using the Borg scale promotes patient autonomy, allowing clinicians to steer individuals into specific intensity ranges, and is widely integrated into clinical practice (73). Remarkably, a more positive affective response was noted when individuals chose their own exercise intensities (109).
However, it's important to note that patients who report RPE may be affected by factors beyond the physical effort of the exercise, including psychological elements and environmental conditions. Furthermore, challenges in interpreting RPE may arise due to factors such as lack of familiarity with exercise training (modes/equipment), lower educational levels, and the use of beta-blockers (73).
In a study involving 2,560 participants of various ages, sexes, and fitness levels, incremental exercise tests revealed correlations between RPE and lactate levels (R2 = 0.71) and between RPE and HR (R2 = 0.55). The mean and standard deviation of RPE values (on Borg's scale of 6–20) for VT1 and VT2 were respectively 10.8 ± 1.8 and 13.6 ± 1.8 (110). Thus, for clinical application, an Borg RPE around 10–11 may reflect VT1, while values around 13–14 may correspond to VT2 (104).
However, caution is advised for healthcare professionals when prescribing moderate to high-intensity workouts to individuals with health complications based solely on RPE assessments, as it is also reported that the reliable agreement between RPE and blood lactate levels concentrations is demonstrated only during low-intensity exercises (111).
Similar to the Borg RPE, the TT can be employed as a supplementary practical method to manage exercise intensity in the daily activities of CVD patients (6, 112), delineating specific training zones during endurance exercise assessments and prescription (97, 113). This simple yet reasonably accurate method uses the comfort level of speech perception as a gauge for exercise intensity, allowing individuals to easily assess the intensity zone of their exercise (113, 114).
Numerous studies have validated the TT as a reliable method for estimating the lactate and VTs across diverse populations, including individuals with CVD (97, 115, 116), overweight and obese individuals (117). The observed parallels between HR and VO2 at TT stages and VTs in individuals with CVD suggest the potential application of TT stages in prescribing endurance exercise (97, 118). Importantly, it has been demonstrated that ischemic patients who can speak comfortably are unlikely to exhibit ECG evidence of myocardial ischemia (119). Moreover, it exhibits a close correlation with RPE (120).
Recently, Althoff et al. (97) highlighted that TT-positive (comfortable speech still possible during exercise) and TT-negative (patient unable to read the paragraph comfortably) are useful instruments to prescribe endurance exercise to patients with CVD. These tools exhibited a moderate relationship with HR at VT1 (r = 0.479) and a strong relationship with HR at VT2 (r = 0.757), respectively. These results are aligned with others (104, 121).
In summary, the TT's equivocal stage, characterized by somewhat uncomfortable speech, corresponds to intensity above VT1, while the negative stage, where a person is no longer able to recite the paragraph comfortably, aligns with intensity above VT2 (104).
Figure 1 provides a practical guidance on utilizing the TT and the RPE scale to monitor exercise intensities.
Figure 1. Practical guidance for monitoring exercise intensity using the talk test and the rating of perceived exertion scale. Adapted from: Bok et al. (104) and Festa et al. (113). VT1, first ventilatory threshold; VT2, second ventilatory threshold. Talk Test positive: comfortable speech still possible during exercise; Talk Test equivocal stage: characterized by somewhat uncomfortable speech; Talk Test negative: patient unable to read the paragraph comfortably.
Prediction equations for HRpeak are also used in CR contexts (122–125), although prescribing exercises based on the predicted values of HRpeak can be quite divergent, especially in patients with CVD (14, 78, 126, 127), mainly due to negative chronotropic influence of the beta-blockers, and disease-related dysfunctions (14). Although recently recommended in a guideline (69), it is important to emphasize that even equations for estimating HRpeak developed in samples of patients with CVD show significant dispersion of individual responses (124–126) and are not based on individual physiological responses to physical effort, but rather on age and resting parameters, such as resting HR (HRrest) (124, 125), ejection fraction, and hemoglobin level (125). Mytinger et al. (127) reported that HRpeak estimation equations are outdated for these patients and should not be used as frequently as done by healthy populations.
The increased dispersion between the actual individual HRpeak measurements and the predicted values may result in significant inaccuracies in the identification of exercise prescription domains (126). Therefore, considering evidence that intensity domains prescribed as a percentage of measured HRpeak can be highly inconsistent with guideline-based recommendations due to individual variability in HR response to exercise (72, 78, 80–85), determining exercise intensity based on estimated values may result in even greater inconsistencies. Hence, this approach could compromise the accuracy of CR prescriptions, potentially affecting efficacy and safety (126).
In this context, even in low-resource settings, it is advisable for the exercise intensity prescription to always depend on an objective assessment of individual physiological variables during effort. Therefore, at least a submaximal functional test should always be performed, along with subjective methods such as Borg RPE and/or TT, to adjust the prescription.
As previously stated, structured exercise training, aimed at enhancing physical fitness and prognosis, stands as a cornerstone in contemporary comprehensive CR (2–4, 128–133). The guidelines provide evidence-based best practice recommendations for CR, reducing disparities in the implementation treatments and in the development of a universal, evidence-based CR program (67).
However, the quantification of exercise training frequency, duration, and particularly intensity differs across current international CR guidelines (79). Numerous guidelines, position statements, and policy documents worldwide have proposed various thresholds for assessing exercise intensity prescription of an exercise-based CR program (79) (Table 1).
The majority of CR guidelines advise determining endurance exercise training intensity by referencing peak effort indices (such as %VO2peak and %HRpeak) or %HRR. Nonetheless, using peak exercise capacity indices for exercise prescription in CVD patients is challenging. Approximately 15% of general outpatient CR patients (80) and 46% of HF patients (82) do not achieve maximal effort (RER > 1.10) during CPET. In addition, a plateau in VO2 is often not observed (80, 134), and different peak indices are not interchangeable for exercise training prescription (6).
Moreover, the association with subjective methods, Borg and/or the TT, is widely reported. Importantly, the International Council of Cardiovascular Prevention and Rehabilitation Consensus (64) emphasizes the necessity of conducting exercise assessments, even in resource-constrained settings, which may include the use of field-based tests such as walking or step-up protocols. Regarding these functional tests, the 6MWT is the most cited, but most of the documents do not provide specific instructions about intensity prescription determination (Table 1).
It is important to highlight that the patients undergoing CR exhibit significant inconsistencies between guidelines and individual threshold-based intensities (72, 78, 80–85). These observations highlight that strict adherence to guideline-based exercise intensity domains can lead to erroneous prescriptions, especially in challenging situations, such as in patients with low fitness (80). In addition, the guidelines notably delineate exercise intensity domains validated in healthy individuals, who's cardiopulmonary and muscle physiology significantly differs from that of CVD patients (135). These disagreements have sparked discussions about reevaluating the necessity of reconsidering the current guidelines for exercise prescription in the CR setting (13, 80).
Therefore, the optimal approach to avoid relying on the mentioned peak exercise intensity indices is to associate training intensity with VT1 and VT2, which reflect real metabolic responses to submaximal effort in the moderate-intensity domain, are not dependent on maximum effort and can be attained by the majority of CVD individuals (6, 14).
Unfortunately, the direct VTs assessment by CPET is not the reality for many CR services (136). Krieger et al. (136), for instance, found that 50% of 91 American rehabilitation programs used submaximal tests, such as the 6MWT, while only 2% conducted incremental exercise testing. This scenario may be even worse in lower-income countries. As earlier reported, in lower-resource settings, the optimal strategy involves approximating VTs by integrating alternative objective assessments (ideally an ergometry test, or alternatively, another functional test providing individual physiological effort parameters like the 6MWT) alongside subjective methods for guiding prescription adjustments, such as the Borg scale and the Talk Test.
As previously mentioned, the planning and implementation of exercise prescription in CVD should be aligned with the FITT-VP model [F: frequency, I: intensity, T: duration (time), T: type of specific exercise, V: volume, P: progression] (16, 17). In this context, intensity emerges as the most complex component, triggering significant controversies over the reliability and validity of methods used to determine and prescribe training intensity (82, 137). Conversely, frequency, duration, and volume of training can be adjusted by varying session frequency, session duration, or total workload within a specific timeframe (82, 137). Furthermore, as previously detailed, notable inconsistencies and disagreements exist among recommendations from different CR guidelines (6, 78, 80, 84). These factors can often result in suboptimal exercise prescriptions for many individuals and limit the ability to compare outcomes across various training programs in clinical practice and research (13, 78, 82).
A general exercise recommendation for both primary and secondary prevention of CVD includes at least 150 min of light to moderate intensity endurance exercise per week, spread over 3–5 days, along with moderate-intensity dynamic resistance exercises on 2 days (6, 7, 14). While this initial guidance is suitable for starting physical activity, adapting the prescription based on individual conditions, risk profile, and needs is crucial to maximize exercise's clinical benefits and prevent CVD (7). This approach is supported by documented additional clinical benefits from aligning prescription components with specific risk factors (7).
Considering the FITT-VP principles, Figure 2 outlines key recommendations for a personalized endurance exercise prescription in CR (5–7, 21). Other exercise modalities, such as strength and flexibility training, should also be included in the program but are not covered in this article.
Figure 2. Key recommendations for endurance exercise prescription in cardiovascular rehabilitation patients. Adapted from: D'Ascenzi et al. (5), Hansen et al. (6, 7, 21). *Gallardo-Gomez et al. (55). †Wood el al. (29). CR, cardiovascular rehabilitation; CVD, cardiovascular diseases; HDL, High-Density Lipoprotein; HF, heart failure; HIIT, high intensity interval training; VTs, ventilatory thresholds.
In a recent study (78) involving 972 maximal treadmill CPET on patients with CVD, a novel approach was proposed, using multivariable equations to estimate HR at VTs, based on parameters individually assessed during an exercise test without gas exchange analyses (HRpeak, HRrest, and METpeak), according to the Equations 1, 2.
The equations demonstrated significant R2 for both VTs (0.726 for VT1 and 0.901 for VT2). Additionally, the method showed superior accuracy in internal validation, with a mean absolute percentage error (MAPE) of 6.0% for VT1 and 4.3% for VT2, compared to other guideline-based methods relying on measured and estimated %HRpeak (MAPE ranging from 9.5% to 23.8% for VT1 and 5.8% to 19.3% for VT2).
While this suggests their potential as a valid alternative when CPET is unavailable, additional studies are necessary to externally validate these treadmill equations for HR at VTs. Furthermore, since the equations were developed solely in the context of treadmill assessments, their applicability in cycle-ergometer exams remains untested.
However, equations to predict HR at VTs using cycle-ergometer assessments were recently developed in a European database of cardiometabolic disease patients and externally validated in a South American sample (138). These cycle-ergometer prediction equations, which also utilize variables measured during an exercise test without gas analysis, demonstrated excellent coefficients of determination, with R2 values of 0.77 for VT1 and 0.88 for VT2 (Equations 3, 4).
In the external validation, these predictive equations for HR at VTs showed superiority over widely used guideline-directed intensity domains for %HRpeak and %HRR, exhibiting lower and more consistent MAPE values (VT1: 7.1%, VT2: 5.0%) in comparison to the range observed in the guideline-based exercise intensity domains (VT1: 6.8%–21.3%, VT2: 5.1%–16.7%) (138). Additionally, these novel equations showed ergometer interchangeability, as the validation sample included both treadmill and cycle-ergometer assessments.
Another important finding of this study was a suggestion to adjust the %HRR method, by using 42% for VT1% and 77% for VT2 (138). These adjusted values for moderate intensity prescription demonstrated the same MAPE values of the predictive equations and were more consistent than the current guideline recommendations for %HRR.
In summary, assessing and prescribing endurance exercise intensity for patients with CVD involves various methods. The CPET stands as the gold standard, and utilizing VT1 and VT2 to tailor prescriptions based on individual physiological responses is the optimal approach to exercise prescription. Alternatives include simpler exercise tests without gas exchange analysis, offering less precision but practicality through measurements like HRpeak and workload. Importantly, novel equations to predict HR at these thresholds have been developed and validated, as well as a range-adjusted %HRR derived from CR patients, enhancing the accuracy of exercise intensity prescriptions when CPET is unavailable. Especially in resource-limited settings, submaximal functional tests like the 6-min walk test play a vital role, and subjective tools such as the Borg RPE and the Talk Test should always be applied as an addition to objective methods.
The following clinical cases illustrate the prescription of endurance exercise intensity based on HR using different approaches, both with and without gas exchange analysis.
Male, 58 years. Weight: 76.3 kg, Height: 1.70 m, BMI: 26.4 kg/m2. Active smoker, dyslipidemia, family history of premature CAD.
Clinical History:
4 weeks before the first assessment in CR: acute event of anterior myocardial infarction with successful primary angioplasty (occlusion of the left anterior coronary artery—uniarterial disease).
• Medications: Antiplatelets, Metoprolol 50 mg/day, Ramipril 10 mg/day, and Rosuvastatin 20 mg/day.
• Echocardiogram with hypokinetic dysfunction in the anterior wall with a left ventricular ejection fraction of 45%.
• Treadmill CPET: Test interrupted by fatigue in lower limbs; Absence of myocardial ischemia; Maximal effort (RERpeak: 1.30); Reduced CRF (VO2peak: 58% of predicted values—Wasserman); Peak treadmill load equivalent to 7.4 METs (FRIEND equation) (139).
Figure 3 presents additional exercise test information, while Figure 4 illustrates the solution.
Figure 3. Exercise test information of clinical case 1. HR, heart rate; HRR, heart rate reserve; HRrest, rest heart rate; VO2, oxygen uptake; VT1, first ventilatory threshold; VT2, second ventilatory threshold.
Figure 4. Solution of clinical case 1: prescribing moderate endurance exercise intensity using various heart rate-based approaches. CPET, cardiopulmonary exercise test; HR, heart rate; HRpeak, peak heart rate; HRR, heart rate reserve; HRrest, rest heart rate; METpeak, peak metabolic equivalent of tasks; VO2, oxygen uptake; VTs, ventilatory thresholds; VT1, first ventilatory threshold; VT2, second ventilatory threshold; %HRpeak, percentage of peak heart rate; %HRR, percentage oof heart rate reserve.
Interpretation:
The optimal method for exercise prescription involves utilizing the VTs identified during CPET, resulting in a moderate-intensity prescription of 94–106 bpm. It is evident that relying on %HRpeak can lead to a significant error in the prescription range: the upper limit guided by %HRpeak is, in fact, the lower limit based on threshold-based prescription. This discrepancy can result in under-training and likely diminish the benefits of exercise training based solely on HRpeak.
Utilizing prescription guidance through %HRR or estimating HR at VTs using equations leads to range-based values similar to those identified by CPET. Therefore, a prescription based on two or more parameters (HRrest and HRpeak, with or without information about CRF) can be more individualized and, arguably, more beneficial.
Male, 62 years. Weight: 77.2 kg, Height: 1.78 m, BMI: 24.4 kg/m2. Former smoker, dyslipidaemia, diabetes mellitus, hypertension.
• Clinical history:
Chronic coronary artery disease with coronary artery bypass graft 10 years in the past.
• Medications: Antiplatelets, Metoprolol 75 mg/day, Ramipril 10 mg/day and Rosuvastatine/Ezetimiibe 20/10 mg/day, Metformine 2 g/day, Dapagliflozin 10 mg/day.
• Echocardiogram with hipocontratile disfunction in inferior wall with left ventricular ejection fraction of 40%.
• Cycle-ergometer CPET: Test interupted by dispnea; Absence of myocardial ischemia; Maximal effort (RER peak: 1.26); Reduced CRF (VO2peak: 71% of predicted values—Wasserman); Peak load equivalent to 6.3 MET (FRIEND equation) (140).
Figure 5 presents additional exercise test information, while Figure 6 illustrates the solution.
Figure 5. Exercise test information of clinical case 2. HR, heart rate; HRR, heart rate reserve; HRrest, rest heart rate; VO2, oxygen uptake; VT1, first ventilatory threshold; VT2, second ventilatory threshold.
Figure 6. Solution of clinical case 2: prescribing moderate endurance exercise intensity using various heart rate-based approaches. CPET, cardiopulmonary exercise test; HR, heart rate; HRpeak, peak heart rate; HRR, heart rate reserve; HRrest, rest heart rate; VO2, oxygen uptake; VTs, ventilatory thresholds; VT1, first ventilatory threshold; VT2, second ventilatory threshold; %HRpeak, percentage of peak heart rate; %HRR, percentage oof heart rate reserve.
Interpretation:
The preferable method, guided by CPET's VTs, resulted in a moderate-intensity prescription ranging from 85 to 108 bpm (VT1 to VT2). Once again, the prescription based on %HRpeak exhibited lower accuracy, resulting in lower-intensity training recommendation compared to the threshold-based method. This discrepancy could lead to undertraining when relying on exercise prescription based on HRpeak. Additionally, the prescription guided by both %HRR and equations demonstrated higher accuracy, despite presenting different numerical values, but closer to those identified by CPET.
When collaborating with patients, healthcare professionals should integrate evidence, guidelines, theories, techniques, and tools related to behaviour change, as well as individual patients' requirements and values (57). It is crucial to select and implement the best possible approach, considering the available resources, with a continuous focus on strategies to enhance the quality of the delivery of CR, especially in low-resource settings.
It is advisable to implement a personalized approach that takes into account the patient's preferences and capabilities, allowing them to select their exercise intensity rather than imposing it. This becomes particularly vital when seeking sustained commitment over the long term (109).
Recognizing the importance of improving general physical activity advice, Festa et al. (113) recently suggested integrating the RPE scale, TT, and weekly training distribution as practical tools in the WHO guidelines for the general population to enhance the establishment of endurance training zones. An RPE < 11 (Borg 6–20) or <4 (Borg modified 0–10), or the ability to speak comfortably, signifies low-intensity exercise. Conversely, a Borg score ≥11 or Borg modified ≥4, or the inability to speak comfortably, indicates moderate to high-intensity exercise. Such approaches could provide valuable assistance to individuals in healthcare or training settings and professionals who lack adequate tools for creating exercise programs.
Therefore, optimizing the prescription of exercise intensity in CR settings and providing personalized physical activity advice, by correlating the intensity component with individual physiological changes promotes a more consistent and impactful exercise routine, effectively enhancing fitness levels among the general population and individuals with CVD (141). This additionally benefit decision support systems for CR, such as EXPERT tool, aiding in the integration of a defined methodology for assessing exercise intensity (142). Moreover, it contributes to enhancing access to healthcare services through the utilization of the internet and telemonitoring. This advancement is pivotal for the evolution of new practices in CR, including home-based training programs (143).
Figure 7 illustrates, with increasing accuracy, the currently most recommended and commonly used methods for assessing and prescribing endurance exercise intensity in CR.
Figure 7. Schematic illustration of endurance exercise intensity methods, ranging from minimal to optimal standards, considering high and low resource settings. LVEF, left ventricular ejection; Hb, hemoglobin; HR, heart rate; HRpeak, peak heart rate; HRR, heart rate reserve; HRrest, rest heart rate; METpeak, peak metabolic equivalent of tasks; SWT, shuttle walk test; VT1, first ventilatory threshold; VT2, second ventilatory threshold; %HRpeak, percentage of peak heart rate; %HRR, percentage oof heart rate reserve; RPE, Ratings of Perceived Exertion; 6MWT, six-minute walk test.
To sum up, this document has provided a practical and critical update on the prescription of endurance exercise intensity for CVD patients, encompassing worldwide guideline recommendations and addressing applicability in clinical practice across various resource availability realities, from minimal to optimal. The optimal approach is to guide intensity prescription based on VTs obtained via CPET. In the absence of CPET, the next best option is an individual assessment through an exercise test without gas exchange analyses. In low-resource settings where this option is also unavailable, performing at least another objective submaximal functional test, like 6MWT, can provide direct measure of the physical condition and should be the minimal approach. Additionally, objective methods should always be complemented by subjective ones, such as Borg RPE and TT, to adjust prescription intensity and consider patient preferences and motivations.
JM: Conceptualization, Writing – original draft. MM: Writing – review & editing. KV: Writing – review & editing. GC: Conceptualization, Writing – review & editing. DH: Conceptualization, Supervision, Writing – review & editing.
The author(s) declare financial support was received for the research, authorship, and/or publication of this article.
The authors (JGPOM and MM) received fundings from Special Research Fund (BOF) from Hasselt University, Belgium (Number: BOF23DOCBL10 and BOF23KV10) and from Coordenação de Aperfeiçoamento de Pessoal de Nível Superior [CAPES (Coordination for the Advancement of Higher Education Personnel)], Brazil.
We extend our heartfelt gratitude to the laboratories staff.
The authors declare that the research was conducted in the absence of any commercial or financial relationships that could be construed as a potential conflict of interest.
The reviewer HH declared a past co-authorship with the author DH to the handling editor.
All claims expressed in this article are solely those of the authors and do not necessarily represent those of their affiliated organizations, or those of the publisher, the editors and the reviewers. Any product that may be evaluated in this article, or claim that may be made by its manufacturer, is not guaranteed or endorsed by the publisher.
1. Verboven K, Hansen D. Critical reappraisal of the role and importance of exercise intervention in the treatment of obesity in adults. Sports Med. (2021) 51(3):379–89. doi: 10.1007/s40279-020-01392-8
2. Anderson L, Oldridge N, Thompson DR, Zwisler AD, Rees K, Martin N, et al. Exercise-based cardiac rehabilitation for coronary heart disease: cochrane systematic review and meta-analysis. J Am Coll Cardiol. (2016) 67(1):1–12. doi: 10.1016/j.jacc.2015.10.044
3. Taylor RS, Walker S, Smart NA, Piepoli MF, Warren FC, Ciani O, et al. Impact of exercise rehabilitation on exercise capacity and quality-of-life in heart failure: individual participant meta-analysis. J Am Coll Cardiol. (2019) 73(12):1430–43. doi: 10.1016/j.jacc.2018.12.072
4. Uddin J, Zwisler AD, Lewinter C, Moniruzzaman M, Lund K, Tang LH, et al. Predictors of exercise capacity following exercise-based rehabilitation in patients with coronary heart disease and heart failure: a meta-regression analysis. Eur J Prev Cardiol. (2016) 23(7):683–93. doi: 10.1177/2047487315604311
5. D'Ascenzi F, Cavigli L, Pagliaro A, Focardi M, Valente S, Cameli M, et al. Clinician approach to cardiopulmonary exercise testing for exercise prescription in patients at risk of and with cardiovascular disease. Br J Sports Med. (2022) 56(20):1180–7. doi: 10.1136/bjsports-2021-105261
6. Hansen D, Abreu A, Ambrosetti M, Cornelissen V, Gevaert A, Kemps H, et al. Exercise intensity assessment and prescription in cardiovascular rehabilitation and beyond: why and how: a position statement from the secondary prevention and rehabilitation section of the European association of preventive cardiology. Eur J Prev Cardiol. (2022) 29(1):230–45. doi: 10.1093/eurjpc/zwab007
7. Hansen D, Coninx K, Beckers P, Cornelissen V, Kouidi E, Neunhauserer D, et al. Appropriate exercise prescription in primary and secondary prevention of cardiovascular disease: why this skill remains to be improved among clinicians and healthcare professionals. A call for action from the EXPERT network. Eur J Prev Cardiol. (2023) 30(18):1986–95. doi: 10.1093/eurjpc/zwad232
8. Bull FC, Al-Ansari SS, Biddle S, Borodulin K, Buman MP, Cardon G, et al. World health organization 2020 guidelines on physical activity and sedentary behaviour. Br J Sports Med. (2020) 54(24):1451–62. doi: 10.1136/bjsports-2020-102955
9. Hansen D, Niebauer J, Cornelissen V, Barna O, Neunhauserer D, Stettler C, et al. Exercise prescription in patients with different combinations of cardiovascular disease risk factors: a consensus statement from the EXPERT working group. Sports Med. (2018) 48(8):1781–97. doi: 10.1007/s40279-018-0930-4
10. Taylor RS, Walker S, Ciani O, Warren F, Smart NA, Piepoli M, et al. Exercise-based cardiac rehabilitation for chronic heart failure: the EXTRAMATCH II individual participant data meta-analysis. Health Technol Assess. (2019) 23(25):1–98. doi: 10.3310/hta23250
11. Kraal JJ, Vromen T, Spee R, Kemps HMC, Peek N. The influence of training characteristics on the effect of exercise training in patients with coronary artery disease: systematic review and meta-regression analysis. Int J Cardiol. (2017) 245:52–8. doi: 10.1016/j.ijcard.2017.07.051
12. Ismail H, McFarlane JR, Dieberg G, Smart NA. Exercise training program characteristics and magnitude of change in functional capacity of heart failure patients. Int J Cardiol. (2014) 171(1):62–5. doi: 10.1016/j.ijcard.2013.11.045
13. Davos CH. Do we have to reconsider the guidelines for exercise intensity determination in cardiovascular rehabilitation? Eur J Prev Cardiol. (2019) 26(18):1918–20. doi: 10.1177/2047487319871870
14. Carvalho T, Milani M, Ferraz AS, Silveira ADD, Herdy AH, Hossri CAC, et al. Brazilian cardiovascular rehabilitation guideline 2020. Arq Bras Cardiol. (2020) 114(5):943–87. doi: 10.36660/abc.20200407
15. Fletcher GF, Ades PA, Kligfield P, Arena R, Balady GJ, Bittner VA, et al. Exercise standards for testing and training: a scientific statement from the American Heart Association. Circulation. (2013) 128(8):873–934. doi: 10.1161/CIR.0b013e31829b5b44
16. Ambrosetti M, Abreu A, Corra U, Davos CH, Hansen D, Frederix I, et al. Secondary prevention through comprehensive cardiovascular rehabilitation: from knowledge to implementation. 2020 update. A position paper from the secondary prevention and rehabilitation section of the European association of preventive cardiology. Eur J Prev Cardiol. (2021) 28(5):460–95. doi: 10.1177/2047487320913379
17. Bushman BA. Developing the P (for progression) in a FITT-VP exercise prescription. ACSM’s Health Fit J. (2018) 22(3):6–9. doi: 10.1249/FIT.0000000000000378
18. Hansford HJ, Wewege MA, Cashin AG, Hagstrom AD, Clifford BK, McAuley JH, et al. If exercise is medicine, why don't we know the dose? An overview of systematic reviews assessing reporting quality of exercise interventions in health and disease. Br J Sports Med. (2022) 56(12):692–700. doi: 10.1136/bjsports-2021-104977
19. Taylor JL, Bonikowske AR, Olson TP. Optimizing outcomes in cardiac rehabilitation: the importance of exercise intensity. Front Cardiovasc Med. (2021) 8:734278. doi: 10.3389/fcvm.2021.734278
20. Taylor RS, Dalal HM, McDonagh STJ. The role of cardiac rehabilitation in improving cardiovascular outcomes. Nat Rev Cardiol. (2022) 19(3):180–94. doi: 10.1038/s41569-021-00611-7
21. Hansen D, Beckers P, Neunhauserer D, Bjarnason-Wehrens B, Piepoli MF, Rauch B, et al. Standardised exercise prescription for patients with chronic coronary syndrome and/or heart failure: a consensus statement from the EXPERT working group. Sports Med. (2023) 53:2013–37. doi: 10.1007/s40279-023-01909-x
22. Martin BJ, Arena R, Haykowsky M, Hauer T, Austford LD, Knudtson M, et al. Cardiovascular fitness and mortality after contemporary cardiac rehabilitation. Mayo Clin Proc. (2013) 88(5):455–63. doi: 10.1016/j.mayocp.2013.02.013
23. Kokkinos P, Faselis C, Samuel IBH, Pittaras A, Doumas M, Murphy R, et al. Cardiorespiratory fitness and mortality risk across the spectra of age, race, and sex. J Am Coll Cardiol. (2022) 80(6):598–609. doi: 10.1016/j.jacc.2022.05.031
24. Lee DC, Sui X, Artero EG, Lee IM, Church TS, McAuley PA, et al. Long-term effects of changes in cardiorespiratory fitness and body mass index on all-cause and cardiovascular disease mortality in men: the aerobics center longitudinal study. Circulation. (2011) 124(23):2483–90. doi: 10.1161/CIRCULATIONAHA.111.038422
25. Gevaert AB, Adams V, Bahls M, Bowen TS, Cornelissen V, Dorr M, et al. Towards a personalised approach in exercise-based cardiovascular rehabilitation: how can translational research help? A ‘call to action’ from the section on secondary prevention and cardiac rehabilitation of the European association of preventive cardiology. Eur J Prev Cardiol. (2020) 27(13):1369–85. doi: 10.1177/2047487319877716
26. Iannetta D, Rouleau CR, Chirico D, Fontana FY, Hauer T, Wilton SB, et al. An evaluation of the role of the exercise training dose for changes in exercise capacity following a standard cardiac rehabilitation program. Int J Cardiol. (2023) 379:104–10. doi: 10.1016/j.ijcard.2023.03.036
27. Mitchell BL, Lock MJ, Davison K, Parfitt G, Buckley JP, Eston RG. What is the effect of aerobic exercise intensity on cardiorespiratory fitness in those undergoing cardiac rehabilitation? A systematic review with meta-analysis. Br J Sports Med. (2019) 53(21):1341–51. doi: 10.1136/bjsports-2018-099153
28. Recchia F, Leung CK, Yu AP, Leung W, Yu DJ, Fong DY, et al. Dose-response effects of exercise and caloric restriction on visceral adiposity in overweight and obese adults: a systematic review and meta-analysis of randomised controlled trials. Br J Sports Med. (2023) 57(16):1035–41. doi: 10.1136/bjsports-2022-106304
29. Wood G, Taylor E, Ng V, Murrell A, Patil A, van der Touw T, et al. Determining the effect size of aerobic exercise training on the standard lipid profile in sedentary adults with three or more metabolic syndrome factors: a systematic review and meta-analysis of randomised controlled trials. Br J Sports Med. (2021) 56(18):1032–41. doi: 10.1136/bjsports-2021-103999
30. Wood G, Taylor E, Ng V, Murrell A, Patil A, van der Touw T, et al. Estimating the effect of aerobic exercise training on novel lipid biomarkers: a systematic review and multivariate meta-analysis of randomized controlled trials. Sports Med. (2023) 53(4):871–86. doi: 10.1007/s40279-023-01817-0
31. Cornelissen VA, Smart NA. Exercise training for blood pressure: a systematic review and meta-analysis. J Am Heart Assoc. (2013) 2(1):e004473. doi: 10.1161/JAHA.112.004473
32. Leal JM, Galliano LM, Del Vecchio FB. Effectiveness of high-intensity interval training versus moderate-intensity continuous training in hypertensive patients: a systematic review and meta-analysis. Curr Hypertens Rep. (2020) 22(3):26. doi: 10.1007/s11906-020-1030-z
33. Costa EC, Hay JL, Kehler DS, Boreskie KF, Arora RC, Umpierre D, et al. Effects of high-intensity interval training versus moderate-intensity continuous training on blood pressure in adults with pre- to established hypertension: a systematic review and meta-analysis of randomized trials. Sports Med. (2018) 48(9):2127–42. doi: 10.1007/s40279-018-0944-y
34. Carpes L, Costa R, Schaarschmidt B, Reichert T, Ferrari R. High-intensity interval training reduces blood pressure in older adults: a systematic review and meta-analysis. Exp Gerontol. (2022) 158:111657. doi: 10.1016/j.exger.2021.111657
35. Grotle AK, Macefield VG, Farquhar WB, O'Leary DS, Stone AJ. Recent advances in exercise pressor reflex function in health and disease. Auton Neurosci. (2020) 228:102698. doi: 10.1016/j.autneu.2020.102698
36. Hamer M. The anti-hypertensive effects of exercise: integrating acute and chronic mechanisms. Sports Med. (2006) 36(2):109–16. doi: 10.2165/00007256-200636020-00002
37. Baffour-Awuah B, Man M, Goessler KF, Cornelissen VA, Dieberg G, Smart NA, et al. Effect of exercise training on the renin-angiotensin-aldosterone system: a meta-analysis. J Hum Hypertens. (2024) 38(2):89–101. doi: 10.1038/s41371-023-00872-4
38. Moreau KL, Clayton ZS, DuBose LE, Rosenberry R, Seals DR. Effects of regular exercise on vascular function with aging: does sex matter? Am J Physiol Heart Circ Physiol. (2024) 326(1):H123–37. doi: 10.1152/ajpheart.00392.2023
39. Cruz LG, Bocchi EA, Grassi G, Guimaraes GV. Neurohumoral and endothelial responses to heated water-based exercise in resistant hypertensive patients. Circ J. (2017) 81(3):339–45. doi: 10.1253/circj.CJ-16-0870
40. Wenner MM, Welti LM, Dow CA, Greiner JJ, Stauffer BL, DeSouza CA. Aerobic exercise training reduces ET-1-mediated vasoconstriction and improves endothelium-dependent vasodilation in postmenopausal women. Am J Physiol Heart Circ Physiol. (2023) 324(6):H732–8. doi: 10.1152/ajpheart.00674.2022
41. Pattyn N, Beulque R, Cornelissen V. Aerobic interval vs. continuous training in patients with coronary artery disease or heart failure: an updated systematic review and meta-analysis with a focus on secondary outcomes. Sports Med. (2018) 48(5):1189–205. doi: 10.1007/s40279-018-0885-5
42. Yue T, Wang Y, Liu H, Kong Z, Qi F. Effects of high-intensity interval vs. moderate-intensity continuous training on cardiac rehabilitation in patients with cardiovascular disease: a systematic review and meta-analysis. Front Cardiovasc Med. (2022) 9:845225. doi: 10.3389/fcvm.2022.845225
43. Gomes-Neto M, Duraes AR, Reis H, Neves VR, Martinez BP, Carvalho VO. High-intensity interval training versus moderate-intensity continuous training on exercise capacity and quality of life in patients with coronary artery disease: a systematic review and meta-analysis. Eur J Prev Cardiol. (2017) 24(16):1696–707. doi: 10.1177/2047487317728370
44. Gomes-Neto M, Duraes AR, Conceicao LSR, Saquetto MB, Ellingsen O, Carvalho VO. High intensity interval training versus moderate intensity continuous training on exercise capacity and quality of life in patients with heart failure with reduced ejection fraction: a systematic review and meta-analysis. Int J Cardiol. (2018) 261:134–41. doi: 10.1016/j.ijcard.2018.02.076
45. Ellingsen O, Halle M, Conraads V, Stoylen A, Dalen H, Delagardelle C, et al. High-intensity interval training in patients with heart failure with reduced ejection fraction. Circulation. (2017) 135(9):839–49. doi: 10.1161/CIRCULATIONAHA.116.022924
46. Pattyn N, Vanhees L, Cornelissen VA, Coeckelberghs E, De Maeyer C, Goetschalckx K, et al. The long-term effects of a randomized trial comparing aerobic interval versus continuous training in coronary artery disease patients: 1-year data from the SAINTEX-CAD study. Eur J Prev Cardiol. (2016) 23(11):1154–64. doi: 10.1177/2047487316631200
47. McGregor G, Powell R, Begg B, Birkett ST, Nichols S, Ennis S, et al. High-intensity interval training in cardiac rehabilitation: a multi-centre randomized controlled trial. Eur J Prev Cardiol. (2023) 30(9):745–55. doi: 10.1093/eurjpc/zwad039
48. Taylor JL, Holland DJ, Keating SE, Leveritt MD, Gomersall SR, Rowlands AV, et al. Short-term and long-term feasibility, safety, and efficacy of high-intensity interval training in cardiac rehabilitation: the FITR heart study randomized clinical trial. JAMA Cardiol. (2020) 5(12):1382–9. doi: 10.1001/jamacardio.2020.3511
49. Christle JW, Knapp S, Geisberger M, Cervenka M, Moneghetti K, Myers J, et al. Interval endurance and resistance training as part of a community-based secondary prevention program for patients with diabetes Mellitus and coronary artery disease. J Cardiopulm Rehabil Prev. (2020) 40(1):17–23. doi: 10.1097/HCR.0000000000000426
50. Jimenez-Pavon D, Lavie CJ. High-intensity intermittent training versus moderate-intensity intermittent training: is it a matter of intensity or intermittent efforts? Br J Sports Med. (2017) 51(18):1319–20. doi: 10.1136/bjsports-2016-097015
51. Maaloul R, Ben Dhia I, Marzougui H, Turki M, Kacem FH, Makhlouf R, et al. Is moderate-intensity interval training more tolerable than high-intensity interval training in adults with obesity? Biol Sport. (2023) 40(4):1159–67. doi: 10.5114/biolsport.2023.123323
52. Coswig VS, Barbalho M, Raiol R, Del Vecchio FB, Ramirez-Campillo R, Gentil P. Effects of high vs moderate-intensity intermittent training on functionality, resting heart rate and blood pressure of elderly women. J Transl Med. (2020) 18(1):88. doi: 10.1186/s12967-020-02261-8
53. Combes A, Dekerle J, Bougault V, Daussin FN. Physiological comparison of intensity-controlled, isocaloric intermittent and continuous exercise. Eur J Sport Sci. (2018) 18(10):1368–75. doi: 10.1080/17461391.2018.1491627
54. Viana RB, de Lira CAB, Naves JPA, Coswig VS, Del Vecchio FB, Ramirez-Campillo R, et al. Can we draw general conclusions from interval training studies? Sports Med. (2018) 48(9):2001–9. doi: 10.1007/s40279-018-0925-1
55. Gallardo-Gomez D, Salazar-Martinez E, Alfonso-Rosa RM, Ramos-Munell J, Del Pozo-Cruz J, Del Pozo Cruz B, et al. Optimal dose and type of physical activity to improve glycemic control in people diagnosed with type 2 diabetes: a systematic review and meta-analysis. Diabetes Care. (2024) 47(2):295–303. doi: 10.2337/dc23-0800
56. Yu H, Zhao X, Wu X, Yang J, Wang J, Hou L. High-intensity interval training versus moderate-intensity continuous training on patient quality of life in cardiovascular disease: a systematic review and meta-analysis. Sci Rep. (2023) 13(1):13915. doi: 10.1038/s41598-023-40589-5
57. Verdicchio C, Freene N, Hollings M, Maiorana A, Briffa T, Gallagher R, et al. A clinical guide for assessment and prescription of exercise and physical activity in cardiac rehabilitation. A CSANZ position statement. Heart Lung Circ. (2023) 32(9):1035–48. doi: 10.1016/j.hlc.2023.06.854
58. Ezekowitz JA, O'Meara E, McDonald MA, Abrams H, Chan M, Ducharme A, et al. 2017 Comprehensive update of the Canadian cardiovascular society guidelines for the management of heart failure. Can J Cardiol. (2017) 33(11):1342–433. doi: 10.1016/j.cjca.2017.08.022
59. Pelliccia A, Sharma S, Gati S, Back M, Borjesson M, Caselli S, et al. 2020 ESC guidelines on sports cardiology and exercise in patients with cardiovascular disease. Eur Heart J. (2021) 42(1):17–96. doi: 10.1093/eurheartj/ehaa605
60. Pavy B, Iliou MC, Verges-Patois B, Brion R, Monpere C, Carre F, et al. French Society of cardiology guidelines for cardiac rehabilitation in adults. Arch Cardiovasc Dis. (2012) 105(5):309–28. doi: 10.1016/j.acvd.2012.01.010
61. Dendale P, Dereppe H, De Sutter J, Laruelle C, Vaes J, Lamotte M, et al. Position paper of the Belgian working group on cardiovascular prevention and rehabilitation: cardiovascular rehabilitation. Acta Cardiol. (2008) 63(6):673–81. doi: 10.2143/AC.63.6.2033383
62. Niebauer J, Mayr K, Tschentscher M, Pokan R, Benzer W. Outpatient cardiac rehabilitation: the Austrian model. Eur J Prev Cardiol. (2013) 20(3):468–79. doi: 10.1177/2047487312446137
63. KNGF. Clinical practice guideline for physical therapy in patients undergoing cardiac rehabilitation. Dutch J Phys Ther. (2011) 121(4):1–47.
64. Grace SL, Turk-Adawi KI, Contractor A, Atrey A, Campbell NR, Derman W, et al. Cardiac rehabilitation delivery model for low-resource settings: an international council of cardiovascular prevention and rehabilitation consensus statement. Prog Cardiovasc Dis. (2016) 59(3):303–22. doi: 10.1016/j.pcad.2016.08.004
65. Grace SL, Turk-Adawi KI, Contractor A, Atrey A, Campbell N, Derman W, et al. Cardiac rehabilitation delivery model for low-resource settings. Heart. (2016) 102(18):1449–55. doi: 10.1136/heartjnl-2015-309209
66. Makita S, Yasu T, Akashi YJ, Adachi H, Izawa H, Ishihara S, et al. JCS/JACR 2021 guideline on rehabilitation in patients with cardiovascular disease. Circ J. (2022) 87(1):155–235. doi: 10.1253/circj.CJ-22-0234
67. Kim C, Sung J, Lee JH, Kim WS, Lee GJ, Jee S, et al. Clinical practice guideline for cardiac rehabilitation in Korea. Ann Rehabil Med. (2019) 43(3):355–443. doi: 10.5535/arm.2019.43.3.355
68. Herdy AH, Lopez-Jimenez F, Terzic CP, Milani M, Stein R, Carvalho T, et al. South American Guidelines for cardiovascular disease prevention and rehabilitation. Arq Bras Cardiol. (2014) 103(2 Suppl 1):1–31. doi: 10.5935/abc.2014s003
69. ACPICR. Standards for Physical Activity and Exercise in the Cardiovascular Population 2023. Available online at: https://www.acpicr.com/data/Page_Downloads/ACPICR2023StandardsReaderlayout.pdf (Accessed January 15, 2024).
70. McCreery C, Cradock K, Fallon N, Duffy R, Doherty VO, Kingston C. Irish Association of Cardiac Rehabilitation: Cardiac Rehabilitation Guidelines 2013. Available online at: https://iacronline.ie//wp-content/uploads/2022/09/IACR-Guidelines2013.pdf (Accessed January 15, 2024).
71. American College of Sports Medicine. ACSM’s Guidelines for Exercise Testing and Prescription. 11 ed. Philadelphia: Wolters Kluwer (2022).
72. Anselmi F, Cavigli L, Pagliaro A, Valente S, Valentini F, Cameli M, et al. The importance of ventilatory thresholds to define aerobic exercise intensity in cardiac patients and healthy subjects. Scand J Med Sci Sports. (2021) 31(9):1796–808. doi: 10.1111/sms.14007
73. Mezzani A, Hamm LF, Jones AM, McBride PE, Moholdt T, Stone JA, et al. Aerobic exercise intensity assessment and prescription in cardiac rehabilitation: a joint position statement of the European association for cardiovascular prevention and rehabilitation, the American association of cardiovascular and pulmonary rehabilitation and the Canadian association of cardiac rehabilitation. Eur J Prev Cardiol. (2013) 20(3):442–67. doi: 10.1177/2047487312460484
74. Weatherwax RM, Harris NK, Kilding AE, Dalleck LC. Incidence of V O2max responders to personalized versus standardized exercise prescription. Med Sci Sports Exerc. (2019) 51(4):681–91. doi: 10.1249/MSS.0000000000001842
75. Mezzani A, Hamm LF, Jones AM, McBride PE, Moholdt T, Stone JA, et al. Aerobic exercise intensity assessment and prescription in cardiac rehabilitation: a joint position statement of the European association for cardiovascular prevention and rehabilitation, the American association of cardiovascular and pulmonary rehabilitation, and the Canadian association of cardiac rehabilitation. J Cardiopulm Rehabil Prev. (2012) 32(6):327–50. doi: 10.1097/HCR.0b013e3182757050
76. Iannetta D, Keir DA, Fontana FY, Inglis EC, Mattu AT, Paterson DH, et al. Evaluating the accuracy of using fixed ranges of METs to categorize exertional intensity in a heterogeneous group of healthy individuals: implications for cardiorespiratory fitness and health outcomes. Sports Med. (2021) 51(11):2411–21. doi: 10.1007/s40279-021-01476-z
77. Baldwin J, Snow RJ, Febbraio MA. Effect of training status and relative exercise intensity on physiological responses in men. Med Sci Sports Exerc. (2000) 32(9):1648–54. doi: 10.1097/00005768-200009000-00020
78. Milani J, Milani M, Cipriano GFB, Hansen D, Cipriano Junior G. Exercise intensity domains determined by heart rate at the ventilatory thresholds in patients with cardiovascular disease: new insights and comparisons to cardiovascular rehabilitation prescription recommendations. BMJ Open Sport Exerc Med. (2023) 9(3):e001601. doi: 10.1136/bmjsem-2023-001601
79. Price KJ, Gordon BA, Bird SR, Benson AC. A review of guidelines for cardiac rehabilitation exercise programmes: is there an international consensus? Eur J Prev Cardiol. (2016) 23(16):1715–33. doi: 10.1177/2047487316657669
80. Hansen D, Bonne K, Alders T, Hermans A, Copermans K, Swinnen H, et al. Exercise training intensity determination in cardiovascular rehabilitation: should the guidelines be reconsidered? Eur J Prev Cardiol. (2019) 26(18):1921–8. doi: 10.1177/2047487319859450
81. Iannetta D, Inglis EC, Mattu AT, Fontana FY, Pogliaghi S, Keir DA, et al. A critical evaluation of current methods for exercise prescription in women and men. Med Sci Sports Exerc. (2020) 52(2):466–73. doi: 10.1249/MSS.0000000000002147
82. Jamnick NA, Pettitt RW, Granata C, Pyne DB, Bishop DJ. An examination and critique of current methods to determine exercise intensity. Sports Med. (2020) 50(10):1729–56. doi: 10.1007/s40279-020-01322-8
83. Keltz RR, Hartley T, Huitema AA, McKelvie RS, Suskin NG, Keir DA. Do clinical exercise tests permit exercise threshold identification in patients referred to cardiac rehabilitation? Can J Cardiol. (2023) 39(11):1701–11. doi: 10.1016/j.cjca.2023.07.029
84. Pymer S, Nichols S, Prosser J, Birkett S, Carroll S, Ingle L. Does exercise prescription based on estimated heart rate training zones exceed the ventilatory anaerobic threshold in patients with coronary heart disease undergoing usual-care cardiovascular rehabilitation? A United Kingdom perspective. Eur J Prev Cardiol. (2020) 27(6):579–89. doi: 10.1177/2047487319852711
85. Marcin T, Eser P, Prescott E, Prins LF, Kolkman E, Bruins W, et al. Training intensity and improvements in exercise capacity in elderly patients undergoing European cardiac rehabilitation—the EU-CaRE multicenter cohort study. PLoS One. (2020) 15(11):e0242503. doi: 10.1371/journal.pone.0242503
86. Carvalho T, Freitas OGA, Chalela WA, Carlos Alberto Cordeiro Hossri CAC, Milani M, Buglia S, et al. Diretriz Brasileira de Ergometria em População Adulta. Arq Bras Cardiol. (2024) 121(3):e20240110. doi: 10.36660/abc.20240110
87. Guazzi M, Adams V, Conraads V, Halle M, Mezzani A, Vanhees L, et al. Clinical recommendations for cardiopulmonary exercise testing data assessment in specific patient populations. Circulation. (2012) 126(18):2261–74. doi: 10.1161/CIR.0b013e31826fb946
88. Casillas JM, Hannequin A, Besson D, Benaim S, Krawcow C, Laurent Y, et al. Walking tests during the exercise training: specific use for the cardiac rehabilitation. Ann Phys Rehabil Med. (2013) 56(7–8):561–75. doi: 10.1016/j.rehab.2013.09.003
89. Oliveira MF, Zanussi G, Sprovieri B, Lobo DM, Mastrocolla LE, Umeda II, et al. Alternatives to aerobic exercise prescription in patients with chronic heart failure. Arq Bras Cardiol. (2016) 106(2):97–104. doi: 10.5935/abc.20160014
90. Laboratories ATSCoPSfCPF. ATS statement: guidelines for the six-minute walk test. Am J Respir Crit Care Med. (2002) 166(1):111–7. doi: 10.1164/ajrccm.166.1.at1102
91. Giannitsi S, Bougiakli M, Bechlioulis A, Kotsia A, Michalis LK, Naka KK. 6-minute walking test: a useful tool in the management of heart failure patients. Ther Adv Cardiovasc Dis. (2019) 13:1753944719870084. doi: 10.1177/1753944719870084
92. Carvalho EE, Costa DC, Crescencio JC, Santi GL, Papa V, Marques F, et al. Heart failure: comparison between six-minute walk test and cardiopulmonary test. Arq Bras Cardiol. (2011) 97(1):59–64. doi: 10.1590/s0066-782X2011005000056
93. Forman DE, Fleg JL, Kitzman DW, Brawner CA, Swank AM, McKelvie RS, et al. 6-min walk test provides prognostic utility comparable to cardiopulmonary exercise testing in ambulatory outpatients with systolic heart failure. J Am Coll Cardiol. (2012) 60(25):2653–61. doi: 10.1016/j.jacc.2012.08.1010
94. Boxer R, Kleppinger A, Ahmad A, Annis K, Hager D, Kenny A. The 6-minute walk is associated with frailty and predicts mortality in older adults with heart failure. Congest Heart Fail. (2010) 16(5):208–13. doi: 10.1111/j.1751-7133.2010.00151.x
95. Wegrzynowska-Teodorczyk K, Rudzinska E, Lazorczyk M, Nowakowska K, Banasiak W, Ponikowski P, et al. Distance covered during a six-minute walk test predicts long-term cardiovascular mortality and hospitalisation rates in men with systolic heart failure: an observational study. J Physiother. (2013) 59(3):177–87. doi: 10.1016/S1836-9553(13)70182-6
96. Calegari L, Moreira I, Falkowski A, Reolao JBC, Karsten M, Deresz LF. Agreement between heart rate at first ventilatory threshold on treadmill and at 6-min walk test in coronary artery disease patients on beta-blockers treatment. J Exerc Rehabil. (2021) 17(5):362–8. doi: 10.12965/jer.2142488.244
97. Althoff A, Vieira AM, Silveira LSD, Benetti M, Karsten M. Aerobic exercise prescription in cardiac rehabilitation based on heart rate from talk test stages and 6-minute walk test. Arq Bras Cardiol. (2023) 120(9):e20230086. doi: 10.36660/abc.20230086
98. Muller Pde T, Christofoletti G, Zagatto AM, Paulin FV, Neder JA. Reliability of peak O2 uptake and O2 uptake kinetics in step exercise tests in healthy subjects. Respir Physiol Neurobiol. (2015) 207:7–13. doi: 10.1016/j.resp.2014.12.001
99. Chae G, Ko EJ, Lee SW, Kim HJ, Kwak SG, Park D, et al. Stronger correlation of peak oxygen uptake with distance of incremental shuttle walk test than 6-min walk test in patients with COPD: a systematic review and meta-analysis. BMC Pulm Med. (2022) 22(1):102. doi: 10.1186/s12890-022-01897-0
100. Pepera G, McAllister J, Sandercock G. Long-term reliability of the incremental shuttle walking test in clinically stable cardiovascular disease patients. Physiotherapy. (2010) 96(3):222–7. doi: 10.1016/j.physio.2009.11.010
101. Singh SJ, Puhan MA, Andrianopoulos V, Hernandes NA, Mitchell KE, Hill CJ, et al. An official systematic review of the European respiratory society/American thoracic society: measurement properties of field walking tests in chronic respiratory disease. Eur Respir J. (2014) 44(6):1447–78. doi: 10.1183/09031936.00150414
102. Osailan AM. Cardiopulmonary response during incremental shuttle walking test in a hallway versus on treadmill in phase IV cardiac rehabilitation: a cross-sectional study. Sci Rep. (2023) 13(1):12806. doi: 10.1038/s41598-023-39999-2
103. Alotaibi JF, Doherty P. Evaluation of determinants of walking fitness in patients attending cardiac rehabilitation. BMJ Open Sport Exerc Med. (2016) 2(1):e000203. doi: 10.1136/bmjsem-2016-000203
104. Bok D, Rakovac M, Foster C. An examination and critique of subjective methods to determine exercise intensity: the talk test, feeling scale, and rating of perceived exertion. Sports Med. (2022) 52(9):2085–109. doi: 10.1007/s40279-022-01690-3
105. Parfitt G, Evans H, Eston R. Perceptually regulated training at RPE13 is pleasant and improves physical health. Med Sci Sports Exerc. (2012) 44(8):1613–8. doi: 10.1249/MSS.0b013e31824d266e
106. Parfitt G, Alrumh A, Rowlands AV. Affect-regulated exercise intensity: does training at an intensity that feels 'good' improve physical health? J Sci Med Sport. (2012) 15(6):548–53. doi: 10.1016/j.jsams.2012.01.005
107. Zanettini R, Centeleghe P, Franzelli C, Mori I, Benna S, Penati C, et al. Validity of the talk test for exercise prescription after myocardial revascularization. Eur J Prev Cardiol. (2013) 20(2):376–82. doi: 10.1177/2047487312438982
108. Chen MJ, Fan X, Moe ST. Criterion-related validity of the borg ratings of perceived exertion scale in healthy individuals: a meta-analysis. J Sports Sci. (2002) 20(11):873–99. doi: 10.1080/026404102320761787
109. Oliveira BR, Deslandes AC, Santos TM. Differences in exercise intensity seems to influence the affective responses in self-selected and imposed exercise: a meta-analysis. Front Psychol. (2015) 6:1105. doi: 10.3389/fpsyg.2015.01105
110. Scherr J, Wolfarth B, Christle JW, Pressler A, Wagenpfeil S, Halle M. Associations between borg’s rating of perceived exertion and physiological measures of exercise intensity. Eur J Appl Physiol. (2013) 113(1):147–55. doi: 10.1007/s00421-012-2421-x
111. Gurovich AN, Heiser B, Hayes C, Marshall E, Roath S, Kabous NG. Clinical markers of exercise intensity as a surrogate for blood lactate levels only during low-intensity exercise in patients with coronary artery disease. Cardiopulm Phys Ther J. (2018) 29(4):144–51. doi: 10.1097/cpt.0000000000000082
112. Nielsen SG, Buus L, Hage T, Olsen H, Walsoe M, Vinther A. The graded cycling test combined with the talk test is reliable for patients with ischemic heart disease. J Cardiopulm Rehabil Prev. (2014) 34(4):276–80. doi: 10.1097/HCR.0000000000000067
113. Festa RR, Jofre-Saldia E, Candia AA, Monsalves-Alvarez M, Flores-Opazo M, Penailillo L, et al. Next steps to advance general physical activity recommendations towards physical exercise prescription: a narrative review. BMJ Open Sport Exerc Med. (2023) 9(4):e001749. doi: 10.1136/bmjsem-2023-001749
114. Saini M, Kulandaivelan S, Devi P, Saini V. The talk test-A costless tool for exercise prescription in Indian cardiac rehabilitation. Indian Heart J. (2018) 70(Suppl 3):S466–70. doi: 10.1016/j.ihj.2018.09.009
115. Reed JL, Pipe AL. The talk test: a useful tool for prescribing and monitoring exercise intensity. Curr Opin Cardiol. (2014) 29(5):475–80. doi: 10.1097/HCO.0000000000000097
116. Sorensen L, Larsen KSR, Petersen AK. Validity of the talk test as a method to estimate ventilatory threshold and guide exercise intensity in cardiac patients. J Cardiopulm Rehabil Prev. (2020) 40(5):330–4. doi: 10.1097/HCR.0000000000000506
117. Orizola-Caceres I, Cerda-Kohler H, Burgos-Jara C, Meneses-Valdes R, Gutierrez-Pino R, Sepulveda C. Modified talk test: a randomized cross-over trial investigating the comparative utility of two “talk tests” for determining aerobic training zones in overweight and obese patients. Sports Med Open. (2021) 7(1):23. doi: 10.1186/s40798-021-00315-9
118. Persinger R, Foster C, Gibson M, Fater DC, Porcari JP. Consistency of the talk test for exercise prescription. Med Sci Sports Exerc. (2004) 36(9):1632–6.15354048
119. Cannon C, Foster C, Porcari JP, Skemp-Arlt KM, Fater DC, Backes R. The talk test as a measure of exertional ischemia. Am J Med Sports. (2004) 6:52–6.
120. Woltmann ML, Foster C, Porcari JP, Camic CL, Dodge C, Haible S, et al. Evidence that the talk test can be used to regulate exercise intensity. J Strength Cond Res. (2015) 29(5):1248–54. doi: 10.1519/JSC.0000000000000811
121. Brawner CA, Vanzant MA, Ehrman JK, Foster C, Porcari JP, Kelso AJ, et al. Guiding exercise using the talk test among patients with coronary artery disease. J Cardiopulm Rehabil. (2006) 26(2):72–5; quiz 6–7. doi: 10.1097/00008483-200603000-00002
122. Sr F, Naughton J, Haskell W. Physical activity and the prevention of coronary heart disease. Ann Clin Res. (1971) 3:404–32.4945367
123. Tanaka H, Monahan KD, Seals DR. Age-predicted maximal heart rate revisited. J Am Coll Cardiol. (2001) 37(1):153–6. doi: 10.1016/s0735-1097(00)01054-8
124. Keteyian SJ, Kitzman D, Zannad F, Landzberg J, Arnold JM, Brubaker P, et al. Predicting maximal HR in heart failure patients on beta-blockade therapy. Med Sci Sports Exerc. (2012) 44(3):371–6. doi: 10.1249/MSS.0b013e318234316f
125. Magrì D, Piepoli M, Gallo G, Corrà U, Metra M, Paolillo S, et al. Old and new equations for maximal heart rate prediction in patients with heart failure and reduced ejection fraction on beta-blockers treatment. Results from the mecki score dataset. Eur J Prev Cardiol. (2022) 29(12):1680–8. doi: 10.1093/eurjpc/zwac099
126. Milani JGPO, Milani M, Bernardelli Cipriano GF, Cipriano G. Estimation of the maximal heart rate for exercise prescription in heart failure patients: are we there yet? Eur J Prev Cardiol. (2022) 30(5):419–21. doi: 10.1093/eurjpc/zwac236
127. Mytinger M, Nelson RK, Zuhl M. Exercise prescription guidelines for cardiovascular disease patients in the absence of a baseline stress test. J Cardiovasc Dev Dis. (2020) 7(2). doi: 10.3390/jcdd7020015
128. Vanhees L, Fagard R, Thijs L, Staessen J, Amery A. Prognostic significance of peak exercise capacity in patients with coronary artery disease. J Am Coll Cardiol. (1994) 23(2):358–63. doi: 10.1016/0735-1097(94)90420-0
129. Hung RK, Al-Mallah MH, McEvoy JW, Whelton SP, Blumenthal RS, Nasir K, et al. Prognostic value of exercise capacity in patients with coronary artery disease: the FIT (henry ford ExercIse testing) project. Mayo Clin Proc. (2014) 89(12):1644–54. doi: 10.1016/j.mayocp.2014.07.011
130. Bjarnason-Wehrens B, Nebel R, Jensen K, Hackbusch M, Grilli M, Gielen S, et al. Exercise-based cardiac rehabilitation in patients with reduced left ventricular ejection fraction: the cardiac rehabilitation outcome study in heart failure (CROS-HF): a systematic review and meta-analysis. Eur J Prev Cardiol. (2020) 27(9):929–52. doi: 10.1177/2047487319854140
131. Long L, Anderson L, Dewhirst AM, He J, Bridges C, Gandhi M, et al. Exercise-based cardiac rehabilitation for adults with stable angina. Cochrane Database Syst Rev. (2018) 2(2):CD012786. doi: 10.1002/14651858.CD012786.pub2
132. Long L, Mordi IR, Bridges C, Sagar VA, Davies EJ, Coats AJ, et al. Exercise-based cardiac rehabilitation for adults with heart failure. Cochrane Database Syst Rev. (2019) 1:CD003331. doi: 10.1002/14651858.CD003331.pub5
133. Rauch B, Davos CH, Doherty P, Saure D, Metzendorf MI, Salzwedel A, et al. The prognostic effect of cardiac rehabilitation in the era of acute revascularisation and statin therapy: a systematic review and meta-analysis of randomized and non-randomized studies—the cardiac rehabilitation outcome study (CROS). Eur J Prev Cardiol. (2016) 23(18):1914–39. doi: 10.1177/2047487316671181
134. Bowen TS, Cannon DT, Begg G, Baliga V, Witte KK, Rossiter HB. A novel cardiopulmonary exercise test protocol and criterion to determine maximal oxygen uptake in chronic heart failure. J Appl Physiol (1985). (2012) 113(3):451–8. doi: 10.1152/japplphysiol.01416.2011
135. Garber CE, Blissmer B, Deschenes MR, Franklin BA, Lamonte MJ, Lee IM, et al. Quantity and quality of exercise for developing and maintaining cardiorespiratory, musculoskeletal, and neuromotor fitness in apparently healthy adults: guidance for prescribing exercise. Med Sci Sports Exerc. (2011) 43(7):1334–59. doi: 10.1249/MSS.0b013e318213fefb
136. Krieger J, McCann N, Bluhm M, Zuhl M. Exercise prescription and progression practices among US cardiac rehabilitation clinics. Clin Pract. (2022) 12(2):194–203. doi: 10.3390/clinpract12020023
137. Faggian S, Centanini A, Quinto G, Vecchiato M, Ermolao A, Battista F, et al. The many faces of exercise intensity: a call to agree on definitions and provide standardized prescriptions. Eur J Prev Cardiol. (2024). doi: 10.1093/eurjpc/zwae034. [Epub ahead of print]38271589
138. Milani J, Milani M, Machado FVC, Wilhelm M, Marcin T, D'Ascenzi F, et al. Accurate prediction equations for ventilatory thresholds in cardiometabolic disease when gas exchange analysis is Unavailable: development and validation. Eur J Prev Cardiol. (2024). doi: 10.1093/eurjpc/zwae149. [Epub ahead of print]
139. Kokkinos P, Kaminsky LA, Arena R, Zhang J, Myers J. New generalized equation for predicting maximal oxygen uptake (from the fitness registry and the importance of exercise national database). Am J Cardiol. (2017) 120(4):688–92. doi: 10.1016/j.amjcard.2017.05.037
140. Kokkinos P, Kaminsky LA, Arena R, Zhang J, Myers J. A new generalized cycle ergometry equation for predicting maximal oxygen uptake: the fitness registry and the importance of exercise national database (FRIEND). Eur J Prev Cardiol. (2018) 25(10):1077–82. doi: 10.1177/2047487318772667
141. MacIntosh BR, Murias JM, Keir DA, Weir JM. What is moderate to vigorous exercise intensity? Front Physiol. (2021) 12:682233. doi: 10.3389/fphys.2021.682233
142. Hansen D, Dendale P, Coninx K, Vanhees L, Piepoli MF, Niebauer J, et al. The European association of preventive cardiology exercise prescription in everyday practice and rehabilitative training (EXPERT) tool: a digital training and decision support system for optimized exercise prescription in cardiovascular disease. Concept, definitions and construction methodology. Eur J Prev Cardiol. (2017) 24(10):1017–31. doi: 10.1177/2047487317702042
Keywords: exercise, physical activity, cardiac rehabilitation, cardiovascular health, cardiovascular disease, heart disease risk factors, exercise test, health
Citation: Milani JGPO, Milani M, Verboven K, Cipriano G Jr and Hansen D (2024) Exercise intensity prescription in cardiovascular rehabilitation: bridging the gap between best evidence and clinical practice. Front. Cardiovasc. Med. 11:1380639. doi: 10.3389/fcvm.2024.1380639
Received: 1 February 2024; Accepted: 1 August 2024;
Published: 27 August 2024.
Edited by:
Konstantinos Koskinas, Inselspital University Hospital Bern, SwitzerlandReviewed by:
Henner Hanssen, University of Basel, SwitzerlandCopyright: © 2024 Milani, Milani, Verboven, Cipriano and Hansen. This is an open-access article distributed under the terms of the Creative Commons Attribution License (CC BY). The use, distribution or reproduction in other forums is permitted, provided the original author(s) and the copyright owner(s) are credited and that the original publication in this journal is cited, in accordance with accepted academic practice. No use, distribution or reproduction is permitted which does not comply with these terms.
*Correspondence: Dominique Hansen, ZG9taW5pcXVlLmhhbnNlbkB1aGFzc2VsdC5iZQ==
†These authors share senior authorship
Disclaimer: All claims expressed in this article are solely those of the authors and do not necessarily represent those of their affiliated organizations, or those of the publisher, the editors and the reviewers. Any product that may be evaluated in this article or claim that may be made by its manufacturer is not guaranteed or endorsed by the publisher.
Research integrity at Frontiers
Learn more about the work of our research integrity team to safeguard the quality of each article we publish.